- 1Jiangsu Co-Innovation Center for Modern Production Technology of Grain Crops, Yangzhou University, Yangzhou, China
- 2Jiangsu Key Laboratory of Crop Genetics and Physiology/Jiangsu Key Laboratory of Crop Cultivation and Physiology, Agricultural College of Yangzhou University, Yangzhou, China
- 3Research Institute of Rice Industrial Engineering Technology of Yangzhou University, Yangzhou, China
- 4School of Food Science and Engineering, Yangzhou University, Yangzhou, China
Background: Simulated oral processing can be used to evaluate the palatability of cooked rice. Previously, we established a simulated oral processing method using a texture analyzer equipped with a multiple extrusion cell probe (TA/MEC). However, the relationship between oral processing and starch fine structure remains unknown.
Methods: In this study, we analyzed the oral processing properties using TA/MEC and characterized the starch fine structure of japonica rice by size-exclusion chromatography (SEC) and fluorophore-assisted capillary electrophoresis (FACE). The relationship between starch fine structure and oral processing of cooked japonica rice was further investigated.
Results: Cooked rice structure contains fast-breakdown (Type I structure), slow-breakdown (Type II structure) and unbreakable structures (Type III structure). Fast-breakdown and slow-breakdown structure were positively correlated with the content of amylose and shorter amylopectin branches. The content of longer amylopectin branches was positively correlated with the contribution of unbreakable structure.
Conclusion: The results indicated that cooked japonica rice varieties with more amylose and shorter amylopectin branches tend to form a harder texture and need more work to break down the fast and slow breakdown structures related to rice kernel fragmentation. Meanwhile, cooked japonica rice varieties possess stronger molecular entanglements due to their longer amylopectin branches and contribute more to the breakdown of unbreakable structures. These results can guide breeders to select rice varieties with desirable eating qualities for cultivation.
Introduction
Rice is a key staple food for human beings and its yield has greatly increased owing to the combination of rice breeding and cultivation technology. However, rice quality, especially eating quality, needs further improvement. The primary consideration of rice customers has shifted from grain yield to grain palatability (1). Theoretically, the palatability of rice can be evaluated by amylose content, gel consistency, and gelatinization, which significantly correlate with the texture formation of cooked rice (2, 3). Researchers usually evaluate the palatability of cooked rice by a human sensory test, but this method is time-consuming and subjective (4, 5).
Oral processing properties, like the force and work during the first bite, chewing, and swallowing, is a crucial procedure for the consumption and palatability of foods (6, 7). Rice is primarily consumed in the form of polished white rice which possesses a whole-grain structure (8), and its oral processing property plays an essential role in consumer acceptability (9, 10). However, the relationship between oral processing and palatability of cooked rice has not been adequately investigated.
Our previous work established a simulated oral processing method by monitoring changes in force and work during mastication using a texture analyzer equipped with a multiple extrusion cell probe (TA/MEC) (11). We found that this method can be used to evaluate the mouthfeel and palatability of cooked rice during mastication (12, 13). In this method, the structure of cooked rice is divided into three types: fast breakdown structure (defined as Type I structure), slow breakdown structure (defined as Type II structure), and unbreakable structure (defined as Type III structure). Based on previous research, the Type I structure is related to the fragmentation of rice kernel, the Type III structure represents a structure that cannot be broken even after much mastication, while the Type II structure lies between these which is related to the fragmentation of rice kernel and the enzymatic degradation of rice matrix (11). The work and proportion of these structures can be used to evaluate rice palatability (11).
Starch consists of two polymers, namely linear amylose and branched amylopectin. Besides, starch is the most abundant component in rice grains. Thus, the structure and content of starch is closely related to the palatability of cooked rice (14). Previous research has confirmed that the texture of cooked rice closely relate to the fine structure of starch, which include the content of amylose, degree of polymerization (DP), and side chain length of amylopectin (15–17). However, the relationship between the oral processing of cooked rice and starch fine structure is still unknown. Thus, this study chose different japonica rice varieties as materials and established the relationship between their oral processing properties and starch fine structure. This study can assist researchers in improving rice palatability by using a simulated oral processing method and choosing rice varieties with certain starch fine structures.
Materials and methods
Materials
Twelve japonica rice varieties were chosen and planted at Shatou town research farm (Yangzhou city, Jiangsu province, China, 32°32′ N, 119°49′ E) from May to November 2019. All rice varieties were planted under the same cultivation conditions for high yield and quality and milled using Xiba LTJM-2099 rice-milling machine (Zhejiang Boliheng Corporation, China). Protease (≥3.5 units/mg solid) and isoamylase (≥10,000,000 units/mg protein) were purchased from Sigma-Aldrich Chemical Co. (St. Louis, MO, USA). Pullulan standards (molecular weight: (Mw) 180 Da ∼ 1.2 × 106 Da) were purchased from Polymer Standards Service (PSS, Mainz, Germany). Simulated saliva (pH 6.8) and low-temperature α-amylase (2,000 U/g solid) were purchased from Ke Lei Biological Technology Co., Ltd. (Shanghai, China) and Shanghai Yuanye Bio-Technology Co., Ltd. (Shanghai, China), respectively. All other chemicals used in this study were of reagent grade.
Size-exclusion chromatography
The molecular size distributions of whole branched starch (Rh, nm) and debranched starch (Mw, Da) were analyzed using an LC-20AD (Shimadzu, Kyoto, Japan) size-exclusion chromatography (SEC) system equiped with a RID-10A detector, according to the method described by Gilbert (16, 18, 19). Starch was isolated from rice kernels using protease to cleave proteins and anhydrous ethanol to remove lipids. The starch was debranched using isoamylase in order to analyze the chains. All the starch samples (2 mg/mL) were dissolved in DMSO/LiBr solution (0.5%, w/w). Whole starch molecules were separated using a combination of GRAM pre-column, GRAM 30, and GRAM 3000 analytical columns (PSS), while starch chains were separated using a combination of GRAM pre-column, GRAM 100, and GRAM 1000 analytical columns (PSS). The mobile phase was DMSO/LiBr solution (0.5%, w/w) with an elution rate of 0.3 and 0.6 mL/min for branched and debranched starches, respectively. The column oven temperature was maintained at 80 °C. Pullulan standards (PSS) with different Mw(180 Da ∼ 1.2 × 106 Da) were used for calibration and calculation. The data were analyzed using XPS Peak Fit software v. 4.1. After peak fitting, the SEC data were calculated to characterize the molecular size distributions of the branched and debranched starch samples.
Fluorophore-assisted capillary electrophoresis
The chain length distributions (CLD) of amylopectin were analyzed according to the method described by Gilbert (20). Amylopectin in the starches was firstly debranched using isoamylase, then labeled with 8-aminopyrene-1,3,6-trisulfonic acid. After that, the samples was analyzed using a PA-800 Plus fluorophore-assisted capillary electrophoresis (FACE) system (Beckman Coulter, Brea, CA, USA) with a solid-state laser-induced fluorescence detector and an argon-ion laser as the excitation source.
Simulated oral processing
The oral processing properties of the cooked rice were measured according to a previous method (11). Milled rice was cooked at a rice/water ratio of 1:1.3 using a rice cooker. The oral processing properties of the cooked rice were measured using a TA.XTplus TA/MEC (Stable Micro System, Surrey, UK). Briefly, cooked rice (30 g) together with simulated saliva (3.6 mL, pH6.8) and low-temperature α-amylase (0.01 g, ezyme activity: 2000 U/g solid) was added to the MEC probe according to the in situ oral processing of human subjects (11). Cyclic compression was conducted 25 times to destroy the structure of the rice kernels. The extrusion distance was set to 93 mm, and both the test and post-test speeds were set as 5 mm/s. The work during each chewing cycle was calculated according to the force versus distance curves and analyzed by fitting to the double-exponential decay function (Eq. (1)):
where wn is the work during each compression cycle (n), w1 and w2 are the contributions to the loss of energies per cycle with a decay rate given by n1 and n2, respectively. Besides, w∞ is the work per cycle even after an infinite number of compression cycles.
In this method, wn can be divided into three parts: wType I, wType II, and wType III, which represent the work per cycle to break down Type I (Eq. (2)), Type II (Eq. (3)), and Type III (Eq. (4)) structures, respectively. In addition, the fractions of wType I (fType I), wType II (fType II), and wType III (fType III) during each compression cycle can be calculated according to Eqs. (5), (6), and (7).
During the whole oral processing, the total work to break down Type I (WType I), Type II (WType II), and Type III (WType III) structures were calculated according to Eq. (8), Eq. (9) and Eq. (10) and their corresponding fractions (FType I, FType II, FType III) were calculated according to Eq. (11), Eq. (12) and Eq. (13), respectively.
Statistical analyses
Correlations between the starch fine structure ans the oral processing properties of cooked rice were analyzed using IBM SPSS® AmosTM 19 (SPSS Inc., Chicago, IL, USA). Both Pearson and Spearman rank correlations were analyzed at p < 0.05 and p < 0.01 for significant and quite significant correlations, respectively.
Results and discussion
Starch fine structure of japonica rice
Molecular size distributions of branched and debranched starches
The molecular size distributions of branched starches extracted from different japonica rice varieties are shown in Figure 1A. Two peaks were observed in the molecular size distribution curves, which were analyzed using XPS peak fitting software (Figure 1A). The lower and higher peaks around 10 to 20 nm and 60 to 100 nm are associated with amylose and amylopectin, respectively, in rice starch (21). Based on the structural parameters summarized in Table 1, the average Rh values of the whole rice starch (Rhsum) ranged from 49.58 to 74.98 nm. As previously mentioned, the SEC method can only semi-quantitatively analyze the whole starch molecular size distribution and cannot calculate amylose content because of the unavoidable shear scission effects and unsatisfactory separation of amylose and amylopectin (22, 23). Thus, branched rice starches were thoroughly debranched and characterized using SEC to analyze their fine structures (Figures 1B,b).
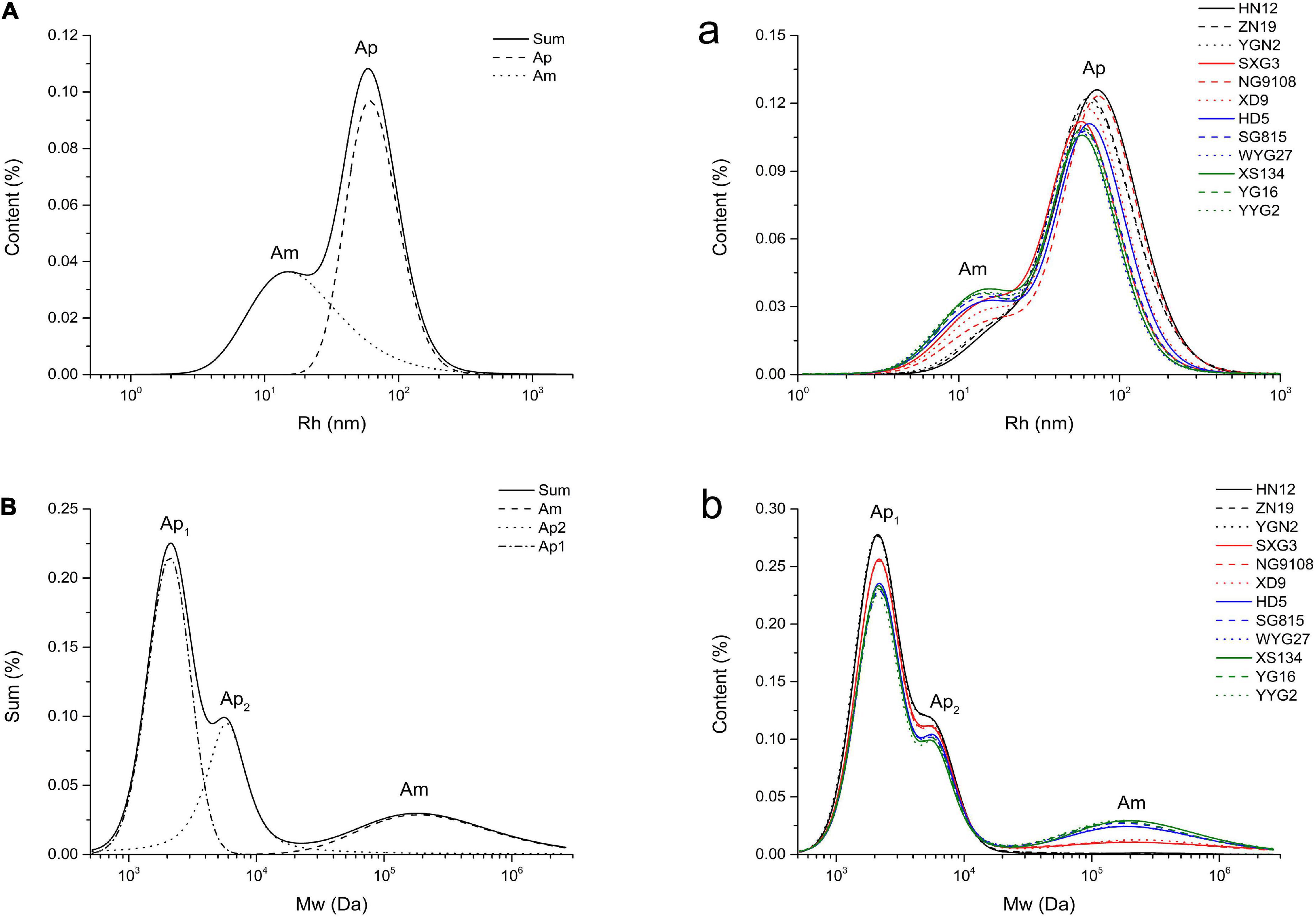
Figure 1. Molecular size distributions of whole branched starches (A/a, Rh) and debranched starches (B/b, Mw) extracted from japonica rice.
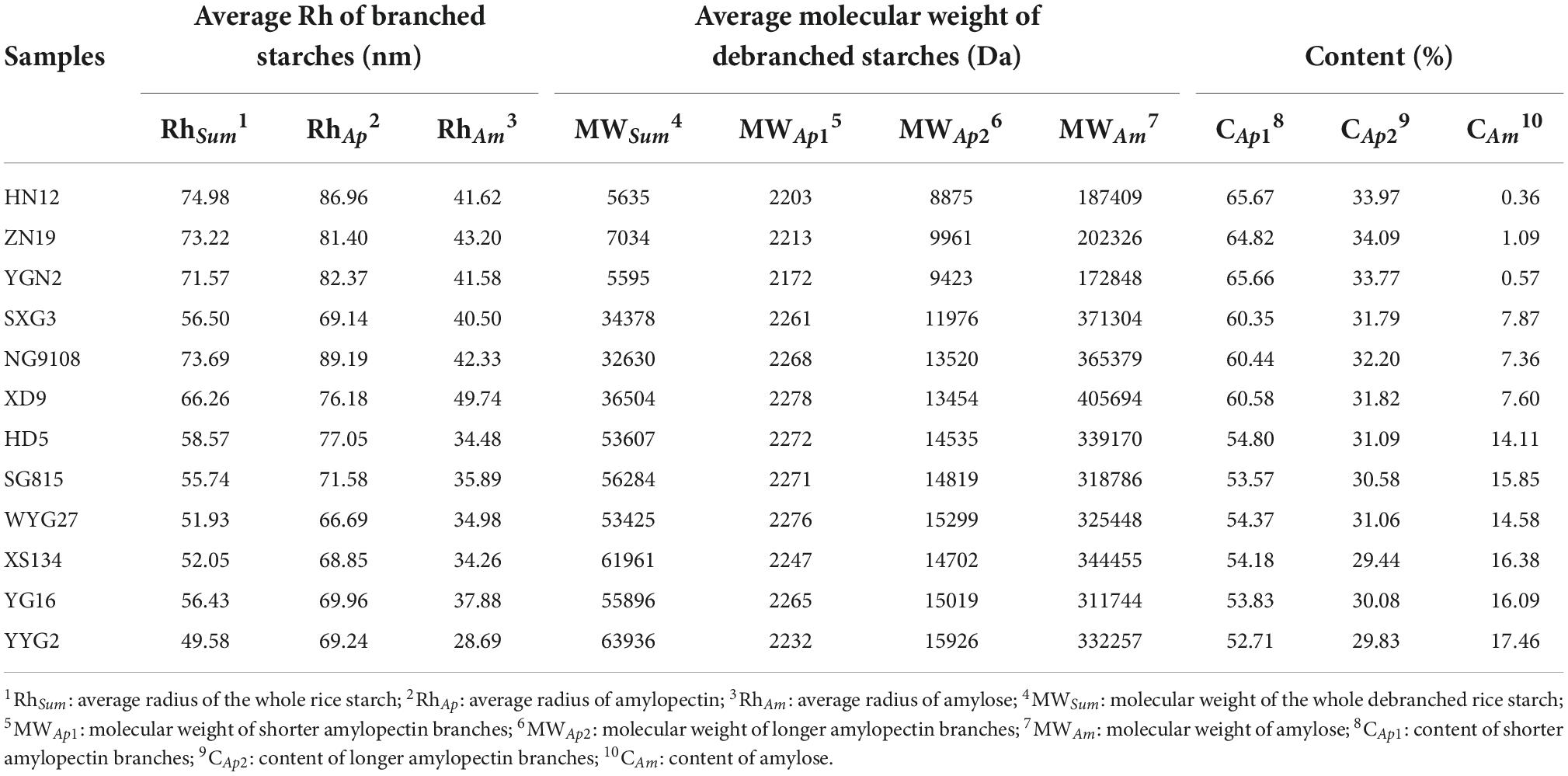
Table 1. Starch structural parameters of whole branched starch and debranched starch extracted from japonica rice.
After debranching, the α-1,6-glycosidic bonds at branching points in amylopectin were cleaved, leading to the generation of short linear starch chains. The Mw distribution curves of the debranched rice starches show three peaks, including two larger peaks of amylopectin branches and one smaller peak of amylose branches. The peak at approximately 2 × 103 Da (Ap1) represents shorter amylopectin branches that are confined to a single lamella, whereas the peak at approximately 5 × 103 to 6 × 103 Da (Ap2) is associated with longer amylopectin branches that span two or more lamellae (16, 24, 25). The peak at approximately 2 × 105 Da (Am) represents amylose (26). The Mw and content of the different starch branches were analyzed by calculating the area under the curve of each peak after peak fitting (Figure 1B); all the structural parameters are shown in Table 1.
The amylose content (CAm) of the japonica rice varieties used in this study ranged from 0.36% to 17.46%. Waxy japonica rice starches, including HN12, ZN19, and YGN2, showed a very limited amount of Am (CAm < 2%) and shorter Am and Ap2 chains. Meanwhile, the CAm values of SXG3, NG9108, and XD9 was approximately 7% to 8%, and these rice varieties have been defined as soft japonica rice. Soft japonica rice starches had longer Am chains, whereas other common japonica rice starches (CAm: 14.11% to 17.46%) had fewer Ap1 and Ap2 chains and much longer Ap2 chains. The japonica rice varieties used in this study showed different fine starch structures, including Mw and chain length of both amylose and amylopectin.
Chain-length distributions of amylopectin
Compared to SEC, FACE can separate and characterize the distributions of individual chains (27). FACE can measure the content of starch chains with DP values ranging from 6 to 100; these starch chains are defined as amylopectin (Ap) chains (26). Theoretically, the CLD of amylopectin can be fractionated into five parts, namely the A Chain (6 ≤ DP ≤ 12), B1 Chain (13 ≤ DP ≤ 24), B2 Chain (25 ≤ DP ≤ 36), B3 Chain (37 ≤ DP ≤ 65), and C Chain (DP ≥ 66) (27, 28). The CLD of amylopectin measured using FACE are shown in Table 2.
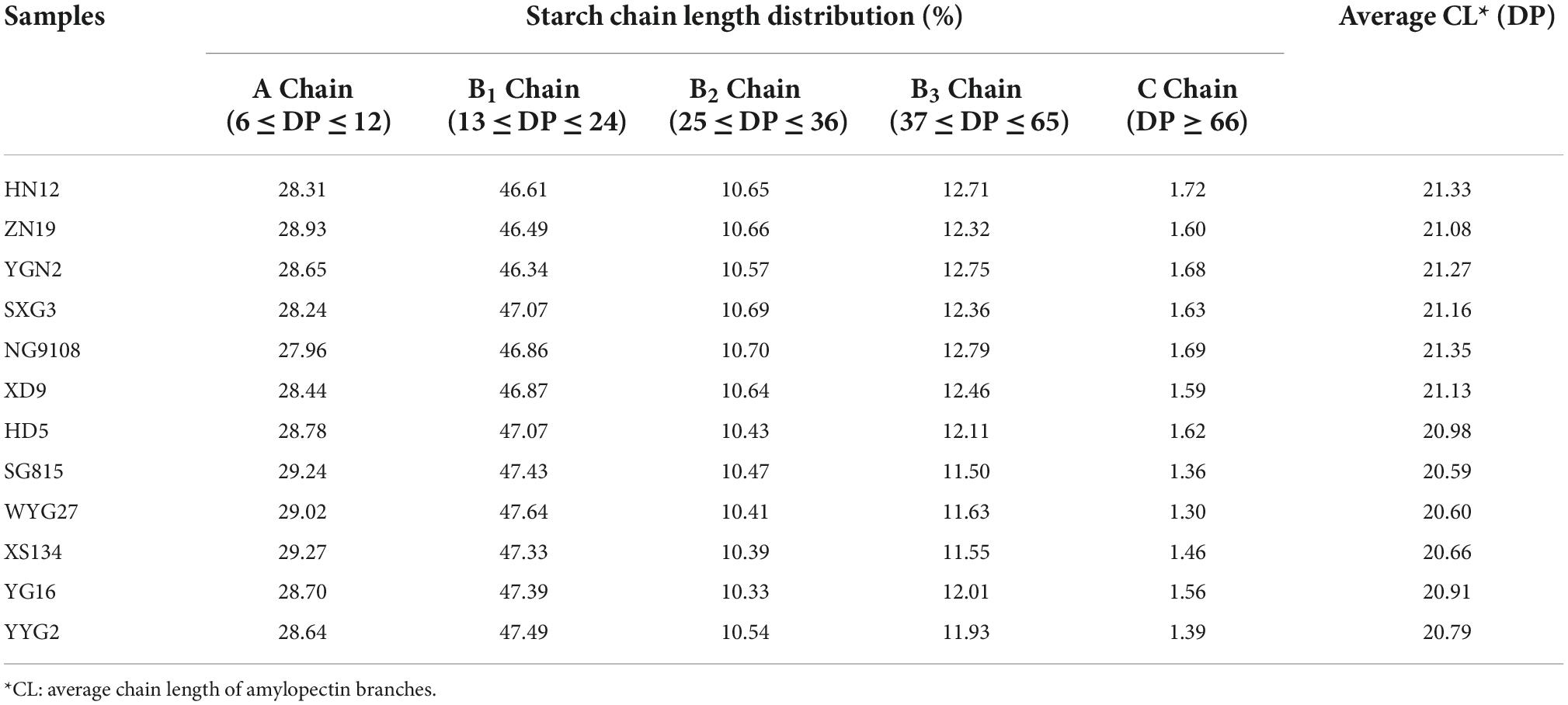
Table 2. Chain length distribution (CLD) analysis of debranched rice starches extracted from japonica rice.
The results indicated that amylopectin extracted from waxy and semi-waxy japonica rice varieties had longer chain lengths and more B3 and C chains than common rice varieties. All the japonica rice amylopectins had higher amounts of the B1 Chain (46.34 to 47.64%) than other fractionated parts. In addition, the amount of the A Chain fraction in all samples was similar, which is related to the determination of starch crystalline polymorphs (27).
Simulated oral processing of cooked japonica rice
TA/MEC was used to measure the oral processing properties of cooked japonica rice (11). The work and force applied to the cooked rice during each chewing cycle were used to characterize their oral processing properties. The results were shown in Figure 2 and Table 3.
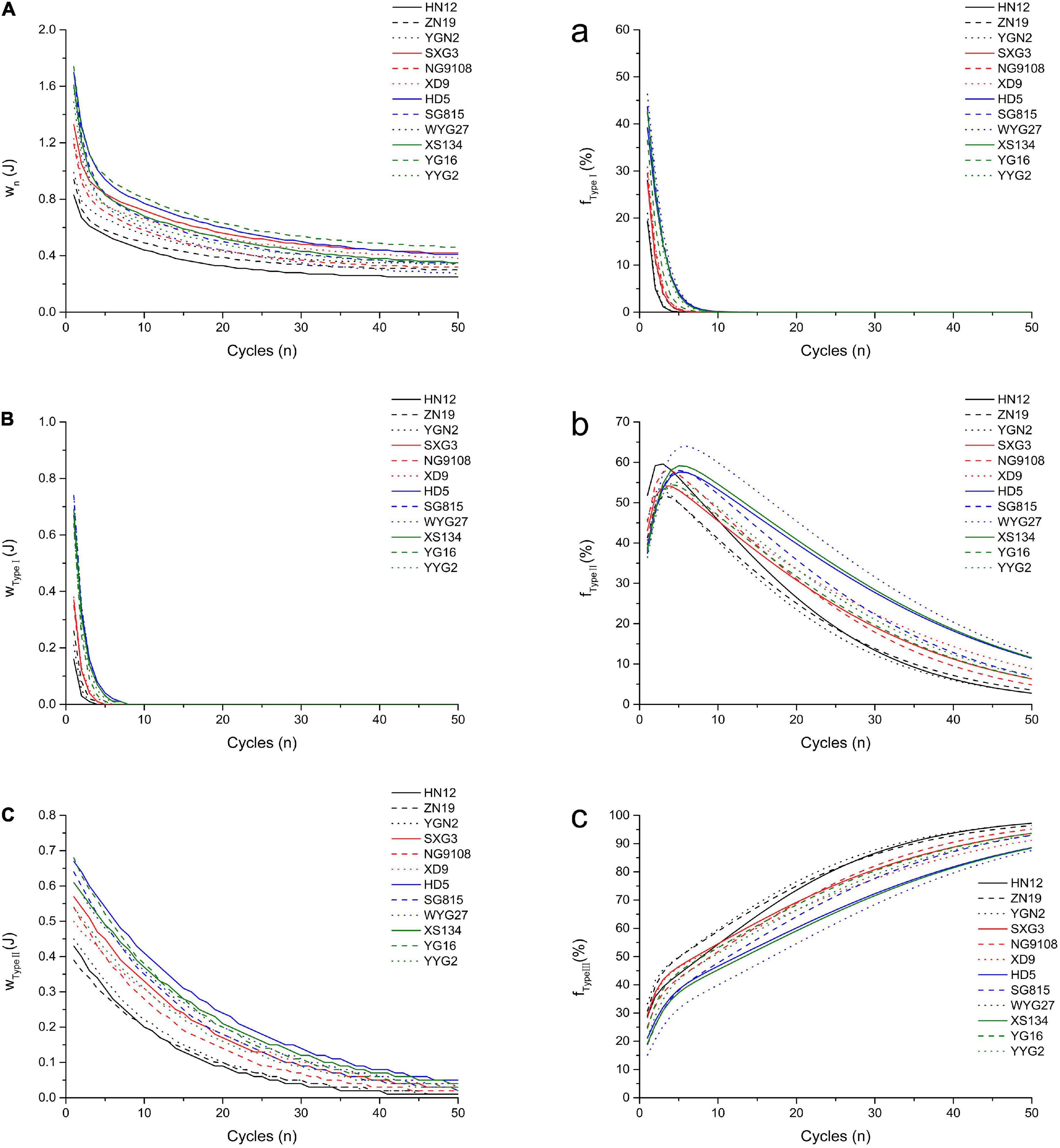
Figure 2. Simulated oral processing of cooked japonica rice. The fitted work of three type structures during each extrusion cycle (A) Type I; (B) Type II; (C) Type III. The fraction of the work contributed to the breakdown of different structures per extrusion cycle (a) Type I; (b) Type II; (c) Type III.
The results showed that wType I and its fraction (fType I) decreased rapidly with the compression cycles, and this can be used to analyze and quantify the fast breakdown structure (Type I) in cooked japonica rice. However, wType II decreased slowly with the compression cycles, which represents the slow breakdown structure (Type II) in cooked rice. The fraction of wType II (fType II) first increased and then decreased, while the fraction of wType III (fType III) increased gradually with the number of compression cycles. These results indicate that the Type I structure plays a more important role in the initial stage of chewing cooked rice, especially within five compression cycles. After approximately five compression cycles, more work per chewing cycle was required to break down the Type II structure. However, the type III structure gradually became the dominant factor affecting the oral processing properties of cooked rice from approximately the tenth compression cycle to the end.
Different japonica rice varieties showed a significant variance in oral processing properties. Based on the double-exponential decay function parameters, common japonica rice varieties (HD5, SG815, YG16, etc.) possess higher w1, w2, and w∞ values, indicating that these rice varieties require more work to break down Type I, Type II, and Type III structures during oral processing than waxy japonica rice (HN12, ZN19, and YGN2) and soft japonica rice varieties (SXG3, NG9108, and XD9). Meanwhile, japonica rice varieties with larger n1 and n2 values require more extrusions to break down the Type I and Type II structures. W1 is the work done during the first bite, and its value closely relates to the hardness of cooked rice (6). These results indicated that the hardness of cooked common japonica rice was higher than that of other japonica rice varieties. During entire oral processing, more work is required to break down the three structure types for common japonica rice varieties, while the contributions of the different structure types to the oral processing properties perform differently. Common japonica rice varieties possess higher FType I values and lower FType III values than waxy and soft japonica rice varieties. These results indicate that the Type I structure contributes more to the oral processing properties of common japonica rice varieties, whereas the Type III structure in cooked waxy japonica rice varieties plays a more important role in the formation of oral processing properties.
Correlations between starch fine structure and simulated oral processing of japonica rice
The relationships between starch fine structure and simulated oral processing of japonica rice varieties were further investigated by Pearson and Spearman rank correlation analysis, and the results are summarized in Table 4. Pearson correlation can analyze the linear correlations, while Spearman rank correlation can reflect non-linear correlations. Both Pearson and Spearman correlation tests show that the double-exponential decay function parameters, including w1, n1, and w2, were significantly and negatively correlated with Rhsum. W1, WType I, WType II, FType I, and FType II showed the same relationship with Rhsum. However, w∞ and WType III showed no significant relationship with Rhsum, but FType III significantly and positively related to Rhsum. These results indicate that japonica rice varieties with smaller starch molecular sizes tend to form harder textures and need more work to break down the Type I and Type II structures. Simultaneously, the Type I and Type II structures in japonica rice varieties with smaller starch molecular sizes contribute more to the oral processing properties, whereas the Type III structure contributes less. In japonica rice starches, the Rhsum of amylopectin is significantly larger than that of amylose. Thus, japonica rice varieties with larger Rhsums possess less amylose.
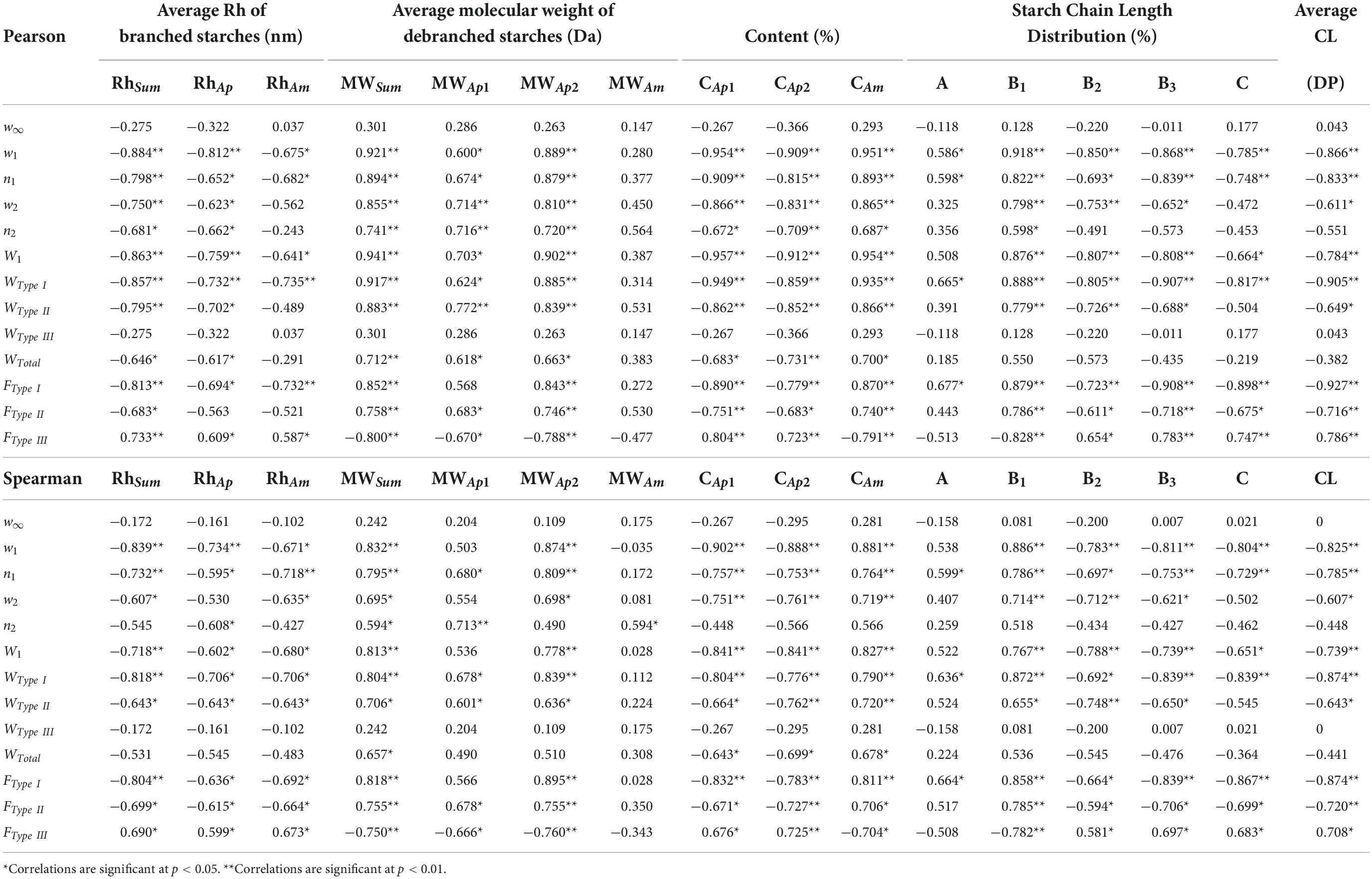
Table 4. Correlation coefficients between starch fine structure and simulated oral processing of cooked japonica rice.
The relationship between amylose content and oral processing properties of japonica rice varieties suggests that japonica rice varieties with higher amylose content possess higher w1, n1, w2, and n2 values, have a harder texture, and need more work to break the Type I and Type II structures down. In addition, the FType I and FType II values were significantly and positively correlated with the amylose content of japonica rice starches, while the FType III values showed a significant and negative correlation with the amylose content of japonica rice starches. These results indicate that Type I and Type II structures in japonica rice varieties with higher amylose content contribute more to the oral processing properties, while the Type III structure contributes less.
The relationship between the CLD of amylopectin parameters and the oral processing properties of japonica rice varieties was further analyzed. Theoretically, A- and B1-chains represent shorter branches on the outer side of amylopectin, while B2-, B3-, and C-chains represent longer branches on the inner side (27). The content of B1-chains with the highest amounts of amylopectin fractions significantly and positively correlated with w1, n1, w2, n2,W1, WType I, WType II, FType I, and FType II, and significantly and negatively correlated with FType III. However, the content of longer side chains in amylopectin, including B2-, B3-, and C-chains, and the average chain length of amylopectin, showed an opposite correlation with the oral processing parameters of cooked japonica rice. These results indicate that japonica rice varieties with more short side chains (including A- and B1-chains) tend to form a harder texture and need more work to break the Type I and Type II structures down during the whole oral processing of cooked japonica rice.
In summary, amylose content is the most critical factor affecting the oral processing properties of cooked japonica rice. Cooked japonica rice varieties with higher amylose content contain more fast breakdown structure and slow breakdown structure, and both contribute more to oral processing properties. In contrast, cooked japonica rice varieties with longer side chains possess fewer fast breakdown structure and slow breakdown structure, while the role of the unbreakable structure is more important in forming oral processing properties.
Conclusion
This study provides a new perspective on the relationship between starch fine structures and oral processing properties of cooked japonica rice. The oral processing properties of cooked japonica rice can be determined by evaluating the amount of work contributed to the breakdown of different structures and their corresponding fractions. This study found, for the first time, that amylose content together with shorter branches in amylopectin significantly positively correlated with the amount of work contributed to the fast breakdown structure and slow breakdown structure, as well as their corresponding fractions during oral processing of cooked japonica rice. Furthermore, cooked japonica rice varieties with longer branches in amylopectin contained fewer fast breakdown structure and slow breakdown structure, while the contribution of the unbreakable structure to oral processing became more important.
We confirmed the fast breakdown structure is controlled by the fragmentation of rice kernel; the unbreakable structure is closely related to the undigested substance, which is controlled by the molecular entanglements among rice components during oral processing, while the slow breakdown structure is mainly controlled by the combination of rice kernel fragmentation and enzymatic degradation (11). Cooked japonica rice varieties with more amylose and shorter branches in amylopectin tend to form a harder texture, and more work is needed to contribute to the rice kernel fragmentation relating to the fast breakdown structure and slow breakdown structure. Meanwhile, cooked japonica rice varieties with longer branches of amylopectin possess stronger molecular entanglements and contribute more to the breakdown of the unbreakable structure. In summary, this study, for the first time, established the relationship between the oral processing properties of cooked japonica rice and its fine starch structure. This research offers a useful method to evaluate rice palatability and offers rice breeders new insights into selecting rice varieties with desirable oral processing properties with the required starch fine structure for cultivation.
Data availability statement
The original contributions presented in this study are included in the article/supplementary material, further inquiries can be directed to the corresponding author.
Author contributions
GL: methodology, investigation, data curation, and writing – original draft. RW, SL, and MX: methodology and investigation. LG and HZ: writing – review editing. HW: funding acquisition and writing – review editing. All authors contributed to the article and approved the submitted version.
Funding
This research was funded by the National Natural Science Foundation of China (grant nos. 31971841 and 32202085), Outstanding Youth Science Fund Project of Natural Science Foundation of Jiangsu Province (grant no: BK20220115), Earmarked fund for China Agriculture Research System (grant no: CARS-01-28), Earmarked Fund for Jiangsu Agricultural Industry Technology System, China (grant no: JATS [2021]502). A project funded by the Priority Academic Program Development of Jiangsu Higher Education Institutions (PAPD).
Conflict of interest
The authors declare that the research was conducted in the absence of any commercial or financial relationships that could be construed as a potential conflict of interest.
Publisher’s note
All claims expressed in this article are solely those of the authors and do not necessarily represent those of their affiliated organizations, or those of the publisher, the editors and the reviewers. Any product that may be evaluated in this article, or claim that may be made by its manufacturer, is not guaranteed or endorsed by the publisher.
Abbreviations
SEC, size-exclusion chromatography; FACE, fluorophore-assisted capillary electrophoresis; Mw, molecular weight; CLD, chain length distributions; DP, degree of polymerization; CAm, amylose content; Ap, amylopectin; TA/MEC, a texture analyzer equipped with a multiple extrusion cell probe; Type I structure, fast breakdown structure; Type II structure, slow breakdown structure; Type III structure, unbreakable structure.
References
1. Tian ZX, Qian Q, Liu QQ, Yan MX, Liu XF, Yan CJ, et al. Allelic diversities in rice starch biosynthesis lead to a diverse array of rice eating and cooking qualities. Proc Natl Acad Sci U.S.A. (2009) 106:21760–5. doi: 10.1073/pnas.0912396106
2. Cagampang GB, Perez CM, Juliano BO. A gel consistency test for eating quality of rice. J Sci Food Agric. (2010) 24:1589–94. doi: 10.1002/jsfa.2740241214
3. Little RR, Hiller GB, Son E. Differential effect of dilute alkali on 25 varieties of milled white rice. Cereal Chem. (1958) 35:111–26.
4. Guinard J-X, Mazzucchelli R. The sensory perception of texture and mouthfeel. Trends Food Sci Tech. (1996) 7:213–9. doi: 10.1016/0924-2244(96)10025-X
5. Champagne ET, Bett KL, Vinyard BT, Mcclung AM, Ii FEB, Moldenhauer K, et al. Correlation between cooked rice texture and rapid visco analyser measurements. Cereal Chem. (1999) 76:764–71. doi: 10.1094/cchem.1999.76.5.764
6. Chen J. Food oral processing—A review. Food Hydrocolloid. (2009) 23:1–25. doi: 10.1016/j.foodhyd.2007.11.013
7. He Y, Wang X, Chen J. Current perspectives on food oral processing. Annu Rev Food Sci T. (2022) 13:167–92. doi: 10.1146/annurev-food-052720-103054
8. Kaur B, Ranawana V, Henry J. The glycemic index of rice and rice products: A review, and table of GI values. Crit Rev Food Sci Nutr. (2016) 56:215–36. doi: 10.1080/10408398.2012.717976
9. Asimi S, Ren X, Zhang M, Zhongyan X, Amjad S, Liu D, et al. In vitro mastication of cooked rice: How it influences the bolus characteristics. J Food Process Eng. (2021) 45:e13922. doi: 10.1111/jfpe.13922
10. Sivakamasundari SK, Priyanka S, Moses JA, Anandharamakrishnan C. Impact of oral mastication on the in vitro digestibility of pigmented and non-pigmented rice varieties. Biointerface Res App. (2021) 12:1148–60. doi: 10.33263/briac121.11481160
11. Liu GD, Zhang C, Zhang SH, Liu XX, Luo JF, Gao H, et al. Simulated oral processing of cooked rice using texture analyzer equipped with multiple extrusion cell probe (TA/MEC) [Article]. LWT Food Sci Technol. (2021) 138:7. doi: 10.1016/j.lwt.2020.110731
12. Liu G, Zhang C, Gao H, Zhang H, Wei H. Water migration, texture and oral processing properties of semi-waxy rice during retrogradation. J Food Sci. (2021) 86:5100–6. doi: 10.1111/1750-3841.15959
13. Liu G, Liu S, Gao H, Zhang H, Wei H. High-level nitrogen application decreases eating quality of rice by affecting pasting, rheological, water migration, and oral processing properties. ACS Food Sci Technol. (2021) 1:754–61. doi: 10.1021/acsfoodscitech.0c00118
14. Li C, Ji Y, Li E. Understanding the influences of rice starch fine structure and protein content on cooked rice texture. Starch Stärke. (2022):2100253. doi: 10.1002/star.202100253
15. Li H, Prakash S, Nicholson TM, Fitzgerald MA, Gilbert RG. Instrumental measurement of cooked rice texture by dynamic rheological testing and its relation to the fine structure of rice starch. Carbohydr Polym. (2016) 146:253–63. doi: 10.1016/j.carbpol.2016.03.045
16. Li H, Prakash S, Nicholson TM, Fitzgerald MA, Gilbert RG. The importance of amylose and amylopectin fine structure for textural properties of cooked rice grains. Food Chem. (2016) 196:702–11. doi: 10.1016/j.foodchem.2015.09.112
17. Li H, Fitzgerald MA, Prakash S, Nicholson TM, Gilbert RG. The molecular structural features controlling stickiness in cooked rice, a major palatability determinant [Article]. Sci Rep. (2017) 7:43713. doi: 10.1038/srep43713
18. Liu W-C, Halley PJ, Gilbert RG. Mechanism of degradation of starch, a highly branched polymer, during extrusion. Macromolecules. (2010) 43:2855–64. doi: 10.1021/ma100067x
19. Li E, Cao P, Cao W, Li C. Relations between starch fine molecular structures with gelatinization property under different moisture content. Carbohydr Polym. (2022) 278:118955. doi: 10.1016/j.carbpol.2021.118955
20. Wu AC, Li E, Gilbert RG. Exploring extraction/dissolution procedures for analysis of starch chain-length distributions. Carbohydr Polym. (2014) 114:36–42. doi: 10.1016/j.carbpol.2014.08.001
21. Tikapunya T, Zou W, Yu W, Powell PO, Fox GP, Furtado A, et al. Molecular structures and properties of starches of Australian wild rice. Carbohydr Polym. (2017) 172:213–22. doi: 10.1016/j.carbpol.2017.05.046
22. Li E, Yang X, Li C. Combined effects of starch fine molecular structures and storage temperatures on long-term rice amylopectin retrogradation property. Int J Biol Macromol. (2022) 201:458–67. doi: 10.1016/j.ijbiomac.2022.01.092
23. Cave RA, Seabrook SA, Gidley MJ, Gilbert RG. Characterization of starch by size-exclusion chromatography: The limitations imposed by shear scission. Biomacromolecules. (2009) 10:2245. doi: 10.1021/bm900426n
24. Thattai M, Wu AC, Morell MK, Gilbert RG. A parameterized model of amylopectin synthesis provides key insights into the synthesis of granular starch. PLoS One. (2013) 8:e65768. doi: 10.1371/journal.pone.0065768
25. Li C, Gong B. Insights into chain-length distributions of amylopectin and amylose molecules on the gelatinization property of rice starches. Int J Biol Macromol. (2020) 155:721–9. doi: 10.1016/j.ijbiomac.2020.04.006
26. Vilaplana F, Hasjim J, Gilbert RG. Amylose content in starches: Toward optimal definition and validating experimental methods. Carbohydr Polym. (2012) 88:103–11. doi: 10.1016/j.carbpol.2011.11.072
27. Hanashiro I, Abe JI, Hizukuri S. A periodic distribution of the chain length of amylopectin as revealed by high-performance anion-exchange chromatography. Carbohydr Res. (1996) 283:151–9. doi: 10.1016/0008-6215(95)00408-4
Keywords: japonica rice, oral processing, starch fine structure, eating quality, palatability
Citation: Liu G, Wang R, Liu S, Xu M, Guo L, Zhang H and Wei H (2022) Relationship between starch fine structure and simulated oral processing of cooked japonica rice. Front. Nutr. 9:1046061. doi: 10.3389/fnut.2022.1046061
Received: 16 September 2022; Accepted: 17 October 2022;
Published: 03 November 2022.
Edited by:
Sen Ma, Henan University of Technology, ChinaReviewed by:
Jinhu Tian, Zhejiang University, ChinaHongyan Li, Beijing Technology and Business University, China
Copyright © 2022 Liu, Wang, Liu, Xu, Guo, Zhang and Wei. This is an open-access article distributed under the terms of the Creative Commons Attribution License (CC BY). The use, distribution or reproduction in other forums is permitted, provided the original author(s) and the copyright owner(s) are credited and that the original publication in this journal is cited, in accordance with accepted academic practice. No use, distribution or reproduction is permitted which does not comply with these terms.
*Correspondence: Haiyan Wei, hywei@yzu.edu.cn