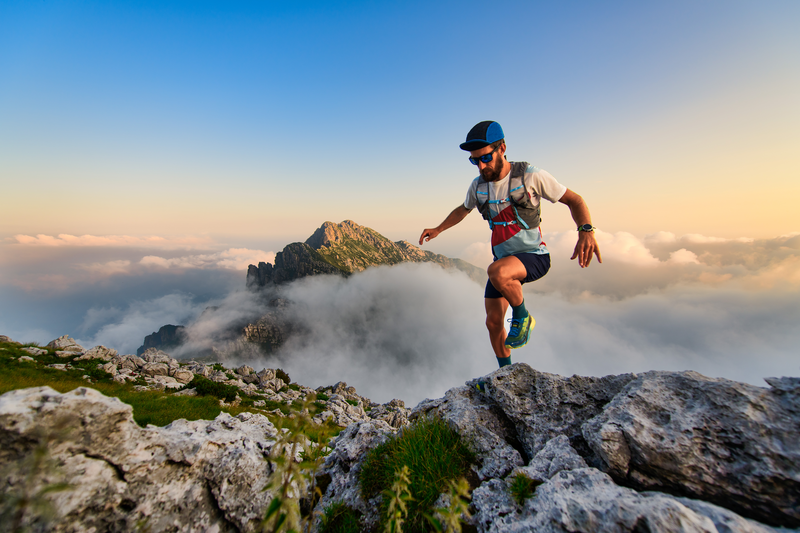
95% of researchers rate our articles as excellent or good
Learn more about the work of our research integrity team to safeguard the quality of each article we publish.
Find out more
ORIGINAL RESEARCH article
Front. Nutr. , 29 November 2022
Sec. Nutrition and Microbes
Volume 9 - 2022 | https://doi.org/10.3389/fnut.2022.1043055
This article is part of the Research Topic Impacts of Diet Pattern and Food Choice on Gut Microbiome Structure and Function View all 4 articles
The impact of fermentation by Levilactobacillus brevis YSJ3 on sleep-promoting components (SPCs) of carrot juice was evaluated. The contents of acetic acid, isovaleric acid, butyric acid, and γ-aminobutyric acid (GABA) significantly increased after fermentation. The beneficial effects of fermented carrot juice (FCJ) on sleep were evaluated in animal experiments. Behavioral test reveal SPCs-enriched FCJ could effectively relieve anxiety. The sleep duration in the FCJ group were extended compared to the control (NC) group and the unfermented carrot juice (UCJ) group. Moreover, the relative abundances of Ruminiclostridium and Akkermansia in the FCJ group and PC group, respectively, increased significantly, compared to the NC group the UCJ group. The contents of gut short-chain fatty acids in the FCJ group were significantly higher than that in the NC group and the UCJ group. The levels of GABA and 5-hydroxytryptamine in the brain for the FCJ group also increased significantly, compared to the NC group and the UCJ group. It indicated that SPCs-enriched FCJ effectively improved sleep in mice, which might be related to the fermentation of carrot juice and the compounds produced during the fermentation.
Insufficient sleep is increasingly problematic in modern society. Sufficient sleep is important for maintaining physical and mental health; yet, nearly a third of adults get less than 6 h of sleep a day (1). Insufficient sleep has been associated with a variety of adverse medical problems, such as obesity, diabetes, cardiovascular disease, neurocognitive impairment, and weakened immunity (2–5). Benzodiazepines and non-benzodiazepines are widely used to address insufficient sleep (6). However, these drugs are not without serious side effects, such as drug dependence and withdrawal reactions (7). As a result, diet modifications and dietary supplements have become alternate approaches to complementary and alternative medicine (8).
According to a recent report, many foods (animal-based foods, fruits, and vegetables) contain sleep-promoting components (SPCs), such as neurotransmitters (8) and short-chain fatty acids (SCFAs) (9). In particular, γ-aminobutyric acid (GABA), 5-hydroxytryptamine (5-HT), and butyrate have been linked to sleep (10–12). Indeed, dietary supplements of these components can effectively improve sleep quality (8). In addition, probiotics are also considered to be SPCs. Lin et al. found that Limosilactobacillus fermentum PS150™ could be used as a potential dietary supplement to improve sleep in pentobarbital-induced mice (13). Lactic fermentation is widely used in food processing, and lactic fermentation can enrich many active substances in foods, such as glucosinolates, peptides, SCFAs, neurotransmitters, vitamins, and exopolysaccharides (14). For example, Fuente et al. used Levilactobacillus brevis POM and Lactiplantibacillus plantarum (TR-7, TR-71, TR-14) to assist in the fermentation of an orange beverage, with high antioxidant activity (15). Wei et al. used Lactiplantibacillus plantarum B7 and C8-1 to ferment bog bilberry juice, and the GABA concentration increased significantly (16). It should be noted that there are no reports on the relationship between lactic fermentation and SPCs in vegetable juice.
In recent years, the relationship between gut microbiota and the gut–brain axis has attracted increasing attention (17, 18). The gut microbiota interferes with gastrointestinal physiology, metabolism, and immune function of hosts and affect the function and behavior of the central nervous system (CNS) (19–21). Bravo et al. found that anxiety and depressive behaviors were reduced in mice fed Lacticaseibacillus rhamnosus, but no changes in neurochemistry and behavior were found in mice with vagus nerve resection, suggesting the vagus nerve is the main communication route between gut microbiota and the brain (22). At present, a few diets have been shown to reduce anxiety-like behaviors and have physiological effects on the brain-gut axis (23). A recent study showed that schisandra chinensis improved anxiety- and depression-like behavior (24). Nevertheless, the effects of consuming vegetable-based fermentation juice rich in SPCs on sleep have not been studied.
This study aimed to investigate whether SPCs can be produced in FCJ by Levilactobacillus brevis (L. brevis) YSJ3. Furthermore, we investigated the effects of FCJ rich in SPCs on sleep. The results provide a theoretical basis for the application and development of SPCs-rich FCJ as a functional food.
Carrots were purchased from the local market (Provincial Academy of Agricultural Sciences vegetable farm, 200 Shiqiao Road, Hangzhou, Zhejiang, China). The carrots were cleaned, and roots were removed. They were cut into pieces and steamed in a steamer (Supor ZN28YK809-150, Zhejiang Supor Co., Ltd., Zhejiang, China). Then, using a 1:3 ratio of material to liquid (w/w), pure water was added, and the carrot was homogenized (high-speed blender; Ranbem-775, Guangdong, China). The carrot juice was filtered through a standard sample sieve with a diameter of 200 mm. Sugar (5% w/v; food grade) and sodium glutamate (0.5% w/v; food grade) were added. The mixture was stirred until dissolved, transferred to a 20-mL glass test tube, sealed, and sterilized in a vertical pressure steam sterilizer (YXQ-50SII, Shanghai Boxun Medical Biological Instrument Corp., China). The suitable condition for sterilization was 121°C high-pressure sterilization for 15 min (15, 25).
Current research suggested that L. brevis has good GABA synthesis ability (26). In our previous research, a high GABA-producing strain-L. brevis YSJ3 from Yeshanjun pickle (a traditional vegetable fermented food, Supplementary Figure 1) in Jingning She Autonomous County was screened. We found the GABA production from L. brevis YSJ3 reach about 760 μg/mL, which higher than the production of other L. brevis (Supplementary Table 1). Thus, L. brevis YSJ3 was selected as the fermentation stain. And L. brevis YSJ3 were deposited at the General Microbiology Center of China (CGMCC No.23307). The bacteria were identified by 16S rRNA gene sequence and subjected to whole genome sequencing using a combination of PacBio RS II Single Molecule Real Time (SMRT) and Illumina sequencing platforms. The genome of strain YSJ3 was deposited in NCBI under the accession numbers CP092264-CP092271. Chromosome genome maps of L. brevis YSJ3 chromosome are shown in Supplementary Figure 2. L. brevis YSJ3 were stored at −80°C in De Man, Rogosa and Sharpe (MRS, 20 g/L glucose, 4 g/L yeast extract, 10 g/L peptone, 8 g/L beef powder, 2 g/L dipotassium phosphate, 2 g/L ammonium citrate dibasic, 0.04 g/L manganese sulfate, 0.2 g/L magnesium sulfate, 1 mL/L tween-80, and 5 g/L sodium acetate) broth (CM1175, OXOID, Basingstoke, UK) containing 20% (v/v) glycerol (Sinopharm Chemical Reagent Co., Ltd., China) until use. L. brevis YSJ3 were propagated twice in MRS broth at 37°C for 30 h. Subsequently, the activated strain (8%, v/v) was inoculated in sterilized carrot juice and cultured at 37°C for 0, 12, 24, 36, and 48 h.
After 0, 12, 24, 36, and 48 h of culture, the cell viability of FCJ was determined by plate counting method (15). The sample was diluted with sterilized deionized water to obtain an appropriate cell density for measurement. Next, 100 μL of the sample was transferred to MRS agar (CM1163, OXOID, Basingstoke, UK) plates and incubated at 37°C for 48 h. The pH value of the sample was measured with a pH meter (METTLER TOLEDO, Zurich, Switzerland). Lactic acid concentration was tested by lactic acid content (LA) detection kit (Solatbio, Beijing, China).
The SCFAs produced in FCJ were determined by gas chromatography-mass spectrometry (GC-MS; Agilent 5977B-7890B, Agilent Technologies, Palo Alto, CA, USA). The sample treatment method was modified according to a previously reported method (27). Carrot juice was fermented for 0, 12, 24, 36, and 48 h. The samples were centrifuged at 8,000 rpm for 20 min (Tabletop Centrifuge; Velocity 18R 5426026, Dynamica Scientific Ltd., Livingston, UK). Then, the supernatant was acidified by adding 50% concentrated sulfuric acid (Shanghai Lingfeng Chemical Reagent Co., Ltd., China) at a ratio of 24:1 (v/v). The mixture was kept at room temperature for 5 min and vortexed (Vortex finder; Scientific Industries, Inc., Bohemia, NY, USA) mixed once every 60 s. Then, the mixture was centrifuged at 5,000 × g for 10 min. The supernatant was combined with anhydrous ether (Shanghai Lingfeng Chemical Reagent Co., Ltd., China) at a ratio of 1:1 (v/v). The mixture was vortexed for 30 s and centrifuged at 5,000 × g for 10 min. Finally, the ether layer was collected and stored in a 2-mL injection bottle until further analysis. Acetic acid, propionic acid, isobutyric acid, butyric acid, isovaleric acid, and valeric acid were used as the standard solutions (Shanghai Macklin Biochemical Co., Ltd., China).
HP-FFAP column (30 m × 250 μm × 0.25 μm; Agilent Technologies, Palo Alto, CA, USA) was used for chromatographic analysis. The program to run was: 2 min at 90°C, increase to 150°C at 12°C/min, increase to 220°C at 20°C/min, and hold for 4.5 min. The run time was 15 min. Helium was the carrier gas, with a flow rate of 1 mL/min, and splitless mode was used for sample injection. The ion source, MS transfer line temperatures and mode selection were determined by reference to the reports (28, 29).
High performance liquid chromatography-triple quadrupole mass spectrometry (LC-MS, SCIEX 5500 +, AB SCIEX, Massachusetts, USA) was used to determine the neurotransmitters produced in FCJ (30). First, the carrot juice samples fermented for 0, 12, 24, 36, and 48 h were centrifuged at 8,000 rpm for 20 min. The supernatant was combined with two times the volume of methanol (Merck KGaA, Darmstadt, Germany) and mixed via vortexing. The mixture was centrifuged at 12,000 rpm for 15 min at 4°C. The supernatant was collected and stored in a 2-mL injection bottle until further analysis. GABA (Shanghai Macklin Biochemical Co., Ltd., China) and 5-HT (Shanghai Aladdin Biochemical Technology Co., Ltd., China) were used as the standard solutions.
Chromatographic separation was performed using a ZORBAX Eclipse Plus C18 column (3.0 × 100 mm) with 1.8 μm particle size (Agilent Technologies Inc., Palo Alto, CA, USA). The injection volume was 1 μL. Eluent A consisted of ultra-pure water (Hangzhou Wahaha Group Co., Ltd., China) with 2 mM ammonium formate (Merck KGaA, Darmstadt, Germany) and 0.01% (v/v) formic acid (FA) (Merck KGaA, Darmstadt, Germany), and eluent B consisted of 100% methanol. The following gradient was applied: 0–0.5 min, 5% B; 0.5–5 min, 5–80% B; 5–6 min, 80% B; 6–6.1 min, 80–5% B; and 6.1–8 min, 5% B. The total runtime was 8 min, and the flow rate was 0.40 mL/min. The multiple reaction monitoring (MRM) transitions, declustering potential (DP), and collision energy (CE) are shown in Supplementary Table 2.
Specific pathogen free (SPF) ICR mice (male; 6 weeks of age) were purchased from Slack Laboratory Animal Co., Ltd. (Shanghai, China). Forty mice were randomly divided into four groups for treatment (n = 10): control (NC), unfermented carrot juice (UCJ), fermented carrot juice (FCJ), and positive control (PC) groups. Mice were placed in cages and subjected to a 12-h light/dark cycle in a room with temperature (23 ± 3°C) and humidity control (55 ± 15%). After acclimatization for 1 week, the mice in UCJ and FCJ group were orally given 10 mL/kg body weight (BW) of the UCJ and FCJ, respectively. The FCJ was fermented by L. brevis YSJ3 for 48 h. The number of viable bacteria was 108 CFU/mL. The mice in NC group were received an equal volume of 0.85% aseptic normal salin by oral gavage. Meanwhile, the PC group was received daily doses (10 mg⋅kg–1⋅day–1) of GABA solution (1 mg/mL, Yuanye Biotechnology Co., LTD., Shanghai, China) by oral gavage. The dose of 10 mg⋅kg–1⋅day–1 GABA was equivalent to consumption of 48.65 mg GABA day–1 by a 60 kg human according to formula for dose translation (31). The process took place between 8:00 and 16:00 every day for 37 days. The animals underwent a series of behavioral tests and sleep improvement tests for 7 days before the end of the study. In each behavioral test, the experimenters treated each animal blindly. At the end of the experiment, the mice were fasted for 12 h, and anesthetized by ether and dissected. Blood was collected from the eyeballs and stored at 4°C for 4 h for coagulation. Then, the serum was obtained by centrifugation at 5,000 rpm at 4°C for 30 min. The mice were sacrificed by cervical dislocation, and the intestinal tract and intestinal contents were dissected. The mouse skull was cut open, and the brain was quickly separated, weighed, and immersed in liquid nitrogen. All serum, brain, and fecal samples were stored at -80°C until extracted for further testing. A schematic of the experimental process is shown in Figure 1A. The research was carried out in compliance with the International Guiding Principles for Biomedical Research Involving Animals. All experimental protocols were approved by the Animal Ethics Committee of Zhejiang Academy of Agricultural Sciences (Committee approval #2022ZAASLA47).
Figure 1. Effects of control (NC) group, unfermented carrot juice (UCJ) group, fermented carrot juice (FCJ) group, and positive control (PC) group on behavioral test and sleep improvement test in mice. (A) Schematic representation of the experimental procedure; (B) captured images for OFT; (C) the time spent at the central area for OFT; (D) the time spent at open arms for EPM; (E) the time spent at light box for LDB; (F) the frequency of entry into the light box for LDB; (G) sodium pentobarbital-induced sleep duration test; (H) sub-threshold sodium pentobarbital dose-induced sleep test. Data are mean ± SEM (n = 10). Different letters indicate significant difference (p < 0.05).
The open field experimental box was a square box (50 × 50 × 40 cm). The color of the bottom was different from that of the mice to some extent. Open field tests (OFTs) were performed by following a modified protocol, as previously described (32–34). After 30 min of intragastric administration, each mouse was placed at the center of the device, and its activity within 5 min was recorded. After each mouse test, the instrument was washed with 75% ethanol to eliminate any possible odor cues. During the 5-min experiment, the movement routes and the time spent at the central area were recorded.
The light–dark box (LDB) consists of a light box and a dark box (100 × 50 × 50 cm), separated by a perforated partition (10 × 10 cm) in the middle. The light box provides a certain intensity of light. LDB tests were performed by following a modified protocol, as previously described (32). During the test, a mouse was gently placed in the central area of the light box in the LDB device, with its head facing the partition. The video recording system was used to track and record the behavioral performance of the mice in the LDB for 10 min. At the end of the test for each mouse, the instrument was cleaned with 75% alcohol before testing the next mouse. The time the mice spent in the light box and the frequency of entry into the light box were recorded. The mouse was considered to have completed the shuttle activity when its limbs completely entered the box from one side to the other.
The elevated plus maze (EPM) is composed of two open arms and two closed arms, each with a cross shape, suspended at a certain height. The length of the device was 70 cm, and the length of the arm was 30 cm. The width was 5 cm, and the height was 15 cm (32). The mouse was gently placed in the central platform region of the EPM device, with its head facing one of the open arms. The video recording system was used to track and record the behavioral performance of the mice in the EPM for 5 min. After each mouse was tested, the maze was cleaned with 75% alcohol before the next mouse was tested. The time the mice spent in the open arms was recorded. Mice were considered to be in the arm when their limbs were completely in one arm.
The dose of pentobarbital sodium intraperitoneal injection was preliminarily determined prior to the experiment. Thirty minutes after the last dose, each mouse was intraperitoneally injected 55 mg/kg.bw to induce sleep. The time from the disappearance of the righting reflex to the recurrence of the righting reflex was recorded as the sleep time of mice, and we recorded whether pentobarbital sodium prolonged the sleep duration in each group (34–37).
A preliminary experiment was conducted to determine the maximum subthreshold dose. Thirty minutes after the last dose, each mouse was intraperitoneally injected 40 mg/kg.bw to induce sleep. If the righting reflex of mice disappeared for more than 1 min, sleep was considered successful. The sleep onset ratio within 30 min was recorded (34–37).
Fecal genomic deoxyribonucleic acid (DNA) was extracted using DNA Kit (Omega Bio-tek, Norcross, GA, USA) according to the manufacturer’s instructions. 16S rRNA gene sequencing by the hypervariable regions V3–V4 (primer pairs 338F 5-ACTCCTACGGGAGGCAGCAG-3, and 806R 5-GGACTACH-VGGGTWTCTAAT-3) were performed by Shanghai Majorbio Bio-pharm Technology Co., Ltd. (Shanghai, China) using Illumina MiSeq platform (Illumina, San Diego, USA). The data processing and analysis is described in detail in Supplementary Methods.
Colon content samples were collected and determined by GC-MS (27). Briefly, colon content particles (0.1 g) were mixed with 1,200 μL ultrapure water, mashed, and swirled for 1 min. To the suspension, 50 μL of 50% sulfuric acid was added, and the mixture was kept at room temperature for 5 min and mixed every 60 s. After the sample was centrifuged at 5,000 × g for 10 min, the supernatant (500 μL) was transferred to a 1.5-mL centrifuge tube, and 500 μL anhydrous ether was added for 30 s. The sample was centrifuged at 5,000 × g for 10 min, and the upper ether layer was collected for GC-MS analysis. The instrumental analysis method was the same as described above.
The mice were killed by removing cervical vertebra, and the entire brain was removed by ice bath craniotomy. After the brain was weighed, ultra-pure water was added to the homogenate at the ratio of 1:1 (m/v), and it underwent ultrasonication for 10 s. Then, trifluoroacetic acid (TFA) (Macklin, China) was added in a volume ratio 5:1, and the protein was precipitated by whirlpool mixing. Next, the supernatant was centrifuged at 12,000 rpm for 20 min at 4°C and analyzed by LC-MS. The preparation method for serum and fecal samples was the same as that of the brain homogenate. GABA, 5-HT, 5-hydroxyindole-3-acetic acid (5-HIAA, Shanghai Aladdin Biochemical Technology Co., Ltd., China), dopamine (DA, Shanghai Macklin Biochemical Co., Ltd., China), and norepinephrine (NE, Shanghai Macklin Biochemical Co., Ltd., China) were used as the standard solutions. The instrumental analysis method was the same as described above.
Statistical analysis was performed using GraphPad Prism 9.0 software and SPSS 19.0 software. All data are expressed as mean ± standard deviation. All data in Table 1 were carried out with at least three replicates, and repeated measures ANOVA was used to analyze significance.
Table 1. L. brevis YSJ3 growth, pH, lactic acid, short chain fatty acids, and neurotransmitters in fermented carrot juice at different fermented time.
In mice experiments, a priori sample size calculations were not conducted. Behavioral test and sleep improvement test were carried out in 10 mice (n = 10). In addition, for the detection and analysis of gut microbes, four samples were used (n = 4). And each sample were collected from 2 to 3 mice (e.g., the fecal from the first and second mice was gathered together as the first sample). For the concentration of SCFAs in mice feces and the concentration of neurotransmitters in mice feces, brain, and serum, six mice were randomly selected from each experimental group (n = 6). All data were performed with a normal distribution (Shapiro-Wilk test). If assumptions were met normal distribution, one-way ANOVA were performed with Tukey’ multiple comparisons test. If assumptions were not met normal distribution, non-parametric test (Kruskal-Wallis) were performed with Dunn’s multiple comparisons test. The p-value of multiple comparisons (padj) was adjusted by family-wise significance and confidence levels of 0.05 (95% confidence interval). Statistical significance was acknowledged when p-values were below 0.05 (p < 0.05).
The cell viability of L. brevis YSJ3 in carrot juice after 12, 24, 36, and 48 h of fermentation was measured (Table 1). The bacteria were not detected in UCJ, which was used as the control group to evaluate the growth of L. brevis YSJ3. As the fermentation time increased, the colony numbers of L. brevis YSJ3 also increased. The fastest growth rate was observed from 0 to 12 h. When the fermentation time was 12 h, L. brevis YSJ3 entered a stable growth stage. The viable count of L. brevis YSJ3 remained around 2 × 108 CFU/mL. This indicates that L. brevis YSJ3 can grow in carrot juice due to its sugar content, which provides adequate energy and a carbon source for the bacteria. Fuente et al. and Multari et al. found that lactic acid bacteria could grow and live in plant-based fermented juices (15, 38). In addition, Table 1 shows that the pH of the FCJ decreased with fermentation time, and the lowest pH (3.78) was reached after 48 h of fermentation. On the contrary, the level of lactic acid increased with fermentation time. Thus, the higher bacterial growth decreases the pH and increases LA via organic acid metabolism, such as lactic acid and acetic acid. This result is in agreement with previously reported results by Hashemi et al. (39). Therefore, carrot juice rich in sugar is a suitable medium for the growth of lactic acid bacteria.
Certain probiotic strains (mainly lactic acid bacteria) are known to produce SCFAs and sleep-promoting neurotransmitters, GABA and 5-HT, by fermentation (14, 26). For this reason, these compounds were identified in L. brevis YSJ3 FCJ at different fermentation stages. The SCFAs concentration in FCJ at 12, 24, 36, and 48 h was determined by GC-MS (Table 1). Only butyric acid was detected in the UCJ, at a concentration of 39.30 ± 0.54 mg/L. As fermentation progressed, the content of acetic acid, isovaleric acid, and butyric acid significantly increased. The maximum concentration of acetic acid, isovaleric acid, and butyric acid reached 4912.14 ± 15.14, 18.68 ± 0.44, and 46.29 ± 0.67 mg/L, respectively. In contrast, propionic acid, isobutyric acid, and valeric acid were not detected in the FCJ. These results indicate that L. brevis YSJ3 in carrot juice produced acetic acid, isovaleric acid, and butyric acid, and these three fatty acids are typical metabolic products of organic acid metabolism by L. brevis. GABA and 5-HT concentrations in FCJ at 0, 12, 24, 36, and 48 h fermentation were determined by LC-MS (Table 1). No 5-HT was detected in unfermented and FCJ. However, GABA content significantly increased with increasing fermentation time from 0.52 ± 0.01 to 74.08 ± 0.28 mg/L. Therefore, L. brevis YSJ3 FCJ was rich in SCFAs and GABA, which would effectively improve sleep.
BW of mice showed no statistically significant differences among all the groups (Supplementary Table 3). OFT, LDB, and EPM tests are widely used for detecting anxiety-like behavior in animals (40). The captured images for OFT show the FCJ and PC groups were more frequently entered the central area then the UCJ and NC groups (Figure 1B). And the images of FCJ group and PC group were not different. It demonstrated that mice in FCJ group had a similar effect to mice in PC group. In addition, the FCJ and PC groups spent significantly more time in the central area (34.25 ± 13.67 and 41.25 ± 3.5 s, respectively), compared with the UCJ group (6.25 ± 5.85 s) and NC group (12.00 ± 2.94 s) (Figure 1C). Similarly, EPM and LDB tests showed that the FCJ and PC groups spent significantly more time in the open arms and light box (Figures 1D–F). Furthermore, the effects of FCJ, UCJ, PC, and NC treatments on sodium pentobarbital-induced sleeping behaviors are shown in Figure 1G. Sleep duration more than doubled after oral administration of FCJ and GABA compared to UCJ and saline. The sleep onset ratio of the FCJ and PC groups was 30 and 27%, respectively (Figure 1H). This represents a significant increase, compared with the UCJ groups (10%). These results demonstrate that SPCs-enriched FCJ could effectively relieve anxiety and improve sleep.
To assess the effect of FCJ on gut microbiota composition, the mice feces was detected by bacterial 16S rRNA sequencing. In the Venn diagram, the number of OTUs was not significantly different in all groups, and most OTUs were shared (Figure 2A). In addition, the α diversity indices for the UCJ and FCJ groups, including Shannon, Ace, and Chao1, were not significantly different, compared to the NC group (Figures 2B–D). This suggests that the microbial richness and diversity did not change after oral administration of normal saline and fermented and UCJ. In addition, PCoA shows that the beta diversity of the FCJ group was separated from the NC group (Figure 2E). But, the FCJ group and UCJ group did not differ. This indicates that some ingredients in carrot juice may change the composition and structure of mice gut microbiota. To identify specific taxa, relative abundances were assessed at the phylum and genus levels (Supplementary Tables 4, 5). Interestingly, Ruminiclostridium and Akkermansia found in the FCJ group and PC group, respectively, which was significantly higher than that in the NC group and UCJ group, as indicated by LEfSe (LDA Effect Size) multi-level species difference discriminant analysis and linear discriminant analysis (LDA) (Figures 2F,G).
Figure 2. Control (NC), unfermented carrot juice (UCJ), fermented carrot juice (FCJ), and positive control (PC) group gut microbiota composition (n = 4). (A) Venn diagram of OTU in the feces; (B) Shannon diversity indexes; (C) Ace diversity indexes; (D) Chao 1 diversity indexes; (E) PCoA analysis; (F) LEfSe multi-level species difference discriminant analysis; (G) linear discriminant analysis. *p < 0.05 was considered to indicate significant difference.
Currently, there is growing evidence linking changes in gut microbiota to gut metabolic changes (9). In particular, SCFAs can be produced by gut microbiota. Thus, acetic acid, propionic acid, isobutyric acid, butyric acid, isovaleric acid, and valeric acid were detected in mice colon content by GC-MS. The concentrations of acetic acid and butyric acid were higher than that of other SCFAs, and they observably improved in the FCJ and PC groups, compared to the NC and UCJ groups (Figures 3A,B). The concentration of propanoic acid, isobutyric acid, valeric acid, and isovaleric acid was not significantly different among the four groups. In addition, the total concentration of SCFAs in the FCJ and PC groups reached 43.83 and 45.06 mg/g, respectively, which were significantly higher than 24.85 and 25.93 mg/g in the NC and UCJ groups, respectively (Figure 3C).
Figure 3. The content of SCFAs and neurotransmitters in mice gut, serum, and brain. (A) The content of acetic acid in gut; (B) the content of butyric acid in gut; (C) the content of total SCFAs in gut; (D) the content of GABA in mice gut, serum, and brain; (E) the content of 5-HT in mice gut and brain. Different letters indicate significant difference (p < 0.05). Six mice were randomly selected from each experimental group (n = 6).
The content of neurotransmitters, such as GABA, 5-HT, 5-HIAA, DA, and NE, affects sleep quality and quantity (41). These five neurotransmitters were measured in mice gut and brain by LC-MS. Figures 3D,E show that the levels of GABA and 5-HT significantly increased in the brain after oral administration of GABA and FCJ. It is worth noting that the GABA content was not significantly different in the serum of all groups. The content was only about 0.6 ng/mL. Thus, GABA in the gut was possible not absorbed by the intestine into the bloodstream. But there was another possibility that the time of measurement may be missed in circulating GABA concentrations. In addition, the level of 5-HIAA, DA, and NE was not significantly different in mice gut and brain between the four groups (Supplementary Table 6).
Increasing evidence suggests that the incorporation of probiotics, SCFAs, and neurotransmitters as dietary supplements can mitigate insomnia and promote sleep quality or quantity (8, 22, 42). In our research, an SPCs-rich L. brevis YSJ3 FCJ by was developed to improve sleep function. L. brevis YSJ3 could increase the content of SCFAs and sleep-promoting neurotransmitters, such as acetic acid, isovaleric acid, butyric acid, and GABA. L. brevis are considered to be an SCFAs-producing strain via organic acid metabolism (26). In addition, L. brevis exhibited a strong ability to produce GABA, compared to other lactic acid bacteria (34). These GABA-producing bacteria synthesize GABA mainly via the glutamate decarboxylase (GAD) system (43, 44). The entire genome of L. brevis YSJ3 had been sequenced in our previous research (Supplementary Figure 2). The results of gene prediction show that L. brevis YSJ3 had a complete set of gad operons, including GadA/B, GadC, and GadR (Supplementary Figure 3). Furthermore, we proved that L. brevis YSJ3 can produce GABA in vitro from MRS broth (Supplementary Figure 4). Therefore, GABA can be produced and accumulated in FCJ via L. brevis YSJ3 fermentation. More importantly, mice treated with daily supplementation of SPCs-rich FCJ increased their entry frequency and time spent at the central area, open arms, and light box. Additionally, sleep improvement tests show that sleep duration of mice improved significantly after supplementation with FCJ.
The latest studies have suggested that certain dietary foods and supplements containing prebiotics, probiotics, and SPCs can improve sleep by affecting microbiota composition (24, 27). We compared the composition and diversity of bacterial communities from mice fecal samples from the FCJ, UCJ, PC, and NC groups. Alpha diversity analysis revealed that microbial richness and diversity did not change upon oral administration of GABA, FCJ, and UCJ. Similar results were reported by Song et al. and Tang et al. (41, 45). However, the beta diversity of the FCJ group was distinguished from that of the NC group and overlapped with that of the UCJ group, suggesting that some ingredients in carrot juice may change the composition and structure of mice gut microbiota. At the genus level, the relative abundances of Ruminiclostridium and Akkermansia significantly increased in the FCJ group and PC group, respectively, compared with those in the NC group and UCJ group. It is worth noting that these genera are all SCFAs-producing bacteria. Changes in probiotic levels could alter the ability to produce SCFAs. In this study, the fecal SCFAs level of the FCJ group was markedly higher than that of the NC and UCJ groups, especially acetic acid and butyric acid. Interestingly, butyrate has a positive effect on sleep via a sensory mechanism (42). Moreover, acetate has been shown to modulate the production of gut neurotransmitters (46). The high level of gut acetic acid and butyric acid was partly food-derived and partly from SCFAs-producing gut bacteria. Thus, consumption of foods rich in acetic acid and butyric acid can effectively improve the level of SCFAs in the gut.
GABA, a SPCs, can inhibit arousal systems to promote sleep. In general, GABA interacts through the vagus nerve and the production of other neurotransmitters to affect sleep (34, 47). In addition, some reports have proven that GABA can cross the blood brain barrier (48). Thus, we determined the content of GABA in the gut, serum, and brain of mice in all groups. The content of GABA in the gut and brain of the FCJ and PC groups was significantly increased compared with the NC group. However, a low level of GABA was found in mice serum, and no significant difference was determined for all groups. We speculated that gut-GABA may not interfere with sleep by crossing the blood-brain barrier. But the reasons need to be further verified. Indeed, intestinal tissues and vagal afferent neurons have a variety of GABA receptors, such as ionotropics GABAA receptor and metabotropic GABAB receptors (49). GABA can bind to these receptors to modulate the production and secretion of other neurotransmitters in the gut and brain. Our results revealed that only 5-HT in the brain of mice substantially increased after oral administration of FCJ and GABA. However, the level of 5-HIAA, DA, and NE was not significantly different in mice gut and brain. Many reports have suggested that probiotic administration, especially Lactobacillus and Bifidobacterium, can also improve sleep (31, 50–53). These strains perform functional communication by changing gut microbiota composition to increase the levels of several sleep-promoting metabolites (17, 22). Furthermore, these gut metabolites exert their effects through the vagus nerve (17). Therefore, oral administration of GABA and the consumption of L. brevis YSJ3-rich foods contribute to the improvement of sleep. But, the detailed mechanism is not clear, which needs further validation. In this study, we only have focused on the effects of SCFAs, GABA, and probiotics on sleep. However, FCJ also contain other substances, which may be related to sleep. In addition, this is a limitation of this paper in sample size and mixed fecal samples for gut microbiota analysis. In the further, a large sample size of gut microbiota analysis is required. Furthermore, detecting neurotransmitter levels at single time points may affect the accuracy of the results and conclusions. Therefore, the promoting sleep mechanisms of carrot-based fermentation juice need to be more detailed and in-depth studied.
In this work, the contents of SPCs (acetic acid, isovaleric acid, butyric acid, and GABA) increased significantly in carrot juice fermented by L. brevis YSJ3. The results of OFT, LDB, EPM, and sleep improvement tests show that oral administration of FCJ improved anxiety and sleep of mice. Moreover, the levels of SCFAs and GABA in the gut, as well as GABA and 5-HT in the brain, were significantly increased in the FCJ group, compared with NC group. The results support that SPCs-enriched FCJ has potential functionality to improve sleep. This probably linked to the fermentation of carrot juice and the compounds produced during the fermentation.
The original contributions presented in the study are included in the article/Supplementary material, further inquiries can be directed to the corresponding author/s. The data presented in the study are deposited in the NCBI repository, accession number PRJNA903512.
This animal study was reviewed and approved by the Animal Ethics Committee of Zhejiang Academy of Agricultural Sciences (Committee approval #2022ZAASLA47).
DYL and JZ prepared the fermented carrot juice, sleep-promoting components test, the animal experiments, and significantly contributed to writing the manuscript. JC performed all animal experiment. CZ was responsible for data processing and article revision. HY and DQL were responsible for designing and managing the entire study. All authors read and approved the final manuscript.
This work was supported by the National Natural Science Foundation of China (Nos. 32101920 and 32172180) and the Key Research and Development Program of Zhejiang Province (No. 2020c04008).
We gratefully acknowledge the technical support for GSMS and LCMS by Central Laboratory of Zhejiang Academy of Agricultural Sciences.
The authors declare that the research was conducted in the absence of any commercial or financial relationships that could be construed as a potential conflict of interest.
All claims expressed in this article are solely those of the authors and do not necessarily represent those of their affiliated organizations, or those of the publisher, the editors and the reviewers. Any product that may be evaluated in this article, or claim that may be made by its manufacturer, is not guaranteed or endorsed by the publisher.
The Supplementary Material for this article can be found online at: https://www.frontiersin.org/articles/10.3389/fnut.2022.1043055/full#supplementary-material
1. Zhang SL, Bai L, Goel N, Bailey A, Jang CJ, Bushman FD, et al. Human and rat gut microbiome composition is maintained following sleep restriction. Proc Natl Acad Sci USA. (2017) 114:E1564–71. doi: 10.1073/pnas.1620673114
2. Moller-Levet CS, Archer SN, Bucca G, Laing EE, Slak A, Kabiljo R, et al. Mortality associated withshort sleep duration: the evidence, the possible mechanisms, and the future. Proc Natl Acad Sci USA. (2013) 110:E1132–41. doi: 10.1016/j.smrv.2009.07.006
3. Markwald RR, Melanson EL, Smith MR, Higgins J, Perreault L, Eckel RH, et al. Impact of insufficient sleep on total daily energy expenditure, food intake, and weight gain. Proc Natl Acad Sci USA. (2013) 110:5695–700.
4. Yuan RK, Zitting KM, Duffy JF, Vujovic N, Wang W, Quan SF, et al. Chronic sleep restriction while minimizing circadian disruption does not adversely affect glucose tolerance. Front Physiol. (2021) 12:764737. doi: 10.3389/fphys.2021.764737
5. Prather AA, Gurfein B, Moran P, Daubenmier J, Acree M, Bacchetti P, et al. Tired telomeres: poor global sleep quality, perceived stress, and telomere length in immune cell subsets in obese men and women. Brain Behav Immun. (2015) 47:155–62. doi: 10.1016/j.bbi.2014.12.011
6. Wagner J, Wagner ML. Non-benzodiazepines for the treatment of insomnia. Sleep Med Rev. (2000) 4:551–81.
7. Chapoutot M, Peter-Derex L, Bastuji H, Leslie W, Schoendorff B, Heinzer R, et al. Cognitive behavioral therapy and acceptance and commitment therapy for the discontinuation of long-term benzodiazepine use in insomnia and anxiety disorders. Int J Env Res. (2021) 18:10222. doi: 10.3390/ijerph181910222
8. Briguglio M, Dellosso B, Panzica G, Malgaroli A, Banfi G, Dina CZ, et al. Dietary neurotransmitters: a narrative review on current knowledge. Nutrients. (2018) 10:591.
9. Kenneth JOR, Michael KC, Gerard MM, Emily GK, María RA, Christine F, et al. Short chain fatty acids: microbial metabolites for gut-brain axis signalling. Mol Cell Endocrinol. (2022) 546:111572.
10. Li D, Li L, Xiao GN, Limwachiranon J, Xu YQ, Lu HY, et al. Effects of elevated CO2 on energy metabolism and γ-aminobutyric acid shunt pathway in postharvest strawberry fruit. Food Chem. (2018) 265:281–9. doi: 10.1016/j.foodchem.2018.05.106
11. Ni YH, Hu L, Yang S, Ni LY, Ma LY, Zhao YF, et al. Bisphenol a impairs cognitive function and 5-HT metabolism in adult male mice by modulating the microbiota-gut-brain axis. Chemosphere. (2021) 282:130952. doi: 10.1016/j.chemosphere.2021.130952
12. Lin SS, Feng Y, Hu LF, Lin CL, Ye R, Yuan ZZ. Jiaotaiwan increased GABA level in brain and serum, improved sleep via increasing NREM sleep and REM sleep, and its component identification. J Ethnopharmacol. (2022) 285:114866. doi: 10.1016/j.jep.2021.114866
13. Lin A, Shih CT, Huang CL, Wu CC, Lin CT, Tsai YC. Hypnotic effects of Lactobacillus fermentum PS150(TM) on pentobarbital-induced sleep in mice. Nutrients. (2019) 11:2409. doi: 10.3390/nu11102409
14. Chang YH, Jeong CH, Cheng WN, Choi Y, Shin DM, Lee S, et al. Quality characteristics of yogurts fermented with short-chain fatty acid-producing probiotics and their effects on mucin production and probiotic adhesion onto human colon epithelial cells. J Dairy Sci. (2021) 104:7415–25. doi: 10.3168/jds.2020-19820
15. Fuente BDL, Luz C, Puchol C, Meca G, Barba FJ. Evaluation of fermentation assisted by Lactobacillus brevis pom, and Lactobacillus plantarum (TR-7, TR-71, TR-14) on antioxidant compounds and organic acids of an orange juice-milk based beverage. Food Chem. (2021) 343:128414. doi: 10.1016/j.foodchem.2020.128414
16. Wei M, Wang SY, Gu P, Ouyang XY, Liu SX, Li YQ, et al. Comparison of physicochemical indexes, amino acids, phenolic compounds and volatile compounds in bog bilberry juice fermented by Lactobacillus plantarum under different pH conditions. J Food Sci Tech Mys. (2018) 55:2240–50. doi: 10.1007/s13197-018-3141-y
17. Sen P, Molinero-Perez A, O’Riordan KJ, Mccafferty CP, O’Halloran KD, Cryan JF. Microbiota and sleep: awakening the gut feeling. Trends Mol Med. (2021) 27:935–45. doi: 10.1016/j.molmed.2021.07.004
18. Song XR, Wang LY, Liu YN, Zhang X, Weng PF, Liu LL, et al. The gut microbiota–brain axis: role of the gut microbial metabolites of dietary food in obesity. Food Res Int. (2022) 153:110971. doi: 10.1016/j.foodres.2022.110971
19. Forsythe P, Bienenstock J, Kunze WA. Vagal pathways for microbiome-brain-gut axis communication. Microb Endocrinol Microb Gut Brain Axis Health Dis. (2014) 817:115–33.
20. Wang Y, Kasper LH. The role of microbiome in central nervous system disorders. Brain Behav Immun. (2014) 38:1–12.
21. Ghaisas S, Maher J, Kanthasamy A. Gut microbiome in health and disease: linking the microbiome-gut-brain axis and environmental factors in the pathogenesis of systemic and neurodegenerative diseases. Pharmacol Therapeut. (2016) 158:52–62. doi: 10.1016/j.pharmthera.2015.11.012
22. Bravo JA, Forsythle P, Chew MV, Escaravage E, Savignac HM, Dinan TG, et al. Ingestion of Lactobacillus strain regulates emotional behavior and central GABA receptor expression in a mouse via the vagus nerve. Proc Natl Acad Sci USA. (2011) 108:16050–5. doi: 10.1073/pnas.1102999108
23. Wu M, Tian T, Mao Q, Zou T, Zhou CJ, Xie J, et al. Associations between disordered gut microbiota and changes of neurotransmitters and short-chain fatty acids in depressed mice. Trans Psychiat. (2020) 10:350. doi: 10.1038/s41398-020-01038-3
24. Neroni B, Evangelisti M, Radocchia G, Di Nardo G, Pantanella F, Villa MP, et al. Relationship between sleep disorders and gut dysbiosis: what affects what? Sleep Med. (2021) 87:1–7.
25. Sireswar S, Dey G, Biswas S. Influence of fruit-based beverages on efficacy of Lacticaseibacillus rhamnosus GG (Lactobacillus rhamnosus GG) against DSS-induced intestinal inflammation. Food Res Int. (2021) 149:110661. doi: 10.1016/j.foodres.2021.110661
26. Cataldo PG, Villegas JM, Giori G, Saavedra L, Hebert EM. Enhancement of γ-aminobutyric acid (GABA) production by Lactobacillus brevis CRL2013 based on carbohydrate fermentation. Int J Food Microbiol. (2020) 333:108792.
27. Tong LJ, Hao HN, Zhang XY, Zhang Z, Lv YY, Zhang LW, et al. Oral administration of bovine milk-derived extracellular vesicles alters the gut microbiota and enhances intestinal immunity in mice. Mol Nutr Food Res. (2020) 64:1901251. doi: 10.1002/mnfr.201901251
28. Han X, Guo JL, You YL, Yin MW, Ren CL, Zhan JC, et al. A fast and accurate way to determine short chain fatty acids in mouse feces based on GC-MS. J Chromatogr B. (2018) 1099:73–82. doi: 10.1016/j.jchromb.2018.09.013
29. Zhang J, Zhang C, Lei P, Xin X, Liu D, Yi H. Isolation, purification, identification, and discovery of the antibacterial mechanism of ld-phenyllactic acid produced by Lactiplantibacillus plantarum CXG9 isolated from a traditional Chinese fermented vegetable. Food Control. (2022) 132:108490.
30. Wang LS, Zhang MD, Tao X, Zhou YF, Liu XM, Pan RL, et al. LC-MS/MS-based quantification of tryptophan metabolites and neurotransmitters in the serum and brain of mice. J Chromatogr B. (2019) 1112:24–32. doi: 10.1016/j.jchromb.2019.02.021
31. Reagan-Shaw S, Nihal M, Ahmad N. Dose translation from animal to human studies revisited. FASEB J. (2008) 22:659.
32. Borbely E, Hajna Z, Nabi L, Scheich B, Tekus V, Laszlo K, et al. Hemokinin-1 mediates anxiolytic and anti-depressant-like actions in mice. Brain Behav Immun. (2017) 59:219–32. doi: 10.1016/j.bbi.2016.09.004
33. Lim DW, Park J, Han D, Lee J, Kim YT, Lee C. Anti-inflammatory effects of asian fawn lily (erythronium japonicum) extract on lipopolysaccharide-induced depressive-like behavior in mice. Nutrients. (2020) 12:3809. doi: 10.3390/nu12123809
34. Yu LL, Han X, Cen S, Duan H, Feng SS, Xue YH, et al. Beneficial effect of GABA-rich fermented milk on insomnia involving regulation of gut microbiota. Microbiol Res. (2020) 233:126409. doi: 10.1016/j.micres.2020.126409
35. Um MY, Yoon M, Lee J, Jung J, Cho S. A novel potent sleep-promoting effect of turmeric: turmeric increases non-rapid eye movement sleep in mice via histamine H-1 receptor blockade. Mol Nutr Food Res. (2021) 65:2100100. doi: 10.1002/mnfr.202100100
36. Qian JJ, Zheng L, Su GW, Huang MT, Luo DH, Zhao MM. Identification and screening of potential bioactive peptides with sleep-enhancing effects in bovine milk casein hydrolysate. J Agr Food Chem. (2021) 69:11246–58. doi: 10.1021/acs.jafc.1c03937
37. Kim YR, Lee SY, Lee SM, Shim I, Lee MY. Effect of Hibiscus syriacus linnaeus extract and its active constituent, saponarin, in animal models of stress-induced sleep disturbances and pentobarbital-induced sleep. Biomed Pharmacother. (2022) 146:112301. doi: 10.1016/j.biopha.2021.112301
38. Multari S, Carafa I, Barp L, Caruso M, Licciardello C, Larche R, et al. Effects of Lactobacillus spp. on the phytochemical composition of juices from two varieties of Citrus sinensis L. osbeck: ‘tarocco’ and ‘washington navel’. LWT Food Sci Technol. (2020) 125:109205.
39. Hashemi SMB, Mousavi Khaneghah A, Barba FJ, Nemati Z, Sohrabi Shokofti S, Alizadeh F. Fermented sweet lemon juice (Citrus limetta) using Lactobacillus plantarum LS5: chemical composition, antioxidant and antibacterial activities. J Funct Foods. (2017) 38:409–14.
40. Kuniishi H, Ichisaka S, Yamamoto M, Ikubo N, Matsuda S, Futora E, et al. Early deprivation increases high-leaning behavior, a novel anxiety-like behavior, in the open field test in rats. Neurosci Res. (2017) 123:27–35. doi: 10.1016/j.neures.2017.04.012
41. Tang QH, Xiong J, Wang JW, Cao Z, Liao SQ, Xiao Y, et al. Queen bee larva consumption improves sleep disorder and regulates gut microbiota in mice with PCPA-induced insomnia. Food Biosci. (2021) 43:101256.
42. Szentirmai E, Millican NS, Massie AR, KapAs L. Butyrate, a metabolite of intestinal bacteria, enhances sleep. Sci Rep. (2019) 9:7035. doi: 10.1038/s41598-019-43502-1
43. Wang YS, Luo ZS, Huang XD, Yang KL, Gao SJ, Du RX. Effect of exogenous γ-aminobutyric acid (GABA) treatment on chilling injury and antioxidant capacity in banana peel. Sci Hortic. (2014) 168:132–7.
44. Xiao TT, Shah NP. Lactic acid produced by Streptococcus thermophilus activated glutamate decarboxylase (GadA) in Lactobacillus brevis NPS-QW145 to improve γ-amino butyric acid production during soymilk fermentation. LWT Food Sci Technol. (2020) 137:110474.
45. Song YG, Shan BX, Zeng SF, Zhang J, Jin C, Liao Z, et al. Raw and wine processed Schisandra chinensis attenuate anxiety like behavior via modulating gut microbiota and lipid metabolism pathway. J Ethnopharmacol. (2021) 266:113426. doi: 10.1016/j.jep.2020.113426
46. O’Riordan KJ, Collins MK, Moloney GM, Knox EG, Aburto MR, Fulling CM, et al. Short chain fatty acids: microbial metabolites for gut-brain axis signalling. Mol Cell Endocrinol. (2022) 546:111572.
47. Han MQ, Yuan SY, Zhang JC. The interplay between sleep and gut microbiota. Brain Res Bull. (2022) 180:131–46.
48. Boonstra B, Kleijn RD, Colzato LS, Alkemade A, Forstman BU, Nieuwenhuis S. Neurotransmitters as food supplements: the effects of gaba on brain and behavior. Front Psychol. (2015) 6:1520. doi: 10.3389/fpsyg.2015.01520
49. Hyland NP, Cryan JF. A gut feeling about gaba: focus on gabab receptors. Front Psychol. (2010) 1:124. doi: 10.3389/fphar.2010.00124
50. Horii Y, Nakakita Y, Fujisaki Y, Yamamoto S, Itoh N, Miyazaki K, et al. Effects of intraduodenal injection of Lactobacillus brevis SBC8803 on autonomic neurotransmission and appetite in rodents. Neurosci Lett. (2013) 539:32–7. doi: 10.1016/j.neulet.2013.01.037
51. Nishida K, Sawada D, Kuwano Y, Tanaka H, Rokutan K. Health benefits of Lactobacillus gasseri CP2305 tablets in young adults exposed to chronic stress: a randomized, double-blind, placebo-controlled study. Nutrients. (2019) 11:1859. doi: 10.3390/nu11081859
52. Marotta A, Sarno E, Del Casale A, Pane M, Mogna L, Amoruso A, et al. Effects of probiotics on cognitive reactivity, mood, and sleep quality. Front Psychol. (2019) 10:164. doi: 10.3389/fpsyt.2019.00164
Keywords: Levilactobacillus brevis YSJ3, fermented carrot juice, sleep-promoting components, improved sleep, mice
Citation: Liu D, Zhang J, Chen J, Zhang C, Yi H and Liu D (2022) Carrot-based fermentation juice rich in sleep-promoting components improved sleep in mice. Front. Nutr. 9:1043055. doi: 10.3389/fnut.2022.1043055
Received: 13 September 2022; Accepted: 10 November 2022;
Published: 29 November 2022.
Edited by:
J. Philip Karl, U.S. Army Research Institute of Environmental Medicine (USARIEM), United StatesCopyright © 2022 Liu, Zhang, Chen, Zhang, Yi and Liu. This is an open-access article distributed under the terms of the Creative Commons Attribution License (CC BY). The use, distribution or reproduction in other forums is permitted, provided the original author(s) and the copyright owner(s) are credited and that the original publication in this journal is cited, in accordance with accepted academic practice. No use, distribution or reproduction is permitted which does not comply with these terms.
*Correspondence: Huaxi Yi, eWloeEBvdWMuZWR1LmNu; Daqun Liu, ZGFxdW4ubGl1QGhvdG1haWwuY29t
†These authors have contributed equally to this work
Disclaimer: All claims expressed in this article are solely those of the authors and do not necessarily represent those of their affiliated organizations, or those of the publisher, the editors and the reviewers. Any product that may be evaluated in this article or claim that may be made by its manufacturer is not guaranteed or endorsed by the publisher.
Research integrity at Frontiers
Learn more about the work of our research integrity team to safeguard the quality of each article we publish.