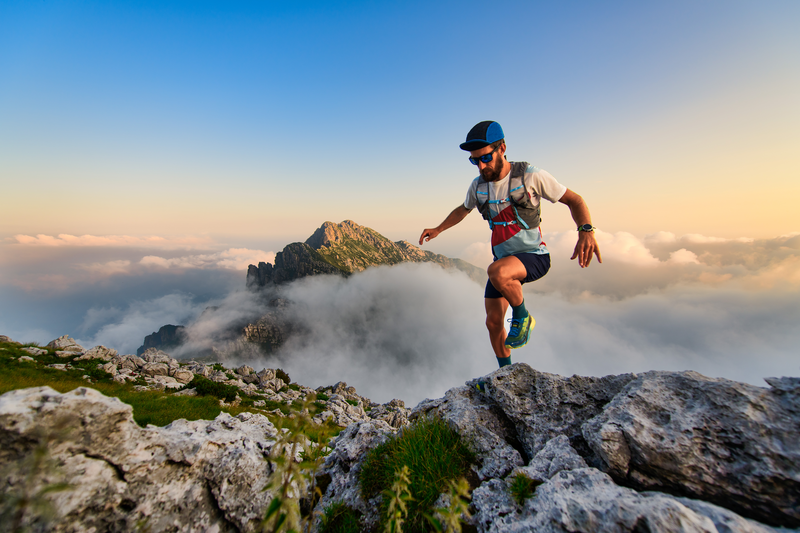
94% of researchers rate our articles as excellent or good
Learn more about the work of our research integrity team to safeguard the quality of each article we publish.
Find out more
ORIGINAL RESEARCH article
Front. Nutr. , 30 November 2022
Sec. Food Chemistry
Volume 9 - 2022 | https://doi.org/10.3389/fnut.2022.1042590
Introduction: The extensive occurrence of acrylamide in heat processing foods has continuously raised a potential health risk for the public in the recent 20 years. Machine learning emerging as a robust computational tool has been highlighted for predicting the generation and control of processing contaminants.
Methods: We used the least squares support vector regression (LS-SVR) as a machine learning approach to investigate the effects of flavone carbon and oxygen glycosides on acrylamide formation under a low moisture condition. Acrylamide was prepared through oven heating via a potato-based model with equimolar doses of asparagine and reducing sugars.
Results: Both inhibition and promotion effects were observed when the addition levels of flavonoids ranged 1–10,000 μmol/L. The formation of acrylamide could be effectively mitigated (37.6%–55.7%) when each kind of flavone carbon or oxygen glycoside (100 μmol/L) was added. The correlations between acrylamide content and trolox-equivalent antioxidant capacity (TEAC) within inhibitory range (R2 = 0.85) had an advantage over that within promotion range (R2 = 0.87) through multiple linear regression.
Discussion: Taking ΔTEAC as a variable, a LS-SVR model was optimized as a predictive tool to estimate acrylamide content (R2inhibition = 0.87 and R2promotion = 0.91), which is pertinent for predicting the formation and elimination of acrylamide in the presence of exogenous antioxidants including flavonoids.
Acrylamide, a recognized food endogenous contaminant, was first discovered in baked and fried foods via the Swedish National Food Administration (SNFA). International Agency for Research on Cancer has classified acrylamide as a probable carcinogen to humans (Group 2A substance) (1). Numerous laboratories and institutes have been devoted to investigating the formation and reduction of acrylamide for its widely acknowledged neural, genetic and reproductive toxicities (2, 3). Maillard reaction between the amino acid asparagine and reducing sugars can generate acrylamide within a wide range, depending on reaction time, reaction temperature, pH, etc. (4, 5). Several key intermediates like Schiff base, Amadori products and 3-aminopropionamide have been verified to contribute to the generation of acrylamide (6).
Maillard reaction, the way to the formation of acrylamide, usually occurs during heat processing such as frying, baking and roasting, which is actually under a low-moisture condition. Water activity is an important index in food matrices due to its affection of lipid oxidation rates, the growth rate of food microorganism, browning rate and enzyme activity. The Maillard reaction usually occurs at a water activity of 0.3–0.7 with the maximum reaction rate also being affected by the type of food (7), which indicates water activity can influence acrylamide formation. Some previous studies have revealed that low initial water activity of the system results in a high formation of acrylamide, pointing out that acrylamide formation occurs to a large extent only when the moisture content is below 5% (8, 9).
Given that acrylamide is a Maillard reaction associated product, the major challenge is to simultaneously reduce the formation of acrylamide and remain original color, taste, texture and flavor properties in food (3). Three major mitigation recipes were recommended to reduce acrylamide formation, including the alteration of raw materials, the optimization of heat processing parameters, and the addition of exogenous substances (10). Several studies have found that herbal extracts could effectively inhibit the generation of acrylamide (11). For instance, the formation of acrylamide can be reduced by green tea extracts in fried potatoes (12) and fried chicken drumsticks and chicken wings (13). Flavone carbon and oxygen glycosides inhibit the acrylamide generation by trapping carbohydrates and decreasing lipid oxidation presumably (14). Hitherto, few studies have discussed the effect of flavone carbon and oxygen glycosides on the acrylamide reduction in a low-moisture system.
Many studies have found different effects of various herbal extracts on acrylamide formation in the low-moisture system. Our previous study reported the extracts from both bamboo leaves and green tea could inhibit the acrylamide generation (15). However, oleuropein as a typical polyphenolic compound could promote the generation of acrylamide although many phenolic acids such as caffeic acid, chlorogenic acid, ellagic acid, epicatechin acid, punicalagin and tyrosol could reduce the formation of acrylamide in an asparagine-fructose model system (16). These results indicated that natural antioxidants may possess both reduction and promotion effects on the acrylamide formation. However, it is still unclear how typical natural antioxidants exert their dose-dependent way to the effect on the formation of acrylamide. Additionally, previous studies showed the valuable application of artificial intelligence in food research field (17, 18). Support vector regression (SVR) has unique advantages that it does not need a large number of training samples for developing models and is not affected by the presence of outliers (19).
In the current study, we aim to investigate and compare the dual effect of two typical kinds of flavonoids, flavone carbon and oxygen glycosides, as the representative of natural antioxidants on the acrylamide generation, and employ the machine learning models to associate the concentration of acrylamide with the antioxidant properties of Maillard reaction products (MRP) and predict the chemoprevention of acrylamide.
Apigenin-7-O-glucoside, luteolin-7-O-glucoside, and luteolin-4′-O-glucoside were supplied by Extrasynthese Co. (Lyon, France). Homoorientin, orientin, isovitexin, and vitexin were isolated and purified from antioxidants of bamboo leaves by preparative high-performance liquid chromatography (HPLC) on the basis of our previous studies (20). Acrylamide, L-asparagine monohydrate, D-(+)-glucose monohydrate, 2,2-diphenyl-1-picrylhydrazyl (DPPH), 2,2-azino-bis-(3-ethylbenzothiazoline-6-sulfonic acid) (ABTS), 6-hydroxy-2,5,7,8-tetramethylchromane-2-carboxylic acid (trolox), potassium persulfate and 2,4,6-tri(2-pyridyl)-s-triazine (TPTZ) were purchased from Sigma-Aldrich (St. Louis, MO, USA). D3-labeled acrylamide (isotopic purity ≥ 99%) was supplied by Cambridge Isotope Laboratories (Andover, MA, USA). Atlantis potato powder was purchased from Sanjiang (Group) Potato Products Co., Ltd. (Lintao, Gansu, China).
A potato-based equimolar asparagine-reducing sugar system was used to investigate the role of flavone carbon and oxygen glycosides. Oven heating, a high-speed and short-time internal heating method, was used to reach the heating temperature that the Maillard reaction needed according to previous work (20). Potato powder (50 g) and the reactant powder of asparagine (0.14 mol) and glucose (0.14 mol) were grinded carefully to ensure adequate reaction. On the basis of our previous study using HPLC (21), the original concentrations of asparagine (0.037 mg) and glucose (0.015 mg) measured in potato powder (100 mg) could be negligible compared with the equimolar addition concentrations of asparagine (1 mmol) and glucose (1 mmol) used in the experiment.
On account of mimicking the reduction of acrylamide by flavonoids in the asparagine-glucose reaction system, gradient addition levels of each kind of flavone carbon and oxygen glycosides were added into the treatment groups, while phosphate buffer (0.1 mol/L, pH 6.80) was added into the control groups. A series of working solutions of standards (100, 500, 1,000, 5,000, 10,000, 50,000, 100,000, 500,000, and 1,000,000 μmol/L) was diluted in phosphate buffer. Then, 100 μL working solution was added into the low-moisture system. The oven was adjusted to the predesigned temperature (180°C). The mixed reactant powders were hermetically sealed in hard beakers and then went through the oven-heating process under the selected heating temperature (180°C) at a set time (15 min), the optimal heating conditions when the acrylamide generation reached the maximal level were used according to previous study (22). After the heating stage, the hard-beakers were carefully taken out from the oven and transferred into an ice bath immediately to stop further reaction. The cooled reaction products were stored at 4°C to avoid Maillard reaction for further use. The concentrations of flavone carbon and oxygen glycosides in reaction solutions (10 ml) for the sample treatment were 1, 5, 10, 50, 100, 500, 1,000, 5,000, and 10,000 μmol/L in different treatment groups.
The method used for the sample treatment and the analysis of acrylamide by UHPLC-MS/MS were conducted according to our previous study (23). Firstly, PBS (10 ml, 0.1 mol/L, pH 6.8) was used to dissolve the cooled MRP, followed by the addition of D3-acrylamide (500 μL, 2 μg/ml). Then, the mixed solution (2 ml) was liquid-liquid extracted using ethyl acetate (2 ml) thrice. The organic phase was fused and dried with nitrogen gas. Then, 1.5 ml water was used to redissolve the residue and Oasis HLB cartridges (6 ml, 200 mg; Waters Technology, Milford, MA, USA) were used for solid phase extraction (SPE) purification according to previous study (22). Finally, the collected eluent was used for injection for the UHPLC-MS/MS analysis.
We used an Acquity ultra-high performance liquid chromatograph equipped with vacuum degasser, sample tray and autosampler (Waters, Milford, MA, USA) to conduct the UHPLC analysis. We completed the UHPLC separation by using a Waters UPLC BEH C18 column (50 mm × 2.1 mm i.d., 1.7 μm). Tandem mass spectrometry was performed on a Quattro Ultima Pt tandem mass spectrometer (Micromass Company Inc., Manchester, UK) using an electrospray source in a positive ion mode (ESI+) to detect characteristic precursors and product ions of acrylamide. The conditions used for the electrospray source were as follows: capillary voltage, 3.5 kV; cone voltage, 50 V; source temperature, 100°C; desolvation gas temperature, 350°C; desolvation gas flow, 400 L/h nitrogen; cone gas flow, 45 L/h nitrogen; argon collision gas pressure to 3 × 10–3 mbar for MS/MS, which gave a highest acrylamide response in this study. The collision energy (CE) was optimized for each multiple reaction monitored (MRM) transition (20).
Three antioxidant evaluation methods [DPPH, ABTS, and ferric ion reducing antioxidant power (FRAP) assays] were conducted to investigate the antioxidant characteristics of MRP. (i) DPPH assay was used to determine the radical scavenging activity of MRP by a spectrophotometer (Shengke Instrument Equipment Co., Ltd., Shanghai, China) based on a modified procedure (24). An aliquot of sample (20 μL) after SPE treatment was reacted with 5 ml of DPPH solution (74 mg/L) at room temperature in the dark for 1 h. UV-Vis readings were recorded at 520 nm. A series of aqueous trolox solutions (0–0.4 mmol/L) were measured in the same condition and used to calibrate the results. (ii) ABTS assays was conducted according to a previous work (25). An aliquot of sample treatment solution (5 μL) was added into 5 ml of ABTS+ solution (70 μmol/L) and reacted for 20 min. The decline of absorbance was recorded at 730 nm in 10 min. Aqueous trolox solutions (0–0.12 mmol/L) were measured and used to calibrate the results. (iii) FRAP assay was also conducted on the basis of previous study with modifications (21). The FRAP reagent included TPTZ (10 mmol/L), FeCl3 (20 mmol/L), and acetate buffer (0.3 mol/L) in a certain ratio (1:1:10). SPE-treated sample (6 μL) was added into 4.5 ml FRAP working solution. Then, the absorbance was measured at 595 nm after vortex mixing. Calibration procedure was conducted using a linear range of 0–0.3 mmol/L Trolox. These results were all expressed as Trolox equivalent antioxidant capacity (TEAC) and figured as μmol trolox per ml.
All the experiments were performed in triplicate. The concentrations of acrylamide were expressed as the mean ± standard deviation (SD) values. This study measured the inhibitory effects of four flavone carbon glycosides and three flavone oxygen glycosides at 10 different addition levels for each on the formation of acrylamide in MRP. Given that each experiment was performed in triplicate, we thus obtained 210 groups of experimental data. Data from each group contained the concentration of acrylamide generated from the low-moisture system and three TEAC values from three different antioxidant evaluation methods.
The LS-SVR modeling approach was used for predicting the effect of flavone carbon and oxygen glycosides on acrylamide generation via triple TEAC variables. The training and predicting data sets had been first assigned. Data were divided into two parts according to the effect on acrylamide generation. In each part, we used the software of Matlab R2010b (The MathWorks, Natick, MA, USA) to sort data. The middle one of every three data was taken out as the prediction of the effect, and the rest were applied for building the model. The LS-SVR model was operated via the Matlab R2010b combining with LS-SVR toolbox (LS-SVR v 1.5, Suykens, Leuven, Belgium).
To compare the regression efficiency of LS-SVR approach with the routine model, data were simultaneously fitted using the multiple linear regression (MLR) and partial least squares regression (PLS) models, based both on linear model and quadratic model. Model performance was evaluated using determination coefficient of calibration (R2C), determination coefficient of validation (R2V), determination coefficient of prediction (R2P), root mean square error of calibration (RMSEC), root mean square error of validation (RMSEV), and root mean square error of prediction (RMSEP) as indicators. Statistical significance of differences was evaluated by two-way ANOVA test and correlations were evaluated by calculating the Pearson’s correlation coefficient using SPSS (version 22.0, IBM Corp., Armonk, NY, USA). Differences were considered significant at p < 0.05 in all statistical tests.
To observe the relationships between the formation of acrylamide and the addition of flavone carbon and oxygen glycosides in the low-moisture system, a dose-response study was conducted in the potato-based system. Both inhibition and promotion effects were observed when the addition levels of flavonoids ranged from 1 to 10,000 μmol/L (Figure 1). In addition, there was an effect of types and addition levels of flavone carbon and oxygen glycosides on the production of acrylamide, which is shown in Supplementary Table 1. In detail, a significant dose-response inhibitory effect could be found by the addition of flavonoids within a range of 1–100 μmol/L. However, the inhibitory tendency of acrylamide formation decreased when the addition level surpassed 100 μmol/L. What’s more, the acrylamide content was dramatically promoted when the addition levels of flavonoids ranged 1,000–10,000 μmol/L. As a result, the optimal addition level of flavone carbon and oxygen glycosides with their ability of reducing the acrylamide content was 100 μmol/L, at which orientin, homoorientin, vitexin and isovitexin among flavone carbon glycosides exerted their maximal inhibitory rates of 47.9%, 55.7%, 44.3%, and 46.9%, respectively, while in the mean time apigenin-7-O-glucoside, luteolin-7-O-glucoside and luteolin-4′-O-glucoside among flavone oxygen glycosides maximally reduced the acrylamide content by 37.6%, 46.2%, and 47.5%, respectively. Furthermore, the maximal promotion effects of orientin, homoorientin, vitexin, isovitexin, apigenin-7-O-glucoside, luteolin-7-O-glucoside and luteolin-4′-O-glucoside could reach 1.62-fold, 1.45-fold, 1.62-fold, 1.61-fold, 1.36-fold, 1.32-fold, and 1.38-fold for the acrylamide concentration when compared to the control group at their addition levels of 10,000 μmol/L. These unexpected adverse effects seemed like violating the definition of flavone carbon and oxygen glycosides as acrylamide inhibitors. Diverse factors are put forward to clarify the so-called “antioxidant paradox” contributing to the dual effect of flavonoids on the formation of acrylamide, including the intrinsic properties of antioxidants, the antioxidants of products and the antioxidant activity of model system. Some promotion mechanisms have been proved to take part in the reaction, including trapping carbonyl compounds (26) and preventing against lipid oxidation (27). However, flavonoids can simultaneously provide carbonyl groups for acrylamide formation (28), inhibit the acrylamide elimination (29) and accelerate the conversion from 3-APA to acrylamide (30). Previous study similarly reported the phenomenon of both promotion and reduction of acrylamide content in wheat bread by the addition of rosemary, dittany and catechin compounds in a dose-dependent way, and clarified the role of rosemary extract and catechin compounds in the acrylamide formation during the Maillard reaction (31). The addition of polyphenols (0.1%, m/m) including catechin, quercetin, gallic acid, ferulic acid and caffeic acid significantly reduced acrylamide by 16.2–92.5% in a model wheat bread system, but in the case of quercetin, a promotion effect was observed with the increase of its concentration (32). Yuan et al. (33) reported that not only the type but also the concentration of phenolic compounds might play a significant role in the formation of acrylamide. Especially, ascorbic acid, a typical water-soluble antioxidant, exerted an inhibitory effect up to 58% within an addition range of 0.2–1.2%, whereas showed a promotion effect with the addition percentage of 1.5% in an asparagine-glucose model system. Furthermore, an equimolar asparagine-glucose model system indicates that different vitamin A and E homologue concentrations could determine their functionality either as antioxidants or pro-oxidants (34).
Figure 1. U-shaped correlations between acrylamide concentrations and treatment levels of (A) flavone carbon glycosides or (B) flavone oxygen glycosides. Data are shown as the mean ± SD (n = 3). Values with different letters indicate significant differences between different additional levels at the same flavonoid (P < 0.05).
For the effect of flavonoids, we initially revealed that a flavonoid-rich antioxidant extract from bamboo leaves effectively reduced the formation of acrylamide in cookies in a non-linear manner (35). Among different types of flavonoids, we also demonstrated that catechin compounds as flavanols and their derivatives showed better but non-linear inhibitory effect on the reduction of acrylamide in a microwave heating model system (36). Qi et al. (37) investigated the effect of proanthocyanidins with different oligomer structures in a fried potato crisp model and observed that oligomer-1, oligomer-2 and oligomer-4 proanthocyanidins (100 μg/ml) could effectively reduce the formation of acrylamide by 41.2%, 46.1%, and 39.6%, respectively, but they did not observe any promotion effect. In view of chemical structures, B-type proanthocyanidins had stronger inhibitory effect than A-type, but unit composition and degree of polymerization had no significant inhibitory effect based on the same mass. In the current study, all the selected flavonoids showed a maximal inhibitory effect when the addition level was 100 μmol/L. In detail, the inhibitory effect of four flavone carbon glycosides ranged 44.3–55.7%, in which homoorientin exerted a maximal effect (n = 3, Figure 1A); the inhibitory effect of three flavone oxygen glycosides spanned 37.6–47.5%, in which the mitigation effect of luteolin-4′-O-glucoside should be preferred (n = 3, Figure 1B). The above results recommended an overall better inhibitory effect of flavone carbon glycosides on the acrylamide generation than the effect of flavone oxygen glycosides, indicating a role of phenolic hydroxyl groups in reducing the acrylamide formation rather than alcoholic hydroxyl groups in the chemical structures of flavone glycosides. As a matter of fact, various activities of flavonoids and polyphenols hinges on their functional groups in the chemical structures such as number and position of phenolic hydroxyl groups, steric effects, and molecular properties (38). Santos et al. (39) investigated the association between chemical structures and antioxidant capacity of hydrolysable tannins and flavonoids by DPPH and ORAC assays and molecular descriptors obtained through density functional theory, and found that the cluster with more active compounds was mostly comprised of monomeric and dimeric ellagitannins. What’s more, the effect of heating processing plays a significant role in the antioxidant activities of flavonoids. Depending on Arrhenius law, the activation energy was 51.4 kJ/mol for luteolin and 120 kJ/mol for luteolin-7-O-glucoside, indicating glycosylated flavonoids may be more resistant than aglycon flavonoids to heat treatment (40). In the current study, although flavone carbon glycosides and oxygen glycosides have the same aglycone structure, the former performed better in the inhibition of acrylamide formation than the latter (e.g., isovitexin > apigenin-7-O-glucoside), which may be ascribed to the difference of phenolic hydroxyl group numbers. In other words, the insertion of oxygen glucoside eliminates one phenolic hydroxyl of the aglycon, while the introduction of carbon glucoside maintains the original number of phenolic hydroxyls compared with the aglycon.
The principle of these representative assays is to measure the antioxidant properties via the single electron transfer-induced elimination of radicals (41), and the mechanism can be used to determine the antioxidant role of flavone carbon and oxygen glycosides used in the current study. The parabolic-shape correlations between TEACDPPH/TEACABTS/TEACFRAP values and the addition levels of flavone carbon and oxygen glycosides is shown in Figures 2, 3. We found the lowest TEAC values when the addition levels of all the flavone carbon and oxygen glycosides were 100 μmol/L, which was used to achieve the maximal inhibition effect on acrylamide formation. In addition, we also identified a significant correlation between acrylamide contents and TEAC values in MRP using Pearson’s correlation test (RTEAC–DPPH = 0.68–0.89, RTEAC–ABTS = 0.48–0.88, and RTEAC–FRAP = 0.58–0.84), which is presented as heat maps in Supplementary material (Supplementary Figure 1). These results were in agreement with some previous studies, reporting similar associations between the acrylamide concentrations and the total antioxidant capacity in model reaction system and real food (21, 36, 42). These results demonstrated that the TEAC values of MRP might be an appropriate predictor for the acrylamide generation and its inhibition/promotion change by the addition of flavone carbon and oxygen glycosides.
Figure 2. U-shaped correlations between treatment levels of flavone carbon glycosides and (A) TEAC-DPPH values, (B) TEAC-ABTS values, or (C) TEAC-ferric ion reducing antioxidant power (FRAP) values in Maillard reaction products. Data are shown as the mean ± SD (n = 3). Values with different letters indicate significant differences between different additional levels at the same flavonoid (P < 0.05).
Figure 3. U-shaped correlations between treatment levels of flavone oxygen glycosides and (A) TEAC-DPPH values, (B) TEAC-ABTS values, or (C) TEAC-FRAP values in Maillard reaction products. Data are shown as the mean ± SD (n = 3). Values with different letters indicate significant differences between different additional levels at the same flavonoid (P < 0.05).
Our study linked the addition of flavone glycosides with the TEAC values of MRP. Based on the possible formation of antioxidant-sugar adducts in model systems, phenolic antioxidants such as flavonoids may interact with sugar fragments and/or reactive carbonyl compounds, generate various adducts through electrophilic aromatic substitution reactions and thus reduce the formation of acrylamide in model Maillard reaction systems (43). However, several studies reported positive, negative or no effects and thus revealed ambiguous role of phenolic and other antioxidants on the formation of acrylamide when investigating their effect on acrylamide (42, 44, 45). Therefore, the variability in experimental parameters such as thermal conditions, food matrix and the addition level of antioxidants may explain the conflicting observations regarding their efficacy as acrylamide inhibitors and/or promotors (43). For instance, the co-formation profile of both toxic acrylamide and beneficial antioxidants changed during different stages of coffee roasting process, indicating the effect of thermal processing and food matrix on the correlation between acrylamide formation and antioxidant property of MRP (46). Although numerous studies reported dose-dependent reduction or mitigation effect of various phenolic antioxidants on the formation of acrylamide in both model system and food (32, 47), we uniquely observed non-linear U-shape tendency of both antioxidant capacity of MRP and acrylamide concentrations with the increase of addition levels of all the selected flavone glycosides. Thermal processing with the addition of flavone glycosides exhibits higher antioxidant capacity due to the generation of MRP such as melanoidins but simultaneously produces related harmful compounds such as acrylamide, indicating we should get insight into the optimal heat processing procedures based on simultaneous consideration of bioactive compounds, sensory characteristics, and safety (48). Meanwhile, compared with the control group, the addition of flavone glycosides at their high levels may promote the formation of acrylamide but not enhance the antioxidant capacity of MRP, suggesting these types of flavone glycosides may reduce the generation of melanoidins, which can be used as a color indicator for controlling the thermal processing and Maillard reaction (49). Further studies are still warranted to unravel how flavone carbon and oxygen glycosides control both acrylamide formation and antioxidant capacity of MRP.
In consideration of the correlation between the inhibition/promotion effect of flavonoids on the acrylamide formation and the antioxidant capacity of MRP, the least squares support vector regression (LS-SVR) models were used to predict the inhibition/promotion effect using triple antioxidant measurement variables. By comparison against LS-SVR and PLS models, consensus LS-SVR model gives more stable and accurate prediction results. Therefore, consensus strategy like LS-SVR modeling may be a promising way for quantitative modeling of complex samples (50). The antioxidant properties of MRP were regarded as three independent variables and the corresponding acrylamide concentration was served as dependent variable. We used the radial basis function as a kernel function in the current study. γ and δ2, two important parameters, were determined before applying LS-SVR model. Among these, γ, called penalty factor, minimizes the structural and empirical risk and also plays a leading part in the improvement of the generalization in this model. δ2, called the width of the kernel function, controls the regression error and reflects the sensitivity given by the input variables. In this study, the parameters were selected by using grid searching technique to ensure the accuracy of the model. According to previous experiments, the ranges of γ and δ2 for acrylamide concentration are set and shown in Table 1 and Supplementary Table 2. The optimization procedures are shown in Figure 4 and Supplementary Figure 2. As shown in Table 1, the Rc2 and Rp2 of the inhibition range of acrylamide were 0.87 and 0.82, respectively, while they were 0.91 and 0.77 when the prediction fell into the promotion range of acrylamide, respectively.
Figure 4. The LS-SVR models were established to predict the reduction effect of acrylamide, and the model performance including the optimization of γ and δ2 was investigated when the addition level of flavonoids was (A) in the range of 1–100 μmol/L (inhibition range) or (B) in the range of 100–10,000 μmol/L (promotion range).
Table 1. Results of LS-SVR models for predicting acrylamide concentrations based on antioxidant properties.
Furthermore, we compared the LS-SVR model with other models such as PLS and MLR for predicting both inhibition and promotion effects of flavone glycosides on the generation of acrylamide. The graphs of three linear models are shown in Figure 5 and the relevant data about inhibition or promotion rates are shown in Table 2. The graphs of three quadratic models are shown in Supplementary Figure 3 and the relevant data are shown in Supplementary Table 3. When R2C and R2P values are larger and the difference between them is smaller, and RMSEC and RMSEP values are smaller and the difference between them is smaller, then the performance of the corresponding model is better. In the prediction of inhibition range, LS-SVR model (0.87 of R2C, 0.82 of R2P, 24.41 of RMSEC and 29.04 of RMSEP) exhibited better predictive ability compared with PLS model (0.84 of R2C, 0.79 of R2P, 26.8 of RMSEC and 30.61 of RMSEP) and MLR model (0.85 of R2C, 0.80 of R2P, 26.23 of RMSEC and 30.38 of RMSEP). The similar phenomenon occurred in respect of the prediction of promotion range and dual effect range. It is clear that the quadratic model predictions seem unqualified. In conclusion, compared with the traditional multiple linear regression model including PLS and MLR, LS-SVR model can better predict inhibition and promotion effects of flavone carbon and oxygen glycosides on the formation of acrylamide.
Figure 5. Graphs of three models (PLS, MLR, and LS-SVR) for estimating the correlation between predicted concentrations and measured values and showing both inhibition and promotion effects on acrylamide formation. Inhibition range of PLS, MLR, and LS-SVR model are presented in panels (A–C), respectively; Promotion range of PLS, MLR, and LS-SVR model are presented in panels (D–F), respectively.
Table 2. Statistical variables of training and testing data of inhibition or promotion rate (%) in the PLS, MLR, LS-SVR models.
In this work, we found that flavone carbon and oxygen glycosides have both inhibition and promotion effects on the formation of acrylamide using an asparagine-glucose model under a low-moisture system. When the addition levels ranged 1–100 μmol/L, the flavone carbon and oxygen glycosides conducted inhibition for the acrylamide formation which could reach the maximum inhibition rate (37.5–55.7%) till the addition level was 100 μmol/L. On the other hand, when the addition concentration ranged 1,000–10,000 μmol/L, the system demonstrated promotion effect and the maximal promotion effect could reach 1.33-fold to 1.62-fold for control while the addition level is 10,000 μmol/L. Taking the phenolic hydroxyl group numbers into consideration, the inhibition/promotion effect may be related to the number of phenolic hydroxyl groups and the differences of thermal resistance. The reduction or promotion effects of flavone carbon and oxygen glycosides could be affected by the addition level and the chemical structure. Our study here also identified a linear relationship between the acrylamide content and the antioxidant capacities of MRP. Using TEAC values as variables, a LS-SVR model could serve as a predictive tool for estimating the generation and reduction of acrylamide in a low-moisture system. Compared with the traditional MLR models, the machine learning approaches like LS-SVR could fit a better correlation coefficient to predict the change of acrylamide content in the food system. However, the mechanistic role of flavone carbon and oxygen glycosides in affecting acrylamide formation still remains unclear. Further studies should continuously focus on the inhibition or promotion effect of flavone carbon and oxygen glycosides on the kinetics of acrylamide. Meanwhile, further optimized prediction by various machine learning approaches may emphasize the interaction between confounding factors and the estimated results, and focus on the fusion of machine learning and experimental design to precisely estimate the reduction/promotion of typical food contaminants by accelerating and improving data processing and real-time decision-making.
The raw data supporting the conclusions of this article will be made available by the authors, without undue reservation.
YZ conceived and designed the study, was the guarantor of the work and, as such, has full access to all the data in the study, takes responsibility for the integrity of the data, and accuracy of the data analysis. LW, FZ, JW, QW, XC, and JC performed the experiment. QW, XC, and JC contributed to data collection. LW and JW drafted the manuscript. QW and XC interpreted the data. LW, FZ, JW, and YZ critically revised the manuscript. All authors read and approved the manuscript.
We thank the financial support from the National Natural Science Foundation of China (Grant No. 21976156) and the China National Program for Support of Top-notch Young Professionals.
The authors declare that the research was conducted in the absence of any commercial or financial relationships that could be construed as a potential conflict of interest.
All claims expressed in this article are solely those of the authors and do not necessarily represent those of their affiliated organizations, or those of the publisher, the editors and the reviewers. Any product that may be evaluated in this article, or claim that may be made by its manufacturer, is not guaranteed or endorsed by the publisher.
The Supplementary Material for this article can be found online at: https://www.frontiersin.org/articles/10.3389/fnut.2022.1042590/full#supplementary-material
ABTS, 2,2-azino-bis-(3-ethylbenzothiazoline-6-sulfonic acid); DPPH, 2,2-diphenyl-1-picrylhydrazyl; HPLC, high-performance liquid chromatography; LS-SVR, least squares support vector regression; MRM, multiple reaction monitoring; MRP, Maillard reaction products; SNFA, Swedish National Food Administration; TEAC, trolox equivalent antioxidant capacity; TPTZ, 2,4,6-tri(2-pyridyl)-s-triazine; trolox, 6-hydroxy-2,5,7,8-tetramethylchromane-2-carboxylic acid; UHPLC-MS/MS, ultra-high performance liquid chromatography tandem mass spectrometry; SPE, solid phase extraction.
1. International Agency for Research Center. IARC Monographs on the Evaluation of Carcinogenic Risks to Humans, Some Industrial Chemicals. Vol. 60. Lyon: IARC Press (1994). p. 560
2. Pedreschi F, Mariotti MS, Granby K. Current issues in dietary acrylamide: formation, mitigation and risk assessment. J Sci Food Agric. (2014) 94:9–20. doi: 10.1002/jsfa.6349
3. Xu Y, Cui B, Ran R, Liu Y, Chen H, Kai G, et al. Risk assessment, formation, and mitigation of dietary acrylamide: current status and future prospects. Food Chem Toxicol. (2014) 69:1–12. doi: 10.1016/j.fct.2014.03.037
4. Mottram DS, Wedzicha BL, Dodson AT. Acrylamide is formed in the Maillard reaction. Nature. (2002) 419:448–9. doi: 10.1038/419448a
5. Stadler RH, Blank I, Varga N, Robert F, Hau J, Guy PA, et al. Acrylamide from Maillard reaction products. Nature. (2002) 419:449–50. doi: 10.1038/419449a
6. Vinci RM, Mestdagh F, De Meulenaer B. Acrylamide formation in fried potato products - Present and future, a critical review on mitigation strategies. Food Chem. (2012) 133:1138–54. doi: 10.1016/j.foodchem.2011.08.001
7. Eichner K, Karel M. Influence of water content and water activity on the sugar-amino browning reaction in model systems under various conditions. J Agric Food Chem. (1972) 20:218–23. doi: 10.1021/jf60180a025
8. Bassama J, Brat P, Bohuon P, Hocine B, Boulanger R, Günata Z. Acrylamide kinetic in plantain during heating process: precursors and effect of water activity. Food Res Int. (2011) 44:1452–8.
9. Elmore JS, Koutsidis G, Dodson AT, Mottram DS, Wedzicha BL. Measurement of acrylamide and its precursors in potato, wheat, and rye model systems. J Agric Food Chem. (2005) 53:1286–93. doi: 10.1021/jf048557b
10. Zhang Y, Ren Y, Zhang Y. New research developments on acrylamide: analytical chemistry, formation mechanism, and mitigation recipes. Chem Rev. (2009) 109:4375–97. doi: 10.1021/cr800318s
11. Kahkeshani N, Saeidnia S, Abdollahi M. Role of antioxidants and phytochemicals on acrylamide mitigation from food and reducing its toxicity. J Food Sci Technol. (2015) 52:3169–86. doi: 10.1007/s13197-014-1558-5
12. Morales G, Jimenez M, Garcia O, Mendoza MR, Beristain CI. Effect of natural extracts on the formation of acrylamide in fried potatoes. LWT Food Sci Technol. (2014) 58:587–93. doi: 10.1016/j.lwt.2014.03.034
13. Demirok E, Kolsarici N. Effect of green tea extract and microwave pre-cooking on the formation of acrylamide in fried chicken drumsticks and chicken wings. Food Res Int. (2014) 63:290–8. doi: 10.1016/j.foodres.2014.04.003
14. Capuano E, Oliviero T, Açar OÇ, Gökmen V, Fogliano V. Lipid oxidation promotes acrylamide formation in fat-rich model systems. Food Res Int. (2010) 43:1021–6. doi: 10.1016/j.foodres.2010.01.013
15. Zhang Y, Zhang Y. Effect of natural antioxidants on kinetic behavior of acrylamide formation and elimination in low-moisture asparagine-glucose model system. J Food Eng. (2008) 85:105–15. doi: 10.1016/j.jfoodeng.2007.07.013
16. Oral RA, Dogan M, Sarioglu K. Effects of certain polyphenols and extracts on furans and acrylamide formation in model system, and total furans during storage. Food Chem. (2014) 142:423–9. doi: 10.1016/j.foodchem.2013.07.077
17. Lu H, Zheng H, Hu Y, Lou H, Kong X. Bruise detection on red bayberry (Myrica rubra Sieb. & Zucc.) using fractal analysis and support vector machine. J Food Eng. (2011) 104:149–53.
18. Zheng H, Lu H. A least-squares support vector machine (LS-SVM) based on fractal analysis and CIELab parameters for the detection of browning degree on mango (Mangifera indica L.). Comput Electron Agric. (2012) 83:47–51.
19. Burges CJ. A tutorial on support vector machines for pattern recognition. Data Min Knowl Discov. (1998) 2:121–67.
20. Zhang Y, Jiao J, Liu C, Wu X, Zhang Y. Isolation and purification of four flavone C-glycosides from antioxidant of bamboo leaves by macroporous resin column chromatography and preparative high-performance liquid chromatography. Food Chem. (2007) 107:1326–36. doi: 10.1016/j.foodchem.2007.09.037
21. Cheng J, Chen XY, Zhao S, Zhang Y. Antioxidant-capacity-based models for the prediction of acrylamide reduction by flavonoids. Food Chem. (2015) 168:90–9. doi: 10.1016/j.foodchem.2014.07.008
22. Zhang Y, Jiao J, Cai Z, Zhang Y, Ren Y. An improved method validation for rapid determination of acrylamide in foods by ultra-performance liquid chromatography combined with tandem mass spectrometry. J Chromatogr A. (2007) 1142:194–8. doi: 10.1016/j.chroma.2006.12.086
23. Zhang Y, Chen X, Cheng J, Jin C, Zhang Y. The reduction effect of dietary flavone C–and O-glycosides on the formation of acrylamide and its correlation and prediction with the antioxidant activity of Maillard reaction products. RSC Adv. (2014) 4:24147–55.
24. Fadda A, Serra M, Molinu MG, Azara E, Barberis A, Sanna D. Reaction time and DPPH concentration influence antioxidant activity and kinetic parameters of bioactive molecules and plant extracts in the reaction with the DPPH radical. J Food Compos Anal. (2014) 35:112–9. doi: 10.1016/j.jfca.2014.06.006
25. Floegel A, Kim D-O, Chung S-J, Koo SI, Chun OK. Comparison of ABTS/DPPH assays to measure antioxidant capacity in popular antioxidant-rich US foods. J Food Compos Anal. (2011) 24:1043–8. doi: 10.1016/j.jfca.2011.01.008
26. Cheng K-W, Zeng X, Tang YS, Wu J-J, Liu Z, Sze K-H, et al. Inhibitory mechanism of naringenin against carcinogenic acrylamide formation and nonenzymatic browning in Maillard model reactions. Chem Res Toxicol. (2009) 22:1483–9. doi: 10.1021/tx9001644
27. Napolitano A, Morales F, Sacchi R, Fogliano V. Relationship between virgin olive oil phenolic compounds and acrylamide formation in fried crisps. J Agric Food Chem. (2008) 56:2034–40. doi: 10.1021/jf0730082
28. Hamzalıoğlu A, Gökmen V. Role of bioactive carbonyl compounds on the conversion of asparagine into acrylamide during heating. Eur Food Res Technol. (2012) 235:1093–9.
29. Hidalgo FJ, Delgado RM, Zamora R. Role of mercaptans on acrylamide elimination. Food Chem. (2010) 122:596–601. doi: 10.1016/j.foodchem.2010.03.016
30. Cai Y, Zhang Z, Jiang S, Yu M, Huang C, Qiu R, et al. Chlorogenic acid increased acrylamide formation through promotion of HMF formation and 3–aminopropionamide deamination. J Hazard Mater. (2014) 268:1–5. doi: 10.1016/j.jhazmat.2013.12.067
31. Hedegaard RV, Granby K, Frandsen H, Thygesen J, Skibsted LH. Acrylamide in bread. Effect of prooxidants and antioxidants. Eur Food Res Technol. (2008) 227:519–25. doi: 10.1007/s00217-007-0750-5
32. Mildner-Szkudlarz S, Różńska M, Piechowska P, Waśkiewicz A, Zawirska-Wojtasiak R. Effects of polyphenols on volatile profile and acrylamide formation in a model wheat bread system. Food Chem. (2019) 297:125008. doi: 10.1016/j.foodchem.2019.125008
33. Yuan Y, Shu C, Zhou B, Qi X, Xiang J. Impact of selected additives on acrylamide formation in asparagine/sugar Maillard model systems. Food Res Int. (2011) 44:449–55. doi: 10.1016/j.foodres.2010.09.025
34. Kuek SL, Tarmizi AHA, Abd Razak RA, Jinap S, Sanny M. Vitamin A and E homologues impacting the fate of acrylamide in equimolar asparagine-glucose model system. Antioxidants. (2021) 10:993. doi: 10.3390/antiox10070993
35. Li D, Chen Y, Zhang Y, Lu B, Jin C, Wu X, et al. Study on mitigation of acrylamide formation in cookies by 5 antioxidants. J Food Sci. (2012) 77:1144–9. doi: 10.1111/j.1750-3841.2012.02949.x
36. Cheng J, Chen XY, Lu HP, Chen Q, Zhang Y. Antioxidant-related and kinetic studies on the reduction effect of flavanols and their derivatives and esterified flavanols and their derivatives on acrylamide formation in a microwave heating model system. RSC Adv. (2014) 4:43378–86.
37. Qi Y, Zhang H, Wu G, Zhang H, Gu L, Wang L, et al. Mitigation effects of proanthocyanidins with different structures on acrylamide formation in chemical and fried potato crisp models. Food Chem. (2018) 250:98–104. doi: 10.1016/j.foodchem.2018.01.012
38. Wen L, Jiang Y, Yang J, Zhao Y, Tian M, Yang B. Structure, bioactivity, and synthesis of methylated flavonoids. Ann N Y Acad Sci. (2017) 1398:120–9. doi: 10.1111/nyas.13350
39. Santos SC, Fortes GAC, Camargo LTFM, Camargo AJ, Ferri PH. Antioxidant effects of polyphenolic compounds and structure-activity relationship predicted by multivariate regression tree. LWT Food Sci Technol. (2021) 137:110366. doi: 10.1016/j.lwt.2020.110366
40. Chaaban H, Ioannou I, Chebil L, Slimane M, Gérardin C, Paris C, et al. Effect of heat processing on thermal stability and antioxidant activity of six flavonoids. J Food Process Preserv. (2017) 41:e13203. doi: 10.1111/jfpp.13203
41. Huang MM, Wang Q, Chen XY, Zhang Y. Unravelling effects of flavanols and their derivatives on acrylamide formation via support vector machine modelling. Food Chem. (2017) 221:178–86. doi: 10.1016/j.foodchem.2016.10.060
42. Ou S, Shi J, Huang C, Zhang G, Teng J, Jiang Y, et al. Effect of antioxidants on elimination and formation of acrylamide in model reaction systems. J Hazard Mater. (2010) 182:863–8. doi: 10.1016/j.jhazmat.2010.06.124
43. Constantinou C, Koutsidis G. Investigations on the effect of antioxidant type and concentration and model system matrix on acrylamide formation in model Maillard reaction systems. Food Chem. (2016) 197:769–75. doi: 10.1016/j.foodchem.2015.11.037
44. Bassama J, Brat P, Bohuon P, Boulanger R, Günata Z. Study of acrylamide mitigation in model system: effect of pure phenolic compounds. Food Chem. (2010) 123:558–62. doi: 10.1016/j.foodchem.2010.04.071
45. Gómez-Narváez F, Mesías M, Delgado-Andrade C, Contreras-Calderón J, Ubillús F, Cruz G, et al. Occurrence of acrylamide and other heat-induced compounds in panela: relationship with physicochemical and antioxidant parameters. Food Chem. (2019) 301. doi: 10.1016/j.foodchem.2019.125256
46. Schouten MA, Tappi S, Angeloni S, Cortese M, Caprioli G, Vittori S, et al. Acrylamide formation and antioxidant activity in coffee during roasting–a systematic study. Food Chem. (2021) 343:128514. doi: 10.1016/j.foodchem.2020.128514
47. Fu Z, Yoo MJY, Zhou W, Zhang L, Chen Y, Lu J. Effect of (-)-epigallocatechin gallate (EGCG) extracted from green tea in reducing the formation of acrylamide during the bread baking process. Food Chem. (2018) 242:162–8. doi: 10.1016/j.foodchem.2017.09.050
48. Zhang Y, Li X, Lu X, Sun H, Wang F. Effect of oilseed roasting on the quality, flavor and safety of oil: a comprehensive review. Food Res Int. (2021) 150:110791. doi: 10.1016/j.foodres.2021.110791
49. Wang Y, Chen X, Zhao C, Miao J, Mao X, Li X, et al. Effects of temperature during processing with wine on chemical composition, antioxidant capacity and enzyme inhibition activities of Angelica Sinensis Radix. Int J Food Sci Technol. (2017) 52:1324–32. doi: 10.1111/ijfs.13403
Keywords: acrylamide formation, flavone carbon and oxygen glycosides, dual effect, flavonoid structure, least squares support vector regression
Citation: Wang L, Zhang F, Wang J, Wang Q, Chen X, Cheng J and Zhang Y (2022) Machine learning prediction of dual and dose-response effects of flavone carbon and oxygen glycosides on acrylamide formation. Front. Nutr. 9:1042590. doi: 10.3389/fnut.2022.1042590
Received: 12 September 2022; Accepted: 14 November 2022;
Published: 30 November 2022.
Edited by:
Apollinaire Tsopmo, Carleton University, CanadaReviewed by:
Otniel Freitas-Silva, Embrapa Food agroindustry, BrazilCopyright © 2022 Wang, Zhang, Wang, Wang, Chen, Cheng and Zhang. This is an open-access article distributed under the terms of the Creative Commons Attribution License (CC BY). The use, distribution or reproduction in other forums is permitted, provided the original author(s) and the copyright owner(s) are credited and that the original publication in this journal is cited, in accordance with accepted academic practice. No use, distribution or reproduction is permitted which does not comply with these terms.
*Correspondence: Yu Zhang, eV96aGFuZ0B6anUuZWR1LmNu
†These authors have contributed equally to this work
Disclaimer: All claims expressed in this article are solely those of the authors and do not necessarily represent those of their affiliated organizations, or those of the publisher, the editors and the reviewers. Any product that may be evaluated in this article or claim that may be made by its manufacturer is not guaranteed or endorsed by the publisher.
Research integrity at Frontiers
Learn more about the work of our research integrity team to safeguard the quality of each article we publish.