- 1Department of Molecular Genetics, Brain Research Institute, Niigata University, Niigata, Japan
- 2Research Institute for Bioscience Products and Fine Chemicals, Ajinomoto Co., Inc., Kawasaki, Japan
- 3Department of Psychiatry, Asahikawa Keisenkai Hospital, Asahikawa, Japan
- 4Department of Neurology, Nitobe Memorial Nakano General Hospital, Tokyo, Japan
- 5Kurumi Clinic, Tokyo, Japan
- 6Department of Neurology, Brain Research Institute, Niigata University, Niigata, Japan
- 7Department of Neurology, Nishiniigata Chuo Hospital, Niigata, Japan
- 8Department of Neurosurgery, Kofu Neurosurgical Hospital, Kofu, Japan
- 9Mishima Hospital, Niigata, Japan
- 10Department of Psychiatry, Sunagawa City Medical Center, Sunagawa, Japan
- 11Division of Neurology, Department of Medicine, Showa University School of Medicine, Tokyo, Japan
- 12Faculty of Medical Sciences, Second Department of Internal Medicine, University of Fukui, Fukui, Japan
- 13Department of Neurology, Midori Hospital, Niigata, Japan
- 14Kawashima Neurology Clinic, Fujisawa, Japan
- 15Southern TOHOKU Medical Clinic, Koriyama, Japan
- 16Department of Neurology, Takatsuki General Hospital, Takatsuki, Japan
- 17Institute for Innovation, Ajinomoto Co., Inc., Kawasaki, Japan
- 18Research Team for Social Participation and Community Health, Tokyo Metropolitan Institute of Gerontology, Tokyo, Japan
- 19Institute of Education, Tokyo Medical and Dental University, Tokyo, Japan
- 20Department of Nursing, Ashikaga University, Ashikaga, Japan
Background: Nutritional epidemiology has shown that inadequate dietary protein intake is associated with poor brain function in the elderly population. The plasma free amino acid (PFAA) profile reflects nutritional status and may have the potential to predict future changes in cognitive function. Here, we report the results of a 2-year interim analysis of a 3-year longitudinal study following mild cognitive impairment (MCI) participants.
Method: In a multicenter prospective cohort design, MCI participants were recruited, and fasting plasma samples were collected. Based on clinical assessment of cognitive function up to 2 years after blood collection, MCI participants were divided into two groups: remained with MCI or reverted to cognitively normal (“MCI-stable,” N = 87) and converted to Alzheimer’s disease (AD) (“AD-convert,” N = 68). The baseline PFAA profile was compared between the two groups. Stratified analysis based on apolipoprotein E ε4 (APOE ε4) allele possession was also conducted.
Results: Plasma concentrations of all nine essential amino acids (EAAs) were lower in the AD-convert group. Among EAAs, three branched-chain amino acids (BCAAs), valine, leucine and isoleucine, and histidine (His) exhibited significant differences even in the logistic regression model adjusted for potential confounding factors such as age, sex, body mass index (BMI), and APOE ε4 possession (p < 0.05). In the stratified analysis, differences in plasma concentrations of these four EAAs were more pronounced in the APOE ε4-negative group.
Conclusion: The PFAA profile, especially decreases in BCAAs and His, is associated with development of AD in MCI participants, and the difference was larger in the APOE ε4-negative population, suggesting that the PFAA profile is an independent risk indicator for AD development. Measuring the PFAA profile may have importance in assessing the risk of AD conversion in the MCI population, possibly reflecting nutritional status.
Clinical trial registration: [https://center6.umin.ac.jp/cgi-open-bin/ctr/ctr_view.cgi?recptno=R000025322], identifier [UMIN000021965].
Introduction
Alzheimer’s disease (AD) is a neurodegenerative disorder that occurs with high frequency in old age (1) and has significant social and economic consequences (2). For example, Japan is a superaging society, and the dementia population is estimated to grow from 4.4 million in 2018 to over 7 million by 2025, or one in five people aged 65 years or older (3). Caring for patients with cognitive decline requires the support of multiple parties, resulting in a significant social and economic burden (2). The accumulation of amyloid-β (Aβ) in the brain is hypothesized to play a causative, initiating role in AD. Although it is expected that some anti-amyloid agents will be approved as drugs, challenges in terms of coverage, price, and convenience are expected to remain. Even if the development of cognitive impairments could be delayed by pharmacotherapy, it is economically challenging to continue the treatment for such a long time after the preclinical phase.
From this perspective, a lifestyle modification approach is one of the realistic options to address dementia. When considered in relation to body mass index (BMI), low BMI is a clear risk factor for AD in elderly individuals. A recent study of 3,632 participants in the Framingham study reported that a lower BMI is associated with a higher risk of dementia in old age (>50 years) (4). In the Japanese population, it has also been reported that lower BMI is associated with a higher incidence of dementia (5). Inadequate dietary intake triggers low BMI and prefrailty/frailty, and the prefrailty/frailty leads to malnutrition, forming a vicious cycle of deteriorating health outcomes (6).
In this regard, changing dietary habits can be a feasible option. A long-term randomized controlled trial called the Finnish Geriatric Intervention Study to Prevent Cognitive Impairment and Disability (FINGER), a multidomain lifestyle-based intervention that includes dietary intervention to improve vascular and lifestyle-related risk factors, was found to maintain cognitive function and reduce the risk of cognitive decline in older adults at high risk of dementia (7). The World Health Organization (WHO) guidelines for cognitive decline and dementia risk reduction recommends a healthy, balanced diet for all adults (8). In particular, recent findings in nutritional epidemiological studies have shown that protein intake is particularly important for maintaining brain function in the elderly population (9–12). Recently, the quality of protein has also been studied, and a low amino acid score of breakfast has been associated with cognitive impairment (13).
Precision biomarkers linking nutritional status and cognitive decline have been intensively investigated (14) and several candidate markers based on cerebrospinal fluid (CSF) or blood samples were reported in both rodent model and clinical studies (15–18). We previously demonstrated the following as the first report of this clinical study (19). We cross-sectionally compared the concentrations of plasma free amino acid (PFAA) and albumin (Alb) in multicenter clinical study with 219 elderly individuals with MCI and 220 cognitively normal (CN) individuals. We found that the concentrations of essential amino acids (EAAs), such as Lys, His, and Thr, and Alb were lower in the MCI group (19). We are currently following up these individuals with MCI for up to 3 years to assess AD development and are developing a prognostic blood-based biomarker focusing on PFAAs. Here, we report an interim analysis mainly based on the 2-year follow-up status of 155 MCI individuals. Additionally, a stratified analysis based on apolipoprotein E ε4 (APOE ε4) allele possession is reported.
Materials and methods
Ethics statement
This study was carried out in accordance with the Declaration of Helsinki and approved by the ethics committee of respective institutions, including Niigata University and Ajinomoto Co., Inc. This study was registered at the University Hospital Medical Information Network Clinical Trials Registry under the number UMIN000021965. Written informed consent was obtained from all participants before participation in this study. All clinical information of the participants was anonymized prior to data analysis.
Participants
This is the interim analysis of a 3-year longitudinal study. Participants with MCI (N = 352) were recruited between September 2016 and December 2021 from the following 14 medical institutions: Niigata University Medical and Dental Hospital, Asahikawa Keisenkai Hospital, Nitobe Memorial Nakano General Hospital, Kurumi Clinic, Nishiniigata Chuo Hospital, Kofu Neurosurgical Hospital, Mishima Hospital, Sunagawa City Medical Center, Showa University Hospital, University of Fukui Hospital, Midori Hospital, Kawashima Neurology Clinic, Southern TOHOKU Medical Clinic, and Takatsuki General Hospital. Participants who had passed more than 2 years after inclusion were included in the current analysis (Figure 1).
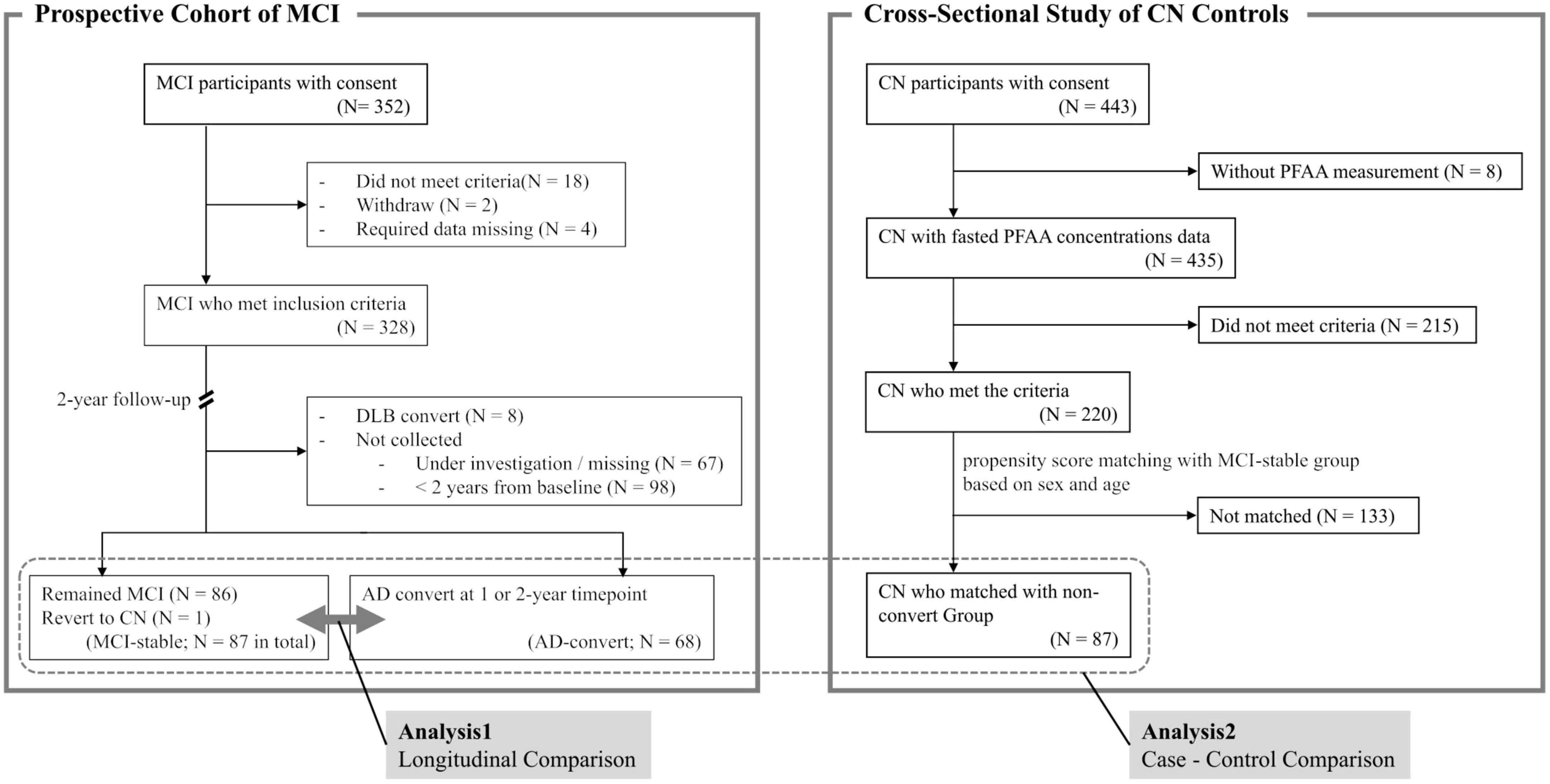
Figure 1. Summary of study design. MCI, mild cognitive impairment; AD, Alzheimer’s disease; DLB, dementia with Lewy bodies; CN, cognitively normal; PFAA, plasma free amino acid.
Control participants (CN, N = 220) who received comprehensive health examinations were recruited from community-dwelling adults at four different cities across Japan (Hatoyama Town, Mitsuke City, Ashikaga City, and Kawasaki City) and from CN participants who visited Sunagawa City Medical Center (19).
Cognitive evaluation and inclusion and exclusion criteria
Since this clinical study is a continuation of the previous report, the study is basically proceeding according to the protocol reported in the previous report (19). Neuropsychological assessments were conducted to evaluate cognitive function of the participants. The Mini-Mental State Examination (MMSE) was used to assess general cognitive function. The Wechsler Memory Scale-Revised Logical Memory II (WMS-R LM II) subtest or Clinical Dementia Rating (CDR) was used to assess memory function and the clinical severity of dementia. The Geriatric Depression Scale-15 (GDS-15) was used for the assessment of the degree of depression.
The following are the inclusion and exclusion criteria for the current study as previously described (19). The common criteria for MCI and CN participants were as follows: (1) aged 50 years or older; (2) not diagnosed as having dementia; (3) living independently; (4) able to undergo neuropsychological tests with an MMSE score of 24 points or higher; and (5) no depression and a GDS-15 score less than six points. In addition, the participant was included in the MCI group if either of the following was applicable: (1) WMS-R LM II score of 11 points or less if the participant had 16 years or more of education, nine points or less if the participant had 8 to 15 years of education, and six points or less if the participant had 7 years or less of education; and (2) a global CDR score of 0.5, which is comparable to the criteria used in the Japanese Alzheimer’s Disease Neuroimaging Initiative (J-ADNI) (20). For the CN participants who were recruited from community-dwelling older adults, the following criteria were additionally applied: (1) an MMSE score of 28–30 points or diagnosed as cognitively normal by a dementia specialist and (2) living independently. The exclusion criteria for MCI and CN were as follows: (1) the consumption of meals or any amino acid formulations, supplements, or beverages within 10 h before blood drawing; (2) less than 6 years of education; (3) current or previous treatment for alcohol addiction; (4) complications of cancer or liver cirrhosis; (5) dialysis treatment; (6) other neurodegenerative or psychiatric disorders; and (7) assessment as ineligible by medical doctors.
Plasma free amino acid analysis
At baseline, 5 ml of venous blood were collected from antecubital veins under fasting conditions in the morning. The blood samples were collected into tubes containing EDTA 2Na as an anticoagulant and were immediately (<1 min) placed in ice water or an ice-cold cooling container (Forte Grow Medical Co., Ltd., Tochigi, Japan). PFAA concentrations were analyzed by high-performance liquid chromatography (HPLC)–electrospray ionization (ESI)–mass spectrometry (MS) by precolumn derivatization. The analytical methods in details have been described elsewhere (21, 22). Concentrations of the following 22 amino acids were determined: alanine (Ala), aminobutyric acid (α-ABA), arginine (Arg), asparagine (Asn), citrulline (Cit), glutamic acid (Glu), glutamine (Gln), glycine (Gly), histidine (His), isoleucine (Ile), leucine (Leu), lysine (Lys), methionine (Met), ornithine (Orn), phenylalanine (Phe), proline (Pro), serine (Ser), taurine (Tau), threonine (Thr), tryptophan (Trp), tyrosine (Tyr), and valine (Val).
Blood biochemistry and apolipoprotein E genotype
The BMI of the participants was calculated based on their height and weight. The following blood parameters were measured in all participants: hemoglobin A1c (HbA1c), Alb, fasting blood glucose, and creatinine. In addition, the following blood variables were measured in all MCI participants: white blood cell (WBC) count, red blood cell (RBC) count, hemoglobin (Hb), hematocrit (Ht), platelet (PLT) count, insulin, total protein (TP), high-density lipoprotein cholesterol (HDLC), low-density lipoprotein cholesterol (LDLC), prealbumin, C-reactive protein (CRP), triglyceride (TG), blood urea nitrogen (BUN), uric acid (UA), calcium (Ca), iron (Fe), aspartate aminotransferase (AST), alanine aminotransferase (ALT), γ-glutamyl transpeptidase (γ-GTP), and folate. In all MCI participants, the following red blood cell indices were calculated based on RBC, Hb, and Ht measurements; mean corpuscular volume (MCV), mean corpuscular hemoglobin (MCH), and mean corpuscular hemoglobin concentration (MCHC).
Genomic DNAs were extracted from peripheral blood of all MCI participants using an automated DNA isolation system (QuickGene-Auto240L, Kurabo, Osaka, Japan). We determined APOE genotypes (rs429358 and rs7412) using TaqMan® PCR Assays (Applied Biosystems, Foster City, CA, USA). In accordance with previous studies (23, 24), participants with one or two alleles of ε4 were defined as the APOE ε4-positive group and those without the ε4 allele as the APOE ε4-negative group.
Follow-up
Follow-up of MCI participants was conducted at the recruiting hospital 1 and 2 years after blood collection and involved a clinical diagnosis of cognitive function, such as AD or MCI, by a medical doctor specializing in dementia. Participants who remained with MCI (N = 86) or revert to CN (N = 1) at the 2-year timepoint were categorized as the “MCI-stable” group collectively, and participants who developed AD at either the 1- or 2-year timepoint (N = 68) were categorized as the “AD-convert” group. Participants who did not return to the hospital at the 2-year timepoint were excluded from the current analysis. Participants who developed dementia types other than AD (such as dementia with Lewy bodies or vascular dementia) were also excluded.
Statistical analysis
Baseline characteristics and plasma free amino acid profiles
To describe the distributions of baseline characteristics and PFAA profiles for both MCI-stable and AD-converted individuals, means and standard deviations or proportions are calculated. Welch’s t-test or Fisher’s exact test was applied to assess differences in baseline characteristics between the MCI-stable and AD-convert groups. Mono- or multivariable logistic regression was used to assess differences in baseline PFAA profiles. Multivariable models were used to adjust for potential cofounding factors such as age, sex, BMI, and APOE ε4 allele possession.
Cognitively normal control dataset preparation
To conduct comparison analysis between CN and MCI-stable or CN and AD-convert, CN individuals were selected using propensity score matching so that the number of matched CN was the same as that of MCI-stable participants (N = 87). Because it is known that the PFAA profile varies with age and sex (25), age and sex were used to calculate propensity scores.
Receiver operating characteristic curve analysis
To determine the capabilities of each PFAA to discriminate AD-converts, receiver operating characteristic (ROC) curve analysis was performed. In the comparison between the MCI-stable and AD-convert groups, the MCI-stable label was fixed as the control class label. On the other hand, in the comparison between CN and MCI-stable or CN and AD-convert, the CN label was fixed as a control class label. Therefore, an area under the ROC curve (AUC of ROC) value < 0.5 indicated that the amino acid concentration was lower in the case group than in the control group, whereas an AUC of ROC value > 0.5 indicated the reverse.
Stratified analysis by apolipoprotein E ε4 status
In the stratified analysis, the dataset of MCI individuals was split according to APOE ε4 positive/negative status. Mono- and multivariable logistic regression models were fit to each dataset to assess differences in baseline PFAA profiles. In multivariable analysis, age, sex, and BMI were used as cofounding factors.
Software
All statistical analyses were conducted using the R (ver. 4.0.3) software (R Foundation for Statistical Computing, Vienna, Austria).
Results
Baseline characteristics of the mild cognitive impairment-stable and Alzheimer’s disease-convert groups
Table 1 summarizes the baseline characteristics of MCI-stable and AD-convert individuals included in this study (MCI-stable; N = 87, AD-convert; N = 68). There were no obvious differences in age or sex between MCI-stable and AD-converted individuals. BMI was slightly lower in the AD-convert group, but the difference was not statistically significant. The MMSE score was significantly lower and the APOE ε4-positive ratio was significantly higher in the AD-convert group. Regarding biochemical tests, LDL-cholesterol was significantly higher and ALT and γ-GTP were significantly lower in the AD-convert group.
Baseline plasma free amino acid profiles of mild cognitive impairment-stable and Alzheimer’s disease-convert groups
Table 2 shows the baseline concentrations of 22 PFAAs of MCI-stable and AD-converted individuals. Concentrations of all nine EAAs were lower in AD-converted individuals. In particular, the concentrations of three branched-chain amino acids (BCAAs), Val, Leu, and Ile, were significantly lower in the AD-convert group even in the model adjusted for potential confounding factors. The concentration of His was also significantly lower in the AD-converted group. Regarding non-essential amino acids (NEAAs), Ser, Gly, and Gln were slightly higher in AD-converted individuals but were not significant in any statistical models. The results were similar when case group was expanded to all cause dementia (N = 76) (Supplementary Tables 1, 2). Additionally, we applied ROC curve analysis for 22 PFAAs (Figure 2A and Supplementary Figure 1).
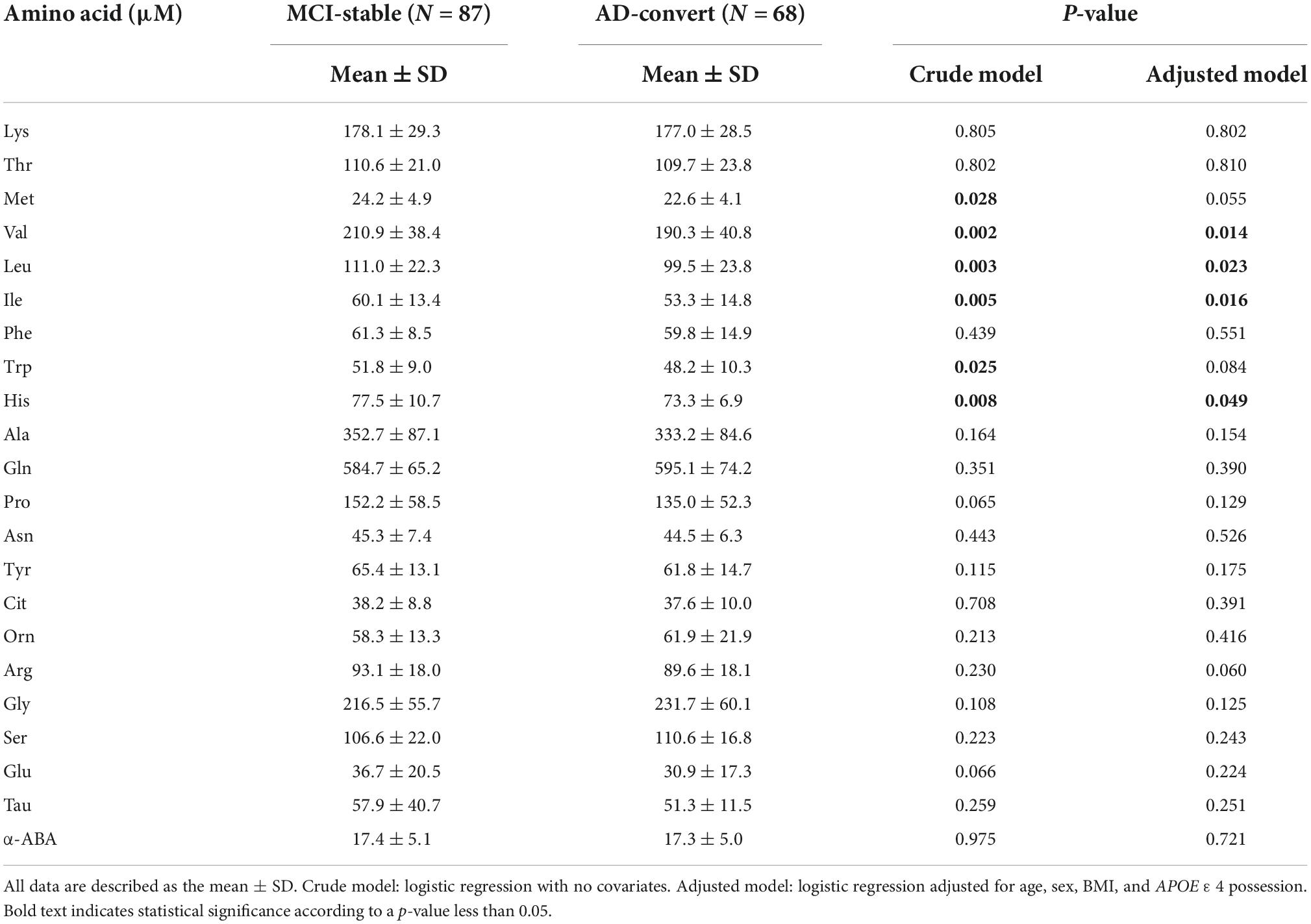
Table 2. Comparison of plasma free amino acid (PFAA) concentrations between mild cognitive impairment (MCI)-stable and Alzheimer’s disease (AD)-convert.
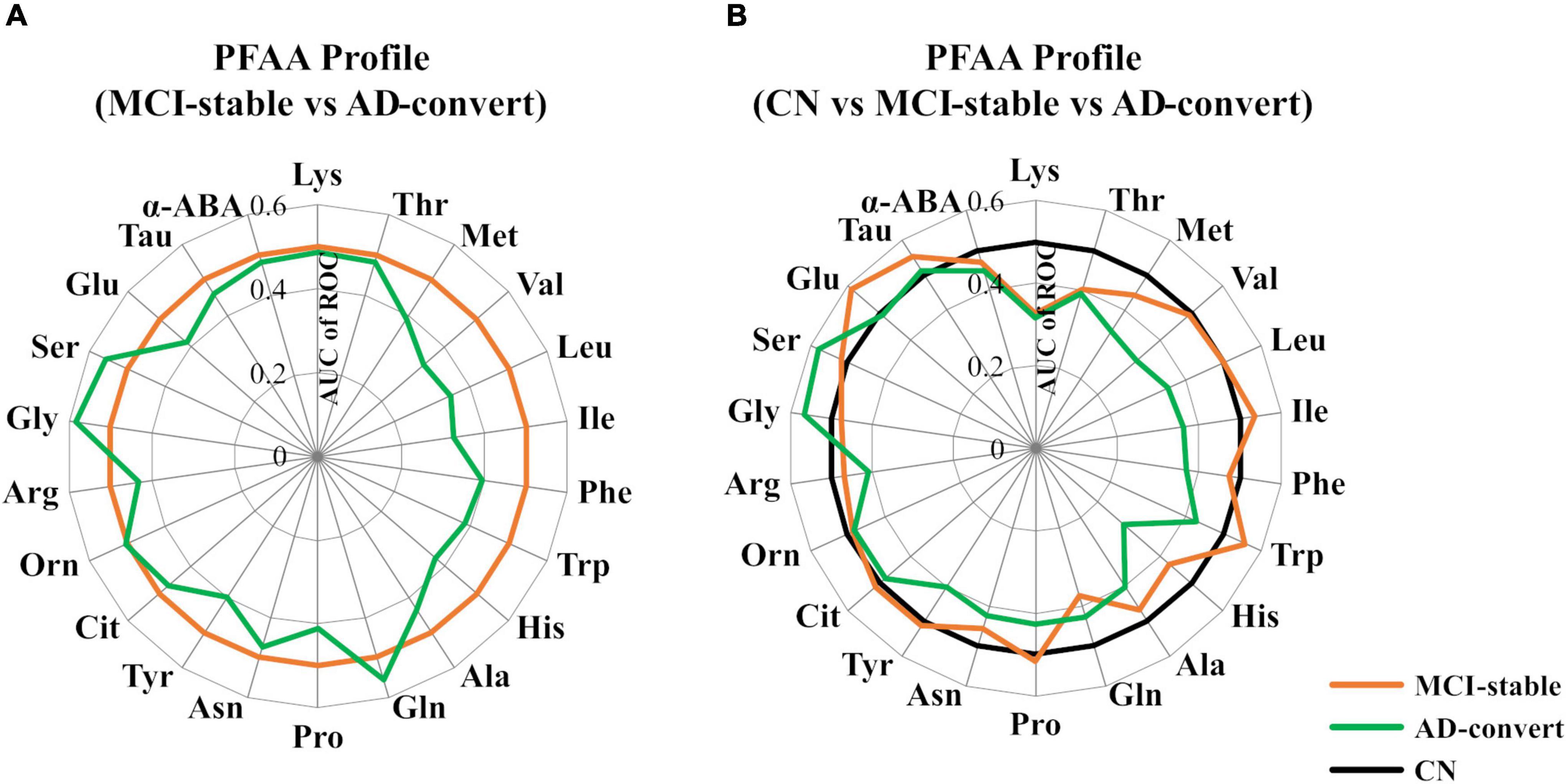
Figure 2. Comparison of plasma free amino acid (PFAA) concentrations among mild cognitive impairment (MCI)-stable, Alzheimer’s disease (AD)-convert, and cognitively normal (CN) individuals. Differences in PFAA concentrations between groups are described by the receiver operating characteristic (ROC) of an area under the curve (AUC). (A) The MCI-stable group was set as the control. (B) The CN group was set as the control. In the CN group, taurine data were partially missing (N = 48 were missing).
Baseline plasma free amino acid profiles of cognitively normal and mild cognitive impairment who developed/did not develop Alzheimer’s disease
We also applied ROC curve analysis setting CN participants as the control group (Figure 2B and Supplementary Table 3). Interestingly, in the comparison between CN and AD-converted individuals, the concentrations of plasma Met, Val, Leu, and His were lower in the AD-convert group, whereas in the comparison between CN and MCI-stable individuals, these amino acids did not show notable differences. In contrast, the plasma concentration of Lys was lower in both the AD-converted and MCI-stable groups than in the CN group. Plasma Gln was significantly lower and Glu was significantly higher only in the MCI-stable group.
Stratified analysis by apolipoprotein E ε4 positive/negative status
To further investigate the relationship of genetic risk factors with the PFAA profile, we conducted a comparison between the MCI-stable and AD-converted groups, stratifying by APOE ε4 positive/negative status. Because the plasma concentrations of the three BCAAs were highly correlated with each other (r > 0.87), the sum of the three BCAA concentrations was used as a representative indicator. In the APOE ε4-negative group, difference in the sum of three BCAA concentrations between the MCI-stable and AD-converted groups was statistically significant, whereas it was not significant in the APOE ε4-positive group (Figure 3). Similarly, differences in three individual BCAAs, His, Gly, and Glu between the MCI-stable and AD-converted groups were greater in the APOE ε4-negative group than in the APOE ε4-positive group (Supplementary Table 4 and Supplementary Figures 2, 3).
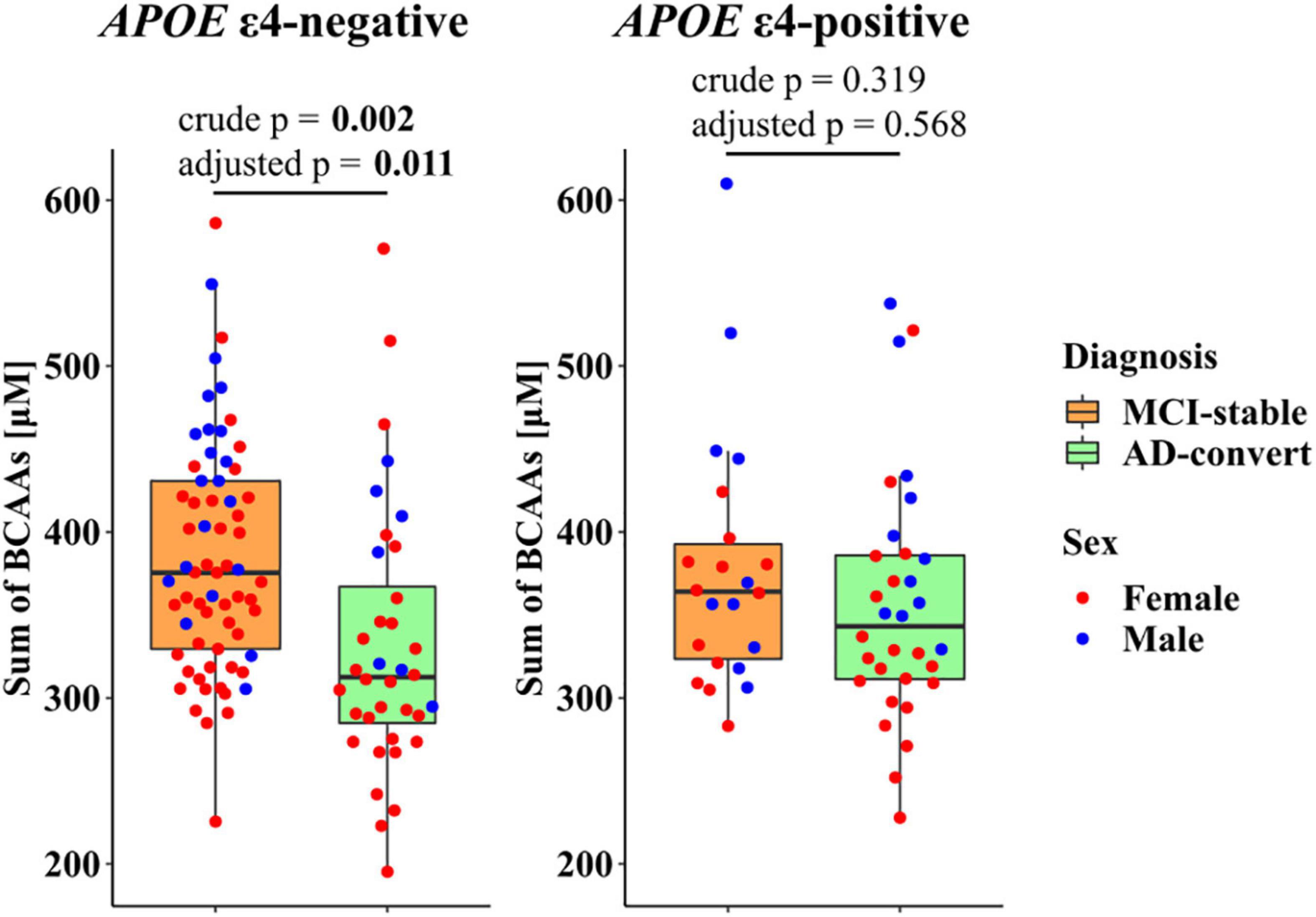
Figure 3. Stratified analysis of plasma branched-chain amino acid (BCAA) concentrations in mild cognitive impairment (MCI) individuals by apolipoprotein E ε4 (APOEε4) positive/negative status. Red and blue dots represent females and males, respectively. Crude p: p-value calculated based on the logistic regression model with no covariates. Adjusted p: p-value calculated based on the logistic regression model adjusted for age, sex, and BMI. Bold text indicates statistical significance according to a p-value less than 0.05.
Discussion
In the current analysis, we investigated the association of AD development within 2 years and the baseline PFAA profile of MCI participants. The AD-converted group showed lower concentrations of EAAs, especially three BCAAs: Val, Leu, and Ile. Additionally, in the stratified analysis, the association of plasma BCAA concentrations and AD development was more pronounced in the APOE ε4-negative group.
Recent findings in nutritional epidemiology have shown that protein intake is important for maintaining brain function in the elderly. For example, the amount of protein intake of dementia patients is significantly lower compared with healthy elderly individuals (9, 10, 26). The protein intake in the elderly is positively associated with memory function (27, 28). Elderly individuals with higher levels of protein intake have a lower risk of MCI (11), and lower levels of Aβ accumulation in the brain (12). These reports provide some insight into the relationship between protein intake and the risk of developing dementia and AD. In a previous study, we reported that MCI individuals showed lower plasma EAA concentrations than CN individuals based on case-control design analysis, demonstrating that among EAAs, Lys, Thr, and Met showed the largest differences (19). In contrast, the current longitudinal study revealed that plasma BCAA concentrations showed the greatest difference between the MCI-stable and AD-converted groups among the MCI population. It is known that some individuals with MCI convert to AD, while others do not, and it is intriguing that these differences are reflected in the differences in plasma BCAA and His concentrations. It is worth noting that in the current study, the differences in serum concentrations of Alb and prealbumin, which are traditionally used indicators of nutritional status, were not statistically significant between the MCI-stable and AD-converted groups (Table 1). Recent position paper by American Society for Parenteral and Enteral Nutrition (ASPEN) pointed out that serum Alb and prealbumin do not serve as valid proxy measures of total body protein or total muscle mass and they must be recognized as inflammatory markers associated with “nutrition risk” (29). Rather, our study indicates that PFAA may be suitable indicator in this context. Interestingly, one study showed that low concentration of serum Val was associated with both brain atrophy and cognitive decline, but not CSF Aβ1–42 level nor CSF Aβ1–42/total tau ratio (30). The concentration of BCAAs in the blood might reflect AD pathology other than Aβ or tau accumulation, such as inflammation or oxidative stress in the brain. Compared to Lys and Thr, which are commonly decreased in MCI compared to CN participants, circulating BCAAs and His may be related to pathological conditions and/or nutritional status, and future detailed studies are warranted.
Recently, multiple reports have emerged on the relationship between changes in the PFAA profile and dementia. An NMR-based metabolomics study based on eight prospective cohorts with 22,623 participants showed that lower serum BCAA concentrations were associated with an increased risk of all types of dementia and AD (31). Recently, a study based on circulating plasma metabolomic data of 110,655 participants in the UK Biobank reported similar results regarding the decrease of plasma BCAAs and the development of dementia (32). It should be noted that in our study, a similar association was found even in the East Asian population. Eating habits and body composition vary across countries, which makes it difficult to compare epidemiological findings between different cultural areas. For example, the average meat consumption per person in Japan is less than half of that in the US (33), and the average BMI and obesity rate of Japanese people are the lowest among OECD member countries (34). Additionally, higher milk consumption was associated with a decrease in executive function or global cognition in a US cohort study (35), whereas higher dairy and milk consumption was associated with a lower risk of all-cause dementia or vascular dementia in two independent Japanese cohort studies (36, 37). Nonetheless, a similar association between the PFAA profile and AD development among diverse food cultures suggests that plasma BCAA concentrations have universal importance in this context.
In this study, differences in APOE ε4 possession showed different strengths of association. The Rotterdam Study, which is a previous study on the relationship between lifestyle and the APOE allele, demonstrated that a healthy lifestyle including a healthy diet reduced the risk of dementia in individuals without the APOE ε4 allele (38). This suggests that a healthy lifestyle, including a balanced diet, is an independent protective factor against dementia, and APOE ε4-negative individuals are more sensitive to lifestyle modification, which is in accordance with our findings that APOE ε4-negative MCI individuals had a stronger association between the PFAA profile and AD development. However, the importance of EAAs in APOE ε4-positive individuals needs future investigation because in our stratified analysis, plasma EAA concentrations of the AD-converted group were lower even in the APOE ε4-positive population, but the effect size might be smaller than that in the APOE ε4-negative population.
Precision biomarkers are key to the preventions tailored to an individual’s biological and nutritional status (14). When considering nutritional interventions based on the current results, the molecular mechanism that links circulating EAAs and development of dementia is important. As a lower plasma BCAA concentration is associated with the progression of sarcopenia (39, 40) one reason may be the loss of muscle strength and the associated decrease in the amount of activity. A decline in handgrip strength has been demonstrated to be an important indicator of dementia (41). Additionally, daily exercise has been reported to help prevent dementia (42). A higher non-grain-derived BCAA intake was associated with handgrip strength among Korean older adults (43), and nutritional intake that supports daily exercise might be desirable. Another perspective is that low-protein diets have been reported to decrease essential amino acid influx to the brain (44) and cause inflammation in the brain, which may be one of the contributing factors of dementia (45, 46). In a previous study using tauopathy model mice, a decrease in circulating EAAs triggered inflammation in the brain. EAA ingestion reversed this effect by competitively inhibiting kynurenine, an initiator of a pathway inducing neuroinflammatory gliosis and neurotoxicity in the brain (45). A recent randomized clinical trial also suggested dietary EAA supplementation improved cognitive function in middle-aged and older adults (47). Another group reported that supplementation with a BCAA mixture in senior malnourished patients improved both MMSE scores and mitochondrial function in peripheral blood mononuclear cells (48). In summary, maintaining an adequate concentration of circulating amino acids by a balanced diet may be protective against cognitive decline potentially by (1) maintaining muscle mass, (2) preventing brain inflammation, and (3) improving mitochondrial function. Future intervention studies on these populations are needed.
This study has several limitations. First, our study did not include molecular imaging or CSF biomarkers, so we were unable to stratify participants with MCI or CN based on the underlying molecular pathology. Second, the study design is based on observational research, and there could be reverse causality. Progressing MCI individuals might have poorer appetite and/or more abnormal eating habits compared to stable MCI individuals, which might have reflected to the imbalance of PFAA profile. Third, factors that may affect PFAAs and cognitive function, such as diet, muscle mass, and physical activity were not included in the current analysis. In future studies, it will be particularly important to examine the correlation with protein nutritional status. Follow-up of the participants through 3 years is currently ongoing, and the relationship with the conversion to AD will be analyzed with other indicators that we were unable to analyze this time in future analyses.
This interim analysis of a multicenter longitudinal study revealed a significant relationship with development of AD and the PFAA profile, especially a decrease in circulating BCAAs and His among MCI participants. In the stratified analysis by APOE ε4 allele positive/negative status, the difference in the PFAA profile with or without AD development was more pronounced in the APOE ε4-negative group, suggesting that the PFAA profile is an independent risk indicator of genetic factors. Further studies are necessary to clarify the relationship between the PFAA profile and lifestyle factors, including nutritional intake.
Data availability statement
The original contributions presented in the study are included in the article/Supplementary material, further inquiries can be directed to the corresponding authors.
Ethics statement
The studies involving human participants were reviewed and approved by the Ethics Committee on Genetic Analysis of Niigata University and Ethics Committee of Ajinomoto Co., Inc. The patients/participants provided their written informed consent to participate in this study.
Author contributions
TI and KN: conceptualization and funding acquisition. MK: data curation, formal analysis, software, and visualization. FM, STor, CN, KK, TTo, TTa, YKu, NM, STan, KU, KO, SY, TH, SN, RY, NK, CK, HT, AK, YYo, EK, MY, HK, YYa, HJ, and MK: investigation. HK, YYa, MK, YKa, and KN: methodology. TI, HK, and STou: project administration. MK, HK, YKa, and KN: resources. KN: supervision. MK and KN: validation. TI, HK, YYa, MK, and KN: writing—original draft. TI, MK, and KN: writing—review and editing. All authors have read and agreed to the published version of the manuscript.
Funding
This work was funded by Ajinomoto Co., Inc.
Acknowledgments
The authors would like to thank Kho Tanaka, Masahiro Morishita (Mishima Hospital), Atsushi Kimura (Showa University School of Medicine), Haruo Hanyu (Southern TOHOKU Medical Clinic), and Yasushi Furukawa (Ajinomoto Co., Inc.) for organizing the multicenter clinical research. The kind support of Tomoyuki Tagami (Ajinomoto Co., Inc.) in supporting recruitment and follow-up of study participants was deeply appreciated. Wataru Sato (Ajinomoto Co., Inc.) was acknowledged for providing valuable insights into the statistical analysis.
Conflict of interest
TI, FM, STor, CN, TTa, NM, STan, KU, KO, TH, SN, NK, CK, HT, AK, EK, and MY’s respective institutions or research organizations were paid for joint research or case incorporation by Ajinomoto Co., Inc. MK, HK, YYa, STou, YKa, HJ, and KN are full-time employees of Ajinomoto Co., Inc. Ajinomoto Co., Inc. funded the study and participated in the study design, implementation, analysis, and interpretation of the data. This does not alter the authors’ adherence to all of the journal policies.
The remaining authors declare that the research was conducted in the absence of any commercial or financial relationships that could be construed as a potential conflict of interest.
Publisher’s note
All claims expressed in this article are solely those of the authors and do not necessarily represent those of their affiliated organizations, or those of the publisher, the editors and the reviewers. Any product that may be evaluated in this article, or claim that may be made by its manufacturer, is not guaranteed or endorsed by the publisher.
Supplementary material
The Supplementary Material for this article can be found online at: https://www.frontiersin.org/articles/10.3389/fnut.2022.1040476/full#supplementary-material
References
1. Sperling R, Aisen P, Beckett L, Bennett D, Craft S, Fagan A, et al. Toward defining the preclinical stages of Alzheimer’s disease: recommendations from the National Institute on Aging-Alzheimer’s Association workgroups on diagnostic guidelines for Alzheimer’s disease. Alzheimers Dement. (2011) 7:280–92. doi: 10.1016/j.jalz.2011.03.003
2. El-Hayek Y, Wiley R, Khoury C, Daya R, Ballard C, Evans A, et al. Tip of the iceberg: Assessing the global socioeconomic costs of Alzheimer’s Disease and related dementias and strategic implications for stakeholders. J Alzheimers Dis. (2019) 70:323–41. doi: 10.3233/JAD-190426
3. Leroi I, Watanabe K, Hird N, Sugihara T. “Psychogeritechnology” in Japan: Exemplars from a super-aged society. Int J Geriatr Psychiatry. (2018) 33:1533–40. doi: 10.1002/gps.4906
4. Li J, Joshi P, Ang T, Liu C, Auerbach S, Devine S, et al. Mid- to late-life body mass index and dementia risk: 38 Years of follow-up of the framingham study. Am J Epidemiol. (2021) 190:2503–10. doi: 10.1093/aje/kwab096
5. Yokomichi H, Kondo K, Nagamine Y, Yamagata Z, Kondo N. Dementia risk by combinations of metabolic diseases and body mass index: Japan Gerontological Evaluation Study Cohort Study. J Diabetes Investig. (2020) 11:206–15. doi: 10.1111/jdi.13103
6. Wei K, Nyunt M, Gao Q, Wee S, Yap K, Ng T. Association of frailty and malnutrition with long-term functional and mortality outcomes among community-dwelling older adults: Results from the singapore longitudinal aging study 1. JAMA Netw Open. (2018) 1:e180650. doi: 10.1001/jamanetworkopen.2018.0650
7. Ngandu T, Lehtisalo J, Solomon A, Levalahti E, Ahtiluoto S, Antikainen R, et al. A 2 year multidomain intervention of diet, exercise, cognitive training, and vascular risk monitoring versus control to prevent cognitive decline in at-risk elderly people (FINGER): a randomised controlled trial. Lancet. (2015) 385:2255–63. doi: 10.1016/S0140-6736(15)60461-5
8. World Health Organization. Risk reduction of cognitive decline and dementia WHO guidelines. Geneva: World Health Organization (2019).
9. Nes M, Sem S, Rousseau B, Bjorneboe G, Engedal K, Trygg K, et al. Dietary intakes and nutritional status of old people with dementia living at home in Oslo. Eur J Clin Nutr. (1988) 42:581–93.
10. Sanders C, Wengreen H, Schwartz S, Behrens S, Corcoran C, Lyketsos C, et al. Nutritional status is associated with severe dementia and mortality: The cache county dementia progression study. Alzheimer Dis Assoc Disord. (2018) 32:298–304. doi: 10.1097/WAD.0000000000000274
11. Roberts R, Roberts L, Geda Y, Cha R, Pankratz V, O’Connor H, et al. Relative intake of macronutrients impacts risk of mild cognitive impairment or dementia. J Alzheimers Dis. (2012) 32:329–39. doi: 10.3233/JAD-2012-120862
12. Fernando W, Rainey-Smith S, Gardener S, Villemagne V, Burnham S, Macaulay S, et al. Associations of dietary protein and fiber intake with brain and blood amyloid-beta. J Alzheimers Dis. (2018) 61:1589–98. doi: 10.3233/JAD-170742
13. Kinoshita K, Otsuka R, Takada M, Tsukamoto-Yasui M, Nishita Y, Tange C, et al. Low amino acid score of breakfast is associated with the incidence of cognitive impairment in older japanese adults: A community-based longitudinal study. J Prev Alzheimers Dis. (2022) 9:151–7. doi: 10.14283/jpad.2021.25
14. Yassine H, Samieri C, Livingston G, Glass K, Wagner M, Tangney C, et al. Nutrition state of science and dementia prevention: recommendations of the Nutrition for Dementia Prevention Working Group. Lancet Healthy Longev. (2022) 3:e501–12. doi: 10.1016/s2666-7568(22)00120-9
15. Basun H, Forssell L, Almkvist O, Cowburn R, Eklof R, Winblad B, et al. Amino acid concentrations in cerebrospinal fluid and plasma in Alzheimer’s disease and healthy control subjects. J Neural Transm Park Dis Dement Sect. (1990) 2:295–304. doi: 10.1007/BF02252924
16. Gonzalez-Dominguez R, Garcia-Barrera T, Gomez-Ariza J. Metabolite profiling for the identification of altered metabolic pathways in Alzheimer’s disease. J Pharm Biomed Anal. (2015) 107:75–81. doi: 10.1016/j.jpba.2014.10.010
17. Pan X, Nasaruddin M, Elliott C, McGuinness B, Passmore A, Kehoe P, et al. Alzheimer’s disease-like pathology has transient effects on the brain and blood metabolome. Neurobiol Aging. (2016) 38:151–63. doi: 10.1016/j.neurobiolaging.2015.11.014
18. Polis B, Samson A. Role of the metabolism of branched-chain amino acids in the development of Alzheimer’s disease and other metabolic disorders. Neural Regen Res. (2020) 15:1460–70. doi: 10.4103/1673-5374.274328
19. Ikeuchi T, Yano Y, Sato W, Morikawa F, Toru S, Nishimura C, et al. Development of a novel nutrition-related multivariate biomarker for mild cognitive impairment based on the plasma free amino acid profile. Nutrients. (2022) 14:637. doi: 10.3390/nu14030637
20. Iwatsubo T, Iwata A, Suzuki K, Ihara R, Arai H, Ishii K, et al. Japanese and north american Alzheimer’s Disease neuroimaging initiative studies: Harmonization for international trials. Alzheimers Dement. (2018) 14:1077–87. doi: 10.1016/j.jalz.2018.03.009
21. Nakayama A, Imaizumi A, Yoshida H. Methods for absolute quantification of human plasma free amino acids by high-performance liquid chromatography/electrospray ionization mass spectrometry using precolumn derivatization. Methods Mol Biol. (2019) 2030:207–18. doi: 10.1007/978-1-4939-9639-1_16
22. Yoshida H, Kondo K, Yamamoto H, Kageyama N, Ozawa S, Shimbo K, et al. Validation of an analytical method for human plasma free amino acids by high-performance liquid chromatography ionization mass spectrometry using automated precolumn derivatization. J Chromatogr B Analyt Technol Biomed Life Sci. (2015) 998-999:88–96. doi: 10.1016/j.jchromb.2015.05.029
23. Lopez O, Jagust W, Dulberg C, Becker J, DeKosky S, Fitzpatrick A, et al. Risk factors for mild cognitive impairment in the Cardiovascular Health Study Cognition Study: part 2. Arch Neurol. (2003) 60:1394–9. doi: 10.1001/archneur.60.10.1394
24. Small G, Ercoli L, Silverman D, Huang S, Komo S, Bookheimer S, et al. Cerebral metabolic and cognitive decline in persons at genetic risk for Alzheimer’s disease. Proc Natl Acad Sci USA. (2000) 97:6037–42. doi: 10.1073/pnas.090106797
25. Yamamoto H, Kondo K, Tanaka T, Muramatsu T, Yoshida H, Imaizumi A, et al. Reference intervals for plasma-free amino acid in a Japanese population. Ann Clin Biochem. (2016) 53:357–64. doi: 10.1177/0004563215583360
26. Thomas D, Chung A, Dickerson J, Tidmarsh S, Shaw D. Tryptophan and nutritional status of patients with senile dementia. Psychol Med. (1986) 16:297–305. doi: 10.1017/s0033291700009119
27. Goodwin J, Goodwin J, Garry P. Association between nutritional status and cognitive functioning in a healthy elderly population. JAMA. (1983) 249:2917–21.
28. La Rue A, Koehler K, Wayne S, Chiulli S, Haaland K, Garry P. Nutritional status and cognitive functioning in a normally aging sample: a 6-y reassessment. Am J Clin Nutr. (1997) 65:20–9. doi: 10.1093/ajcn/65.1.20
29. Evans D, Corkins M, Malone A, Miller S, Mogensen K, Guenter P, et al. The use of visceral proteins as nutrition markers: An ASPEN position paper. Nutr Clin Pract. (2021) 36:22–8. doi: 10.1002/ncp.10588
30. Toledo J, Arnold M, Kastenmuller G, Chang R, Baillie R, Han X, et al. Metabolic network failures in Alzheimer’s disease: A biochemical road map. Alzheimers Dement. (2017) 13:965–84. doi: 10.1016/j.jalz.2017.01.020
31. Tynkkynen J, Chouraki V, van der Lee S, Hernesniemi J, Yang Q, Li S, et al. Association of branched-chain amino acids and other circulating metabolites with risk of incident dementia and Alzheimer’s disease: A prospective study in eight cohorts. Alzheimers Dement. (2018) 14:723–33. doi: 10.1016/j.jalz.2018.01.003
32. Zhang X, Hu W, Wang Y, Wang W, Liao H, Zhang X, et al. Plasma metabolomic profiles of dementia: a prospective study of 110,655 participants in the UK Biobank. BMC Med. (2022) 20:252. doi: 10.1186/s12916-022-02449-3
33. Food and Agriculture Organization of the United Nations. OECD-FAO Agricultural Outlook 2021-2030. Rome: Food and Agriculture Organization (2021).
34. Organisation for Economic Co-operation and Development. Health at a Glance 2021. Paris: Organisation for Economic Co-operation and Development (2021).
35. Petruski-Ivleva N, Kucharska-Newton A, Palta P, Couper D, Meyer K, Graff M, et al. Milk intake at midlife and cognitive decline over 20 Years. The atherosclerosis risk in communities (ARIC) Study. Nutrients. (2017) 9:1134. doi: 10.3390/nu9101134
36. Ozawa M, Ohara T, Ninomiya T, Hata J, Yoshida D, Mukai N, et al. Milk and dairy consumption and risk of dementia in an elderly Japanese population: the Hisayama Study. J Am Geriatr Soc. (2014) 62:1224–30. doi: 10.1111/jgs.12887
37. Yamada M, Kasagi F, Sasaki H, Masunari N, Mimori Y, Suzuki G. Association between dementia and midlife risk factors: The radiation effects research foundation adult health study. J Am Geriatr Soc. (2003) 51:410–4. doi: 10.1046/j.1532-5415.2003.51117.x
38. Licher S, Ahmad S, Karamujic-Comic H, Voortman T, Leening M, Ikram M, et al. Genetic predisposition, modifiable-risk-factor profile and long-term dementia risk in the general population. Nat Med. (2019) 25:1364–9. doi: 10.1038/s41591-019-0547-7
39. Yamada M, Kimura Y, Ishiyama D, Nishio N, Tanaka T, Ohji S, et al. Plasma amino acid concentrations are associated with muscle function in older japanese women. J Nutr Health Aging. (2018) 22:819–23. doi: 10.1007/s12603-018-1014-8
40. Nagao K, Kimura T. Use of plasma-free amino acids as biomarkers for detecting and predicting disease risk. Nutr Rev. (2020) 78:79–85. doi: 10.1093/nutrit/nuaa086
41. Hatabe Y, Shibata M, Ohara T, Oishi E, Yoshida D, Honda T, et al. Decline in handgrip strength from midlife to late-life is associated with dementia in a japanese community: The Hisayama Study. J Epidemiol. (2020) 30:15–23. doi: 10.2188/jea.JE20180137
42. Sofi F, Valecchi D, Bacci D, Abbate R, Gensini G, Casini A, et al. Physical activity and risk of cognitive decline: a meta-analysis of prospective studies. J Intern Med. (2011) 269:107–17. doi: 10.1111/j.1365-2796.2010.02281.x
43. Park S, Chae M, Park H, Park K. Higher branched-chain amino acid intake is associated with handgrip strength among korean older adults. Nutrients. (2021) 13:1522. doi: 10.3390/nu13051522
44. Sato H, Tsukamoto-Yasui M, Takado Y, Kawasaki N, Matsunaga K, Ueno S, et al. Protein deficiency-induced behavioral abnormalities and neurotransmitter loss in aged mice are ameliorated by essential amino acids. Front Nutr. (2020) 7:23. doi: 10.3389/fnut.2020.00023
45. Sato H, Takado Y, Toyoda S, Tsukamoto-Yasui M, Minatohara K, Takuwa H, et al. Neurodegenerative processes accelerated by protein malnutrition and decelerated by essential amino acids in a tauopathy mouse model. Sci Adv. (2021) 7:eabd5046. doi: 10.1126/sciadv.abd5046
46. Zadori D, Veres G, Szalardy L, Klivenyi P, Vecsei L. Alzheimer’s Disease: Recent concepts on the relation of mitochondrial disturbances, excitotoxicity, neuroinflammation, and kynurenines. J Alzheimers Dis. (2018) 62:523–47. doi: 10.3233/JAD-170929
47. Suzuki H, Yamashiro D, Ogawa S, Kobayashi M, Cho D, Iizuka A, et al. Intake of seven essential amino acids improves cognitive function and psychological and social function in middle-aged and older adults: A double-blind, randomized, placebo-controlled trial. Front Nutr. (2020) 7:586166. doi: 10.3389/fnut.2020.586166
48. Buondonno I, Sassi F, Carignano G, Dutto F, Ferreri C, Pili F, et al. From mitochondria to healthy aging: The role of branched-chain amino acids treatment: MATeR a randomized study. Clin Nutr. (2020) 39:2080–91. doi: 10.1016/j.clnu.2019.10.013
Glossary
AD, Alzheimer’s disease; Ala, alanine; Alb, albumin; ALT, alanine transaminase; APOE, apolipoprotein E; APOE ε4, apolipoprotein E ε4; Arg, arginine; Asn, asparagine; ASPEN, American Society for Parenteral and Enteral Nutrition; AST, aspartate aminotransferase; AUC, an area under the curve; Aβ, amyloid-β; BCAA, branched-chain amino acid; BMI, body mass index; BUN, blood urea nitrogen; Ca, calcium; CDR, Clinical Dementia Rating; Cit, citrulline; CN, cognitively normal; CRP, C-reactive protein; CSF, cerebrospinal fluid; DBP, diastolic blood pressure; DLB, dementia with Lewy bodies; EAA, essential amino acid; ESI, electrospray ionization; Fe, iron; GDS-15, Geriatric Depression Scale-15; Gln, glutamine; Glu, glutamic acid; Gly, glycine; Hb, hemoglobin; HbA1c, hemoglobin A1c; HDLC, high-density lipoprotein cholesterol; His, histidine; HPLC, high-performance liquid chromatography; Ht, hematocrit; Ile, isoleucine; LDLC, low-density lipoprotein cholesterol; Leu, leucine; Lys, lysine; MCH, mean corpuscular hemoglobin; MCHC, mean corpuscular hemoglobin concentration; MCI, mild cognitive impairment; MCV, mean corpuscular volume; Met, methionine; MMSE, Mini-Mental State Examination; MS, mass spectrometry; NEAA, non-essential amino acid; Orn, ornithine; PFAA, plasma free amino acid; Phe, phenylalanine; PLT, platelet; Pro, proline; RBC, red blood cell; ROC, receiver operating characteristic; SBP, systolic blood pressure; Ser, serine; Tau, taurine; TG, triglyceride; Thr, threonine; TP, total protein; Trp, tryptophan; Tyr, tyrosine; UA, uric acid; Val, valine; WBC, white blood cell; WMS-R, Wechsler Memory Scale-Revised; WMS-R LM II, Wechsler Memory Scale-Revised Logical Memory II; α-ABA, aminobutyric acid; γ-GTP, γ-glutamyl transpeptidase.
Keywords: multicenter clinical study, protein malnutrition, biomarker discovery, MMSE–Mini-Mental State Examination, APOE
Citation: Ikeuchi T, Kanda M, Kitamura H, Morikawa F, Toru S, Nishimura C, Kasuga K, Tokutake T, Takahashi T, Kuroha Y, Miyazawa N, Tanaka S, Utsumi K, Ono K, Yano S, Hamano T, Naruse S, Yajima R, Kawashima N, Kaneko C, Tachibana H, Yano Y, Kato Y, Toue S, Jinzu H, Kitamura A, Yokoyama Y, Kaneko E, Yamakado M and Nagao K (2022) Decreased circulating branched-chain amino acids are associated with development of Alzheimer’s disease in elderly individuals with mild cognitive impairment. Front. Nutr. 9:1040476. doi: 10.3389/fnut.2022.1040476
Received: 09 September 2022; Accepted: 24 November 2022;
Published: 14 December 2022.
Edited by:
Simon McArthur, Queen Mary University of London, United KingdomReviewed by:
Baruh Polis, Yale University, United StatesRick I. Meijer, Radboud University Medical Center, Netherlands
Copyright © 2022 Ikeuchi, Kanda, Kitamura, Morikawa, Toru, Nishimura, Kasuga, Tokutake, Takahashi, Kuroha, Miyazawa, Tanaka, Utsumi, Ono, Yano, Hamano, Naruse, Yajima, Kawashima, Kaneko, Tachibana, Yano, Kato, Toue, Jinzu, Kitamura, Yokoyama, Kaneko, Yamakado and Nagao. This is an open-access article distributed under the terms of the Creative Commons Attribution License (CC BY). The use, distribution or reproduction in other forums is permitted, provided the original author(s) and the copyright owner(s) are credited and that the original publication in this journal is cited, in accordance with accepted academic practice. No use, distribution or reproduction is permitted which does not comply with these terms.
*Correspondence: Kenji Nagao, kenji.nagao.df2@asv.ajinomoto.com; Takeshi Ikeuchi, ikeuchi@bri.niigata-u.ac.jp
†These authors share senior authorship