- 1Centro de Investigación y Desarrollo en Alimentos de la Universidad Veracruzana, Xalapa, Mexico
- 2CONACyT-Centro de Investigación y Desarrollo en Alimentos, Universidad Veracruzana, Xalapa, Mexico
- 3CIIDIR-Oaxaca, Instituto Politécnico Nacional, Santa Cruz Xoxocotlán, Mexico
The fruits and seeds of Cucurbita ficifolia Bouché are sources of minerals, vitamins, and functional compounds with nutraceutical and preventive potential against cardiovascular diseases and diseases derived from eating disorders. C. ficifolia is native from Mesoamerica and is currently cultivated in temperate zones from Mexico to South America and Asia. This study evaluated the fruit mesocarps of C. ficifolia for physicochemical parameters, antioxidant activity, and phenolic compound contents in a collection of farmers’ landraces. Germplasm is cultivated by traditional farmers in the temperate zones of two municipalities from Oaxaca, Mexico. The results show that the content of soluble solid contents (SSC), pH, total sugars (TS), and flavonoids are influenced by the fruit geographical origin (municipalities) and implicitly by their agroecological cultivation conditions (Huamelúlpam: SSC = 6.22 °Brix, pH = 5.44, TS = 0.52 mg G g–1, flavonoids = 1.24 mg CE g–1; Yanhuitlán: SSC = 6.69, pH = 5.33, TS = 0.55, flavonoids = 1.30). Among populations preserved by traditional farmers, significant differences, and wide variability were found for all parameters evaluated (Huamelúlpam: SSC = 4.9–7.3, pH = 5.5–5.8, TS = 0.4–0.7, protein = 5.8–11.4, polyphenols = 1.9–4.8, flavonoids = 1.0–1.5, DPPH = 4.3–10.6, and FRAP = 4.8–11.8; Yanhuitlán: SSC = 4.3–8.9, pH = 4.8–5.6, TS = 0.4–0.7, protein = 5.0–15.3, polyphenols = 1.9–4.9, flavonoids = 0.8–1.9, DPPH = 5.3–10.5, and FRAP = 4.5–12.6). Eight compounds were identified by UPLC-MS: L-phenylalanine, an amino acid that is regularly associated with proteins; vanillin, a phenolic aldehyde with its functional groups including aldehyde, hydroxyl, and ether; and six phenolic acids: 4-hydroxybenzoic acid, 4-hydroxyphenylacetic acid, vanillic acid, 4-coumaric acid, ferulic acid, and salicylic acid, all with potential health effects. The C. ficifolia fruit mesocarp has bioactive compounds with high antioxidant activity with the potential to both improve diet and to obtain other benefits against nontransmissible diseases derived from food and its associated risk factors.
1 Introduction
Less known horticultural plants have recently gained more popularity. They include high contents of nonnutritive, nutritive, and bioactive compounds such as flavonoids, phenolics, anthocyanins, phenolic acids, as well as, sugars, essential oils, carotenoids, vitamins, and minerals. Certain lesser-known fruits also have distinct flavors and tastes, excellent medicinal value, and health care functions (1–3).
The specie Cucurbita ficifolia Bouché belongs to the Cucurbitaceae family and is distributed from southern Mexico to Central and South America in temperate to humid temperate climates at altitudes of 1,000–3,000 m. Both the immature and ripe fruits are widely consumed by indigenous communities as part of typical cuisine, in traditional sweets and cool drinks or as fodder for domestic animals; the dark to creamy-white seeds are consumed as a condiment for dishes or as snacks. Fruits are globular, oval-elliptical, oblong, or ovoid globe with a polar diameter of 20–50 cm, with an epicarp or rigid shell and creamy-white, lemon green to dark green coloration with white longitudinal stripes toward the apex; the pulp or mesocarp and endocarp are white with a granular-fibrous texture and a slightly sweet taste. Fruits have been used in traditional medicine to relieve gastrointestinal disorders, hemorrhoids, and fever and receive different names from the original peoples of Mexico (i.e., “tzilacayotli” from Nahuatl or “gueeto-xembe” from Zapoteco). A plant can produce up to 50 fruits with an average weight of 6 to 7 kg (4, 5) (Figure 1).
Leafy vegetables are sources of natural antioxidants such as phenolics, flavonoids, betalains, xanthophylls, violaxanthin, ascorbic acids, carotenoids with strong antioxidant potential, betacyanins, betaxanthins, chlorophyll a, chlorophyll b, and beta-carotenes that have high radical quenching ability. These are also sources of essential minerals, including K, Ca, Mg, P, and S, and microelements, including Fe, Cu, Mn, Zn, Na, Mo, and B; they also supply protein, dietary fiber, carbohydrates, and vitamins, for human nutrition (6–15).
Phytochemical analyses of C. ficifolia pulp and different biological tests in vitro and in vivo with extracts of this species suggest the wide potential that they may have in family community health improvement and in pharmacology. Fruits have shown a hypoglycemic preventive and corrective effect against type 2 diabetes mellitus (16). Moya-Hernández et al. (17) pointed out that the aqueous extracts of ripe fruits lower glucose in rats with induced diabetes and that the response is a product of the joint action of all the phenolic compounds rather than of individual compounds. In similar models, Alarcón-Aguilar et al. (18) also found a favorable response, but by oral rather than intraperitoneal administration. Banderas-Dorantes et al. (19) compared the hypoglycemic effect of the C. ficifolia extract in relation to the induced effect by glibenclamide in rat aortic rigs, where they showed that the effect is explained by the ATP mechanism-sensitive potassium channels. Diaz-Flores et al. (20) attributed the hypoglycemic effect to its antioxidant properties in the glutathione redox cycle. It was also proposed that the effect is due to the D-chiro-inositol content in the fruit (21). However, the study of the mechanisms of hypoglycemic action, mainly by using aqueous extracts, is still in progress.
Alshammari et al. (22) reported that C. ficifolia fruit extracts have antimutagenic or anticarcinogenic potential against MCF-7 breast cancer cells, and their observations show that they inhibit cell division and induce p53/caspase-mediated apoptosis. Roman-Ramos et al. (23) proposed that the content of D-chiro-inositol in the fruit generates high antioxidant and anti-inflammatory activity in rats with diabetes induced with streptozotocin. Xia and Wang (24) pointed out that treatment with aqueous extracts of C. ficifolia in rats with induced type 1 diabetes for 30 days generated a significant reduction in blood glucose, triglycerides, and low-density lipoprotein (LDL) and an increase in the level of high-density lipoprotein (HDL). Bayat et al. (25) found similar responses by combinations of C. ficifolia fruits and yogurt in induced type 2 diabetes. The mechanisms of action of extracts of C. ficifolia against diabetes based on their composition have not yet been clarified; therefore, studies with greater precision are needed, as well as a focus on other mechanisms such as hepatoprotective, anticancer, antimicrobial, and antiulcer activities (26), including changes in composition due to environmental, genotypic, or varietal interactions. In the present work, it was important to know if there are differences between physicochemical parameters and compound with antioxidant activity from “chilacayote” landraces, so the proposed objective was to evaluate the variation in physicochemical parameters, phenolic compound contents and antioxidant activity in the fruit mesocarp or pulp of a collection of landraces from Oaxaca, Mexico, the center of origin and diversification of C. ficifolia, for current and potential reassessment purposes of pre-Columbian Mesoamerican food origin.
2 Materials and methods
2.1 Origin of landraces evaluated
Cucurbita ficifolia fruits from different populations were collected in land parcels and backyards of farmers in the municipalities of San Martin Huamelúlpam (Huamelúlpam) and Santo Domingo Yanhuitlán (Yanhuitlán), Oaxaca, Mexico. Four to six fruits were collected in a mature state per population and farmer, harvested in the 2021 crop cycle and after transported to the laboratory. The municipalities have a sub-temperate to temperate climate with rainfall of 7,000–1,000 mm distributed from June to November, average annual temperatures of 14–18°C, and average altitudes of 2,200 m. Yanhuitlán is located at 17° 31′ 1″ LN and 97° 19′ 59″ LW, and Huamelúlpam is located at 17° 23′ 48″ LN and 97° 36′ 24″’ LW in southeastern Mexico within the region known as Mesoamerica and its communities identified with the Mixteco indigenous group. The C. ficifolia populations collected and evaluated were made up of 10 populations from Huamelúlpam (ID: 01–10 H) and 10 from Yanhuitlán (ID: 01–10 Y).
2.2 Sampling and extract preparations
Once the hard epicarp or shell was removed, the seedless fruit mesocarp was obtained to make five different extract types: Aqueous (5 mg with 10 mL of solvent), ethanolic (1 g in 30 mL of solvent), acetone (5 g in 10 mL of 70% solvent acidified to 1% with HCl), protein [1 g with 6 mg of PVPP (polyvinylpolypyrrolidone) in 25 mL of KCl buffer solution with pH 13] and juice (20 mL), small pieces of mesocarp were processed in an electric domestic extractor (Breville JE95XL, CA, USA). The aqueous, acetone and protein extracts, and juice, were homogenized at 50 rpm for 1 min (DAIHAN-Brand HG-15-A Gangwon, Korea) and after centrifuged (Hettich, Universal 32R, Tuttlingen, Germany) at 4,000 rpm for 20 min. The mixture was filtered through two layers of cheesecloth, and the filtrate was used for further analysis. The ethanolic extract was left to stand for 24 h and was later centrifuged and filtered for analysis.
2.3 Evaluation of chemical composition of pulp or mesocarp
Soluble solid contents (SSC) and pH: SSC content was analyzed in a digital refractometer (ATAGO, PR-32, Tokyo, Japan) and expressed as °Brix from 0.1 mL of the aqueous extract (27). The pH was measured in the aqueous extract using a potentiometer (Oakton 510, Vernon Hills, USA) according to the method described by the AOAC (28).
Total sugars (TS): Total sugar content was evaluated by the phenol-sulfuric acid method described by Dubois et al. (29). A 1:20 dilution of the ethanolic extract was made from which 1 mL was taken and 100 μL of an 85% (p/v) phenol solution was added; then, it was homogenized with a vortex and 5 mL of H2SO4 conc. were added and homogenized again. It was incubated for 20 min in a water bath at 30°C, and the absorbance was read at 490 nm with a spectrophotometer (JENWAY 6305, Stafordshire, UK). Quantification was performed with reference to a standard calibration curve of anhydrous D-glucose (0.006–0.120 mg mL–1, r2 = 0.999). The results were expressed as mg of glucose per gram of sample on dry weight (mg G g–1 dw).
Protein: Protein content was estimated based on the method described by Bradford (30). A total of 500 μL of Bradford’s reagent was added to 500 μL of the extract, and the absorbance was read at 595 nm with a spectrophotometer. The concentration was calculated based on the bovine albumin standard curve (0.0005–0.018 mg mL–1, r2 = 0.995). The results were expressed as mg of bovine albumin (BA) per gram of sample on dry weight (mg BA g–1 dw).
Total polyphenols: Total polyphenol content was evaluated using the method described by Singleton and Rossi (31). First, 1 mL of distilled water and 200 μL of Folin–Ciocalteu were added and left to stand for 5–8 min. Then, 2 mL of 7% (w/v) Na2CO3 and 1.4 mL of distilled water were added to 400 μL of the acetone extract, vortexed and incubated for 1 h at room temperature, and the absorbance at 750 nm was measured using a spectrophotometer. Quantification was made with reference to the gallic acid standard curve (0.02–0.16 mg mL–1, r2 = 0.999), and the results were expressed in mg gallic acid equivalents per gram of sample on dry weight (mg GAE g–1 dw).
Total flavonoids: The determination of total flavonoid contents was made based on the method set up by Zhishen et al. (32). First, 75 μL of NaNO2 was added to 250 μL of the juice, incubated for 5 min and vortexed. Then, 150 μL of AlCl3 was added to the solution, and 1 min later, 500 μL NaOH was added to equal 3 mL with distilled water. The solution was stirred again, and finally, the absorbance at 510 nm was measured using a spectrophotometer. The flavonoid concentration was estimated based on the catechin standard curve (0.008–0.05 mg mL–1, r2 = 0.996), and the results were reported in mg equivalents of catechin per gram of sample on dry weight (mg CE g–1 dw).
Antioxidant activity: The antioxidant activity was evaluated by the DPPH (2,2-diphenyl-1-picrylhydrazyl) method described by Brand-Williams et al. (33) and by the FRAP method (ferric reducing antioxidant power) reported by Benzie and Strain (34). In the DPPH method, 2.9 mL of the DPPH solution was added to 100 μL of acetone extract in the dark. Subsequently, it was incubated at room temperature for 30 min, and the absorbance at 517 nm was recorded with a spectrophotometer. The reaction was estimated based on the Trolox standard curve (6-hydroxy-2,5,7,8-tetramethylchroman-2-carboxylic acid; 0.16–0.96 μmol mL–1, r2 = 0.997). In FRAP, the reaction of 100 μL of the acetone extract and 3 mL of FRAP reagent (300 mM C2H3NaO2, 10 mM TPTZ, FeCl3 6H2O in a 10:1:1 ratio) was started. It was incubated for 30 min in a water bath at 37°C, and the absorbance at 593 nm was recorded with a spectrophotometer. Additionally, in this case, the reaction was estimated based on the Trolox standard curve (0.14–1.4 μmol mL–1; r2 = 0.999). In both cases, the results were reported as μmol equivalents of Trolox on dry weight (μmol TE g–1 dw).
2.4 Identification of phenolic compounds by ultra-performance liquid chromatography-mass spectrometry
Accelerated extraction was performed using solvents (ASE 350, Thermo Scientific, Sunnyvale, CA, USA) based on the method described by Juárez-Trujillo et al. (35) and Monribot et al. (36). A 300.6 mg sample of the lyophilized fruit mesocarp (10 H originating from Huamelúlpam) was mixed with 100 mg of diatomaceous soil. The cell was filled with methanol to a pressure of 1,500 psi and heated at 60°C for 5 min. The extract was concentrated in a rotary evaporator (Büchi RII, Switzerland). Subsequently, 100 mg of the dried methanolic extract was dissolved in methanol with 0.1% formic acid (MS grade, Sigma-Aldrich, St. Louis, MO, USA), filtered with 0.5 μm (PTFE Syringe Filter, Agilent, Palo Alto, CA, USA) and placed in 2 mL UPLS vials until analysis in triplicate.
Phenolic compound identification and quantification were performed with an ultrahigh resolution liquid chromatograph (UPLC, Agilent 1,290 infinity) coupled to a triple quadrupole mass spectrometer (MS-MS, Agilent 6460). Chromatographic analysis was performed with an Agilent Eclipse Plus C18 column (2.1 mm × 50 mm, 1.8 Microns) worked at 40°C. For the elution of compounds, water with 0.1% formic acid (A) and acetonitrile with 0.1% formic acid (B) were used as mobile phases. The gradient conditions were as follows: 0 min 1% B, from 0.1 to 40 min linear gradient 1–40% B, 40.1–42 min linear gradient 40–90% B, 42.1–44 min isocratic 90% B isocratic, 44.1–46 min linear gradient 90–1% B, 46.1–47 min 1% B isocratic (total run time 47 min). The flow rate was 0.3 mL min–1, and the injection volume was 2 μL.
The identification and quantification of phenolic compounds was determined according to the analytical conditions described by Juárez-Trujillo et al. (35), and the results were expressed as g per gram of sample in dry weight (μg g−1 dw).
2.5 Statistical analysis
Once the description database was integrated with the physicochemical parameters, phenolic compounds and antioxidant activity for each sample, analysis of variances was performed using a completely random linear model to evaluate the effect of municipalities of origin on the composition and differences between nested populations in municipalities. Subsequently, mean comparisons were made using the Tukey method (p < 0.05), all using the SAS statistical program (37).
3 Results
3.1 Analysis of variance
In the analysis of variance, significant differences (p < 0.05, 0.01) were found between the municipalities of origin of the samples in SSC, pH, total sugars, and flavonoid content. Among populations within the municipalities of origin, the differences were significant (p < 0.01) for all the variables evaluated. These results show that the variation in SSC, total sugars, and flavonoids among populations is greater than the variation between municipalities of origin of the populations, based on the variance estimated for each source of variation (Table 1).
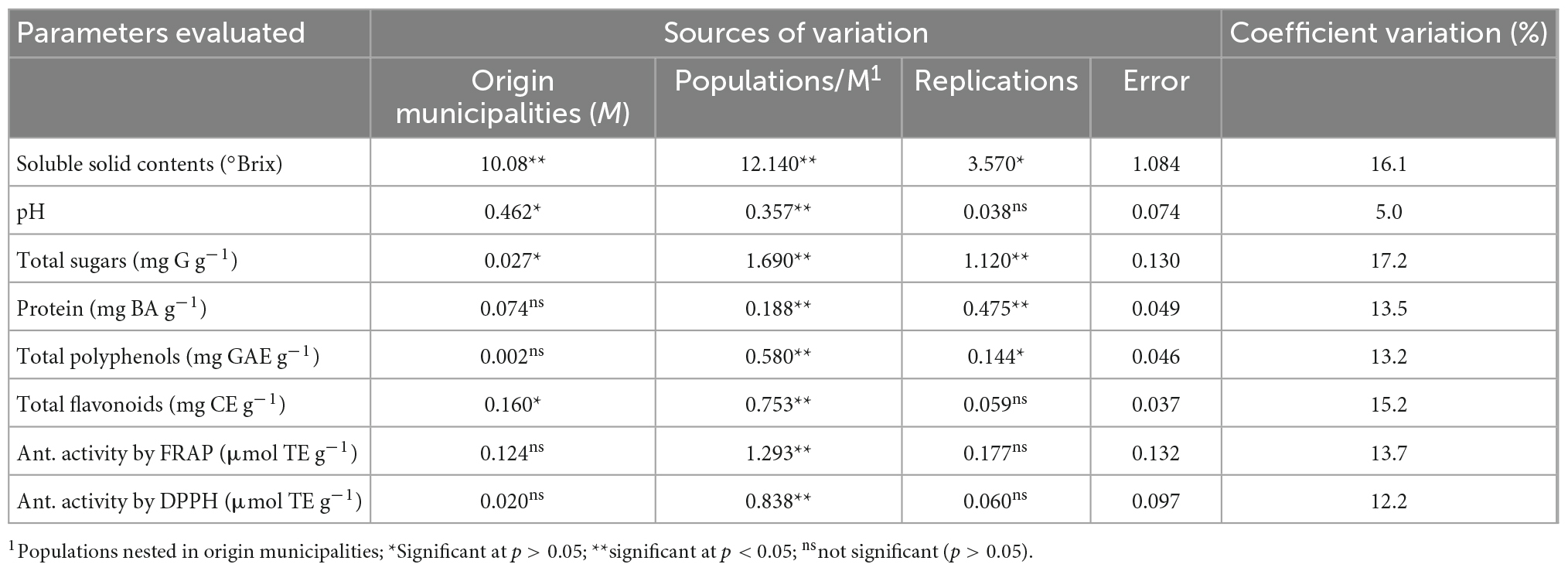
Table 1. Significance of square means of the analysis of variance of physicochemical parameters, phenolic compounds, and antioxidant activity in the pulp of C. ficifolia fruits.
3.2 Chemical composition of mesocarp or pulp
Significant differences in SSC, TS, and pH were recorded between the municipality of origin, with a lower average concentration of soluble solids and sugars in Huamelúlpam and a slightly lower pH concentration in Yanhuitlán. In contrast, the amount of protein was similar between municipalities. The variation between populations in SSC, TS, and pH ranged from 4.30 (08 Y) to 8.97 (07 Y) °Brix, 0.40 (06 Y) to 0.70 (09 H) mg G g–1 and from 4.81 (07 Y) to 5.79 (01 H), respectively. In protein, the variation was from 5.02 (05 Y) to 15.31 (08 Y) mg BA g–1, with significant differences between populations within each municipality of origin (Table 2). Greater variation was also found between populations within the municipality of Yanhuitlán.
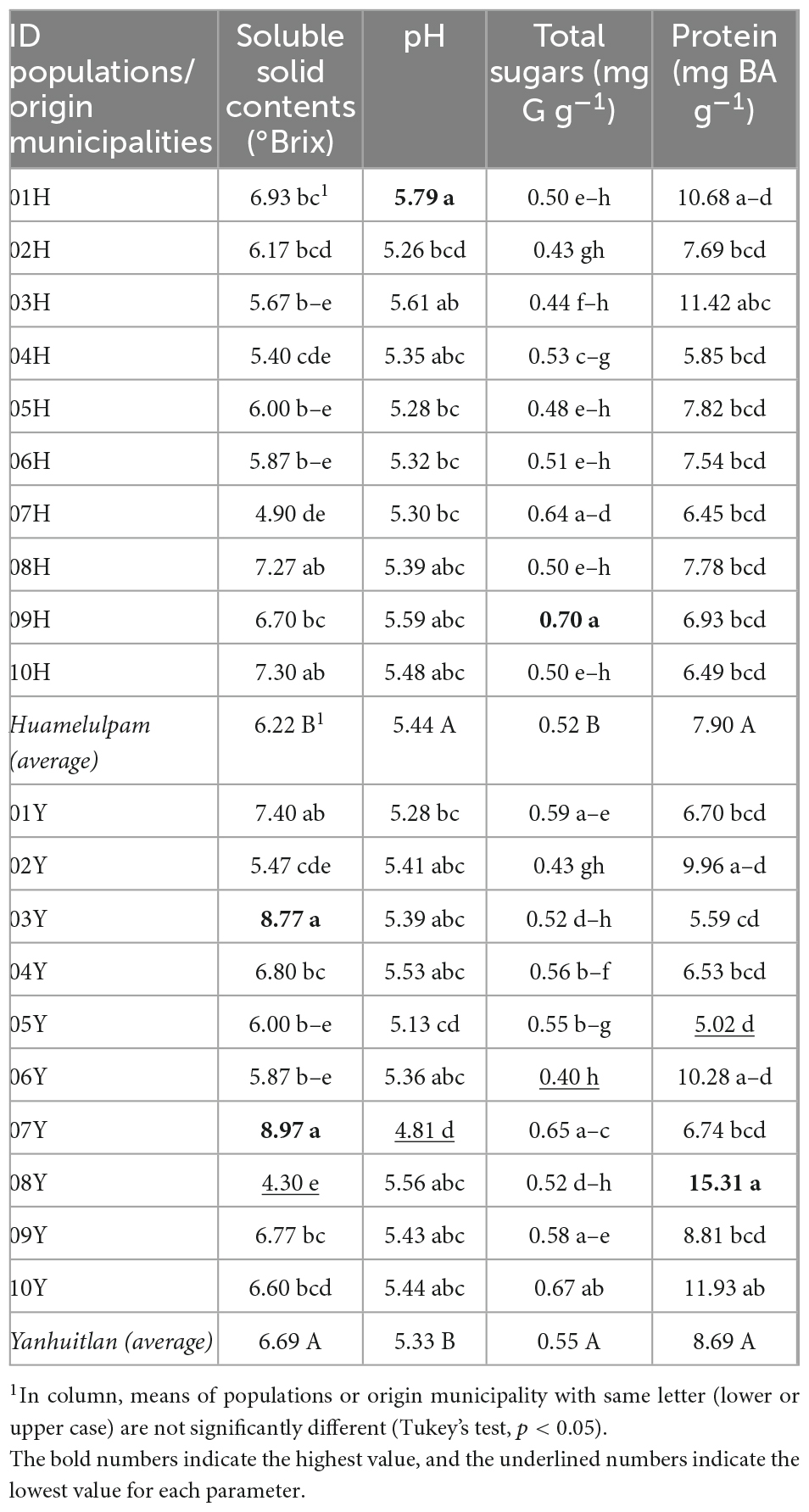
Table 2. Variation in soluble solid contents, pH, sugars, and protein content between C. ficifolia populations from two municipalities of Oaxaca, Mexico.
In total polyphenol contents and antioxidant activity, no significant differences were found (p > 0.05) between municipalities of origin of the evaluated populations; in flavonoids, the average of Yanhuitlán was higher than that of Huamelúlpam. Between populations, the variation in the total polyphenol and flavonoid concentrations ranged from 1.94 (05 Y) to 4.89 (06 Y) mg GAE g–1 and from 0.86 (03 Y) to 1.87 (08 Y) mg CE g–1, respectively. The antioxidant activity varied from 4.36 (08 H) to 10.63 (06 H) and from 4.57 (03 Y) to 12.59 (08 Y) μmol TE g–1 evaluated by DPPH and FRAP methods, respectively (Table 3). The variation between populations within the municipalities of Huamelúlpam and Yanhuitlán in total polyphenols was equivalent, 1.97–4.83 and 1.94–4.89 mg GAE g–1, respectively, but not in total flavonoid concentrations with values of 1.05–1.54 and 0.86–1.87 mg CE g–1, respectively, and shows that the landrace and area of origin influence the fruit mesocarp composition of C. ficifolia.
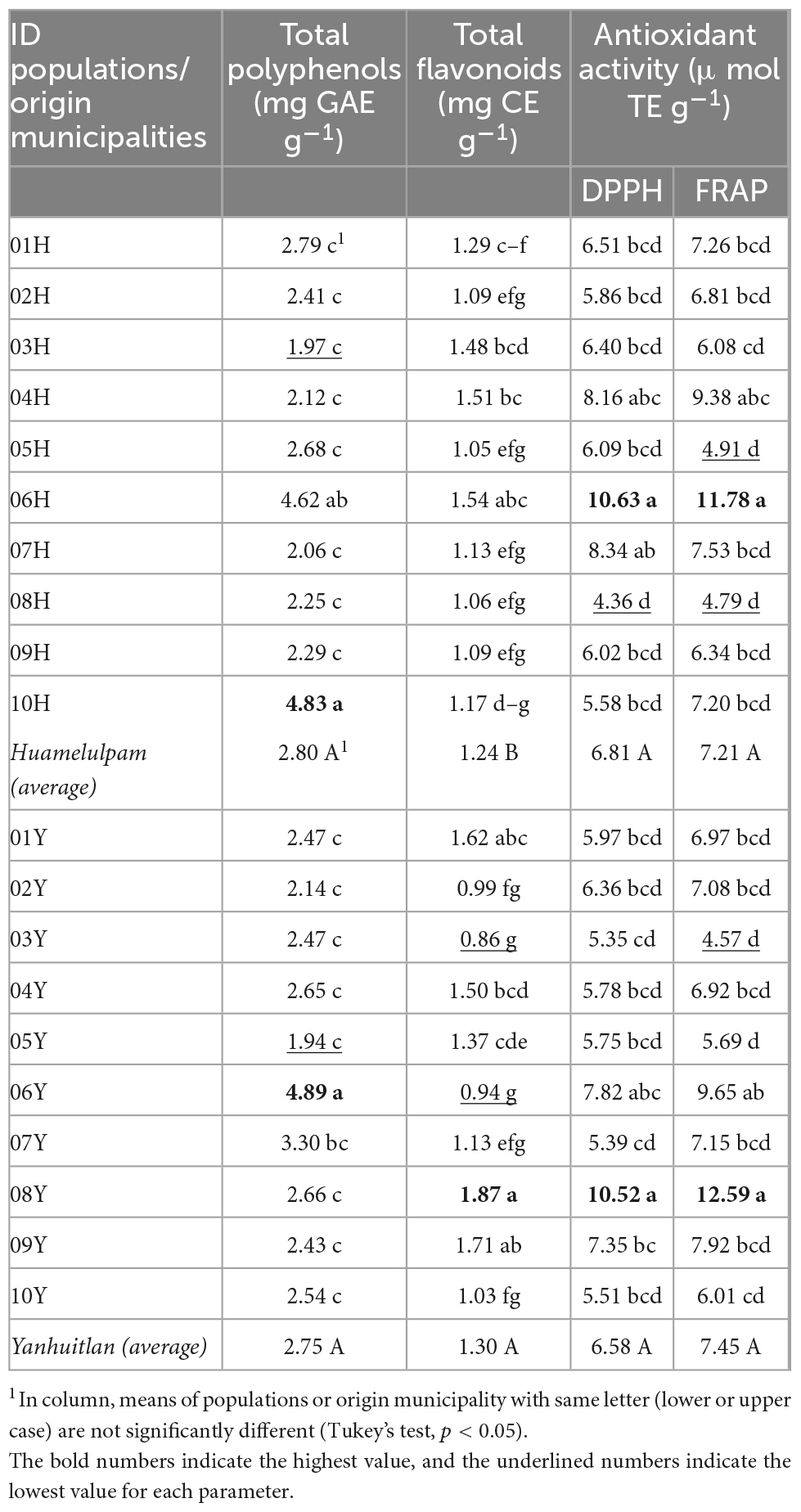
Table 3. Variation in total polyphenol and flavonoid contents and antioxidant activity between C. ficifolia populations from two municipalities of Oaxaca, Mexico.
Through UPLC-MS analysis, the following compounds were identified: L-phenylalanine, 4-hydroxybenzoic acid, 4-hydroxyphenylacetic acid, vanillic acid, vanillin, 4-coumaric acid, ferulic acid, and salicylic acid, with concentrations of 230.68 ± 14.9, 11.70 ± 0.1, 1.70 ± 0.1, 0.57 ± 0.03, 0.12 ± 0.01, 2.04 ± 0.11, 0.05, and 0.10 ± 0.01 μg g–1, respectively (Figure 2). These are an essential amino acid (L-phenylalanine), a phenolic aldehyde (vanillin), and six phenolic acids that contribute to the antioxidant capacity and functional properties of the fruit mesocarp of C. ficifolia.
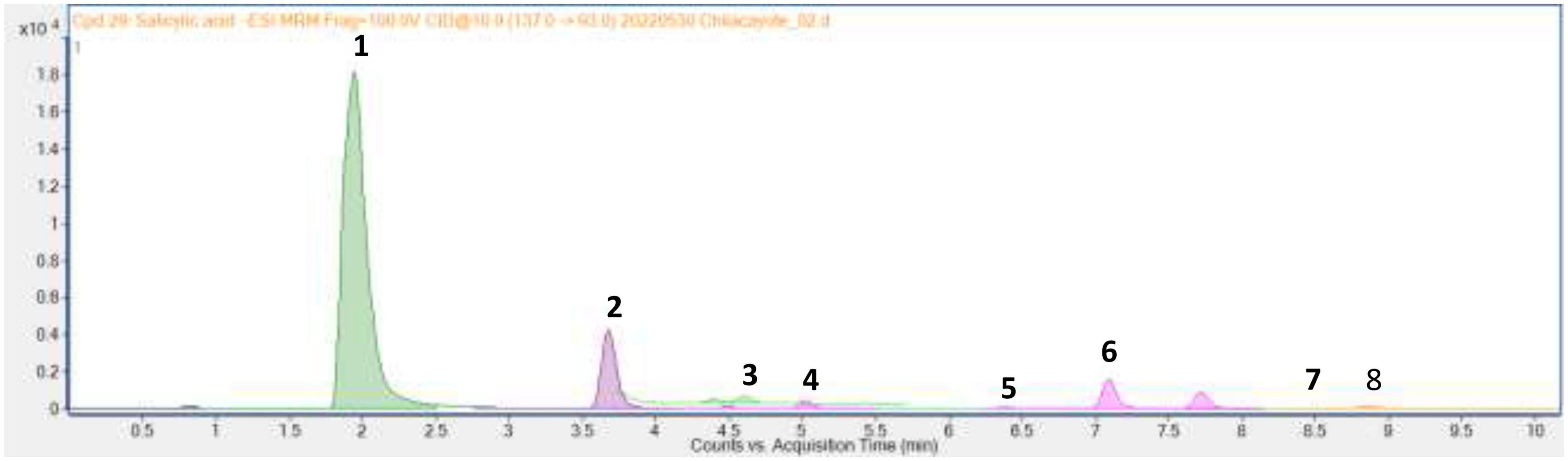
Figure 2. Chromatogram of methanol extract of C. ficifolia pulp where: 1, L-phenylalanine; 2, 4-hydroxybenzoic acid; 3, 4-hydroxyphenylacetic acid; 4, vanillic acid; 5, vanillin; 6, 4-coumaric acid; 7, ferulic acid; 8, ferulic acid.
4 Discussion
4.1 Chemical composition of mesocarp or pulp
In Mexico, Central and South America, Europe and Asia, the immature and ripe fresh fruits of C. ficifolia are consumed (4, 5), but it continues to be underutilized, unknown or consumed less often than the fruits of C. pepo, C. moschata, and C. maxima. Moya-Hernández et al. (17) showed that C. ficifolia fruits undergo different physiological processes from a few grams (primordia) to more than 7.2 kg in weight (>45 g after anthesis), and consequently, their external and internal composition changes, including the seed composition (38). That is, by changing the fruit composition, they can change their current and potential nutritional-nutraceutical value. In the present study, all the fruits evaluated were ripe with a rigid external epicarp and varied in weight from 7 to 9 kg. The average values of SSC in the fruits collected in Huamelúlpam and Yanhuitlán ranged from 4.30 to 8.97 °Brix, similar to values estimated by Moya-Hernández et al. (17), where these authors recorded a variation of 5.0–6.0 °Brix in the fruit’s development stage from 10 to 55 days after anthesis and slightly different from the interval of the present work of 4.30–8.97 °Brix. The estimation of SSC shows the amount of sugars and organic acids in the fruit pulp. In this work, the sugar concentration was similar to the average estimated by Jacobo-Valenzuela et al. (39) in C. moschata fruits (6.42 ± 2.26 °Brix) but slightly higher than the values reported by Martínez-Valdivieso et al. (40) in different landraces of C. pepo (3.4–4.7 °Brix) and lower than the values determined by Biesiada et al. (41) in C. maxima (9.4–10.9 °Brix) under increased application of organic matter (manure). The results show that the sugars contained in the C. ficifolia fruits provide a certain palatability similar to that of other cucurbits used fresh (vegetable) or processed during the preparation of traditional dishes.
The mesocarp of C. ficifolia fruits was acidic since among populations, it varied from 4.81 to 5.79, slightly lower than the range reported by Martínez-Valdivieso et al. (40) for C. pepo landraces (6.5–6.9) and for C. moschata (6.77 ± 0.7) registered by Jacobo-Valenzuela et al. (39), but similar to the records of Moya-Hernández et al. (17) in C. ficifolia (5.4–6.1). In this work, each population had its own patterns, and the pH showed a proportional relationship to the total sugar amount (0.2 ≤ r ≤ 0.7), for example, in the cases of 1 H, 03 H, 04 Y and 06 Y. In other cases, the relationship was similar in acidity or pH, and sugar content influenced the taste perception for C. ficifolia fruit consumers and could influence food preferences. The sugar content varied from 0.40 to 0.7 mg G g–1, slightly lower than that reported by Bressani (42), 0.702–0.777 mg G g–1.
The amount of protein in fruit pulp varied from 5.02 to 15.31 mg AB g–1, where four native populations from Yanhuitlán had contents higher than 9.95 mg AB g–1, but only two populations from Huamelúlpam exceeded that value. This is relevant from the nutritional point of view because it is possible to select populations among farmers with high protein values. The results are lower than the values reported in C. moschata (165.3 mg of AB g–1) and C. maxima (182 mg of AB g–1) using the Kjeldahl method (43, 44). Men et al. (45) pointed out that the protein content in C. moschata stands for 0.8–19.6%, but for the same species, Jacobo-Valenzuela et al. (39) showed that they represent 1.4%. Based on the proportionality showed for C. moschata and respecting the differences between species, it is possible to infer that C. ficifolia can supplement the diet for protein, among other contributions.
In different plant-based foods, the bioactive compounds and antioxidant activity are responsible of the functional activity once they are consumed more regularly or are included in a greater proportion of daily diets. Phenolics include coumarins; phenolic acids, such as hydroxybenzoic acids and hydroxycinnamic acids; flavonoids, such as flavonols, flavones, flavanols, flavanones, isoflavones, anthocyanins, and chalcones; and nonflavonoids, such as tannins, lignans, and stilbenes (46–51).
In C. ficifolia pulp, a variation in total polyphenol content was determined from 1.94 to 4.89 mg GAE g–1 dw. These values are consistent with the estimates of Moya-Hernández et al. (7), who shown that the concentration of gallic acid in C. ficifolia decreases from 16.97 to 0.24 mg g–1 from 15 to 45 days after anthesis (settling to fruit ripening). The values reported here are significantly lower than those reported by Oyeleke et al. (52) for C. maxima and C. mixta (30–45 mg GAE g–1 dw), while those determined by Hussain et al. (53) in C. maxima (1.34 ± 0.2 mg GAE g–1 dw) are slightly higher, as are the records of Priori et al. (54) in C. moschata (0.26–0.79 mg GAE g–1 fw). Similarities and differences in C. ficifolia polyphenols with respect to other cucurbits depend on distinct factors, such as the variety, stage of fruit development, plant growth conditions, laboratory methodologies, and their interactions. However, the food contribution of C. ficifolia fruit for consumers and its potential effects on health are relevant.
The pulp flavonoid content varied from 0.86 to 1.87 mg CE g–1, lower values than those found by Oyeleke et al. (52) in C. mixta and C. maxima (5–9 mg QE g–1) and by Mokhtar et al. (55) in C. moschata (28.66 mg QE g–1). Although these values are reported in terms of quercetin equivalents (QE), they are similar to those reported in catechin equivalents (CE), such as the records of Jacobo-Valenzuela et al. (39) in C. moschata (1.38 ± 0.54 mg CE g–1) and by Hussain et al. (53) in C. maxima (0.77 mg CE g–1). In these and other cases, the laboratory protocols, reference standards and extraction solutions used influence the flavonoid estimations. For example, Enneb et al. (44) determined significant differences in the estimation of C. moschata pulp flavonoids comparing the use of methanol (close to 30 mg QE g–1) vs. hexane, chloroform, and acetate (5–12 mg QE g–1) with a quercetin standard base. Despite the differences in estimated values, C. ficifolia pulp can contribute flavonoids to the diet, which have an antioxidant effect on the health of consumers and have benefits against coronary heart disease prevention and the antimutagenicity of certain cancer cells and antiviral processes are still under study (56).
The identification of six phenolic compounds, a vanillin and an amino acid by UPLC-MS in C. ficifolia pulp helps to describe the composition and its potential effects for consumers. L-phenylalanine, 4-hydroxybenzoic acid, 4-hydroxyphenylacetic acid, and 4-coumaric acid were the most relevant compounds in relation to the concentration in the evaluated population (230.7–1.7 μg g–1). These registered compounds differ from most compounds found by Enneb et al. (44) by LC-EST-MS in C. moschata: quinic acid, syringic acid, p-coumaric acid and trans-cinnamic acid. In contrast, this agrees with Mokhtar et al. (55) who found in vanillin and ferulic acid from a total of 33 phenolic compounds determined in C. moschata by HPLC-DAD-ESI-MS, and in 4-hydroxybenzoic acid and ferulic acid determined by HPLC-DAD in C. moschata and C. pepo by Kulczynski and Gramza-Michalowska (57). In fruit pulp of C. ficifolia, Moya-Hernández et al. (17) determined the presence of gallic acid, chlorogenic acid, catechin, epicatechin, syringic acid, and myricetin by HPLC-DAD, and Fortis-Barrera et al. (21) found D-chiro-inositol in C. ficifolia pulp extracts. All these compounds confer anti-inflammatory and antioxidant activity ability in oxidative stress conditions. This makes the complex of phenolic compounds present in the pulp of C. ficifolia confer functional and beneficial properties against risk factors for diabetes and other chronic degenerative diseases (55, 58, 59).
The antioxidant activity varied from 4.36 to 10.63 and from 4.57 to 12.59 μmol TE g–1 dw, based on DPPH and FRAP methods, respectively. These are higher values than those recorded by Mokhtar et al. (39) in C. moschata (0.048–0.065 μmol TE g–1 dw), by Kulczynski et al. (59) for C. moschata and C. pepo (0.48–1.50 μmol TE g–1 dw) and by Priori et al. (54) in C. moschata (0.71–3.58 μmol TE g–1 fw), all evaluated by the DPPH method. By the FRAP method, Kulczynski et al. (59) recorded variations from 1.89 to 5.16 μmol TE g–1 dw in C. moschata and C. pepo, and Nawirska-Olszanska et al. (60) determined values from 2.08 to 8.07 μmol TE g–1 dw in C. moschata and in C. ficifolia that recorded 2.10–2.27 μmol TE g–1 dw, values that coincide with part of this work. This shows that C. ficifolia pulp could be an important source of compounds with antioxidant activity, which could have an effect on factors that induce oxidative stress.
Physicochemical parameter analysis and the evaluation of phenolic compounds and antioxidant activity in 20 populations of C. ficifolia showed that the geographical origin and the populations preserved by small farmers present differences and high variability in pulp composition and can influence the potential benefits of its frequent consumption. The identification of eight compounds by UPLC-MS shows nutritional-nutraceutical potential. For example, L-phenylalanine and 4-hydroxybenzoic acid, are the main compounds identified and quantified in this work. Fortis-Barrera et al. (21) point out that the antioxidant and anti-inflammatory effect obtained in vitro with the application of C. ficifolia extracts is explained, in part, by its content of D-chiro-inositol, but they do not rule out that it is the product of other compounds present in the pulp. This same processing of C. ficifolia extract was used by Roman-Ramos et al. (23) in biological models in rats with induced diabetes and oral administration of the extract, where they concluded that it helps control diabetes mellitus and has hypoglycemic activities. Bayat et al. (25) pointed out that C. ficifolia juice combined with yogurt favored blood sugar control, LDL cholesterol reduction, and anti-inflammatory activity in the control of type 2 diabetes.
Alashammari et al. (22) used whole fruit flour and chloroform extraction, with later elimination, and found D-glucopyranosylamine, n-hexadecanoic and 1–4-cyclooctadiene as major components, then evaluated their effect on the growth of breast cancer cells and found that it induces MCF-7-cell death because it inhibits cell division and is proposed as a preventive agent. In these and other cases, aqueous or methanolic extracts are evaluated with more regularity using the seedless pulp or juice, but without isolation of specific compounds, and the results support the hypoglycemic effect and factors related to diabetes (19, 20, 24). These advances also show that it is necessary to continue expanding the knowledge of the C. ficifolia fruit composition and to evaluate its potential health effects.
5 Conclusion
We found wide variation in physicochemical parameters (SSC, pH, total sugars, and protein), total polyphenols and flavonoids, and antioxidant activity (evaluated by DPPH and FRAP methods) in fruits of 20 C. ficifolia native populations collected through two municipalities from Oaxaca, México (Huamelúlpam and Yanhuitlán). In addition, eight compounds were identified by UPLC-MS: L-phenylalanine (amino acid), vanillin (phenolic aldehyde), and six phenolic acids (4-hydroxybenzoic acid, 4-hydroxyphenylacetic acid, vanillic acid, 4-coumaric acid, ferulic acid, and salicylic acid), all with potential health effects. Specifically, L-phenylalanine and 4-hydroxybenzoic acid were the compounds with major concentrations, based on UPLCS-MS evaluation, and they have nutritional potential. Consequently, these results encourage us to recommend C. ficifolia fruit pulp consumption due to their potential effects on health as well as complement earlier studies about their composition and their effects on diabetes control.
Data availability statement
The original contributions presented in this study are included in the article/supplementary material, further inquiries can be directed to the corresponding author.
Author contributions
JA-J, EA-B, and JC-S: conceptualization and methodology. GM-Q, JA-J, and JC-S: investigation and writing. All authors read and agreed to the published version of the manuscript.
Funding
This research was funded by the Instituto Politécnico Nacional-Mexico by project no. SIP-20220820.
Acknowledgments
We acknowledge all families from the municipalities of San Martín Huamelulpam and Santo Domingo Yanhuitlán, Oaxaca, Mexico, who shared their C. ficifolia fruits (“chilacayota”) and experiences on preparations of the fruit for consumption. GM-Q thanks the scholarship from the CONACyT (no. 478457) to study a Master Science in Food Sciences. We also acknowledge José Antonio Guerrero-Analco and Juan Luis Monribot-Villanueva from the Department of Advanced Molecular Studies (REMAV), Institute of Ecology (INECOL), for the extraction, identification, and quantification of phenolic compounds.
Conflict of interest
The authors declare that the research was conducted in the absence of any commercial or financial relationships that could be construed as a potential conflict of interest.
Publisher’s note
All claims expressed in this article are solely those of the authors and do not necessarily represent those of their affiliated organizations, or those of the publisher, the editors and the reviewers. Any product that may be evaluated in this article, or claim that may be made by its manufacturer, is not guaranteed or endorsed by the publisher.
References
1. Dogan H, Ercisli S, Temim E, Hadziabulic A, Tosun M, Yilmaz S, et al. Diversity of chemical content and biological activity in flower buds of a wide number of wild grown caper (Capparis ovate Desf.) genotypes from Turkey. Comptes Rendus Acad Bulg Sci. (2014) 67:1593–600.
2. Grygorieva O, Klymenko S, Kuklina A, Vinogradova Y, Vergun O, Sedlackova V, et al. Evaluation of Lonicera caerulea L. genotypes based on morphological characteristics of fruits germplasm collection. Turk J Agric For. (2021) 45:850–60. doi: 10.3906/tar-2002-14
3. Saran P, Singh S, Solanki V, Choudhary R, Manivel P. Evaluation of Asparagus adscendens accessions for root yield and shatavarin IV content in India. Turk J Agric Forest. (2021) 45:475–83. doi: 10.3906/tar-2006-42
4. Lira-Saade R. Taxonomic and Ecogeographic Studies of Latin-American Cucurbitaceae with Economic Importance. Systematic and Ecogeographic Studies on Crop Genepools 9. Rome: International Plant Genetic Resources Institute (1995). p. 281.
5. Delgado-Paredes G, Rojas-Idrogo C, Sencie-Tarazona A, Vásquez-Núñez L. Caracterización de frutos y semillas de algunas cucurbitáceas en el norte del Perú. Rev Fitotec Mex. (2014) 37:7–20.
6. Sarker U, Rabbani M, Oba S, Eldehna W, Al-Rashood S, Mostafa N, et al. Phytonutrients, colorant pigments, phytochemicals, and antioxidant potential of orphan leafy Amaranthus species. Molecules. (2022) S27:2899. doi: 10.3390/molecules27092899
7. Sarker U, Iqbal M, Hossain M, Oba S, Ercisli S, Muresan C, et al. Colorant pigments, nutrients, bioactive components, and antiradical potential of danta leaves (Amaranthus lividus). Antioxidants. (2022) 11:1206. doi: 10.3390/antiox11061206
8. Sarker U, Oba S, Ercisli S, Assouguem A, Alotaibi A, Ullah R. Bioactive phytochemicals and quenching activity of radicals in selected drought-resistant Amaranthus tricolor vegetable amaranth. Antioxidants. (2022) 11:578. doi: 10.3390/antiox1103057
9. Sarker U, Lin Y, Oba S, Yoshioka Y, Hoshikawa K. Prospects and potentials of underutilized leafy amaranths as vegetable use for health-promotion. Plant Physiol Biochem. (2022) 182:104–23. doi: 10.1016/j.plaphy.2022.04.011
10. Sarker U, Oba S, Daramy M. Nutrients, minerals, antioxidant pigments and phytochemicals, and antioxidant capacity of the leaves of stem amaranth. Sci Rep. (2020) 10:3892. doi: 10.1038/s41598-020-60252-7
11. Sarker U, Oba S. Nutraceuticals, antioxidant pigments, and phytochemicals in the leaves of Amaranthus spinosus and Amaranthus viridis weedy species. Sci Rep. (2019) 9:20413. doi: 10.1038/s41598-019-50977-5
12. Sarker U, Hossain M, Oba S. Nutritional and antioxidant components and antioxidant capacity in green morph Amaranthus leafy vegetable. Sci Rep. (2020) 10:1336. doi: 10.1038/s41598-020-57687-3
13. Abeyrathne E, Nam K, Huang X, Ahn D. Plant- and animal-based antioxidants’ structure, efficacy, mechanisms, and applications: a review. Antioxidants. (2022) 11:1025. doi: 10.3390/antiox11051025
14. Sarker U, Oba S. Nutritional and bioactive constituents and scavenging capacity of radicals in Amaranthus hypochondriacus. Sci Rep. (2020) 10:19962. doi: 10.1038/s41598-020-71714-3
15. Sarker U, Oba S. Nutrients, minerals, pigments, phytochemicals, and radical scavenging activity in Amaranthus blitum leafy vegetables. Sci Rep. (2020) 10:3868. doi: 10.1038/s41598-020-59848-w
16. Bayat A, Jamali Z, Hajianfar H, Beni M. Effects of Cucurbita ficifolia intake on type 2 diabetes: review of current evidences. Shiraz E Med J. (2014) 15:e20586. doi: 10.17795/semj20586
17. Moya-Hernández A, Bosquez-Molina E, Verde-Calvo J, Blancas-Flores G, Trejo-Aguilar G. Hypoglycemic effect and bioactive compounds associated with the ripening stage of the Cucurbita ficifolia Bouche fruit. J Sci Food Agric. (2020) 100:5171–81. doi: 10.1002/jsfa.10566
18. Alarcón-Aguilar F, Hernandez-Galicia E, Campos-Sepulveda A, Xolalpa-Molina X, Rivas-Vilchis J, Vazquez-Carrillo L, et al. Evaluation of the hypoglycemic effect of Cucurbita ficifolia Bouche (Cucurbitaceae) in different experimental models. J Ethnopharmacol. (2002) 82:185–9. doi: 10.1016/s0378-874100176-9
19. Banderas-Dorantes T, Roman-Ramos R, Zamilpa A, García-Macedo R, Díaz M, Campos M, et al. Influence of two hypoglycemic Cucurbitaceae (Cucurbita ficifolia Bouche and Ibervillea sonorae Greene) on ATP-sensitive potassium channels in rat aortic rings. Bol Latinoam Caribe Plant Med Aromat. (2012) 11:510–9.
20. Díaz-Flores M, Angeles-Mejía S, Baiza-Gutman L, Medina-Navarro R, Hernández-Saavedra D, Ortega-Camarillo C, et al. Effect of an aqueous extract of Cucurbita ficifolia Bouché on the glutathione redox cycle in mice with STZ-induced diabetes. J Ethnopharmacol. (2012) 144:101–8. doi: 10.1016/j.jep.2012.08.036
21. Fortis-Barrera A, Alarcón-Aguilar F, Banderas-Dorantes T, Díaz-Flores M, Roman-Ramos R, Cruz M, et al. Cucurbita ficifolia Bouche (Cucurbitaceae) and D-chiro-inositol modulate the redox state and inflammation in 3T3-L1 adipocytes. J Pharm Pharmacol. (2013) 65:1563–76. doi: 10.1111/jphp.12119
22. Alshammari G, Balakrishnan A, Alshatwi A, Al-Khalifa A. Cucurbita ficifolia fruit extract induces Tp53/caspase-mediate apoptosis in MCF-7 breast cancer cells. Biomed Res Int. (2020) 2020:3712536. doi: 10.1155/2020/3712536
23. Roman-Ramos R, Almanza-Perez J, Fortis-Barrera A, Angeles-Mejia S, Banderas-Dorantes T, Zamilpa-Alvarez A, et al. Antioxidant and anti-inflammatory effect of the hypoglycemic fraction from Cucurbita ficifolia Bouche in streptozotocin-induced diabetes mice. Am J Chin Med. (2012) 40:97–110. doi: 10.1142/S0192415X12500085
24. Xia T, Wang Q. Effect of Cucurbita ficifolia fruit extract on lipid profile and oral glucose tolerance in streptozotocin-induced diabetic rats. J Food Biochem. (2009) 33:416–24. doi: 10.1111/j.1745-4514.2009.00229.x
25. Bayat A, Azizi-Soleiman F, Heidari-Beni M, Feizi A, Iraj B, Ghiasvand R, et al. Effect of Cucurbita ficifolia and probiotic yogurt consumption on blood glucose, lipid profile, and inflammatory marker in type 2 diabetes. Int J Prev Med. (2016) 7:30. doi: 10.4103/2008-7802.175455
26. Yadav D, Chauban P, Mishra M, Kwak M. Potential health benefits of Cucurbita ficifolia: an updated review. Prog Nutr. (2020) 22:e2020002. doi: 10.23751/pn.v22i3.9555
27. Association of Official Analytical Chemist. A.O.A.C. Official Methods of Analysis. 15th ed. Washington, DC: Association of Official Analytical Chemist (1990).
28. Association of Official Analytical Chemist. A.O.A.C. Official Method of Analysis Method 942.15. 18th ed. Washington, DC: Association of Official Analytical Chemist (2005).
29. Dubois M, Gilles K, Hamilton J, Rebers P, Smith F. Colorimetric method for determination of sugars and related substances. Anal Chem. (1956) 28:350–6. doi: 10.1021/ac60111a017
30. Bradford M. A rapid and sensitive method for the quantitation of microgram quantities of protein utilizing the principle of protein-dye binding. Anal Biochem. (1976) 72:248–54. doi: 10.1006/abio.1976.9999
31. Singleton V, Rossi J. Colorimetry of total phenolics with phosphomolybdic phosphotungstic acid reagents. Am J Enol Vitic. (1965) 16:144–58. doi: 10.1038/nprot.2007.102
32. Zhishen J, Mengcheng T, Jianming W. The determination of flavonoid contents in mulberry and their scavenging effects on superoxide radicals. Food Chem. (1999) 64:555–9. doi: 10.1016/S0308-814600102-2
33. Brand-Williams W, Cuvelier M, Berset C. Use of a free radical method to evaluate antioxidant activity. LWT Food Sci Technol. (1995) 28:25–30. doi: 10.1016/S0023-643880008-5
34. Benzie I, Strain J. The ferric reducing ability of plasma (FRAP) as a measure of “antioxidant power”: the FRAP assay. Anal Biochem. (1996) 239:70–6. doi: 10.1006/abio.1996.0292
35. Juárez-Trujillo N, Monribot-Villanueva J, Jiménez-Fernández V, Suárez-Montaño R, Aguilar-Colorado Á, Guerrero-Analco J, et al. Phytochemical characterization of izote (Yucca elephantipes) flowers. J Appl Bot Food Qual. (2018) 91:202–10. doi: 10.5073/JABFQ.2018.091.02
36. Monribot-Villanueva J, Elizalde-Contreras J, Aluja M, Segura-Cabrera A, Birke A, Guerrero-Analco J, et al. Endorsing and extending the repertory of nutraceutical and antioxidant sources in mangoes during postharvest shelf life. Food Chem. (2019) 285:119–29. doi: 10.1016/j.foodchem.2019.01.136
37. SAS Institute Inc. Base SAS ® 9.1.3 Procedures Guide. 2nd ed. Cary, NC: SAS Institute Inc (2006).
38. Carrillo W, Carrillo C, Carpio C, Morales D, Vilcacundo E, Álvares M, et al. Characterization of fatty acids in sambo oil (Cucurbita ficifolia L.) from Ecuador. Asian J Pharm Clin Res. (2018) 11:403–6. doi: 10.22159/ajpcr.2018.v11i2.15545
39. Jacobo-Valenzuela N, Zazueta-Morales J, Gallegos-Infante J, Aguilar-Gutierrez F, Camacho-Hernández I, Rocha-Guzmán N, et al. Chemical and physicochemical characterization of winter squash (Cucurbita moschata D.). Not Bot Hortic Agrobot Cluj. (2011) 39:34–40. doi: 10.15835/nbha3915848
40. Martínez-Valdivieso D, Gómez P, Font R, Alonso-Moraga A, Río-Celestino M. Physical and chemical characterization in fruit from 22 summer squash (Cucurbita pepo L.) cultivars. LWT Food Sci Technol. (2015) 64:1225–33. doi: 10.1016/j.lwt.2015.07.023
41. Biesiada A, Nawirska A, Kucharska A, Sokól-Letowska A. The effect of nitrogen fertilization methods on yield and chemical composition of pumpkin (Cucurbita maxima) fruits before and after storage. Veg Crops Res Bull. (2009) 70:203–11. doi: 10.2478/v10032-009-0020-0
42. Bressani R. Caracterización Química y Nutricional de la Semilla, Pulpa y Cáscara de Chilacayote (Cucurbita ficifolia Bouché) y Aplicaciones en el Desarrollo de Productos. Technical Report [FODECYT No. 023-2008]. Guatemala: Universidad del Valle de Guatemala (2015).
43. Łozicki A, Koziorzębska A, Halik G, Dymnicka M, Arkuszewska E, Niemiec T, et al. Effect of ensiling pumpkin (Cucurbita maxima D.) with dried sugar beet pulp on the content of bioactive compounds in silage and its antioxidant potential. Anim Feed Sci Technol. (2015) 206:108–13. doi: 10.1016/j.anifeedsci.2015.05.012
44. Enneb S, Drine S, Bagues M, Triki T, Boussora F, Nagaz K, et al. Phytochemical profiles and nutritional composition of squash (Cucurbita moschata D.) from Tunisia. S Afr J Bot. (2020) 130:165–71. doi: 10.1016/j.sajb.2019.12.011
45. Men X, Choi S, Han X, Kwon H, Jang G, Choi Y, et al. Physicochemical, nutritional, and functional properties of Cucurbita moschata. Food Sci Biotechnol. (2020) 30:171–83. doi: 10.1007/s10068-020-00835-2
46. Sarker U, Hossain M, Iqbal M, Oba S. Bioactive components, and radical scavenging activity in selected advance lines of salt-tolerant vegetable amaranth. Front Nutr. (2020) 7:587257. doi: 10.3389/fnut.2020.587257
47. Sarker U, Oba S. Nutraceuticals, phytochemicals, and radical quenching ability of selected drought-tolerant advance lines of vegetable amaranth. BMC Plant Biol. (2020) 20:564. doi: 10.1186/s12870-020-02780-y
48. Sarker U, Oba S. Polyphenol and flavonoid profiles and radical scavenging activity in leafy vegetable Amaranthus gangeticus. BMC Plant Biol. (2020) 20:499. doi: 10.1186/s12870-020-02700-0
49. Sarker U, Oba S. Phenolic profiles, and antioxidant activities in selected drought-tolerant leafy vegetable amaranth. Sci Rep. (2020) 10:18287. doi: 10.1038/s41598-020-71727-y
50. Sarker U, Oba S. Drought stress enhances nutritional and bioactive compounds, phenolic acids, and antioxidant capacity of Amaranthus leafy vegetable. BMC Plant Biol. (2018) 18:258. doi: 10.1186/s12870-018-1484-1
51. Sarker U, Oba S. Antioxidant constituents of three selected red and green color Amaranthus leafy vegetable. Sci Rep. (2019) 9:18233. doi: 10.1038/s41598-019-52033-8
52. Oyeleke A, Oluwajuyitan D, Oluwamukomi O, Enujiugha N. Amino acid profile, functional properties and in-vitro antioxidant capacity of Cucurbita maxima and Cucurbita mixta fruit pulps and seeds. Eur J Nutr Food Saf. (2019) 10:224–224. doi: 10.9734/ejnfs/2019/v10i430117
53. Hussain A, Kausar T, Din A, Murtaza M, Jamil M, Noreen S, et al. Determination of total phenolic, flavonoid, carotenoid, and mineral contents in peel, flesh, and seed of pumpkin (Cucurbita maxima). J Food Process Preserv. (2021) 45:e15542. doi: 10.1111/jfpp.15542
54. Priori D, Valduga E, Villela J, Mistura C, Vizzotto M, Valgas R, et al. Characterization of bioactive compounds, antioxidant activity and minerals in landraces of pumpkin (Cucurbita moschata) cultivated in Southern Brazil. Food Sci Technol. (2017) 37:33–40. doi: 10.1590/1678-457X.05016
55. Mokhtar M, Bouamar S, Di-Lorenzo A, Temporini C, Daglia M, Riazi A. The influence of ripeness on the phenolic content, antioxidant, and antimicrobial activities of pumpkins (Cucurbita moschata Duchesne). Molecules. (2021) 26:3623. doi: 10.3390/molecules26123623
56. Yao L, Jiang Y, Shi J, Tomás-Barberán F, Datta N, Singanusong R, et al. Flavonoids in food and their health benefits. Plant Food Hum Nutr. (2004) 59:113–22. doi: 10.1007/s11130-004-0049-7
57. Kulczyński B, Gramza-Michalowska A. The profile of secondary metabolites and other bioactive compounds in Cucurbita pepo L. and Cucurbita moschata pumpkin cultivars. Molecules. (2019) 24:2945. doi: 10.3390/molecules24162945
58. Huerta-Reyes M, Tavera-Hernández R, Alvarado-Sansininea J, Jiménez-Estrada M. Selected species of the Cucurbitaceae family used in Mexico for the treatment of diabetes mellitus. Molecules. (2022) 27:3440. doi: 10.3390/molecules27113440
59. Kulczyński B, Sidor A, Gramza-Michalowska A. Antioxidants potential of phytochemicals in pumpkin varieties belonging to Cucurbita moschata and Cucurbita pepo species. CyTA J Food. (2020) 18:472–84. doi: 10.1080/19476337.2020.1778092
Keywords: functional foods, antioxidant compounds, landraces, Mesoamerica, bioactive compounds
Citation: Moreno-Quiroga G, Alba-Jiménez J. E, Aquino-Bolaños EN and Chávez-Servia JL (2023) Phenolic compounds and antioxidant activity in Cucurbita ficifolia fruits, an underrated fruit. Front. Nutr. 9:1029826. doi: 10.3389/fnut.2022.1029826
Received: 27 August 2022; Accepted: 01 December 2022;
Published: 11 January 2023.
Edited by:
Ante Lončarić, University of Osijek, CroatiaReviewed by:
Umakanta Sarker, Bangabandhu Sheikh Mujibur Rahman Agricultural University, BangladeshJuan Facundo Massolo, National University of La Plata, Argentina
Copyright © 2023 Moreno-Quiroga, Alba-Jiménez, Aquino-Bolaños and Chávez-Servia. This is an open-access article distributed under the terms of the Creative Commons Attribution License (CC BY). The use, distribution or reproduction in other forums is permitted, provided the original author(s) and the copyright owner(s) are credited and that the original publication in this journal is cited, in accordance with accepted academic practice. No use, distribution or reproduction is permitted which does not comply with these terms.
*Correspondence: J. L. Chávez-Servia, ✉ jchavezs@ipn.mx