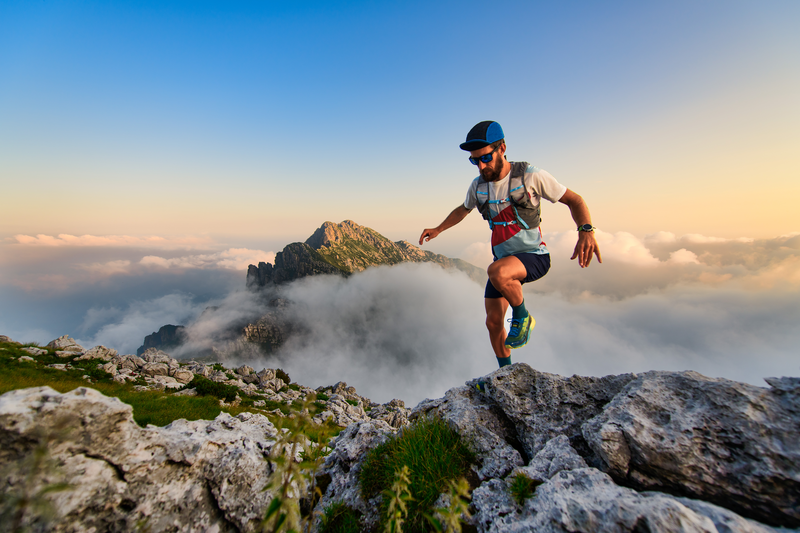
94% of researchers rate our articles as excellent or good
Learn more about the work of our research integrity team to safeguard the quality of each article we publish.
Find out more
ORIGINAL RESEARCH article
Front. Nutr. , 11 November 2022
Sec. Clinical Nutrition
Volume 9 - 2022 | https://doi.org/10.3389/fnut.2022.1029793
This article is part of the Research Topic Evidence-Based on Health Benefits: Probiotics, Micronutrients, and Edible Plants View all 19 articles
The present study is aimed to investigate the antioxidative potential and ameliorative effects of Lens culinaris Medikus sprouts hydroalcoholic extract (LSHE) on CCl4-induced oxidative stress in rats. The research has been carried out in two successive stages. Firstly, the highest phenolic content and antioxidant activity of L. culinaris sprouts were assessed at 20 ± 1°C and 90–93% RH during sprouting. Total phenolic content (TPC), total carotenoids (TC), total flavonoids (TF), total flavonols (TFL), DPPH-RSA, and vitamin C contents of L. culinaris seeds and 6-days sprouts were determined. Subsequently, phenolics by HPLC analysis of L. culinaris seeds, 3rd and 6th-day sprouts were identified and quantified. Results indicated that 6th-day sprouts contained considerable phenolics with superior antioxidant capacity, thus selected to be examined for biological activity in a rat's module consisting of five groups. G1, normal rats orally received distilled water. G2 received 1.0 mL kg−1 of CCl4 and olive oil (1:1) intraperitoneally (i.p.) twice a week. G3 received CCl4 (i.p.) and 50 mg GAE kg−1 of LSHE daily/orally. G4 received CCl4 (i.p.) 100 mg kg−1 of LSHE orally/daily. G5 (reference group) treated by intramuscular injection (i.m.) of vit. E+Selenium (Vit. E+Se, 50 mg kg−1 twice a week). The weight gain, relative weight of organs, hypoglycemic and hypolipidemic efficiencies, liver's and kidneys' functions, and antioxidant biomarkers were examined. LSHE enhanced the weight gain recovery % and significantly reduced fasting blood glucose. The hypolipidemic effect of LSHE was dramatically reduced triglycerides (TG), total cholesterol (CHO), high- and low-density lipoproteins (HDL-c and LDL-c), and very-low-density lipoproteins (VLDL-c). Administration of 50 and 100 LSHE mg kg−1 ameliorated liver and kidney function in dose-dependent manure. Intriguingly, LSHE considerably reduced malondialdehyde (MDA) while significantly raising reduced glutathione (GSH), superoxide dismutase (SOD), and catalase (CAT) in a dose-dependent manner. In conclusion, biochemical examinations confirmed the therapeutic efficacy of LSHE as a functional product. It encouraged us to recommend L. culinaris sprout production for attenuating hepatotoxicity and nephrotoxicity, as well as being beneficial and profitable for controlling oxidative stress complications.
An imbalance among the production of reactive oxygen species (ROS) and the body's detoxification or repair mechanisms is known as oxidative stress. When the cell's redox state is disturbed, toxic peroxides and free radicals are produced, harming lipids and DNA (1). DNA strands can be broken by oxidative stress, which causes underlying damage. Indirect base damage is caused by ROS, which generates the harmful free radicals. Because some reactive oxidative species serve as cellular messengers in redox signaling, oxidative stress can interfere with cellular communication. Oxidative stress is viewed as a potential factor in the development of cancer and Alzheimer's disease in humans (2), atherosclerosis (3), and depression (4). Researchers are looking for safe and effective plant-based bioactive inhibitory compounds to combat oxidative stress.
Pulses demonstrated a significant relationship between total polyphenol and tocopherol concentration and antioxidant activity (5, 6). The amount of polyphenol, carotenoid, and tocopherol bioactive in pulses varies depending on type and cultivar (5). Lentils are among the most nutrient-dense and health-beneficial foods, according to Faris et al. (6). It includes many necessary macronutrients, such as functional proteins and carbs, essential micronutrients, and bioactive phytochemicals. Recent classifications classify lentils as a preventive and therapeutic functional food (6, 7). As one of the top five superfoods, lentils (L. culinaris) have recently attracted more and more attention. In addition to being high in protein, they also contain significant amounts of dietary fiber, folate, iron, and potassium (8). The abilities of green lentil extracts to act as an antioxidant and as a radical scavenger were initially documented by Amarowicz et al. (9). Using HPLC-PAD and HPLC-ESI-MS methods, phenolics were also discovered in the crude extracts. Green lentils mainly included quercetin diglycoside, trans-p-coumaric acid, procyanidin dimers, catechin and epicatechin glucosides, quercetin, and epicatechin glucosides. Xu et al. (10) examined 11 samples of Lentils to determine their antioxidant capacities and four main phenolic groups, phenolic acids, anthocyanins, flavones, and flavan-3-ols—in each sample. Different cultivars of lentils showed considerable variations in their unique phenolic compounds and chemical and cellular antioxidant activities. The highest levels of total polyphenol and antioxidant activity were found in the large and small green lentils, while the split red lentil was among the cultivars of pulses with the least amount of antioxidant activity and the lowest overall polyphenol content (5). To make functional foods or nutraceuticals to enhance consumer health, food processors may use lentils with high phenolic content and antioxidant capabilities (7, 10, 11).
A remarkable variety of secondary metabolites, minerals, and bioactive substances found in lentils have shown promise in treating and preventing numerous chronic human diseases. In vivo and in vivo studies confirmed the positive correlation between their bioactive compounds, antioxidant capacity, and related health effects (5, 6, 11, 12). However, lentils' anticancer, hypoglycemic, hypocholesterolemic, and blood pressure-reducing properties and their potential to alleviate disease were examined (6, 7, 13). It has been claimed that lentil extracts have a variety of pharmacological effects both in vivo and in vitro, including antidiabetic, hypotensive, hypolipidemic, and cardioprotective effects (7). A study has also shown that the phenolic extract from lentils possesses direct ROS scavenging abilities (14, 15). According to Jung et al. (15), the phenolic extract of L. culinaris can serve as a possible source of nutraceuticals with hepatoprotective properties since it partially protects liver cells from oxidative stress by triggering the cellular antioxidant system. According to Goudarzi et al. (12), sodium arsenite (SA) can significantly increase oxidative stress while also depleting antioxidant reserves and blocking the actions of antioxidant enzymes. The oxidative hepatotoxicity caused by SA may be considerably reduced with red lentil extract (RLE). Its medicinal potential might be a cheap, secure, herbal antioxidative medication to treat SA toxicity.
Researchers struggle to improve the nutritional quality of lentils and ensure their abundant supply of bioactive phytochemicals that have health-promoting activity. Sprouts are a phytonutrient-rich vegetable food that is a good source of proteins, minerals, vitamins, flavonoids, polyphenols, glucosinolates, and isothiocyanates (16). Sprouting processes an applicable procedure to enhance phenolic content, antioxidant capacity, glycemic index, and potential bioaccessibility (17). Swieca et al. (18) confirmed that sprouting improved the nutraceutical value of lentil sprouts regarding their antioxidant potential. Interestingly, according to Reed et al. (19), sprouts are regarded as “functional foods,” defined as foods with additional health-promoting or disease-prevention benefits to their primary nutritional value. Studies on sprouts' nutritional value, phytochemical makeup during production or storage, and investigations into their microbiological, bioactive, and technological aspects have been suggested (20). The nutritional advantages and sensory acceptance of food products created with other sprouts were recently reviewed in this context (21).
Until now, nobody has looked into the bio-changes in phytochemicals during lentil sprouting. Therefore, the TPC, AOA, TC, TF, TFL, and vitamin C, as well as HPLC analysis of phenolic compounds during sprouting, were studied first. Consequently, a hydroalcoholic extract of 6-days green lentil sprouts (LSHE) was prepared.
Secondly, the antioxidative potential, hepatoprotective, and nephroprotective efficacy of green lentil sprouts hydroalcoholic extract (LSHE) on CCl4-induced oxidative stress were investigated.
Green lentil seeds (L. culinaris Medikus) were purchased from the Al-Tamimi market (https://www.tamimimarkets.com) in Al Qassim region, K.S.A. Plant expert (Dr. Mokded Rabhi) from the College of Agriculture and Veterinary Medicine at Qassim University in K.S.A., carried out the plant's verification. The broken, sick, and dusty seeds were eliminated. Green lentil sprouts were made from cleaned seeds. Until they were used for analysis, raw or milled seeds (American model laboratory mill, model ES2097) were kept in freezer-plastic bags at a temperature of 18 ± 1°C.
The seeds were sanitized into a solution of sodium hypochlorite (1%) for 3 min before sprouting in batches of 500 g. The seeds were evenly distributed on 7 × 35 cm plastic trays after being rinsed three times in sterilized distilled water (sd.H2O). The seed germinator was then filled with the seeds. The germination procedure was done in an atomizer-equipped temperature-controlled seed germinator (Easygreen, Canada) with a relative humidity of 90–93% with faith light. The temperature of the germinator was kept at 20 ± 1°C. For the first 3 days, 10.0 mL of sd.H2O/tray was sprayed onto lentil samples thrice daily. Beginning at the germination process and continuing for up to 6 days, appropriate samples were taken daily. Lentil sprouts were frozen overnight at −18 ± 1°C, then freeze-dried (CHRIST, Alpha 1-2 LD plus, Germany) for 96 h at −48°C under the pressure of 0.032 mbar. Freeze-dried sprouts were crumbled in a small mill (Thomas Wiley, USA) to obtain a homogenous powder, then kept in the dark containers at 4 ± 1°C for HPLC and phytochemical analysis. Lentil sprouts were individually germinated in identical settings for 6 days. Progressively, Lentil sprouts were dried using a 24-hdrying program according to Barakat et al. (22) and Al-Qabba et al. (23). The dried sprouts were milled, sieved, and kept under cold storage until extraction. Lentil sprouts hydroalcoholic extract (LSHE) was carried out by extracting about 500 g of lentil sprouts three times with 2,500 mL of 50% ethanol. The filtered extract was concentrated in a rotary evaporator at 40°C, then frozen overnight and freeze-dried (CHRIST, Alpha 1–2 LD plus, Germany) (24). Freeze-dried samples were powdered by porcelain morsel to make homogeneous powder which was kept under cooling conditions until used.
According to Yawadio Nsimba et al. (25), the TPC of L. culinaris seeds and sprouts was determined using the Folin-Ciocalteu reagent. In brief, a suitable sample was extracted with 70% methanol. Aliquots of clear supernatant were mixed with (1:10) diluted Folin-Ciocalteu reagent for 5 min before being stopped with Na2CO3 (7.5 %). The optical density (OD) was measured after 60 min and compared to the standard curve of Gallic acid (GA) solution (R2 = 0.99), and the TPC content was expressed as milligrams of Gallic acid equivalents (GAE) per 100 g (mg of GAE 100 g−1 DW). For TC determination, 1 g of the freeze-dried sample was repeatedly extracted with a mixture of acetone and petroleum ether (1:1, v/v), according to Yuan et al. (26). The upper phase was collected, washed with water several times, and combined with crude extracts. The petroleum ether will be added to the solution to prepare a known volume. The TC content was determined spectrophotometrically at 451 nm and expressed as mg 100 g−1 dw. The upper phase was collected, washed with water several times, and combined with crude extracts. The petroleum ether will be added to the solution to prepare a known volume. The TC content was determined spectrophotometrically at 451 nm and expressed as mg 100 g−1 dw. The TF content of L. culinaris seeds and sprouts using a methanolic extract was determined. Aliquots of clear extract were mixed with 2% AlCl3, then measured after 60 min at 420 nm. The TFL content of L. culinaris seeds and sprouts was determined by combining aliquots of methanolic extracts with sodium acetate (5 %). After 5 min, AlCl3 (2%) was added, and the OD was measured after 150 min at 440 nm, according to Mohdaly et al. (27). The content of TF and TFL were expressed as mg quercetin equivalent (QE) per g−1 (mg QE 100 g−1).
The vitamin C content using the 2,6-dichloro phenol indophenol titrimetric method was determined according to Silva et al. (28); data were expressed as mg 100 g−1 fw. The radical scavenging activity (DPPH-RSA) of L. culinaris seeds and sprouts was examined spectrophotometrically according to Barakat and Rohn (29), and antiradical activity value was presented as μmol TE 100 g−1.
According to Kim et al. (30), using an HPLC system HP1100 (Agilent Technologies, Palo Alto, CA, USA) equipped with an autosampler, quaternary pump, and diode array detector DAD, as well as an Altima C18, 5 × 150 mm, 4.6 mm ID column and an Altima C18, 5 mm guard column (Alltech), the phenolic compounds in L. culinaris and its sprouts were determined. At a flow rate of 1 mL min-1, 10 μl of the extracted samples were injected, and separation was carried out at 25°C. The gradient of acetic acid concentrations in the solvent system was A (acetic acid 2.5%), B (acetic acid 8%), and C (Acetonitrile 100%). For identification and quantification, each peak's retention times and mass spectra were compared to external standards and stored; then, phenolic compounds were expressed in mg Kg−1.
This study used Wistar rats (40 adult males) weighing 175–200 g. All the experiments received approval from the Institutional Animal Ethics Committee (IAEC) of QU, KSA (No. 21-18-09 on Thursday, May 19, 2022), Qassim University, SA. Under typical laboratory conditions, animals were housed in polypropylene cages with air conditioning and kept at 24°C. Rats were placed in new cages under controlled circumstances of 24°C, 40–45% relative humidity, and a 12-h light/dark cycle after being exposed to the environment for 10 days. Randomly, five groups of eight rats each were assigned to the groups. The rats' body weight (BW) was noted along with their identification labels. Rats were given a commercial standard pellet diet and unlimited access to water (31). The following procedures were used to treat the rats for six consecutive weeks. Group 1 (normal rats, NR) received 2 mL of distilled water orally/daily and an intraperitoneal injection (i.p.) of olive oil (1.0 mL kg−1) twice a week. For oxidative stress and hepatotoxicity induction in experimental animals. Rats were administrated (i.p.) with a fresh solution of CCl4 and olive oil (1:1) at a dose of 1.0 mL kg−1 twice a week and 2 ml of distilled water orally/daily (32). After 1 week, rats were randomly divided into four groups, eight each, and one of those groups was labeled as Group 2 and located and positive control. Group 3 received CCl4 (i.p.) twice a week in addition to 50 mg GAE kg−1 of LSHE given orally daily. Group 4 received CCl4 (i.p.) twice a week in addition to 100 mg kg−1 of LSHE administered orally daily. According to Asuku et al. (33) and Gaber et al. (34), Group 5 (reference group) received an intramuscular injection (i.m.) of Vit. E+Se (Selepherol, Vetoquinol Co., France) at 50 mg kg−1 twice a week and 2 mL distilled water orally daily. At the end of the 6th week, animals fasted for 12 h with free access to water. According to Leila et al. (35), rats were anesthetized. Blood was collected from the heart puncture and then treated to separate blood serum by centrifugation at 4,000 x g for 30 min for use in various biochemical measures. Appropriate kits and a blood chemistry analyzer (HumaLyzer 4000, Germany) were used to determine the biochemical parameters. Rats' liver, kidneys, and spleen were removed during the dissection of sacrificed animals. The following equation was used to determine the relative weight (RW) of the organs:
Alanine aminotransferase (ALT, UL−1), aspartate aminotransferase (AST, UL−1), alkaline phosphatase (ALP, UL−1), and total bilirubin (T. Bili, mg dL−1) in blood serum were measured using specific and approved kits following the manufacturing instructions. According to the manufacturer's instructions, kidney functions such as albumin (g dL−1), total protein (T. Protein, g dL−1), urea (mg dL−1), and creatinine (mg dL−1) concentrations were measured. Albumin concentrations were subtracted from T. Protein concentrations to calculate globulin (g dL−1). Dividing the urea concentration by 0.47, blood urea nitrogen (BUN, mg dL−1) was calculated. All biochemical test kits were bought from Human Co. in Wiesbaden, Germany. According to Nwagha et al. (36), the atherogenic index (AI) was calculated. An enzymatic colorimetric test kit was used to determine fasting blood glucose (mg dL−1). High-density lipoproteins (HDL-c, mg dL−1) and total cholesterol (CHO, mg dL−1), and triglycerides (TG, mg dL−1), according to manufacturer instructions, were examined. According to Friedewald et al. (37), low-density lipoproteins (LDL, mg dL−1) and very-low-density lipoproteins (VLDL, mg dL−1) were mathematically calculated.
According to the described method by Beutler et al. (38), reduced-glutathione (GSH, μg dL−1) was determined. According to Ohkawa et al. (39), lipid peroxidation was evaluated by detecting thiobarbituric acid reactive substance (TBARS), and the measured malondialdehyde (MDA) concentration was expressed as nmol mL−1. Superoxide dismutase (SOD, U L−1) activity was determined following the protocol of Giannopolitis and Ries (40). Catalase (CAT, U L-1) activity was assessed using the Aebi technique (41). All oxidative-stress biomarkers were determined using a blood chemistry analyzer (HumaLyzer 4000, Germany).
The SPSS (Ver. 22.0 for Windows) was used to conduct the statistical analysis. According to Steel et al. (42), one-way ANOVA was used to assess the statistical significance, p-values of 0.05 were used for the post-hoc test, and means of the experimental results and standard error were presented.
The quantitative analysis of phytochemicals such as TPC, TC, TF, and TFL, as well as related antioxidant activity using DPPH radical scavenging and vitamin C content in L. culinaris sprouts, was performed. The TPC content of green lentil seeds (GLS) was 379.76 mg GAE 100 g−1, as illustrated in Table 1. The TC content of GLS was 14.15 g 100 g−1. Both TF and TFL contents in GLS were 16.32 and 11.17 mg QE 100 g−1, respectively. Furthermore, the development of antioxidant activity was tracked using DPPH-RSA. The results showed 479.42 mol of TE 100 g−1 in GLS. The vitamin C content of GLS was 42.91 mg 100 g−1. On the 6th day, significant increases in TPC, TC, TF, and TFL, as well as Vit. C were observed.
Table 1. Total phenolic, carotenoids, flavonoids, flavonols contents, and potential antioxidant capacities of L. culinaris during sprouting at 20 ± 1°C and 90–93% RH (mean ± SE), n = 6.
The quantitative analysis of phenolics in extracts of L. culinaris seeds and sprouts was carried out; the data are illustrated in Table 2. Thirteen separated phenolic acids and five flavonoids were identified in GLS and its sprouts in detectable amounts. The most abundant phenolics were p-Hydroxy benzoic acid (71.34 mg Kg−1), followed by p-coumaric acid (38.46 mg Kg−1) and Vanillic acid (21.40 mg Kg−1). The GLS is abundant in TF content, as demonstrated in Table 2. TF such as Naringenin (112.62 mg Kg−1) and Quercetin (35.29 mg Kg−1) were identified and found in higher amounts, followed by Myricetin (28.58 mg Kg−1), Resveratrol (19.00 mg Kg−1) and Kaempferol (15.27 mg Kg−1). On the 3rd day of sprouting, Benzoic acid, Rosmarinic acid, and Syringic acid as phenolic acids were presented in 42.73, 28.48, and 28.48 mg Kg−1, respectively. Similarly, new flavonoids have been shown in reasonable amounts with an increase in detected flavonoids in GLS except for Naringenin. Rutin and Catechin were quantified at 50.02 and 4.19 mg Kg−1 in the 3rd day's sprouts. Seven flavonoids, eleven phenolic acids, and their derivatives were identified and measured on the 6-day. The most abundant phenolic acids were Rosmarinic acid, p-Hydroxy benzoic acid, and p-coumaric acid. In contrast, the most abundant flavonoids were Kaempferol, followed by Myricetin, Naringenin, Resveratrol, Quercetin, Rutin, and Catechin. On the 6th day, phenolic acids and flavonoids were remarkably increased, whereas the flavonoids recorded increases higher than phenolic acids and their derivatives.
Table 2. Quantitative analysis of phenolic compounds in L. culinaris during sprouting at 20 ± 1 °C and 90–93% RH (mean ± SE), n = 3.
The weight gain, RW of organs, and hypoglycemic efficiency of GLR extracts in CCl4-induced oxidative stress and hepatotoxicity in rats were scrutinized; data are presented in Table 3. Injection of CCl4 directly affected the rats' weight during the 1st week; however, virtually little weight gain was observed in G2 rats in the 6th week. The most efficient treatment in recovering rats' weight was administering 100 mg GAE Kg−1 LSHE compared with G1 or G5 at the end of the 6th week. LSHE significantly improved weight gain associatively in a dose-dependent manner. For organs' relative weight, the injected group exhibited significant increases in organs' weight. Treating rats with LSHE or Vit. E+Se showed a positive attenuation. After the 6th week, LSHE at 50 or 100 mg GAE Kg−1 exhibited a potent efficacy in reducing fasting blood glucose but not better Vit. E+Se at 50 mg Kg−1, as shown in Table 3.
Table 3. Effect of L. culinaris sprouts hydroalcoholic extract on weight gain%, organs' weight, and FBG in CCl4-induced oxidative stress and hepatotoxicity in rats (mean ± SE), n = 8.
The hypolipidemic efficiency of LSHE at 50 and 100 mg GAE Kg−1 and Vit. E+Se at 50 mg kg−1 on CCl4-induced oxidative stress and hepatotoxicity in rats were determined; data are shown in Table 4. The CCl4-induced oxidative stress and hepatotoxicity in rats were observed to significantly raise the levels of TG, CHO, LDL-c, and VLDL-c. However, CCl4 injection resulted in significantly lower HDL-c levels than in control rats (G1). The lipid profile was improved in dose-dependent manure by administering LSHE at 50 or 100 mg GAE Kg−1. The most effective therapy for enhancing the blood profile was LSHE with 100 mg GAE Kg−1, which showed no significance when compared to G1 or G5. However, comparing G5 (41.55%), administrating LSHE at 50 or 100 mg GAE Kg−1 reduced the TG level by 33.56 and 34.44%, respectively. Interestingly, the rate of CHO reduction was 33.11, 39.55, and 41.61% for treating rats with 50, 100 mg GAE Kg−1 of LSHE and 50 mg Kg−1 Vit. E+Se, respectively. HDL-c increase was recorded as 12.92, 41.95, and 34.78%, whereas LDL-c decrease was noted as 47.12, 65.37, and 64.64% after LSHE at 50, 100 mg GAE Kg−1 or 50 mg Kg−1 Vit. E+Se treatments, respectively. VLDL-c level was improved with treatments associatively in a type and dose-dependent manner. LSHE with 100 mg LSHE Kg−1 was significantly better than 50 mg LSHE Kg−1. Fascinatingly, when CCl4 was injected, the AI significantly raised compared to normal rats (G1). Indeed the most efficient treatments for attenuating the atherogenicity complication were those giving 100 mg GAE Kg−1 of LSHE, which presented better attenuation than 50 mg GAE Kg−1 of LSHE and even normal rats. The superior effect was recorded for using 50 mg Kg−1 Vit. E+Se did not differ significantly from using 100 mg GAE Kg−1 of LSHE.
Table 4. Effect of hydroalcoholic extract of L. culinaris sprouts on lipid profile and Atherogenic index in CCl4-induced oxidative stress and hepatotoxicity in rats (mean ± SE), n = 8.
CCl4 injection considerably raised serum AST, ALT, and ALP enzyme levels in G2 rats as oxidative stress and hepatotoxicity complications compared to normal rats (GI). The T. Bili level was significantly increased in CCl4-treated rats (Figure 1). Administration of LSHE at 50 or 100 mg GAE Kg−1 and Vit. E+Se at 50 mg Kg−1 improved the liver's function. A high level of LSHE was better than a low level of LSHE or Vit. E+Se to improve liver functions. Interestingly, giving LSHE reduced the modifications in liver functions caused by CCl4 injection to be close to typical values in GI (Figure 1). The ALT level attenuated by 28.68, 38.22, and 35.94% when 50 and 100 mg GAE LSHE Kg−1 and 50 Kg−1 Vit. E+Se were given, respectively. Similarly, ATS and ALP improved by 20.01, 30.44, and 32.71% and 19.83, 28.48, and 28.80%, respectively. However, in comparison to NR in G1, LSHE, and Vit. E+Se significantly enhanced some liver functions such as T. Bili and the liver enzymes as shown in (ALT, ALP, and AST). T. Bili level was attenuated by 26.00, 28.67, and 36.67% when rats were administrated when 50 and 100 mg GAE LSHE Kg−1 and 50 Kg−1 Vit. E+Se, respectively.
Figure 1. Effect of hydroalcoholic extract of L. culinaris sprouts on liver's functions in CCl4-induced oxidative stress and hepatotoxicity in rats (mean ± SE), n = 8. (A) ALT: Alanine aminotransferase. (B) AST, Aspartate Aminotransferase. (C) ALP, Alkaline phosphatase. (D) T. Bili, Total bilirubin, a,b,c,d: Bars not sharing similar letters are statistically different at p < 0.05.
The nephroprotective efficiency of LSHE at 50 or 100 mg GAE Kg−1 and Vit. E+Se at 50 mg Kg−1 on CCl4-induced oxidative stress and hepatotoxicity in rats were studied; results are demonstrated in Table 5. CCl4 injection considerably increased serum urea, BUN, and creatinine levels in G2 rats compared to NR in GI. Conversely, albumin, T. Protein, and globulin levels were drastically decreased in CCl4-treated rats (Table 5). LSHE at 50 or 100 mg GAE Kg−1 and Vit. E+Se at 50 mg Kg−1 treatments significantly attenuated urea, creatinine, and BUN alterations caused by CCl4 problems. Albumin, T. Protein, and Globulin levels were also raised to nearly normal levels in the GI (Table 5). The most effective enhancement was evidently recorded with LSHE at 100 mg GAE Kg−1 even sometimes better than using Vit. E+Se at 50 mg Kg−1 compared to normal rats (G1).
Table 5. Effect of hydroalcoholic extract of L. culinaris sprouts on kidneys' functions in CCl4-induced oxidative stress and hepatotoxicity in rats (mean ± SE), n = 8.
Injection of CCl4 dramatically decreased GSH, SOD, and CAT levels and elevated MDA levels in the blood serum of G2 compared to NR in G1, as shown in Figure 2. The activity of the antioxidant enzymes GSH, CAT, and SOD was significantly improved in the treated rats treated with 50 or 100 mg GAE Kg−1 and Vit. E+Se at 50 mg Kg−1 and the levels of MDA were significantly decreased, as shown in Figure 2. On the other hand, treatment of 50 mg LSHE Kg−1 exuded minimal diminution in GSH, CAT, and SOD and inhibited the autoxidation process, resulting in low MDA levels. Compared to the CCl4-group (G2), the most effective treatment for GSH, DMA, CAT, and SOD was LSHE with 100 mg Kg−1, which showed improved rates of 76.36, 29.47, 37.82, and 31.72 %, respectively. Comparing rats treated with CCl4 and normal rats (G1), it is interesting to note that rats treated with 50 mg Kg−1 of vit. E+Se dramatically improved their enzymatic defense system (G2).
Figure 2. Effects of oral administration of hydroalcoholic extract of L. culinaris sprouts on antioxidant biomarkers in CCl4-induced oxidative stress and hepatotoxicity in rats (mean ± SE), n = 8. (A) GSH: Reduced glutathione, (B) Malondialdehyde, (C) CAT: Catalase, and (D) SOD: Superoxide dismutase. G1–G5: Experimental groups see materials and methods, a,b,c,d: Bars not sharing similar letters are statistically different at p < 0.05.
Functional foods regulate diabetes through blood pressure regulation, activation of antioxidant enzymes, interaction with gut microbiota, suppressing of pro-inflammatory cytokine overproduction, and presenting antioxidative potential (23, 43, 44). A capable strategy, particularly when phenolics are incorporated, has been described (32) with superior antioxidant activity (45). The valuable phytochemical content and antioxidant activities of LSHE corresponded to Amarowicz et al. and Xu and Chang (9, 10). Indeed, biologically active components, such as phenolic acids and flavonoids, demonstrate antioxidant capacity by stopping lipid oxidation chain reactions in vitro and in vivo (9, 10). The phenolics' ability to scavenge and inhibit free radicals is caused by the phenolic hydroxyl groups located in polyphenols (46, 47). An efficient antioxidant component has been presented in various phenolic acids inhibiting hydrogen peroxide formation, superoxide anion, and hydroxyl radicals (48, 49). A direct correlation exists between abundant polyphenols concentration and their antioxidant function (9, 10, 23, 50).
Consumption of sprout extracts could help reduce cellular oxidation, as confirmed in the current study (23, 51). Interestingly during sprouting, phenolics and antioxidants increased (18). Zhang et al. (52) evaluated the total phenolic composition and contents, antioxidant activities (DPPH, FRAP, ORAC), and inhibitory properties of phenolic extracts from 20 Canadian lentil cultivars (L. culinaris) against α-glucosidase and pancreatic lipase (52). All extracts showed antioxidant and radical-scavenging properties as revealed by the total antioxidant activity (TAA) method, a β-carotene-linoleate model system, a reducing power assay, and the DPPH scavenging activity assay (9).
The increased number of phenolics in L. culinaris sprouts increased than its seeds with the progression of the sprouting period; results were agreed with Swieca and Gawlik-Dziki (18). Remarkably, present research noticed a considerable amount of identified phenolics higher than confirmed previously (9). However, Amarowicz et al. (9) indicated increased Rosmarinic acid, p-coumaric acid, and p-Hydroxy benzoic acid, which was consistently observed in the current study. It is worth mentioning that the phenolics profile in the cotyledon and seed coat of lentils vary and are affected by numerous factors (53, 54). L. culinaris seeds and sprouts show superior flavonoids content, similarly presented in 4 lentil varieties (55). Zhang et al. (52) supported our study regarding identified phenolics in L. culinaris seeds. In the present study, twenty-one phenolic compounds were identified, with the majority being flavonoids, including catechin/epicatechin glucosides, kaempeferol glycosides, and procyanidins. Amarowicz et al. (9). Twenty compounds (procyanidins, hydroxycinnamates, flavonols, gallates, dihydroflavonols, and dihydrochalcones) were detected and quantified in the crude extracts by HPLC-PAD and HPLC–ESI-MS procedures. The dominant phenolics in GL were epicatechin glucosides, catechin, procyanidin dimers, quercetin diglycoside, and trans-p-coumaric acid.
In addition to being beneficial against various metabolic illnesses, biologically active substances such as phenolic components have been defined as useful antioxidant substances, including hydroxyl radicals, hydrogen peroxide, and superoxide anion (56, 57). Interestingly, quantifying phenolics in L. culinaris sprouts indicated considerable numbers of phenolic acids and flavonoids, which increased significantly with increased sprouting time as confirmed (17, 18, 23) to process biological and nutritional benefits (20, 21).
Indeed, oxidative stress is thought to lead to many metabolic impairments, such as hypoglycemia (58–60). Our recent in vivo study indicated that LSHE stated substantial reductions in FBG in rats in a dose-dependent manner, as similarly indicated (13, 23, 51). These results strengthen our analysis, which confirms that LSHE possesses hypoglycemic effects because of rich polyphenols as effective antioxidants capable of modulating glucose levels (9, 15). Practically, the administration of LSHE was extremely beneficial in body weight recovery in a dose-dependent manner (57, 61).
Our results demonstrated the efficacy of LSHE as a rich source of antioxidants that alleviated liver malfunctions and elevated serum lipids among CCl4-intoxicated rat groups. It might be due to increasing and supporting rats' serum with bioactive dietary antioxidants (9, 15, 62). Administration of LSHE at 50 or 100 mg GAE Kg−1 improved the lipid profile in dose-dependent manure. The highly efficient therapy for improving the blood profile was LSHE with 100 mg GAE Kg−1 which presented no significance compared to normal rats (G1) or (G5). Obviously, administrating 50 mg Kg−1 Vit. E+Se showed the highest improvement rate (41.55%) in lipid profile because of high antioxidant content, as similarly observed (15, 62). Excitingly, the VLDL-c level was adjusted associatively in a dose-dependent manner with LSHE treatments. Our results align with Morise et al. (63), who explained that flaxseed oil rich in α-linolenic acid caused an elevated cholesterol secretion, causing depletion of the intrahepatic pool of cholesterol resulting in cholesterol synthesis increases. Additionally, α-linolenic acid reduced hepatic lipid accumulation by stimulating β-oxidation and suppressing fatty acid synthesis (12, 15, 64). Concerning TG, high antioxidants can be assigned to a decrease in the hepatic synthesis of fatty acids, decreasing the triacylglycerol concentration in the liver and reducing autoxidation, which attenuates VLDL-c accumulation (15, 65). Interestingly, 100 mg GAE Kg−1 of LSHE was more effective than 50 mg GAE Kg−1 of LSHE or even normal rats in attenuating the atherogenicity issue. Better results were obtained with 50 mg Kg−1 vit. E+Se, which did not significantly differ from 100 mg GAE Kg−1 of LSHE (15).
CCl4 insertion in a rat enlarged its liver by accumulating fats inside liver cells (66). Elevated serum enzymes activities levels (AST, ALT) signify cellular leak and loss of efficient integrity of cell membranes in the liver because of CCl4 intoxication. Administration of LSHE or Vit. E+Se significantly improved the levels of liver enzymes (ALT, AST) which consistently agreed (51, 62). Similarly, Saxena et al. (67) and Jung et al. (15) have confirmed the effects of the plant-based extract on elevated serum AST and ALT enzymes against oxidative stress induced by CCl4. T.Bili indicates that liver damage and CCl4 had a considerably higher level than in treated groups (Vit. E+Si and LSHE) or the NR group. Incidentally, LSHE was also efficient as Vit. E+Se presented. A current study has also indicated that in valuable amounts, LSHE contains rosmarinic acid, p-coumaric acid, vanillic acid, p-Hydroxy benzoic acid, and Benzoic acid, as well as high content of flavonoids such as kaempferol, myricetin, quercetin, resveratrol, Naringenin, and rutin. Antioxidative and anti-inflammatory efficiency in rats with hepatic damage has been proven with these compounds (68). Due to more polyphenols (20, 21), LSHE effectively attenuated oxidative stress complications. Therefore, LSHE may offer superior liver protection by blocking the development of liver fibrosis and suppressing TGF-β1 (68).
A correlation between nephrotoxicity and oxidative stress has been exhibited in many investigational models (69), and our study's results in kidney functions proved the same pattern as the organ function markers (61). Presented data clearly showed the recovery in all Kidney functions with orally administered LSHE for up to 6 weeks in dose-dependent manure. The elevated levels of Albumin, T. Protein, and Globulin and decreases in Urea, Creatinine, and BUN were highly meaningful in G4 and G5 compared to all other treated groups. An enhancement of kidney function parameters to the normal level in CCl4-injected rats fed Anastatica hierochuntica ethanolic and aqueous extracts (61) Samsum Ant Pachycondyla sennaarensis Venom (69) was demonstrated. Concerning the positive impact of LSHE on Kidneys' function attenuation, it was previously described that caffeic acid, carnosic acid, rosemarinic acid, and essential oil are accountable for the body's protection against free radical attack through occurred oxidative stress (6, 9, 15).
As established by the catabolite MDA indicator, tissue damage and lipid peroxidation are mediated by generated ROS (70). ROS enhances the risk of tissue injury and causes lipid peroxidation, as ascertained by the catabolite malondialdehyde marker (70). Earlier experiments indicated that CCl4 i.p. injection drastically decreased SOD, CAT, GPx, and GSH activities but considerably enhanced the MDA level (23, 71). Owning rich polyphenols content and AOA in LSHE, administrating 100 mg LSHE kg−1 was more efficient in attenuating autoxidation. The enzymatic antioxidant defense system such as SOD, CAT, and glutathione enzymes are essential scavengers of active radicals (68). LSHE attenuated GSH, CAT, SOD, and MDA levels close to the NR and equal to administrating Vit. E+Se. Previous studies have reported that consuming L. culinaris (6, 9, 15) increased serum antioxidant enzymes (51, 72). Concerning the present study's observations, administering LSHE orally increased the antioxidant enzymes SOD and CAT levels and decreased lipid peroxidation in CCl4-injected rats (57). The efficiency was significantly improved when LSHE was given at 100 mg Kg−1. As phenolics and antioxidants increase during sprouting (18), the consumption of sprouts extracts could help reduce cellular oxidation (23, 51). Also, LSHE attenuated the MDA and restored the total antioxidant defense in the CCl4-treated rats. This protective efficiency may be due to the potent antioxidative capacity of LSHE in the presence of high polyphenols, which effectively diminishes the complications related to oxidative stress (20, 21, 49, 73, 74).
This study investigated and confirmed the antioxidative potential of L. culinaris Medikus. The current study looked into a rat module's antioxidative, hepatoprotective and nephroprotective properties of L. culinaris sprouts extract. It is possible to conclude that the L. culinaris sprouts extract is high in phenolic compounds, particularly flavonoids with high antioxidant capacity. Phenolic analysis revealed that L. culinaris sprouts contained significant amounts of TF, which support its functional and therapeutic properties. Compared to vit. E+Se administration of LSHE at 50 and 100 mg Kg−1 protects rats against CCl4 oxidative stress. The protective efficacy could be attributed to the high concentration of phenolics (e.g., rosmarinic acid, p-hydroxybenzoic acid, vanillic acid, p-coumaric acid, benzoic acid, kaempferol, naringenin, myricetin, resveratrol, and quercetin) which may modulate glucose levels and reduce hepatotoxicity complications. In addition, biochemical examinations have confirmed this superior activity. As a result, the findings could aid in explaining the therapeutic efficacy of LSHE as a functional product. It encouraged us to recommend L. culinaris sprout production for combining oxidative stress, as well as being beneficial and profitable for controlling oxidative stress complications.
The original contributions presented in the study are included in the article/supplementary material, further inquiries can be directed to the corresponding author/s.
The animal study was reviewed and approved by the Committee of Research Ethics, Deanship of Scientific Research, Qassim University (No. 21-18-09 on Thursday, May 19, 2022), SA, governed by the Control and Supervision of Experiments on Animals (CPCSEA) Committee of the National Committee of BioEthics (NCBE), which implements regulations related to the ethics of research on living creatures.
SIA and HB: research design. ASA and RIA: experiment performance. SIA and HB: experiment operation assistance. SIA and RMA: main supervision and research leadership. RAA, RMG, and MA: draft manuscript writing. AAHA: validation and formal analysis. TA and HB: manuscript writing. All authors contributed to the article and approved the submitted version.
The authors extend their appreciation to the Deputyship for Research and Innovation, Ministry of Education, Saudi Arabia for funding this research work through the project number (QU-IF-2-1-2-26891). The authors also thank to Qassim University for technical support.
The authors declare that the research was conducted in the absence of any commercial or financial relationships that could be construed as a potential conflict of interest.
All claims expressed in this article are solely those of the authors and do not necessarily represent those of their affiliated organizations, or those of the publisher, the editors and the reviewers. Any product that may be evaluated in this article, or claim that may be made by its manufacturer, is not guaranteed or endorsed by the publisher.
ALT, Alanine aminotransferase; AOA, Antioxidant activity; AST, Aspartate aminotransferase; CAT, Catalase; CHO, Total cholesterol; DPPH, 1,1-diphenyl-2-picryl hydrazine; dw, Dry weight; GA, Gallic acid; GAE, Gallic acid equivalent; GSH, Reduced-glutathione; HDL-c, High-density lipoproteins; LDL-c, Low-density lipoproteins; LSHE, Lens culinaris Medikus sprouts hydroalcoholic extract; MDA, Malonaldehyde; QE, Quercetin equivalent; RSA, Radical scavenging activity; SE, Standard error; SOD, Superoxide dismutase; TBA, Thiobarbituric acid; TC, Total carotenoids; TE, Trolox equivalent; TF, Total flavonoids; TFL, Total flavonols; TG, Triglycerides; TPC, Total phenolic compounds; VLDL-c, Very Low-density lipoproteins.
1. Pizzino GA-OX, Irrera NA-O, Cucinotta MA-O, Pallio GA-O, Mannino F, Arcoraci VA-O, et al. Oxidative stress: harms and benefits for human health. Oxid Med Cell Longev. (2017) 5:6763. doi: 10.1155/2017/8416763
2. Romá-Mateo C, Aguado C, García-Giménez JL, Ibáñez-Cabellos JS, Seco-Cervera M, Pallardó FV, et al. Increased oxidative stress and impaired antioxidant response in Lafora disease. Mol Neurobiol. (2015) 51:932–46. doi: 10.1007/s12035-014-8747-0
3. Bonomini F, Tengattini S, Fabiano A, Bianchi R, Rezzani R. Atherosclerosis and oxidative stress. Histology Histopathology. (2008) 13:129–42. Available online at: http://hdl.handle.net/10201/29669
4. Jiménez-Fernández S, Gurpegui M, Díaz-Atienza F, Pérez-Costillas L, Gerstenberg M, Correll CU. Oxidative stress and antioxidant parameters in patients with major depressive disorder compared to healthy controls before and after antidepressant treatment: results from a meta-analysis. J Clin Psychiatry. (2015) 76:1658–67. doi: 10.4088/JCP.14r09179
5. Padhi EMT, Liu R, Hernandez M, Tsao R, Ramdath DD. Total polyphenol content, carotenoid, tocopherol and fatty acid composition of commonly consumed canadian pulses and their contribution to antioxidant activity. J Funct Foods. (2017) 38:602–11. doi: 10.1016/j.jff.2016.11.006
6. Faris M, Issa AY. Role of Lentils (Lens culinaris L) in human health and nutrition: a review. Med J Nutrition Metab. (2013) 6:3–16. doi: 10.1007/s12349-012-0109-8
7. Ganesan K, Xu B. Polyphenol-rich lentils and their health promoting effects. Int J Mol Sci. (2017) 18:2390. doi: 10.3390/ijms18112390
8. Kahraman A. Nutritional components and amino acids in lentil varieties. Selcuk J Agr Food Sci. (2016) 30:34–8. Available online at: https://dergipark.org.tr/en/pub/selcukjafsci/issue/37961/438425
9. Amarowicz R, Estrella I, Hernández T, Robredo S, Troszyńska A, Kosińska A, et al. Free radical-scavenging capacity, antioxidant activity, and phenolic composition of green lentil (Lens culinaris). Food Chem. (2010) 121:705–11. doi: 10.1016/j.foodchem.2010.01.009
10. Xu B, Chang SKC. Phenolic substance characterization and chemical and cell-based antioxidant activities of 11 lentils grown in the Northern United States. J Agric Food Chem. (2010) 58:1509–17. doi: 10.1021/jf903532y
11. Hall C, Hillen C, Garden Robinson J. Composition, nutritional value, and health benefits of pulses. Cereal Chem. (2017) 94:11–31. doi: 10.1094/CCHEM-03-16-0069-FI
12. Goudarzi M, Kalantari H, Kalantar M. Ameliorative effects of red lentil extract on sodium arsenite induced oxidative stress in experimental rats. Toxicol Lett. (2016) 258:S285–S. doi: 10.1016/j.toxlet.2016.06.1994
13. Peñas E, Limón RI, Martínez-Villaluenga C, Restani P, Pihlanto A, Frias J. Impact of elicitation on antioxidant and potential antihypertensive properties of lentil sprouts. Plant Foods Hum Nutr. (2015) 70:401–7. doi: 10.1007/s11130-015-0508-3
14. Lee SH, Lee SO. Polyphenol contents and antioxidant activities of lentil extracts from different cultivars. J Korean Soc Food Sci Nutr. (2016) 45:973–9. doi: 10.3746/jkfn.2016.45.7.973
15. Jung Y-S, Lee S-H, Chun SY, Kim DH, Jang BI, Han M-H, et al. In vitro and in vivo protective effects of lentil (Lens culinaris) extract against oxidative stress-induced hepatotoxicity. Molecules. (2022) 27:59. doi: 10.3390/molecules27010059
16. Moreno DA, Pérez-Balibrea S, García-Viguera C. Phytochemical quality and bioactivity of edible sprouts. Nat Prod Commun. (2006) 1:1934578X0600101120. doi: 10.1177/1934578X0600101120
17. Swieca M, Gawlik-Dziki U. Effects of sprouting and postharvest storage under cool temperature conditions on starch content and antioxidant capacity of green pea, lentil and young mung bean sprouts. Food Chem. (2015) 185:99–105. doi: 10.1016/j.foodchem.2015.03.108
18. Swieca M, Gawlik-Dziki U, Kowalczyk D, Złotek U. Impact of germination time and type of illumination on the antioxidant compounds and antioxidant capacity of Lens culinaris sprouts. Sci Hortic. (2012) 140:87–95. doi: 10.1016/j.scienta.2012.04.005
19. Montemurro M, Pontonio E, Gobbetti M, Rizzello CG. Investigation of the nutritional, functional and technological effects of the sourdough fermentation of sprouted flours. Int J Food Microbiol. (2019) 302:47–58. doi: 10.1016/j.ijfoodmicro.2018.08.005
20. Peñas E, Martínez-Villaluenga C. Advances in production, properties and applications of sprouted seeds. Foods. (2020) 9:790. doi: 10.3390/foods9060790
21. Miyahira RF. Lopes JdO, Antunes AEC. The use of sprouts to improve the nutritional value of food products: a brief review. Plant Foods Hum Nutr. (2021) 76:143–52. doi: 10.1007/s11130-021-00888-6
22. Barakat H, Spielvogel A, Hassan M, El-Desouky A, El-Mansy H, Rath F, et al. The antifungal protein AFP from Aspergillus giganteus prevents secondary growth of different Fusarium species on barley. Appl Microbiol Biotechnol. (2010) 87:617–24. doi: 10.1007/s00253-010-2508-4
23. Al-Qabba MM, El-Mowafy MA, Althwab SA, Alfheeaid HA, Aljutaily T, Barakat H. Phenolic profile, antioxidant activity, and ameliorating efficacy of Chenopodium quinoa sprouts against CCl4-Induced oxidative stress in rats. Nutrients. (2020) 12:2904. doi: 10.3390/nu12102904
24. Hernández-Saavedra D, Pérez-Ramírez IF, Ramos-Gómez M, Mendoza-Díaz S, Loarca-Piña G, Reynoso-Camacho R. Phytochemical characterization and effect of Calendula officinalis, Hypericum perforatum, and Salvia officinalis infusions on obesity-associated cardiovascular risk. Med Chem Res. (2016) 25:163–72. doi: 10.1007/s00044-015-1454-1
25. Yawadio Nsimba R, Kikuzaki H, Konishi Y. Antioxidant activity of various extracts and fractions of Chenopodium quinoa and Amaranthus spp. seeds. Food Chem. (2008) 106:760–6. doi: 10.1016/j.foodchem.2007.06.004
26. Yuan GF, Sun J, Yuan Q, Wang QM. Effects of different cooking methods on health-promoting compounds of broccoli. J Zhejiang University-SCIENCE B. (2009) 10:580–8. doi: 10.1631/jzus.B0920051
27. Mohdaly AAA, Hassanien MFR, Mahmoud A, Sarhan MA, Smetanska I. Phenolics extracted from potato, sugar beet, and sesame processing by-products. Int J Food Prop. (2012) 16:1148–68. doi: 10.1080/10942912.2011.578318
28. Silva CR, Simoni JA, Collins CH, Volpe PL. Ascorbic acid as a standard for iodometric titrations. An analytical experiment for general chemistry. J Chem Edu. (1999) 76:1421. doi: 10.1021/ed076p1421
29. Barakat H, Rohn S. Effect of different cooking methods on bioactive compounds in vegetarian, broccoli-based bars. J Funct Foods. (2014) 11:407–16. doi: 10.1016/j.jff.2014.10.009
30. Kim K-H, Tsao R, Yang R, Cui SW. Phenolic acid profiles and antioxidant activities of wheat bran extracts and the effect of hydrolysis conditions. Food Chem. (2006) 95:466–73. doi: 10.1016/j.foodchem.2005.01.032
31. Reeves PG, Nielsen FH, Fahey GC Jr. AIN-93 Purified diets for laboratory rodents: Final report of the american institute of nutrition ad hoc writing committee on the reformulation of the AIN-76a rodent diet. J Nutr. (1993) 123:1939–51. doi: 10.1093/jn/123.11.1939
32. Barakat H, Almundarij TI. Phenolic compounds and hepatoprotective potential of Anastatica hierochuntica ethanolic and aqueous extracts against CCl4-induced hepatotoxicity in rats. J Tradit Chin Med. (2020) 40:947. doi: 10.3390/nu13092973
33. Asuku O, Atawodi SE, Onyike E. Antioxidant, hepatoprotective, and ameliorative effects of methanolic extract of leaves of Grewia mollis Juss. on carbon tetrachloride–treated albino rats. J Med Food. (2012) 15:83–8. doi: 10.1089/jmf.2010.0285
34. El-Desoky G, Abdelreheem M, Abdulaziz AO, ALOthman Z, Mahmoud M, Yusuf K. Potential hepatoprotective effects of vitamin E and selenium on hepatotoxicity induced by malathion in rats. Af J Pharmacy Pharmacol. (2012) 6:806–13. doi: 10.5897/AJPP11.628
35. Moradabadi L, Montasser Kouhsari S, Fehresti Sani M. Hypoglycemic effects of three medicinal plants in experimental diabetes: inhibition of rat intestinal α-glucosidase and enhanced pancreatic insulin and cardiac Glut-4 mRNAs expression. Iran J Pharm Res. (2013) 12:387–97. Available online at: https://www.ncbi.nlm.nih.gov/pmc/articles/PMC3813273/
36. Nwagha U, Ikekpeazu E, Ejezie F, Neboh E, Maduka I. Atherogenic index of plasma as useful predictor of cardiovascular risk among postmenopausal women in Enugu, Nigeria. Afr Health Sci. (2010) 10:248–52. Available online at: https://www.ajol.info/index.php/ahs/article/view/62873
37. Friedewald WT, Levy RI, Fredrickson DS. Estimation of the concentration of low-density lipoprotein cholesterol in plasma, without use of the preparative ultracentrifuge. Clin Chem. (1972) 18:499–502. doi: 10.1093/clinchem/18.6.499
38. Beutler E. Improved method for the determination of blood glutathione. J Lab Clin Med. (1963) 61:882–8.
39. Ohkawa H, Ohishi N, Yagi K. Assay for lipid peroxides in animal tissues by thiobarbituric acid reaction. Anal Biochem. (1979) 95:351–8. doi: 10.1016/0003-2697(79)90738-3
40. Giannopolitis CN, Ries SK. Superoxide dismutases: I. Occurrence in higher plants. Plant Physiol. (1977) 59:309–14. doi: 10.1104/pp.59.2.309
42. Steel RG. Pinciples and Procedures of Statistics A Biometrical Approach. 3rd ed. Boston: McGraw-Hill. (1997).
43. Mirmiran P, Bahadoran Z, Azizi F. Functional foods-based diet as a novel dietary approach for management of type 2 diabetes and its complications: a review. World J Diab. (2014) 5:267–81. doi: 10.4239/wjd.v5.i3.267
44. Alharbi YM, Sakr SS, Albarrak SM, Almundarij TI, Barakat H, Hassan MFY. Antioxidative, antidiabetic, and hypolipidemic properties of probiotic-enriched fermented camel milk combined with Salvia officinalis leaves hydroalcoholic extract in streptozotocin-induced diabetes in rats. Antioxidants. (2022) 11:668. doi: 10.3390/antiox11040668
45. Khalifa I, Barakat H, El-Mansy HA, Soliman S. Optimizing bioactive substances extraction procedures from Guava, olive and potato processing wastes and evaluating their antioxidant capacity. J Food Chem Nanotechnol. (2016) 2:170–7. doi: 10.17756/jfcn.2016-027
46. Tosun M, Ercisli S, Sengul M, Ozer H, Polat T, Ozturk E. Antioxidant properties and total phenolic content of eight Salvia species from Turkey. Biol Res. (2009) 42:175–81. doi: 10.4067/S0716-97602009000200005
47. Ollanketo M, Peltoketo A, Hartonen K, Hiltunen R, Riekkola ML. Extraction of sage (Salvia officinalis L) by pressurized hot water and conventional methods: antioxidant activity of the extracts. Eur Food Res Technol. (2002) 215:158–63. doi: 10.1007/s00217-002-0545-7
48. Farhat MB, Chaouch-Hamada R, Sotomayor JA, Landoulsi A, Jordán MJ. Antioxidant potential of Salvia officinalis L. residues as affected by the harvesting time. Ind Crops Prod. (2014) 54:78–85. doi: 10.1016/j.indcrop.2014.01.001
49. Roby MHH, Sarhan MA, Selim KAH, Khalel KI. Evaluation of antioxidant activity, total phenols and phenolic compounds in thyme (Thymus vulgaris L), sage (Salvia officinalis L), and marjoram (Origanum majorana L) extracts. Ind Crops Prod. (2013) 43:827–31. doi: 10.1016/j.indcrop.2012.08.029
50. Ebrahimzadeh MA, Nabavi SM, Nabavi SF, Bahramian F, Bekhradnia AR. Antioxidant and free radical scavenging activity of H. officinalis L var angustifolius, V odorata, B hyrcana and C speciosum. Pak J Pharm Sci. (2010) 23:29–34.
51. Jia L, Wang T, Sun Y, Zhang M, Tian J, Chen H, et al. Protective effect of selenium-enriched red radish sprouts on carbon tetrachloride-induced liver injury in mice. J Food Sci. (2019) 84:3027–36. doi: 10.1111/1750-3841.14727
52. Zhang B, Deng Z, Ramdath DD, Tang Y, Chen PX, Liu R, et al. Phenolic profiles of 20 Canadian lentil cultivars and their contribution to antioxidant activity and inhibitory effects on α-glucosidase and pancreatic lipase. Food Chem. (2015) 172:862–72. doi: 10.1016/j.foodchem.2014.09.144
53. Dueñas M, Hernández T, Estrella I. Phenolic composition of the cotyledon and the seed coat of lentils (Lens culinaris L). Eur Food Res Technol. (2002) 215:478–83. doi: 10.1007/s00217-002-0603-1
54. Singh Ak, Rehal J, Kaur A, Jyot G. Enhancement of attributes of cereals by germination and fermentation: a review. Crit Rev Food Sci Nutr. (2015) 55:1575–89. doi: 10.1080/10408398.2012.706661
55. López-Amorós ML, Hernández T, Estrella I. Effect of germination on legume phenolic compounds and their antioxidant activity. J Food Compos Anal. (2006) 19:277–83. doi: 10.1016/j.jfca.2004.06.012
56. Eva Y, Annisa A, Andrafikar. Effectiveness of jicama probiotic yoghurt (Pachyrhizus erosus) on blood glucose in diabetic mice. KnE Life Sci. (2019)4:250–61.
57. Hasanein P, Felehgari Z, Emamjomeh A. Preventive effects of Salvia officinalis L. against learning and memory deficit induced by diabetes in rats: Possible hypoglycaemic and antioxidant mechanisms. Neurosci Lett. (2016) 622:72–7. doi: 10.1016/j.neulet.2016.04.045
58. Prevention. CfDCa. Diabetes Report Card 2019. Atlanta, GA: Centers for Disease Control and Prevention, US Dept of Health and Human Services (2020).
59. Zafar M, Naqvi SN-u-H. Effects of STZ-Induced diabetes on the relative weights of kidney, liver and pancreas in albino rats: a comparative study. Int J Morphol. (2010) 28:19. doi: 10.4067/S0717-95022010000100019
60. Maritim AC, Sanders RA, Watkins JB III. Diabetes, oxidative stress, and antioxidants: a review. J Biochem Mol Toxicol. (2003) 17:24–38. doi: 10.1002/jbt.10058
61. Almundarij TI, Alharbi YM, Abdel-Rahman HA, Barakat H. Antioxidant activity, phenolic profile, and nephroprotective potential of Anastatica hierochuntica ethanolic and aqueous extracts against ccl4-induced nephrotoxicity in rats. Nutrients. (2021) 13:2973.
62. Ismail RS, El-Megeid AA, Abdel-Moemin AR. Carbon tetrachloride-induced liver disease in rats: the potential effect of supplement oils with vitamin E and C on the nutritional status. Ger Med Sci. (2009) 7:Doc05. doi: 10.3205/000064
63. Morise A, Sérougne C, Gripois D, Blouquit M-F, Lutton C, Hermier D. Effects of dietary alpha linolenic acid on cholesterol metabolism in male and female hamsters of the LPN strain. J Nutr Biochem. (2004) 15:51–61. doi: 10.1016/j.jnutbio.2003.10.002
64. Fan JG, Zhong L, Xu ZJ, Tia LY, Ding XD Li MS, et al. Effects of low-calorie diet on steatohepatitis in rats with obesity and hyperlipidemia. World J Gastroenterol. (2003) 9:2045–9. doi: 10.3748/wjg.v9.i9.2045
65. Park Y, Harris WS. Omega-3 fatty acid supplementation accelerates chylomicron triglyceride clearance. J Lipid Res. (2003) 44:455–63. doi: 10.1194/jlr.M200282-JLR200
66. De Bleserc PJ, Jannes P, Van Buul-Offers SC, Hoogerbrugge CM, Van Schravendijk CFH, Niki T, et al. Insulinlike growth factor—Ii/Mannose 6-Phosphate receptor is expressed on CCl4-exposed rat fat-storing cells and facilitates activation of latent transforming growth factor-? in cocultures with sinusoidal endothelial cells. Hepatology. (1995) 21=:1429-37. doi: 10.1002/hep.1840210529
67. Saxena S, Shahani L, Bhatnagar P. Hepatoprotective effect of Chenopodium quinoa seed against CCL4-induced liver toxicity in Swiss albino male mice. Asian J Pharmaceutical Clin Res. (2017) 10:273–6. doi: 10.22159/ajpcr.2017.v10i11.20918
68. Lin T-A, Ke B-J, Cheng C-S, Wang J-J, Wei B-L, Lee C-L. Red quinoa bran extracts protects against carbon tetrachloride-induced liver injury and fibrosis in mice via activation of antioxidative enzyme systems and blocking TGF-β1 pathway. Nutrients. (2019) 11:395. doi: 10.3390/nu11020395
69. Ebaid H, Al-Tamimi J, Hassan I, Alhazza I, Al-Khalifa M. Antioxidant bioactivity of samsum ant Pachycondyla sennaarensis venom protects against CCl4-induced nephrotoxicity in mice. Oxid Med Cell Longev. (2014) 2014:763061. doi: 10.1155/2014/763061
70. Ng S-C, Anderson A, Coker J, Ondrus M. Characterization of lipid oxidation products in quinoa (Chenopodium quinoa). Food Chem. (2007) 101:185–92. doi: 10.1016/j.foodchem.2006.01.016
71. Dai N, Zou Y, Zhu L, Wang H-F, Dai M-G. Antioxidant properties of proanthocyanidins attenuate carbon tetrachloride (CCl4)–induced steatosis and liver injury in rats via CYP2E1 regulation. J Med Food. (2014) 17:663–9. doi: 10.1089/jmf.2013.2834
72. Watanabe M, Ayugase J. Effects of buckwheat sprouts on plasma and hepatic parameters in type 2 diabetic db/db mice. J Food Sci. (2010) 75:H294–H9. doi: 10.1111/j.1750-3841.2010.01853.x
73. Walch SG, Tinzoh LN, Zimmermann BF, Stuhlinger W, Lachenmeier DW. Antioxidant capacity and polyphenolic composition as quality indicators for aqueous infusions of salvia officinalis l (sage tea). Front Pharmacol. (2011) 2:79. doi: 10.3389/fphar.2011.00079
Keywords: Lens culinaris, sprouts, antioxidative potential, hepatoprotection, nephroprotection
Citation: Barakat H, Alshimali SI, Almutairi AS, Alkhurayji RI, Almutiri SM, Aljutaily T, Algheshairy RM, Alhomaid RM, Aljalis RA, Alkhidhr MF and Abdellatif AAH (2022) Antioxidative potential and ameliorative effects of green lentil (Lens culinaris M.) sprouts against CCl4-induced oxidative stress in rats. Front. Nutr. 9:1029793. doi: 10.3389/fnut.2022.1029793
Received: 27 August 2022; Accepted: 27 October 2022;
Published: 11 November 2022.
Edited by:
Piyameth Dilokthornsakul, Chiang Mai University, ThailandReviewed by:
Fatma Rahmouni, University of Sfax, TunisiaCopyright © 2022 Barakat, Alshimali, Almutairi, Alkhurayji, Almutiri, Aljutaily, Algheshairy, Alhomaid, Aljalis, Alkhidhr and Abdellatif. This is an open-access article distributed under the terms of the Creative Commons Attribution License (CC BY). The use, distribution or reproduction in other forums is permitted, provided the original author(s) and the copyright owner(s) are credited and that the original publication in this journal is cited, in accordance with accepted academic practice. No use, distribution or reproduction is permitted which does not comply with these terms.
*Correspondence: Hassan Barakat, aGFhLm1vaGFtZWRAcXUuZWR1LnNh
Disclaimer: All claims expressed in this article are solely those of the authors and do not necessarily represent those of their affiliated organizations, or those of the publisher, the editors and the reviewers. Any product that may be evaluated in this article or claim that may be made by its manufacturer is not guaranteed or endorsed by the publisher.
Research integrity at Frontiers
Learn more about the work of our research integrity team to safeguard the quality of each article we publish.