- 1Department of Chronic Diseases, Metabolism and Aging, Translational Research Center for Gastrointestinal Disorders, KU Leuven, Leuven, Belgium
- 2Laboratory of Lifestock Physiology, Department of Animal and Human Health, KU Leuven, Leuven, Belgium
- 3Nutrition & Obesity Unit, Clinical and Experimental Endocrinology, Department of Chronic Diseases, Metabolism and Aging, KU Leuven, Leuven, Belgium
- 4Department of Endocrinology, University Hospitals Leuven, Leuven, Belgium
- 5Leuven Food Science and Nutrition Research Centre, KU Leuven, Leuven, Belgium
Introduction: Bariatric surgery, currently the most effective treatment for morbidly obese patients, may induce macronutrient malabsorption depending on the type of procedure. Macronutrient malabsorption affects the supply of substrates to the colon, subsequent microbial fermentation and possibly colonic health.
Methods: Using isotope technology, we quantified the extent of macronutrient and bile acid malabsorption and its impact on colonic protein fermentation in patients after Roux-en-Y gastric bypass (RYGB) and sleeve gastrectomy (SG) and in controls. Participants consumed a single test meal (day 0) that contained intrinsically labeled (13C, 15N, and 2H) egg protein for quantification of protein digestion, malabsorption and fermentation, respectively, together with a transit marker and a marker for bile acid malabsorption. They collected breath samples up to 6 h and all urine and stool for 48 and 72 h, respectively. Food intake was registered from day –3 to day 2.
Results: Malabsorption of fat, protein and carbohydrates differed between groups (p = 0.040; p = 0.046; and p = 0.003, respectively) and was slightly higher in RYGB but not in SG patients compared to controls. Protein fermentation was increased in both RYGB and SG patients compared to controls (p = 0.001) and was negatively correlated to 2H-recovery as a marker of transit (ρ = −0.47, p = 0.013).
Conclusion: The limited macronutrient malabsorption likely does not affect the nutritional status of the patient. However, the higher protein fermentation may affect colonic health and warrants further investigation.
Introduction
Bariatric surgery (BS) is currently the most efficient treatment to induce long-term weight loss and improve health in patients with morbid obesity (1). Worldwide, laparoscopic Roux-en-Y gastric bypass (RYGB) and sleeve gastrectomy (SG) are the most common types of BS (2). After RYGB, the stomach is converted to a small gastric pouch and a large part of the small intestine is bypassed. SG only involves a vertical resection of the stomach along the greater curvature, leaving the intestines intact (3).
Originally, these procedures were intended to induce weight loss by restricting caloric intake (small gastric volume) and, in the case of RYGB, by reducing nutrient absorption in the small intestine (reduced absorptive surface) (4). Nowadays, several studies have associated the increased secretion of the gut hormones GLP-1, CCK, and PYY, altered bile acid signaling and microbiota composition to the weight loss and the metabolic benefits seen after RYBG and SG (5, 6).
Even if malabsorption is not a major driver for weight loss after BS, it remains essential to quantify the extent of macronutrient malabsorption for several reasons. First, knowledge on the extent of macronutrient malabsorption may improve dietary guidelines for patients after surgery and aid in preventing undernutrition (7, 8). Secondly, food components that are not digested and absorbed in the small intestine, reach the colon where they serve as substrate for the residing microbiota. Changes in substrate availability may drive the alterations in microbiota composition observed after BS resulting in altered interactions with bile acids and farnesoid X receptor (FXR) signaling. Activation of the FXR regulates hepatic bile acid metabolism, glucose and lipid metabolism (9). Thirdly, changes in supply of substrates to the colonic microbiota also affect the type and amount of metabolites produced by bacterial fermentation. Those metabolites are in close contact with the intestinal cells and play a role in colonic health. Short chain fatty acids are the main metabolites from carbohydrate fermentation and are considered beneficial. In contrast, some protein fermentation metabolites such as p-cresol, ammonia and hydrogen sulfide may harm colonic cells by disrupting the epithelial barrier and inducing DNA damage (10, 11).
In this study, we applied isotope technology to quantify macronutrient and bile acid malabsorption in weight-stable RYGB and SG patients (6–18 months after surgery), and in a control group. Furthermore, we evaluated the impact of surgery on colonic protein fermentation. As the small intestinal anatomy is rearranged after RYGB but not SG, we hypothesized that macronutrient malabsorption is affected to a greater extent after RYGB than after SG.
Materials and methods
Subjects
Roux-en-Y gastric bypass and SG patients that had surgery 6–18 months ago were recruited at the Obesity Clinic of the University Hospital Leuven (Leuven, Belgium). Unoperated subjects with a BMI between 18 and 30 kg/m2 were recruited as controls (CTR). Exclusion criteria were abdominal surgery (except appendectomy, cholecystectomy, and bariatric surgery) and kidney, liver, lung and gastro-intestinal disease. Participants were free from antibiotics and pre-and probiotics for 1 month and from antidiarrheal drugs and laxatives for 2 weeks prior to the start of the study. Subjects on a specific diet, including a vegan, vegetarian, lactose-or gluten-free diet, pregnant or breastfeeding women and subjects that participated in a clinical study with radiation exposure in the past year were excluded. The study was approved by the Ethics Committee of UZ/KU Leuven and registered at ClinicalTrials.Gov (clinical trial number: NCT04345328). All subjects signed written informed consent.
Study design
Participants performed a single test day (day 0) but started to complete a dietary record at home at day −3. The study is schematically presented in Figure 1 and the details of all procedures are described in the subsequent paragraphs. On day −1, they were asked to avoid fiber-rich food (only white bread, white pasta, and white rice, maximal one piece of fruit and no cabbage, legumes, or onions) and alcohol. On the morning of day 0, they came to the laboratory in fasted state for the test day. After collection of baseline breath samples, participants consumed an intrinsically stable isotope labeled pancake test meal with a glass of water, a gelatine capsule containing 14C-glycocholic acid (185 kBq, PerkinElmer, Shelton, CT, USA) to assess bile acid malabsorption and a capsule with 3H-polyethylene glycol (3H-PEG, 185 kBq, PerkinElmer) as a marker of total transit. Additional breath samples for analysis of 13C, 14C, and H2 were collected at regular time points up to 6 h after the test meal. Upon completion of the breath sampling, participants left the laboratory and could have lunch and dinner ad libitum but kept recording food intake. Furthermore, they collected all stools for the next 72 h and stored them immediately at −20°C. Urine was collected for 48 h in dedicated recipients to which 500 mg neomycin was added to prevent bacterial growth. On day 3, all samples and diaries were returned to the lab. The fecal collection was weighed, homogenized and lyophilized. Urine samples were weighed, aliquoted and stored at −20°C until analysis.
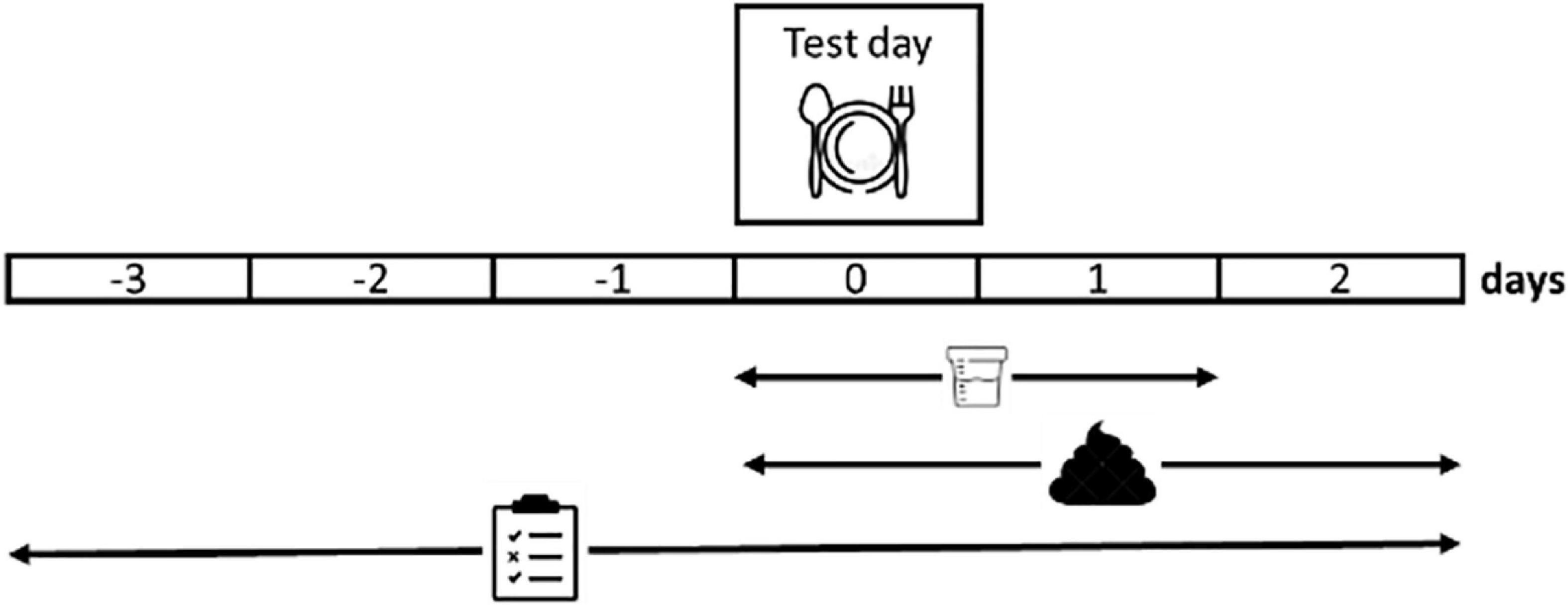
Figure 1. Schematic overview of the study design. Participants self-report their dietary intake from 3 days before a test day (day 0) up to 2 days after the test day. The test day is performed in the laboratory and consists of the consumption of an isotope labeled standard meal and the collection of breath samples (6 h), urine and feces. Urine collection is continued up to 48 h and feces collection up to 72 h after the standard meal.
Production of stable isotope labeled eggs
Eggs were intrinsically labeled with 13C, 15N, and 2H by feeding 2 laying hens (Hisex white, body weight of about 2 kg) at peak egg production with standard feed (Farm 3 Mash, HobbyFirst, Schoten, Belgium) supplemented (3 g/kg) with L-[1-13C]-leucine, L-[15N]-leucine and L-[ring-2H5]-phenylalanine (Euriso-top, Saint-Aubin, France; >98 mole%). The hens incorporated these amino acids into their egg proteins. Dietary L-[ring-2H5]-phenylalanine is partly hydroxylated by the hen’s metabolism and incorporated into the egg protein as both L-[ring-2H4]-tyrosine and L-[ring-2H5]-phenylalanine. From day 14, the eggs were collected daily and lyophilized. The 15N- and 13C-abundance was measured using an elemental analyzer coupled to an isotope ratio mass spectrometry (IRMS) (ANCA-2020, Europe Scientific, Crew, UK). The 2H-abundance of the eggs was assessed with gas chromatography mass spectrometry (GC-MS, Trace GC 1300 and DSQ II XL, Thermo Electron Corporation, Wathham, MA, USA), after hydrolysis of the egg proteins and derivatization of the resulting amino acids, as described previously (12). The pooled eggs had a 13C-abundance of 1.18 atom%, a 15N-abundance of 1.64 atom% and a L-[ring-2H4]-tyrosine abundance of 3.78 mole%.
Pancake test meal
The pancake test meal was prepared by adding 100 mL of water to a mix of 24.7 g of lyophilized labeled egg, 3.75 g of unlabeled lyophilized egg white, 17 g of wheat flour, 7 g of sugar, 3 g of milk powder and baking the resulting dough with 6 g of butter. The pancake was consumed with an additional 5 g of sugar. The total caloric content of the meal was 326 kcal and consisted of 18 g of protein, 16 g of fat and 27 g of carbohydrates (13).
Self-reported dietary intake
Participants digitally registered their food intake for 6 days using the mobile application or website of MyFitnessPal (MyFP). To ensure accurate registration, we provided a manual on how to use MyFP. Participants were asked to weigh all consumed food and drinks on a kitchen scale. Upon delivery, the food diaries were checked for irregularities and corrected if required. Macronutrient composition was calculated manually using the portion size and the nutritional information from the label of branded items and from the Belgian Composition Data Table for generic items. Results were expressed in absolute amounts (g/day) or as% of energy intake (EN%) using 4 kcal/g for protein and carbohydrate, 9 kcal/g for fat and 2 kcal/g for dietary fiber.
Protein digestion
Protein digestion was estimated from the rate of 13CO2 appearance in breath after ingestion of intrinsically labeled proteins (14, 15). Breath samples for quantification of 13C were collected by blowing through a straw in an Exetainer® (Labco Ltd., Ceredigion, UK) and the isotopic abundance of CO2 was measured using IRMS (ABCA, Sercon, Crewe, UK). CO2-production was assumed to be 300 mmol per m2 body surface area per hour, with body surface area being calculated by the weight-height formula of Haycock et al. (16). Results were expressed as% of administered dose of 13C recovered per hour (13C-recovery/h) and as cumulative% of administered dose of 13C over 6 h (6 h 13C-recovery).
Bile acid malabsorption
A 14C-glycocholic acid breath test was used to assess bile acid malabsorption (17). Breath samples for analysis of 14C were collected by blowing through a pipette into a vial containing 2 mmol of hyamine hydroxide until discoloration of the thymolphtalein color indicator, which corresponds to the capture of 2 mmol CO2. The amount of 14CO2 was measured by β-scintillation counting (PerkinElmer, Shelton, CT, USA) after addition of 10 ml Emulsifier-Safe™ (PerkinElmer). Results were expressed as disintegrations per minute (dpm) and converted to% of administered dose of 14C/h (14C-recovery/h). Results were classified as positive or negative for bile acid malabsorption based on visual inspection of the curves.
Carbohydrate malabsorption
As hydrogen is not produced by mammalian enzymes but only upon bacterial metabolism of carbohydrates, excretion of hydrogen (H2) in breath was measured as an indication of malabsorption of the carbohydrates (starch and sugar) in the pancake test meal (18). Breath H2 was measured using GC with thermal conductivity detection (19) and used to calculate the positive incremental area under the curve (iAUC).
Fat malabsorption
Fat malabsorption was expressed as% fat excretion ([fat excretion/fat intake] × 100). Fat intake was calculated from the food diary analysis and was expressed as g fat/day. Excretion of fat in feces was quantified by extracting 200 mg lyophilized feces in petroleum ether, using a continuous Soxhlet extraction (20). The petroleum ether extract was dried and the amount of fat measured gravimetrically. Results were expressed as g fat excreted per day.
3H-recovery
The tritium (3H) content in lyophilized fecal samples was measured by liquid scintillation counting after oxidation to [3H]-H20 (Sample Oxidizer, model 307, PerkinElmer). Results of 3H-recovery were expressed as% of the administered 3H dose recovered over 72 h. This 72-h 3H-recovery was used as a measure of total transit (21).
Protein malabsorption
Protein malabsorption was calculated as the% difference between 15N intake and fecal 15N excretion. Intake of 15N was calculated from the measured enrichment and total N content of the labeled egg. For 15N excretion, total N content and 15N-abundance of lyophilized fecal samples were determined (22). Total amount of excreted 15N was calculated from the amount of 15N in the fecal sample, the% of dry weight and the total fecal output. The 15N-recovery in feces over 72 h was corrected for total transit by dividing the% 15N recovery by the% 3H recovery.
Protein fermentation
Urinary recovery of p-[ring-2H4]cresol was measured to estimate protein fermentation. After thawing, urine was analyzed for p-[ring-2H4]-cresol content using GC-MS, as described previously (23). Results were expressed as the% of administered dose of L-ring[2H4]tyrosine.
Statistical analysis
Sample size calculation was performed using protein malabsorption as the outcome because we considered protein malabsorption as an important factor impacting colonic fermentation and gut health. Based on previous data from our lab that indicate a 5.7% protein malabsorption in physiological conditions (24) and an increase of 20% protein malabsorption reported after RYGB (25), we calculated that 10 subjects in each group would be sufficient to provide a 90% chance for detecting such difference at the 5% level of significance. Unfortunately, we managed to only include 8 SG patients that fulfilled all criteria.
The residuals of all variables were tested for normality and equal variance using a Shapiro-Wilk and Levene’s test, respectively. In case normality or equal variance were not obtained after transformation, Kruskall–Wallis or Welch’s ANOVA, respectively, was applied. For post-hoc pairwise comparisons, the Tukey–Kramer test was used. Continuous variables were compared across the 3 groups, using a one-way ANOVA. Whereas a Fisher’s Exact test was applied for categorical variables. Using ANCOVA, group comparison of protein fermentation was adjusted for 72-h 3H-recovery, protein malabsorption and fiber intake, whereas 3H-recovery was adjusted for fiber intake. Fat malabsorption was adjusted for bile acid malabsorption status. Additionally, Pearson or Spearman correlations were applied, depending on the normality of the data. Data were analyzed using SAS 9.4 (SAS Institute, Cary, NC, USA). Significance level was set at a p-value < 0.05.
Results
Study population
Ten RYGB patients, 8 SG patients, and 10 CTR were included in the study. From the 113 subjects screened, 85 did not meet the inclusion criteria or did not wish to participate. One SG patient had insufficient CO2 in the breath samples for reliable 13C-abundance analysis and was excluded from the protein digestion analysis.
Age, weight, and BMI did not differ across the three groups (Table 1). Time after surgery was similar for both surgery groups and the proportion of men and women did not differ across the groups.
Dietary intake
Total energy intake was lower in the surgery groups than in the CTR group (Table 2). RYGB patients ate significantly less carbohydrates and fat but similar amounts of protein compared to CTR, resulting in a higher EN% from protein in the RYGB than in the CTR group (p = 0.029). EN% from carbohydrates and fats was not different between groups. Fiber intake in SG but not RYGB patients was lower than in CTR (p = 0.033 and p = 0.622, respectively).
3H-recovery
3H-recovery was different between groups (ANOVA, p = 0.005). RYGB patients had a lower 3H-recovery than CTR, reflecting a slower transit (p = 0.005, Figure 2), whereas SG did not differ from RYGB (p = 0.665) and had a borderline non-significant difference with CTR (p = 0.054). Surprisingly, fiber intake did not correlate with total transit (ρ = 0.19, p = 0.325). After correction for fiber intake, the group effect on 72-h 3H-recovery remained significant (p = 0.012).
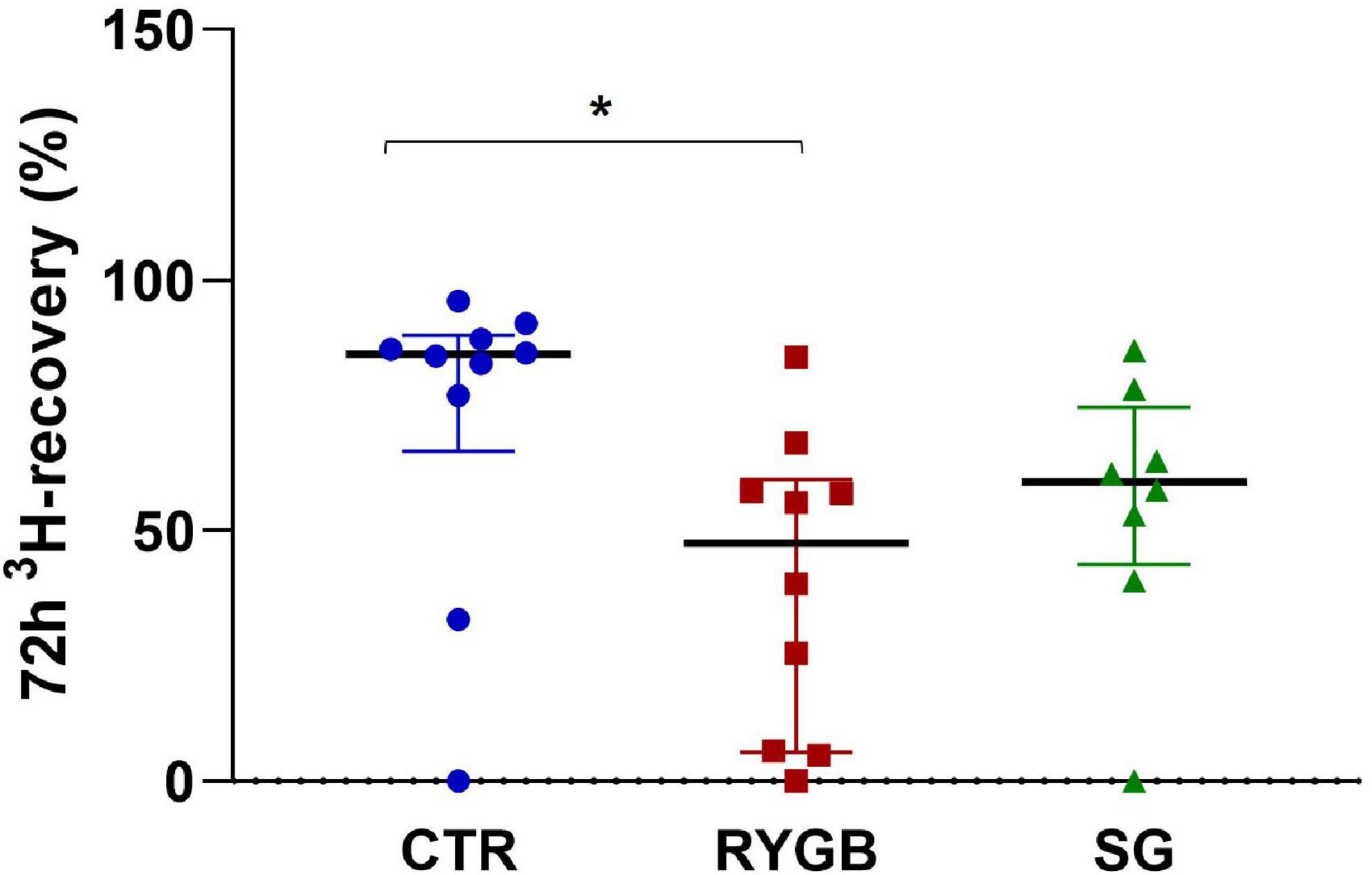
Figure 2. Median (IQR) 72-h 3H-recovery in feces of controls and bariatric surgery patients. Asterix indicates significance at the 0.05 level (one-way ANOVA with post-hoc Tukey–Kramer). CTR, control; RYGB, Roux-and-Y Gastric Bypass; SG, sleeve gastrectomy.
Protein digestion, malabsorption, and fermentation
Breath 6-h 13C-recovery was different across groups (ANOVA p = 0.015), with lower recovery in RYGB patients than in CTR (p = 0.039) and SG patients (p = 0.025; Figure 3A), indicating a lower protein digestion after RYGB. SG patients did not differ from CTR (p = 0.901). Time to maximum 13C-recovery (tmax) differed across the groups (ANOVA p = 0.003) with the surgery groups having a shorter tmax (110 and 105 min, for RYGB and SG patients, respectively) than the CTR group (150 min) (Figure 3B).
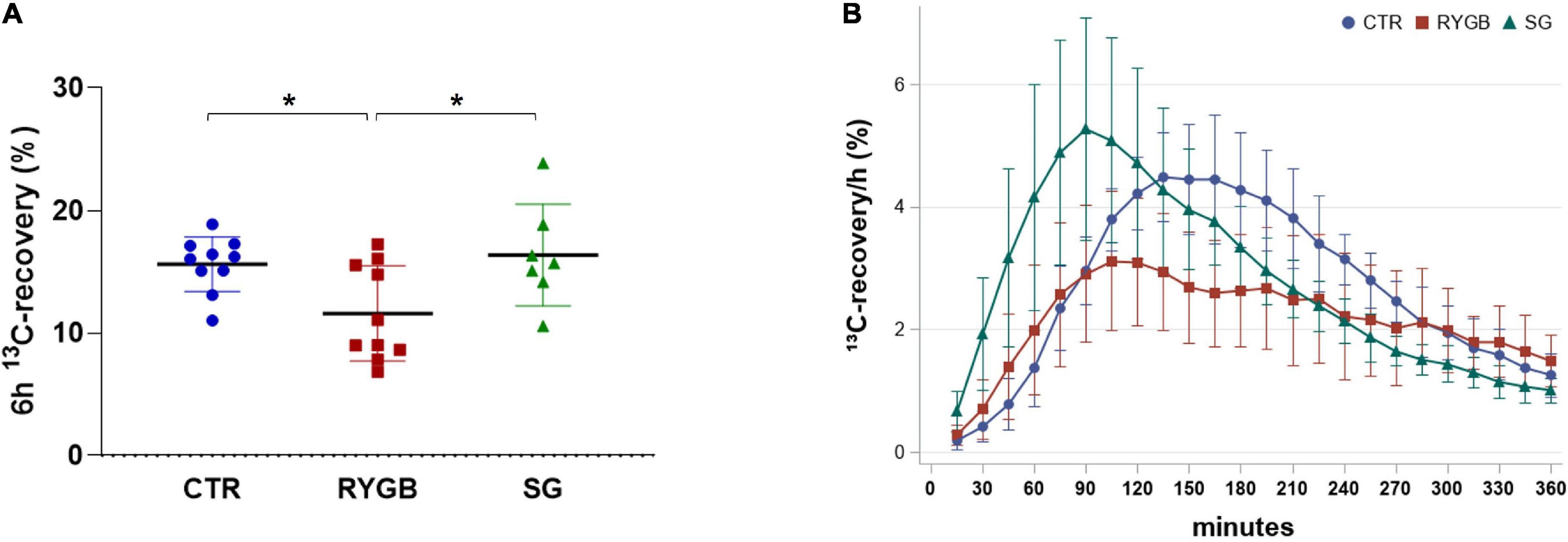
Figure 3. 13C-recovery in breath of controls and bariatric surgery patients. (A) Mean (SD) 6-h 13C-recovery and (B) 13C-recovery/h over time. Values are displayed as mean (SD) and N = 27. Asterix indicates significance at the 0.05 level (one-way ANOVA with post-hoc Tukey–Kramer). CTR, control; RYGB, Roux-and-Y Gastric Bypass; SG, sleeve gastrectomy.
The corrected 72-h 15N-recovery differed between the three groups (ANOVA p = 0.046; Figure 4A) although pairwise comparisons did not yield differences between groups. After exclusion of the outlier in the SG group, 15N-recovery was higher after RYGB than after SG (p = 0.016). Higher protein malabsorption was associated with lower protein digestion (ρ = −0.47, p = 0.020).
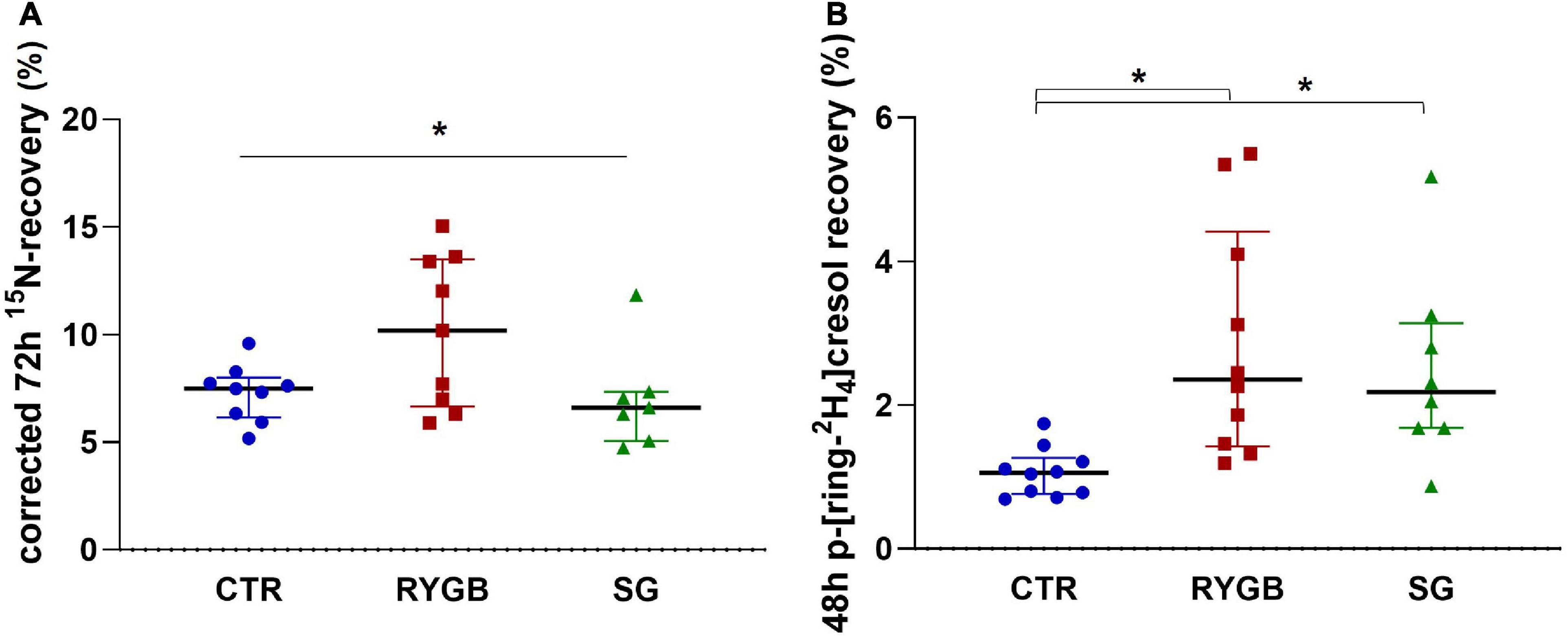
Figure 4. (A) Median (IQR) 72-h 15N-recovery (% 15N × 100/% 3H) in feces and (B) median (IQR) 48-h p-ring[2H4]cresol recovery (% of administered p-ring[2H4]tyrosine) in urine of controls and bariatric surgery patients. Asterix indicates significance at the 0.05 level (one-way ANOVA with post-hoc Tukey–Kramer). CTR, control; RYGB, Roux-and-Y Gastric Bypass; SG, sleeve gastrectomy.
Protein fermentation, evaluated from the p-[ring-2H4]cresol-recovery in urine, was different across groups (ANOVA p = 0.001), with higher fermentation in both surgery groups than in the CTR group (RYGB: p = 0.001 and SG: p = 0.006; Figure 4B) but no difference between surgery groups (p = 0.856). The p-[ring-2H4]cresol-recovery correlated negatively with 3H-recovery (ρ = −0.47, p = 0.013), indicating that a higher degree of protein fermentation was associated with a slower total transit. In contrast, protein fermentation did not correlate with protein malabsorption (ρ = 0.13, p = 0.524), nor with fiber intake (ρ = −0.19, p = 0.334). Group had an effect on protein fermentation, regardless of 3H-recovery (p = 0.003), protein malabsorption (p = 0.005) or fiber intake (p = 0.002).
Carbohydrate malabsorption
The iAUC of breath H2 was different across group (ANOVA p = 0.003). The RYGB group had higher breath H2 excretion than CTR and SG, (Figure 5A, p = 0.005 and p = 0.014, respectively), indicating more carbohydrate malabsorption after RYGB. CTR and SG subjects exhibited a similar extent of carbohydrate malabsorption (p = 0.978, Figure 5A).
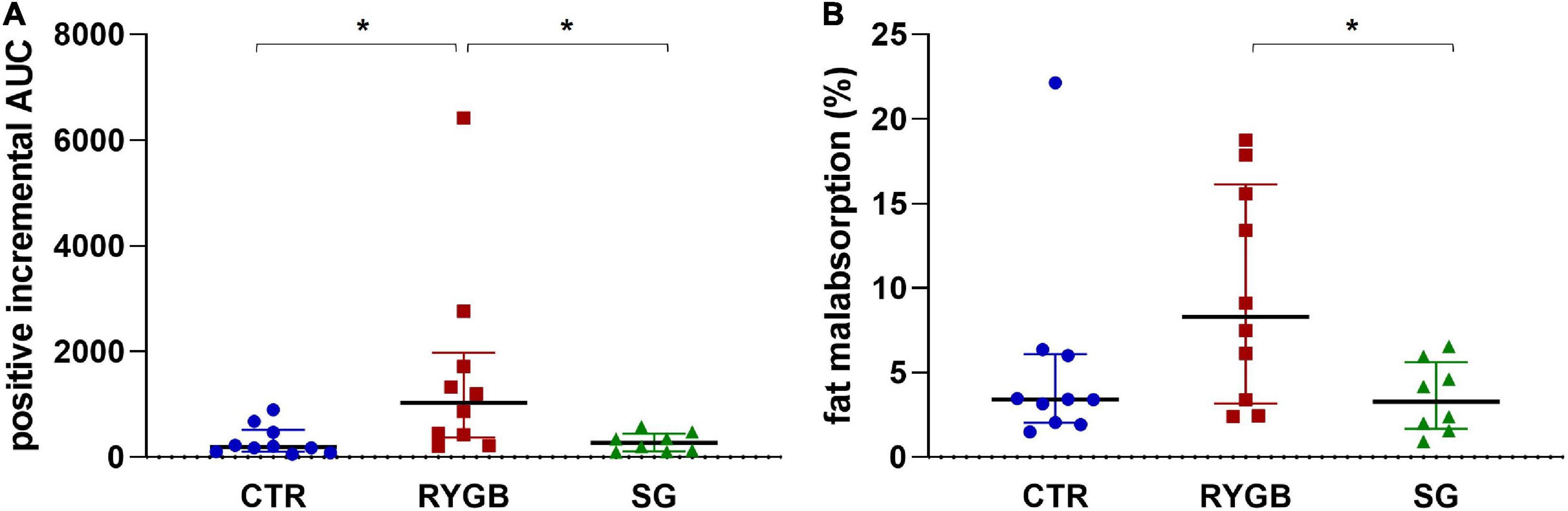
Figure 5. (A) Median (IQR) positive iAUC of H2 in breath and (B) median (IQR) fat malabsorption. Asterix indicates significance at the 0.05 level (one-way ANOVA with post-hoc Tukey–Kramer). AUC, area under the curve; RYGB, Roux-en-Y gastric bypass; SG, sleeve gastrectomy; CTR, control.
Fat malabsorption
Fat malabsorption differed across group (ANOVA p = 0.040) and was higher in RYGB patients than in SG patients (p = 0.043; Figure 5B). The CTR group did not differ from the surgery groups (RYGB: p = 0.138 and SG: p = 0.766) due to the presence of an outlier. After exclusion of this outlier, fat malabsorption was higher in RYGB than CTR (p = 0.025). The group effect on fat malabsorption remained significant (p = 0.004) after adjusting for bile acid malabsorption status.
Bile acid malabsorption
BS did not influence bile acid malabsorption as the proportion of subjects with bile acid malabsorption was similar across the three groups (p = 1.00). Only 1 CTR and 1 RYGB patient suffered from bile acid malabsorption.
Discussion
We hypothesized that the impact of BS on the assimilation of nutrients depends on the type of procedure. As the extent of anatomical rearrangement and nutrient rerouting is more pronounced after RYGB than after SG, we expected a higher level of malabsorption after RYGB. Indeed, our results indicate that RYGB, but not SG, slightly increased fat, protein and carbohydrate malabsorption compared to a control group with similar BMI that did not undergo surgery. Remarkably, the extent of protein fermentation was not only higher in RYGB but also in SG patients.
Fat malabsorption after RYGB has been reported previously (25–27) and is confirmed in the present study. In contrast, SG does not induce fat malabsorption. Absorption of dietary fat requires the concerted action of pancreatic lipase, to hydrolyze the triglycerides in the small intestine, and bile acids to form micelles for absorption of the fatty acids. A disturbance of either process will result in fat malabsorption. Occurrence of bile acid malabsorption could be ruled out as a causative factor since the bile acid breath test showed similar results across the groups. Similarly, previous studies comparing fecal bile acid secretion before and after RYGB excluded bile acid malabsorption (25, 26). Secretion of pancreatic enzymes is induced by secretion of cholecystokinin and secretin upon exposure of duodenal enteric endocrine cells to nutrients (28). As the duodenum is bypassed after RYGB, pancreatic stimulation is reduced resulting in lower secretion of digestive enzymes (29). Also asynchronization between the release of enzymes and passage of nutrients as a result of the structural changes to the GI tract may reduce fat digestion (30). Furthermore, supplementation of RYGB patients with pancreatic supplements (40,000 USP units per meal for 3 months) reduced, but did not normalize, the fat malabsorption, indicating that the malabsorption was at least partly due to enzyme deficiency (30). The fact that the duodenum remains intact after SG may explain the preservation of normal fat digestion after SG. As luminal digestion of fat is hardly compensated by non-pancreatic mechanisms, malabsorption of fat and steatorrhea are generally more severe and occur before malabsorption of protein and carbohydrates in case of pancreatic insufficiency (31). Hence, it is not surprising that malabsorption of fat is the most pronounced finding after BS.
We used egg proteins intrinsically labeled with three stable isotopes (13C, 15N, and 2H) to non-invasively evaluate the extent of protein assimilation, i.e., digestion, malabsorption, and fermentation. The 13C-protein breath test is a validated tool to quantify the extent of small intestinal protein digestion (32). The fractional fecal loss of 15N from orally administered egg protein was quantified as a measure of protein malabsorption. Quantified in this way, protein malabsorption might be overestimated due to tracer recycling, i.e., secretion into the colon of 15N that had been absorbed in the small intestine (33). However, previous studies in our lab using this technique showed that protein malabsorption was slightly lower in healthy subjects compared to healthy ileostomy subjects in identical conditions (24), suggesting a slight underestimation rather than overestimation of the protein losses, probably due to nitrogen salvage from the colon (34). Finally, the extent of protein fermentation was estimated from the urinary recovery of p-[ring-2H4]cresol (23). p-[ring-2H4]Cresol is not produced by human enzymes but only by bacterial metabolism in the colon from undigested protein containing p-[ring-2H4]tyrosine. It is readily absorbed from the colonic lumen and excreted in urine after sulfate- or glucuronide conjugation in the mucosa and liver. As p-[ring-2H4]cresol does not accumulate in the body of healthy subjects, its urinary excretion reflects its colonic generation (23).
Protein digestion was lower after RYGB compared to CTR and SG, and correlated negatively with protein malabsorption. Most likely, the lower protein digestion after RYGB is also due to the reduced stimulation of the pancreas and lower absorption surface due to the anatomical rearrangements. Indeed, secretion rates of pancreatic trypsin were lower in serial aspirates from the common channel of 13 RYGB patients than in the duodenal aspirates of 7 healthy controls (29). Furthermore, the time to maximal protein digestion rate was shorter after RYGB and SG compared to controls and probably reflects a faster gastric emptying rate. We were not able to directly measure gastric emptying rate as the isotopes that are standardly used in gastric emptying breath tests (13C or 14C) were already used for protein digestion and bile acid malabsorption, respectively. Similarly, a recent study that applied an isotope dilution technique to compare the absorption of intrinsically 15N labeled caseinate in RYGB and SG patients to controls found a higher systemic appearance rate of ingested phenylalanine in RYGB patients, indicating a faster protein digestion and suggesting a faster gastric emptying. However, total (6h) systemic recovery of ingested phenylalanine was similar across the 3 groups, suggesting that protein assimilation was unaffected after RYGB (35). A potential explanation for the apparent discrepancy with the results in the current study might lie in the different type of test meal (solid vs. liquid) in both studies. We used a solid meal that requires mechanical degradation in the stomach to particles less than 1–2 mm in size before they can pass through the pyloric sphincter to the duodenum (36). As the gastric pouch of RYGB no longer has a pyloric sphincter to govern the passage of the stomach content to the small intestine, larger particles may enter the jejunum, contributing to the compromised intestinal digestion. This complication plays no part when using liquid test meals neither in SG patients, where the pyloric sphincter and the small intestinal anatomy remain conserved.
Protein fermentation is increased after RYGB but also after SG, despite a normal protein digestion and absorption in the latter group, suggesting that other factors than protein malabsorption are involved. In addition to the type and amount of substrates supplied to the colon and the composition of the microbiota, transit time is a major factor that determines microbial metabolism (37). In a cross-sectional study in 98 adults with increased metabolic risk, a slow colonic transit was associated with a shift from carbohydrate fermentation to protein fermentation (38). In the present study, total transit was slower in both surgery groups compared to controls, and was negatively associated with protein fermentation. Constipation is a common problem after BS (39) that has been, at least partly, explained by a low fiber intake. Nevertheless, fiber intake and transit time were not correlated in our study. An alternative explanation for the slow transit might be the reported increase in post-prandial levels of glucagon-like peptide-1 (GLP-1) and peptide-YY (PYY) after bariatric surgery which delay intestinal transit (40). Unfortunately, these gut hormones were not quantified in this study.
The modest increase in breath hydrogen in RYGB patients indicates that the carbohydrate fraction in the test meal (11.6 g of starch, 12 g of sucrose, and 1.5 g of lactose) is incompletely digested in some patients. Carbohydrate digestion requires pancreatic α-amylase to hydrolyze starch into maltose, maltotriose and α-limit dextrins and the brushborder disaccharidases sucrase-isomaltase, lactase, maltase-glucoamylase and trehalase that further convert disaccharides into monosaccharides. The amount of lactose in the test meal is probably too low to explain the increased hydrogen since even lactase-deficient subjects are able to digest up to 12 g lactose per day (41). Studies that administered glucose and measured its systemic absorption found no effect of RYGB (35, 42), suggesting that carbohydrate digestion rather than absorption is reduced. Since pancreatic amylase is a very stable enzyme that is secreted in a large excess, the impaired carbohydrate absorption is probably not due to a lack of pancreatic enzyme but either to asynchronization between the secretion and nutrient passage or a too short contact time with the brush border enzymes (30). Nevertheless, supplementation with oral pancreatic enzymes in RYGB patients suppressed the late rise in breath H2 (28).
The implications of the macronutrient malabsorption after RYGB on the nutritional status of the patient may be limited. The energy deficit due to malabsorption has been estimated at not more than 200 kcal/day which is considerably lower than the energy deficit induced by the lower food intake (42). However, the increase in protein fermentation observed in both surgery groups may require further investigation. Indeed, despite prospective cohort studies consistently reporting a substantial reduction in all-cancer risk and mortality, observational data suggest that colorectal cancer (CRC) risk might actually increase after BS (43). A plausible hypothesis, raised by Hull et al. (43), implies that changes in colonic microbial metabolites due to altered dietary intake, macronutrient malabsorption, altered transit and persistent low microbial diversity may drive colorectal carcinogenesis together with other pro-carcinogenic factors like exposure to secondary bile acids and local inflammation. However, this hypothesis needs to be further investigated.
The main strength of the study is the use of proteins intrinsically labeled with 3 different stable isotopes allowed estimating protein digestion, malabsorption and fermentation simultaneously and accurately. Unfortunately, such tests are not available for fat malabsorption, and therefore we estimated fat intake from dietary records and used the golden standard to measure fat excretion. Hydrogen excretion in breath is standardly measured as a qualitative indication of carbohydrate malabsorption.
A limitation of the study is the limited number of participants, although that was based on a sample size calculation using protein malabsorption as the outcome parameter. Unfortunately, we were able to only include 8 patients that underwent SG instead of 10. Moreover, the breath samples from one patient in the SG group were not of sufficient quality for reliable analysis resulting in a missing value for protein digestion. For 2 other parameters, one subject (not the same) was an outlier. Due to the small groups and to be transparent, we report the statistical analysis with and without the outlier included. The limited number of participants precluded more detailed investigation in the origin of the variability observed for several parameters, in particular in the RYGB groups. Several factors may have affected this variability such as the time after surgery, the amount of weight loss, the length of the biliopancreatic limb or dietary intake and should be further investigated in studies specifically designed for this purpose.
Finally, we eliminated subjects on lactose-, glutenfree, vegetarian and vegan diets which implicates that the results of the study can not be extrapolated to those groups. Animal protein is on average better digestible than plant based protein (44). Therefore, we expect that BS patients on a vegetarian or vegan protein might experience a higher rather than lower degree of protein malabsorption.
In conclusion, RYGB but not SG patients experience higher fat, carbohydrate and protein malabsorption compared to controls. Bile acid malabsorption was not a driver for the fat malabsorption. Protein fermentation was increased after both surgery types and was associated with a slow transit. It would be interesting to evaluate whether treating the post-operative constipation would normalize the protein fermentation. Furthermore, the implications of the increased protein fermentation on colonic health need further investigation.
Data availability statement
The raw data supporting the conclusions of this article will be made available by the authors, without undue reservation.
Ethics statement
The studies involving human participants were reviewed and approved by the Ethics Committee of UZ/KU Leuven. The patients/participants provided their written informed consent to participate in this study.
Author contributions
CE and KV acquired funding, conceived and designed the study, wrote the manuscript, and had primary responsibility for the final content. DV and JB provided essential materials. CE, AL, and GV conducted the research. CE performed the statistical analyses. ML, BV, and KV supervised the study. All authors read and approved the final manuscript.
Funding
This study was supported by a grant from the Internal Funds of the Research Council of KU Leuven (Grant number: C14/15/074). Charlotte Evenepoel acknowledges the Research Foundation - Flanders (FWO – Vlaanderen, Brussels, Belgium) for her position as doctoral researcher (Grant number: 1S51318N| 1S51320N).
Conflict of interest
The authors declare that the research was conducted in the absence of any commercial or financial relationships that could be construed as a potential conflict of interest.
Publisher’s note
All claims expressed in this article are solely those of the authors and do not necessarily represent those of their affiliated organizations, or those of the publisher, the editors and the reviewers. Any product that may be evaluated in this article, or claim that may be made by its manufacturer, is not guaranteed or endorsed by the publisher.
References
1. Colquitt J, Pickett K, Loveman E, Frampton G. Surgery for weight loss in adults. Cochrane Database Syst Rev. (2014) 2014:Cd003641. doi: 10.1002/14651858.CD003641.pub4
2. Angrisani L, Santonicola A, Iovino P, Formisano G, Buchwald H, Scopinaro N. Bariatric surgery worldwide 2013. Obes Surg. (2015) 25:1822–32. doi: 10.1007/s11695-015-1657-z
3. Lannoo M, Dillemans B. Laparoscopy for primary and secondary bariatric procedures. Best Pract Res Clin Gastroenterol. (2014) 28:159–73. doi: 10.1016/j.bpg.2013.11.013
4. Elder K, Wolfe B. Bariatric surgery: a review of procedures and outcomes. Gastroenterology. (2007) 132:2253–71. doi: 10.1053/j.gastro.2007.03.057
5. Evers S, Sandoval D, Seeley R. The physiology and molecular underpinnings of the effects of bariatric surgery on obesity and diabetes. Annu Rev Physiol. (2017) 79:313–34. doi: 10.1146/annurev-physiol-022516-034423
6. Seeley R, Chambers A, Sandoval D. The role of gut adaptation in the potent effects of multiple bariatric surgeries on obesity and diabetes. Cell Metab. (2015) 21:369–78. doi: 10.1016/j.cmet.2015.01.001
7. Lupoli R, Lembo E, Saldalamacchia G, Avola C, Angrisani L, Capaldo B. Bariatric surgery and long-term nutritional issues. World J Diabetes. (2017) 8:464–74. doi: 10.4239/wjd.v8.i11.464
8. Stein J, Stier C, Raab H, Weiner R. The nutritional and pharmacological consequences of obesity surgery. Aliment Pharmacol Ther. (2014) 40:582–609. doi: 10.1111/apt.12872
9. Kuipers F, Bloks V, Groen A. Beyond intestinal soap–bile acids in metabolic control. Nat Rev Endocrinol. (2014) 10:488–98. doi: 10.1038/nrendo.2014.60
10. Gilbert M, Ijssennagger N, Kies A, van Mil S. Protein fermentation in the gut; implications for intestinal dysfunction in humans, pigs, and poultry. Am J Physiol Gastrointest Liver Physiol. (2018) 315:G159–70. doi: 10.1152/ajpgi.00319.2017
11. Louis P, Hold G, Flint H. The gut microbiota, bacterial metabolites and colorectal cancer. Nat Rev Microbiol. (2014) 12:661–72. doi: 10.1038/nrmicro3344
12. Geypens B, Claus D, Gorris N, Evenepoel P, Luypaerts A, Rutgeerts P, et al. Determination of deuterated phenylalanine and tyrosine in egg protein by GCQ. J High Res Chromatogr. (1999) 22:465–8. doi: 10.1002/(sici)1521-4168(19990801)22:83.0.co;2-s
13. Cloetens L, Swennen K, De Preter V, Broekaert W, Courtin C, Delcour J, et al. Effect of arabinoxylo-oligosaccharides on proximal gastrointestinal motility and digestion in healthy volunteers. E SPEN Eur E J Clin Nutr Metab. (2008) 3:e220–5. doi: 10.1016/j.eclnm.2008.05.008
14. Evenepoel P, Hiele M, Luypaerts A, Geypens B, Buyse J, Decuypere E, et al. Production of egg proteins, enriched with L-leucine-13C1, for the study of protein assimilation in humans using the breath test technique. J Nutr. (1997) 127:327–31. doi: 10.1093/jn/127.2.327
15. Geboes K, Bammens B, Luypaerts A, Malheiros R, Buyse J, Evenepoel P, et al. Validation of a new test meal for a protein digestion breath test in humans. J Nutr. (2004) 134:806–10. doi: 10.1093/jn/134.4.806
16. Haycock G, Schwartz G, Wisotsky D. Geometric method for measuring body surface area: a height-weight formula validated in infants, children, and adults. J Pediatr. (1978) 93:62–6. doi: 10.1016/s0022-3476(78)80601-5
17. Fromm H, Hofmann A. Breath test for altered bile-acid metabolism. Lancet. (1971) 298:621–5. doi: 10.1016/s0140-6736(71)80068-5
18. Houben E, De Preter V, Billen J, Van Ranst M, Verbeke K. Additional value of CH4 measurement in a combined (13)C/H2 lactose malabsorption breath test: a retrospective analysis. Nutrients. (2015) 7:7469–85. doi: 10.3390/nu7095348
19. Robb P, Wolever T, Hassanein J. Use of breath hydrogen to quantify carbohydrate malabsorption: lack of effect of soluble fibre (guar). Nutr Res. (1991) 11:1349–56.
20. Van De Kamer J, Ten Bokkel Huinink H, Weyers H. Rapid method for the determination of fat in feces. J Biol Chem. (1949) 177:347–55.
21. Krag E, Krag B, Lenz K. A comparison of stable and 3H-labelled polyethylene glycol 4000 as non-absorbable water phase markers in the human ileum and faeces. Scand J Gastroenterol. (1975) 10:105–8.
22. De Preter V, Vanhoutte T, Huys G, Swings J, Rutgeerts P, Verbeke K. Effect of lactulose and Saccharomyces boulardii administration on the colonic urea-nitrogen metabolism and the bifidobacteria concentration in healthy human subjects. Aliment Pharmacol Ther. (2006) 23:963–74. doi: 10.1111/j.1365-2036.2006.02834.x
23. De Preter V, Geboes K, Verbrugghe K, De Vuyst L, Vanhoutte T, Huys G, et al. The in vivo use of the stable isotope-labelled biomarkers lactose-[15N]ureide and [2H4]tyrosine to assess the effects of pro- and prebiotics on the intestinal flora of healthy human volunteers. Br J Nutr. (2004) 92:439–46. doi: 10.1079/bjn20041228
24. Evenepoel P, Claus D, Geypens B, Hiele M, Geboes K, Rutgeerts P, et al. Amount and fate of egg protein escaping assimilation in the small intestine of humans. Am J Physiol. (1999) 277(5 Pt 1):G935–43. doi: 10.1152/ajpgi.1999.277.5.G935
25. Odstrcil E, Martinez J, Santa Ana C, Xue B, Schneider R, Steffer K, et al. The contribution of malabsorption to the reduction in net energy absorption after long-limb Roux-en-Y gastric bypass. Am J Clin Nutr. (2010) 92:704–13. doi: 10.3945/ajcn.2010.29870
26. Moreland A, Santa Ana C, Asplin J, Kuhn J, Holmes R, Cole J, et al. Steatorrhea and hyperoxaluria in severely obese patients before and after Roux-en-Y gastric bypass. Gastroenterology. (2017) 152:1055–67.e3. doi: 10.1053/j.gastro.2017.01.004
27. Kumar R, Lieske J, Collazo-Clavell M, Sarr M, Olson E, Vrtiska T, et al. Fat malabsorption and increased intestinal oxalate absorption are common after Roux-en-Y gastric bypass surgery. Surgery. (2011) 149:654–61. doi: 10.1016/j.surg.2010.11.015
28. Keller J, Layer P. Human pancreatic exocrine response to nutrients in health and disease. Gut. (2005) 54(Suppl. 6):vi1–28. doi: 10.1136/gut.2005.065946
29. O’Keefe S, Rakitt T, Ou J, El H II, Blaney E, Vipperla K, et al. Pancreatic and intestinal function post Roux-en-Y gastric bypass surgery for obesity. Clin Transl Gastroenterol. (2017) 8:e112. doi: 10.1038/ctg.2017.39
30. Chaudhary A, Domínguez-Muñoz J, Layer P, Lerch M. Pancreatic exocrine insufficiency as a complication of gastrointestinal surgery and the impact of pancreatic enzyme replacement therapy. Dig Dis. (2020) 38:53–68. doi: 10.1159/000501675
31. Layer P, Keller J. Pancreatic enzymes: secretion and luminal nutrient digestion in health and disease. J Clin Gastroenterol. (1999) 28:3–10. doi: 10.1097/00004836-199901000-00002
32. Evenepoel P, Geypens B, Luypaerts A, Hiele M, Ghoos Y, Rutgeerts P. Digestibility of cooked and raw egg protein in humans as assessed by stable isotope techniques. J Nutr. (1998) 128:1716–22. doi: 10.1093/jn/128.10.1716
33. Kayser B, Acheson K, Decombaz J, Fern E, Cerretelli P. Protein absorption and energy digestibility at high altitude. J Appl Physiol. (1992) 73:2425–31. doi: 10.1152/jappl.1992.73.6.2425
34. Jackson A. Salvage of urea-nitrogen and protein requirements. Proc Nutr Soc. (1995) 54:535–47. doi: 10.1079/pns19950022
35. Svane M, Bojsen-Møller K, Martinussen C, Dirksen C, Madsen J, Reitelseder S, et al. Postprandial nutrient handling and gastrointestinal hormone secretion after Roux-en-Y gastric bypass vs sleeve gastrectomy. Gastroenterology. (2019) 156:1627–41.e1. doi: 10.1053/j.gastro.2019.01.262
36. Sensoy I. A review on the food digestion in the digestive tract and the used in vitro models. Curr Res Food Sci. (2021) 4:308–19. doi: 10.1016/j.crfs.2021.04.004
37. Macfarlane S, Macfarlane G. Regulation of short-chain fatty acid production. Proc Nutr Soc. (2003) 62:67–72. doi: 10.1079/PNS2002207
38. Roager H, Hansen L, Bahl M, Frandsen H, Carvalho V, Gøbel R, et al. Colonic transit time is related to bacterial metabolism and mucosal turnover in the gut. Nat Microbiol. (2016) 1:16093. doi: 10.1038/nmicrobiol.2016.93
39. Afshar S, Kelly S, Seymour K, Woodcock S, Werner A, Mathers J. The effects of bariatric procedures on bowel habit. Obes Surg. (2016) 26:2348–54. doi: 10.1007/s11695-016-2100-9
40. Jacobsen S, Olesen S, Dirksen C, Jørgensen N, Bojsen-Møller K, Kielgast U, et al. Changes in gastrointestinal hormone responses, insulin sensitivity, and beta-cell function within 2 weeks after gastric bypass in non-diabetic subjects. Obes Surg. (2012) 22:1084–96. doi: 10.1007/s11695-012-0621-4
41. Shaukat A, Levitt M, Taylor B, MacDonald R, Shamliyan T, Kane R, et al. Systematic review: effective management strategies for lactose intolerance. Ann Intern Med. (2010) 152:797–803. doi: 10.7326/0003-4819-152-12-201006150-00241
42. Mahawar K, Sharples A. Contribution of malabsorption to weight loss after Roux-en-Y gastric bypass: a systematic review. Obes Surg. (2017) 27:2194–206. doi: 10.1007/s11695-017-2762-y
43. Hull M, Markar S, Morris E. Cancer risk after bariatric surgery – Is colorectal cancer a special case? Nat Rev Gastroenterol Hepatol. (2018) 15:653–4. doi: 10.1038/s41575-018-0070-1
Keywords: bariatric surgery, isotope technology, macronutrient malabsorption, protein fermentation, protein digestion
Citation: Evenepoel C, Vandermeulen G, Luypaerts A, Vermeulen D, Lannoo M, Van der Schueren B, Buyse J and Verbeke K (2023) The impact of bariatric surgery on macronutrient malabsorption depends on the type of procedure. Front. Nutr. 9:1028881. doi: 10.3389/fnut.2022.1028881
Received: 26 August 2022; Accepted: 30 December 2022;
Published: 12 January 2023.
Edited by:
Edda Cava, San Camillo-Forlanini Hospital, ItalyReviewed by:
Ingrid Larsson, Sahlgrenska University Hospital, SwedenThomas Alexander Lutz, University of Zurich, Switzerland
Copyright © 2023 Evenepoel, Vandermeulen, Luypaerts, Vermeulen, Lannoo, Van der Schueren, Buyse and Verbeke. This is an open-access article distributed under the terms of the Creative Commons Attribution License (CC BY). The use, distribution or reproduction in other forums is permitted, provided the original author(s) and the copyright owner(s) are credited and that the original publication in this journal is cited, in accordance with accepted academic practice. No use, distribution or reproduction is permitted which does not comply with these terms.
*Correspondence: Kristin Verbeke, a3Jpc3Rpbi52ZXJiZWtlQGt1bGV1dmVuLmJl