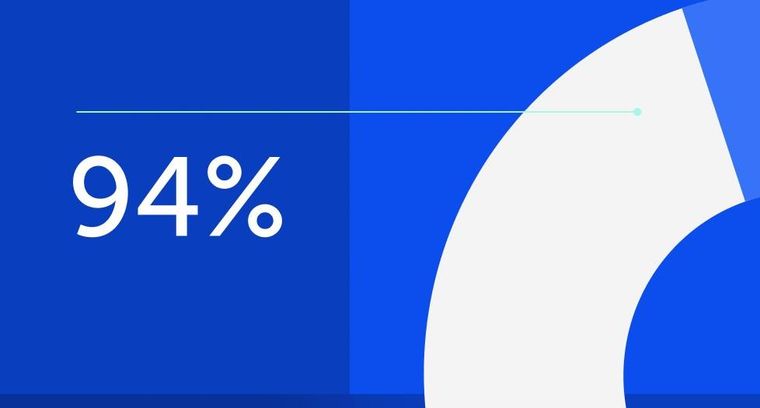
94% of researchers rate our articles as excellent or good
Learn more about the work of our research integrity team to safeguard the quality of each article we publish.
Find out more
ORIGINAL RESEARCH article
Front. Nutr., 28 October 2022
Sec. Nutrition and Food Science Technology
Volume 9 - 2022 | https://doi.org/10.3389/fnut.2022.1025080
This article is part of the Research TopicNovel Technologies for Enrichment, Extraction, and Determination of Phenolic Compounds in Foods - Volume 1View all 13 articles
In this study, a strain of Lactobacillus plantarum Y2 was isolated from the ginkgo peel, and showed adequate adaptation to the ginkgo biloba kernel juice. After 48 h of fermentation, the number of viable cells in the stable growth phase was remained at 10.0 Log CFU/mL, while the content of total organic acid increased by 5.86%. Phenolic substances were significantly enriched, and the content of total phenolic substances increased by 9.72%, and the content of total flavonoids after fermentation exceeded 55.33 mg/L, which was 3.6 times that of the unfermented ginkgo juice. The total relative content of volatile flavor compounds increased by 125.48%, and 24 new volatile flavor substances were produced. The content of total sugar, total protein, and total free amino acid decreased to 44.85, 67.51, and 6.88%, respectively. Meanwhile, more than 82.25% of 4′-O-methylpyridoxine was degraded by lactic acid fermentation, and the final concentration in ginkgo biloba kernel juice was lower than 41.53 mg/L. In addition, the antioxidant and antibacterial activities of fermented ginkgo biloba kernel juice were significantly enhanced. These results showed that LAB fermentation could effectively improve the nutritional value and safety of ginkgo biloba kernel juice.
Ginkgo biloba L., one of the oldest living tree species on earth (1), has been reported to have great development potential in the field of functional food (2). Meanwhile, ginkgo biloba kernel juice contains various active ingredients such as ginkgo phenolic acids, flavonoids, and polysaccharides, etc., making it widely used in food, health products, cosmetics, medicine and other fields (3). In particular, the special physiologically active components such as flavonoids and ginkgoid acid contained in it have the functions of anti-oxidation, anti-aging, anti-inflammatory, anti-allergic, and inhibiting nerve cell apoptosis (4). Recent studies on ginkgo have mainly focused on ginkgo leaves and relatively few studies on ginkgo kernels (5). Therefore, it is necessary and meaningful to further develop and utilize ginkgo kernels.
4′-O-methylpyridoxine (MPN) is a vitamin 6 derivative which can cause in pregnancy, radiation sickness, seborrheic dermatitis, and other pathologies. Interestingly, only a threshold concentration of MPN is beneficial to the human body; a higher concentration can lead to toxic reactions in the human body. The MPN content in medicine ranged from 0 to 9.77 μg/mL (6), and in ginkgo kernels ranged from 172.8 to 404.2 μg/g, indicating that excessive consumption of ginkgo kernels was harmful to human health (7). As MPN concentrations severely limit the development and utilization of ginkgo kernels, MPN degradation during the production process of ginkgo is a vital concern that needs to be addressed.
Lactic acid bacteria (LAB) have been widely used in the fermentation of pickles, yogurt, soy sauce and tempeh, owing to their ability to regulate the human intestinal flora, promote the absorption of nutrition substances, kill harmful flora and the toxins, and ameliorate food flavor (8). Moreover, the secondary metabolites in the fermentation process with LAB have several health benefits, such as promoting the activity of antioxidant enzymes in cells, and increasing the content of beneficial substances. For example, Verón found that LAB fermentation could increase the activities of ferulic acid, caffeic acid derivatives and intracellular antioxidant enzymes, and enhance the overall antioxidant capacity of the fermentation broth (9).
Currently, studies on ginkgo mainly focuses on ginkgo leaves, while there are relatively few studies on ginkgo kernels. Ginkgo products mainly include canned ginkgo kernel, ginkgo wine, and beverages (10, 11). However, only a few studies had been conducted on fermented silver almonds. In this study, a LAB strain was selected from ginkgo peel to ferment ginkgo biloba kernel juice. The physiological and biochemical indices of fermented ginkgo biloba kernel juice were analyzed, and the antioxidation activity and bacteriostatic ability were evaluated. The results of this study will enrich the physiological and biochemical studies of LAB-fermented ginkgo biloba kernel, and improve the reference for the development of ginkgo products.
Ginkgo was sourced from the campus of Jiangsu Ocean University (Lianyun Gang, Jiangsu province), and the LAB strains were screened from the surface of the ginkgo fruit. The ginkgo fruit was inoculated in MRS culture medium (g: mL, 1:1.5) and cultured at 37°C for 24 h. The fermentation broth was spreaded on MRS plates to obtain single colonies which were underlined on MRS plates and placed in a 37°C incubator for 24 h to obtain single colonies. The obtained single colonies were then grown in MRS medium, with 40% glycerol stocks stored and frozen in a −40°C refrigerator. These LAB strains were identified using 16S-rRNA, physiological and biochemical tests in accordance with conventional methods (12).
The ginkgo fruit was boiled for 5–10 min, and the shell and seed coat were removed. After washing with water, the shelled ginkgo kernels were crushed and broken up in a certain proportion (ginkgo biloba kernels: distilled water = 1:2.2, g:mL), and the starch dissolution rate was required to reach more than 80%. The α-amylase (20 U/g, ginkgo mass) and saccharification enzyme (30 U/g, ginkgo mass) were added, and then ginkgo biloba kernel juice was saccharified at 50°C for 2 h. After the enzymatic hydrolysis the ginkgo biloba kernel juice was completed. It was filtered through a 100-mesh filter, and pasteurized in a water bath at 90°C for 20 min (13). In a 500 mL sealed Erlenmeyer flask, 1% (v/v) inoculum of ginkgo biloba kernel juice was inoculated to ensure an initial viable count of approximately 5.0 Log CFU/mL. The fermentation process was performed in an incubator at 37°C for 48 h. At the end of fermentation, the bacterial cells were removed by centrifugation (10,000 g, 10 min, 4°C), and the supernatant was collected for further chemical analysis.
Viable cell counts were determined using the standard plate method (14). The fermentation broth was first diluted to an appropriate concentration, then the diluted liquid was spread on a plate and the colonies that grew were counted. A precision pH meter (PHS-3C, Shanghai INESA Scientific Instrument Co., Ltd., Shanghai, China) was used to measure the pH value of each sample of ginkgo biloba kernel juice fermentation broth and the bacteriostatic activity was assessed by measuring the diameter of the outward inhibition zone of the Oxford cup using a Vernier caliper (15). The centrifuged supernatant (10 mL) was concentrated in vacuo to 2 ml, and then screening through a 0.22 μm membrane screening. The bacterial pathogens were Escherichia coli CICC 10003, Staphylococcus aureus CICC 23656, and Bacillus cereus s CICC 23828.
The total sugar content was determined using the sulfuric acid phenol method (16). The principle of this method was that polysaccharides were hydrolyzed into monosaccharides under the action of sulfuric acid, and then rapidly dehydrated to form uronic derivatives, which were then combined with phenol to form orange-yellow compounds. The absorbance values at 470 nm of the orange-yellow compounds were linearly related to the monosaccharide concentration. A standard curve was established using anhydrous glucose and the results were expressed in μg/mL of glucose equivalents. Protein was detected using the Coomassie brilliant blue method G250 (17). A standard curve was established using a Bovine Serum Albumin (BSA) standard solution, and the total protein equivalent was expressed in mg/mL.
The organic acid spectrum was analyzed using a the Shimadzu LC-2010A system (Shimadzu, Tokyo, Japan), following the method of Luo Ke with some modifications (18). The chromatographic column was an Agilent TC-C18 column (4.6 × 25 mm, 5 μm), the detection wavelength was 210 nm, 0.08 M KH2PO4 solution (adjusted to pH 2.5 with phosphoric acid) was used for isocratic elution. The column temperature was 30°C, the flow rate was 0.7 mL/min, and the injection volume was 20 μL. The free amino acids were quantified using Adeyeye’s method with slight modifications. The analytical column was 2622PH 4.6 mm I.D. × 60 mm, the flow rate was 0.40 mL/min, the column temperature was 57°C, the reaction temperature was 135°C, and the detection wavelength was 570 nm, and the injection volume was 20 μL.
The total flavonoids was determined according to the method of Kwaw et al. (19). A calibration curve was constructed with rutin and the results were expressed in μg/mL of rutin equivalents. The total phenolic content of crude polyphenols in fermented or unfermented ginkgo biloba kernel was determined using the Folin-Ciocalteu method. A calibration curve constructed using gallic acid and the results were expressed in mg/L of gallic acid equivalents. Phenolic acid and flavonoids content were determined according to the method described by Chiang et al. (20). The column was Inertsil ODS-3 5 μm (4.6 × 250 mm, 5 μm), and the column temperature was 25°C. The mobile phase was composed of solution A (1% acetic acid in water) and the solution B (1% acetic acid in methanol), and the flow rate was 0.6 mL/min. The gradient set was as follows: 0–10 min, 10–26% B; 10–25 min, 26–40% B; 25–45 min, 40–65% B; 45–55 min, 65–95% B; 55–58 min, 95–10% B; 58–65 min, 10% B. The wavelength for determination of phenolic acids and flavonoids was 280 and 350 nm, respectively. The concentration of each phenolic compound was calculated according to the standard calibration curve, and the results were expressed in mg/mL.
Total antioxidant capacity was measured in the same way as previously described (21). The total antioxidant capacity was calculated as follows: total antioxidant capacity = (Measured OD − Control OD)/0.01/30 × total reaction volume/sampling volume × sample dilution ratio before testing. The ABTS+-SA assay was performed using the method described by Tao et al. (21). Trolox was used as the standard, and the results were expressed in mmol/LTrolox equivalents. The Iron Reducing Antioxidant Ability (FRAP) of the fermented samples was evaluated by the method of Yan et al. (22).
The volatile components were separated, collected, and analyzed using a triple quadrupole GC-MS (Trace 1310/TSQ 9000, Thermo Scientific) (23). The headspace vial was filled with nitrogen to expel the air in the vial, and 5 mL tested sample was taken into the Thermo RSH autosampler for extraction. The extraction head was placed in the gas chromatography injection port, the injection port temperature was 250°C, the aging was 30 min, the oven temperature was set to 80°C, the water bath was set for 20 min, the adsorption extraction was 30 min, and the extraction head was inserted into the injection port for analysis for 5 min. The MPN content was determined reference to the method described by Yoshimura using the Agilent 1260 fluorescence detector (detection wavelength was 291 nm) (24).
All experiments were performed three times in the same way, and all samples were analyzed in triplicate. The significance analysis was performed using SPSS Statistics 20 (IBM Corp., NY, USA), and the significance was assessed at p < 0.05 value. The principal component analysis (PCA) was performed using Origin 2018 (Origin Lab Corp., UK), and all data results were expressed as mean ± standard deviation.
In this study, nine LAB strains were isolated from the surface of ginkgo fruit. The developmental tree established by the BLAST program and the results of the physiology and biochemistry of the strains confirmed that all the selected strains were all Lactobacillus plantarum (Table 1 and Figure 1). Next, the acid-producing and antibacterial activities of the nine strains were evaluated. The pH of ginkgo biloba kernel juice, fermented by different strains, decreased, indicating that these strains had good acid production capacity. Among them, the pH of ginkgo biloba kernel juice fermented by the strain Y2 had a lower pH than the other strains, indicating that Y2 has a stronger growth and metabolism in ginkgo biloba kernel juice (25). In addition, the pH of ginkgo biloba kernel juice fermented by the strain Y2 had the best antibacterial effect on pathogenic bacteria (E. coli, S. aureus, and B. cereus), with inhibition zone diameters of 13.77 ± 0.32, 21.54 ± 0.58, and 15.57 ± 0.53 mm, respectively. When the acidity of fermented ginkgo biloba kernel juice is high, the pH value of the medium decreases, reducing conductivity for pathogenic bacterial growth (26). Lactic acid and compounds such as bacteriocins and synthetic hydrogen peroxide produced by the fermentation of lactic acid bacteria inhibit microbial growth (27). In conclusion, the strain Y2 had a good growth and metabolic status in ginkgo biloba kernel juice, and was selected as an effective fermentation strain for further experiments.
Table 1. The pH change and antibacterial activity determination of Ginkgo biloba kernel juice fermented by LAB strains used in this study.
Figure 1. The colonial morphology (A, MRS plate), gram staining picture (B) and colonial morphology the hylogenetic tree (C) of Lactobacillus plantarum Y2.
Cell viability is a functional feature used to evaluate bacterial growth (28). The strain Y2 grew well in ginkgo biloba kernel juice without any nutritional supplements according to the number of viable cells of strain Y2 in the fermenting process, showing that ginkgo biloba kernel juice could be used as a fermentation substrate (Figure 2A). The strain Y2 grew rapidly in the logarithmic phase from 4 to 8 h, and the number of viable bacteria increased significantly, indicating that Y2 was suitable for growth in ginkgo biloba kernel juice. The number of viable cells started at 5.0 ± 0.02 log CFU/mL and grew rapidly to 10.0 ± 0.03 log CFU/mL after 8 h of fermentation. After 24 h of fermentation, the number of viable cells stabilized at 12.1 ± 0.08 log CFU/mL. After 48 h of fermentation, the number of viable cells of LAB Y2 remained at 8.4 ± 0.03 log CFU/mL. The initial pH of ginkgo biloba kernel juice was 6.2 ± 0.04 (Figure 2B). In the early stage of fermentation, a large amount of lactic acid was produced, and the pH value of the fermentation broth decreased rapidly. After 12 h of fermentation, the rate of pH continued to gradually slow down reaching a final pH of 3.27 ± 0.07 at 48 h. According to the characteristics of lactic acid bacteria fermentation and acid production, Y2 was a LAB with strong acid production ability and good fermentation effect. The changes in antioxidant activity during the fermentation of ginkgo biloba kernel juice were shown in Figures 2C,D. The ABTS+ radical scavenging capacity and FRAP reducing capacity increased with time. After 48 h of fermentation, the free radical scavenging rate of ABTS+ increased from 4.52 ± 0.72 to 13.69 ± 0.32 mM, and the reducing capacity of FRAP increased from 0.35 ± 0.01 to 0.62 ± 0.02 mM. After 0–4 h of fermentation, the ABTS+ free radical scavenging rate and FRAP reducing ability increased significantly, and the antioxidant activity of the fermentation broth improved significantly. After 24 h of fermentation, the lifting speed approached slowly, and the antioxidant activity was basically stable at the end of fermentation.
Figure 2. Viable cell count (A), changes of pH (B), ABTS+ free radical scavenging ability (C), FRAP reducing antioxidant capacity (D) of Ginkgo biloba kernel juice fermented by strain Y2.
Carbon source catabolism could provide energy for the growth of Lactobacillus and indirectly promote an increase in organic acid content (29). The total sugar content decreased significantly from 12 to 36 h of fermentation, indicating that the lactic acid bacteria in the stable growth period consumed large amounts of carbon sources (Table 2). As fermentation entered the decay period (36–48 h of fermentation), lower substrate pH and reduced nutrients limited the growth of Y2 and indirectly affected its sugar metabolism, resulting in a stabilization of the total sugar contents (30). The consumption rate exceeded 44.85% after the fermentation ended.
Table 2. Total sugar, total protein and total phenolic content of Ginkgo biloba kernel juice fermented by strain Y2.
During the fermentation, the organic acids were transformed into each other over time, and the total organic acid content increased by about approximately 5.86% after 48 h of fermentation. The components of all fermentation time periods were well separated from the control samples according to the component map (Figure 3A), showing that the fermentation of Y2 significantly changed the organic acid composition in ginkgo biloba kernel juice. According to the loading diagram (Figure 3B), malic acid, tartaric acid, citric acid, pyruvic acid and quinic acid all had high positive values for PCI, indicating that the content of these substances decreased during the fermentation process. While the decrease in malic acid was particularly obvious, levels of oxalic acid did not observe any changes during fermentation. Lactic acid, shikimic acid, fumaric acid, and succinic acid had high negative values for PCI, reflecting a general increase in content after fermentation.
Figure 3. Principal component analysis of changes in organic acids (A,B), free amino acids (C,D) and phenolic acids (E,F) during fermentation of Ginkgo biloba kernel juice by Y2 for 48 h.
Ginkgo kernel was rich in a variety of natural organic acids, of which malic acid had the highest content (926.91 ± 5.35 μg/mL), followed by pyruvic acid (80.27 ± 0.27 μg/mL), citric acid (43.77 ± 0.55 μg/mL) and tartaric acid (632.34 ± 19.98 μg/mL) (Table 3). Lactic acid was the main organic acid produced by the consumption of sugars by lactic acid bacteria in ginkgo biloba kernel juice, and the lactic acid content continued to increase throughout the fermentation process. The initial lactic acid content was 403.33 ± 17.77 μg/mL and increased to 722.58 ± 29.76 μg/mL after 48 h of fermentation. The production of abundant lactic acid reduced the pH value of the fermentation substrate. Pyruvate and citric acid could be decompose into various products such as lactic acid and acetic acid during fermentation (31). Malic acid, which was a good carbon source, observed the highest proportion of total organic acids. The content of malic acid decreased by about 25.25% during the fermentation process, while the content of lactic acid and succinic acid increased. Tartaric acid could be decomposed into various products, such as gluconic acid, which was then oxidized to 2-keto-D-gluconic acid (2-KGA) and 5-keto-D-gluconic acid (5-KGA) (32). During fermentation, the tartaric acid content gradually decreased, and the reduction rate was about 37.42%. In conclusion, lactic acid bacteria fermented ginkgo biloba kernel juice could transform and generate a variety of organic acids. Organic acid was the base of taste substance of ginkgo biloba kernel juice, and was the precursor of many flavor substances. Volatile flavor substances such as acids, alcohols, and aldehydes will be produced in the process of microbial metabolism. Therefore, we evaluated the changes of flavor substances before and after fermentation (Table 5).
Lactic acid bacteria could degrade macromolecular proteins into small peptide chains, amino acid proteases and peptidases through their own reproductive metabolism and hydrolysis during fermentation (33). Under the catalytic reaction, the proteases could degrade the protein into smaller peptides that are degraded into the small-molecule free amino acids by the action of the peptidase (34). The total protein content of ginkgo biloba kernel juice decreased continuously during the fermentation process, and approximately 67.51% of the metabolism was consumed at the end of fermentation (Table 2).
Among all free amino acids, glutamic acid had the highest content (74.22 ± 0.01 μg/mL), followed by arginine (55.68 ± 0.01 μg/mL) and alanine (20.83 ± 0.05 μg/mL) (Table 4). Glutamate, free histidine and alanine levels decreased significantly after fermentation. During the fermentation process, under the action of lactic acid bacteria decarboxylase, Glu is converted into γ-aminobutyric acid, and Asp is converted into Ala (35). The combination of aspartic acid, glutamic acid and Na+ makes the sample umami, which would give ginkgo biloba juice rich flavor substances. In addition, the content of aromatic amino acids (Phe) decreased significantly after fermentation, while the content of another aromatic tyrosine (Tyr) increased, owing to the effect of amino acid invertase on the fermentation process (36). The content of branched-chain amino acids (Leu, Ile) also decreased significantly after fermentation, then were metabolized into keto acids, alcohols and fatty acids through amino acid converting enzymes. Phe and Thr could be converted into benzyl alcohol, phenylethanol and acetaldehyde with certain flavor under the action of an amino acid converting enzyme. Arginine is converted to citrulline by the enzyme arginine deiminase during lactic acid fermentation, which is further broken down into ornithine and free ammonia (37).
The total free amino acid content increased by approximately 6.88% after 48 h of fermentation. The effects of lactic acid fermentation on free amino acid profiles were studied using principal component analysis (PCA). In principal component analysis, PC1 and PC2 accounted for 98.2 and 1.8% of the data variance, respectively. As shown in the composition diagram (Figure 3C), the samples of unfermented ginkgo biloba kernel juice were located on the positive side of PC1, and the samples fermented for 48 h were distributed on the negative side. This indicated that lactic acid bacteria had a significant effect on the change in free amino acid content during the fermentation of ginkgo biloba kernel juice. Specific changes in the free amino acids were observed through in the loading diagram (Figure 3D). Phe, Thr, Leu, Ile, Asp, Ser, Ala, and free histidines were all positively correlated with PC1, indicating that these amino acids would be affected by the fermentation process. Whereas, Lys, Val, Met, Gly, Cys, Tyr, and Arg were all negatively correlated with PC2, indicating that their content increased with the fermentation time.
Ginkgo kernels were also rich in a variety of phenolic substances, which led to the production of many secondary metabolites during fermentation, including phenolic acids, stilbenes, lignins, and aromatic compounds (38). Therefore, it is necessary to study the effect of LAB fermentation on the phenolic components in ginkgo biloba kernel juice.
As shown in Table 1, the total phenolic content of ginkgo biloba kernel juice at the beginning of fermentation was 116.51 ± 0.51 mg/L. It decreased significantly within 4 h of fermentation, and then increased slowly. After 48 h of fermentation, the total phenolic content of the samples was approximately 9.72% higher than that of the unfermented samples. Procyanidin B2 is an oligomer, dimer and polymer of catechin (39). The highest content of flavonoids in ginkgo kernel was about 7.32 ± 0.06 μg/mL of catechin and about 125.92 ± 0.56 μg/mL of procyanidin B2 (Table 5). Procyanidin B2 increased by approximately 20.93%, and catechin increased by approximately 30.46% after 4 h of fermentation. The increase in the total flavonoid content could result in an increase in the antioxidant activity of the fermented samples. In the process of fermenting ginkgo biloba kernel juice, the improvement in antioxidant activity was highly correlated with the transformation of phenolic substances (40). Previous studies have reported that phenolic compounds could be used as reducing agents as well as free radical scavengers and singlet oxygen quenchers (41). Phenols could be converted into each other, and the antioxidant activity of the fermentation broth can be improved (42). It was also shown that the antioxidant capacity of phenolic substances in ginkgo biloba kernel juice was significantly improved after Y2 fermentation (Figures 2C,D). There is also a certain relationship between FRAP and phenolic content, and the presence of phenolic substances can effectively reduce Fe3+ to Fe2+ form (43). Therefore, the increase in phenolic substances was an important factor affecting the antioxidant activity of fermentation.
As shown in Figures 3E,F, principal component analysis (PCA) was used to analyze the effect of Y2 fermentation on phenolic components in ginkgo biloba kernel juice. After 48 h of fermentation, phloridzin, epicatechin and gallate in the samples were metabolized to form gallic acid and phloretin with a strong antioxidant quality (44). L. plantarum fermentation could produce some phenolic acid decarboxylases, such as ferulic acid. These extracellular enzymes act on phenolic components, and from a chemical point of view, glycosylation, methylation and other types of substitutions are carried out, resulting in structural changes. This lead to the mutual conversion of phenolic substances in the sample, thereby changing their oxidative activity (45). The p-hydroxybenzoic acid content decreased by approximately 17.95% after fermentation. Further, the content of chlorogenic acid was the highest at 4 h of fermentation (3.64 ± 0.08 μg/mL), and it increased by about 20.17% at the end of fermentation. The increase in chlorogenic acid content leads to an increase in pharmacological functions, such as antioxidant, antiviral, anti-cancer and antibacterial capacities (46). During the fermentation period of 4–8 h, the content of chlorogenic acid gradually decreased, potentially under the action of extracellular enzyme hydrolysis, which indirectly led to an increase in caffeic acid, p-coumaric acid and ferulic acid. Phenolic acids not only have various pharmacological effects such as anti-inflammatory, antioxidant, anti-mutation, and anti-cardiovascular disease, but also have co-color effects (such as ferulic acid), which can enhance the stability of their own colors after fermentation (47). There are few studies on the volatile flavor compounds after fermentation, and further research is needed.
A total of 64 volatile flavor compounds were identified in ginkgo biloba kernel juice before and after fermentation, including three kinds of aldehydes, 18 kinds of alcohols, 9 kinds of ketones, 8 kinds of esters, 29 kinds of hydrocarbons, 6 kinds of phenols, 3 kinds of heterocycles, 7 kinds of acids, and two other types. From the analysis results, the fermented samples and the unfermented samples were quite different, and the similarities of volatile flavor substances were low. The relative content and species of ginkgo biloba kernel juice in alcohols, acids, and heterocyclic compounds were significantly increased after 48 h of fermentation (Figure 4).
Figure 4. Changes in relative content (A) and number of species (B) of volatile flavor compounds in Ginkgo biloba kernel juice fermented by Y2 for 48 h.
The main aroma substances in unfermented ginkgo biloba kernel juice were 2-ethyl-1-hexanol, 1-octanol, and n-octanol (Table 6). The total relative content of volatile flavor compounds increased by 125.48% after fermention. Among them, the total relative content of alcohol increased by about 104.69%, potentially due to the emergence of new generation of various volatile flavor substances. After 48 h of fermentation, the contents of 1-octanol and n-octanol increased significantly, the contents of 2-ethyl-1-hexanol and 1-octanol decreased, and 1-heptanol, 1-octen-3-ol and 2-octen-1-ol newly formed. The relatively high contents of newly generated alcohols were geraniol (1.81%), 1-octen-3-ol (2.65%) and 1-heptanol (3.24%). These alcohols were mainly produced by metabolism during microbial processes, degradation of unsaturated fatty acids, and reduction of carbon-based compounds during fermentation. Aldehydes are unstable compounds, and the relative content of aldehydes increased by about 126.5%, among which the highest content was 16.15% 2,5-dimethylbenzaldehyde. The relative content of hydrocarbons increased by about 32.92%, while the types of hydrocarbons decreased after fermentation. Meanwhile, the relative content of newly generated aromatic compounds was higher, of which 1,6-dimethyl-4-(1-methylethyl)-naphthalene accounted for about 2.18% of the total relative content after fermentation. The increase of total content of alcohols and esters in ginkgo biloba kernel juice could mpart stronger fruity and floral aromas (48). Under the metabolic activity of lactic acid bacteria, more aldehydes are reduced to alcohols and acids, and the increase of alcohols will increase the corresponding esters, which is also the reduction of aldehydes in fermented ginkgo biloba kernel juice, while alcohol, the reasons for the increase in esters (49). The content of acids increased by about 786.01%, and the relative content of acids increased significantly. The strain Y2 produced a large amount of acids during the fermentation process, among which the new acids were 2.16% methoxy-acetic acid, 1.02% dodecanoic acid, 0.29% 2-methylbutyric acid, 0.24% 2-ethylhexanoic acid. Among them, the relative contents of L-lactic acid and propionic anhydride decreased after the fermentation, while the relative content of acetic anhydride was high (4.18%). Meanwhile, the relative content of phenols changed scarcely, and eugenol (7.48%) and 3-ethylphenol (0.29%) were newly formed at the end of fermentation. The former was produced by the reaction of guaiacol and allyl alcohol, and had strong antibacterial activity. The latter had an aromatic odor and was widely used in organic synthesis and as a solvent (50). Future research will focus on the transformation mechanism between these molecules and their effect on the flavor of ginkgo biloba kernel juice.
4′-O-methylpyridoxine (MPN), also known as ginkgo toxin, is an anti-vitamin B6 compound, and one of the toxic components in ginkgo kernels. If ingested in large amounts, adverse symptoms, such as vomiting, clonic seizures, and loss of consciousness could occur (51). Relevant studies had shown that MPN could be effectively converted into 4′-O-methylpyridoxine-5-glycoside (MPNG) after heating, microwave irradiation and boiling (52). In this study, the MPN content in ginkgo biloba kernel juice was identified, by comparison with standard chemicals determined by high performance liquid phase (HPLC) analysis (Figure 5). The content of MPN was reduced by roughly 82.25% after fermentation for 48 h, indicating that strain Y2 could effectively gradate MPN. Relevant studies have shown that lactic acid bacteria have the function of adsorbing toxins during the fermentation process. During the growth of lactic acid bacteria, a large number of polysaccharides and peptidoglycan were produced on the cell wall, which played a key role in adsorbing the toxic substance 4′-O-methylpyridoxine in ginkgo biloba kernel juice (53). It also had been reported that MPN could be phosphorylated to form 4′-O-methylpyridoxine-5′-phosphate (MPNP) under the action of phosphoric acid produced during LAB fermentation (54). However, there are few studies on the degradation mechanism of 4′-O-methylpyridoxine content by LAB fermentation, and we will further investigate how strain Y2 bio-transformed MPN.
This work showed that LAB fermentation was a potential way to improve the beneficial value and safety of ginkgo biloba kernel juice. The total relative content of volatile flavor substances increased by about 125.48%, and 24 new substances were added. The biotransformation and biosynthesis content of the phenols was increased, and the total phenolic concentration increased by about 9.72%, which indirectly led to the enhance of antioxidant capacity of the fermentation. The increase of the aromatic amino acids and volatile flavor substances reflected the metabolism of lactic acid bacteria in fruit juice. In addition, 4′-O-methylpyridoxine, as one of the toxic substances in ginkgo biloba kernel, showed a degradation rate above 82.25%, and the total ginkgo acid content in the final product was less than 41.53 mg/L. As few reports about the degradation of 4′-O-methylpyridoxine by fermentation, further studied will focus on the degradation mechanism. Our results showed that the LAB fermented ginkgo biloba kernel juice had broad application prospects.
The original contributions presented in this study are included in the article/supplementary material, further inquiries can be directed to the corresponding author/s.
JY and YS performed the experiments and analyzed the data. JC, YC, HZ, and TG drafted the manuscript. FX and SP analyzed and discussed the data. YT and JL contributed to the writing—review, editing, and funding acquisition. All authors have read and agreed to the published version of the manuscript.
This research was funded by the Natural Science Research General Project of Jiangsu Higher Education Institutions (Nos. 20KJB550008 and 21KJB550003), the National Natural Science Foundation of China (Nos. 32100037 and 32201964), Science and Technology Projects of Jiangsu (No. BE2021353), Jiangsu Planned Projects for Postdoctoral Research Funds (No. 2021K364C), Postdoctoral Research Funds of Lianyungang city (No. ZKK2021006), and the Innovative Project of the Priority Academic Program Development of Jiangsu Higher Education Institutions (No. 2021JSPAPD20).
The authors declare that the research was conducted in the absence of any commercial or financial relationships that could be construed as a potential conflict of interest.
All claims expressed in this article are solely those of the authors and do not necessarily represent those of their affiliated organizations, or those of the publisher, the editors and the reviewers. Any product that may be evaluated in this article, or claim that may be made by its manufacturer, is not guaranteed or endorsed by the publisher.
1. Dubber MJ, Kanfer I. Determination of terpene trilactones in Ginkgo biloba solid oral dosage forms using HPLC with evaporative light scattering detection. J Pharm Biomed Anal. (2006) 41:135–40. doi: 10.1016/j.jpba.2005.11.010
2. Lim HB, Kim DH. Correction to: effects of roasting conditions on physicochemical properties and antioxidant activities in Ginkgo biloba seeds. Food Sci Biotechnol. (2018) 27:1549. doi: 10.1007/s10068-018-0454-6
3. Hosoda S, Kawazoe Y, Shiba T, Numazawa S, Manabe A. Anti-obesity effect of Ginkgo vinegar, a fermented product of Ginkgo seed coat, in mice fed a high-fat diet and 3T3-L1 preadipocyte cells. Nutrients. (2020) 12:230. doi: 10.1186/s12906-019-2756-5
4. Ahlemeyer B, Krieglstein J. Pharmacological studies supporting the therapeutic use of Ginkgo biloba extract for Alzheimer’s disease. Pharmacopsychiatry. (2003) 36:8–14. doi: 10.1055/s-2003-40454
5. Ademiluyi AO, Oyeniran OH, Jimoh TO, Oboh G, Boligon AA. Fluted pumpkin (Telfairia occidentalis) seed modulates some markers of erectile function in isolated rat’s corpus cavernosum: influence of polyphenol and amino acid constituents. J Food Biochem. (2019) 43:e13037. doi: 10.1111/jfbc.13037
6. Arenz A, Klein M, Fiehe K. Occurrence of neurotoxic 4′-O-methylpyridoxine in Ginkgo biloba leaves, Ginkgo medications and Japanese Ginkgo food. Planta Med. (1996) 62:548–51. doi: 10.1055/s-2006-957967
7. Lu Y, Sun L, Li C, Wang X, Li W, Zhao T, et al. Comparative mass spectrometry analysis of N-glycans from the glycoproteins of eight allergy-inducing plants. Food Chem. (2022) 384:132440. doi: 10.26914/c.cnkihy.2020.022032
8. Prete R. Lactic acid bacteria exopolysaccharides producers: a sustainable tool for functional foods. Foods. (2021) 10:1653. doi: 10.3390/FOODS10071653
9. Verón HE, Gauffin Cano P, Fabersani E, Sanz Y, Isla MI, Fernández Espinar MT, et al. Cactus pear juice fermented with autochthonous Lactobacillus plantarum S-811. Food Funct. (2019) 10:1085–97. doi: 10.1039/C8FO01591K
10. Wang J, Ren S, Fang XX. Data comparison of the effects of feeding Ginkgo leaf fermentation and Chinese herbal medicine on meat ducks. J Phys. (2019) 1423:012018. doi: 10.1088/1742-6596/1423/1/012018
11. Hu P, Song W, Shan YJ. Lactobacillus paracasei sub sp. paracasei M5L induces cell cycle arrest and calreticulin translocation via the generation of reactive oxygen species in HT-29 cell apoptosis. Food Funct. (2015) 6:2257–65. doi: 10.1039/c5fo00248f
12. Yi L, Qi T, Hong Y, Deng L, Zeng K. Screening of bacteriocin-producing lactic acid bacteria in Chinese homemade pickle and dry-cured meat, and bacteriocin identification by genome sequencing. LWT. (2020) 125:109177. doi: 10.1016/j.lwt.2020.109177
13. Binte Abu Bakar SY, Salim M, Clulow AJ, Hawley A, Pelle J, Geddes DT, et al. Impact of pasteurization on the self-assembly of human milk lipids during digestion. J Lipid Res. (2022) 63:100183. doi: 10.1016/J.JLR.2022.100183
14. Kaprasob R, Kerdchoechuen O, Laohakunjit N, Sarkar D, Shetty K. Fermentation-based biotransformation of biaoctive phenolics and volatile compounds from cashew apple juice by screening lactic acid bacteria. Process Biochem. (2017) 59:141–9. doi: 10.1016/j.procbio.2017.05.019
15. Bungenstock L, Abdulmawjood A, Reich F. Evaluation of antibacterial properties of lactic acid bacteria from traditionally and industrially produced fermented sausages from Germany. PLoS One. (2020) 15:e0230345. doi: 10.1371/journal.pone.0230345
16. Cai H, Tian Z. Determination of total sugar content in strawberries by phenol-sulfuric acid method. Jilin Agric. (2019) 4:46. doi: 10.14025/j.cnki.jlny.2019.04.011
17. Lü X, Li D, Huang Y, Zhang Y. Application of a modified Coomassie Brilliant Blue protein assay in the study of protein adsorption on carbon thin films. Surf Coat Technol. (2007) 201:6843–6. doi: 10.1016/j.surfcoat.2006.09.019
18. Luo K. Effect of organic acids on the morphology and particle size of titanium dioxide (E171) in processed food. J Hazard Mater. (2022) 432:128666. doi: 10.1016/J.JHAZMAT.2022.128666
19. Kwaw E, Ma Y, Tchabo W. Effect of fermentation parameters and their optimization on the phytochemical properties of lactic-acid-fermented mulberry juice. J Food Meas Charact. (2017) 11:1462–73. doi: 10.1007/s11694-017-9525-2
20. Chiang ELC, Lee S, Medriano CA. Assessment of physiological responses of bacteria to chlorine and UV disinfection using a plate count method, flow cytometry, and viability PCR. J Appl Microbiol. (2021) 132:1788–801. doi: 10.1111/JAM.15325
21. Shi HT, Wang BY, Bian CZ, Han YQ, Qiao HX. Fermented Astragalus in diet improved laying performance, egg quality, antioxidant and immunological status and intestinal microbiota in laying hens. AMB Express. (2020) 10:159. doi: 10.21203/rs.3.rs-30575/v2
22. Yan Z, Xie L, Li M. Phytochemical components and bioactivities of novel medicinal food – Peony roots. Food Res Int. (2020) 140:109902. doi: 10.1016/j.foodres.2020.109902
23. Matsubara H, Imamura Y. GC-MS analysis of volatile sulfuric compounds in sweet potato shochu and the identification of off-flavor substances. J JA Soc Brew Chem. (2011) 106:493–501. doi: 10.6013/jbrewsocjapan.106.493
24. Yoshimura T, Udaka N. High performance liquid chromatographic determination of ginkgotoxin and ginkgotoxin−5’−glucoside in Ginkgo biloba seeds. J Liq Chromatogr R T. (2007) 29:605–16. doi: 10.1080/10826070500531466
25. Piao M, Liu J, Liu Q, Kim D, Dhungana SK, Kim J, et al. Development of antioxidative soy sauce fermented with enzymatic hydrolysates of Eupolyphaga sinensis. J Pure Appl Micro. (2016) 10:2511–9.
26. Pereira A, Maciel TC, Rodrigues S. Probiotic beverage from cashew apple juice fermented with Lactobacillus casei. Food Res Int. (2011) 44:1276–83. doi: 10.1016/j.foodres.2010.11.035
27. Tang HW, Phapugrangkul P, Fauzi HM. Lactic acid bacteria bacteriocin, an antimicrobial peptide effective against multidrug resistance: a comprehensive review. Int J Pept Res Ther. (2022) 28:1–14. doi: 10.1007/s10989-021-10317-6
28. Kamatani T, Fukunaga K, Miyata K. Construction of a system using a deep learning algorithm to count cell numbers in nanoliter wells for viable single-cell experiments. Sci Rep. (2017) 7:16831. doi: 10.1038/s41598-017-17012-x
29. Deutscher J. The mechanisms of carbon catabolite repression in bacteria. Curr Opin Microbiol. (2008) 11:87–93. doi: 10.1016/j.mib.2008.02.007
30. Tang H, Qian B, Xia B, Zhuan Y, Yao Y, Gan R, et al. Screening of lactic acid bacteria isolated from fermented Cornus officinalis fruits for probiotic potential. J Food Saf. (2018) 38:e12565. doi: 10.1111/jfs.12565
31. Dudley EG, Steele JL. Succinate production and citrate catabolism by cheddar cheese nonstarter lactobacilli. J Appl Microbiol. (2005) 98:14–23. doi: 10.1111/j.1365-2672.2004.02440.x
32. Kotera U, Umehara K, Kodama T, Yamada K. Isolation method of highly tartaric acid producing mutants of gluconobacter suboxydans. Agric Biol Chem. (2014) 36:1307–13. doi: 10.1080/00021369.1972.10860418
33. Farrell K, Musaus M, Navabpour S, Martin K, Ray WK, Helm RF, et al. Proteomic analysis reveals sex-specific protein degradation targets in the amygdala during fear memory formation. Front Mol Neurosci. (2021) 14:716284. doi: 10.3389/FNMOL.2021.716284
34. Diana M, Rafecas M, Quílez J. Free amino acids, acrylamide and biogenic amines in gamma-aminobutyric acid enriched sourdough and commercial breads. J Cereal Sci. (2014) 60:639–44. doi: 10.1016/j.jcs.2014.06.009
35. Nishimura K, Ijiri D, Shimamoto S, Takaya M, Ohtsuka A, Goto T. Genetic effect on free amino acid contents of egg yolk and albumen using five different chicken genotypes under floor rearing system. PLoS One. (2021) 16:e0258506. doi: 10.1371/JOURNAL.PONE.0258506
36. Yvon M, Rijnen L. Flavour formation by amino acid catabolism. Biotechnol Adv. (2006) 24:238–42. doi: 10.1016/j.biotechadv.2005.11.005
37. Mira de Orduña R, Liu SQ, Patchett ML, Pilone GJ. Kinetics of the arginine metabolism of malolactic wine lactic acid bacteria Lactobacillus buchneri CUC-3 and Oenococcus oeni Lo111. J Appl Microbiol. (2000) 89:547–52. doi: 10.1046/j.1365-2672.2000.01135.x
38. Manach C, Scalbert A, Morand C, Rémésy C, Jiménez L. Polyphenols: food sources and bioavailability. Am J Clin Nutr. (2004) 79:727–47. doi: 10.1093/ajcn/79.5.727
39. Crozier A, Jaganath IB, Clifford MN. Chem inform abstract: dietary phenolics: chemistry, bioavailability and effects on health. Nat Prod Rep. (2009) 26:1001–43. doi: 10.1002/chin.200946244
40. Chen Y, Wang Y, Chen J, Tang H, Wang C, Li Z, et al. Bioprocessing of soybeans (Glycine max L.) by solid-state fermentation with Eurotium cristatum YL-1 improves total phenolic content, isoflavone aglycones, and antioxidant activity. RSC Adv. (2020) 10:16928–41. doi: 10.1039/C9RA10344A
41. Emmanuel K, Yongkun M, William T. Effect of Lactobacillus strains on phenolic profile, color attributes and antioxidant activities of lactic-acid-fermented mulberry juice. Food Chem. (2018) 250:148–54. doi: 10.1016/j.foodchem.2018.01.009
42. Xiao Y, Wu X, Yao X, Chen Y, Ho CT, He C, et al. Metabolite profiling, antioxidant and α-glucosidase inhibitory activities of buckwheat processed by solid-state fermentation with Eurotium cristatum YL-1. Food Res Int. (2021) 143:110262. doi: 10.1016/j.foodres.2021.110262
43. Vadivel V, Stuetz W, Scherbaum V, Biesalski HK. Total free phenolic content and health relevant functionality of Indian wild legume grains: effect of indigenous processing methods. J Food Compos Anal. (2011) 24:935–43. doi: 10.1016/j.jfca.2011.04.001
44. Tao Y, Wu P, Dai Y, Luo X, Manickam S, Li D, et al. Bridge between mass transfer behavior and properties of bubbles under two-stage ultrasound-assisted physisorption of polyphenols using macroporous resin. Chem Eng J. (2022) 436:135158. doi: 10.1016/J.CEJ.2022.135158
45. Amrane-Abider M, Nerín C, Tamendjari A, Serralheiro MLM. Phenolic composition, antioxidant and antiacetylcholinesterase activities of Opuntia ficus-indica peel and flower teas after in vitro gastrointestinal digestion. J Sci Food Agric. (2022) 102:4401–9. doi: 10.1002/JSFA.11793
46. Matsuda R, Sakagami H, Amano S, Iijima Y, Sano M, Uesawa Y, et al. Inhibition of neurotoxicity/anticancer activity of bortezomib by caffeic acid and ahlorogenic acid. Anticancer Res. (2022) 42:781–90. doi: 10.21873/anticanres.15536
47. Cao YX, Wu ZF, Weng PF. Effect of phenolic acids on color and volatile flavor substances during storage of bayberry fermented wine. Food Sci. (2021) 42:78–85. doi: 10.7506/spkx1002-6630-20200712-161
48. Zhen S, Dong H, Chun-hui Z, Hai L, Xia L, Zhi-bin L, et al. Profile analysis of the volatile flavor compounds of quantitative marinated chicken during processing. Sci Agric Sin. (2016) 49:3030–45. doi: 10.3864/j.issn.0578-1752.2016.15.017
49. Le Y, Yang H. Xanthan gum modified fish gelatin and binary culture modulates the metabolism of probiotics in fermented milk mainly via amino acid metabolism pathways. Food Res Int. (2022) 161:111844. doi: 10.1016/J.FOODRES.2022.111844
50. Chen Y, Li P, Liao L, Qin Y, Jiang L, Liu Y. Characteristic fingerprints and volatile flavor compound variations in Liuyang Douchi during fermentation via HS-GC-IMS and HS-SPME-GC-MS. Food Chem. (2021) 361:130055. doi: 10.1016/j.foodchem.2021.130055
51. Lim HB, Kim DH. Effect of heat treatment on 4′-O-methylpyridoxine (MPN) content in Ginkgo biloba seed extract solution. J Sci Food Agric. (2018) 98:5153–6. doi: 10.1002/jsfa.9017
52. Hao G. Determination of native contents of 4′- O -methylpyridoxine and its glucoside in raw and heated Ginkgo biloba seeds by high-performance liquid chromatography. J Food Meas Charact. (2020) 14:917–24. doi: 10.1007/s11694-019-00341-y
53. Haskard CA, El-Nezami HS, Kankaanpaa PE. Surface binding of aflatoxin B1 by lactic acid bacteria. Appl Environ Microbiol. (2001) 67:3086–91. doi: 10.3969/j.issn.1004-8456.2003.03.005
Keywords: ginkgo biloba kernel juice, lactic acid bacteria fermentation, phenolic substances, free amino acids (FAAs), volatile flavor substances
Citation: Yang J, Sun Y, Chen J, Cheng Y, Zhang H, Gao T, Xu F, Pan S, Tao Y and Lu J (2022) Fermentation of ginkgo biloba kernel juice using Lactobacillus plantarum Y2 from the ginkgo peel: Fermentation characteristics and evolution of phenolic profiles, antioxidant activities in vitro, and volatile flavor compounds. Front. Nutr. 9:1025080. doi: 10.3389/fnut.2022.1025080
Received: 22 August 2022; Accepted: 13 October 2022;
Published: 28 October 2022.
Edited by:
Yu Xiao, Hunan Agricultural University, ChinaReviewed by:
Hongshun Yang, National University of Singapore, SingaporeCopyright © 2022 Yang, Sun, Chen, Cheng, Zhang, Gao, Xu, Pan, Tao and Lu. This is an open-access article distributed under the terms of the Creative Commons Attribution License (CC BY). The use, distribution or reproduction in other forums is permitted, provided the original author(s) and the copyright owner(s) are credited and that the original publication in this journal is cited, in accordance with accepted academic practice. No use, distribution or reproduction is permitted which does not comply with these terms.
*Correspondence: Jing Lu, amluZ2x1QGpvdS5lZHUuY24=
Disclaimer: All claims expressed in this article are solely those of the authors and do not necessarily represent those of their affiliated organizations, or those of the publisher, the editors and the reviewers. Any product that may be evaluated in this article or claim that may be made by its manufacturer is not guaranteed or endorsed by the publisher.
Research integrity at Frontiers
Learn more about the work of our research integrity team to safeguard the quality of each article we publish.