- 1College of Health Management, Shangluo University, Shangluo, Shaanxi, China
- 2Shaanxi Key Laboratory of Bioresources, 2011 QinLing-Bashan Mountains Bioresources Comprehensive Development C. I. C., Qinba State Key Laboratory of Biological Resources and Ecological Environment, College of Bioscience and Bioengineering, Shaanxi University of Technology, Hanzhong, Shaanxi, China
- 3College of Society and Science, Tibet Cultural University, Xianyang, China
- 4College of Food Science and Technology, Northwest University, Xi’an, Shaanxi, China
- 5Department of General Surgery, Institute of Precision Diagnosis, Treatment of Digestive System Tumors, Shenzhen University General Hospital, Shenzhen University, Shenzhen, Guangdong, China
- 6Department of Medical Pharmacology, Faculty of Medicine, Atatürk University, Erzurum, Türkiye
- 7State Key Laboratory of Biobased Material and Green Papermaking, Qilu University of Technology, Shandong Academy of Sciences, Jinan, China
- 8Department of Pharmacology, Faculty of Veterinary Medicine, Cairo University, Giza, Egypt
Asparagus root (AR) is utilized globally as a traditional herbal medicine because it contains various bioactive compounds, such as polyphenols, flavonoids, saponins, and minerals. The composition profiles of AR are strongly affected by its botanical and geographical origins. Although minerals and heavy metals are minor constituents of AR, they play a crucial role in determining its quality and efficacy. A comprehensive classification of AR, its phytochemistry, and its pharmacology were reviewed and interpreted herein. Potentially eligible articles (in English) were identified through an electronic search of the Web of Science database (2010–2022) and Google (2001–2022). We used the primary search term “Asparagus roots” combined with the words “pharmacology,” “bioactive compounds,” “physicochemical properties,” and “health benefits” to find the relevant literature. We screened the titles, keywords, and abstracts of the publications obtained from the database. A full copy of the article was obtained for further assessment if deemed appropriate. Different asparagus species might potentially be used as herbal medicines and functional foods. Phytochemical studies have revealed the presence of various bioactive compounds as valuable secondary metabolites. The dominant class of bioactive compounds in AR is flavonoids. Furthermore, AR displayed significant pharmacological effects, such as antioxidant, antimicrobial, antiviral, anticancer, anti-inflammatory, and antidiabetic effects, as shown in animal and human studies. This review provides a valuable resource to enable a thorough assessment of the profile of Asparagus root as a functional ingredient for the pharmaceutical and food industries. In addition, it is anticipated that this review will provide information to healthcare professionals seeking alternative sources of critical bioactive compounds.
1. Introduction
The Asparagus crop (Family Asparagaceae, including approximately 300 species) is native to the eastern Mediterranean region and Asia. The Asparagus crop grows in various soil types (1). Under optimum climate conditions, asparagus could attain growth rates up to 1 cm/h and be harvested once the plant reaches a height close to 21 cm (2). The asparagus plant can be continuously harvested for up to 40 years or until there is a decline in productivity and quality owing to exposure to microbial agents, such as Fusarium, Phytophthora, Stemphylium, Phomopsis asparagi (Sacc.) Bubak, and Cercospora asparagi Sacc. species. The regeneration of the rootstock is therefore necessary for sustained productivity. Residual asparagus roots from the main crop are considered waste and are commonly left to rot in fields. However, crops that cannot be degraded after replanting new asparagus plants would cause some problems. The reason is that residual autotoxins present in original asparagus roots might have allopathic properties, which hinder the growth of new asparagus plants (3). This was accounted mainly for Fusarium, the primary cause of root rot disease. Asparagus officinalis, a versatile plant with a unique flavor, is one of the most popular fresh vegetables worldwide. It was reported that autotoxins in residual asparagus roots might negatively impact sequential crops over time. Hence, physically removing the roots would be better (3). While farmers may be aware of the autotoxicity of asparagus roots (AR), some may consider root removal an unrealistic economic solution due to the costs involved.
However, residual Asparagus roots contain several bioactive compounds, such as saponins, polyphenols, and flavonoids, which have application value in nutraceutical and pharmaceutical processing (4). Most importantly, these bioactive compounds extracted from AR would enable farmers to generate additional revenue from the sale of AR, thus subsidizing the cost of removing the roots (5). On the other hand, removing AR would improve the growth of new asparagus since its allopathic effect was circumvented, thus increasing farmers’ incomes. Such streamlining processes to capture more value from AR via the recovery of bioactive compounds with a wide range of health benefits would maximize crop utilization while reducing waste.
Studies on Asparagus and reviews are likely to focus on pharmacological activities because Asparagus racemosus, one of the most investigated varieties, has long been used in traditional medicine. Therefore, providing scientific evidence that AR is a valuable byproduct rather than a waste product is crucial to encourage the farming community to ‘harvest’ AR and use it in the nutraceutical and medicinal industries. Therefore, we aimed to provide comprehensive information on the biological activities of major metabolites and the contribution of these compounds in pharmacological and health status to develop AR in the pharmacy and functional food industry. In this review article, potential eligible studies (in English) were identified through an electronic search of the Web of Science database (2010-2022)1 and Google (2001–2022). We used the primary search term “Asparagus roots” combined with the words “pharmacology,” “bioactive compounds,” “physicochemical properties,” and “health benefits” to find the relevant literature. We screened the titles, keywords, and abstracts of the publications obtained from the database. A full copy of the article was obtained for further assessment if deemed appropriate.
2. Literature analysis of asparagus roots research
CiteSpace software is a visual analysis software developed by Dr. Chenchaomei of Redsell University in the United States, which can be used to analyze the potential multivariate, time-sharing, dynamic and other knowledge in scientific literature. This article takes the Web of Science (WOS) as the core database with ‘Asparagus roots’ as the keyword to search the literature published from 2010 to 2022. The CiteSpace analysis tool and the bibliometric analysis platform2 were used to analyze the development status, development trend and research hot spots of asparagus to predict future research trends and provide a reference for the research and development of asparagus.
2.1. Analysis of annual documents, research countries and institutes
As shown in Figure 1, the top three countries in the number of documents published on AR research were India, China, and Japan in 2010, and in 2021, they were China, India, and Poland. The number of papers published each year ranged from 16 to 34. Research on asparagus has not encountered outbreaks. As the first breeding country and the first exporting country of asparagus, the production of asparagus in China is fairly simple, primarily as a vegetable. Byproduct processing is insufficient, and market efficiency needs to be improved. The roots of asparagus are potential raw materials for functional foods. Therefore, we comprehensively reviewed the research on asparagus roots.
Table 1 was obtained by analyzing the literature of major research institutions in the field of asparagus roots in the world from 2010 to 2022. The research institution with the most papers published is Naresuan University, followed by Gifu University. Interestingly, among the top 10 institutions, none are from China. According to the ranking of total citations, Naresuan University is also the number, followed by Gifu University and Poznań University of Life Sciences. From Table 1, we can see that the published papers on AR were widely distributed to many institutes instead of being deeply researched by several major institutes.
By analyzing the sending countries and institutions, we can show the distribution, reserves and cooperation of scientific research forces in the field of AR. As shown in Figure 2A, countries cooperate closely in AR; cross-country cooperation mainly focuses on the leading countries, and other countries are the nodes.
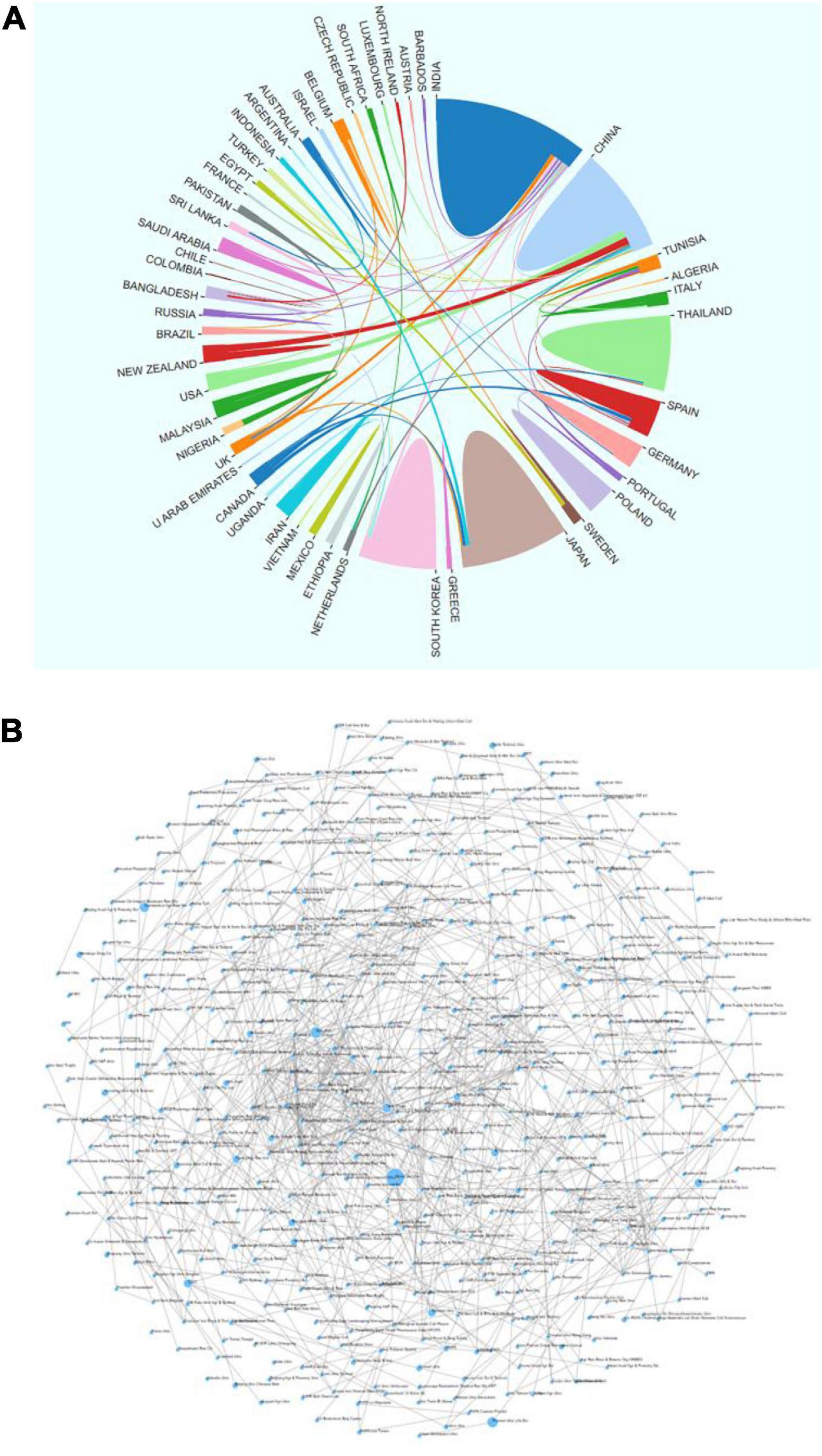
Figure 2. Cooperation between national/regional research institutions of asparagus root (AR) research globally from 2010 to 2022. (A) Cooperation between countries and regions; (B) cooperation between research institutions.
India and China are not only at the forefront of AR research but also maintain close cooperation with each other. The cooperation networks with India and China are 11 and 9 countries, respectively. These countries have made outstanding contributions to global AR research.
As seen from the institutional cooperation relationship (Figure 2B), the institutions at the cooperation nodes are the Naresuan Univ, Pozna Univ Life Sci and Univ Otago. From the analysis of research institutions, it can be seen that in the research of AR, one research institution is the center, and other research institutions focus on it. However, the research cooperation links between several major research institutions are slightly insufficient.
2.2. Analysis of research direction
The research direction in this field can be seen from the amount of literature published in relevant journals. According to the statistical findings of the industry fields of the selected documents in the WOS database (Figure 3). As shown, the research direction is in the three major research fields of Plant Science, Horticulture and Pharmacology Pharmacy (Figure 3B). Through the analysis of the journals published by the selected literature through the online analysis platform of literature metrology, it is found that the top journal is “ACTA Horticulture,” which, with the most papers published and the most frequently cited, is not a top journal. This might suggest that although AR research has been recognized internationally, the academic quality of AR research needs improvement, and the research depth needs to be strengthened.
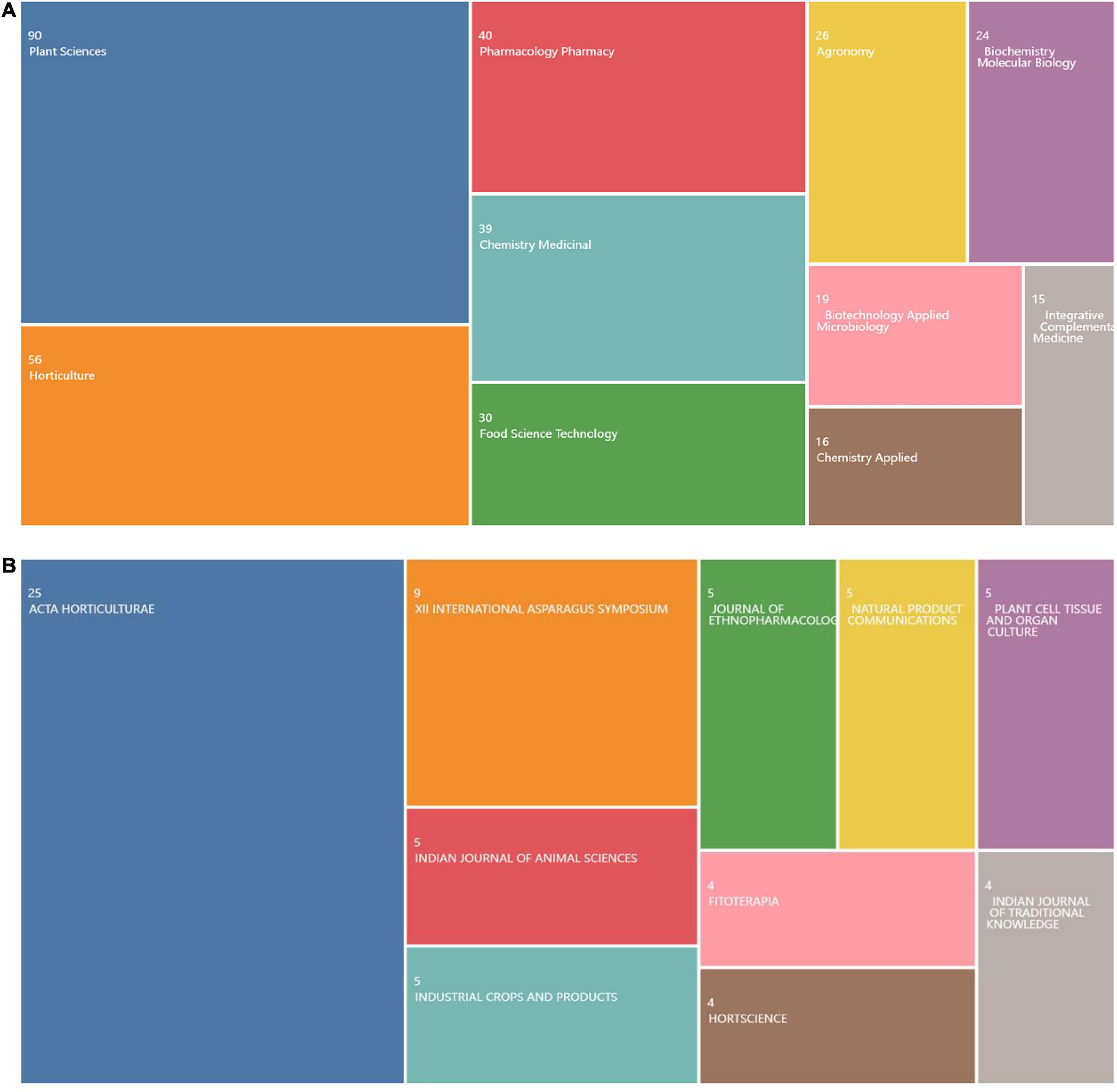
Figure 3. Research direction of asparagus root (AR) from 2010 to 2022. (A) Ranking of the research direction of AR in Web of Science (WOS). (B) Ranking of the Global Journal publishers of AR.
2.3. Analysis of research hotspots
Research hotspots are the focus that scholars pay attention to and discuss at a certain stage in a specific field. Keywords are the refinement and generalization of the content expressed in an article, so they are often used to analyze the hot spots in a particular field. Figure 4 shows that from 2010 to 2022, the high-frequency keywords for AR research included “antioxidant capacity,” “extract,” “plant growth,” and “antioxidant activity.” This shows that functional research on AR is limited to antioxidants.
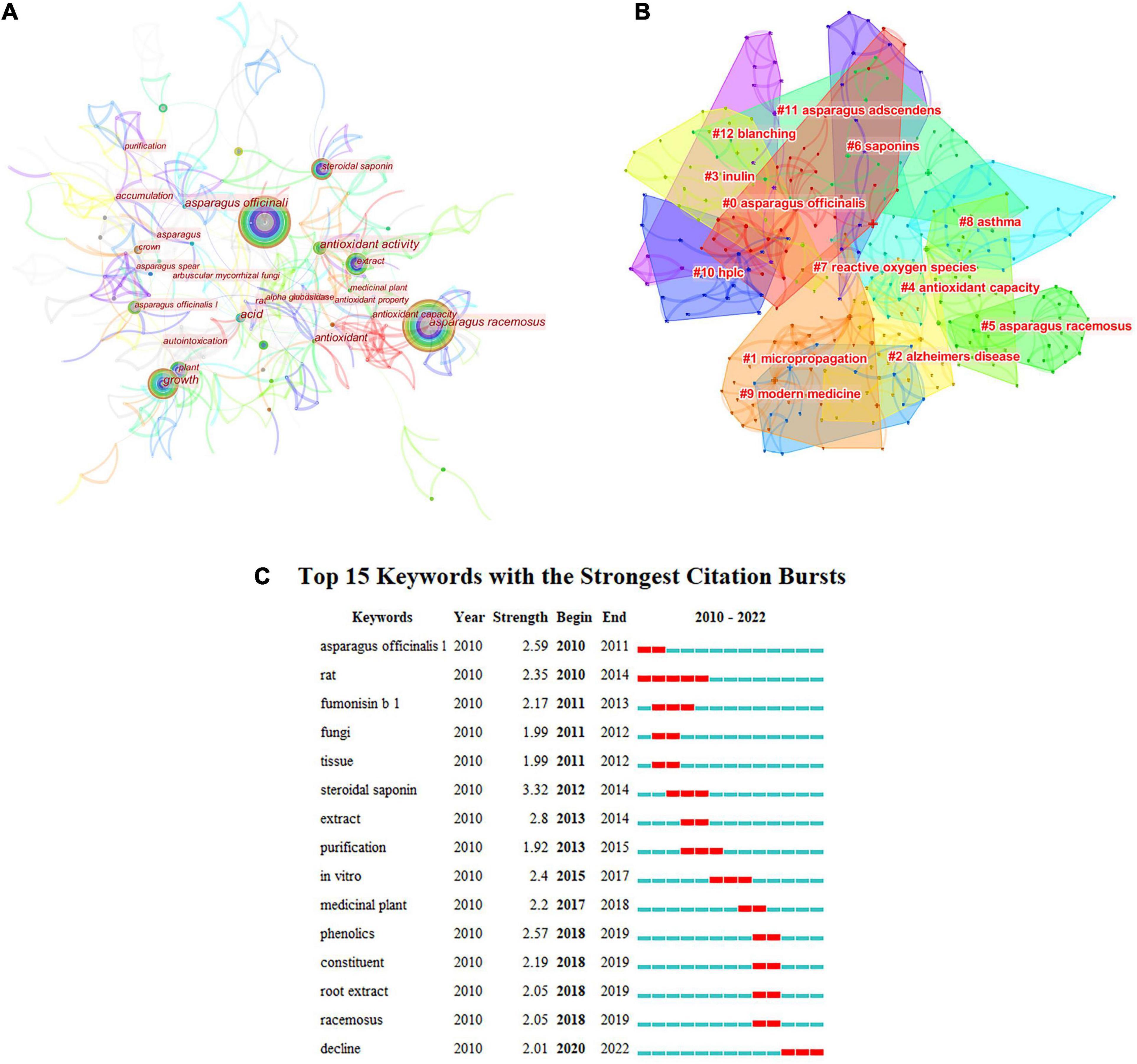
Figure 4. Analysis of keywords. (A) Keyword node map of asparagus; (B) clustering map of asparagus; (C) keyword emergence map.
Figure 4B shows the cluster analysis of AR keywords in the WOS database. Keyword cluster analysis can further explain the internal relationship between keywords. Through cluster calculation, q = 0.8203 > 0.3 and s = 0.9328 > 0.7 show effective clustering. Among the 11 clustering modules, the modules related to sturgeon and sturgeon names were removed. AR research has focused on AR bioactivities, such as antioxidants.
Figure 4C shows the emergence analysis of keywords for AR research in the WOS database. From the emergence of keywords, it can be seen that more asparagus plants (rats, fumonisin, fungi, and so on) were studied in the early stage, and keywords such as root extract and phenolics have gradually appeared since 2018. This also shows that research on asparagus is limited.
3. Taxonomy of asparagus
Asparagus is a perennial flowering herb belonging to the genus Asparagus, similar to Cousins leek and garlic in the Liliaceae family (2). The Asparagus shoots are classified according to color to white (grown in the absence of light) or green (developed in the presence of light). Generally, asparagus can rise to 100–150 cm and have a stout stem. Asparagus has feathery needle-like leaves. The plant attains a 6–32 mm long and 1 mm wide rosette, clustered of 4–15. Its root type is indeterminate and fascicular. The flower looks bell-shaped, greenish-white to yellowish, 4.5–6.5 mm long, and with six flowers fused at the bottom. They are usually individuals or two or three clusters at the junction of branchlets. They are typically dioecious but sometimes monoecious. The fruits are small red berries (approximately 6–10 mm in diameter) and poisonous to humans. The taxonomy of Asparagus can be summarized as follows:
Kingdom: Plantae
Phylum: Angiospermae
Class: Monocotyledoneae
Tribe: Asparagaceae
Suborder: Lily suborder
Order: Liliaceae
Family: Liliaceae
Genus: Asparagus
Species: Asparagus officinalis
Distribution: China, Germany, France, Spain, United States, Japan
4. Traditional health benefits of asparagus extract
Many bioactive compounds in asparagus may have health benefits (6, 7). In plants, bioactive compounds may impact numerous functions, such as flavor, color development, and defense systems against ultraviolet (UV) radiation (1). In particular, saponins, polyphenols, and flavonoids have been reported to have health benefits, as summarized in Table 2. For example, it was reported that there are antiglycation (7) and anticancer activities (6) for bioactive compounds from A. racemosus roots and antinociceptive and antioxidant effects for A. officinalis roots (8).
Three species of asparagus, including A. cochinchinensis, A. officinalis, and A. racemosus, have been widely studied for their health benefits (6). Rodríguez et al. (20) concluded that most bioactive compounds in asparagus are mainly located in the lower portion of the plant. The majority of published articles on A. officinalis (4, 21–23) have predominantly focused on the plant spears (stems) rather than roots as a source of bioactive compounds, as a spear is an edible part and A. officinalis is the only species that is commonly eaten. On the other hand, due to its long history of use in folk and traditional medicine, A. racemosus has been extensively investigated as a medicinal plant (15).
5. Phytochemistry of asparagus root
Phytochemical compounds, such as ferulic acid, isoferulic acid, malic acid, citric acid, asparagusic acid, caffeic acid, and fumaric acid (Figure 5), are typically extracted from dried A. officinalis roots (24, 25). The plant’s origin, species, and portions are important factors when investigating bioactive compounds. Different species have different profiles and quantities of bioactive compounds, and different plant sections contain different concentrations (26). A. officinalis roots are a valuable source of fructooligosaccharides (1), which may be isolated and characterized by mass spectrometry (MS), high-performance liquid chromatography (HPLC), NMR, and TLC (24). Furthermore, although common phytochemicals are readily detected via classical liquid chromatography, more complex compounds would require gas chromatography–mass spectrometry (GC-MS) for analysis (25). For example, A. racemosus roots (ARs) extract subjected to GC-MS analysis showed the presence of 2- furancarboxaldehyde, 1,2-dithiolane-3-carboxylic acid [synonyms: tetranorlipoic acid], 1,6-anhydro-β-d-talopyranase, tetradecanoic acid, n-hexadecanoic acid, oleic acid, 4 H pyran- 4 one, 2,3 dihydro–3,5 dihydroxy–6 methyl, and 9,12- octadecadienoic acid (27). Janani and Singaravadivel (28) confirmed compounds 2-furancarboxaldehyde, 1,2-dithiolane-3-carboxylic acid, 1,6-anhydro-β-d-talopyranase, tetradecanoic acid, n-hexadecanoic acid, oleic acid, 4 H pyran-4 one, 2,3 dihydro-3,5 dihydroxy-6 methyl, and 9,12-octadecadienoic acid in ARs. These results from various studies suggest that the source of AR specifically influences the composition of phytochemical compounds in the roots of various asparagus species and ARs. In another study, the phytochemical compounds found in the ethanol extract of ARs were investigated by Ravishankar et al. (29). The determined compounds were alkaloids, carbohydrates, glycosides, phenolic compounds, tannins, saponins, steroids, and flavonoids. No protein was found in the AR extract. The authors demonstrated that different compounds were extracted in different extraction solvents. For instance, alkaloids, tannins, and proteins were present in the aqueous extracts, whereas steroids and saponins were predominantly found in alcoholic extracts of ARs. Different extraction solvents could solvate different phenolic compounds from ARs, affecting the biological activity of the extracts, e.g., the antibacterial activity (8). Many bioactive compounds (such as steroids, phytosterols, carbohydrates, tannins, anthraquinones, saponins, glycosides, flavonoids, terpenoids, amino acids, and alkaloids) were reported by Ahmad et al. (7) from aqueous extracts of ARs. The results mentioned above suggest that most bioactive compounds in asparagus roots can be dissolved in strongly polar solvents. Furthermore, it appears that the same AR species contain different compositions of bioactive compounds due to regional effects of climate and other environmental factors (30). It is well-documented that biosynthesis of phenolic compounds occurs during photosynthesis, implying that sunshine exposure could affect the composition and amount of individual phenolics. Thus, environmental effects may be a major factor in the bioactive composition in AR. In this context, Dhwaj and Singh analyzed the methanol extracts of ARs and provided a very comprehensive profile of bioactive compounds, e.g., alkaloids, carbohydrates, glycosides, phenolic compounds, tannins, flavonoids, amino acids, proteins, steroids, terpenes, gum, and mucilage (31). Similarly, Sharma et al. isolated and identified a new steroidal sapogenin molecule from ARs and tested its bioactivity in an immune-suppressed animal model that highlighted its potent immunostimulant activity (32). The type of saponins and their composition vary with the asparagus root variety. For instance, Sautour et al. isolated and identified six new steroidal saponins (1–6) from A. acutifolius L. roots and evaluated their antifungal activity against human pathogenic yeasts, including Candida albicans, C. glabrata, and C. tropicalis, and noticed a very high activity with a minimum inhibitory concentration (MIC) between 12.5 and 100 μg/ml. Various techniques have been used to purify and identify bioactive compounds in AR (24). Pahwa and Goel. detected 3-heptadecanone, 8-hexadecenoic acid, methyl pentacosanoate, tetratriacontane, tritriacontane, methyl palmitate, tetracosyl tetracosanoate, palmitic acid, stearic acid, asparanin C, asparanin D, asparoside C, asparoside D, 3-β-O-{β-D-2-tetracosylxylopyranosyl}-stigmasterol, 3-β-O-{β-d- glucopyranosyl (1–2)-α-l-arabinopyranosyl}-stigmasterol, β-sitosterol-β-D-glucoside, shatavarin IV, stigmasterol, sarsasapogenin, xanthophyll, ascorbic acid, α-tocopherol, and β-carotene in A. adscendens Roxb roots by TLC and high-performance thin-layer chromatography (HPTLC) (33). Sharma et al. (34) identified shatavaroside A/B and 3-0-[α-L-rhamnopyranosyl-(1→2)-α-L-rhamnopyranosyl-(1→4)-0-β-D-glucopyranosyl]-25(S)-5β spirostan-3β-ol as potent immunostimulant compounds from ARs. In another species (35), investigated aspastipuline and 5-hydroxyaspastipuline from A. stipularis Forssk roots. Aspastipuline exhibited remarkable cytotoxic activity against the MCF-7 cell line and displayed moderate antiproliferative activity with an IC50 (half-maximal inhibitory concentration) value of 4.7 μM. The details mentioned above indicate that a wide range of bioactive compounds with multiple biological functions could be found in AR. This bioactive compound varies in terms of existence and quantity among various ARs due to ecological and genetic factors.
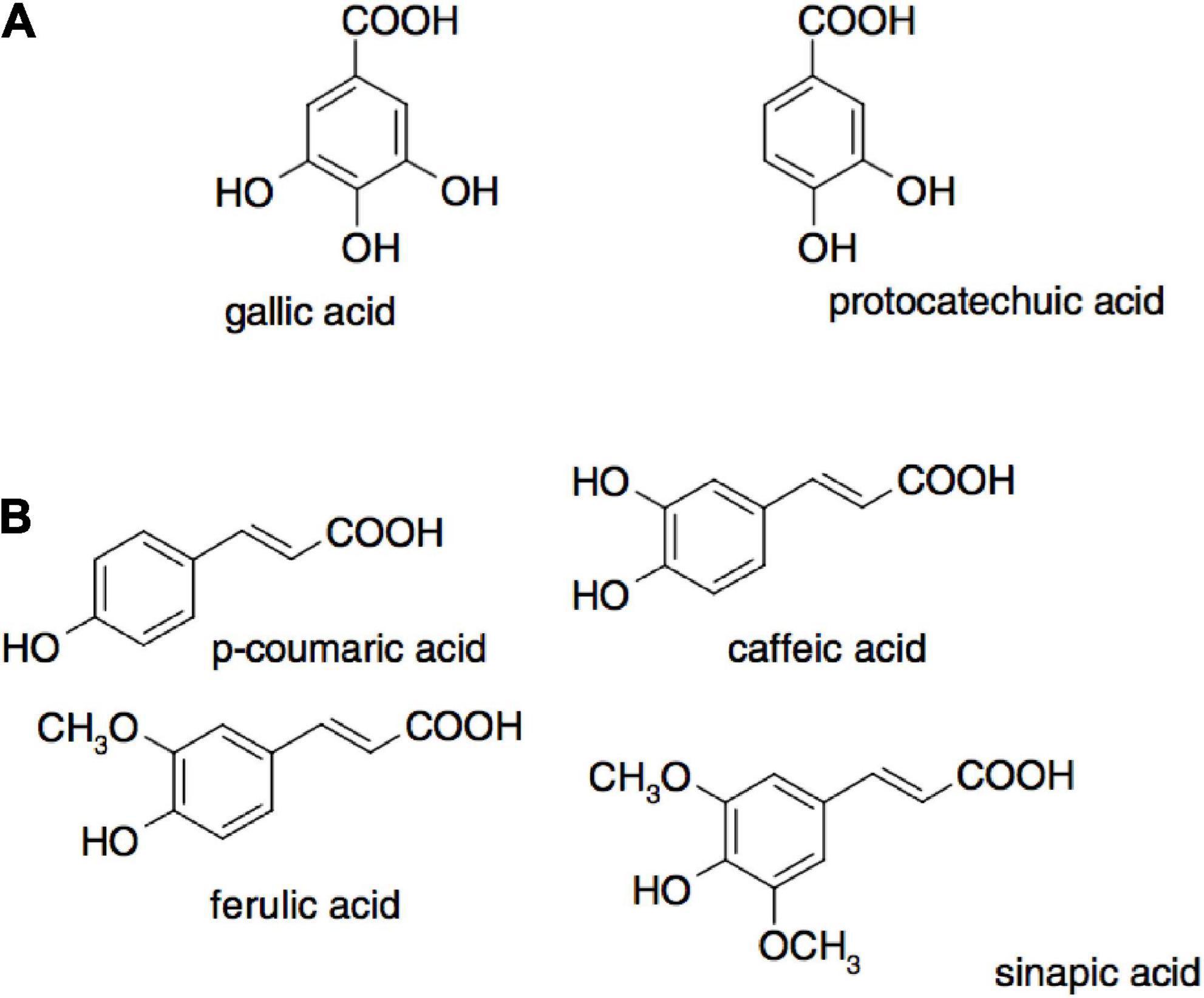
Figure 5. Examples of phenolic acids where (A) are hydroxybenzoic acids and (B) are hydroxycinnamic acids (38).
6. Bioactive compounds in asparagus
Polyphenols are ubiquitous and are present in plants. They possess various physiological activities, including antiatherogenic, antialgenic, antimicrobial, anti-inflammatory, antithrombotic, and vasodilatory and cardioprotective effects (36). Therefore, there is much interest in plants containing polyphenols, as they can provide several beneficial effects on health attributed to their antioxidant activity (7, 8).
The antioxidant activity of polyphenols is attributed to their ability to donate hydrogen, scavenge free radicals, and chelate metal ions (36). The activity depends on their structure, including the positions and number of hydroxyl groups compared to the carboxyl functional group (37). The main classification of polyphenols includes flavonoids, phenolic acids, tannins, stilbenes, and lignans. The in vivo antioxidant activities from asparagus roots are shown in Table 3, and examples of phenolic acids (hydroxybenzoic acids and hydroxycinnamic acids) that exhibit antioxidant activity are presented in Figure 5 (38).
The most consumed polyphenols in the human diet are flavonoids. They have a basic structure of a flavan nucleus with 15 carbon atoms arranged in three rings (C6-C3-C6) (39). Depending on the oxidative state of the central C ring, flavonoids can be categorized into five subgroups: flavones, flavonols flavanones, flavanols, and anthocyanins (Figure 6) (38). The antioxidant activity depends on their structure and the availability of hydroxyl groups, which allows them to scavenge radicals. It must be noted that the separation of flavonoids is challenging, as they have similar structures (36).
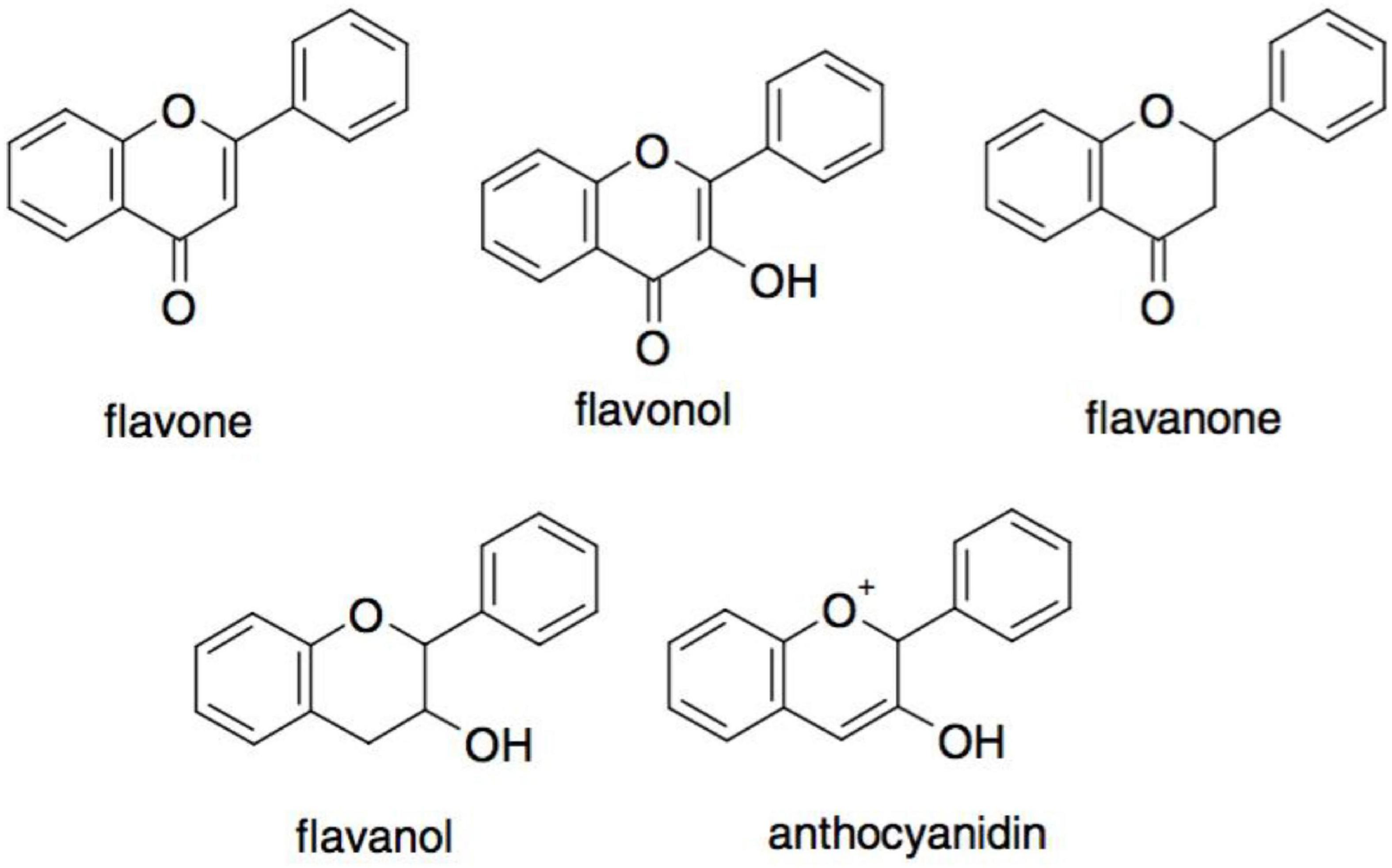
Figure 6. Different major subclasses of flavonoids (38).
6.1. Polyphenols and flavonoids in asparagus
The phenolic and flavonoid contents in asparagus have been investigated in the literature. Asparagus contains different polyphenols, including hydroxycinnamic and ferulic acids, in moderate amounts as determined by HPLC analysis (51). In this context, Nile and Park (22) found that ARs had a higher polyphenol content of 28.17 GAE/100 g compared to other medicinal plants, such as Butea monosperma, Withania somnifera, Tephrosia purpurea, Vitex negudo, and Plumbago zeylanica, with polyphenol contents of 24.07 GAE/100 g, 24.02 GAE/100 g, 18.02 GAE/100 g, 12.45 GAE/100 g, and 10.21 GAE/100 g, respectively. This result may indicate that asparagus roots are a good source of polyphenols. Furthermore, some studies have investigated the association between polyphenols and the antioxidant activity of asparagus and found good agreement between the total polyphenols (as determined by HPLC and colorimetric assays) and the antioxidant activity (37).
Solana et al. (52) investigated the flavonoid and phenolic compounds in whole A. officinalis and found that rutin is the most abundant phenolic compound. Rutin, also known as sophorin, quercetin-3-rutinoside, and ruthenium, is similar to saponin structures because of its aromatic configuration. Rutin is a glycoside hydrolyzed into aglycone and quercetin by enzymes in the human gut microbiota (53). Rutin has been shown to improve colitis, modulate the signaling of tumor necrosis factor-alpha and nuclear factor kappa B activity, reduce myeloperoxidase activity and modulate the levels of proinflammatory cytokines (54).
Caffeic acid was found to be the main phenolic compound in the methanol extract of fresh A. officinalis roots. A wider array of bioactive compounds, including 1,3-0-di-trans-p-coumaroylglycerol (1.7 mg/100 g extract), tetracosanoic acid (12.1 mg/100 g extract), 4′7-dimethylkaempferol (4.9 mg/100 g extract), rutin (211.3 mg/100 g extract), quercetin (7.0 mg/100 g extract), L-asparagine (3.7 mg/100 g extract), caffeic acid (12.0 mg/100 g extract), ferulic acid (5.9 mg/100 g extract), and inosine (5.3 mg/100 g extract), were characterized in A. officinalis roots by HPLC and NMR (55).
6.2. Lignans, isoflavones, and saponins
AR contains phytonutrients, such as lignans, isoflavones, and saponins, that have health benefits. These phytonutrients may prevent the formation of cancerous tumors, lower blood pressure, reduce the risk of stomach ulcers and slow down the degeneration of cells (39). Phytoestrogens are naturally occurring polycyclic phenols found in AR. There are two classes of phytoestrogens in ARs: isoflavones and lignans (56). Flavonoids may partly contribute to breast cancer prevention due to their antiestrogenic properties. Huang et al. identified rutin and quercetin in A. officinalis roots (55). In this study, rutin was obtained for the first time. On the other hand, lignans are dimers of phenylpropanoid (C6-C3) units linked by the central carbons of their side chains. In this context, Huang et al. (56) first separated four lignan types, (+)-nyasol, 3′-methoxynyasin, syringaresinol-4′, 4″-0-bis-β-D-glucoside, and syringaresinol-4-0-β-D-glucopyranoside, from A. officinalis roots.
AR also provides opportunities to develop value-added food products that enhance health benefits. Li et al. (57) found that the total saponin content in A. officinalis roots was 3.6 times higher than that in A. officinalis spears. Moreover, Shatavarin saponin glycosides from ARs have shown moderate protection against Cu+-induced oxidation of human low-density lipoprotein (LDL) with no inhibition of lipid peroxidation as determined by thiobarbituric acid reactive assay (TBARs) (58). Isoflavones, asparagamine, racemosol, polysaccharides, and mucilage were also reported by Chawla et al. (59).
Saponins naturally occur as glycosides in plant tubers, roots, leaves, and seeds. Over 100 plant families contain saponins, and studies have shown that at least 150 types of saponins have significant anticarcinogenic properties (60). Saponins are characterized by soap-like foaming properties and their sweet or bitter taste (depending on the saponin type) (61). Depending on their chemical structure, saponins can be grouped under many classifications: tirucallanes, dammaranes, hopanes, lupanes, taraxasternaes, oleananes, cycloartanes ursanes, lanostanes, steroidal compounds, and cucurbitanes (Figure 7) (62). These different classifications of saponins are due to other positions, types, and amounts of sugar moieties attached via a glycosidic link. Hence, the hydrophobic and hydrophilic components of the compound are responsible for the ability of saponins to have soap-like properties.
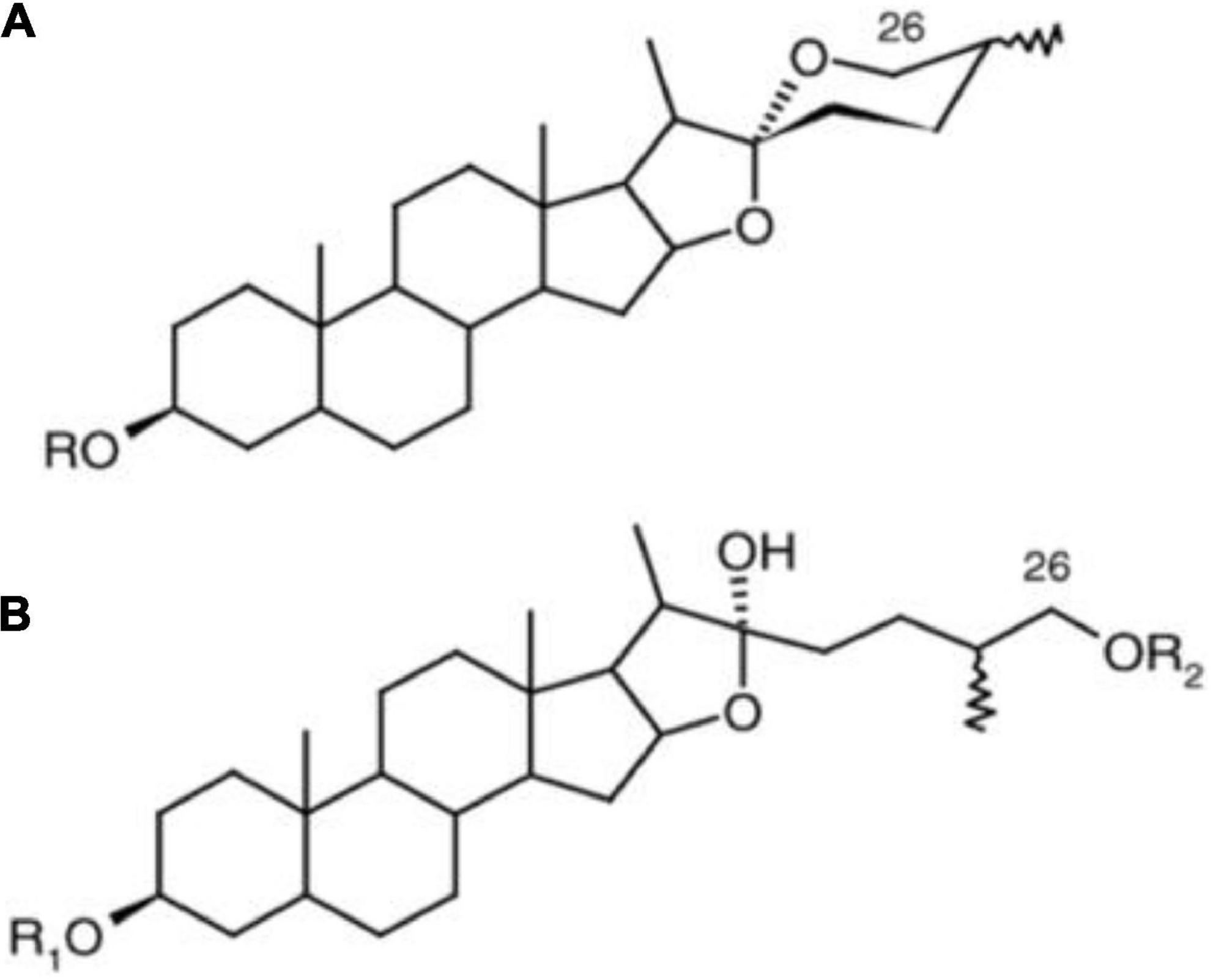
Figure 7. Different structures of steroidal saponins (A) steroidal spirostane and (B) steroidal furostane, where R = sugar moiety (62).
6.2.1. Saponins health properties
Saponins can act as anti-carcinogens as they force cancerous cells to undergo apoptosis (60). Apoptosis is a natural form of cell death, as it instructs the cell to self-destruct. This is different from necrosis, which is caused by external factors and has adverse side effects. Therefore, saponins have been seen as ideal candidate drugs to treat cancer. Saponins appear to prevent a wide range of cancers, as highlighted in Table 2. The literature has described saponins as vital in folk medicine (61). They provide antioxidant, anti-osteogenic, and anti-inflammatory effects.
6.2.2. Saponins in asparagus
In asparagus, the main type of saponin present is steroidal (60). Steroidal saponins contain a steroidal aglycone with a 27-carbon chain skeleton in a six-ring structure. In some plant sources, in the 26th position, the hydroxyl group is occupied in a glycosidic bond, maintaining the aglycone structure as pentacyclic (63). These structures are shown in Figure 8.
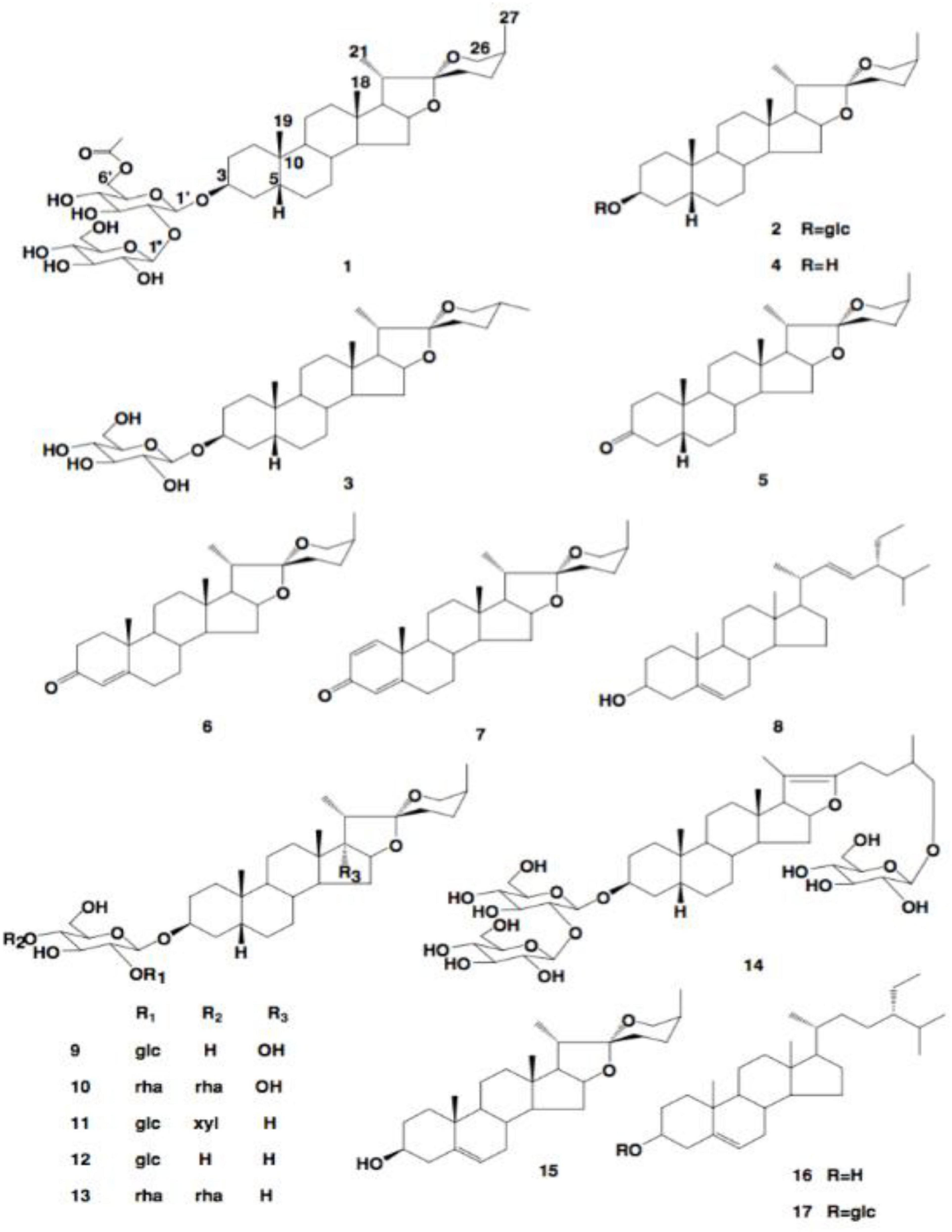
Figure 8. The structures of saponins 1–18 (63).
There is a vast amount of research on the health benefits of saponins extracted from asparagus. A potential reason for the wide range of health benefits could be the ability of saponins to interact with reactive oxygen species (64). As saponins exhibit aglycone moieties, many different saponins are present in the asparagus root and spear (60). Huang et al. found seventeen other saponins in A. officinalis roots (Table 4) (55). They also determined the structures of these saponins. These structures are presented in Figure 8. Saponins 1–17 have an aromatic configuration with different side chains depending on the type of compound. Huang et al. also investigated the in vitro cytotoxicity of these saponins against various cancer cells (65). Specifically, saponin 1 had an IC50 value of 5.50 μM against a nasopharyngeal CNE cancer cell line; saponin 11 had an IC50 value of 1.46 μM against a mouse leukemia L1210 cell line; and saponin 12 was effective against a wide range of cancer cells, including esophageal Eca-109, leukemia L1210, gastric MGC-803, nasopharynx KB, and lung LTEP-a-2 cells, with IC50 values of 4.03, 4.32, 3.72, 1.38, and 3.16 μM, respectively. Therefore, saponins 1, 11, and 12 had significant inhibitory effects against tumor cell proliferation, and saponins 2, 3, 4, 6, and 13 inhibited tumor cells moderately (55). Lee et al. (66) investigated the cytotoxic effects of A. cochinchinensis root extracts and found a new saponin, methyl protodioscin (18), responsible for inhibiting A549 lung cells with an IC50 of 59.1 μM. This saponin was also found to reduce cholesterol levels in rats.
6.3. Minerals value in asparagus root
Trace elements such as Ca, Mg, Fe, copper (Cu), and zinc (Zn) are present in AR (81). The trace elements from AR are summarized in Table 5. Several studies have investigated the trace elements in AR cultivars, including A. officinalis, A. racemosus, and A. curillus roots, such as Mg, P, potassium (K), and sodium (Na) consumed at levels of >100 mg/day for a healthy body (Table 6). In contrast, microminerals such as Cu, Fe, Zn, cobalt (Co), and manganese (Mn) are only needed in minimal quantities (<100 mg/day) (82). It was found that the concentration of trace elements was influenced by different altitudes and parts of the entire plant (83). Negi et al. reported that A. curillus (Buch.-Ham.) ex-Roxb roots exhibited the highest concentrations of Zn, Cu, Na, K, Ca, and Li, but Mn, Fe, and Co were the highest in A. curillus leaves (84). Meanwhile, the concentrations of Cu and Mn were low, Zn, Co, Na, and lithium (Li) concentrations were moderate, and K, Ca, and Fe concentrations were very high in A. curillus roots collected from four different altitudes in three seasons. Therefore, the results show that AR is a promising source of extracts that can treat many diseases (85). In another study, Li et al. reported that Ca in A. officinalis roots was 7.5 times higher than that in A. officinalis spears. In comparison, the content of Fe in A. officinalis roots was 15 times higher than that in A. officinalis spears (86). The presence of Ca in the AR powder correlates with the analytical results for galactagogues.
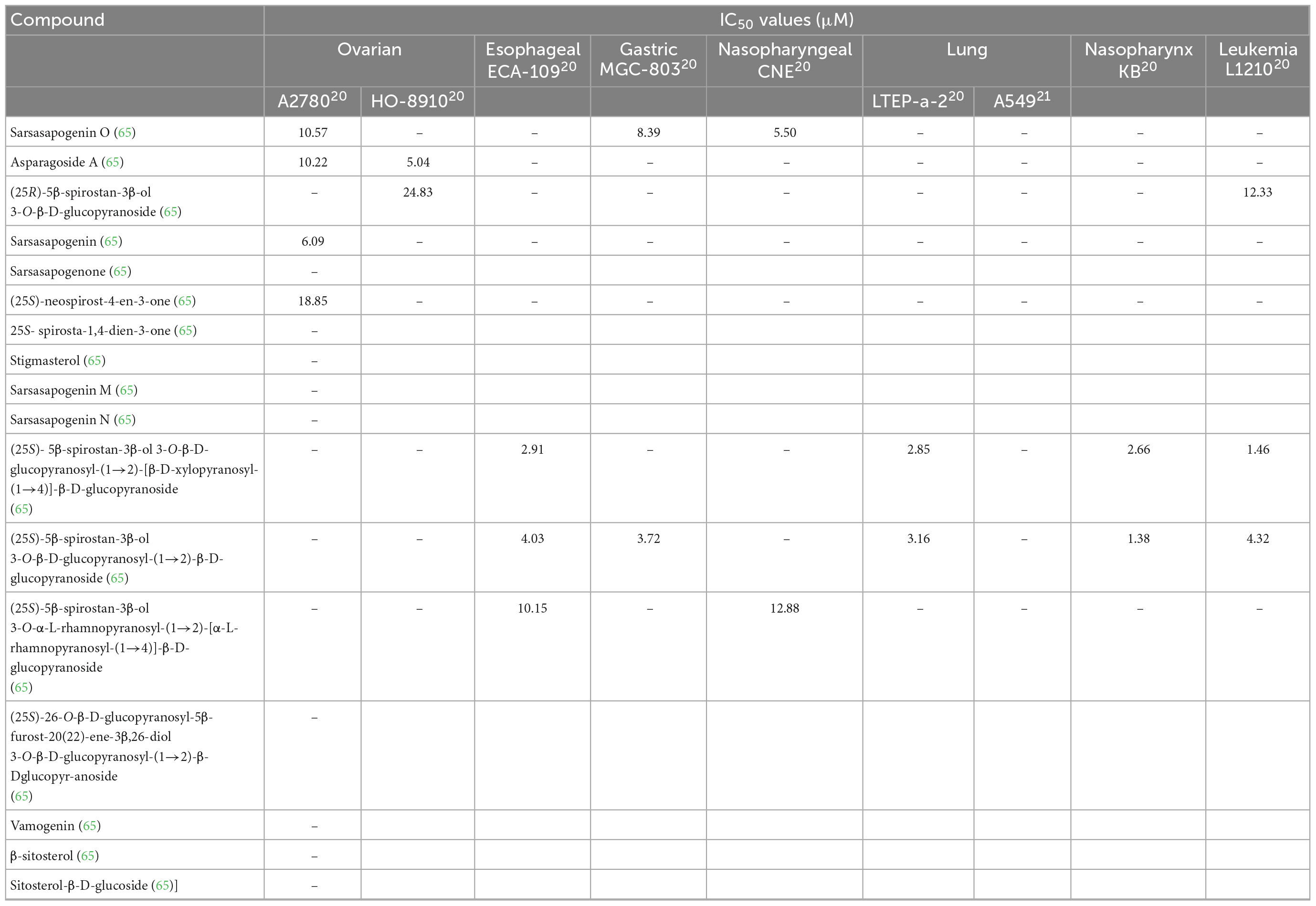
Table 5. Cytotoxicity of saponins and minerals obtained from A. officinalis and A. cochinchinensis roots.
7. Pharmacological activities
7.1. Protection against stomach ulcers
Stomach ulcers, commonly known as peptic ulcers (including gastric and duodenal ulcers), are common gastrointestinal disorders affecting millions of people. It is rarely fatal (89). Many herbal drugs are used as ayurvedic preparations and in Indian traditional medicinal systems to manage peptic ulcers (90). The methanolic extract of A. racemosus root indicated antisecretory. Antiulcer activity against a non-steroidal anti-inflammatory drug (indomethacin) and pyloric ligation (PL) that induced gastric ulcers in rats showed that crude extracts significantly reduced total acidity, free acidity, and gastric secretion at doses of 25–100 mg/kg for 5 days and protected rats considerably against duodenal ulcers at a dose of 50 mg/kg, twice daily (91). This suggests that A. racemosus roots have enhanced mucosal defensive factors, including the life span of cells, mucus secretion and cellular mucus, and antioxidant effects.
7.2. Insulin secretory activity (antidiabetic activity)
Herbal medicines are cheaper than modern synthetic drugs for treating widespread and rapidly growing diseases. The evaluation of more herbal plants for the treatment of diabetes is increasing. A study by Hannan et al. (92) reported that the ethanol extract of A. racemosus root significantly improved insulin secretion from the perfused pancreas. Therefore, A. racemosus root might be used to treat diabetes in clinical settings (93).
7.3. Hypolipidemic activity
Coronary heart diseases are caused by plasma cholesterol, high low-density lipoprotein-cholesterol, and triglyceride levels in the blood, with hyperlipidemic/hypercholesterolemia constituting the major risk factor for atherosclerosis and cardiovascular diseases (94). Cholesterol, along with the generation of reactive oxygen species (ROS), raises serum lipid levels and plays a vital role in the causation of coronary artery disease (CAD) and atherosclerosis. The roots of asparagus have been shown to reduce cholesterol levels (95). To investigate the antihypercholesterolemia activity of A. racemosus roots, a previous study induced hypercholesterolemia in 3-month-old male albino rats with an atherogenic diet, including 0.75 g% cholesterol and 1.5 g% bile salt in a regular diet (43). It was concluded that hyperlipidemia treated at 5 and 10 g% doses of AR powder for 4 weeks led to significantly increased plasma high-density lipoprotein (HDL) fractions (P < 0.05, 19–27%) and decreased total plasma lipid (TL) (14–21%), total cholesterol (TC) (25–35%), low-density lipoproteins (LDL) (32–45%) and AI (atherogenic index) (37–49%) compared to the standard drug atorvastatin. In addition, AR root enhanced the concentrations of SOD, hepatic catalase, and ascorbic acid in hypercholesteremic rats, which might be due to the presence of bioactive compounds, including steroids, flavonoids, glycosides, alkaloids, and phenolic compounds, as confirmed by HPTLC (59).
7.4. Antiurolithiatic activity
Ethylene glycol is formed at high concentrations of these ions (calcium, phosphate, and oxalate), contributing to renal stone formation. For example, the ethanolic extract of A. racemosus root significantly reduced the elevated creatinine levels, calcium, oxalate, and phosphate ions in the urine of adult male albino Wistar rats fed 0.75% ethylene glycol water. It also increased the urinary level of magnesium, a crystallization inhibitor. These results were supported by histopathological findings that presented signs of improvement after treatment with the ethanolic extract (44).
7.5. Effect on diuretic activity
ARs treat acute toxicity and diuretic activity (45). A decoction of AR treated urinary tract infections in ancient times (96). Evaluation of acute toxicity and diuretic activity was carried out by feeding Wistar albino rats 800, 1,600, and 3,200 mg/kg AR water extract (45). The Lipschitz test evaluated the diuretic activity compared with the standard drug furosemide. Urine was collected by housing each animal in separate metabolic cage output at 24 h and was analyzed by colorimetry. The urine electrolytes increased significantly in P+, K+, and Cl– concentrations at 3,200 mg/kg body weight, higher than the standard drug furosemide. It was also evaluated that animals showed no behavioral, autonomic, or central nervous system changes even at the maximum single dose of 3,200 mg/kg. Phytochemical screening of ARs revealed steroidal saponins, shatavarins I, II, III, and IV, and isoflavones (72).
7.6. Antitussive activity
In another study, A. racemosus roots were tested for antitussive activity. Jaiswal et al. (97) prepared a methanol extract of A. racemosus roots at 200 and 400 mg/kg doses, which was used to recover sulfur-induced coughing in Wistar albino mice. The authors also produced 40 and 58.5% inhibition of sulfur-induced cough at doses of 200 and 400 mg/kg compared with the inhibition of codeine phosphate of 36 and 55.45% at 10 and 20 mg/kg doses. Therefore, A. racemosus roots showed significant antitussive activity at 200 and 400 mg/kg (97).
7.7. Anti-dyspepsia and antidiarrheal activity
Asparagus racemosus is also used in Ayurveda for treating dyspepsia because it possesses an effect comparable to the allopathic drug metoclopramide. Metoclopramide is used to decrease gastric emptying time in patients diagnosed with dyspepsia (48). Diarrhea occurs widely in developing countries and is a fatal disease, killing approximately 2.2 million people per year worldwide, most of whom are infants and children below the age of 5 years (49). Therefore, ethanolic and aqueous extracts of ARs were investigated for antidiarrheal activity against castor oil-induced diarrhea in rats compared to Loperamide (a traditional medicine for treating diarrhea as a positive control) (49). The release of ricinoleic acid from castor oil results in inflammation and irritation of the intestinal mucosa, causing prostaglandins, which stimulate motility and secretion. It is well-known that prostaglandin E causes diarrhea in experimental animals and human beings. Therefore, ethanolic and aqueous extracts of ARs could be attributed to the inhibition of prostaglandin biosynthesis, which in turn inhibits gastrointestinal motility and secretion depending on the composition of saponins, alkaloids, flavonoids, sterols, and terpenes in ARs (96).
7.8. Neuroprotective action
Neuronal degeneration plays a vital role in the specific area of the brain in neurodegenerative disorders and the development of the nervous system. Methanolic extract of ARs was tested against kainic acid-induced striatal neuronal and hippocampal damage in mice at 18 mg/kg. After treatment, 10.81–14.0 and 10.0 nmol NADPH oxidized/mg protein in the hippocampus and striatum, respectively, were obtained in glutathione peroxidase (GPx) assays, and 0.83–1.92 and 1.17–2.31 nmol NADPH oxidized/mg protein in the hippocampus and striatum, respectively, were obtained in the glutathione GSH assay. This result suggested that the methanolic extract of ARs plays the role of an antioxidant by attenuating free radical-induced oxidative damage (46). Another study investigated the neuroprotective effects of a methanolic extract from ARs in Swiss albino mice and clinical patients (98). The results demonstrated that cytological effects were significantly higher in the total normal cells of various regions of the hippocampus (CA1, CA3, CA4, and Dg) in the order Dg > CA4 > CA1 > CA3 compared to the control. In a further study, evaluating the neuroprotective effects of ethanolic extracts from ARs significantly reduced ethanol-induced oxidative stress and cognitive impairment in Swiss albino male rats at 100 and 200 mg/kg b.w. (P < 0.05, P < 0.01), decreased retention transfer latency (RTL), escape latency (EL) and AChE (acetylcholinesterase) activity in the EPM, MWM and TBARS level tests after 21 days. Furthermore, in passive avoidance (PA), novel object recognition (NOR) testing and biochemical studies, such as lipid peroxidation (TBARS) content and AChE tests, ethanolic extract from A. racemosus roots markedly increased regency latency (RL), DI (discrimination index) and TSTQ (target quadrant) and time spent in the annuli (TSA) at a dose of 200 mg/kg b.w. (P < 0.05, P < 0.01) on days 11 and 21 compared to the disease control (99).
7.9. Miscellaneous
Asparagus root has been used for over 2,000 years as a medicine prescription (8). AR was used to produce commercial medicinal products in the modern era for clinical settings. Cancer patients taking medications containing AR reported a significant improvement in health outcomes (100). In India, many highly processed asparagus root products and AR powder are popular in the public market (101). In a randomized controlled trial of lactating mothers with symptoms of deficient lactation, Gupta and Shaw found that the oral administration of ARs improved prolactin hormone levels more than threefold compared to the control group (13). No acute toxicity effects were detected. Another study showed improved quality of life for patients with advanced non-small cell lung cancer after treatment with Yiqi Yangyin Jiedu Decoration, a Chinese herbal recipe containing AR (102).
8. Potential toxicity of bioactive compounds in AR
Some studies have shown that AR might possess teratogenicity effects (103). For example, methanolic extracts of ARs (100 mg/kg/day for 60 days) showed increased resorption of fetuses, swelling in legs, and intrauterine growth retardation in Charles Foster rats (103). Considering these adverse effects observed in animal studies, AR might harm the human fetus and should be avoided during pregnancy Goel et al. (103).
An acute toxicity study by Kumar et al. (45) reported no fatalities in rats administered 800 mg/kg, 1,600 mg/kg, and 3,200 mg/kg of aqueous extracts of ARs. However, the authors reported significant diuretic activity when 3,200 mg/kg aqueous extracts of ARs were given. The ethanolic and aqueous extracts of AR did not show any toxic effects at doses ranging from 50 mg/kg to 1 g/kg b.w. for 4 weeks in Wistar albino rats (104).
9. Conclusion and future prospects
The genus Asparagus is becoming a more valuable plant because of its uses as folk medicine and food. AR is not as popular as asparagus spears because of incomplete research and insufficient evidence regarding their clinical applications. Various studies have concluded that the plant is rich in the alkaloid mimosine, which has anticancer properties. Further efforts are required to elaborate upon the anticarcinogenic effect of mimosine and its isolation at a commercial scale for therapeutic applications in humans. A large number of studies have been performed regarding its antivenom activity. Thus, different asparagus species might have the potential to be used as herbal medicines and functional foods. Furthermore, phytochemical studies have demonstrated the presence of various bioactive compounds as valuable secondary metabolites. The dominant class of bioactive compounds in AR is flavonoids. AR displayed significant pharmacological effects, such as antioxidant, antimicrobial, antiviral, anticancer, anti-inflammatory, and antidiabetic effects, as shown in studies conducted in animals and humans. Some phytochemical compounds have been identified and quantified through NMR, HPL, and TLC. We believe high-throughput techniques, such as Q-TOF-MS, would identify compounds that ordinary instruments might not recognize. This plant could supply a sound basis for developing therapeutic formulations of herbal medicine to treat various health ailments.
Author contributions
YG: conceptualization, design of the work, writing—review and editing, supervision, and funding acquisition. ZL: visualization, resources, and writing—review and editing. YW, YZ, and HA: conception or design of the work, resources, and investigation. MY: writing—review and editing and supervision. JP, TY, and XZ: data acquisition, formal analysis, conceptualization, and writing—review and editing. AIH and AMA: formal analysis and writing—review and editing. All authors contributed to the article and approved the submitted version.
Funding
This study was funded by the Special Support Plan for High-Level Talents in Shaanxi Province (for JP) and the Foreign Expert Project of the Ministry of Science and Technology, and the Key Research and Development Program of Shaanxi Province, China (2020NY-111).
Conflict of interest
The authors declare that the research was conducted in the absence of any commercial or financial relationships that could be construed as a potential conflict of interest.
Publisher’s note
All claims expressed in this article are solely those of the authors and do not necessarily represent those of their affiliated organizations, or those of the publisher, the editors and the reviewers. Any product that may be evaluated in this article, or claim that may be made by its manufacturer, is not guaranteed or endorsed by the publisher.
Abbreviations
AR, asparagus root; UV, ultraviolet; IC50, half maximal inhibitory concentration; T2D, type 2 diabetes; TLC, thin-layer chromatography; 1H-NMR, proton nuclear magnetic resonance; MS, mass spectrometry; HPLC, high-performance liquid chromatography; GC-MS, gas chromatography-mass spectrometry; ARs, Asparagus racemosus roots; RP, reversed-phase; LC-MS, liquid chromatography-mass spectrometry; MIC, minimum inhibitory concentration; HPTLC, high-performance thin-layer chromatography; HDL, high-density lipoproteins; TL, total lipid; TC, total cholesterol; LDL, low-density lipoproteins, GPx, glutathione peroxidase; ELISA, enzyme-linked immunosorbent assay; PL, pyloric ligation.
Footnotes
References
1. Siccama JW, Oudejans R, Zhang L, Kabel M, Schutyser M. Steering the formation of cellobiose and oligosaccharides during enzymatic hydrolysis of asparagus fibre. LWT. (2022). 160:113273. doi: 10.1016/j.lwt.2022.113273
2. Walters L. Asparagus Season Shoots Off. (2014). Available online at: http://www.stuff.co.nz/life-style/food-wine/10553507/Asparagus-season-shoots-off
4. Fuentes-Alventosa JM, Jaramillo-Carmona S, Rodríguez-Gutiérrez G, Guillén-Bejarano R, Jiménez-Araujo A, Fernández-Bolaños J, et al. Preparation of bioactive extracts from asparagus by-product. Food Bioprod Process. (2013) 91:74–82. doi: 10.1016/j.fbp.2012.12.004
5. Zhang H, Birch J, Ma ZF, Xie C, Yang H, Bekhit AED, et al. Optimization of microwave-assisted extraction of bioactive compounds from New Zealand and Chinese Asparagus officinalis L. roots. J Food Sci Technol. (2019) 6:799–810. doi: 10.1007/s13197-018-3540-0
6. Sharma A, Kumar A, Jaitak V. Pharmacological and chemical potential of Cassia fistula L- a critical review. J Herb Med. (2020) 26:100407.
7. Ahmad S, Pandey AR, Singh SP, Singh S, Sashidhara KV, Tamrakar AK. Antiglycation activity of β-glucogallin from Asparagus racemosus. Nat Prod Res. (2022) 36:6329–35. doi: 10.1080/14786419.2022.2025799
8. Fathalipour M, Delnavazi MR, Safa O, Zarifinia N, Rafiee B. Antioxidant and antinociceptive effects of hydroalcoholic root extract of Asparagus officinalis L. Physiol Pharmacol. (2020) 24:322–30. doi: 10.32598/ppj.24.4.30
9. Jashni HK, Jahromi HK, Ranjbary AG, Jahromi ZK, Kherameh ZK. Effects of aqueous extract from Asparagus officinalis L. roots on hypothalamic-pituitary-gonadal axis hormone levels and the number of ovarian follicles in adult rats. Int J Reprod Biomed. (2016) 14:75–80. doi: 10.29252/ijrm.14.2.75
10. Hafizur RM, Fatima N, Shaukat S. Immunohistochemical evidence of pancreatic [beta]-cell regeneration in streptozotocin-induced type 2 diabetic rats treated with Gymnema sylvestre extract. J Cytol Histol. (2015) 6:342. doi: 10.4172/2157-7099.1000342
11. Nishimura M, Ohkawara T, Kagami-Katsuyama H, Sato H, Nishihira J. Improvement of blood pressure, glucose metabolism, and lipid profile by the intake of powdered asparagus bottom-stems and cladophylls. J Tradit Complement Med. (2013) 3:250–5. doi: 10.4103/2225-4110.119728
12. Agrawal A, Sharma M, Rai SK, Singh B, Tiwari M, Chandra R. The effect of the aqueous extract of the roots of Asparagus racemosus on hepatocarcinogenesis initiated by diethylnitrosamine. Phytother Res. (2008) 22:1175–82. doi: 10.1002/ptr.2391
13. Gupta M, Shaw BA. Double-blind randomized clinical trial for evaluation of galactogogue activity of Asparagus racemosus willd. Iran J Pharm Res. (2011) 10:167–72.
14. Hossain MI, Sharmin FA, Akhter S, Bhuiyan MA, Shahriar M. Investigation of cytotoxicity and in-vitro antioxidant activity of Asparagus racemosus root extract. ICPJ. (2012) 1:250–7. doi: 10.3329/icpj.v1i9.11615
15. Jagannath N, Chikkannasetty SS, Govindadas D, Devasankaraiah G. Study of antiurolithiatic activity of Asparagus racemosus on albino rats. Indian J Pharmacol. (2012) 44:576–9. doi: 10.4103/0253-7613.100378
16. Jalsrai A, Numakawa T, Kunugi H, Dieterich D, Becker A. The neuroprotective effects and possible mechanism of action of a methanol extract from Asparagus cochinchinensis: in vitro and in vivo studies. Neuroscience. (2016) 322:452–63. doi: 10.1016/j.neuroscience.2016.02.065
17. Fuentes-Alventosa JM, Rodríguez-Gutiérrez G, Jaramillo-Carmona S, Espejo-Calvo JA, Rodríguez-Arcos R, Fernández-Bolaños J, et al. Effect of extraction method on chemical composition and functional characteristics of high dietary fiber powders obtained from asparagus by-products. Food Chem. (2009) 113:665–71. doi: 10.1016/j.foodchem.2008.07.075
18. Zhang H, Birch J, Pei J, Ma ZF, Bekhit AED. Phytochemical compounds and biological activity in Asparagus roots: a review. Int J Food Sci Technol. (2019) 54:966–77. doi: 10.1111/ijfs.13993
19. Lee DY, Choo BK, Yoon T, Cheon MS, Lee HW, Lee AY, et al. Anti-inflammatory effects of Asparagus cochinchinensis extract in acute and chronic cutaneous inflammation. J Ethnopharmacol. (2009) 121:28–34. doi: 10.1016/j.jep.2008.07.006
20. Rodríguez R, Jaramillo S, Rodríguez G, Espejo JA, Guillén R, Fernández-Bolaños J, et al. Antioxidant activity of ethanolic extracts from several asparagus cultivars. J Agric Food Chem. (2005) 53:5212–7. doi: 10.1021/jf050338i
21. Lee EJ, Yoo KS, Patil BS. Development of a Rapid HPLC-UV method for simultaneous quantification of protodioscin and rutin in white and green asparagus spears. J Food Sci. (2010) 75:C703–9. doi: 10.1111/j.1750-3841.2010.01824.x
22. Nile SH, Park SW. HPTLC analysis, antioxidant and antigout activity of Indian plants. Iran J Pharm Res. (2014) 13:531–9.
23. Samad NB, Debnath T, Hasnat A, Pervin M, Kim DH, Jo JE, et al. Phenolic contents, antioxidant and anti-inflammatory activities of Asparagus cochinchinensis (Loureiro) Merrill. J. Food Biochem. (2014) 38:83–91. doi: 10.1111/jfbc.12028
24. Chen G, Lin B, Zheng F, Yu W, Fang X, Shi Q, et al. Comparison of different drying methods for Asparagus [Asparagus cochinchinensis (Lour.) Merr.] root volatile compounds as revealed using gas chromatography ion mobility spectrometry. Front Nutr. (2022) 9:868209. doi: 10.3389/fnut.2022.868209
25. Yang C, Ye Z, Mao L, Zhang L, Zhang J, Ding W, et al. Analysis of volatile organic compounds and metabolites of three cultivars of asparagus (Asparagus officinalis L.) using E-nose, GC-IMS, and LC-MS/MS. Bioengineered. (2022) 13:8866–80. doi: 10.1080/21655979.2022.2056318
26. Fan R, Yuan F, Wang N, Gao Y, Huang Y. Extraction and analysis of antioxidant compounds from the residues of Asparagus officinalis L. J Food Sci Technol. (2015) 52:2690–700. doi: 10.1007/s13197-014-1360-4
27. Sivakumar T, Gajalakshmi D. Phytochemical screening and GC-MS analysis of root extract from Asparagus racemosus L. Int J Pharm Sci Res. (2014) 5:5245–9.
28. Janani S, Singaravadivel K. Screening of phytochemical and GC-MS analysis of some bioactive constituents of Asparagus racemosus. Int J Pharm Res. (2014) 6:428–32.
29. Ravishankar K, Kiranmayi GVN, Lalitha TM, Priyanka T, Ranjith T, Someswarao SBV, et al. Preliminary phytochemical screening and in vitro antibacterial activity on Asparagus racemosus root extract. Int J Pharm Chem Biol Sci. (2012) 2:117–23.
30. Kulczyński B, Kobus-Cisowska J, Kmiecik D, Gramza-Michałowska A, Golczak D, Korczak J. Antiradical capacity and polyphenol composition of asparagus spears varieties cultivated under different sunlight conditions. Acta Sci Pol Technol Aliment. (2016) 15:267–79. doi: 10.17306/J.AFS.2016.3.26
31. Dhwaj AV, Singh R. Reversal effect of Asparagus racemosus wild (liliaceae) root extract on memory deficits of mice. Int J Drug Dev Res. (2011) 3:314–23.
32. Sharma P, Chauhan PS, Dutt P, Amina M, Suri KA, Gupta BD, et al. A unique immuno-stimulant steroidal sapogenin acid from the roots of Asparagus racemosus. Steroids. (2011) 76:358–64. doi: 10.1016/j.steroids.2010.12.006
33. Pahwa P, Goel RK. Asparagus adscendens root extract enhances cognition and protects against scopolamine induced amnesia: an in-silico and in-vivo studies. Chem Biol Interact. (2016) 260:208–18. doi: 10.1016/j.cbi.2016.10.007
34. Sharma U, Kumar N, Singh B, Munshi RK, Bhalerao S. Immunomodulatory active steroidal saponins from Asparagus racemosus. Med Chem Res. (2013) 22:573–9. doi: 10.1007/s00044-012-0048-4
35. Galala AA, Ahmad KF, Zaghloul MG, Mansour ESS. Two new alkaloids from Asparagus stipularis Forssk. roots. Phytochem Lett. (2015) 12:220–3. doi: 10.1016/j.phytol.2015.04.004
36. Xu N, Zhang H, Jia J, Li H, Zhu Z, Fu S, et al. Assessment of contents and health impacts of four metals in Chongming Asparagus—geographical and seasonal aspects. Foods. (2022) 11:624. doi: 10.3390/foods11050624
37. Adouni K, Bendif H, Zouaoui O, Kraujalis P, Flamini G, Rimantas P, et al. Antioxidant activity of extracts obtained by high-pressure extraction procedures from Asparagus stipularis Forssk. South Afr J Bot. (2022) 146:789–93. doi: 10.1016/j.sajb.2021.12.027
38. Balasundram N, Sundram K, Samman S. Phenolic compounds in plants and agri-industrial by-products: antioxidant activity, occurrence, and potential uses. Food Chem. (2006) 99:191–203. doi: 10.1016/j.foodchem.2005.07.042
39. Shahrajabian MH, Sun WL. Asparagus (Asparagus officinalis L.) and pennyroyal (Mentba pulegium L.) impressive advantages with wondrous health-beneficial phytochemicals. Not Sci Biol. (2022) 14:11212.
40. Bhatnagar M, Sisodia SS. Antisecretory and antiulcer activity of Asparagus racemosus Wild. against indomethacin plus pyloric ligation-induced gastric ulcer in rats. J Herb Pharm. (2006) 6:13–20. doi: 10.1080/J157v06n01_02
41. Hannan J, Ali L, Khaleque J, Akhter M, Flatt PR, Abdel-Wahab YH. Antihyperglycaemic activity of Asparagus racemosus roots is partly mediated by inhibition of carbohydrate digestion and absorption, and enhancement of cellular insulin action. Br J Nutr. (2012) 107:1316–23. doi: 10.1017/S0007114511004284
42. Patel DK, Prasad SK, Kumar R, Hemalatha S. An overview on antidiabetic medicinal plants having insulin mimetic property. Asian Pac J Trop Biomed. (2012) 2:320–30. doi: 10.1016/S2221-1691(12)60032-X
43. Visavadiya NP, Narasimhacharya AVRL. Hypolipidemic and antioxidant activities of Asparagus racemosus in hypercholesteremic rats. Indian J Pharmacol. (2005) 37:376–80. doi: 10.4103/0253-7613.19074
44. Christina AJM, Ashok K, Packialakshmi M, Tobin GC, Murugesh N. Antilithiatic effect of Asparagus racemosus wild on ethylene glycol-induced lithiasis in male albino Wistar rats. Methods find. Exp Clin Pharmacol. (2005) 27:633–8. doi: 10.1358/mf.2005.27.9.939338
45. Kumar MCS, Udupa AL, Sammodavardhana K, Rathnakar UP, Shvetha U, Kodancha GP. Acute toxicity and diuretic studies of the roots of Asparagus racemosus Wild in rats. West Indian Med J. (2010) 59:3–5.
46. Parihar MS, Hemnani T. Experimental excitotoxicity provokes oxidative damage in mice brain and attenuation by extract of Asparagus racemosus. J Neural Transm. (2004) 111:1–12. doi: 10.1007/s00702-003-0069-8
47. Mandal N, Samanta SK, ChakrabortyC. “Reply to the comment by marques et al. on: “numerical models of flow patterns around a rigid inclusion in a viscous matrix undergoing simple shear: implications of model parameters and boundary conditions” by N. Mandal, S.K. Samanta, C. Chakraborty.” J Struct Geol. (2006) 28:1375–78.
49. Venkatesan N, Thiyagarajan V, Narayanan S, Arul A, Raja S, Kumar SGV, et al. Anti-diarrhoeal potential of Asparagus racemosus wild root extracts in laboratory animals. J Pharm Pharm Sci. (2005) 8:39–46.
50. Jalalpure SS, Bagewadi V, Shaikh I. Antiepileptic effect of Asparagus racemosus root extracts. J Trop Med Plants. (2009) 10:157–61.
51. Alan O, Turk B, Sen F. A comparative study on the quality attributes, phenolic content and antioxidant activity of cultivated and wild asparagus as influenced by seasonal variations. J Agric Sci. (2022):1–10. S0021859622000569, doi: 10.1017/S0021859622000569
52. Solana M, Boschiero I, Dall’Acqua S, Bertucco A. A comparison between supercritical fluid and pressurized liquid extraction methods for obtaining phenolic compounds from Asparagus officinalis L. J Supercrit Fluid. (2015) 100:201–8. doi: 10.1016/j.supflu.2015.02.014
53. Amaretti A, Raimondi S, Leonardi A, Quartieri A, Rossi M. Hydrolysis of the rutinose-conjugates flavonoids rutin and hesperidin by the gut microbiota and bifidobacteria. Nutrients. (2015) 7:2788–800. doi: 10.3390/nu7042788
54. Rabišková M, Bautzová T, Gajdziok J, Dvořáčková K, Lamprecht A, Pellequer Y, et al. Coated chitosan pellets containing rutin intended for the treatment of inflammatory bowel disease: in vitro characteristics and in vivo evaluation. Int J Pharm. (2012) 422:151–9. doi: 10.1016/j.ijpharm.2011.10.045
55. Huang X, Luo J, Zhang Y, Kong LY. Chemical constituents of Asparagus officinalis. Chin J Nat Med. (2006) 4:181–4.
56. Huang X, Zhang Y, Kong L. Studies on lignan components of Asparagus officinalis. J. Chin. Pharm. (2007) 42:339–48.
57. Li J, Nie L, Liu M, Hu S. The study of asparagus different parts active ingredients. Food Res Dev. (2015) 36:10–2.
58. Onlom C, Phrompittayarat W, Putalun W, Waranuch N, Ingkaninan K. Immunoaffinity knockout of saponin glycosides from Asparagus racemosus to assess anti-lipid peroxidation. Phytochem Anal. (2017) 28:316–23. doi: 10.1002/pca.2678
59. Chawla A, Chawla P, Mangalesh RRC, Roy R. Asparagus racemosus (Willd): biological activities and its active principles. Indo Glob J Pharm Sci. (2011) 1:113–20. doi: 10.35652/IGJPS.2011.11
60. Zhu XL, Zhang W, Gao Y, Zhang GQ. The hypoglycaemic activity of saponins from Asparagus officinalis l. by-products in diabetic rats. Acta Nutr Sin. (2019) 41:270–5.
61. Liao Y, Li Z, Zhou Q, Sheng M, Shi X. Saponin surfactants used in drug delivery systems: a new application for natural medicine components. Int J Pharm. (2021) 603:120709. doi: 10.1016/j.ijpharm.2021.120709
62. Man S, Gao W, Zhang Y, Huang L, Liu C. Chemical study and medical application of saponins as anti-cancer agents. Fitoterapia. (2010) 81:703–14. doi: 10.1016/j.fitote.2010.06.004
63. Sparg SG, Light ME, Van Staden J. Biological activities and distribution of plant saponins. J Ethnopharmacol. (2004) 94:219–43. doi: 10.1016/j.jep.2004.05.016
64. George F, Zohar K, Makkar H, Klaus B. Reflections on ‘the biological action of saponins in animal systems: a review. Br J Nutr. (2022) 127:1034–6. doi: 10.1017/S0007114521004852
65. Huang X-F, Lin Y-Y, Kong L-Y. Steroids from the roots of Asparagus officinalis and their cytotoxic activity. J Integr Plant Biol. (2008) 50:717–22. doi: 10.1111/j.1744-7909.2008.00651.x
66. Lee W, Ku SK, Bae JS. Barrier protective effects of rutin in LPS-induced inflammation in vitro and in vivo. Food Chem Toxicol. (2012) 50:3048–55. doi: 10.1016/j.fct.2012.06.013
67. Yamamori A, Onodera S, Kikuchi M, Shiomi N. Two novel oligosaccharides formed by 1F-fructosyltransferase purified from roots of asparagus (Asparagus officinalis L.). Biosci Biotechnol Biochem. (2002) 66:1419–22. doi: 10.1271/bbb.66.1419
68. Zhang HJ, Sydara K, Tan GT, Ma C, Southavong B, Soejarto DD, et al. Bioactive constituents from Asparagus cochinchinensis. J Nat Prod. (2004) 67:194–200. doi: 10.1021/np030370b
69. Yang CX, Shiomi SS, Yang XP, Jia ZJ. Nor-lignans and steroidal saponins from Asparagus gobicus. Planta Med. (2004) 70:446–51. doi: 10.1055/s-2004-818974
70. Feng J, Chen DF, Sun QZ, Nakamura N, Hattori M. Asparosides A and B, two new steroidal saponins from Asparagus meioclados. J Asian Nat Prod Res. (2002) 4:221–6. doi: 10.1080/10286020290028983
71. Sautour M, Miyamoto T, Lacaille-Dubois MA. Steroidal saponins from Asparagus acutifolius. Phytochemistry. (2007) 68:2554–62. doi: 10.1016/j.phytochem.2007.02.033
72. Hayes PY, Jahidin AH, Lehmann R, Penman K, Kitching W, De Voss JJ. Steroidal saponins from the roots of Asparagus racemosus. Phytochemistry. (2008) 69:796–804. doi: 10.1016/j.phytochem.2007.09.001
73. Kumeta Y, Maruyama T, Wakana D, Kamakura H, Goda Y. Chemical analysis reveals the botanical origin of shatavari products and confirms the absence of alkaloid asparagamine A in Asparagus racemosus. J Nat Med. (2013) 67:168–73. doi: 10.1007/s11418-012-0669-4
74. Shastry RA, Madagundi SD, Habbu PV, Patil BS, Joshi SD, Kulkarni VH. Phytochemical investigation and antiepileptic activity of Asparagus racemosus (wild) root extracts in rodents. RGUHS J Pharm Sci. (2015) 5:97–103. doi: 10.5530/rjps.2015.3.3
75. Handa SS, Suri OP, Gupta VN, Suri KA, Satti NK, Bhardwaj V, et al. Oligospirostanoside from Asparagus racemosus as Immunomodulator. US Patent No. 6649745. (2003).
76. Sabde S, Bodiwala HS, Karmase A, Deshpande PJ, Singh IP. Anti-HIV activity of Indian medicinal plants. J Nat Med. (2011) 65:662–9. doi: 10.1007/s11418-011-0513-2
77. Saxena VK, Chourasia S. A new isoflavone from the roots of Asparagus racemosus. Fitoterapia. (2001) 72:307–9. doi: 10.1016/S0367-326X(00)00315-4
78. Hayes PY, Jahidin AH, Lehmann R, Penman K, Kitching W, De Voss JJ. Structural revision of shatavarins I and IV, the major components from the roots of Asparagus racemosus. Tetrahedron Lett. (2006) 25:6965–9. doi: 10.1016/j.tetlet.2006.07.121
79. Wiboonpun N, Phuwapraisirisan P, Tip-pyang S. Identification of antioxidant compound from Asparagus racemosus. Phytother Res. (2004) 18:771–3. doi: 10.1002/ptr.1526
80. Li YF, Hu LH, Lou FC, Hong JR, Li J, Shen Q. Furostanoside from Asparagus filicinus. J. Asian Nat Prod Res. (2005) 7:43–7. doi: 10.1080/10286020310001617110
81. Thakur S, Sharma S, Thakur S, Rai R. Green synthesis of copper nano-particles using Asparagus adscendens roxb. root and leaf extract and their antimicrobial activities. Int J Curr Microbiol Appl Sci. (2018) 7:683–94. doi: 10.20546/ijcmas.2018.704.077
82. Solayman M, Islam MA, Paul S, Ali Y, Khalil MI, Alam N, et al. Physicochemical properties, minerals, trace elements, and heavy metals in honey of different origins: a comprehensive review. Exp Clin Pharmacol. (2016) 15:219–33. doi: 10.1111/1541-4337.12182
83. Thakur M, Chauhan NS, Bhargava S, Dixit VK. A comparative study on aphrodisiac activity of some ayurvedic herbs in male albino rats. Arch Sex Behav. (2009) 38:1009–15. doi: 10.1007/s10508-008-9444-8
84. Negi JS, Singh P, Pant GJN, Rawat MSM, Pandey H. Variation of trace elements contents in Asparagus racemosus (Willd). Biol Trace Element Res. (2010) 135:275–82. doi: 10.1007/s12011-009-8485-8
85. Tong DW, Chao LI, Chen SL. Determination of copper, lead, cadmium and chromium in asparagus by microwave digestion-gfaas. J Huaihai Inst Technol. (2013) 22:30–4.
86. Li J, Ye L, Liu F, Pang J, Li J, Yao X, et al. Effect of Asparagus root decomposition on seed germination and radical elongation of crops. J Agric Univ Hebei. (2010) 33:43–5.
87. Lee JH, Lim HJ, Lee CW, Son KH, Son JK, Lee SK, et al. Methyl protodioscin from the roots of Asparagus cochinchinensis attenuates airway inflammation by inhibiting cytokine production. Evid Based Complement Alternat Med. (2015) 2015:640846. doi: 10.1155/2015/640846
88. Lohdip AM, Tyonande TT. Analysis of Asparagus africanus (LAM) for some hair growth-stimulating elements. ChemClass J. (2005) 2:93–4.
89. Panfilova H, Samborskyi O, Bogdan N, Tsurikova O, Simonian L. Study of development problems dynamics of ukrainian market of stomach ulcer treatment drugs. Res J Pharm Technol. (2021) 14:2502–10. doi: 10.52711/0974-360X.2021.00441
90. Bhatnagar M, Sisodia SS, Bhatnagar R. Antiulcer and antioxidant activity of Asparagus racemosus wild and Withania somnifera dunal in rats. Ann N Y Acad. (2010) 1056:261–78. doi: 10.1196/annals.1352.027
91. Sairam K, Priyambada S, Aryya NC, Goel RK. Gastroduodenal ulcer protective activity of Asparagus racemosus: an experimental, biochemical and histological study. J Ethnopharmacol. (2003) 86:1–10. doi: 10.1016/S0378-8741(02)00342-2
92. Hannan JMA, Marenah L, Ali L, Rokeya B, Flatt PR, Abdel-Wahab YH. Insulin secretory actions of extracts of Asparagus racemosus root in perfused pancreas, isolated islets and clonal pancreatic β-cells. J Endocrinol. (2007) 192:159–68. doi: 10.1677/joe.1.07084
93. Folane P, Unhale S, Kale P, Shelke S, Biyani KR. Antidiabetic activity of polyherbal formulation containing Citrullus colocynthis, Piper nigrum, Asparagus racemosus, Cinnamomum tamala (cpac) in alloxan induced diabetic rats. World J Pharm Pharm Sci. (2020) 9:1174–88.
94. Muhammad G, Hussain MA, Jantan I, Bukhari SNA. Mimosa pudica L., a high-value medicinal plant as a source of bioactives for pharmaceuticals. Compr Rev Food Sci Food Safe. (2016) 15:303–15. doi: 10.1111/1541-4337.12184
95. Hamdi A, Jiménez-Araujo A, Rodríguez-Arcos R, Jaramillo-Carmona S, Lachaal M, Bouraoui N, et al. Asparagus saponins: chemical characterization, bioavailability and intervention in human health. Nutri Food Sci Int J. (2018) 7:555704.
96. Ratnam KV, Ravishankar K, Kiranmayi GVN. Evaluation of diuretic and antiurolithiatic activities of ethanolic root extracts of Curculigo orchioides. Int J Biol Pharm Res. (2011) 4:12.
97. Jaiswal Y, Liang Z, Ho A, Chen H, Zhao ZA. Comparative tissue-specific metabolite analysis and determination of protodioscin content in asparagus species used in traditional Chinese medicine and ayurveda by use of laser microdissection, UHPLC–QTOF/MS and LC–MS/MS. Phytochem Anal. (2014) 25:514–28. doi: 10.1002/pca.2522
98. Saxena G, Singh M, Meena P, Barber S, Sharma D, Shukla S, et al. Neuroprotective effects of Asparagus racemosus linn root extract: an experimental and clinical evidence. Ann Neurosci. (2007) 14:57–62. doi: 10.5214/ans.0972.7531.2007.140302
99. Uddin MS, Asaduzzaman M, Mamun AA, Iqbal MA, Wahid F, Rony RK. Neuroprotective activity of Asparagus racemosus Linn. against ethanol-induced cognitive impairment and oxidative stress in rats brain: auspicious for controlling the risk of Alzheimer’s disease. J Alzheimers Dis Parkinson. (2016) 6:1–10. doi: 10.4172/2161-0460.1000245
100. Balasubramani SP, Venkatasubramanian P, Kukkupuni SK, Patwardhan B. Plant-based rasayana drugs from ayurveda. Chin J Integr Med. (2011) 17:88–94. doi: 10.1007/s11655-011-0659-5
101. Turner NJ, Łuczaj ŁJ, Migliorini P, Pieroni A, Dreon AL, Sacchetti LE, et al. Edible and tended wild plants, traditional ecological knowledge and agroecology. Crit Rev Plant Sci. (2011) 30:198–225. doi: 10.1080/07352689.2011.554492
102. Liu LS, Liu JX, Li C, Tian JH, Shi ZM. Clinical effect of Yiqi Yangyin Jiedu decoction in treating patients with advanced non-small cell lung cancer. Zhongguo Zhong Xi Yi Jie He Za Zhi. (2008) 8:352–5.
103. Goel RK, Prabha T, Kumar MM, Dorababu M, Prakssh, Singh G. Teratogenicity of Asparagus racemosus Willd. root, a herbal medicine. India J Exp Biol (2006) 44:570–3.
Keywords: asparagus roots, physicochemical properties, pharmacology, health benefits, bioactive compounds
Citation: Guo Y, Liu Z, Wan Y, Zhang Y, Abdu HI, Yang M, Pei J, Yue T, Zhang X, Hacimuftuoglu A and Abd El-Aty AM (2023) Literature analysis on asparagus roots and review of its functional characterizations. Front. Nutr. 9:1024190. doi: 10.3389/fnut.2022.1024190
Received: 21 August 2022; Accepted: 05 December 2022;
Published: 17 April 2023.
Edited by:
Er Sheng Gong, Gannan Medical University, ChinaReviewed by:
Yuanyuan Bian, Shenyang Agricultural University, ChinaBin Peng, Jiangxi Normal University, China
Copyright © 2023 Guo, Liu, Wan, Zhang, Abdu, Yang, Pei, Yue, Zhang, Hacimuftuoglu and Abd El-Aty. This is an open-access article distributed under the terms of the Creative Commons Attribution License (CC BY). The use, distribution or reproduction in other forums is permitted, provided the original author(s) and the copyright owner(s) are credited and that the original publication in this journal is cited, in accordance with accepted academic practice. No use, distribution or reproduction is permitted which does not comply with these terms.
*Correspondence: Jinjin Pei, jinjinpeislg@snut.edu.cn; Tianli Yue, yuetl305@nwsuaf.edu.cn; Xianbin Zhang, zhangxianbin@hotmail.com
†These authors share first authorship