- 1Food and Nutritional Sciences, Chubu University, Kasugai, Japan
- 2Department of Nutrition and Food Hygiene, West China School of Public Health and West China Fourth Hospital, Sichuan University, Chengdu, China
Background and aims: Sichuan dark tea (ST), Zangcha, is a traditional fermented Chinese tea found in Sichuan and Tibet and claimed for beneficial effects against lifestyle-related metabolic disorders. We examined the effects of ST on lipid metabolism and atherosclerosis.
Methods and results: Sichuan dark tea was given to fat-rich diet-induced atherosclerosis model rats in comparison with dark-fermented Chinese Pu-erh tea (PT) and Japanese green tea (GT). After 8 weeks of feeding, ST and PT induced an increase in high-density lipoprotein (HDL)-cholesterol and a decrease in glucose, and ST decreased triglyceride in plasma. ST also induced low pH in the cecum. There was no significant change in their body weight among the fat-feeding groups but a decrease was found in the visceral fat and liver weight in the ST group. Accordingly, ST reduced lipid deposition in the aorta in comparison with PT and GT. ST increased mRNA of LXRα, PPARα, PPARγ, and ABCA1 in the rat liver. The extract of ST stimulated the AMPK pathway to increase the expression of ABCA1 in J774 cells and increased expression of lipoprotein lipase and hormone-sensitive lipase in 3T3L1 cells, consistent with its anti-atherogenic effects in rats. High-performance liquid chromatography analysis showed unique spectra of original specific compounds of caffeine and catechins in each tea extract, but none of them was likely responsible for these effects.
Conclusion: Sichuan dark tea increases plasma HDL and reduces plasma triglyceride to decrease atherosclerosis through AMPK activation. Further study is required to identify specific components for the effects of this tea preparation.
Introduction
Tea is a long-time favored beverage in the history of human culture and is believed to have various beneficial effects on human health. It is produced from the leaves of Camellia sinensis originating in China (C. sinensis var. sinensis) and India (C. sinensis var. assamica). The tea products in China and Japan are all made from the former. Tea is taken as various preparations largely classified into two categories of straight green tea (GT) and its fermented products. Dark-fermented tea is one of the latter popular in China and has empirically been claimed for its beneficial effects on health in tradition. It has therefore become a subject of interest and investigation in health and nutritional sciences, and the evidence has accumulated for the background of the effects against various lifestyle-related metabolic disorders, such as obesity, diabetes, hypertension, and atherosclerosis. Most of these works were carried out for popular and commonly consumed Pu-erh tea (PT) but some were for other types of dark teas as well (1–6). Preliminary findings indicated the activation of peroxisome proliferator-activated receptors (PPARs), liver X receptors (LXRs), and farnesoid X receptors (FXRs) by some fermentation-processed teas (7, 8), and the topic was well summarized in an extensive review article by Zhang (9). On the other hand, the risk of toxicity of PT was also discussed in general for the accumulation of fluoride in old tea leaves and the production of aflatoxins during fermentation (10).
Sichuan dark tea (ST), Zangcha, is one of the traditional dark-fermented teas commonly consumed in the regions of Sichuan and Tibet in China (11). The historical background of this tea is that it originates in the plants in Yunnan Province and their rough old leaves are fermented during long transportation to Tibet through Sichuan. In these days, it is mainly produced in Ya’an area of Sichuan Province, located in the middle of the historic long trail from Yunnan to Tibet. This is a unique and local type of fermented tea that has come down in history with a strong local belief of its beneficial effects on health. Because it has been less popular even among the Chinese, scientific investigation has not been done much in comparison with PT (12–14). Recently, Li et al. have reported the effects of an ST-based formula designed on the idea of “medicine-food homology” (15). The formula reduced triglyceride and raised HDL-cholesterol in the plasma of apolipoprotein E-deficient mice, in association with changes in the intestinal microbial flora (15). The results indicated its potential preventive effect against atherosclerosis development. It is therefore important to investigate this particular dark-fermented Chinese tea in further detail.
Based on these backgrounds, we studied the effects of ST on atherosclerosis development in the fat-rich diet-induced rat model. We chose Chinese PT and Japanese GT, as references, since these were more common types of tea for the previous investigation as described above. It was shown that ST has more specific effects than other types of tea on lipid metabolism and atherosclerosis development in a specific animal model used in this study.
Materials and methods
Tea products and their processing
Three types of tea products were subjected to investigation, representing dark-fermented, and fresh green teas. ST under the product name Zangcha was provided by Guge Tibetan Tea Culture Communication Co., Ltd., in Ya’an, Sichuan, China. Pu-erh tea (PT) was a commercial product of Yunnan Province, China and purchased in Japan through China Trading Co., Ltd., Yokohama. Deep-steamed GT was a commercial product of ITO EN, Tokyo, Japan. For animal experiments, the dry leaves of 20 g were boiled in 1 liter water at 100°C for 45 min using a heat insulation tank (Shaking Bath BW 101, Yamato Kagaku Co., Ltd., Tokyo), cooled to room temperature, and filtered before feeding. For the experiments with cultured cells, the tea extracts were prepared by boiling 20 g of tea leaf in 300 mL water for 45 min followed by filtering and drying with a centrifugal evaporator (CVE-3110 type, EYELA Tokyo Rika Kikai Co., Ltd., Tokyo) at 30°C, 900 rpm and −100 kPa for 45 h as designed based on previous reports (16, 17). The extracts were stocked in a solution of 1 mg/mL with distilled water. For the analysis by high-performance liquid chromatography (HPLC), the tea leaf extract of 2 g boiled in 100 mL water for 45 min was filtered for 0.22 μm and diluted twofold with distilled water.
Animal experiments
Six-week-old male Sprague–Dawley rats were divided into five groups (n = 9 in each) and housed under alternating 12-h light/dark cycles with a relative humidity of 60 ± 10% and a controlled temperature of 22 ± 2°C. The control group was fed a control chow with 5.4% fat in calories (CRF-1, Oriental Yeast, Tokyo) and the other four groups were given a high-fat diet with 16.5% in fat (F2HFD1, Oriental Yeast) for 8 weeks (Table 1). The fat contents of these diets are substantially lower than those used in other previous experimental protocols for rats, such as 13% for control and 45–60% for high fat (18–20), reflecting the nutritional background of Japanese. The control group and plain high-fat diet group (HF) were fed with water, and the experimental groups were fed with the three types of tea (such as ST, PT, and GT) instead of water on top of the high-fat diet, as they were prepared as described above. The tea or water was taken freely as a liquid. The food and liquid intakes were measured three times a week and the body weight was measured once a week for monitoring. At the end of the experiment, blood plasma levels of total cholesterol (TC), HDL-cholesterol (HDL-C), triglyceride (TG), and glucose were measured under the condition of free feeding, by using enzymatic assay kits for lipid parameters provided by Sekisui Medical Co., Ltd., Tokyo and by Glucose CII Test Wako (GOD method, Wako Pure Chemical Industries, Ltd., Tokyo) for blood glucose level. The presence of apolipoprotein E-containing HDL in rat plasma may not likely influence the HDL assay significantly (21) but the assay system was validated for linearity and selectivity for rat plasma. Non-HDL-cholesterol (non-HDL-C) was calculated as [TC]–[HDL-C]. After the animals were euthanized under isoflurane gas, the weight of the liver and that of omentum/perirenal visceral fat was measured and expression of mRNA in the liver was estimated for LXRα, PPARα, PPARγ, ATP binding cassette transporter (ABC)A1, ABCG1, lipoprotein lipase, and hepatic lipase as described in detail below. The contents of the cecum were taken out by a spatula with saline for measuring its pH. The deposition of lipid on the inner wall of the aorta was examined by staining with Oil red O (MUTO PURE CHEMICALS Co., Ltd., Tokyo). The area of the region stained above a threshold density was quantitated by using the Photoshop software, as a relative area of a color-selected region (red) to a focused region (total surface) with a histogram tool as described (22). The protocol for the animal experiments had been approved as 2910063 by the Animal Experiments and Welfare Committee of Chubu University in accordance with its guidelines.
The cells and their culture conditions
The effects of tea extracts were examined in cell line cells modeling macrophages and adipocytes to investigate their background mechanism in preventing atherogenesis and visceral adipogenesis. Mouse monocyte-macrophage cell line cells J774.1 were maintained in RPMI 1640 medium (Sigma-Aldrich, St. Louis) containing 10% fetal bovine serum and 0.05 mg/mL gentamicin solution (Sigma-Aldrich) in a humidified atmosphere of 5% CO2 and 95% air at 37°C. The cells were seeded in culture plates at a density of 3 × 106 cells and cultured for 1 day before use. Mouse immortalized 3T3-L1 fibroblasts were cultured with DMEM medium (Sigma-Aldrich) supplemented with 10% fetal bovine serum in a humidified atmosphere of 5% CO2 and 95% air at 37°C. The cells were differentiated to adipose 2 days after reaching 80% confluence (day 0), by supplementing growth media containing 10 μg/mL insulin (Sigma-Aldrich), 1 μM dexamethasone (Wako), and 0.5 mM 1-methyl-3-isobutyl-xanthine (Sigma-Aldrich) and maintained for 4 to 8 days in growth media containing 1 μg/mL insulin. The effects of the tea extracts were examined on J774 cells and 3T3-L1 cells. The tea extract prepared as described above was added to the cells in culture in a final concentration of 1 and 5 μg/mL for 1/100 and 1/20 of the tea preparation for oral intake. J774.1 cells at an 80% confluent stage were washed with phosphate-buffered saline and treated with various concentrations of the tea extracts in RPMI 1640 medium supplemented with 0.1% bovine serum albumin (Sigma-Aldrich) and 0.05 mg/mL gentamicin solution (Sigma-Aldrich) for 18 h. After differentiation into adipocytes, 3T3-L1 fibroblasts were incubated with various concentrations of the tea extracts in the DMEM medium supplemented with 0.1% bovine serum albumin for 18 h.
Estimation of mRNA expression by real-time quantitative polymerase chain reaction
Total cellular RNA was isolated from frozen livers or tea extracts treated cells using ISOGEN reagent (Nippon Gene, Tokyo). RNA was reverse transcribed to cDNA using iScript™ cDNA Synthesis Kit (BIO-RAD, Hercules). Gene expression was analyzed by real-time quantitative polymerase chain reactions using SYBR PCR Permix Ex Taq Kit (TaKaRa, Kyoto) on a 7300 Real-Time PCR System (Applied Biosystems, Waltham). Primer sequences used in the study are shown as follows: abca1, 5′-AAC AGT TTG TGG CCC TTT TG-3′ and 5′-GAT GAG CCA GAC TTC TGT TGC-3′ for J774.1 cells, 5′-GAA CTG GCT GTG TTC CAT TGA T-3′ and 5′-GAT GAG CCA GAC TTC TGT TGC-3′ for mouse liver, abcg1, 5′-ACG CAG TTC TGC ATC CTC TT-3′ and 5′-CGG AGT TGC TCA AGA CCT TC-3′, lxrα, 5′-TCT GGA GAC ATC TCG GAG GTA-3′ and 5′-GGC TCA CCA GTT TCA TTA GCA-3′, pparα, 5′-GGA CCT TCG GCA GCT GGT-3′ and 5′-TCG GAC TCG GTC TTC TTG ATG-3′, pparγ, 5′-ATA AAG CAT CAG GCT TCC ACT-3′and 5′-GCA CTT CTG AAA CCG ACA GTA-3′, lpl (lipoprotein lipase), 5′-GGG CAT GTT GAC ATT TAC CC-3′ and 5′-GCT GGT CCA CAT CTC AAG T-3′, hl (hepatic lipase), 5′-GAA ACC AGA GCC ATT TGG AA-3′ and 5′-AAT CTG ACA GCC CTG ATT GG-3′, and hsl (hormone-sensitive lipase), 5′-GCA CTA CAA ACG CAA CGA GA-3′ and 5′-TGT GAT CCG CTC AAA CTC GA-3′.
Phosphorylation of 5′ adenosine monophosphate-activated protein kinase
The effect of tea extracts was examined for phosphorylation of AMPK (23). J774.1 cells were preincubated with or without dorsomorphin (DOR), an inhibitor of AMPK (predissolved in DMSO and added to make the final concentration 5 μM), for 1 h. After this treatment, the cells were incubated with or without 1 μg/mL of tea extract or 0.15 mM cAMP, an activator of AMPK, for 1 h. Cells were immediately rinsed with phosphate-buffered saline at 4°C and incubated for 5 min with gentle shaking in Lysis-M reagent (complete Lysis-M, Roche, Basel) containing complete Mini tablets (Complete Mini Protease Inhibitor Cocktail tablets, Roche) and phosphatase inhibitor tablets (PhosSTOP, Roche). The insoluble residue was removed by centrifugation at 15,000 × g for 10 min at 4°C to collect the supernatant as cell lysate. An equal amount of protein in the lysis buffer [50 mM Tris–HCl buffer, pH 7.5, containing 150 mM NaCl, 1% Triton X-100, 1% sodium dodecyl sulfate (SDS), and 10 mM 2Na-EDTA] in the presence of Protease Inhibitor Cocktail (Sigma-Aldrich) was analyzed in SDS-polyacrylamide electrophoresis and immunoblotting by using the monoclonal antibodies against AMPK and phosphorylated AMPK (Invitrogen) and that against ABCA1 peptide following a common sequence of its C-terminus of human and mouse CNFAKDQSDDDHLKDLSLHKN (MABI98-7, MAB Institute, Yokohama, Japan) (24), and by using that against β-actin (A5316, SIGMA) as a reference. The signals were visualized with a chemiluminescence method using ImmunoStar LD or Zeta (Wako) on Image Studio Digits (LI-COR Biosciences, Lincoln).
Analysis of tea extracts by high performance liquid chromatography
An HPLC system for the determination of tea extracts consisted of an 1100 series system (Agilent Technologies, Santa Clara, CA, USA) with a TSKgel ODS-80Ts column (4.6 mm × 250 mm, Tosoh Co., Kyoto, Japan) and an SPD-6AV UV-VIS spectrophotometric detector (Shimadzu Co., Kyoto, Japan) using detection wavelengths at 280 nm. A two-solvent gradient elution using 10 mM KH2PO4 (A) and acetonitrile (B) was performed with a flow rate of 1 mL per min. The mobile phase composition started with 100% solvent A, being increased linearly to 40% solvent B in 20 min, followed by a linear increase of solvent B to 60% in 10 min, and the final conditions being held for an additional 10 min. All samples were microfiltered before injection. The system was calibrated by standard compounds of (–)-epicatechin, (–)-epicatechin gallate, (–)-epigallocatechin, (–)-epigallocatechin gallate, and caffeine were purchased from Nagara Science. Stock standard solutions (500 ppm) were prepared in ascorbic acid and stored at −30°C, from which working standard solutions were prepared weekly by diluting with distilled water. Tea leaf extracts prepared as described above were analyzed according to the conditions for the calibration condition specified above.
Statistical analysis
The data were statistically analyzed by Student’s t-test with one-sided and unpaired testing for unequal dispersion by using Microsoft Excel statistical tool. The level of significance in difference is defined as p < 0.01 and p < 0.05, specified in the legend for tables.
Results
Plasma lipid parameters
Basic feeding parameters of the study are presented in Table 1. The food intake record did not show a statistical difference in any feeding parameter amongst the feeding groups except for fat intake between the control group and the high fat-fed groups as designed and accordingly for the total energy intake as well. The major outcomes of the animal experiment after 8 weeks are presented in Table 2. No significant difference was found in the body weight among the groups at the end of the study while the relative weight of the visceral fat significantly decreased in the ST group against other groups (Table 3). The weight of the liver increased by HF feeding but significantly less in the ST group.
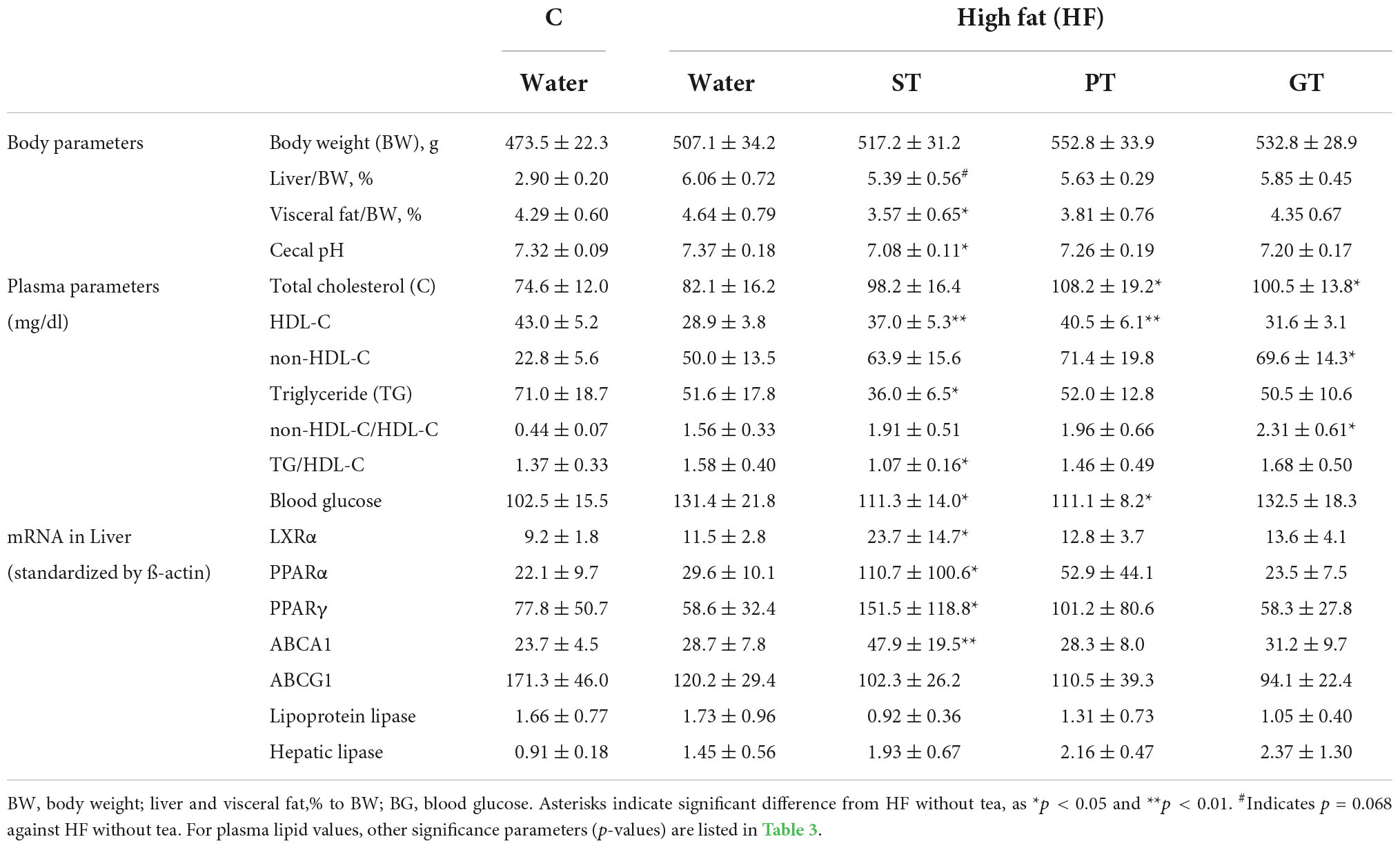
Table 2. Effects of 8 week tea-feeding; control chow (C), High fat chow (HF), Sichuan tea (ST), Pu-erh tea (PT), and Green tea (GT).
Plasma lipid and lipoprotein levels at the end of the study are presented also in Table 2. Significance levels in the difference between the feeding groups are presented in Table 3 as p-values for each t-test combination. Significant changes by feeding HF diet were decreased in HDL-C and increased in non-HDL-C, but no apparent change was observed in TG level. Accordingly, non-HDL-C/HDL-C as a strong atherogenic index showed a marked increase indicating an increase in atherogenesis in this rat model. On the basis of HF diet feeding, HDL-C significantly increased in the ST and PT groups against HF but the GT group showed no difference. Non-HDL-C increased significantly by PT and GT against HF. TG decreased by ST against other groups fed with HF. The parameters of non-HDL-C/HDL-C increased by GT against HF and TG/HDL-C decreased by ST against HF, PT, and GT. Blood glucose decreased in ST and PT but not in GT groups against the HF group. Plasma lipid data are also graphically displayed in Figure 1. The peculiar changes by feeding of ST were demonstrated as an increase in HDL-C and a decrease in TG and accordingly a decrease in TG/HDL-C, in comparison with other tea products.
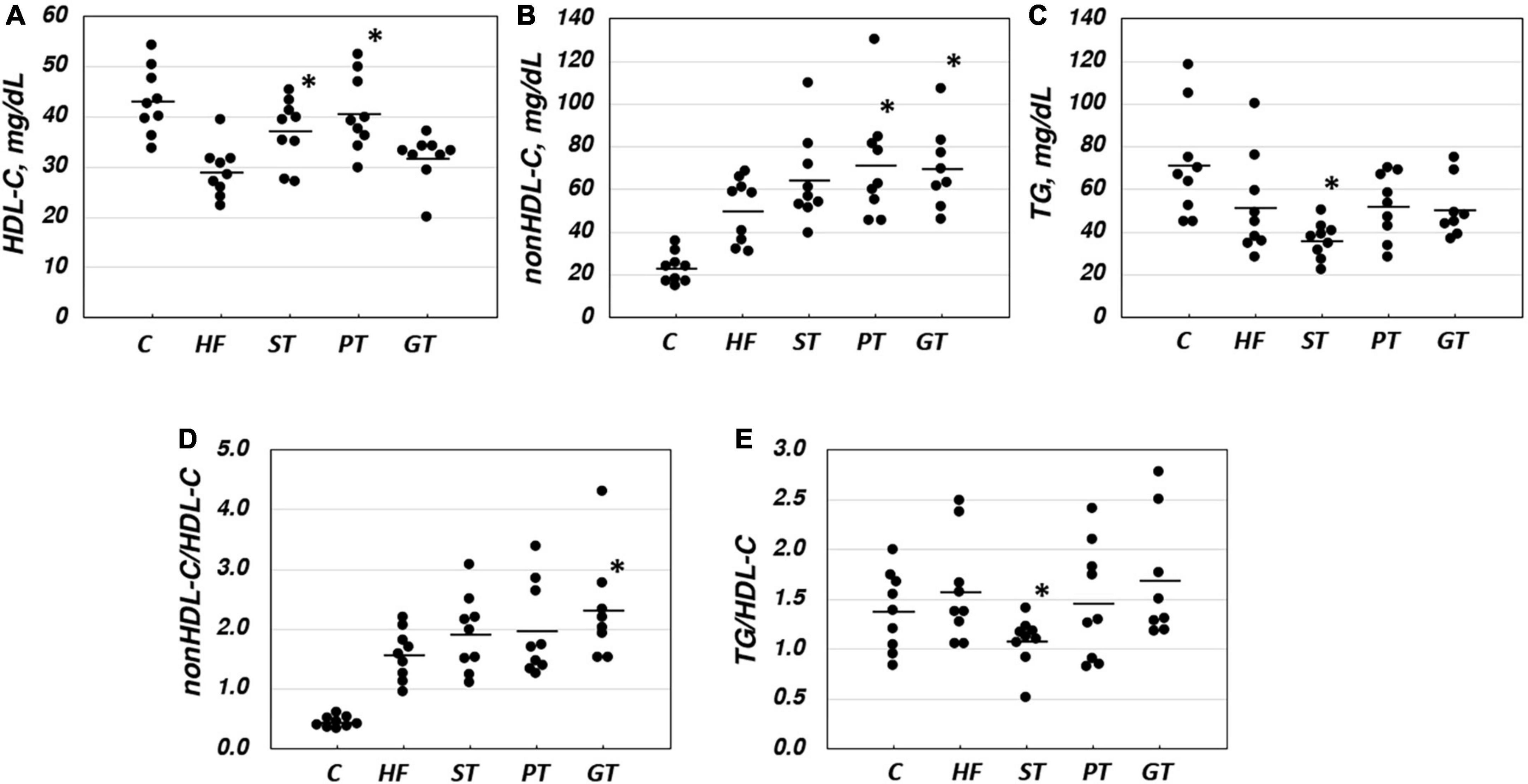
Figure 1. Lipid and lipoprotein parameters in rat plasma after 8-week experimental feeding as indicated in Tables 1, 2 (A, HDL-C; B, nonHDL-C; C, TG; D, nonHDL-C/HDL-C; E, TG/nonHDL-C) (C, control; HF, high fat-fed; ST, PT, and GT, Sichuan tea, Pu-erh tea, and Green tea on top of HF, respectively) (n = 9 for each group). A bar indicates the average value for each group. Total cholesterol and triglyceride (TG) were measured by using an enzymatic method and HDL-C was measured enzymatically by a homogeneous assay system as described in the text. Non-HDL-C was calculated by subtracting HDL-C from total cholesterol. Asterisks indicate a significant difference from the high-fat fed group (HF) as indicated in Table 3. The significance of differences in other combinations of the feeding groups is presented in Table 3.
Expression of the lipid-related genes
Table 2 also shows the data for expression levels of some mRNAs related to lipid and lipoprotein metabolism probed in the liver of the rats after the 8-week experiment. The mRNA of LXRα, PPARα, PPARγ, and ABCA1 was increased by ST against other feeding groups, but the expression of ABCG1, lipoprotein lipase, and hepatic lipase showed no significant difference among the groups. Overall, the anti-atherogenic effect of ST was more prominent than PT and GT (Table 3).
Lipid deposition on the aorta
Lipid deposition in the aortic intima surface was visualized by Oil red O staining after feeding of the tea preparations for 8 weeks, as shown in Figure 2. The area of the stained region relative to the total aortic surface was calculated for each animal and displayed in the graph in the figure. Significance between the feeding groups is presented in Table 3 as the p-values of each t-test between the groups. Lipid deposit significantly increased by HF diet indicating atherogenesis of this diet model, and it was decreased by feeding ST. The feeding groups of PT and GT did not show a significant change in lipid deposit.
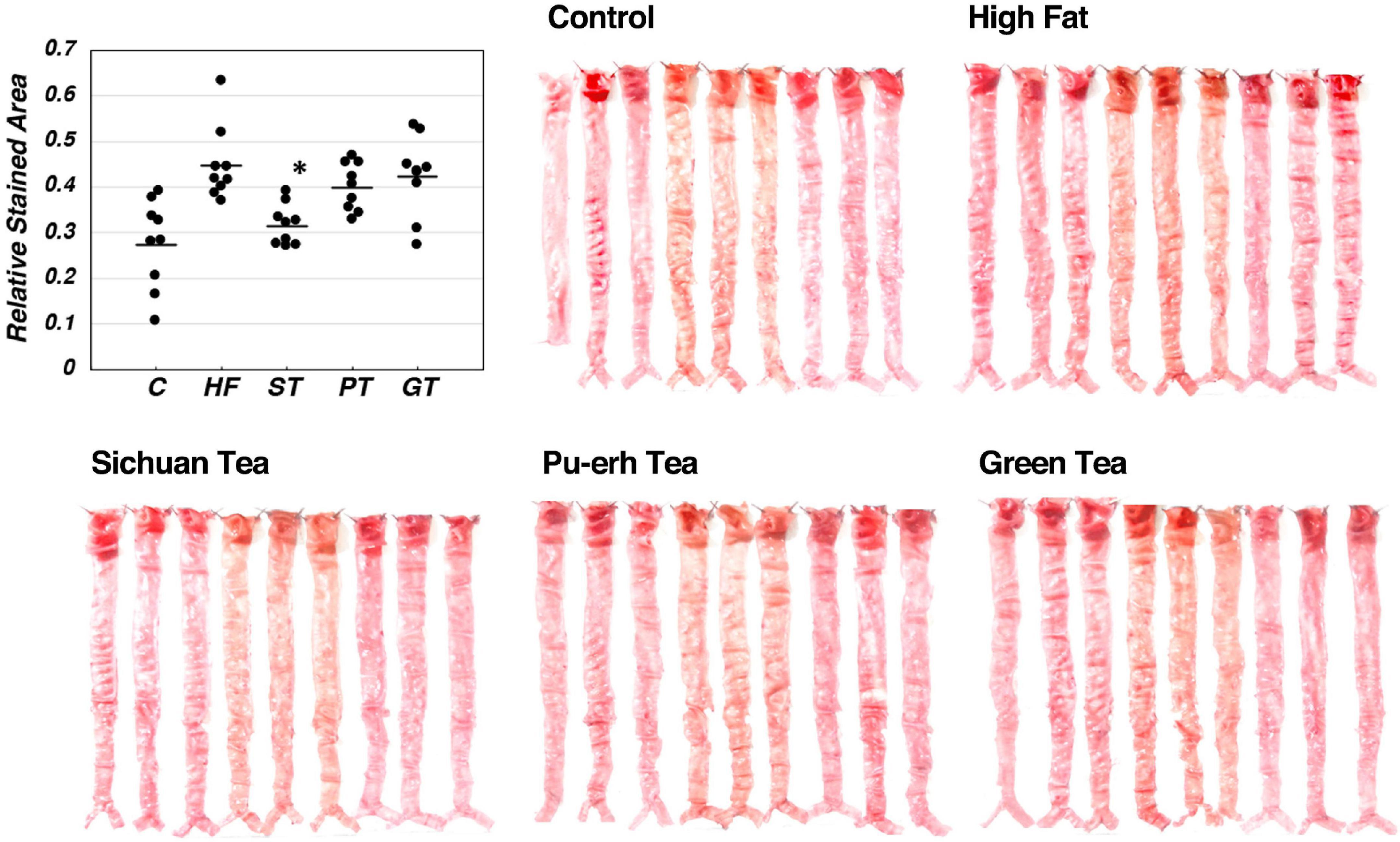
Figure 2. Lipid deposit on the aortic intima after 8-week experimental feeding, as indicated in Table 2 and Figure 1. The aortic intima surface was stained by Oil Red O. Red area was scanned and quantitated by using the Photoshop software as described in the text. The graph shows relative stained area and a bar indicates the average value for each group. The asterisk indicates a significant difference from HF as indicated in Table 3 (n = 9). The significance of differences in other combinations of the feeding groups is presented in Table 3.
Analysis of the tea extracts and their effects on the cells in culture
The increase by ST in mRNA of LXRα, PPARα, PPARγ, and ABCA1 in the rat liver was consistent with the increase in their plasma HDL. In order to investigate a signal to trigger these reactions, stimulation of the AMPK pathway was examined in J774 mouse macrophage cell line cells (Figures 3A,B). A positive control cAMP induced phosphorylation of AMPK as well as an increase of ABCA1 in this system and these effects were canceled by an AMPK inhibitor DOR. The extract from ST also significantly induced phosphorylation of AMPK but no other tea extracts, as well as an increase in the expression of ABCA1. The effects of ST were also canceled by DOR. Thus, AMPK phosphorylation is likely an upper-stream signal for LXRα and PPARs to increase ABCA1 gene expression by ST similar to the case demonstrated with nobiletin (23).
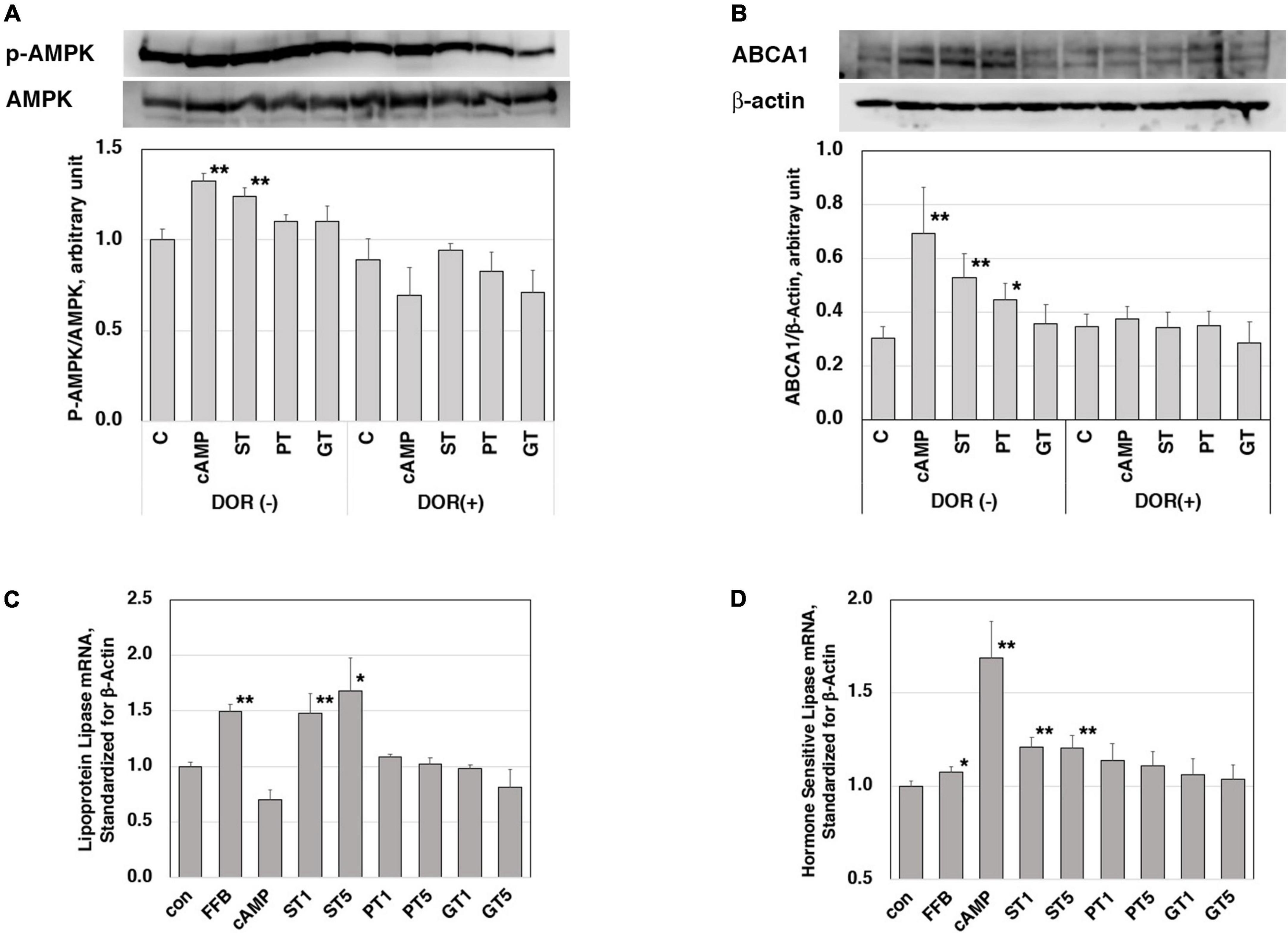
Figure 3. Functions of the tea extracts evaluated in the cell culture system. Stimulation of AMPK phosphorylation in mouse macrophage cell line cells J774 (A,B). Cells were pre-incubated with 5 μM AMPK inhibitor dorsomorphin (DOR) for 1 h and then incubated with or without 0.15 mM cAMP or tea extracts (1 mg/ml) for 4 h. AMPK protein, phosphorylated AMPK (A), and ABCA1 (B) were quantitated in electrophoresis and immunoblotting. Relative phosphorylation was calculated for graphic display (C for control; cAMP as a positive control; ST, PT, and GT for Sichuan tea, Pu-erh tea, and Green tea, respectively). Asterisks ** indicate significant differences from the control by p < 0.01 (n = 3). Enhancement of expression of lipoprotein lipase (C) and hormone-sensitive lipase (D) in mouse adipose cell models 3T3-L1. Cells were stimulated by tea extracts (1 and 5 μg/ml), fenofibric acid (50 μM), and cAMP (0.15 mM). The mRNA of lipoprotein lipase and hormone-sensitive lipase was quantitated by RT-PCR (C for control; FFB, fenofibric acid; ST, PT, and GT for Sichuan tea, Pu-erh tea, and Green tea, respectively). Asterisks indicate significant differences from the control by **p < 0.01 and *p < 0.05. The data presented represent those from 5 to 6 experiments repeated.
Reduction of plasma TG and body fat mass seem to be the other key for the anti-atherogenic effects of ST, so the molecular background for these functions was examined in mouse adipose-like cells 3T3-L1. The expression levels of mRNA of lipases were examined for the effects of the tea extracts (Figures 3C,D). The mRNA of lipoprotein lipase and hormone-sensitive lipase was increased by the respective positive control activator of fenofibrate (PPARα agonist) and cAMP. Among the three tea extracts, ST increased the mRNA of both lipases being consistent with the phenotype induced by ST in fat-fed rats.
Profile of representative bioactive components, such as caffeine and catechins of tea extracts were analyzed by HPLC. Substantial differences were demonstrated in the spectrum of caffeine and four typical catechins (such as epigallocatechin, epicatechin, epigallocatechin gallate, and epicatechin) among the tea preparations examined (Table 4). However, none of those differences were likely consistent to support the specific effects of ST, including the change in caffeine which most likely stimulates the cAMP-related signaling pathway.
Discussion
Sichuan dark tea, a type of tea produced by fermentation in a specific traditional fashion in China, has been claimed to have various beneficial effects against lifestyle-related health disorders, such as diabetes, dyslipidemia, and atherosclerosis as described above. However, solid experimental or clinical evidence have not adequately accumulated to support these claims (12–14). A recent report indicated that ST-related product induced a reduction in TG and an increase of HDL in apolipoprotein E-deficient mice as well as changing the intestinal flora (15). We, therefore, conducted experiments to investigate the effects of ST including verification of the prior findings reported and to look into their molecular background. The atherogenesis model was generated by a modest increase of fat feeding in order to reflect the Japanese nutritional background (18–20). This diet resulted in a significant decrease in HDL-C and an increase in non-HDL-C and accordingly marked an increase in the atherogenic index of non-HDL-C/HDL-C ratio, without apparent change in TG. Lipid deposit in the aortic surface indeed increased by this diet feeding.
Sichuan dark tea feeding increased HDL and reduced plasma TG in rats to confirm the previous findings in mice (15). Both of these animals lack plasma cholesteryl ester transfer protein activity, so these findings are independent effects and unlikely linked (25). ST also induced a significant decrease in visceral fat and blood glucose level. Accordingly, ST reduced fat deposits on the aortic intima. Thus, the potential anti-atherogenic effect of ST was demonstrated likely through altering lipid and lipoprotein metabolism. ST reduced the cecal pH, which may also be consistent with the previous finding of changing the intestinal flora. Pu-erh tea (PT) and GT, shown effective in improving lipid metabolism and atherosclerosis in model animals with severe hyperlipidemia (17, 26–28), were less effective in a current moderate hyperlipidemic rat model.
Plasma HDL level and lipid accumulation in vascular macrophages are regulated by the expression of ABCA1, and its mRNA indeed increased in the liver of ST-fed rats being consistent with the finding in the animals. We previously showed that regulation of ABCA1 expression involves the cAMP-AMPK pathway (29) to activate the propagation loop between LXRα-PPARs (23, 30). AMPK was shown activated by ST to increase ABCA1 expression in a macrophage cell model to support the finding that ST decreased lipid deposition in the aorta. Reduction of plasma TG and body fat mass can also be related to the cAMP-AMPK pathway. The increase of PPARs upregulates LPL expression to reduce plasma TG. The cAMP pathway also increases the expression of hormone-sensitive lipase to mobilize TG from adipocytes to decrease body fat. These hypotheses were consistent with the findings demonstrated in an adipose cell model as the increase of mRNAs of these lipases by the ST extract. Thus, the effect of ST on lipid metabolism can largely be interpreted by its stimulation of the cAMP-AMPK pathway, which eventually achieves the prevention of lipid deposition on the aortic surface. This mechanism has been in fact suggested as a background for anti-atherogenic effects of other teas (31–33).
It was inconclusive at this moment to identify a specific substance for this function of ST among these known tea compounds. Well-known active components in teas are caffeine and catechins, which potentially activate the cAMP-AMPK pathway (34–37). These components are analyzed by HPLC for the extract of the teas used in this study. There was a substantial difference in their composition among ST, PT, and GT, but no specific tendency was identified to interpret or support the specific anti-atherogenic effect of ST. It has been demonstrated various modifications and bioconversion of the authentic bioactive components of tea leaves during their processing by fermentation (38–43). Specific fungal and bacterial spectrums required for fermentation to produce ST make such reactions more complicated (44, 45).
We thus conclude that ST may be more beneficial for the prevention of atherosclerosis development than other types of tea, at least PT and GT, by regularly taking it in a normal beverage-taking fashion. Investigation into the profile of plasma lipoprotein and apolipoprotein in future would provide more supportive information for the hypothesis above discussed. Further study is required to identify a specific compound(s) or any specific combination of such materials contained in ST for its peculiar anti-atherogenic effects. The role of low cecal pH in ST-fed rats is unknown if it is related to the effects on lipid metabolism or more directly to anti-atherosclerotic action. This should be investigated in relation to changes in the intestinal flora by the ST-related product (15).
Conclusion
Sichuan dark tea increased HDL and reduced plasma TG and visceral fat and decreased lipid deposition in the aorta in fat-fed rats, more than Pu-erh tea and Japanese green tea. ST increases ABCA1, lipoprotein lipase, and hormone-sensitive lipase by activation of the AMPK pathway. The results and discussion are graphically summarized in Figure 4. The effect however cannot be attributed to caffeine and catechins in their original forms. Further studies are required to identify the compounds responsible for this specific function.
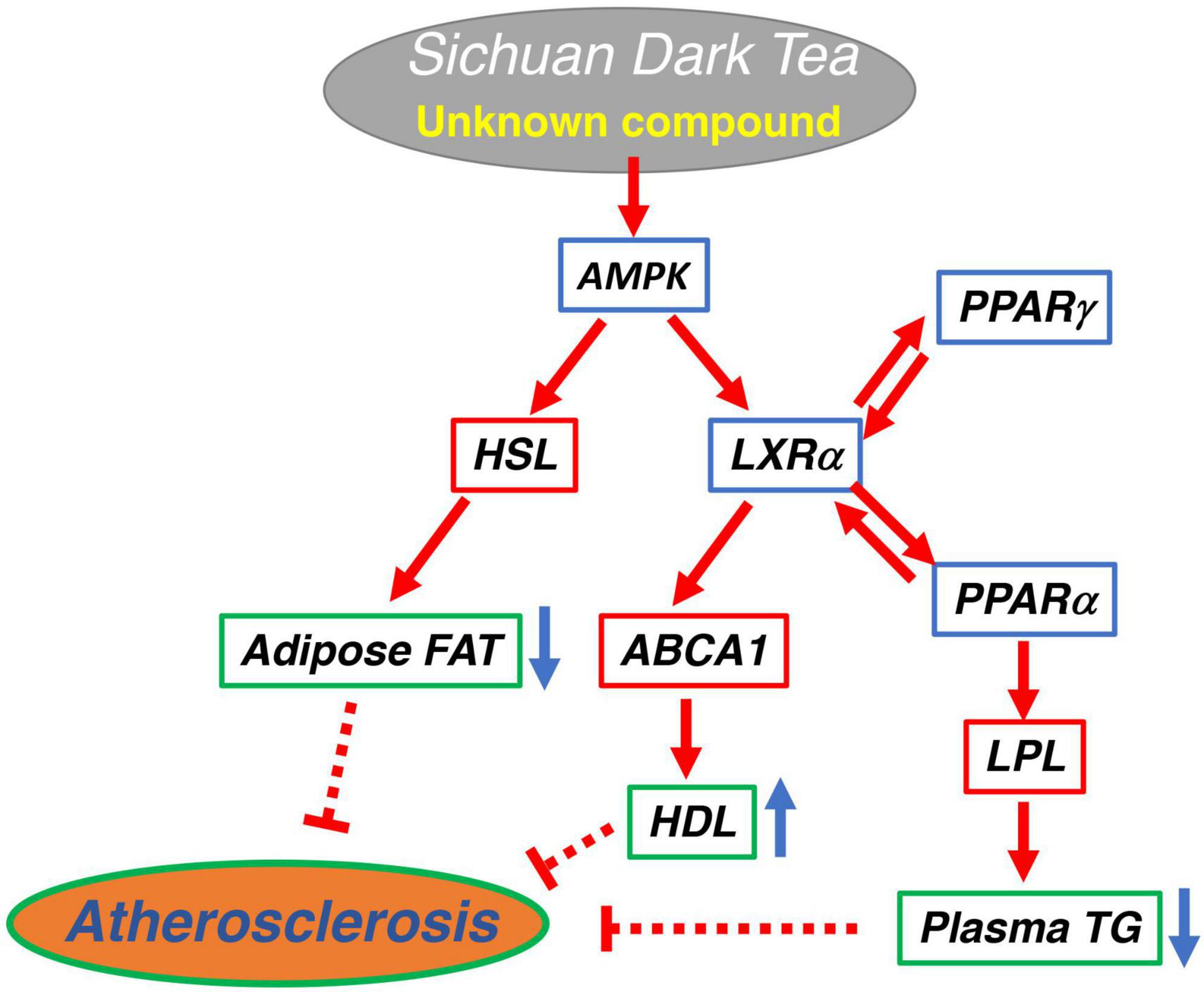
Figure 4. Graphical summary of the potential signaling pathway for anti-atherogenic effect of Sichuan dark tea.
Data availability statement
The raw data supporting the conclusions of this article will be made available by the authors, without undue reservation.
Ethics statement
This animal study was reviewed and approved by the Animal Experiments and Welfare Committee of Chubu University.
Author contributions
RL initiated the project, designed the experiments, carried them out herself, analyzed the data, and wrote the manuscript. TSu performed animal experiments for his master-degree graduate study. TT assisted the animal experiments and helped cellular experiments in vitro with her expertise. TSe contributed to animal experiments and cell culture mainly collaborating with TSu. MT helped animal experiment in evaluating intestinal physiology. XL provided the basic idea for the project on Sichuan black tea and contributed to obtaining the materials. SY supervised designing the projects and experiments, analyzing the data, and writing the manuscript. All authors contributed to the article and approved the submitted version.
Funding
This work was supported by the MEXT-Supported Program for Strategic Founding of Research in Private Universities (S1201007) and by Grants-in-aid from MEXT Japan (24614018, 26461370, 15H02903, 20K11591, and 21K11586).
Acknowledgments
We are thankful to Toshio Wada, Food and Nutritional Sciences, Chubu University for his valuable and helpful technical advice on HPLC analysis of the tea extracts.
Conflict of interest
The authors declare that the research was conducted in the absence of any commercial or financial relationships that could be construed as a potential conflict of interest.
Publisher’s note
All claims expressed in this article are solely those of the authors and do not necessarily represent those of their affiliated organizations, or those of the publisher, the editors and the reviewers. Any product that may be evaluated in this article, or claim that may be made by its manufacturer, is not guaranteed or endorsed by the publisher.
Abbreviations
ST, Sichuan dark tea; PT, Pu-erh tea; GT, Japanese green tea; HDL, high density lipoprotein; LXR, liver X receptor; PPAR, peroxisome proliferator-activated receptor; ABC, ATP-binding cassette transporter; HF, high fat diet group; TC, total cholesterol; HDL-C, HDL-cholesterol; non-HDL-C, non-HDL-cholesterol; TG, triglyceride; AMPK, 5 ′ adenosine monophosphate-activated protein kinase; DOR, dorsomorphin; SDS, sodium dodecylsulfate; HPLC, high performance liquid chromatography.
References
1. Kubota K, Sumi S, Tojo H, Sumi-Inoue Y, I-Chin H, Oi Y, et al. Improvements of mean body mass index and body weight in preobese and overweight Japanese adults with black Chinese tea (Pu-Erh) water extract. Nutr Res. (2011) 31:421–8. doi: 10.1016/j.nutres.2011.05.004
2. Huang Q, Chen S, Chen H, Wang Y, Wang Y, Hochstetter D, et al. Studies on the bioactivity of aqueous extract of pu-erh tea and its fractions: in vitro antioxidant activity and α-glycosidase inhibitory property, and their effect on postprandial hyperglycemia in diabetic mice. Food Chem Toxicol. (2013) 53:75–83. doi: 10.1016/j.fct.2012.11.039
3. Lee LK, Foo KY. Recent advances on the beneficial use and health implications of Pu-Erh tea. Food Res Int. (2013) 53:619–28.
4. Wang Y, Zhang M, Zhang Z, Lu H, Gao X, Yue P. High-theabrownins instant dark tea product by Aspergillus niger via submerged fermentation: α-glucosidase and pancreatic lipase inhibition and antioxidant activity. J Sci Food Agric. (2017) 97:5100–6. doi: 10.1002/jsfa.8387
5. Zhang S, Xuan H, Zhang L, Fu S, Wang Y, Yang H, et al. TBC2health: a database of experimentally validated health-beneficial effects of tea bioactive compounds. Brief Bioinform. (2017) 18:830–6. doi: 10.1093/bib/bbw055
6. Guo H, Fu MX, Wu DT, Zhao YX, Li H, Li HB, et al. Structural characteristics of crude polysaccharides from 12 selected chinese teas, and their antioxidant and anti-diabetic activities. Antioxidants. (2021) 10:1562. doi: 10.3390/antiox10101562
7. Song LB, Huang JN, Liu ZH, Huang H, Wang KB. Study on activity of dark tea extracts on PPARs model. J Tea Sci. (2008) 28:319–25. doi: 10.13305/j.cnki.jts.2008.05.002
8. Song LB, Huang JN, Liu ZH, Huang H, Wang KB. Study on the activity of dark tea extracts to FXR and LXR model. J Tea Sci. (2009) 29:131–5.
9. Zhang L, Ho CT, Zhou J, Santos JS, Armstrong L, Granato D. Chemistry and biological activities of processed Camellia sinensis teas: a comprehensive review. Compr Rev Food Sci Food Saf. (2019) 18:1474–95. doi: 10.1111/1541-4337.12479
10. He M, Lyu X. Application of BRAFO-tiered approach for health benefit-risk assessment of dark tea consumption in China. Food Chem Toxicol. (2021) 158:112615. doi: 10.1016/j.fct.2021.112615
11. Larrick JW. The methyl xanthine hypothesis: does tea consumption by Tibetan natives blunt the effects of high altitude? Med Hypotheses. (1991) 34:99–104. doi: 10.1016/0306-9877(91)90174-w
12. Cao J, Bai X, Zhao Y, Liu J, Zhou D, Fang S, et al. The relationship of fluorosis and brick tea drinking in Chinese Tibetans. Environ Health Perspect. (1996) 104:1340–3. doi: 10.1289/ehp.961041340
13. Wu J, Wang W, Liu Y, Sun J, Ye Y, Li B, et al. Modifying role of GSTP1 polymorphism on the association between tea fluoride exposure and the brick-tea type fluorosis. PLoS One. (2015) 10:e0128280. doi: 10.1371/journal.pone.0128280
14. Xie H, Li X, Ren Z, Qiu W, Chen J, Jiang Q, et al. Antioxidant and cytoprotective effects of tibetan tea and its phenolic components. Molecules. (2018) 23:179. doi: 10.3390/molecules23020179
15. Li L, Shi M, Salerno S, Tang M, Guo F, Liu J, et al. Microbial and metabolomic remodeling by a formula of Sichuan dark tea improves hyperlipidemia in apoE-deficient mice. PLoS One. (2019) 14:e0219010. doi: 10.1371/journal.pone.0219010
16. Wu X, Xie CQ, Zhu QQ, Wang MY, Sun B, Huang YP, et al. Green tea (Camellia sinensis) aqueous extract alleviates postmenopausal osteoporosis in ovariectomized rats and prevents RANKL-induced osteoclastogenesis in vitro. Food Nutr Res. (2018) 62. doi: 10.29219/fnr.v62.1478 [Epub ahead of print].
17. Deng X, Hou Y, Zhou H, Li Y, Xue Z, Xue X, et al. Hypolipidemic, anti-inflammatory, and anti-atherosclerotic effects of tea before and after microbial fermentation. Food Sci Nutr. (2021) 9:1160–70. doi: 10.1002/fsn3.2096
18. Jia YJ, Liu J, Guo YL, Xu RX, Sun J, Li JJ. Dyslipidemia in rat fed with high-fat diet is not associated with PCSK9-LDL-receptor pathway but ageing. J Geriatr Cardiol. (2013) 10:361–8. doi: 10.3969/j.issn.1671-5411.2013.04.007
19. Marques C, Meireles M, Norberto S, Leite J, Freitas J, Pestana D, et al. High-fat diet-induced obesity Rat model: a comparison between wistar and sprague-dawley rat. Adipocyte. (2016) 5:11–21. doi: 10.1080/21623945.2015.1061723
20. Xu Z, Sheng Y, Zeng G, Zeng Z, Li B, Jiang L, et al. Metabonomic study on the plasma of high-fat diet-induced dyslipidemia rats treated with ge gen qin lian decoction by ultrahigh-performance liquid chromatography-mass spectrometry. Evid Based Complement Alternat Med. (2021) 2021:6692456. doi: 10.1155/2021/6692456
21. Sasamoto K, Hirayama S, Kon M, Seino U, Ueno T, Nagao Y, et al. Changes in apolipoprotein E-containing high-density lipoprotein (HDL) have little impact on HDL-cholesterol measurements using homogeneous assays in normolipidemic and dyslipidemic subjects. Clin Chim Acta. (2017) 470:56–63. doi: 10.1016/j.cca.2017.04.018
22. Arakawa R, Tsujita M, Iwamoto N, Ito-Ohsumi C, Lu R, Wu CA, et al. Pharmacological inhibition of ABCA1 degradation increases HDL biogenesis and exhibits antiatherogenesis. J Lipid Res. (2009) 50:2299–305. doi: 10.1194/jlr.M900122-JLR200
23. Tsuboi T, Lu R, Yonezawa T, Watanabe A, Woo JT, Abe-Dohmae S, et al. Molecular mechanism for nobiletin to enhance ABCA1/G1 expression in mouse macrophages. Atherosclerosis. (2020) 297:32–9. doi: 10.1016/j.atherosclerosis.2020.01.024
24. Hu W, Abe-Dohmae S, Tsujita M, Iwamoto N, Ogikubo O, Otsuka T, et al. Biogenesis of HDL by SAA is dependent on ABCA1 in the liver in vivo. J Lipid Res. (2008) 49:386–93. doi: 10.1194/jlr.M700402-JLR200
25. Föger B, Ritsch A, Doblinger A, Wessels H, Patsch JR. Relationship of plasma cholesteryl ester transfer protein to HDL cholesterol. Studies in normotriglyceridemia and moderate hypertriglyceridemia. Arterioscler Thromb Vasc Biol. (1996) 16:1430–6. doi: 10.1161/01.atv.16.12.1430
26. Lin JK, Lin-Shiau SY. Mechanisms of hypolipidemic and anti-obesity effects of tea and tea polyphenols. Mol Nutr Food Res. (2006) 50:211–7. doi: 10.1002/mnfr.200500138
27. Ikeda I. Multifunctional effects of green tea catechins on prevention of the metabolic syndrome. Asia Pac J Clin Nutr. (2008) 17(Suppl. 1):273–4.
28. Xu P, Ying L, Wu J, Kong D, Wang Y. Safety evaluation and antihyperlipidemia effect of aqueous extracts from fermented puerh tea. Food Funct. (2016) 7:2667–74. doi: 10.1039/c5fo01389e
29. Abe-Dohmae S, Suzuki S, Wada Y, Aburatani H, Vance DE, Yokoyama S. Characterization of apolipoprotein-mediated HDL generation induced by cAMP in a murine macrophage cell line. Biochemistry. (2000) 39:11092–9. doi: 10.1021/bi0008175
30. Ogata M, Tsujita M, Hossain MA, Akita N, Gonzalez FJ, Staels B, et al. On the mechanism for PPAR agonists to enhance ABCA1 gene expression. Atherosclerosis. (2009) 205:413–9. doi: 10.1016/j.atherosclerosis.2009.01.008
31. Huang HC, Lin JK. Pu-erh tea, green tea, and black tea suppresses hyperlipidemia, hyperleptinemia and fatty acid synthase through activating AMPK in rats fed a high-fructose diet. Food Funct. (2012) 3:170–7. doi: 10.1039/c1fo10157a
32. Banerjee S, Ghoshal S, Porter TD. Phosphorylation of hepatic AMP-activated protein kinase and liver kinase B1 is increased after a single oral dose of green tea extract to mice. Nutr Res. (2012) 32:985–90. doi: 10.1016/j.nutres.2012.10.005
33. Rocha A, Bolin AP, Cardoso CA, Otton R. Green tea extract activates AMPK and ameliorates white adipose tissue metabolic dysfunction induced by obesity. Eur J Nutr. (2016) 55:2231–44. doi: 10.1007/s00394-015-1033-8
34. Ok WJ, Cho HJ, Kim HH, Lee DH, Kang HY, Kwon HW, et al. Epigallocatechin-3-gallate has an anti-platelet effect in a cyclic AMP-dependent manner. J Atheroscler Thromb. (2012) 19:337–48. doi: 10.5551/jat.10363
35. Wang S, Moustaid-Moussa N, Chen L, Mo H, Shastri A, Su R, et al. Novel insights of dietary polyphenols and obesity. J Nutr Biochem. (2014) 25:1–18. doi: 10.1016/j.jnutbio.2013.09.001
36. Fried NT, Elliott MB, Oshinsky ML. The role of adenosine signaling in headache: a review. Brain Sci. (2017) 7:30. doi: 10.3390/brainsci7030030
37. Olas B, Bryś M. Effects of coffee, energy drinks and their components on hemostasis: the hypothetical mechanisms of their action. Food Chem Toxicol. (2019) 127:31–41. doi: 10.1016/j.fct.2019.02.039
38. Wulandari RA, Amano M, Yanagita T, Tanaka T, Kouno I, Kawamura D, et al. New phenolic compounds from Camellia sinensis L. leaves fermented with Aspergillus sp. J Nat Med. (2011) 65:594–7. doi: 10.1007/s11418-011-0515-0
39. Kanegae A, Sakamoto A, Nakayama H, Nakazono Y, Yakashiro I, Matsuo Y, et al. New phenolic compounds from Camellia sinensis L. fermented leaves. J Nat Med. (2013) 67:652–6. doi: 10.1007/s11418-012-0704-5
40. Xie HF, Kong YS, Li RZ, Nothias LF, Melnik AV, Zhang H, et al. Feature-based molecular networking analysis of the metabolites produced by in vitro solid-state fermentation reveals pathways for the bioconversion of epigallocatechin gallate. J Agric Food Chem. (2020) 68:7995–8007. doi: 10.1021/acs.jafc.0c02983
41. Zhu MZ, Li N, Zhou F, Ouyang J, Lu DM, Xu W, et al. Microbial bioconversion of the chemical components in dark tea. Food Chem. (2020) 312:126043. doi: 10.1016/j.foodchem.2019.126043
42. Gong ZP, Ouyang J, Wu XL, Zhou F, Lu DM, Zhao CJ, et al. Dark tea extracts: chemical constituents and modulatory effect on gastrointestinal function. Biomed Pharmacother. (2020) 130:110514. doi: 10.1016/j.biopha.2020.110514
43. Xiao Y, Li M, Wu Y, Zhong K, Gao H. Structural characteristics and hypolipidemic activity of theabrownins from dark tea fermented by single species Eurotium cristatum PW-1. Biomolecules. (2020) 10:204. doi: 10.3390/biom10020204
44. Yan K, Abbas M, Meng L, Cai H, Peng Z, Li Q, et al. Analysis of the fungal diversity and community structure in sichuan dark tea during pile-fermentation. Front Microbiol. (2021) 12:706714. doi: 10.3389/fmicb.2021.706714
Keywords: atherosclerosis, dark tea, HDL, triglyceride, ABCA1, lipases
Citation: Lu R, Sugimoto T, Tsuboi T, Sekikawa T, Tanaka M, Lyu X and Yokoyama S (2022) Sichuan dark tea improves lipid metabolism and prevents aortic lipid deposition in diet-induced atherosclerosis model rats. Front. Nutr. 9:1014883. doi: 10.3389/fnut.2022.1014883
Received: 09 August 2022; Accepted: 28 October 2022;
Published: 24 November 2022.
Edited by:
Xiaohua Liang, Children‘s Hospital of Chongqing Medical University, ChinaReviewed by:
Hong-Yan Liu, Institute of Urban Agriculture (CAAS), ChinaJian Mao, Jiangnan University, China
Copyright © 2022 Lu, Sugimoto, Tsuboi, Sekikawa, Tanaka, Lyu and Yokoyama. This is an open-access article distributed under the terms of the Creative Commons Attribution License (CC BY). The use, distribution or reproduction in other forums is permitted, provided the original author(s) and the copyright owner(s) are credited and that the original publication in this journal is cited, in accordance with accepted academic practice. No use, distribution or reproduction is permitted which does not comply with these terms.
*Correspondence: Shinji Yokoyama, c3lva295YW1AaXNjLmNodWJ1LmFjLmpw
†Present address: Takumi Sugimoto, Graduate School of Environmental and Life Sciences, Kyoto Prefectural University, Kyoto, Japan; Tomoe Tsuboi, School of Pharmacy, Aichi Gakuin University, Nagoya, Japan