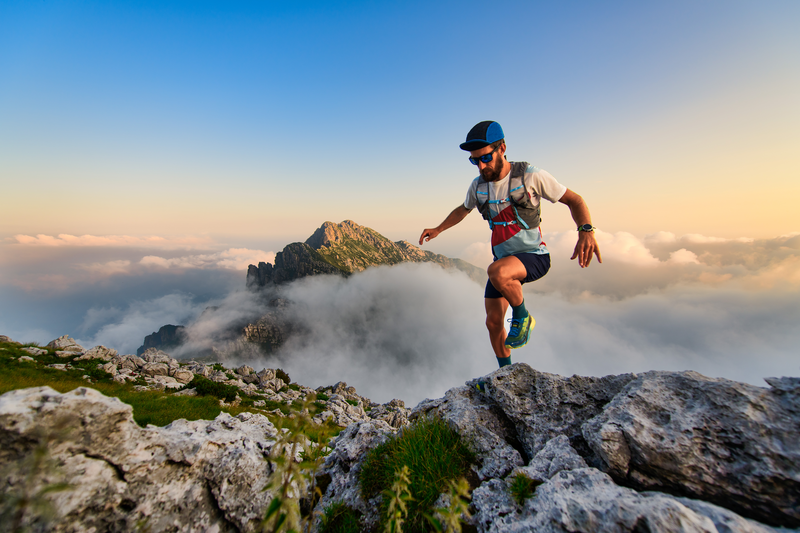
95% of researchers rate our articles as excellent or good
Learn more about the work of our research integrity team to safeguard the quality of each article we publish.
Find out more
REVIEW article
Front. Nutr. , 20 September 2022
Sec. Nutrition and Microbes
Volume 9 - 2022 | https://doi.org/10.3389/fnut.2022.1012087
Osteoarthritis (OA) is a common chronic degenerative disease of articular cartilage in middle-aged and older individuals, which can result in the joint pain and dysfunction, and even cause the joint deformity or disability. With the enhancing process of global aging, OA has gradually become a major public health problem worldwide. Explaining pathogenesis of OA is critical for the development of new preventive and therapeutic interventions. In recent years, gut microbiota (GM) has been generally regarded as a “multifunctional organ,” which is closely relevant with a variety of immune, metabolic and inflammatory functions. Meanwhile, more and more human and animal researches have indicated the existence of gut-bone axis and suggested that GM and its metabolites are closely involved in the pathogenic process of OA, which might become a potential and promising intervention target. Based on the close coordination of gut-bone axis, this review aims to summarize and discuss the mechanisms of GM and its metabolites influencing OA from the aspects of the intestinal mucosal barrier modulation, intestinal metabolites modulation, immune modulation and strategies for the prevention or treatment of OA based on perspectives of GM and its metabolites, thus providing a profound knowledge and recognition of it.
Osteoarthritis (OA) is a common chronic degenerative disease of articular cartilage in middle-aged and older individuals, mainly manifested as the progressive destruction of articular cartilage, subchondral sclerosis and hyperplasia, periarticular osteophyte formation and synovial lesions, which can result in the joint pain and dysfunction, and even cause the joint deformity or disability (1–3). With the enhancing process of global aging, OA has gradually become a major public health problem worldwide and the main source of social medical expenses for middle-aged and older individuals (4). According to the previous study, by 2020, OA has become the fourth most prevalent disease in the world (5, 6). Meanwhile, due to the high prevalence of OA and the consequent severe consequences for patients, their families and the social medical service system, OA has gradually become a continuous research hotspot in current scientific fields (7, 8).
As a kind of complex chronic degenerative disease, the etiology and pathogenesis of OA are still unclear, and the corresponding treatment or intervention approaches are relatively limited. The current strategies of OA mainly include drug treatment, physical intervention and surgeries. Hence, based on the original cognition of OA (progressive destruction of articular cartilage, subchondral sclerosis and hyperplasia, periarticular osteophyte formation, and synovial lesions), a deeper explanation of the underlying mechanisms of OA is crucial for the development of prevention and treatment strategies. Furthermore, more and more evidence has suggested that gut microbiota (GM) and its metabolites play a vital role in the occurrence and progression of OA (9). As the human intestine contains a large number of microorganisms, GM stores a huge genome, which is about 150 times the number of human genes. Thus, GM is considered to be the second gene bank of human beings, making it an inseparable part of the human body (10–12). Moreover, GM plays a crucial role in the health of host from birth, and the interactions between the intestinal epithelial cells, microorganisms and its metabolites are the key mediators between intestinal epithelium and other cell types (13). This interaction also contributes to maturation of intestinal epithelium, intestinal nervous system, intestinal vascular system and innate immune system (14). In addition, GM influences the basic physiological functions of host (such as digestion and absorption, energy metabolism, and immune defense) through the neuromodulation, immune modulation and endocrine modulation, so as to maintain the homeostasis of internal environment (15).
In recent years, more and more researches have suggested that the dysregulation of GM and its metabolites is not only involved in the pathogenic process of the intestinal diseases (such as inflammatory bowel disease, Crohn’s disease, and colon cancer), but also the imbalance of intestinal homeostasis may induce several extraintestinal immune and metabolic diseases (such as osteoporosis, psoriasis, systemic lupus erythematosus, and OA) (16–21). Regarding this, Sheth et al. (22) showed that approximately 10–40% of patients with inflammatory bowel disease may suffer from at least one extraintestinal lesion, with the most frequent occurrences being musculoskeletal lesions, such as OA and osteoporosis. Schule et al. (23) observed patients in a cohort study of inflammatory bowel disease in the Switzerland, and suggested that 57% of them were combined with osteopenia, 20% were combined with osteoporosis, and part of the remaining patients were combined with OA. Hence, the close correlation between GM and the occurrence and progression of OA can be gradually recognized by the academic circles, which also drives more researchers to explore the advanced nature and profound connotation of OA from the perspective of intestinal microecology. On the basis of close coordination of gut-bone axis (24), this review aims to summarize and discuss the mechanisms of GM and its metabolites influencing OA from the aspects of intestinal mucosal barrier modulation, intestinal metabolites modulation, immune modulation and strategies for the prevention or treatment of OA based on the perspectives of GM and its metabolites, thus providing a profound knowledge and recognition of it.
Gut microbiota is the critical factor to activate and maintain intestinal physiological function, and plays an indelible role in maintaining the health and homeostasis of the host. Under normal circumstances, GM maintains a dynamic balance with the host and the external environment (25). Moreover, it is generally recognized that the earliest “immigrants” are the microorganisms in birth canal or the environment that babies come into contact with at birth. At the beginning of the baby’s birth, there is a lot of oxygen in the intestine, mainly due to the colonization of partial bacteria that need oxygen, such as Escherichia coli and Staphylococcus (26). When they gradually exhausted of oxygen and PH in the intestine decreases, some anaerobic bacteria, such as Bifidobacteria and Bacteroidetes, begin to colonize. As the baby’s age increases, various bacteria are in the process of mutual influence. Until the baby is 2 years old, a stable GM structure similar to that of adults can be formed (27). After reaching the old age, the decline of GM diversity with the increase of age may be the result of changes in various lifestyles. Bacteria become less diverse, and the number of beneficial microorganisms, such as Lactobacillus and Bifidobacteria, decreased in number and lost its dominant share (28). These factors also contribute to the imbalance of intestinal microecology and are related to the increased risk of various age-related chronic diseases, such as heart disease, Parkinson’s disease and Alzheimer’s disease (29).
Gut microbiota is symbiotic with host, which affects several vital links, such as nutrition intake, food digestion, systemic metabolism, development of immunity, physiological function maintenance and diseases defense of the human bodies (30, 31). Meanwhile, especially in recent years, more and more human and animal studies have indicated the existence of gut-bone axis and suggested that GM and its metabolites are closely involved in the pathogenic process of OA, which might become a potential and promising intervention target (32, 33). On this basis, Gleason et al. (34) emphasized the role of gut-joint axis in the pathogenic process of OA, and recognized that the gut-joint axis was proposed as an association involving the gastrointestinal microbiome, immune and inflammatory response that it induced, and the overall joint health. Meanwhile, the gut is regarded as an intriguing and novel target for the therapy of OA, and the dietary modification or supplementation with fiber, probiotics, or prebiotics could provide a positive influence on the gut-joint axis (35, 36).
Obesity is a significant risk factor for inducing the OA. In the traditional sense, the excessive joint wear caused by excessive local stress of obese joints is the main cause of OA. However, more and more researches have suggested that excessive joint load cannot fully explain the relationship between obesity and OA (37, 38). Obesity can also increase the prevalence of OA in non-weight-bearing joints, such as hand joints (39, 40). It can be seen that non-mechanical factors are involved in the pathological process of obese OA (41). In this process, the imbalance of GM and its metabolites is a vital trigger factor for the enhancement of inflammation level, and also participates in the occurrence of OA in obese body. Collins et al. (42) showed that the number of intestinal Lactobacillus decreased in obese rats, and the abundance of this bacteria was negatively correlated with the levels of inflammatory factors in the blood, synovial fluid and the Mankin score of joints. Meanwhile, the study also indicated that the imbalance of GM and increase of intestinal permeability resulted in enhancement of lipopolysaccharide (LPS) into the blood, and the inflammatory response induced by LPS may be involved in the pathogenesis of OA. Li et al. (43) emphasized the key significance of analyzing the role of nutrients-GM-bacterial metabolites axis in the pathogenesis of OA from different perspectives such as obesity, aging and gender. From the perspective of obesity, the metabolic inflammation, insulin resistance and other diseases caused by the disorder of GM and its metabolites may accelerate the pathological process of OA.
With regard to this, as for the human researches, a well-known perception is that the prevalence and severity of OA in women are much higher than that in men, and the incidence and severity of OA in women after menopause are significantly higher than that before menopause (44). In this process, GM may modulate the immune response by influencing the sex hormones. For example, Clostridium can convert glucocorticoids into androgens, and the diversity and richness of bacterial species are positively related to the concentration of serum androgens (45, 46). As GM is involved in the excretion and circulation process of sex hormones, the concept of “microgenderome” indicating the role of sex hormone on the GM has been gradually proposed (47). Meanwhile, GM also presents a dynamic change process in body, and the types of GM and beneficial bacteria enhance from the infants. When body begins to decline with age, the abundance and diversity of GM also decreases, and harmful bacteria increases (48). A population-based study conducted by the Xiangya OA team (49) included a total of 1388 middle-aged and older individuals, and revealed that the alterations in the composition of GM were observed among study participants who had symptomatic hand OA, and a low relative abundance of Roseburia but high relative abundance of Bilophila and Desulfovibrio at genus level were associated with prevalent symptomatic hand OA. In a population-based study, Rushing et al. (50) revealed that the participants with obesity and knee plus hand OA had distinct fecal metabolomes characterized by the enhanced products of proteolysis, perturbations in leukotriene metabolism, and alterations in the microbial metabolites compared with healthy controls. Chen et al. (51) analyzed GM of 57 patients with OA and a corresponding number of healthy controls, and observed that the abundance and diversity of GM in the patients with OA reduced significantly, mainly manifested in the decrease of Bifidobacterium longum and Faecalibacterium, while the level of Clostridium enhanced. A prospective study led by Wang et al. (52) included 182 stool samples from overweight OA patients (86 cases) and overweight normal subjects (96 cases) and found that the diversity and richness of GM in the overweight OA patients decreased. Accordingly, there were 9 branches and 87 genera with significant difference between the overweight OA patients and overweight normal subjects. Lee et al. (53) reported that the ratio of Bacteroidetes and Firmicutes can be regarded as one of potential indicators to predict the occurrence and severity of OA and rheumatoid arthritis, but whether other specific species and genera can also play a predictive role needs to be further explored in the future researches. The literatures regarding the GM and OA in population-based studies are summarized in Table 1 (54–56). Hence, it could be acknowledged that more and more evidence indicate that GM participates in the pathological modulation of OA (57), and exhibits differences in composition, abundance and diversity at the different levels. Besides, the gender, age, dietary habits, obesity, lifestyle and other factors might also be the risk factors linking between the GM and OA.
In addition, as for the animal researches, Guan et al. (58) indicated that in the mice with injury-induced OA, the imbalance of GM induced by antibiotics could reduce the serum LPS level and inflammatory response, and further improve the OA after joint injury. Collins et al. (42) explored the link between GM, systemic LPS levels, serum and local inflammatory profiles and OA in a high fat/high sucrose diet-induced obese rat model, and discovered the close interaction relationship between GM and adiposity-derived inflammation and metabolic OA. Ulici et al. (59) observed that compared with specific pathogen free (SPF) mice with injury-induced OA, the germ-free (GF) mice were equipped with better synovial score and articular cartilage structure, indicating the factors related to GM promoted the development of OA after joint injury. Moreover, Hahn et al. (60) compared the early responses to injury-induced OA in the conventional and GF mice, and revealed that the differences in response to injury in GF compared to conventional mice were related to metabolic pathways that modulated the inflammation associated with the innate immune system. The literatures regarding the GM and OA in animal studies are summarized in Table 2 (61–64). Hence, on the basis of these animal studies, the summarized evidence gradually exhibited a direct gut-bone communication that set the stage for novel approaches of exploring OA pathogenesis and potentially new OA therapeutics.
The intestinal barrier is composed of mechanical barrier, chemical barrier, immune barrier and biological barrier. The mechanical barrier is mainly composed of intestinal mucosal epithelial cells, intercellular tight junctions and bacterial membranes, which could effectively prevent harmful tissues, such as bacteria and endotoxin, from entering the blood through the intestinal mucosa (65, 66). The chemical barrier consists of mucus secreted by epithelium, digestive juice and antibacterial materials produced by parasitic bacteria (67). The immune barrier is composed of intestinal mucosal lymphoid tissues (mesenteric lymph nodes and intraepithelial lymphocytes), which could generate local mucosal immune response and specifically secrete secretory immunoglobulin A (sIgA) to prevent the damage of pathogenic antigens (68). The biological barrier is composed of the microspatial structure formed between the normal parasitic flora of intestinal tract and host intestinal tissue (69). Hernandez et al. (70) showed that GM and its metabolites can cause the abnormal intestinal mucosal barrier function by altering intestinal nutrient absorption, inducing the changes in intestinal immune function and abnormal intestinal endothelial transport, thus inducing the occurrence and progression of extraintestinal diseases, such as OA and osteoporosis.
The permeability of intestinal mucosa generally allows substances with a molecular weight >150 KD to readily pass through the intestinal epithelium and then enter into the circulation (71). Therein, the permeation modes of intestinal epithelial permeability include transepithelial pathway and paracellular pathway. The transepithelial pathway is that nutrients are absorbed from the intestinal cavity through the intestinal mucosal epithelium and enter into the circulation, while selectively restricting the passage of harmful substances, such as endotoxin and inflammatory factors (72). The paracellular pathway is the passive diffusion of the intestinal molecules through the epithelial space, which is controlled by the tight junction between the epithelial cells and modulates the passage of intestinal microflora products and other macromolecules (73). The changes of intestinal mucosal permeability are related to various factors, including exogenous factors, nutritional factors, several kinds of cytokines, and effects of immune cells. The alterations of GM can affect the absorption of vitamin B (Vit B), vitamin C (Vit C), vitamin D (Vit D), vitamin K (Vit K) and tetrahydrofolate, metabolism of carbohydrate and fats, the synthesis of short chain fatty acids (SCFAs), thus influencing the stability, growth and progression of intestinal mucosal cells. During this process, the primary focus of metabolites mainly includes SCFAs, 5-hydroxytryptamine (5-HT), tryptophan-related metabolites, and so on.
Importantly, SCFAs are the main products of GM fermenting resistant starch and non-starch polysaccharides (the main components of dietary fiber) (74). When GM is disturbed, the types and contents of SCFAs in the intestine could be significantly altered. Therein, the acetic acid, propionic acid and butyric acid have been generally verified by several researches to be involved in the bone metabolism (75, 76). Collins et al. (42) revealed that high-fat diet (HFD) can significantly reduce the amount of Lactobacillus and the synthesis of SCFAs in the intestine, thereby decreasing the energy supply of intestinal cells, and the bacterial abundance was closely associated with the levels of inflammatory factors in the blood and synovial fluid of the rat models with OA. As one of the significant components of intestinal mucosal barrier, the expression of the tight junction protein, zonula occludens 1 (ZO-1), is prone to be reduced by HFD, and the intestinal permeability also alters, resulting in a large amount of LPS entering systemic circulation (77). Meanwhile, HFD also induces large amounts of saturated fat into the intestine, enhances the number of activated macrophages, thus releasing additional pro-inflammatory mediators and adipokines, directly amplifying the inflammatory response and aggravating the clinical outcome of OA (78). Based on this, LPS widely exists in the outer membrane of intestinal Gram-negative bacteria that can induce inflammatory response, and its excessive translocation into the body circulation might be one of the predisposing factors of OA. LPS can also activate innate immune system cells, such as macrophages and neutrophils, and synthesize the proinflammatory cytokines, including interleukin (IL)-1β, tumor necrosis factor (TNF)-α and so on, resulting in the secondary inflammation in various joints throughout the body (57).
In addition to this, 5-HT could disrupt the dynamic balance between bone formation and bone resorption, thereby influencing the maintenance of bone mass. GM plays an important role in the production of 5-HT, and previous studies have also indicated that GM can stimulate the chromaffin cells to produce 5-HT, and microorganisms can also regulate gastrointestinal motility via the neural secretion mechanism activated by toll like receptors (TLRs) (79, 80). Besides, 5-HT has dual effects on bone metabolism. As a hormone, intestine-derived 5-HT can inhibit bone formation, and as a neurotransmitter, the brain-derived 5-HT can promote bone formation through a series of pathways (81). Tryptophan is a kind of aromatic amino acid, which can act as the biosynthetic precursor of certain microorganisms and host metabolites, thereby modulating the physiological functions of the body (82). Tryptophan is mainly metabolized in intestine through the following three pathways: (1) enterochromaffin cells synthesize most of the 5-HT in the circulation via tryptophan hydroxylase 1 (TPH 1); (2) partial metabolites can induce the indoleamine 2,3-dioxygenase 1 (IDO1) and promotes the metabolism of tryptophan to kynurenine; (3) under the action of GM, tryptophan is directly converted into the indoles and acts as an aromatic hydrocarbon receptor ligand (83).
In addition, GM could also modulate and inhibit the dietary inflammatory response produced in the daily intake of foods, which also play a critical role as antigens in the immune system (84). As a kind of transmembrane transporter, the microbe-associated molecular patterns (MAMPs) could affect the physiological activities of distant organs and tissues after entering the systemic circulation. Kim et al. (85) reported that MAMPs could directly influence the bone remodeling by stimulating innate immune receptors on osteocytes, including modulating the balance between osteoblasts and osteoclasts. When the MAMPs are translocated, the living bacteria could pass through the intestinal mucosal barrier, the translocated bacteria are usually quickly cleared by immune cells, and the uncleared bacteria enter with MAMPs and stay in the intestinal mucosal barrier. After inducing the death of immune cells within the barrier, a small amount of MAMPs may be released into systemic circulation. Furthermore, as MAMPs circulate to distant organs and tissues, such as several joints of limbs, MAMPs can activate the immune response and produce the local inflammation (86). Partial bacteria that pass through the intestinal mucosal barrier can penetrate the natural cells and survive, thus avoiding the impact of inflammatory response (87). Generally, it can be recognized that the changes of intestinal mucosal permeability and the transport of GM-related metabolites across the intestinal mucosal barrier could influence the occurrence and progression of OA to a certain extent.
There is a close relationship between GM and intestinal mucosal immune system, and the degree of change in intestinal microecology determines the stability of intestinal mucosal immune system and systemic immune system. The direct contact between GM, its metabolites and immune cells stimulates the immune response of intestinal mucosal barrier (88–91). The immune cells (such as T cells and dendritic cells) can interact with GM, and migrate to lymph nodes to activate the pro-inflammatory or anti-inflammatory responses. Meanwhile, these cells are also able to release soluble pro-inflammatory or anti-inflammatory mediators or cytokines into the systemic circulation and participate in the modulation of systemic bone remodeling (92, 93). In addition to this, TLR also serve as a bridge between the intestinal mucosal barrier, GM and the innate immune system, and play a crucial role in the formation of GM. The intestine is rich in dietary and microbial antigens, and the human immune system needs to tolerate these antigens to create conditions for survival of GM. TLR is one of the significant immunosensors, which maintains the balance of GM in the whole body (94). TLR exists in a variety of innate immune system cells, such as macrophages, dendritic cells (DCs) and intestinal epithelial cells, which could recognize the pathogenic microorganisms and induce the production of antibacterial products, so as to modulate immune response (95–97). There is also a critical link between the innate immune response and cartilage damage, which affects the development of OA. Articular cartilage damage caused by several factors, such as aging and obesity, might trigger the release of autoantigens and damage-associated molecular patterns (DAMPs), thereby activating the innate immune response (the activation of immune cells, activation of complement system and release of anti-cartilage components in the immune complexes), resulting in the generation of sterile inflammation and further damage to the cartilage, and forming a vicious circle (98, 99).
As a widely reported immunomodulator, GM mainly stimulates the host intestinal wall to collect the lymph nodes through the bacteria itself or cell wall components, and generates immune response to bacteria (100, 101). During this process, the lymphocyte activation transmits the immune response to the entire intestinal mucosa, and then forms secretory IgA globulin to cover the mucosal surface. Regarding this, IgA globulin is the largest amount of immunoglobulin secreted by the body, which could prevent the adhesion of pathogenic microorganisms in intestine from attaching to mucosal surface, neutralize bacterial toxins, and play a synergistic bactericidal role with complement and lysozyme (102, 103). Moreover, GM could directly modulate the immune cells, such as Prevotella copri can promote the differentiation of regulatory T (Tregs) cells and Th17 cells in the intestine, and Tregs cells induce the production of retinoic acid-related orphan receptor γt (RORγt) to further promote the differentiation of Th17 cells (80, 104). On the basis of this, GM could modulate the type II hypersensitivity through Th17 cells and Tregs cells, so as to balance the immune response on the surface of intestinal mucosa (105). Moreover, Ivanov et al. (106) indicated that the segmented filamentous bacteria (SFB) can induce the differentiation of Th17 cells, and Bacteroides fragilis can also induce the differentiation of Th1 cells and Treg cells. Atarashi et al. (107) proposed that when the GM subsets (such as SFB, Citrobacter, Escherichia coli O157 and certain extracellular pathogens) colonized and adhered to the intestinal epithelial cells, the intestinal Th17 cells were then induced and aggregated. In a recent study involving the oral-GM axis, du Teil Espina et al. (108) noticed that one of the causes of the rheumatoid arthritis was the alterations of oral bacteria, and the oral diseases (such as periodontitis) could result in the GM disorder, and its diversity and abundance may also be reduced, while the formation of neutrophils and biofilm plays an indelible role in this process. Besides, intestinal citrullinated proteins mediated by oral pathogens (Porphyromonas gingivalis and Actinobacillus) can promote the production of anti-citrullinated protein antibodies (109, 110). In this process, the overgrown GM might strengthen the response of Th1 cells and further inhibit the activation of Tregs cells by inhibiting the growth of B. fragilis, leading to immune imbalance and the occurrence and progression of rheumatoid arthritis (111). Hence, it is recognized that the in-depth exploration of immune modulation caused by the alterations and dysregulation of GM provides a promising intervention target for the prevention and treatment of OA in the future.
During the process of ongoing researches, the researchers unexpectedly observed that in the treatment of the patients with intestinal or extraintestinal diseases caused by intestinal microecological disorders, partial patients with OA exhibited joint pain relief and improved exercise ability with the advancement of treatment process (112), which provided a novel perspective and ideas for the basic researches and clinical treatment of OA. With regard to this, the intestine is the main site of nutrient absorption in human bodies, and the daily dietary intake and supplementation of auxiliary substances has a profound and dynamic impact on the composition and function of GM (113–115). Diets play a significant role in shaping the structure and function of GM, and the GM triggers numerous signal molecules with host receptors by metabolizing food-derived SCFAs and signaling molecules, such as trimethylamine oxide (TMAO), directly or indirectly influencing the distant organs (bone, kidney, brain, skin, and so on) (116, 117). The beneficial metabolites of specific foods contribute to enriching the intestinal beneficial bacteria to play a positive role in modulating the homeostasis of body (118, 119). Hence, the structure and function of GM can also be modulated by supplementing probiotics and prebiotics. Probiotics cannot only restore the bone loss caused by hypogonadism or ovariectomy, but also work to improve the inflammatory bone-related diseases (120).
Diet is closely related to the pathogenesis of OA, and the selenium, magnesium and vitamins play a significant role in the pathogenesis of OA (121–123). Moreover, high-glucose diets and HFDs are also closely associated with the OA. The glucose plays an important role in the growth and development of articular cartilage, and the disorder of glucose metabolism may aggravate the progression of OA (124). More and more recent studies have revealed that GM also has a regulatory effect on the blood glucose, and the number of Akkermansia muciniphila (A. muciniphila) in the type II diabetic and obese mice is significantly reduced. However, when the mice are fed with polyphenol-rich cranberry extract, the number of A. muciniphila increases significantly, and the glucose tolerance and insulin sensitivity are improved accordingly (125, 126). Similarly, the excessive intake of HFD might also result in the alterations of GM, and Nguyen et al. (127) also reported that the supplementation of Lactobacillus plantarum PH04 could effectively reduce the cholesterol and triglyceride levels of hypercholesterolemia mice. Ramasamy et al. (55) suggested in a pilot study that Parabacteroides, Butyricimonas, Pseudobutyrivibrio, Odoribacter, and Gordonibacter are predominant bacteria in Vit D deficient patients with or without Knee OA, and these results noticed a non-negligible link between GM, Vit D and Knee OA. Thus, it is recognized that the diets-GM axis is closely associated with the glucose and lipid metabolism of the body, and altering the intake of dietary nutrients might be an effective approach to alleviate OA.
In clinical practice, the proper supplementation of microecological regulators tends to be used to correct the intestinal microecological disorders and maintain homeostasis modulation. Currently, the probiotics and prebiotics are widely used in clinic and daily life (128). On one hand, the probiotics are a kind of microorganisms that have beneficial effects on the host after sufficient intake, and play an active role mainly by altering the composition and structure of GM and metabolic activities. By supplementing sufficient probiotics, supporting the beneficial bacteria in the body could improve the resistance to pathogenic bacteria, modulate the function of intestinal mucosal barrier, optimize the intestinal environment, and maintain the immune balance of body (129–131). Tyagi et al. (132) observed that the supplementation of Lactobacillus rhamnosus GG is able to increase the butyric acid-producing bacteria in the intestine of mice, promote the production of butyric acid, and then improve the expression of Wnt10b and increase the bone mass through the Tregs cells, so as to participate in the modulation of bone metabolism. Pan et al. (133) revealed that Lactobacillus casei can correct the imbalance of GM, restore the abundance of Lactobacillus gasseri, Lactobacillus reuteri, and Lactobacillus vaginalis to normal levels, and enhance the abundance of Lactobacillus acidophilus, thereby protecting the bones from destruction in adjuvant-induced arthritis (AIA) rats. Henrotin et al. (134) administered orally a freeze-dried inactivated culture of the Bifidobacterium longum CBi0703 in a spontaneous model of OA in guinea pigs, and observed that the cartilage structural injury and the degradation of type II collagen caused by OA were significantly reduced, which has potential to prevent and treat OA. Sim et al. (135) also regularly fed the tyndallized Clostridium butyricum to the rats to treat or alleviate OA, and experimental results revealed that the levels of inflammatory indicators and bone metabolism markers in the serum were significantly reduced, while the concentrations of interferon-γ (IFN-γ) and glycosaminoglycans were significantly enhanced. As a consequence, the application of tyndallized C. butyricum also effectively protected the cartilage and synovium of knee joint, and reduce the formation of fibrous tissue. In addition to this, by comparing applications of probiotic containing capsules, including the Lactobacillus rhamnosus, Saccharomyces cerevisiae (boulardii), and Bifidobacterium animalis ssp. lactis, and placebo capsules without probiotics to the participants with OA, Taye et al. (136) found that although the reduction of pain scores related to probiotic intervention was relatively small, the application of probiotics had a clinical practical significance for the pain management of OA patients. Moreover, as a newly founded bacterium from human breast milk, TCI633 (Streptococcus thermophilus) is able to produce hyaluronate in gastrointestinal tract. Lyu et al. (137) recruited 80 patients with knee OA and conducted a double-blind controlled study with TCI633 and placebo, and the results suggested that TCI633 might be able to delay the progression of knee OA.
On the other hand, the prebiotics are a kind of indigestible food components that can play a beneficial role in the host by altering the composition of GM and the activity of partial GM, or being fermented by partial GM, and can also stimulate and activate intestinal probiotics (138, 139). Prebiotics are considered as the auxiliary substrates for the bacterial activation and growth promotion, which can resist the action of hydrolytic enzymes and a variety of proteases, and are converted into SCFAs after being fermented by GM (140). Schott et al. (141) observed that Bifidobacterium in the intestine of obese rats were significantly reduced, and the alterations of GM resulted in the accumulation of downstream systemic inflammatory signals along with macrophages to the synovium of joints, which accelerated the progression of OA. However, the restoration of GM by the addition of Fructooligosaccharides decreased the systemic levels of inflammation and alleviated the OA-related symptoms. Therein, the relevant mechanism may be that the supplementation of Fructooligosaccharides can increase the level of Lactobacillus, thus maintaining the intestinal mucosal barrier, preventing bacterial endotoxin or LPS translocation, and inhibiting the proliferation of Enterobacteriaceae. Tanabe et al. (142) further explored the effects of prebiotics on bone metabolism in the aging mice, and the results indicated that Fructooligosaccharides and Glucomannan could inhibit the bone absorption and improve the bone metabolism in aging mice, and further obtain the aim of increasing femoral calcium contents mainly by enhancing the level of Lactobacillus, Bacteroides and Clostridium. Furthermore, Fructooligosaccharides are not easy to be absorbed by the human bodies, and can resist the digestive reaction of intestine, so that Fructooligosaccharides could reach the colon smoothly after intaking it, become the growth matrix to promote GM optimization, and indirectly have corresponding effects on the human bodies (143, 144). Meanwhile, Wu et al. (145) also indicated that the direct oral administration of Fructooligosaccharides could alleviate the inflammatory response of mouse pups without altering the type, quantity and abundance of GM in mice. This result suggested that the prebiotics might also play a direct role in immune modulation without the intervention of GM. Hence, especially for the obesity-related OA (mainly manifested as an inflammatory reaction process), which is related to the obesity-related GM dysregulation, and could restore a healthy microbial community via the use of indigestible prebiotic fiber Fructooligosaccharides. Fructooligosaccharides can induce the alterations in the abundance of key microorganisms in obese individuals, decrease the response characteristics of colon macrophages, reduce the systemic and joint inflammation, protect the articular cartilage, and further alleviate the progression of OA (146, 147). Thus, more and more evidence has revealed that the supplementation of probiotics and prebiotics could reduce the expression of pro-inflammatory cytokines and cartilage-degrading factors in several joint tissues, enhance the production of anti-inflammatory factors and cartilage matrix synthesis cytokines, so as to inhibit combined inflammation and cartilage destruction, and alleviate the development process of OA.
Furthermore, as a research focus in recent years, fecal microbiota transplantation (FMT) often refers to the transplantation of bacteria in the feces of donor to the recipient by intragastric or oral administration, thereby altering the composition, structure and abundance of GM in the recipients (148, 149). During this process, FMT can reshape the intestinal microenvironment, improve the inflammation, immunity and metabolism of the recipient’s gut, and provide a new treatment concept and method for a variety of intestinal and parenteral diseases (150). Huang et al. (151) suggested that FMT from the metabolically compromised human donors was able to accelerate the process of OA in mice, and the alterations of Fusobacterium, Faecalibaterium and Ruminococcaceae exhibited a vital role of these particular microbes in exacerbating the OA. Additionally, glucosamine sulfate and chondroitin sulfate are the proteoglycan-based nutraceuticals. Theoretically, taking it is able to stimulate chondrocytes to produce new collagen and proteoglycans, thus helping body repair cartilage damaged by OA (152, 153). However, partial studies have shown that these compounds can usually reduce pain caused by OA and repair articular cartilage within a few weeks to months after starting treatment (154). Therefore, although more and more OA patients are currently using it worldwide, larger randomized controlled clinical trials are still needed to verify the safety and efficacy of glucosamine sulfate and chondroitin sulfate on OA. Furthermore, the non-steroidal anti-inflammatory drugs (NSAIDs) are commonly used as a treatment method for OA, and several previous reports also revealed the role of NSAIDs in the regulation of OA from the perspective of GM. Boer et al. (155) suggested that the administration of NSAIDs can affect the diversity and abundance of GM, and there is a certain correlation between the abundance of specific bacterial groups and the pain degree of knee OA. Vitetta et al. (156) reported that the pharmaceuticals to treat OA-related pain (such as NSAIDs) may disrupt intestinal barrier integrity and induce intestinal inflammation. In general, for patients who often need to take NSAIDs to alleviate the OA-related pain, the GM disorders characterized by abnormal proliferation of Gram-negative bacteria can induce abnormal intestinal mucosal immune response, which can cause intestinal inflammation and intestinal injury, while probiotics and rifaximin can significantly alleviate intestinal injury induced by NSAIDs (157, 158).
To sum up, with the in-depth researches of the pathogenesis of OA, more and more evidence indicates that there is a close relationship between OA and GM. Although the specific mechanisms of the relationship between intestinal microecology and OA is still unclear and under intensive exploration, it is foreseeable that the characteristics of GM and its metabolites can be applied to diagnose and evaluate the OA or other bone-related diseases in the future. The functional relationship and mechanisms between GM and its metabolites and OA are summarized in Figure 1. Meanwhile, improving the intestinal microecology can also be developed as a potential therapeutic approach to prevent and intervene the development process of OA. Nevertheless, in the process of improving the understanding of GM and bone-related diseases represented by OA, there are still partial unavoidable challenges need to be emphasized, including (1) due to the relatively slow rate of bone alterations, it is difficult to experimentally observe the continuous alterations of GM through the current experiments. Furthermore, among the existing research methods, a common method of utilizing the GM is to transfer the microbiota from the donor to GF animals. When exposed to a new host environment, the structure of transferred microbiota might also alter over time; (2) the preclinical studies related to OA have focused on adult skeletal phenotypes, which require the application of older animals. The adult phenotype may be sensitive to the time of microbial exposure, so it is difficult to assess the GM changes related to mature bones; (3) there are still few methods to effectively utilize GM and its metabolites to obtain the targeted therapeutic effects, which need to be further explored. Hence, in the course of future exploration, repetitive animal studies and clinical trials should be conducted in response to the above challenges to examine the growth, quality, structure, strength and bone healing of the experimental animals and human bones in the presence of GM dysregulation, so that the GM and its metabolites might become a promising target for the prevention and treatment of bone-related diseases represented by OA.
Figure 1. The functional relationship and mechanisms between GM and its metabolites and OA. GM, gut microbiota; OA, osteoarthritis; LPS, lipopolysaccharide; TLR, toll-like receptors; SCFAs, short chain fatty acids; Tregs, regulatory T.
LL, FT, and RX developed the idea for this manuscript. LL, FT, and WX developed the search strategy and performed the review and data extraction. LL and FT wrote the manuscript. All authors edited and agreed the final version.
This work was supported by the grants from National Natural Science Foundation of China (No. 81902201) and the Fundamental Research Funds for Central Universities (No. WK9110000093).
The authors declare that the research was conducted in the absence of any commercial or financial relationships that could be construed as a potential conflict of interest.
All claims expressed in this article are solely those of the authors and do not necessarily represent those of their affiliated organizations, or those of the publisher, the editors and the reviewers. Any product that may be evaluated in this article, or claim that may be made by its manufacturer, is not guaranteed or endorsed by the publisher.
1. Bucci J, Chen X, LaValley M, Nevitt M, Torner J, Lewis CE, et al. Progression of knee osteoarthritis with use of intraarticular glucocorticoids versus hyaluronic acid. Arthritis Rheumatol. (2022) 74:223–6. doi: 10.1002/art.42031
2. Jiang W, Liu H, Wan R, Wu Y, Shi Z, Huang W. Mechanisms linking mitochondrial mechanotransduction and chondrocyte biology in the pathogenesis of osteoarthritis. Ageing Res Rev. (2021) 67:101315. doi: 10.1016/j.arr.2021.101315
3. Petersen ET, Rytter S, Koppens D, Dalsgaard J, Hansen TB, Larsen NE, et al. Patients with knee osteoarthritis can be divided into subgroups based on tibiofemoral joint kinematics of gait – an exploratory and dynamic radiostereometric study. Osteoarthritis Cartilage. (2022) 30:249–59. doi: 10.1016/j.joca.2021.10.011
4. Prieto-Alhambra D, Judge A, Javaid MK, Cooper C, Diez-Perez A, Arden NK. Incidence and risk factors for clinically diagnosed knee, hip and hand osteoarthritis: influences of age, gender and osteoarthritis affecting other joints. Ann Rheum Dis. (2014) 73:1659–64. doi: 10.1136/annrheumdis-2013-203355
5. Abbate LM, Jeffreys AS, Coffman CJ, Schwartz TA, Arbeeva L, Callahan LF, et al. Demographic and clinical factors associated with non-surgical osteoarthritis treatment among patients in outpatient clinics. Arthritis Care Res. (2018) 70:1141–9. doi: 10.1002/acr.23466
6. Paterson KL, Hinman RS, Metcalf BR, Campbell PK, Menz HB, Hunter DJ, et al. Podiatry intervention versus usual general practitioner care for symptomatic radiographic osteoarthritis of the first metatarsophalangeal joint: a randomized clinical feasibility study. Arthritis Care Res. (2021) 73:250–8. doi: 10.1002/acr.24107
7. Conaghan PG, Cook AD, Hamilton JA, Tak PP. Therapeutic options for targeting inflammatory osteoarthritis pain. Nat Rev Rheumatol. (2019) 15:355–63. doi: 10.1038/s41584-019-0221-y
8. Eriksen EF, Shabestari M, Ghouri A, Conaghan PG. Bisphosphonates as a treatment modality in osteoarthritis. Bone. (2021) 143:115352. doi: 10.1016/j.bone.2020.115352
9. Yan Y, Yi X, Duan Y, Jiang B, Huang T, Inglis BM, et al. Alteration of the gut microbiota in rhesus monkey with spontaneous osteoarthritis. BMC Microbiol. (2021) 21:328. doi: 10.1186/s12866-021-02390-0
10. Han H, Yi B, Zhong R, Wang M, Zhang S, Ma J, et al. From gut microbiota to host appetite: gut microbiota-derived metabolites as key regulators. Microbiome. (2021) 9:162. doi: 10.1186/s40168-021-01093-y
11. Yang G, Wei J, Liu P, Zhang Q, Tian Y, Hou G, et al. Role of the gut microbiota in type 2 diabetes and related diseases. Metabolism. (2021) 117:154712. doi: 10.1016/j.metabol.2021.154712
12. Zhang YW, Li YJ, Lu PP, Dai GC, Chen XX, Rui YF. The modulatory effect and implication of gut microbiota on osteoporosis: from the perspective of “brain-gut-bone” axis. Food Funct. (2021) 12:5703–18. doi: 10.1039/d0fo03468a
13. Gershon MD, Margolis KG. The gut, its microbiome, and the brain: connections and communications. J Clin Invest. (2021) 131:e143768. doi: 10.1172/jci143768
14. Jing Y, Yu Y, Bai F, Wang L, Yang D, Zhang C, et al. Effect of fecal microbiota transplantation on neurological restoration in a spinal cord injury mouse model: involvement of brain-gut axis. Microbiome. (2021) 9:59. doi: 10.1186/s40168-021-01007-y
15. Wu Y, Wan J, Choe U, Pham Q, Schoene NW, He Q, et al. Interactions between food and gut microbiota: impact on human health. Annu Rev Food Sci Technol. (2019) 10:389–408. doi: 10.1146/annurev-food-032818-121303
16. Chen BD, Jia XM, Xu JY, Zhao LD, Ji JY, Wu BX, et al. An Autoimmunogenic and proinflammatory profile defined by the gut microbiota of patients with untreated systemic lupus erythematosus. Arthritis Rheumatol. (2021) 73:232–43. doi: 10.1002/art.41511
17. Choi SC, Brown J, Gong M, Ge Y, Zadeh M, Li W, et al. Gut microbiota dysbiosis and altered tryptophan catabolism contribute to autoimmunity in lupus-susceptible mice. Sci Transl Med. (2020) 12:eaax2220. doi: 10.1126/scitranslmed.aax2220
18. Hidalgo-Cantabrana C, Gómez J, Delgado S, Requena-López S, Queiro-Silva R, Margolles A, et al. Gut microbiota dysbiosis in a cohort of patients with psoriasis. Br J Dermatol. (2019) 181:1287–95. doi: 10.1111/bjd.17931
19. Johnson BM, Gaudreau MC, Gudi R, Brown R, Gilkeson G, Vasu C. Gut microbiota differently contributes to intestinal immune phenotype and systemic autoimmune progression in female and male lupus-prone mice. J Autoimmun. (2020) 108:102420. doi: 10.1016/j.jaut.2020.102420
20. Tomofuji Y, Maeda Y, Oguro-Igashira E, Kishikawa T, Yamamoto K, Sonehara K, et al. Metagenome-wide association study revealed disease-specific landscape of the gut microbiome of systemic lupus erythematosus in Japanese. Ann Rheum Dis. (2021) 80:1575–83. doi: 10.1136/annrheumdis-2021-220687
21. Zhang YW, Cao MM, Li YJ, Dai GC, Lu PP, Zhang M, et al. The regulative effect and repercussion of probiotics and prebiotics on osteoporosis: involvement of brain-gut-bone axis. Crit Rev Food Sci Nutr. (2022). [Online ahead of print]. doi: 10.1080/10408398.2022.2047005
22. Sheth T, Pitchumoni CS, Das KM. Musculoskeletal manifestations in inflammatory bowel disease: a revisit in search of immunopathophysiological mechanisms. J Clin Gastroenterol. (2014) 48:308–17. doi: 10.1097/mcg.0000000000000067
23. Schüle S, Rossel JB, Frey D, Biedermann L, Scharl M, Zeitz J, et al. Widely differing screening and treatment practice for osteoporosis in patients with inflammatory bowel diseases in the Swiss IBD cohort study. Medicine. (2017) 96:e6788. doi: 10.1097/md.0000000000006788
24. Hansen MS, Frost M. Alliances of the gut and bone axis. Semin Cell Dev Biol. (2022) 123:74–81. doi: 10.1016/j.semcdb.2021.06.024
25. Wang M, Zhou J, He F, Cai C, Wang H, Wang Y, et al. Alteration of gut microbiota-associated epitopes in children with autism spectrum disorders. Brain Behav Immun. (2019) 75:192–9. doi: 10.1016/j.bbi.2018.10.006
26. Fernández L, Langa S, Martín V, Maldonado A, Jiménez E, Martín R, et al. The human milk microbiota: origin and potential roles in health and disease. Pharmacol Res. (2013) 69:1–10. doi: 10.1016/j.phrs.2012.09.001
27. Ley D, Desseyn JL, Mischke M, Knol J, Turck D, Gottrand F. Early-life origin of intestinal inflammatory disorders. Nutr Rev. (2017) 75:175–87. doi: 10.1093/nutrit/nuw061
28. Coman V, Vodnar DC. Gut microbiota and old age: modulating factors and interventions for healthy longevity. Exp Gerontol. (2020) 141:111095. doi: 10.1016/j.exger.2020.111095
29. Conway J, Ageing ADN. of the gut microbiome: potential influences on immune senescence and inflammageing. Ageing Res Rev. (2021) 68:101323. doi: 10.1016/j.arr.2021.101323
30. Chang EB, Martinez-Guryn K. Small intestinal microbiota: the neglected stepchild needed for fat digestion and absorption. Gut Microbes. (2019) 10:235–40. doi: 10.1080/19490976.2018.1502539
31. Fouhy F, Watkins C, Hill CJ, O’Shea CA, Nagle B, Dempsey EM, et al. Perinatal factors affect the gut microbiota up to four years after birth. Nat Commun. (2019) 10:1517. doi: 10.1038/s41467-019-09252-4
32. Guido G, Ausenda G, Iascone V, Chisari E. Gut permeability and osteoarthritis, towards a mechanistic understanding of the pathogenesis: a systematic review. Ann Med. (2021) 53:2380–90. doi: 10.1080/07853890.2021.2014557
33. Zhang H, Qi L, Shen Q, Wang R, Guo Y, Zhang C, et al. Comparative analysis of the bioactive compounds in chicken cartilage: protective effects of chondroitin sulfate and type ii collagen peptides against osteoarthritis involve gut microbiota. Front Nutr. (2022) 9:843360. doi: 10.3389/fnut.2022.843360
34. Gleason B, Chisari E, Parvizi J. Osteoarthritis can also start in the gut: the gut-joint axis. Indian J Orthop. (2022) 56:1150–5. doi: 10.1007/s43465-021-00473-8
35. Dunn CM, Jeffries MA. The microbiome in osteoarthritis: a narrative review of recent human and animal model literature. Curr Rheumatol Rep. (2022) 24:139–48. doi: 10.1007/s11926-022-01066-6
36. Bonato A, Zenobi-Wong M, Barreto G, Huang Z. A systematic review of microbiome composition in osteoarthritis subjects. Osteoarthritis Cartilage. (2022) 30:786–801. doi: 10.1016/j.joca.2021.12.006
37. Musumeci G, Szychlinska MA, Mobasheri A. Age-related degeneration of articular cartilage in the pathogenesis of osteoarthritis: molecular markers of senescent chondrocytes. Histol Histopathol. (2015) 30:1–12. doi: 10.14670/hh-30.1
38. Schröder A, Nazet U, Muschter D, Grässel S, Proff P, Kirschneck C. Impact of mechanical load on the expression profile of synovial fibroblasts from patients with and without osteoarthritis. Int J Mol Sci. (2019) 20:585. doi: 10.3390/ijms20030585
39. Kolasinski SL, Neogi T, Hochberg MC, Oatis C, Guyatt G, Block J, et al. 2019 American College of Rheumatology/arthritis foundation guideline for the management of osteoarthritis of the hand, hip, and knee. Arthritis Rheumatol. (2020) 72:220–33. doi: 10.1002/art.41142
40. Reyes C, Leyland KM, Peat G, Cooper C, Arden NK, Prieto-Alhambra D. Association between overweight and obesity and risk of clinically diagnosed knee, hip, and hand osteoarthritis: a population-based cohort study. Arthritis Rheumatol. (2016) 68:1869–75. doi: 10.1002/art.39707
41. Qin J, Barbour KE, Murphy LB, Nelson AE, Schwartz TA, Helmick CG, et al. Lifetime risk of symptomatic hand osteoarthritis: the johnston county osteoarthritis project. Arthritis Rheumatol. (2017) 69:1204–12. doi: 10.1002/art.40097
42. Collins KH, Paul HA, Reimer RA, Seerattan RA, Hart DA, Herzog W. Relationship between inflammation, the gut microbiota, and metabolic osteoarthritis development: studies in a rat model. Osteoarthritis Cartilage. (2015) 23:1989–98. doi: 10.1016/j.joca.2015.03.014
43. Li Y, Luo W, Deng Z, Lei G. Diet-intestinal microbiota axis in osteoarthritis: a possible role. Mediat Inflamm. (2016) 2016:3495173. doi: 10.1155/2016/3495173
44. Avci D, Bachmann GA. Osteoarthritis and osteoporosis in postmenopausal women: clinical similarities and differences. Menopause. (2004) 11(6 Pt 1):615–21. doi: 10.1097/01.gme.0000119985.45613.7e
45. Yuan X, Chen R, Zhang Y, Lin X, Yang X. Gut microbiota: effect of pubertal status. BMC Microbiol. (2020) 20:334. doi: 10.1186/s12866-020-02021-0
46. Ridlon JM, Ikegawa S, Alves JM, Zhou B, Kobayashi A, Iida T, et al. Clostridium scindens: a human gut microbe with a high potential to convert glucocorticoids into androgens. J Lipid Res. (2013) 54:2437–49. doi: 10.1194/jlr.M038869
47. Yoon K, Kim N. Roles of sex hormones and gender in the gut microbiota. J Neurogastroenterol Motil. (2021) 27:314–25. doi: 10.5056/jnm20208
48. Ji BW, Sheth RU, Dixit PD, Tchourine K, Vitkup D. Macroecological dynamics of gut microbiota. Nat Microbiol. (2020) 5:768–75. doi: 10.1038/s41564-020-0685-1
49. Wei J, Zhang C, Zhang Y, Zhang W, Doherty M, Yang T, et al. Association between gut microbiota and symptomatic hand osteoarthritis: data from the xiangya osteoarthritis study. Arthritis Rheumatol. (2021) 73:1656–62. doi: 10.1002/art.41729
50. Rushing BR, McRitchie S, Arbeeva L, Nelson AE, Azcarate-Peril MA, Li YY, et al. Fecal metabolomics reveals products of dysregulated proteolysis and altered microbial metabolism in obesity-related osteoarthritis. Osteoarthritis Cartilage. (2022) 30:81–91. doi: 10.1016/j.joca.2021.10.006
51. Chen J, Wang A, Wang Q. Dysbiosis of the gut microbiome is a risk factor for osteoarthritis in older female adults: a case control study. BMC Bioinformatics. (2021) 22:299. doi: 10.1186/s12859-021-04199-0
52. Wang Z, Zhu H, Jiang Q, Zhu YZ. The gut microbiome as non-invasive biomarkers for identifying overweight people at risk for osteoarthritis. Microb Pathog. (2021) 157:104976. doi: 10.1016/j.micpath.2021.104976
53. Lee JY, Mannaa M, Kim Y, Kim J, Kim GT, Seo YS. Comparative analysis of fecal microbiota composition between rheumatoid arthritis and osteoarthritis patients. Genes. (2019) 10:748. doi: 10.3390/genes10100748
54. Wang X, Ning Y, Li C, Gong Y, Huang R, Hu M, et al. Alterations in the gut microbiota and metabolite profiles of patients with Kashin-Beck disease, an endemic osteoarthritis in China. Cell Death Dis. (2021) 12:1015. doi: 10.1038/s41419-021-04322-2
55. Ramasamy B, Magne F, Tripathy SK, Venugopal G, Mukherjee D, Balamurugan R. Association of gut microbiome and vitamin d deficiency in knee osteoarthritis patients: a pilot study. Nutrients. (2021) 13:1272. doi: 10.3390/nu13041272
56. Ning Y, Hu M, Gong Y, Huang R, Xu K, Chen S, et al. Comparative analysis of the gut microbiota composition between knee osteoarthritis and Kashin-Beck disease in Northwest China. Arthritis Res Ther. (2022) 24:129. doi: 10.1186/s13075-022-02819-5
57. Loeser RF, Arbeeva L, Kelley K, Fodor AA, Sun S, Ulici V, et al. Association of increased serum lipopolysaccharide, but not microbial dysbiosis, with obesity-related osteoarthritis. Arthritis Rheumatol. (2022) 74:227–36. doi: 10.1002/art.41955
58. Guan Z, Jia J, Zhang C, Sun T, Zhang W, Yuan W, et al. Gut microbiome dysbiosis alleviates the progression of osteoarthritis in mice. Clin Sci. (2020) 134:3159–74. doi: 10.1042/cs20201224
59. Ulici V, Kelley KL, Azcarate-Peril MA, Cleveland RJ, Sartor RB, Schwartz TA, et al. Osteoarthritis induced by destabilization of the medial meniscus is reduced in germ-free mice. Osteoarthritis Cartilage. (2018) 26:1098–109. doi: 10.1016/j.joca.2018.05.016
60. Hahn AK, Wallace CW, Welhaven HD, Brooks E, McAlpine M, Christiansen BA, et al. The microbiome mediates epiphyseal bone loss and metabolomic changes after acute joint trauma in mice. Osteoarthritis Cartilage. (2021) 29:882–93. doi: 10.1016/j.joca.2021.01.012
61. Jia YJ, Li TY, Han P, Chen Y, Pan LJ, Jia CS. Effects of different courses of moxibustion treatment on intestinal flora and inflammation of a rat model of knee osteoarthritis. J Integr Med. (2022) 20:173–81. doi: 10.1016/j.joim.2022.01.004
62. Li K, Liu A, Zong W, Dai L, Liu Y, Luo R, et al. Moderate exercise ameliorates osteoarthritis by reducing lipopolysaccharides from gut microbiota in mice. Saudi J Biol Sci. (2021) 28:40–9. doi: 10.1016/j.sjbs.2020.08.027
63. Mendez ME, Murugesh DK, Sebastian A, Hum NR, McCloy SA, Kuhn EA, et al. Antibiotic treatment prior to injury improves post-traumatic osteoarthritis outcomes in mice. Int J Mol Sci. (2020) 21:6424. doi: 10.3390/ijms21176424
64. Rios JL, Bomhof MR, Reimer RA, Hart DA, Collins KH, Herzog W. Protective effect of prebiotic and exercise intervention on knee health in a rat model of diet-induced obesity. Sci Rep. (2019) 9:3893. doi: 10.1038/s41598-019-40601-x
65. Groschwitz KR, Hogan SP. Intestinal barrier function: molecular regulation and disease pathogenesis. J Allergy Clin Immunol. (2009) 124:3–20. doi: 10.1016/j.jaci.2009.05.038
66. Otani T, Furuse M. Tight junction structure and function revisited. Trends Cell Biol. (2020) 30:805–17. doi: 10.1016/j.tcb.2020.08.004
67. Celebi Sözener Z, Cevhertas L, Nadeau K, Akdis M, Akdis CA. Environmental factors in epithelial barrier dysfunction. J Allergy Clin Immunol. (2020) 145:1517–28. doi: 10.1016/j.jaci.2020.04.024
68. Doron I, Mesko M, Li XV, Kusakabe T, Leonardi I, Shaw DG, et al. Mycobiota-induced IgA antibodies regulate fungal commensalism in the gut and are dysregulated in Crohn’s disease. Nat Microbiol. (2021) 6:1493–504. doi: 10.1038/s41564-021-00983-z
69. Varela SAM, Matos M, Schlupp I. The role of mate-choice copying in speciation and hybridization. Biol Rev Camb Philos Soc. (2018) 93:1304–22. doi: 10.1111/brv.12397
70. Hernandez CJ, Guss JD, Luna M, Goldring SR. Links between the microbiome and bone. J Bone Miner Res. (2016) 31:1638–46. doi: 10.1002/jbmr.2887
71. Fine RL, Manfredo Vieira S, Gilmore MS, Kriegel MA. Mechanisms and consequences of gut commensal translocation in chronic diseases. Gut Microbes. (2020) 11:217–30. doi: 10.1080/19490976.2019.1629236
72. Brennan CA, Clay SL, Lavoie SL, Bae S, Lang JK, Fonseca-Pereira D, et al. Fusobacterium nucleatum drives a pro-inflammatory intestinal microenvironment through metabolite receptor-dependent modulation of IL-17 expression. Gut Microbes. (2021) 13:1987780. doi: 10.1080/19490976.2021.1987780
73. Buckley A, Turner JR. Cell Biology of Tight Junction Barrier Regulation and Mucosal Disease. Cold Spring Harb Perspect Biol. (2018) 10:a029314. doi: 10.1101/cshperspect.a029314
74. Koh A, De Vadder F, Kovatcheva-Datchary P, Bäckhed F. From dietary fiber to host physiology: short-chain fatty acids as key bacterial metabolites. Cell. (2016) 165:1332–45. doi: 10.1016/j.cell.2016.05.041
75. Lucas S, Omata Y, Hofmann J, Böttcher M, Iljazovic A, Sarter K, et al. Short-chain fatty acids regulate systemic bone mass and protect from pathological bone loss. Nat Commun. (2018) 9:55. doi: 10.1038/s41467-017-02490-4
76. Yan J, Herzog JW, Tsang K, Brennan CA, Bower MA, Garrett WS, et al. Gut microbiota induce IGF-1 and promote bone formation and growth. Proc Natl Acad Sci U S A. (2016) 113:E7554–63. doi: 10.1073/pnas.1607235113
77. Rohr MW, Narasimhulu CA, Rudeski-Rohr TA, Parthasarathy S. Negative effects of a high-fat diet on intestinal permeability: a review. Adv Nutr. (2020) 11:77–91. doi: 10.1093/advances/nmz061
78. Griffin TM, Batushansky A, Hudson J, Lopes EBP. Correlation network analysis shows divergent effects of a long-term, high-fat diet and exercise on early stage osteoarthritis phenotypes in mice. J Sport Health Sci. (2020) 9:119–31. doi: 10.1016/j.jshs.2019.05.008
79. Wang J, Xu W, Wang R, Cheng R, Tang Z, Zhang M. The outer membrane protein Amuc_1100 of Akkermansia muciniphila promotes intestinal 5-HT biosynthesis and extracellular availability through TLR2 signalling. Food Funct. (2021) 12:3597–610. doi: 10.1039/d1fo00115a
80. Zhao CN, Wang P, Mao YM, Dan YL, Wu Q, Li XM, et al. Potential role of melatonin in autoimmune diseases. Cytokine Growth Factor Rev. (2019) 48:1–10. doi: 10.1016/j.cytogfr.2019.07.002
81. Lavoie B, Lian JB, Mawe GM. Regulation of bone metabolism by serotonin. Adv Exp Med Biol. (2017) 1033:35–46. doi: 10.1007/978-3-319-66653-2_3
82. Agus A, Planchais J, Sokol H. Gut microbiota regulation of tryptophan metabolism in health and disease. Cell Host Microbe. (2018) 23:716–24. doi: 10.1016/j.chom.2018.05.003
83. Gao K, Mu CL, Farzi A, Zhu WY. Tryptophan metabolism: a link between the gut microbiota and brain. Adv Nutr. (2020) 11:709–23. doi: 10.1093/advances/nmz127
84. Bolte LA, Vich Vila A, Imhann F, Collij V, Gacesa R, Peters V, et al. Long-term dietary patterns are associated with pro-inflammatory and anti-inflammatory features of the gut microbiome. Gut. (2021) 70:1287–98. doi: 10.1136/gutjnl-2020-322670
85. Kim SJ, Chen Z, Chamberlain ND, Essani AB, Volin MV, Amin MA, et al. Ligation of TLR5 promotes myeloid cell infiltration and differentiation into mature osteoclasts in rheumatoid arthritis and experimental arthritis. J Immunol. (2014) 193:3902–13. doi: 10.4049/jimmunol.1302998
86. Ma X, Claus LAN, Leslie ME, Tao K, Wu Z, Liu J, et al. Ligand-induced monoubiquitination of BIK1 regulates plant immunity. Nature. (2020) 581:199–203. doi: 10.1038/s41586-020-2210-3
87. Kurashima Y, Goto Y, Kiyono H. Mucosal innate immune cells regulate both gut homeostasis and intestinal inflammation. Eur J Immunol. (2013) 43:3108–15. doi: 10.1002/eji.201343782
88. Desai MS, Seekatz AM, Koropatkin NM, Kamada N, Hickey CA, Wolter M, et al. A dietary fiber-deprived gut microbiota degrades the colonic mucus barrier and enhances pathogen susceptibility. Cell. (2016) 167:1339–1353.e21. doi: 10.1016/j.cell.2016.10.043
89. Ren Z, Pan LL, Huang Y, Chen H, Liu Y, Liu H, et al. Gut microbiota-CRAMP axis shapes intestinal barrier function and immune responses in dietary gluten-induced enteropathy. EMBO Mol Med. (2021) 13:e14059. doi: 10.15252/emmm.202114059
90. Shi N, Li N, Duan X, Niu H. Interaction between the gut microbiome and mucosal immune system. Mil Med Res. (2017) 4:14. doi: 10.1186/s40779-017-0122-9
91. Allaire JM, Crowley SM, Law HT, Chang SY, Ko HJ, Vallance BA. The intestinal epithelium: central coordinator of mucosal immunity. Trends Immunol. (2018) 39:677–96. doi: 10.1016/j.it.2018.04.002
92. Yin C, Zhao Q, Li W, Zhao Z, Wang J, Deng T, et al. Biomimetic anti-inflammatory nano-capsule serves as a cytokine blocker and M2 polarization inducer for bone tissue repair. Acta Biomater. (2020) 102:416–26. doi: 10.1016/j.actbio.2019.11.025
93. Alves CH, Farrell E, Vis M, Colin EM, Lubberts E. Animal models of bone loss in inflammatory arthritis: from cytokines in the bench to novel treatments for bone loss in the bedside-a comprehensive review. Clin Rev Allergy Immunol. (2016) 51:27–47. doi: 10.1007/s12016-015-8522-7
94. Perniss A, Schmidt P, Soultanova A, Papadakis T, Dahlke K, Voigt A, et al. Development of epithelial cholinergic chemosensory cells of the urethra and trachea of mice. Cell Tissue Res. (2021) 385:21–35. doi: 10.1007/s00441-021-03424-9
95. Maldonado Galdeano C, Ca.zorla SI, Lemme Dumit JM, Vélez E, Perdigón G. Beneficial effects of probiotic consumption on the immune system. Ann Nutr Metab. (2019) 74:115–24. doi: 10.1159/000496426
96. Sodhi C, Levy R, Gill R, Neal MD, Richardson W, Branca M, et al. DNA attenuates enterocyte Toll-like receptor 4-mediated intestinal mucosal injury after remote trauma. Am J Physiol Gastrointest Liver Physiol. (2011) 300:G862–73. doi: 10.1152/ajpgi.00373.2010
97. Pang XX, Ansari AR, Yang WJ, Niu XY, Dong L, Li HZ, et al. Visfatin regulates inflammatory mediators in mouse intestinal mucosa through toll-like receptors signaling under lipopolysaccharide stress. Arch Immunol Ther Exp. (2021) 69:11. doi: 10.1007/s00005-021-00611-y
98. Lambert C, Zappia J, Sanchez C, Florin A, Dubuc JE, Henrotin Y. The Damage-Associated Molecular Patterns (DAMPs) as potential targets to treat osteoarthritis: perspectives from a review of the literature. Front Med. (2020) 7:607186. doi: 10.3389/fmed.2020.607186
99. Mahon OR, Kelly DJ, McCarthy GM, Dunne A. Osteoarthritis-associated basic calcium phosphate crystals alter immune cell metabolism and promote M1 macrophage polarization. Osteoarthritis Cartilage. (2020) 28:603–12. doi: 10.1016/j.joca.2019.10.010
100. Liébana-García R, Olivares M, Bullich-Vilarrubias C, López-Almela I, Romaní-Pérez M, Sanz Y. The gut microbiota as a versatile immunomodulator in obesity and associated metabolic disorders. Best Pract Res Clin Endocrinol Metab. (2021) 35:101542. doi: 10.1016/j.beem.2021.101542
101. Chen Y, Wang X, Zhang C, Liu Z, Li C, Ren Z. Gut microbiota and bone diseases: a growing partnership. Front Microbiol. (2022) 13:877776. doi: 10.3389/fmicb.2022.877776
102. Pabst O, Slack E. IgA and the intestinal microbiota: the importance of being specific. Mucosal Immunol. (2020) 13:12–21. doi: 10.1038/s41385-019-0227-4
103. Huus KE, Petersen C, Finlay BB. Diversity and dynamism of IgA-microbiota interactions. Nat Rev Immunol. (2021) 21:514–25. doi: 10.1038/s41577-021-00506-1
104. Jetten AM, Cook DN. (Inverse) agonists of retinoic acid-related orphan receptor γ: regulation of immune responses, inflammation, and autoimmune disease. Annu Rev Pharmacol Toxicol. (2020) 60:371–90. doi: 10.1146/annurev-pharmtox-010919-023711
105. Ang QY, Alexander M, Newman JC, Tian Y, Cai J, Upadhyay V, et al. Ketogenic diets alter the gut microbiome resulting in decreased intestinal th17 cells. Cell. (2020) 181:1263–1275.e16. doi: 10.1016/j.cell.2020.04.027
106. Ivanov II, Atarashi K, Manel N, Brodie EL, Shima T, Karaoz U, et al. Induction of intestinal Th17 cells by segmented filamentous bacteria. Cell. (2009) 139:485–98. doi: 10.1016/j.cell.2009.09.033
107. Atarashi K, Tanoue T, Ando M, Kamada N, Nagano Y, Narushima S, et al. Th17 cell induction by adhesion of microbes to intestinal epithelial cells. Cell. (2015) 163:367–80. doi: 10.1016/j.cell.2015.08.058
108. du Teil Espina M, Gabarrini G, Harmsen HJM, Westra J, van Winkelhoff AJ, van Dijl JM. Talk to your gut: the oral-gut microbiome axis and its immunomodulatory role in the etiology of rheumatoid arthritis. FEMS Microbiol Rev. (2019) 43:1–18. doi: 10.1093/femsre/fuy035
109. Hamamoto Y, Ouhara K, Munenaga S, Shoji M, Ozawa T, Hisatsune J, et al. Effect of Porphyromonas gingivalis infection on gut dysbiosis and resultant arthritis exacerbation in mouse model. Arthritis Res Ther. (2020) 22:249. doi: 10.1186/s13075-020-02348-z
110. Sato K, Takahashi N, Kato T, Matsuda Y, Yokoji M, Yamada M, et al. Aggravation of collagen-induced arthritis by orally administered Porphyromonas gingivalis through modulation of the gut microbiota and gut immune system. Sci Rep. (2017) 7:6955. doi: 10.1038/s41598-017-07196-7
111. Stewart L, Edgar JDM, Blakely G, Patrick S. Antigenic mimicry of ubiquitin by the gut bacterium Bacteroides fragilis: a potential link with autoimmune disease. Clin Exp Immunol. (2018) 194:153–65. doi: 10.1111/cei.13195
112. Rida MA, Chandran V. Challenges in the clinical diagnosis of psoriatic arthritis. Clin Immunol. (2020) 214:108390. doi: 10.1016/j.clim.2020.108390
113. Staffas A, Burgos da Silva M, van den Brink MR. The intestinal microbiota in allogeneic hematopoietic cell transplant and graft-versus-host disease. Blood. (2017) 129:927–33. doi: 10.1182/blood-2016-09-691394
114. Weingarden AR, Vaughn BP. Intestinal microbiota, fecal microbiota transplantation, and inflammatory bowel disease. Gut Microbes. (2017) 8:238–52. doi: 10.1080/19490976.2017.1290757
115. Lozupone CA, Stombaugh JI, Gordon JI, Jansson JK, Knight R. Diversity, stability and resilience of the human gut microbiota. Nature. (2012) 489:220–30. doi: 10.1038/nature11550
116. Zhu W, Gregory JC, Org E, Buffa JA, Gupta N, Wang Z, et al. Gut microbial metabolite TMAO enhances platelet hyperreactivity and thrombosis risk. Cell. (2016) 165:111–24. doi: 10.1016/j.cell.2016.02.011
117. Yoo W, Zieba JK, Foegeding NJ, Torres TP, Shelton CD, Shealy NG, et al. High-fat diet-induced colonocyte dysfunction escalates microbiota-derived trimethylamine N-oxide. Science. (2021) 373:813–8. doi: 10.1126/science.aba3683
118. Requena T, Martínez-Cuesta MC, Peláez C. Diet and microbiota linked in health and disease. Food Funct. (2018) 9:688–704. doi: 10.1039/c7fo01820g
119. De Filippis F, Pellegrini N, Vannini L, Jeffery IB, La Storia A, Laghi L, et al. High-level adherence to a Mediterranean diet beneficially impacts the gut microbiota and associated metabolome. Gut. (2016) 65:1812–21. doi: 10.1136/gutjnl-2015-309957
120. McCabe L, Britton RA, Parameswaran N. Prebiotic and probiotic regulation of bone health: role of the intestine and its microbiome. Curr Osteoporos Rep. (2015) 13:363–71. doi: 10.1007/s11914-015-0292-x
121. Messier SP, Mihalko SL, Legault C, Miller GD, Nicklas BJ, DeVita P, et al. Effects of intensive diet and exercise on knee joint loads, inflammation, and clinical outcomes among overweight and obese adults with knee osteoarthritis: the IDEA randomized clinical trial. JAMA. (2013) 310:1263–73. doi: 10.1001/jama.2013.277669
122. Morales-Ivorra I, Romera-Baures M, Roman-Viñas B, Serra-Majem L. Osteoarthritis and the mediterranean diet: a systematic review. Nutrients. (2018) 10:1030. doi: 10.3390/nu10081030
123. Thomas S, Browne H, Mobasheri A, Rayman MP. What is the evidence for a role for diet and nutrition in osteoarthritis? Rheumatology. (2018) 57(Suppl_4):461–74. doi: 10.1093/rheumatology/key011
124. Chen L, Zheng JJY, Li G, Yuan J, Ebert JR, Li H, et al. Pathogenesis and clinical management of obesity-related knee osteoarthritis: impact of mechanical loading. J Orthop Translat. (2020) 24:66–75. doi: 10.1016/j.jot.2020.05.001
125. Hagi T, Belzer C. The interaction of Akkermansia muciniphila with host-derived substances, bacteria and diets. Appl Microbiol Biotechnol. (2021) 105:4833–41. doi: 10.1007/s00253-021-11362-3
126. Remely M, Hippe B, Zanner J, Aumueller E, Brath H, Haslberger AG. Gut microbiota of obese, type 2 diabetic individuals is enriched in Faecalibacterium prausnitzii, Akkermansia muciniphila and Peptostreptococcus anaerobius after weight loss. Endocr Metab Immune Disord Drug Targets. (2016) 16:99–106. doi: 10.2174/1871530316666160831093813
127. Nguyen TD, Kang JH, Lee MS. Characterization of Lactobacillus plantarum PH04, a potential probiotic bacterium with cholesterol-lowering effects. Int J Food Microbiol. (2007) 113:358–61. doi: 10.1016/j.ijfoodmicro.2006.08.015
128. Li D, Liu Y, Yang X, Kong L. The role of probiotics and prebiotics in osteolastogenesis and immune relevance. Curr Med Chem. (2021) 28:5228–47. doi: 10.2174/0929867328666210316115126
129. Dimidi E, Christodoulides S, Scott SM, Whelan K. Mechanisms of action of probiotics and the gastrointestinal microbiota on gut motility and constipation. Adv Nutr. (2017) 8:484–94. doi: 10.3945/an.116.014407
130. da Silva TF, Casarotti SN, de Oliveira GLV, Penna ALB. The impact of probiotics, prebiotics, and synbiotics on the biochemical, clinical, and immunological markers, as well as on the gut microbiota of obese hosts. Crit Rev Food Sci Nutr. (2021) 61:337–55. doi: 10.1080/10408398.2020.1733483
131. Sanders ME, Merenstein DJ, Reid G, Gibson GR, Rastall RA. Probiotics and prebiotics in intestinal health and disease: from biology to the clinic. Nat Rev Gastroenterol Hepatol. (2019) 16:605–16. doi: 10.1038/s41575-019-0173-3
132. Tyagi AM, Yu M, Darby TM, Vaccaro C, Li JY, Owens JA, et al. The microbial metabolite butyrate stimulates bone formation via T regulatory cell-mediated regulation of WNT10B expression. Immunity. (2018) 49:1116–1131.e7. doi: 10.1016/j.immuni.2018.10.013
133. Pan H, Guo R, Ju Y, Wang Q, Zhu J, Xie Y, et al. A single bacterium restores the microbiome dysbiosis to protect bones from destruction in a rat model of rheumatoid arthritis. Microbiome. (2019) 7:107. doi: 10.1186/s40168-019-0719-1
134. Henrotin Y, Patrier S, Pralus A, Roche M, Nivoliez A. Protective actions of oral administration of Bifidobacterium longum CBi0703 in spontaneous osteoarthritis in dunkin hartley guinea pig model. Cartilage. (2021) 13(2_suppl):1204s–13s. doi: 10.1177/1947603519841674
135. Sim BY, Choi HJ, Kim MG, Jeong DG, Lee DG, Yoon JM, et al. Effects of ID-CBT5101 in preventing and alleviating osteoarthritis symptoms in a monosodium iodoacetate-induced rat model. J Microbiol Biotechnol. (2018) 28:1199–208. doi: 10.4014/jmb.1803.03032
136. Taye I, Bradbury J, Grace S, Avila C. Probiotics for pain of osteoarthritis; an N-of-1 trial of individual effects. Complement Ther Med. (2020) 54:102548. doi: 10.1016/j.ctim.2020.102548
137. Lyu JL, Wang TM, Chen YH, Chang ST, Wu MS, Lin YH, et al. Oral intake of Streptococcus thermophil us improves knee osteoarthritis degeneration: a randomized, double-blind, placebo-controlled clinical study. Heliyon. (2020) 6:e03757. doi: 10.1016/j.heliyon.2020.e03757
138. Quigley EMM. Prebiotics and probiotics in digestive health. Clin Gastroenterol Hepatol. (2019) 17:333–44. doi: 10.1016/j.cgh.2018.09.028
139. Vandeputte D, Falony G, Vieira-Silva S, Wang J, Sailer M, Theis S, et al. Prebiotic inulin-type fructans induce specific changes in the human gut microbiota. Gut. (2017) 66:1968–74. doi: 10.1136/gutjnl-2016-313271
140. Wang H, Geier MS, Howarth GS. Prebiotics: a potential treatment strategy for the chemotherapy-damaged Gut? Crit Rev Food Sci Nutr. (2016) 56:946–56. doi: 10.1080/10408398.2012.741082
141. Schott EM, Farnsworth CW, Grier A, Lillis JA, Soniwala S, Dadourian GH, et al. Targeting the gut microbiome to treat the osteoarthritis of obesity. JCI Insight. (2018) 3:e95997. doi: 10.1172/jci.insight.95997
142. Tanabe K, Nakamura S, Moriyama-Hashiguchi M, Kitajima M, Ejima H, Imori C, et al. Dietary fructooligosaccharide and glucomannan alter gut microbiota and improve bone metabolism in senescence-accelerated mouse. J Agric Food Chem. (2019) 67:867–74. doi: 10.1021/acs.jafc.8b05164
143. Sun J, Liu S, Ling Z, Wang F, Ling Y, Gong T, et al. Fructooligosaccharides ameliorating cognitive deficits and neurodegeneration in APP/PS1 transgenic mice through modulating gut microbiota. J Agric Food Chem. (2019) 67:3006–17. doi: 10.1021/acs.jafc.8b07313
144. Wang Z, Tauzin AS, Laville E, Tedesco P, Létisse F, Terrapon N, et al. Harvesting of prebiotic fructooligosaccharides by non-beneficial human gut bacteria. mSphere. (2020) 5:e00771-19. doi: 10.1128/mSphere.00771-19
145. Wu RY, Määttänen P, Napper S, Scruten E, Li B, Koike Y, et al. Non-digestible oligosaccharides directly regulate host kinome to modulate host inflammatory responses without alterations in the gut microbiota. Microbiome. (2017) 5:135. doi: 10.1186/s40168-017-0357-4
146. Mhd Omar NA, Frank J, Kruger J, Dal Bello F, Medana C, Collino M, et al. Effects of high intakes of fructose and galactose, with or without added fructooligosaccharides, on metabolic factors, inflammation, and gut integrity in a rat model. Mol Nutr Food Res. (2021) 65:e2001133. doi: 10.1002/mnfr.202001133
147. Costa GT, Vasconcelos Q, Aragão GF. Fructooligosaccharides on inflammation, immunomodulation, oxidative stress, and gut immune response: a systematic review. Nutr Rev. (2022) 80:709–22. doi: 10.1093/nutrit/nuab115
148. Wang N, Ma S, Fu L. Gut microbiota dysbiosis as one cause of osteoporosis by impairing intestinal barrier function. Calcif Tissue Int. (2022) 110:225–35. doi: 10.1007/s00223-021-00911-7
149. Ma S, Wang N, Zhang P, Wu W, Fu L. Fecal microbiota transplantation mitigates bone loss by improving gut microbiome composition and gut barrier function in aged rats. PeerJ. (2021) 9:e12293. doi: 10.7717/peerj.12293
150. Zhao Z, Ning J, Bao XQ, Shang M, Ma J, Li G, et al. Fecal microbiota transplantation protects rotenone-induced Parkinson’s disease mice via suppressing inflammation mediated by the lipopolysaccharide-TLR4 signaling pathway through the microbiota-gut-brain axis. Microbiome. (2021) 9:226. doi: 10.1186/s40168-021-01107-9
151. Huang Z, Chen J, Li B, Zeng B, Chou CH, Zheng X, et al. Faecal microbiota transplantation from metabolically compromised human donors accelerates osteoarthritis in mice. Ann Rheum Dis. (2020) 79:646–56. doi: 10.1136/annrheumdis-2019-216471
152. Arden NK, Perry TA, Bannuru RR, Bruyère O, Cooper C, Haugen IK, et al. Non-surgical management of knee osteoarthritis: comparison of ESCEO and OARSI 2019 guidelines. Nat Rev Rheumatol. (2021) 17:59–66. doi: 10.1038/s41584-020-00523-9
153. Simental-Mendía M, ánchez-García AS, Vilchez-Cavazos F, Acosta-Olivo CA, Peña-Martínez VM, Simental-Mendía LE. Effect of glucosamine and chondroitin sulfate in symptomatic knee osteoarthritis: a systematic review and meta-analysis of randomized placebo-controlled trials. Rheumatol Int. (2018) 38:1413–28. doi: 10.1007/s00296-018-4077-2
154. Clegg DO, Reda DJ, Harris CL, Klein MA, O’Dell JR, Hooper MM, et al. Glucosamine, chondroitin sulfate, and the two in combination for painful knee osteoarthritis. N Engl J Med. (2006) 354:795–808. doi: 10.1056/NEJMoa052771
155. Boer CG, Radjabzadeh D, Medina-Gomez C, Garmaeva S, Schiphof D, Arp P, et al. Intestinal microbiome composition and its relation to joint pain and inflammation. Nat Commun. (2019) 10:4881. doi: 10.1038/s41467-019-12873-4
156. Vitetta L, Coulson S, Linnane AW, Butt H. The gastrointestinal microbiome and musculoskeletal diseases: a beneficial role for probiotics and prebiotics. Pathogens. (2013) 2:606–26. doi: 10.3390/pathogens2040606
157. Scarpignato C, Hunt RH. Non-steroidal antiinflammatory drug-related injury to the gastrointestinal tract: clinical picture, pathogenesis, and prevention. Gastroenterol Clin North Am. (2010) 39:433–64. doi: 10.1016/j.gtc.2010.08.010
Keywords: osteoarthritis, gut microbiota, metabolites, gut-bone axis, bone
Citation: Liu L, Tian F, Li G-Y, Xu W and Xia R (2022) The effects and significance of gut microbiota and its metabolites on the regulation of osteoarthritis: Close coordination of gut-bone axis. Front. Nutr. 9:1012087. doi: 10.3389/fnut.2022.1012087
Received: 05 August 2022; Accepted: 07 September 2022;
Published: 20 September 2022.
Edited by:
Pradyumna Kumar Mishra, ICMR-National Institute for Research in Environmental Health, IndiaReviewed by:
Vibhor Joshi, ICMR-National Institute for Research in Environmental Health, IndiaCopyright © 2022 Liu, Tian, Li, Xu and Xia. This is an open-access article distributed under the terms of the Creative Commons Attribution License (CC BY). The use, distribution or reproduction in other forums is permitted, provided the original author(s) and the copyright owner(s) are credited and that the original publication in this journal is cited, in accordance with accepted academic practice. No use, distribution or reproduction is permitted which does not comply with these terms.
*Correspondence: Rui Xia, c2x4cjg5MDlAMTYzLmNvbQ==; Wei Xu, eHcxOTk4MDdAMTYzLmNvbQ==
†These authors have contributed equally to this work
Disclaimer: All claims expressed in this article are solely those of the authors and do not necessarily represent those of their affiliated organizations, or those of the publisher, the editors and the reviewers. Any product that may be evaluated in this article or claim that may be made by its manufacturer is not guaranteed or endorsed by the publisher.
Research integrity at Frontiers
Learn more about the work of our research integrity team to safeguard the quality of each article we publish.