- 1Department of Psychiatry, Jining Medical University, Jining, Shandong, China
- 2Department of Psychiatry, Shandong Daizhuang Hospital, Jining, Shandong, China
- 3Department of Sleep Medicine, Shandong Daizhuang Hospital, Jining, Shandong, China
Objectives: Fatty acids (FA) are widely believed to play a role in the pathophysiology of depression. However, the causal relationships between FA and depression remain elusive and warrant further research. We aimed to investigate the potential causal relationship between FA [saturated fatty acids (SFA), mono-unsaturated fatty acids (MUFA), and polyunsaturated fatty acids (PUFA)] and the risk of depression using Mendelian randomization (MR) analysis.
Methods: We conducted a two-sample MR analysis using large-scale European-based genome-wide association studies (GWASs) summary data related to depression (n = 500,199 individuals) and FA [saturated fatty acids (SFA), mono-unsaturated fatty acids (MUFA), and polyunsaturated fatty acids (PUFA)] levels. MR analysis was performed using the Wald ratio and inverse variance-weighted (IVW) methods, and sensitivity analysis was conducted by the simple mode, weighted mode, weighted median method, and MR-Egger method.
Results: We found the causal effects for the levels of oleic acid (OA; OR = 1.07, p = 5.72 × 10–4), adrenic acid (OR = 0.74, p = 1.01 × 10–3), α-linolenic acid (ALA; OR = 2.52, p = 1.01 × 10–3), eicosapentaenoic acid (EPA; OR = 0.84, p = 3.11 × 10–3) on depression risk, after Bonferroni correction. The sensitivity analyses indicated similar trends. No causal effect between the levels of SFA and depression risk was observed.
Conclusion: Our study suggests that adrenic acid and EPA are protective against the risk of depression, while OA and ALA are potential risk factors for depression. Nonetheless, the underlying mechanisms that mediate the association between these FAs and depression risk should be investigated in further experiments.
Introduction
Depression is a common and debilitating mental disorder that seriously affects psychosocial functioning and reduces the quality of life (1). Current evidence suggests that the disability-adjusted life-years (DALYs) due to depressive disorders have steadily increased over the past 30 years in the 10–24 and 25–49 age groups, impeding social and economic development and overwhelming the public health sector (2). Notwithstanding that the past decade has witnessed significant scientific advances, the mechanism and etiology of depression remain unclear, impeding the development of effective preventive treatment regimens and prognostic assessment (3–5). Diet represents a potentially modifiable factor that could be the focus of such preventative measures (6). The emergence of new area of research, “Nutritional Psychiatry” holds the promise of identifying which dietary components are indeed critical for mental health, including psychiatric disease. Moreover, it is essential to identify the populations, conditions, and dosages required for these nutritional interventions to exert preventative and therapeutic effects (7). The relationship between food and depression has received increasing attention (8).
Given that dietary factors may contribute to the onset of depression, a better understanding of this relationship can be harnessed to develop preventive measures for depression. Fatty acids (FA), which make up the bulk of fat in our bodies and food, are crucial for various physiological and pathological processes (9, 10). Common dietary FAs can be grouped as saturated fatty acids (SFA), mono-unsaturated fatty acids (MUFA), and polyunsaturated fatty acids (PUFA) based on the number of double bonds, type of double bonds, and chain length (11). SFAs have been linked to depressive symptoms, such as palmitic acid (PA) was positively associated with depressive symptoms, and arachidonic acid proportions showed negative association with depressive symptoms (12). MUFA fats were reported to have a negative effect on depressive symptoms (13). PUFAs, including long-chain omega-3 (mainly from fish), α-linolenic acid (ALA, a plant-based omega-3), and omega-6 (mainly from vegetable oils), participate in the synthesis, release, reuptake, and binding of neurotransmitters, as well as in the structure and function of the brain (9, 10, 12). Increased consumption of unsaturated FA, particularly PUFA, and decreased consumption of SFA have been found to lessen the risk of depression (14–17). These above findings demonstrate the importance of dietary fat content in research on the pathogenesis and treatment of depression. However, some studies have yielded inconsistent findings (18, 19). A previous randomized controlled trial (RCT) indicated an overall beneficial effect of omega-3 PUFAs on depression symptoms (20). Nevertheless, another large-scale RCT provides no support for the use of omega-3 PUFAs to prevent depression (21). Although it has been suggested that FA might contribute to the pathologic of depression risk, the potential causal relationship between FA and depression remains unclear.
Previous observational studies and meta-analyses have investigated the association between FA and depression risk (18, 20–22). However, observational studies cannot comprehensively evaluate causal links and exclude unreliable and biased factors. RCTs are the gold standard for studying causal links between exposure and outcome, which can be costly, impractical, or unethical (23). Furthermore, conventional observational studies can alter exposure-outcome relationships by confounding variables and reverse causal connections, restricting causal inference (24). Fortunately, in recent years, much emphasis has been placed on delineating the causal genetic variants and biological mechanisms based on genome-wide association studies (GWAS) methodology, generating a wide range of robust associations for various traits and disorders (25, 26). By utilizing genetic variations as a natural experiment, Mendelian randomization (MR) is a novel method to assess the apparent causal relationships between a potential exposure factor and the outcome (27). In addition, MR methods can overcome confounding and reverse causality association problems on causal inference (28–31). Moreover, the Two-sample MR method refers to the application of MR approach to summary-level GWAS results estimated in non-overlapping sets of individuals, but from the same ethnicity. In recent years, MR analysis has been used to examine the causative role of FA on ischemic stroke (32), frailty (33), Alzheimer’s disease (34), and schizophrenia (35). Nonetheless, little MR evidence is available on the relationship between FA and depression. To further analyze the influence of different types of FAs on depression, the causal link between FA and depression was assessed using MR. To conduct a comprehensive MR analysis between FA and depression, we selected 16 types of FAs, including SFAs [palmitic acid (PA), arachidic acid, and stearic acid (SA)], MUFAs [oleic acid (OA), and palmitoleic acid (POA)], omega-3 PUFAs [eicosapentaenoic acid (EPA), α-linolenic acid (ALA), docosapentaenoic acid (DPA), and docosahexaenoic acid (DHA)], omega-6 PUFAs [arachidonic acid (AA), adrenic acid, gamma–linolenic acid (GLA), Dihomo-gamma–linolenic acid (DGLA), and linoleic acid (LA)], total omega-3 PUFA and total omega-6 PUFA.
Materials and methods
Study design
To explore the potential causal links between FA and the risk of depression, we devised a two-sample MR study. The MR method relies on the following assumptions (Figure 1): (1) the selected SNPs were reliably associated with FA; (2) SNPs must be independent of confounding factors; (3) SNPs were associated with the risk of depression only via FA levels.
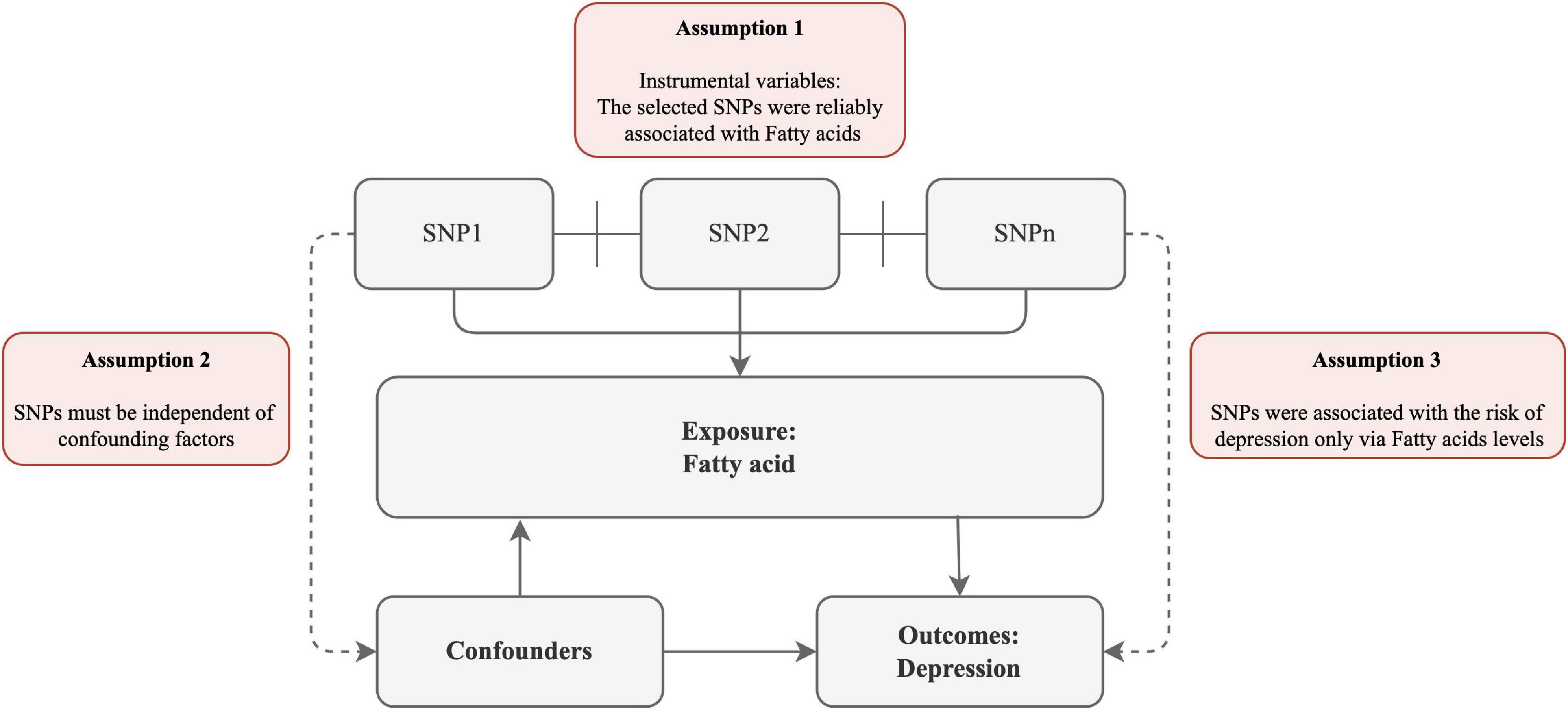
Figure 1. Schematic diagram of the assumptions underpinning a Mendelian randomization analysis of the association between plasma fatty acid (FA) levels and depression. We performed a two-sample MR study to examine the potential causal links between FA and the risk of depression. The MR method relies on the following assumptions: (1) the selected SNPs were reliably associated with FA; (2) SNPs must be independent of confounding factors; (3) SNPs were associated with the risk of depression only via FA levels.
Data sources
To ensure high power in the MR analysis and adequate reproducibility of results, our study collected publicly available GWAS summary data. First, we extracted SNPs associated with total omega-3 PUFA and total omega-6 PUFA at genome-wide significance level (P < 5 × 10–8) from the latest genome-wide association study (GWAS) of circulating PUFA, including 114,999 individuals of European ancestry (36). Next, we utilized several well-known meta-analysis genome-wide association results for the summary-level data of specific types of omega-3 and omega-6 PUFAs (37–40). Overall, we selected 52 SNPs as the genetic instruments for specific types of omega-3 PUFAs [docosapentaenoic acid (DPA, 3 SNPs), docosahexaenoic acid (DHA, 47 SNPs), α-linolenic acid (ALA, 1 SNP), eicosapentaenoic acid (EPA, 1 SNP)] and 64 SNPs as the genetic instruments for specific types of omega-6 PUFAs [arachidonic acid (AA, 2 SNPs), linoleic acid (LA, 57 SNPs), gamma-linolenic acid (GLA, 2 SNPs), dihomo-gamma-linolenic acid (DGLA, 2 SNPs), and adrenic acid (1 SNP)]. Moreover, summary statistics for non-PUFA were acquired from CHARGE (Cohorts for Heart and Aging Research in Genomic Epidemiology) (37). The summary statistics were generated from the European GWAS meta-analysis (n = 8,961 individuals for SFA or MUFA) (37). Moreover, we selected 5 SNPs as the genetic instruments for SFAs [PA (1 SNPs), arachidic acid (1 SNPs), and SA (3 SNPs)] and 5 SNPs as the genetic instruments for MUFAs [OA (1 SNP), and POA (4 SNPs)] (38). Finally, GWAS summary data on depression were obtained from the Psychiatric Genomics Consortium (PGC),1 including 246,363 cases and 561,190 controls (41). Considering the sample overlap between FA and depression GWAS, for total omega-3 and omega-6 PUFA levels, we extracted the SNP information from the meta-analysis of the 33 PGC cohorts, excluding UK Biobank, consisting of 135,548 cases and 344,901 controls (42). All GWAS summary data were mainly based on the GWAS of European cohorts. More details about these GWAS samples used in the present analysis are provided in Table 1.
Selection of genetic instrumental variants
We included the genome-wide significant single nucleotide polymorphisms (SNPs) (p < 5 × 10–8) as instrumental variables (IVs) (37–39, 41, 43). A more accurate effect size estimate with these variants was obtained as follows: genetic variants with high linkage disequilibrium (LD) (R2 > 0.001) were removed, and the distance between each variant was less than 10,000 kb. The SNPs were removed using the “clump” function implemented in the “TwoSampleMR” package (version 0.4.25). Furthermore, we removed palindromic variants with an ambiguous allele frequency from MR analyses (44).
Statistical analysis
To investigate the association between FA and risk of depression, we applied the following MR methods, including Wald ratio, inverse variance weighted (IVW), MR-Egger, simple mode, weighted mode, and weighted median. If there is only one SNP as genetic instrumental variant, we performed MR analysis using the Wald ratio method (45). The Wald ratio method (i.e., the beta coefficient for the effect of the SNP on the outcome divided by the beta coefficient for the effect of the SNP on the exposure) was used to infer the causal association between exposure and the outcome containing only one SNP (45). For multiple SNPs as genetic instrumental variants, we conducted MR analysis by using the other methods, including IVW method, MR-Egger, weighted mode, simple mode, and weighted median. In MR analysis, the IVW method is the conventional method for summary statistics data and may be directly employed to calculate causal effect sizes without requiring individual-level data (46). Using regression analysis of the SNP-FA and the SNP-depression associations, we calculated the mean IVW estimates of SNP ratios (46). The weighted median method was used to estimate the impact effects, which enabled us to find the weighted empirical distribution function for all the selected SNPs. By utilizing the weighted median method, SNPs with stronger effects contribute more to causal estimates, and when fewer SNPs are effective tools, there is less bias toward estimating causal effects (47). The MR-Egger analysis was conducted assuming that the magnitude of the pleiotropic effects and SNP exposure effects are uncorrelated, allowing for a non-zero intercept in the regression model and unbalanced horizontal variability across all SNPs (48). MR-Egger regression performed a regression of the SNP-depression risk on the SNP-FA association. However, the MR-Egger method can provide unbiased estimates even if all selected SNPs are invalid (48). The results were presented as beta and their 95% confidence intervals (CI). The significant threshold after Bonferroni-adjustment for multiple tests was P < 0.003 (0.05/16 = 3.13E-3) since we conducted MR analyses of 16 types of FAs. To visualize the results for statistical analysis and the estimated effects for each SNP, we used the data analysis functionality of the MR-based platform to generate forest plots and scatter plots of the SNP-associated FA and depression risk (49). It is widely acknowledged that MR analysis results may be subject to significant heterogeneity due to differences in inclusion criteria, populations studied, and SNPs, thereby biasing causal effect inference. We conducted MR-Egger regression to assess the possible pleiotropy of SNPs used as IVs. The MR-Egger regression intercept implies the presence of directional horizontal pleiotropy drives the results from MR analyses (48). MR-Egger regression and IVW approaches were used to detect underlying heterogeneity of the association between different genetic variants. The significant heterogeneity was quantified with (p-value for Cochran’s Q statistic < 0.05). All analyses were performed using the TwoSampleMR package (version 0.5.6) in R (version 4.1.2) (49).
Results
Characteristics of single nucleotide polymorphisms associated with fatty acids as instrumental variables
After removing palindromic variants and SNPs with high LD (R2 > 0.001; distance < 1,000 kb), we identified SNPs associated with total omega-3 PUFA (n = 48), total omega-6 PUFA (n = 64), ALA (n = 1), EPA (n = 1), DPA (n = 3), DHA (n = 30), AA (n = 2), adrenic acid (n = 1), GLA (n = 2), DGLA (n = 2), LA (n = 44), POA (n = 4), OA (n = 1), SA (n = 2), arachidic acid (n = 1), and PA (n = 1) in the depression GWAS. These SNPs were not considered weak instrumental variables (F-statistic ≥ 10) and were eligible for MR analyses. Supplementary Table 1 provides details of the SNPs and their correlations to each type of FAs.
Causal effects of omega-3 fatty acids on depression
Our MR analysis indicated that the level of total omega-3 PUFA was not significantly associated with the risk of depression using the IVW method (OR = 0.96, 95% CI = 0.91 to 1.00, p = 0.07; Table 2 and Figure 2). Modified Q statistics indicated no notable heterogeneity (p = 0.73; Supplementary Table 2) across instrument SNP effects. Moreover, there was no horizontal pleiotropy by the MR-Egger intercept test (p = 0.73; Supplementary Table 2). The scatter plot, forest plot, funnel plot, and leave-one-out plot for the MR associations between total omega-3 PUFA and depression are provided in Supplementary Figure 1. Among the four types of omega-3 PUFAs, we detected a significant causal effect for ALA (OR = 2.52, 95% CI = 1.45 to 4.38, p = 1.01E-03) and EPA (OR = 0.84, 95% CI = 0.76 to 0.95, p = 3.11E-03) on depression risk (Table 2 and Figure 2) after Bonferroni correction. However, the levels of DPA (OR = 0.88, 95% CI = 0.79 to 0.97, p = 9.84E-03) and DHA (OR = 0.97, 95% CI = 0.95 to 1.00, p = 3.21E-02) showed no causal association with depression risk after Bonferroni correction. The forest plot, scatter plot, funnel plot, and leave-one-out plot for the MR associations between omega-3 PUFA, DPA, DHA, and depression risk are shown in Supplementary Figures 2, 3.
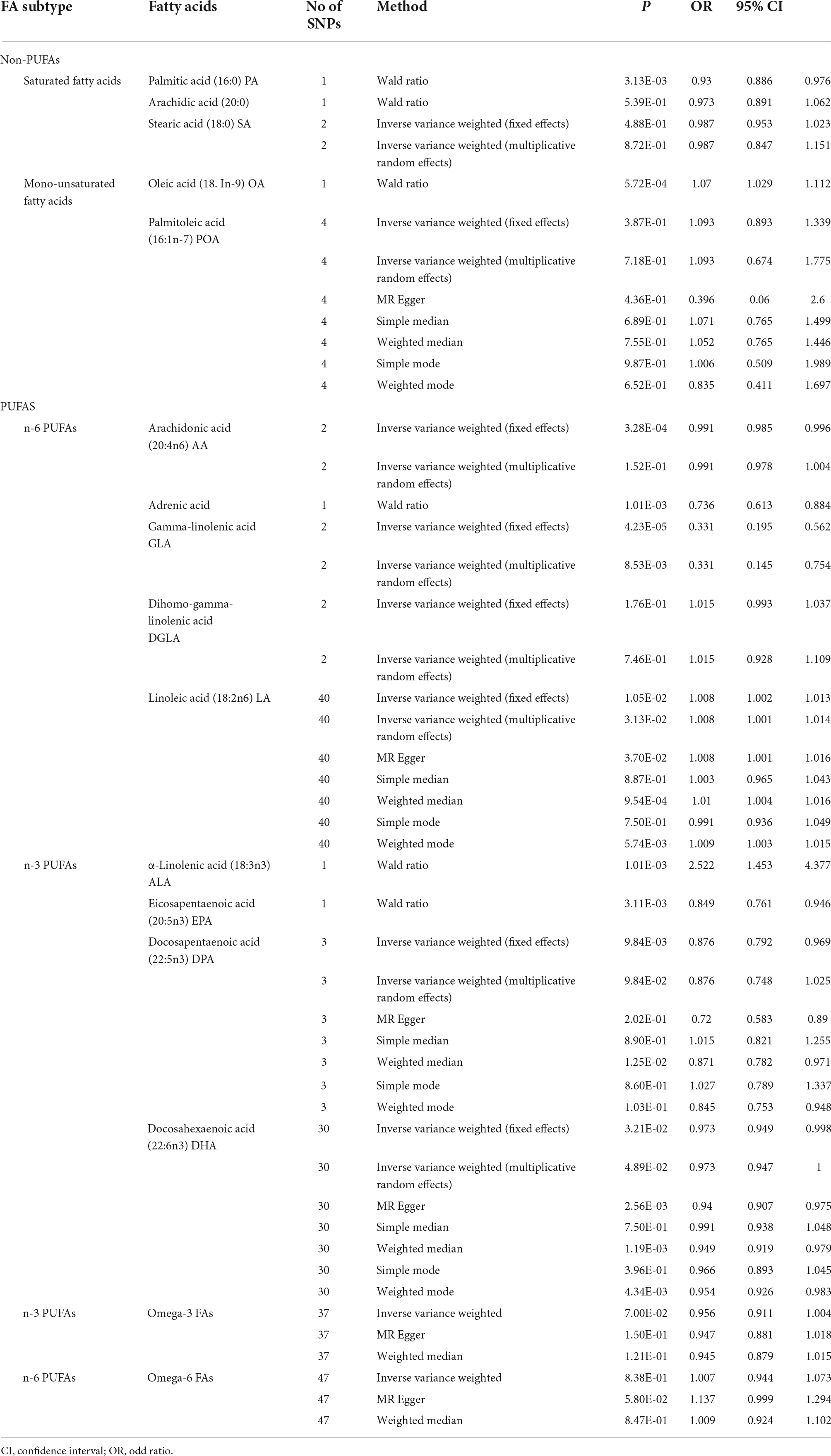
Table 2. Associations of plasma fatty acid levels with risk of depression in Mendelian randomization analyses (Wald ratio, multiplicative random effects inverse-variance weighted model fixed-effects inverse-variance weighted model).
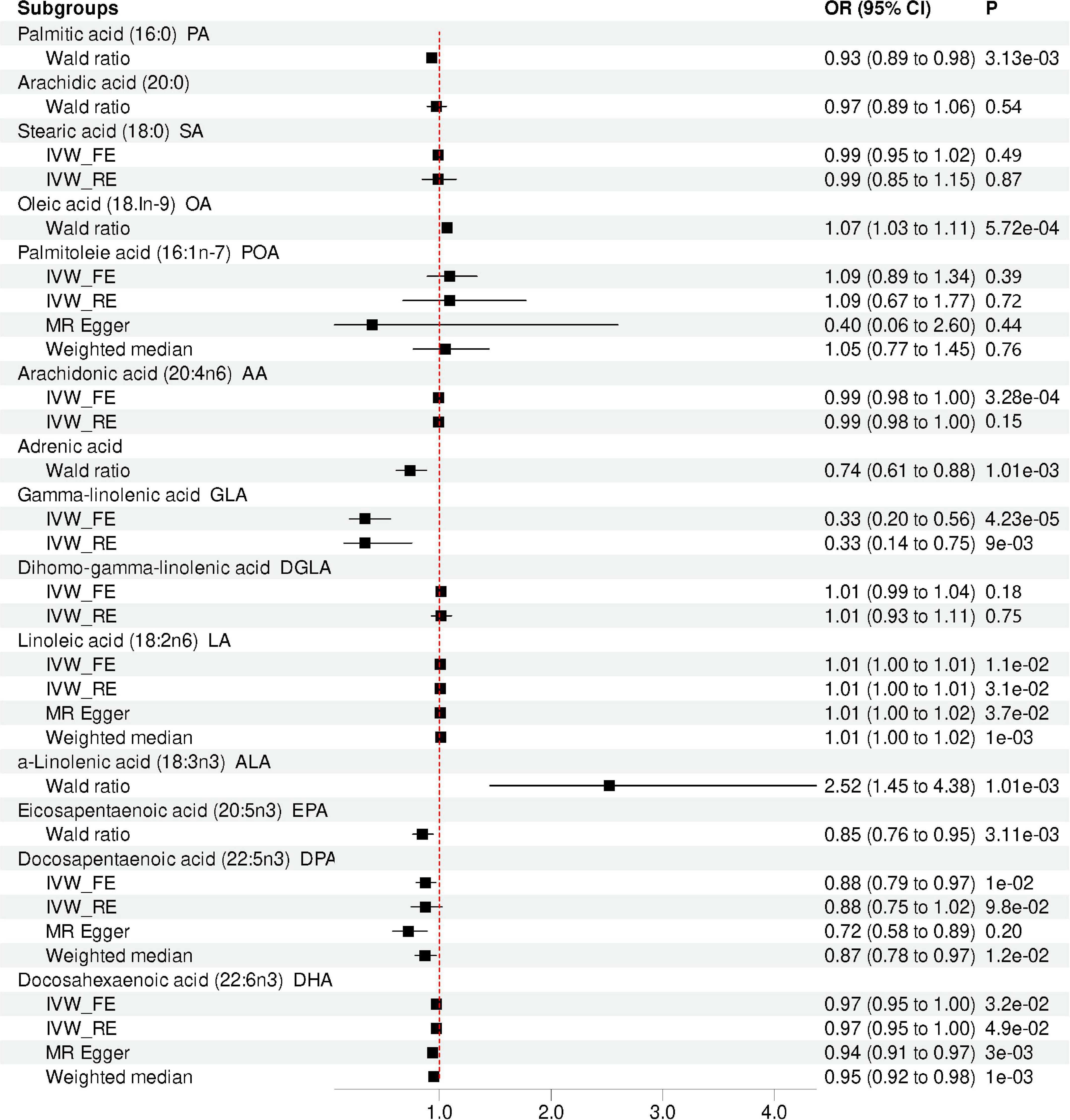
Figure 2. Mendelian randomization analysis showing the effects of different type of FA on depression risk. IVW_FE: fixed-effects inverse-variance weighted model; IVW_RE: random effect inverse variance weighted; CI, confidence interval; OR, odd ratio.
Causal effects of omega-6 fatty acids on depression
Our results suggested that the level of total omega-6 PUFA was not significantly associated with depression using the IVW method (OR = 1.00, 95% CI = 0.94 to 1.07, p = 0.84; Table 2 and Figure 2). Cochran’s Q of IVW analysis revealed no significant heterogeneity among SNPs (p = 0.73; Supplementary Table 2). Additionally, MR-Egger regression analysis showed no horizontal pleiotropy among SNPs between omega-6 FA and depression (p = 0.73; Supplementary Table 2). Supplementary Figure 4 displays scatter plots of the SNP-omega-6 FA and SNP-depression association estimates with five different MR methods, which visualized the causal effect estimates of each SNP on depression. In the funnel plot (Supplementary Figure 5), the causal effect point exhibited a symmetric distribution, indicating that a single SNP as IV was less likely to bias the cause. Among the five specific types of omega-6 PUFAs, genetically predicted level of AA (OR = 0.99, 95% CI = 0.99 to 1.00, p = 3.28E-04), adrenic acid (OR = 0.74; 95% CI = 0.61 to 0.88; p = 1.01E-03), and GLA (OR = 0.33, 95% CI = 0.20 to 0.56, p = 4.23E-05) were associated with depression, after Bonferroni correction. However, AA and GLA exhibited significant heterogeneity in the IVW analyses and was not significantly associated with the depression corrected by the multiplicative random-effects IVW method AA (OR = 0.99, 95% CI = 0.98 to 1.00, p = 0.15) and GLA (OR = 0.33, 95% CI = 0.15 to 0.75, p = 8.53E-03). Genetic predictions demonstrated no significant association between DGLA (OR = 1.02, 95% CI = 0.99 to 1.04, p = 0.18), LA (OR = 1.01, 95% CI = 1.00 to 1.01, p = 0.01) and depression after Bonferroni correction.
Causal effects of saturated fatty acids on depression
Genetic predictions showed an association between PA levels and depression (OR = 0.93; 95% CI = 0.89 to 0.98; p = 3.13E-03), which was no longer observed after Bonferroni correction. Sensitivity analysis based on MR Egger and weighted median methods yielded similar results for the causal effects of PA levels on depression (Table 2 and Figure 2). Heterogeneity and horizontal pleiotropy analyses were not conducted since only one SNP was left. However, after the Bonferroni correction, no association was found between the levels of SA (OR = 0.99, 95%CI: 0.95 to 1.02, p = 0.49), Arachidic acid (OR = 0.97, 95%CI: 0.89 to 1.06, p = 0.54) and depression. The scatter plot, forest plot, funnel plot, and leave-one-out plot for the MR associations between SA and depression are provided in Supplementary Figure 5.
Causal effects of mono-unsaturated fatty acids on depression
There was one single SNP associated with OA conducted using Wald ratio MR analyses were statistically associated with depression (OR = 1.07, 95% CI = 1.03 to 1.11, p = 5.72E-04), surviving after Bonferroni correction. However, after the Bonferroni correction, no association was found between the levels of POA and depression (OR = 1.09, 95% CI = 0.89 to 1.34, p = 0.39). The scatter plot, forest plot, funnel plot, and leave-one-out plot for the MR associations between POA and depression are provided in Supplementary Figure 6.
Discussion
In this work, the MR approach was used to obtain accurate findings regarding the influence of FA on depression. We found that higher levels of adrenic acid and EPA were associated with a decreased risk of depression. In contrast, our MR results suggested that higher levels of OA and ALA could increase the risk of depression. Most importantly, the present investigation found no causal relationship between other omega-3 PUFAs (DHA and DPA), omega-6 PUFAs (AA, GLA, DGLA, and LA), MUFA (POA), and SFA (arachidic acid, PA, and SA) and depression risk. Our study was based on GWAS summary data and provided novel insights into the potential roles of specific FAs on depression risk for follow-up studies.
Our results indicated that elevated ALA levels were associated with increased depression risk, whereas higher EPA levels were associated with decreased depression risk. ALA is the precursor for long-chain PUFAs, including EPA and DHA (50), which are essential components of neuronal membrane phospholipids and regulate the physical and physiological features of neuronal membranes (51). Although the conversion efficiency is reportedly moderate, enough ALA consumption from flaxseed oil can dramatically increase the amounts of neuronal long-chain omega-3 PUFAs in rats (52). It has been established that neurotransmitter signaling in the GABAergic, serotonergic, or dopaminergic systems might be related to the neurobehavioral effects of ALA on depression risk (53). Our result for the causal relationship between EPA and depression is consistent with the earlier MR study (54). Growing evidence implicated that EPA plays a crucial role in the development and nervous system repair of synapse and nerve growth cones which are the basis of the neurodevelopmental hypothesis in depression (55–60). However, previous observational and clinical trial data on the association of omega-3 PUFA with depression have yielded conflicting results. A meta-analysis of observational studies suggested that marine omega-3 PUFA may reduce the risk of depression and promote a favorable mood (18). A cross-sectional observational study found no evidence for associations between DHA and omega-3 index and depression (19). The discrepancy among these observational studies may be explained by residual confounding. Additionally, the effect of supplementation with omega-3 PUFA on the risk of depression has been extensively studied over the years (20–22). For example, a meta-analysis of double-blind randomized placebo-controlled trials showed an overall beneficial effect of omega-3 PUFAs with EPA ≥ 60% at a dosage of ≤1 g/d on depression symptoms, whereas DHA-pure and DHA-major formulations did not yield such benefits (20). However, a large-scale randomized clinical trial involving 18,353 adults aged 50 years or older found no evidence supporting the use of omega-3 supplements in adults to prevent depression (21). Therefore, we hypothesized that omega-3 PUFA supplementation might not be beneficial for the prevention of depression but might be beneficial (specifically EPA) for the treatment of MDD. The confounding factors may be responsible for the inconsistent outcomes. Given that MR techniques might overcome confounding variables and offer the causal effects of FA on depression, we hypothesized that increasing levels of ALA might increase the risk of depression.
Our MR study found no association between total omega-6 PUFA and depression, showing consistent results compared with previous observational studies (61, 62). In a previous cross-sectional observational study, no association was found between omega-6 PUFA levels and depression (61). Meanwhile, observational research revealed that circulating omega-6 PUFA levels were not associated with depression relapse risk (62). As for the specific type of omega-6 PUFAs, the current investigation revealed that the higher level of adrenic acid was associated with a reduced risk of depression. Adrenic acid, the immediate precursor derived from arachidonic acid, is necessary for neuronal development and enrichment of myelinic lipids (63, 64). Adrenic acid is metabolized by cytochrome P450s to a group of epoxy fatty acids and epoxyeicosatrienoic acids, which are the vital lipid mediators playing key role in exerting analgesia and reducing endoplasmic reticulum stress (65). Furthermore, functional studies showed that adrenic acid potently inhibited the formation of the chemoattractant leukotriene B4 (LTB4), which has been proposed as a contributor for chronic neuroinflammatory effects (66–68). Given that, we could pay more attention to the role of the specific type of omega-6 PUFAs in depression, such as adrenic acid. Our findings might provide novel insights into the link between omega-6 PUFA and depression, given the paucity of existing research on the associations between omega-6 PUFA levels and depression.
Our MR analysis found a significant causal effect between specific types of MUFAs and the risk of depression. Little evidence is available on the association between MUFA and the risk of depression. The consumption of OA was positively related to depressive symptoms, according to a cross-sectional study involving 2,793 perimenopausal women (69). Mechanically, neural differentiation depends on OA production (70). Moreover, it has been shown that the composition of FA in the hippocampus and brain of rats with cognitive impairment is altered by OA (71). Hamilton and Fernandes (72) found that co-infusion with OA reduced the abundance of proliferating neural stem cells (NSCs) and suppressed the activation of NSCs in mice models. Therefore, OA might be involved in depression by affecting neural functions. However, the relationships between OA and depression remain largely unclear, warranting further experiments.
Importantly, in the present study, we adopted MR analyses which can avoid reverse causality induced by intrinsic confounders in conventional observational research. Moreover, due to the relatively large sample size, our MR study had more statistical power, which made the findings more accurate. However, some limitations must be considered when interpreting our findings. Our current MR investigation used GWAS summary data, and the study populations were predominantly European. The applicability of the study’s findings to other populations remains unclear, emphasizing the need for more studies. Besides, the original GWAS data of depression and characteristics of subjects could not be retrieved directly. Consequently, no subgroup analysis according to characteristics of subjects (sex, geography, and so on) was conducted. Moreover, the MR approach could only provide a preliminary indication of the causative link between FA and depression. The biological mechanism between FA and depression would be comprehensively investigated the further experiments.
Conclusion
Overall, our MR analyses provide evidence that ALA and OA levels increase the risk of depression, whereas EPA and adrenic acid levels decrease the risk of depression. Nonetheless, more in-depth work is still needed to elucidate the underlying mechanisms that mediate the relationship between ALA, OA, EPA, and adrenic acid levels and depression.
Data availability statement
The original contributions presented in this study are included in the article/Supplementary material, further inquiries can be directed to the corresponding authors.
Author contributions
HL, LZ, and HY designed the study, contributed to analysis and interpretation of data, and wrote the first draft of the manuscript. HL, LZ, XW, XL, RX, CZ, and HY did the statistical analyses and prepared the tables and figures. HY provided further data interpretation. All authors contributed to drafting the work or revising it critically for important intellectual content and made substantial contributions to the concept and design of the study and acquisition, analysis, and interpretation of data.
Funding
This study was funded by the National Natural Science Foundation of China (81901358), Natural Science Foundation of Shandong Province (ZR2019BH001 and ZR2021YQ55), Medical and Health Science and Technology Development Plan of Shandong Province (202003061210, 202103090742, and 202103090692), Jining Key Research and Development Program (2020YXNS047, 2021YXNS024, and 2021YXNS077), and Young Taishan Scholars of Shandong Province (tsqn201909146). The funders had no role in the design and conduction of this study.
Acknowledgments
We gratefully acknowledge all the studies and databases that made GWAS summary data available.
Conflict of interest
The authors declare that the research was conducted in the absence of any commercial or financial relationships that could be construed as a potential conflict of interest.
Publisher’s note
All claims expressed in this article are solely those of the authors and do not necessarily represent those of their affiliated organizations, or those of the publisher, the editors and the reviewers. Any product that may be evaluated in this article, or claim that may be made by its manufacturer, is not guaranteed or endorsed by the publisher.
Supplementary material
The Supplementary Material for this article can be found online at: https://www.frontiersin.org/articles/10.3389/fnut.2022.1010476/full#supplementary-material
Footnotes
References
2. Vos T, Lim SS, Abbafati C, Abbas KM, Abbasi M, Abbasifard M, et al. Global burden of 369 diseases and injuries in 204 countries and territories, 1990–2019: a systematic analysis for the Global Burden of Disease Study 2019. Lancet. (2020) 396:1204–22.
3. Fusar-Poli P, Hijazi Z, Stahl D, Steyerberg EW. The science of prognosis in psychiatry: a review. JAMA Psychiatry. (2018) 75:1289–97.
4. Kim Y, Vadodaria KC, Lenkei Z, Kato T, Gage FH, Marchetto MC, et al. Mitochondria, metabolism, and redox mechanisms in psychiatric disorders. Antioxid Redox Signal. (2019) 31:275–317.
5. Sanacora G, Yan Z, Popoli M. The stressed synapse 2.0: pathophysiological mechanisms in stress-related neuropsychiatric disorders. Nat Rev Neurosci. (2022) 23:86–103. doi: 10.1038/s41583-021-00540-x
6. Firth J, Gangwisch JE, Borisini A, Wootton RE, Mayer EA. Food and mood: how do diet and nutrition affect mental wellbeing? BMJ. (2020) 369:m2382.
7. Sarris J, Logan AC, Akbaraly TN, Amminger GP, Balanzá-Martínez V, Freeman MP, et al. Nutritional medicine as mainstream in psychiatry. Lancet Psychiatry. (2015) 2:271–4.
8. Grosso G. Nutritional psychiatry: how diet affects brain through gut microbiota. Nutrients. (2021) 13:1282.
9. Tapiero H, Ba GN, Couvreur P, Tew KD. Polyunsaturated fatty acids (PUFA) and eicosanoids in human health and pathologies. Biomed Pharmacother. (2002) 56:215–22.
10. Chilton FH, Dutta R, Reynolds LM, Sergeant S, Mathias RA, Seeds MC. Precision nutrition and omega-3 polyunsaturated fatty acids: a case for personalized supplementation approaches for the prevention and management of human diseases. Nutrients. (2017) 9:1165. doi: 10.3390/nu9111165
12. Tsuboi H, Watanabe M, Kobayashi F, Kimura K, Kinae N. Associations of depressive symptoms with serum proportions of palmitic and arachidonic acids, and α-tocopherol effects among male population–a preliminary study. Clin Nutr. (2013) 32:289–93. doi: 10.1016/j.clnu.2012.07.011
13. Wolfe AR, Ogbonna EM, Lim S, Li Y, Zhang J. Dietary linoleic and oleic fatty acids in relation to severe depressed mood: 10 years follow-up of a national cohort. Prog Neuropsychopharmacol Biol Psychiatry. (2009) 33:972–7. doi: 10.1016/j.pnpbp.2009.05.002
14. Freeman MP, Hibbeln JR, Wisner KL, Davis JM, Mischoulon D, Peet M, et al. Omega-3 fatty acids: evidence basis for treatment and future research in psychiatry. J Clin Psychiatry. (2006) 67:1954–67.
15. Messamore E, Almeida DM, Jandacek RJ, Mcnamara RK. Polyunsaturated fatty acids and recurrent mood disorders: phenomenology, mechanisms, and clinical application. Prog Lipid Res. (2017) 66:1–13. doi: 10.1016/j.plipres.2017.01.001
16. Zhou X, Liu L, Lan X, Cohen D, Zhang Y, Ravindran AV, et al. Polyunsaturated fatty acids metabolism, purine metabolism and inosine as potential independent diagnostic biomarkers for major depressive disorder in children and adolescents. Mol Psychiatry. (2019) 24:1478–88. doi: 10.1038/s41380-018-0047-z
17. Bozzatello P, Blua C, Rocca P, Bellino S. Mental health in childhood and adolescence: the role of polyunsaturated fatty acids. Biomedicines. (2021) 9:850.
18. Li F, Liu X, Zhang D. Fish consumption and risk of depression: a meta-analysis. J Epidemiol Community Health. (2016) 70:299–304.
19. van der Wurff ISM, Von Schacky C, Bergeland T, Leontjevas R, Zeegers MP, Kirschner PA, et al. Exploring the association between whole blood omega-3 index, DHA, EPA, DHA, AA and n-6 DPA, and depression and self-esteem in adolescents of lower general secondary education. Eur J Nutr. (2019) 58:1429–39.
20. Liao Y, Xie B, Zhang H, He Q, Guo L, Subramanieapillai M, et al. Efficacy of omega-3 PUFAs in depression: a meta-analysis. Transl Psychiatry. (2019) 9: 190.
21. Okereke OI, Vyas CM, Mischoulon D, Chang G, Cook NR, Weinberg A, et al. Effect of long-term supplementation with marine omega-3 fatty acids vs placebo on risk of depression or clinically relevant depressive symptoms and on change in mood scores: a randomized clinical trial. JAMA. (2021) 326:2385–94.
22. Grosso G, Pajak A, Marventano S, Castellano S, Galvano F, Bucolo C, et al. Role of omega-3 fatty acids in the treatment of depressive disorders: a comprehensive meta-analysis of randomized clinical trials. PLoS One. (2014) 9:e96905. doi: 10.1371/journal.pone.0096905
24. Larsson SC. Mendelian randomization as a tool for causal inference in human nutrition and metabolism. Curr Opin Lipidol. (2021) 32:1–8. doi: 10.1097/MOL.0000000000000721
25. Gallagher MD, Chen-Plotkin AS. The post-GWAS era: from association to function. Am J Hum Genet. (2018) 102:717–30.
26. Uffelmann E, Huang QQ, Munung NS, De Vries J, Okada Y, Martin AR, et al. Genome-wide association studies. Nat Rev Methods Prim. (2021) 1:59.
27. Davies NM, Holmes MV, Davey Smith G. Reading Mendelian randomisation studies: a guide, glossary, and checklist for clinicians. BMJ. (2018) 362:k601. doi: 10.1136/bmj.k601
28. Gao K, Wei C, Zhu J, Wang X, Chen G, Luo Y, et al. Exploring the causal pathway from telomere length to Alzheimer’s disease: an update Mendelian randomization study. Front Psychiatry. (2019) 10:843. doi: 10.3389/fpsyt.2019.00843
29. Chen G, Wang Q, Xue R, Liu X, Yu H. Examining the causal inference of leptin and soluble plasma leptin receptor levels on schizophrenia: a Mendelian randomization study. Front Psychiatry. (2021) 12:753224. doi: 10.3389/fpsyt.2021.753224
30. Chen G, Lv H, Zhang X, Gao Y, Liu X, Gu C, et al. Assessment of the relationships between genetic determinants of thyroid functions and bipolar disorder: a Mendelian randomization study. J Affect Disord. (2022) 298:373–80. doi: 10.1016/j.jad.2021.10.101
31. Yu J, Xue R, Wang Q, Yu H, Liu X. The effects of plasma homocysteine level on the risk of three major psychiatric disorders: a Mendelian randomization study. Front Psychiatry. (2022) 13:841429. doi: 10.3389/fpsyt.2022.841429
32. Yuan T, Si S, Li Y, Li W, Chen X, Liu C, et al. Roles for circulating polyunsaturated fatty acids in ischemic stroke and modifiable factors: a Mendelian randomization study. Nutr J. (2020) 19:70. doi: 10.1186/s12937-020-00582-4
33. Tomata Y, Wang Y, Hägg S, Jylhävä J. Fatty acids and frailty: a Mendelian randomization study. Nutrients. (2021) 13:3539. doi: 10.3390/nu13103539
34. Tomata Y, Larsson SC, Hägg S. Polyunsaturated fatty acids and risk of Alzheimer’s disease: a Mendelian randomization study. Eur J Nutr. (2020) 59: 1763–6.
35. Jones HJ, Borges MC, Carnegie R, Mongan D, Rogers PJ, Lewis SJ, et al. Associations between plasma fatty acid concentrations and schizophrenia: a two-sample Mendelian randomisation study. Lancet Psychiatry. (2021) 8:1062–70. doi: 10.1016/S2215-0366(21)00286-8
36. Borges MC, Haycock PC, Zheng J, Hemani G, Holmes MV, Davey Smith G, et al. Role of circulating polyunsaturated fatty acids on cardiovascular diseases risk: analysis using Mendelian randomization and fatty acid genetic association data from over 114,000 UK Biobank participants. BMC Med. (2022) 20:210. doi: 10.1186/s12916-022-02399-w
37. Lemaitre RN, Tanaka T, Tang W, Manichaikul A, Foy M, Kabagambe EK, et al. Genetic loci associated with plasma phospholipid n-3 fatty acids: a meta-analysis of genome-wide association studies from the CHARGE Consortium. PLoS Genet. (2011) 7:e1002193. doi: 10.1371/journal.pgen.1002193
38. Wu JH, Lemaitre RN, Manichaikul A, Guan W, Tanaka T, Foy M, et al. Genome-wide association study identifies novel loci associated with concentrations of four plasma phospholipid fatty acids in the de novo lipogenesis pathway: results from the Cohorts for Heart and Aging Research in Genomic Epidemiology (CHARGE) consortium. Circ Cardiovasc Genet. (2013) 6:171–83. doi: 10.1161/CIRCGENETICS.112.964619
39. Guan W, Steffen BT, Lemaitre RN, Wu JHY, Tanaka T, Manichaikul A, et al. Genome-wide association study of plasma N6 polyunsaturated fatty acids within the cohorts for heart and aging research in genomic epidemiology consortium. Circ Cardiovasc Genet. (2014) 7:321–31. doi: 10.1161/CIRCGENETICS.113.000208
40. Lemaitre RN, King IB, Kabagambe EK, Wu JH, Mcknight B, Manichaikul A, et al. Genetic loci associated with circulating levels of very long-chain saturated fatty acids. J Lipid Res. (2015) 56:176–84.
41. Howard DM, Adams MJ, Clarke TK, Hafferty JD, Gibson J, Shirali M, et al. Genome-wide meta-analysis of depression identifies 102 independent variants and highlights the importance of the prefrontal brain regions. Nat Neurosci. (2019) 22:343–52. doi: 10.1038/s41593-018-0326-7
42. Wray NR, Ripke S, Mattheisen M, Trzaskowski M, Byrne EM, Abdellaoui A, et al. Genome-wide association analyses identify 44 risk variants and refine the genetic architecture of major depression. Nat Genet. (2018) 50:668–81. doi: 10.1038/s41588-018-0090-3
43. May-Wilson S, Sud A, Law PJ, Palin K, Tuupanen S, Gylfe A, et al. Pro-inflammatory fatty acid profile and colorectal cancer risk: a Mendelian randomisation analysis. Eur J Cancer. (2017) 84:228–38. doi: 10.1016/j.ejca.2017.07.034
44. Bowden J, Davey Smith G, Burgess S. Mendelian randomization with invalid instruments: effect estimation and bias detection through Egger regression. Int J Epidemiol. (2015) 44:512–25.
45. Bautista LE, Smeeth L, Hingorani AD, Casas JP. Estimation of bias in nongenetic observational studies using “mendelian triangulation”. Ann Epidemiol. (2006) 16:675–80.
46. Stephen B, Adam B, Thompson SG. Mendelian randomization analysis with multiple genetic variants using summarized data. Genet Epidemiol. (2013) 37:658–65.
47. Bowden J, Davey SG, Haycock PC, Burgess S. Consistent estimation in Mendelian randomization with some invalid instruments using a weighted median estimator. Genet Epidemiol. (2016) 40:304–14.
48. Jack B, George DS, Stephen B. Mendelian randomization with invalid instruments: effect estimation and bias detection through Egger regression. Int J Epidemiol. (2015) 44:512–25.
49. Hemani G, Zheng J, Elsworth B, Wade KH, Haberland V, Baird D, et al. The MR-base platform supports systematic causal inference across the human phenome. Elife. (2018) 7:e34408. doi: 10.7554/eLife.34408
50. Dawczynski C, Plagge J, Jahreis G, Liebisch G, Höring M, Seeliger C, et al. Dietary PUFA preferably modify ethanolamine-containing glycerophospholipids of the human plasma lipidome. Nutrients. (2022) 14:3055. doi: 10.3390/nu14153055
51. Hishikawa D, Valentine WJ, Iizuka-Hishikawa Y, Shindou H, Shimizu T. Metabolism and functions of docosahexaenoic acid-containing membrane glycerophospholipids. FEBS Lett. (2017) 591:2730–44.
52. Wang DD, Wu F, Ding L, Shi HH, Xue CH, Wang YM, et al. Dietary n-3 PUFA deficiency increases vulnerability to scopolamine-induced cognitive impairment in male C57BL/6 mice. J Nutr. (2021) 151:2206–14. doi: 10.1093/jn/nxab116
53. Chen HF, Su HM. Exposure to a maternal n-3 fatty acid-deficient diet during brain development provokes excessive hypothalamic-pituitary-adrenal axis responses to stress and behavioral indices of depression and anxiety in male rat offspring later in life. J Nutr Biochem. (2013) 24:70–80. doi: 10.1016/j.jnutbio.2012.02.006
54. Sallis H, Steer C, Paternoster L, Davey Smith G, Evans J. Perinatal depression and omega-3 fatty acids: a Mendelian randomisation study. J Affect Disord. (2014) 166:124–31. doi: 10.1016/j.jad.2014.04.077
55. Hibbeln JR, Ferguson TA, Blasbalg TL. Omega-3 fatty acid deficiencies in neurodevelopment, aggression and autonomic dysregulation: opportunities for intervention. Int Rev Psychiatry. (2006) 18:107–18. doi: 10.1080/09540260600582967
56. Robson LG, Dyall S, Sidloff D, Michael-Titus AT. Omega-3 polyunsaturated fatty acids increase the neurite outgrowth of rat sensory neurones throughout development and in aged animals. Neurobiol Aging. (2010) 31:678–87. doi: 10.1016/j.neurobiolaging.2008.05.027
57. Dyall SC. Long-chain omega-3 fatty acids and the brain: a review of the independent and shared effects of EPA, DPA and DHA. Front Aging Neurosci. (2015) 7:52. doi: 10.3389/fnagi.2015.00052
58. Tesei A, Crippa A, Ceccarelli SB, Mauri M, Molteni M, Agostoni C, et al. The potential relevance of docosahexaenoic acid and eicosapentaenoic acid to the etiopathogenesis of childhood neuropsychiatric disorders. Eur Child Adolesc Psychiatry. (2017) 26:1011–30. doi: 10.1007/s00787-016-0932-4
59. Chang JP, Su KP. Nutritional neuroscience as mainstream of psychiatry: the evidence- based treatment guidelines for using omega-3 fatty acids as a new treatment for psychiatric disorders in children and adolescents. Clin Psychopharmacol Neurosci. (2020) 18:469–83. doi: 10.9758/cpn.2020.18.4.469
60. Liu J-H, Wang Q, You Q-L, Li Z-L, Hu N-Y, Wang Y, et al. Acute EPA-induced learning and memory impairment in mice is prevented by DHA. Nat Commun. (2020) 11:5465. doi: 10.1038/s41467-020-19255-1
61. Thesing CS, Bot M, Milaneschi Y, Giltay EJ, Penninx B. Omega-3 and omega-6 fatty acid levels in depressive and anxiety disorders. Psychoneuroendocrinology. (2018) 87:53–62.
62. Thesing CS, Lok A, Milaneschi Y, Assies J, Bockting CLH, Figueroa CA, et al. Fatty acids and recurrence of major depressive disorder: combined analysis of two Dutch clinical cohorts. Acta Psychiatr Scand. (2020) 141:362–73. doi: 10.1111/acps.13136
63. Wilson R, Sargent JR. Lipid and fatty acid composition of brain tissue from adrenoleukodystrophy patients. J Neurochem. (1993) 61:290–7.
64. Jahn R, Scheller RH. SNAREs — Engines for membrane fusion. Nat Rev Mol Cell Biol. (2006) 7:631–43.
65. Singh N, Barnych B, Wagner KM, Wan D, Morisseau C, Hammock BD. Adrenic acid-derived epoxy fatty acids are naturally occurring lipids and their methyl ester prodrug reduces endoplasmic reticulum stress and inflammatory pain. ACS Omega. (2021) 6:7165–74. doi: 10.1021/acsomega.1c00241
66. Simopoulos AP. Omega-3 fatty acids in inflammation and autoimmune diseases. J Am Coll Nutr. (2002) 21:495–505.
67. Brouwers H, Jonasdottir HS, Kuipers ME, Kwekkeboom JC, Auger JL, Gonzalez-Torres M, et al. Anti-inflammatory and proresolving effects of the omega-6 polyunsaturated fatty acid adrenic acid. J Immunol. (2020) 205:2840–9. doi: 10.4049/jimmunol.1801653
68. Regulska M, Szuster-Gluszczak M, Trojan E, Leskiewicz M, Basta-Kaim A. The emerging role of the double-edged impact of arachidonic acid- derived eicosanoids in the neuroinflammatory background of depression. Curr Neuropharmacol. (2021) 19:278–93. doi: 10.2174/1570159X1866620080714453
69. Li D, Tong Y, Li Y. Associations between dietary oleic acid and linoleic acid and depressive symptoms in perimenopausal women: the study of Women’s health across the nation. Nutrition. (2020) 71:110602. doi: 10.1016/j.nut.2019.110602
70. Tabernero A, Lavado EM, Granda B, Velasco A, Medina JM. Neuronal differentiation is triggered by oleic acid synthesized and released by astrocytes. J Neurochem. (2001) 79:606–16.
71. Ulmann L, Mimouni V, Roux S, Porsolt R, Poisson JP. Brain and hippocampus fatty acid composition in phospholipid classes of aged-relative cognitive deficit rats. Prostaglandins Leukot Essent Fatty Acids. (2001) 64:189–95. doi: 10.1054/plef.2001.0260
Keywords: depression, Mendelian randomization, fatty acids (FA), non-polyunsaturated fatty acids (non-PUFA), polyunsaturated fatty acids (PUFA), omega-3 fatty acids, omega-6 fatty acids
Citation: Zeng L, Lv H, Wang X, Xue R, Zhou C, Liu X and Yu H (2022) Causal effects of fatty acids on depression: Mendelian randomization study. Front. Nutr. 9:1010476. doi: 10.3389/fnut.2022.1010476
Received: 03 August 2022; Accepted: 18 November 2022;
Published: 06 December 2022.
Edited by:
Antonio Lucio Teixeira, University of Texas Health Science Center at Houston, United StatesReviewed by:
Estela Jiménez López, University of Castilla–La Mancha, SpainInge Van Der Wurff, Open University of the Netherlands, Netherlands
Copyright © 2022 Zeng, Lv, Wang, Xue, Zhou, Liu and Yu. This is an open-access article distributed under the terms of the Creative Commons Attribution License (CC BY). The use, distribution or reproduction in other forums is permitted, provided the original author(s) and the copyright owner(s) are credited and that the original publication in this journal is cited, in accordance with accepted academic practice. No use, distribution or reproduction is permitted which does not comply with these terms.
*Correspondence: Hao Yu, eXVoYW9AbWFpbC5qbm1jLmVkdS5jbg==; Xia Liu, bGl1eGlhLjA5OUAxNjMuY29t
†These authors have contributed equally to this work