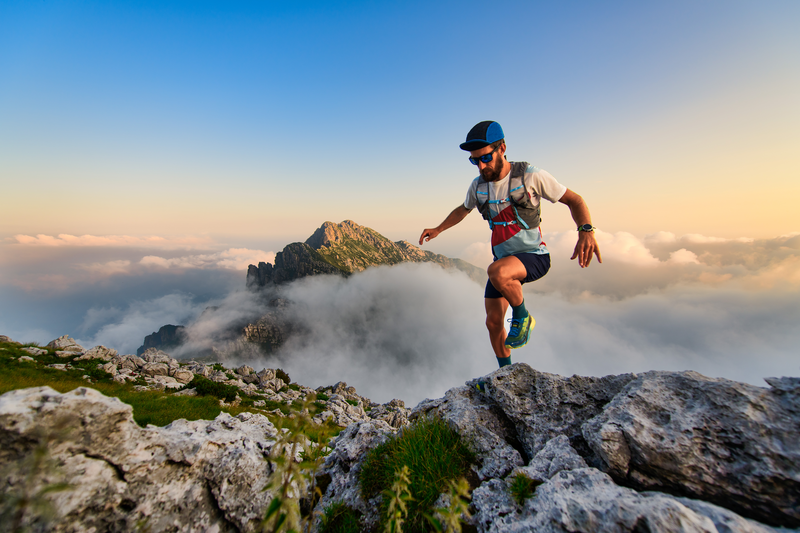
94% of researchers rate our articles as excellent or good
Learn more about the work of our research integrity team to safeguard the quality of each article we publish.
Find out more
REVIEW article
Front. Nutr. , 09 September 2022
Sec. Nutrition and Food Science Technology
Volume 9 - 2022 | https://doi.org/10.3389/fnut.2022.1004588
This article is part of the Research Topic Development of Nutrient Delivery Systems Constructed from Polysaccharides View all 6 articles
In order to solve the increasingly serious environmental problems caused by plastic-based packaging, carrageenan-based films are drawing much attentions in food packaging applications, due to low cost, biodegradability, compatibility, and film-forming property. The purpose of this article is to present a comprehensive review of recent developments in carrageenan-based films, including fabrication strategies, physical and chemical properties and novel food packaging applications. Carrageenan can be extracted from red algae mainly by hydrolysis, ultrasonic-assisted and microwave-assisted extraction, and the combination of multiple extraction methods will be future trends in carrageenan extraction methods. Carrageenan can form homogeneous film-forming solutions and fabricate films mainly by direct coating, solvent casting and electrospinning, and mechanism of film formation was discussed in detail. Due to the inherent limitations of the pure carrageenan film, physical and chemical properties of carrageenan films were enhanced by incorporation with other compounds. Therefore, carrageenan-based films can be widely used for extending the shelf life of food and monitoring the food freshness by inhibiting microbial growth, reducing moisture loss and the respiration, etc. This article will provide useful guidelines for further research on carrageenan-based films.
Food packaging is to separate food items from the surrounding environment, preventing microorganisms, oxygen, and water from contacting food, thus ensuring food quality and extending food shelf life (1, 2). It is estimated that approximately 36% of food packaging materials are petroleum-based plastics, including polyethylene (3, 4), polypropylene (5, 6) and polystyrene (7), due to their low cost, ease of manufacture, and better mechanical properties.
Meanwhile, the production of petroleum-based packaging materials still increases at 8% per year, with recycling rate <5% (8). Most plastics degrades in incineration and landfill, resulting in the production of microplastics smaller than 5 mm in diameter. High concentrations of microplastics will alter phytoplankton community structure, and therefore affect food chain and entire ecosystems (9, 10).
With growing awareness of environmental protection, an alternative food packaging material of bio-based polymers has received much attention, because of abundant resource (11), non-toxicity (12), and easily degrade within a few weeks at a specific temperature and humidity (13, 14). Polysaccharides (15–18), lipids (19, 20), and protein (21–24) are usually used as film-forming substrates for biodegradable packaging materials. In this regard, a hydroxyl-riched carrageenan is considered as a highly promising material, due to excellent gelling and film-forming properties. Carrageenan-based composite films are frequently designed by incorporating functional substances, such as polyphenols and enzymes, into carrageenan substrates (25–27). Over the past 2 years, researches focusing on carrageenan-based films have increased sharply. It was found that the number of publications in the research area of food science technology in 2019-2022 was more than five times that before 2019, when keywords like “carrageenan film” or “carrageenan coating” or “carrageenan packaging” were entered into search box of “topic” within scientific database “Web of Science”. However, only one review published in 2020-2022, which focused on the effect of nanomaterials (such as TiO2, SiO2, and copper sulfide) on carrageenan films, the properties of nanomaterial-enriched carrageenan films and their applications (28). Our study offers an overview of the latest comprehensive development of carrageenan-based biodegradable films for food packaging, focusing on high-level studies over past 3 years (Figure 1).
In order to discuss this topic logically, the source, extraction methods and characteristics of carrageenan were firstly summarized, and then the carrageenan gelation process and driving forces for the formation of carrageenan-based films are highlighted. Finally, properties and applications of carrageenan-based films were discussed in detail (Figure 2).
Carrageenan is composed of alternating copolymers of 3,6-anhydrous-galactose (3,6-AG) and D-galactose linked by α-1,3 and β-1,4-glycosidic bond (27, 29, 30). According to different number and position of sulfate groups, carrageenan can be divided into six basic forms, namely kappa (κ)-, lambda (λ)-, Iota (ι)-, Mu (μ)-, Theta (θ)- Nu (ν)- and ξ (xi)- carrageenan (31). With alkaline pretreatment, μ-, ν- and λ-carrageenan can be translated into κ-, ι-, and θ-carrageenan, respectively (Figure 3). Among them, κ-, ι-, and λ- carrageenans are the most widely used commercial products, which have one, two and three sulfate ester groups in each disaccharide repeat unit, respectively (32). In general, κ-carrageenan exhibits the strongest gelling ability because it contains about 25–30% sulfate groups and 28–35% 3, 6-anhydrogalactose contents (30).
All types of carrageenans are insoluble in organic solvents, oils, and fats, but soluble in water. The water solubility of carrageenan is influenced by multiple factors, such as the sulfate group on the molecule and the content of 3, 6-anhydrogalactose (33). Compared with other commercial carrageenans, κ-carrageenan exhibits lower aqueous solubility, because of low sulfate ester groups and high 3, 6-anhydrogalactose contents (34).
Carrageenan is extracted from red algae, such as Eucheuma Cottonii (35), Mastocarpus stellatus (36), and Hypnea musciformis (37), by multiple methods, including hydrolysis, ultrasonic-assisted and microwave-assisted extraction. The hydrolysis extraction is the most frequently used method, and the carrageenan yield can reach 27% (38). However, the extraction steps are complicated, solvent-consuming and time-consuming. In contrast, ultrasonic-assisted and microwave-assisted extraction methods possess shorter extraction time, lower energy consumption, and higher extraction yields, which have drawn an increasing attention. Ultrasonic-assisted extraction is a physical technology that can generate high pressure and high temperature in a short period of time, thereby generating high-intensity shear force, free radicals and shock waves, and resulting in physically destroying the plant cell wall (39, 40). A 50–55% carrageenan yield can be achieved within 15 min at the ultrasound power of 150 W (38). Since algae with high moisture contents are highly susceptible to microwave radiation, the microwave-assisted extraction is also an auxiliary method that can heat sample and solvent rapidly (41). The parameter settings are critical and have a considerable effect on the carrageenan yield. For example, the yield of carrageenan at a microwave temperature of 105 °C is 30.7% higher than that at 85 °C (42). Also, the carrageenan yield under neutral extraction conditions is approximately 37% higher than under alkaline conditions (43). Furthermore, all of these methods could change the structure, bioactivity, and composition of carrageenan. Therefore, the combination of multiple extraction technologies will be future trends in carrageenan extraction methods.
The gelation of carrageenan is a complex process, involving the coil-helix transition and then the helix aggregation (Figure 4). In the initial stage, the carrageenan in the sol state mostly exists in the form of irregular coils. Then the low-temperature cooling process induces formation of hydrogen bonds between the galactosyl units, which promotes the twisting of the anhydrogalactose sequence in a helix manner (44–46). As for the helix aggregation stage, double helices arranged laterally in a trigonal unit structure are interconnected by intermolecular interactions to form individual junction region in units of six to 10 molecules (44, 47), thereby resulting in forming cubic structured carrageenan gels (45). The number of molecules in the bonding area is affected by the cooling rate, and a large bonding area with more molecules is likely to be formed at the slower cooling rates (48). The higher the number of molecules in the junction zone, the more rigid the gel is. Therefore, the multi-molecular junction region of κ-carrageenan exhibits rigidity when disturbed by shear force, whereas the ι-carrageenan gel, consisting of only two molecules in the linkage region, has a more flexible structure and is less sensitive to shear (41, 49, 50).
In general, some salt ions, such as K+ and Ca2+, have been introduced into the film forming process (33). Because salt ions can partially neutralize the negative charge of sulfate groups in the carrageenan ionic chain through electrostatic interactions, thus reducing the electrostatic repulsion between sulfate groups, and facilitating a disorder-order conformational transition (24, 51). Other components have also been added into the carrageenan matrix through electrostatic interactions, such as gelatin and sodium caseinate. Disordered κ-carrageenan combined electrostatically with gelatin to facilitate the gelation process, leading to a high temperature of κ-carrageenan/gelatin conformational transition than that of pure carrageenan in film-forming process (52). In addition, sodium caseinate and negatively charged λ-carrageenan have been proposed to fabricate edible films. The cross-linking of polymer chains formed by electrostatic interactions could reduce the water vapor permeability of the film and improve moisture barrier properties (53).
As one of the most common non-covalent bonds, hydrogen bond is widely distributed in structure of the carrageenan film and critical for formation of pure carrageenan films. It is mainly explained by that the abundant hydroxyl groups in carrageenan molecules can form intramolecular or intermolecular hydrogen bonds with each other. Moreover, other polysaccharides have commonly been added to carrageenan for improving the properties of the resulting films (45, 54, 55). Agar molecules and κ-carrageenan molecules can be intertwined through hydrogen bonds to form agar/κ-carrageenan mixed double helixes (56). Similarly, arrowroot starch molecules and amylose can combine with carrageenan molecules through hydrogen bonding, resulting in films with superior mechanical properties compared with pure carrageenan film (57).
Direct coating is commonly used for fabricating edible packaging, including spray coating and dipping (58–60). Spray coating is a technology in which high pressure is usually used to atomize the film-forming solution and spray it onto the food surface (59, 60). Although the efficiency of spray coating is high, the operation is complicated and requires the professional equipment. Compared with the spray coating method, the dip coating method has been used for a more extensive range of applications. It involved preparation of the coating solution, dipping the food in coating solutions, removing excess coating solutions, and air-drying of the coating (61, 62). As it is known, the coating thickness closely affects films properties, and the coating thickness by dipping method is generally larger than that prepared by spray method (63). The dipping method is relatively simple to operate, but it requires a large amount of film-forming solutions. In summary, since the coating is directly contacted with the food, films prepared by the direct coating method can interfere with the sensory aspects of consumers, which requires the films with acceptable sensory properties.
Solvent casting is the most common method for manufacturing films, which involves preparation of film-forming solutions, spreading film-forming solutions on a suitable mold and drying. Carrageenan/alginate (64), carrageenan/gelatin (65), and carrageenan/starch (66), composite films are usually formed by this method. Numerous factors can affect the film formation process. For instance, the film-forming mold can have a great impact on the peeling of a film, and molds that are easy to peel off films are teflon, glass plates and so on (67). In addition, the drying temperature and the environment humidity affect the film thickness. Specifically, the thickness and elongation at break of the film decreased with an increase in drying temperature (68). It has also been proven that the tensile strength and water vapor permeability of the film increased with increasing ambient humidity (69). Furthermore, the film prepared with high concentration of carrageenan film-forming solutions has been found to possess a poorly sponge structure and large pores, which will be detrimental to the subsequent utilization of the film (70). It may be due to the delayed delamination during film casting. In summary, this method possesses super characteristic of low cost, simple equipment and easy operation, but it require a long drying time, which is not conducive to its application in large-scale commercial production.
Electrospinning is a non-mechanical technology that makes polymer materials into nanoscale fibers. It can generate a high-voltage electrostatic field that is used to change the surface of a polymer solution droplet and apply an electrical potential between the polymer solution droplet and the collector at the end of the capillary (71, 72). When the applied electric field force is sufficient to overcome the surface tension of the droplet, the spinneret will eject a jet of charged polymer solution. During the spraying process, the solution jet is stretched by force, the solvent gradually evaporates, and then a partially or fully solidified electrospun fiber is obtained (72, 73). The final prepared electrospun fibers possess ultra-fine structure, high porosity, high surface-to-volume ratio, and tailored morphology. Also, they can be used to encapsulate bioactive ingredients to improve antimicrobial properties, oxidation resistance (74, 75), and thermal stability (76) of films. For instance, zein, carrageenan, ZnO nanoparticles and rosemary essential oil were used to form maize protein/κ-carrageenan electrospun fibers by electrostatic spinning, and the developed fibers exhibited better surface hydrophobicity, high antibacterial, as well as antioxidant properties (73). Owing to its simple operation and low cost, electrospinning is one of the most effective methods for preparing one-dimensional materials. However, the weak interaction between electrospun fibers endows the films with poor mechanical properties.
Owing to fast, high efficiency, high energy utilization rate, and suitable for large-scale production, the extrusion method is commonly used for the production of packaging materials. The material is extruded by a twin-screw, passing through a desired shaped die to fabricate films under certain parameter settings (77). Unlike petrochemical materials, biopolymers are more sensitive to processing parameters such as screw speed, temperature, feed rate and screw configuration (78). It has been demonstrated that high extrusion temperature and unsuitable pH will break the polypeptide chain of the protein and affect the protein charge distribution (79). Also, small changes in extrusion processing parameters will have a considerable impact on film properties. In summary, complex parameter settings have been a challenge for the production of biopolymers-based films by extrusion. At present, the extrusion method has been used to prepare some biopolymers-based films, such as pectin-based and gelatin-based films (78, 80). Studies have shown that uniform biopolymers-based films can be produced by precisely controlling extrusion speed, barrel temperature and pH (81). However, the preparation of the carrageenan films by the extrusion method have rarely been investigated, and only related reports on the preparation of carrageenan hydrogels and particles by the extrusion method (82, 83). Therefore, further attempts requires to be made to fabricate the carrageenan film using the extrusion method to increase commercial viability of the production of carrageenan-based packaging films.
Carrageenan is regarded as a promising biomaterial for fabricating food packaging films, due to its unique mechanical behavior, water vapor barrier, surface hydrophobicity, light protection and thermal properties. In this section, it focused on the outstanding properties of carrageenan.
The mechanical behavior of the film is usually characterized by tensile strength and elongation at break (84). The measurement process is as follows: the film sample is cut into small pieces and then subjected to a tensile test to obtain a stress-strain curve (85). After analyzing the trend of the curve, the average value of each measured characteristic can be obtained (86).
As shown in Table 1, the tensile strength (TS) and elongation at break (EAB) of the pure ι-carrageenan film were 2.5 MPa and 1.04%, respectively (85). In comparison with the pure ι-carrageenan film, due to a low content of sulfate and negative charge, the pure κ-carrageenan film exhibited better mechanical properties, with a TS of 42.5 MPa and an EAB of 3.9%, respectively. However, the neat structure of κ-carrageenan made the pure κ-carrageenan film brittle (99). To overcome the limit of κ-carrageenan, ι-carrageenan was mixed with κ-carrageenan to fabricate the mixed carrageenan film and the TS of the mixed film reached 55.2 MPa (87). It could be explained that anhydro-bridges were formed during the mixing process, which reduced the hydrophilicity of the sugar residues, leading to a conformational shift in carrageenan and enhancing its gelation properties (64).
The incorporation of other components into the carrageenan matrix resulted in changes in crosslinking properties of carrageenan, thus affecting the mechanical properties of the carrageenan-based film (84). For example, it has been demonstrated that with the addition of TiO2 nanoparticles increasing from 0 wt to 5 wt%, the tensile strength and modulus of the composite films significantly increased from 39 to 122% (100). It may be explained by that the addition of TiO2 nanoparticles can strengthen the physical interaction between the filler and the carrageenan matrix. Better still, sodium benzoate solution was coated onto the film surface as a photosensitizer and then the film was exposed to the UV light for a photo-crosslinking. The reactive benzoate radicals generated by UV radiation reacted with the tertiary hydrogen atoms of the semi-refined carrageenan polymer, forming polymeric radicals, which promoted the crosslinking of the carrageenan polymer chains. The TS of the resulting film was increased by 36–55%, and the density and water barrier properties of the resulting film were also enhanced (101). However, when the addition of nanomaterials is excessively large or the UV exposure time is excessively long, the resulting film will not exhibit excellent mechanical properties, due to the crystallization of nanoparticles into clusters and the pores and microcracks caused by high level photodegradation (100, 102).
In recent years, due to the increasing environmental issues in the world, natural biodegradable ingredients have also been incorporated into the carrageenan matrix. For instance, the pearl millet starch was mixed with carrageenan to form the composite film with excellent mechanical properties (TS of 28 MPa) (103). It may be ascribed to the formation of hydrogen bonding between the starch and carrageenan molecules, resulting in increasing intermolecular interactions (103). Furthermore, the addition of edible maize protein increased the elongation at break of the film from 10.85 to 12.97% (65). In summary, most additives are mixed with carrageenan through physical interactions, and the mechanical properties of carrageenan films are affected by additives (types and amounts). Therefore, natural additives, such as starch, animal and vegetable proteins, are suitable choices due to the widespread presence of hydroxyl groups in their molecular chains and excellent compatibility with carrageenan matrix.
It is noteworthy that plasticizers also affect the mechanical properties of composite films. The addition of plasticizers increases the fluidity of the film-forming matrix by distorting the hydrogen bonds between adjacent polymer chains. However, the addition of plasticizers at high concentration could increase the intermolecular spacing and reduce the tensile strength of the film (103).
The water vapor barrier of a film is affected by several factors, such as the integrity of the film, the hydrophilic-hydrophobic ratio of the film components, the molecular density of the film and the fluidity of the polymer chains (84). Carrageenan exhibits poor water resistance, which limits its application (104). Therefore, the addition of other ingredients is needed to improve the water vapor permeability of composite films. For example, the cross-linked network formed by zein and carrageenan allows water molecules to pass through a twisted path, thus stopping water vapor outside the food. It has been demonstrated that the addition of zein/carrageenan complex can improve the water vapor permeability of the gelatin film, decreasing from 5.2 ± 0.2 × 10−10 g mm/h mm2 Pa (the pure gelatin film) to 1.2 ± 0.1 × 10−10 g mm/h mm2 Pa (the zein/carrageenan/gelatin film) (105). In addition, the hydrogen bonding interaction between the hydroxyl groups on the benzene ring of curcumin and carrageenan made the curcumin evenly distributed in the carrageenan matrix, thus prolonging the permeation path of water vapor, and reducing the water vapor permeability of the film from 2.08 × 10−10 g mm −2 s−1 atm −1 to 0.98 × 10−10 g mm −2 s−1 atm −1 (98).
The hydrophobic surface protects the film from moisture and facilitates the removal of impurities by water. However, due to its hydrophilicity, carrageenan film exhibits poor moisture-proof effect, which limits its application in food preservation (92). Therefore, the interest in the incorporation of other components into the carrageenan matrix for enhancing hydrophobicity of the films has been growing. For example, the high pressure treatment broke the intermolecular bonds in the starch molecules, allowing hydrogen bonds to form between the starch and carrageenan. Therefore, the pressure-treated starch was dispersed into the carrageenan matrix, forming a compatible system of carrageenan and modified starch with enhanced surface hydrophobicity and tensile strength (66). The addition of fatty acids also had a positive effect on surface hydrophobicity. Compared to the control films without fatty acids, polymer-lipid-carrageenan composite films showed better surface hydrophobicity, due to the generation of strong stabilizing network linked by covalent and non-covalent bonds (93). Proteins were used to mix with κ-carrageenan, and exposed its internal hydrophobic groups, thus improving the hydrophobic properties of the composite films (99). In addition, some small molecules, such as arginine laurate, salt ions can improve the hydrophobicity of carrageenan films (106). The surface activity of the hydrophilic chain of carrageenan was successfully enhanced by the in situ interaction of the polymer with the surfactant arginine laurate. Furthermore, a unidirectionally permeable film was prepared, including an inner layer (a carrageenan film) and an outer layer (a hydrophobic film) (Figure 5), which is beneficial to improve the hydrophobicity of the composite film (65).
The light barrier properties of carrageenan films are vital for food packaging applications. There are two properties of the film to achieve light protection for food, including color and opacity. The color of the carrageenan composite films is measured using a colorimeter and evaluated by the L, a and b values (112). L is for lightness and darkness, ranging from 0 to 100. As for a and b values, they range between−128 and + 127. Specifically, a value represents red (+127)/green (-128), and b value is yellow (+127) /blue (-128). The pure carrageenan film is nearly colorless and transparent, and researchers often enhance the reflection of light by increasing the whiteness of the film to protect food. For example, due to the aggregation and mutual cross-linking of white fibers, the addition of soybean dietary fibers improved the whiteness of the carrageenan film, thus protecting food (107). The addition of colored substances endows the film with color, thus protecting food. For instance, the addition of phenolic extracts (from germinating fenugreek seeds) changed the color of the carrageenan composite films. Specifically, the L value of the film decreased from 91.51 to 66.87, and the color became significantly darker, which can enhance the UV-resistance of the films (108, 109). Curcumin and anthocyanin are often added into carrageenan composite films, which can improve the light barrier properties of the composite films. For example, with the curcumin content of the carrageenan film increasing from 0 to 7%, a value of the film showed an increase from−1.08 to + 21.88, b value of the film increasing from +4.82 to +65.29, L value of the film decreasing from 96.16 to 61.88. The color of the film darkened and deepened in yellow (98). Furthermore, since the color of curcumin and anthocyanin changes with pH, the pigment-loaded carrageenan composite film can be used as an intelligent colorimetric indicator film (110, 111) (Figure 6).
Figure 6. Schematic diagram of the application of the anthocyanin-carrageenan film for monitoring fish freshness.
The opacity of the film can affect food protection of the film. The addition of nanoparticles made the film cloudy, increased the opacity of the film, and prevent light from passing through (112). Similarly, the incorporation of oil droplets into the film can cause light scattering, and the opacity of the film was affected by many factors, such as the content of the oil droplet, the size of the oil droplet, and the light scattering intensity (113). For instance, the light transmittance of the carrageenan film (containing camellia oil) at 350 nm was lower than 20%, while that of the pure carrageenan film was about 60%. It indicated that the addition of camellia oil can enhance UV-resistance and light-resistance of the films (94).
The thermal properties of carrageenan films determine the ability of the film to adapt to the environment temperature. Thermal properties are usually determined using thermogravimetric analysis and differential scanning calorimetry (90, 114). Thermogravimetric analysis involves weighing the film into a crucible, scanning it at an appropriate rate over a range of temperatures, and plotting the mass loss of the sample as a function of temperature (90). Differential scanning calorimetry is plotted as a function of heat flow rate and temperature (115).
In the film-forming process, the addition of the plasticizer causes the carrageenan chains to be separated and the intermolecular interaction forces to be reduced, thus affecting the thermal property of the film. For example, the glass transition temperature of the carrageenan films (with plasticizers) dropped below 30 °C, while the glass transition temperature of pure κ-carrageenan was 84.72 °C (35). Therefore, the selection of the plasticizer is critical for thermal properties of the film. Glycerol and sorbitol are commonly used as plasticizers in the film-forming process and have different effect on the thermal performance of the film. It was found that the melting temperature of the film decreased sharply with increasing glycerin concentration, while the melting temperature of the film did not decrease as sharply with increasing sorbitol concentration (35). Moreover, the film (with glycerin) still exhibited good stability at around 200 °C, which can meet the needs of most food packaging (35, 116).
In order to endow carrageenan films with a wide range of applications, the incorporation of substances that enhance the internal structural stability of the carrageenan, can improve thermal properties of the composite film. Transglutaminase, an enzyme that occurring almost all organisms, can significantly enhance the double helix structure of carrageenan through crosslinking. As the concentration of transglutaminase increased, the melting temperature of the film increased from 49.7 °C to above 80 °C (117). In addition, the incorporation of the natural peptide ε-polylysine can exhibit excellent compatibility with carrageenan and promote the formation of the ordered and tightly arranged network structure in the carrageenan gel, thus improving the thermal stability of the carrageenan film. It was proven that the decomposition temperature of the film (with ε-polylysine) was much higher than that of the pure carrageenan film (118).
Fruits and vegetables are rich in vitamins and minerals, which play an important role in human health. However, they are susceptible to spoilage. Approximately 20% of fruits and vegetables are discarded each year in developed countries, thus leading to huge economic losses (119). Generally, main factors affecting the spoilage of fruits and vegetables are water content and respiration rate. It has been proven that an extremely high water content (about 75–95%) and high respiration rate endowed fruits and vegetables susceptible to bacteria and fungi, therefore resulting in spoilage (120). So, it is necessary to develop preservation methods for extending the shelf life of fruits and vegetables.
The carrageenan film has excellent mechanical and protective properties, therefore it is favored in the preservation packaging of fresh fruits and vegetables (Table 2). The mechanical properties of the carrageenan film allow the film to maintain intact during the transport and distribution of fruits and vegetables, thus avoiding mechanical damage to the products (123). Furthermore, the addition of oxygen and ethylene scavengers into the carrageenan film can slow down the respiration rate of fruits and vegetables and inhibit the growth of aerobic bacteria (125). Although carrageenan exhibits a weak water vapor barrier (104), the addition of other components could effectively reduce the water vapor permeability of the film, thus preventing the loss of internal moisture in fruits and vegetables through pores and cracks on the surface (123).
It has demonstrated that carrageenan-zinc oxide nanocomposite coatings (Figure 7) can reduce physical and biological damage to mangoes (122). On the 19th day, coated mangoes still retained initial firmness, a slight discoloration and decay occurred in the coated mangoes on the 33rd day. The shelf life of coated mangoes was extended to 14 days, compared with uncoated mangoes (122). Because the addition of zinc oxide nanoparticles allowed the composite coating to significantly increase the tensile strength by 43%, reduce the water vapor transmission by 9%, and inhibit the growth of E. coli, compared with that of pure carrageenan coatings (122). Compared with the mango, strawberry is more prone to spoilage. Therefore, it is imperative to develop a method for strawberry preservation. The carrageenan coating has been used for strawberry packaging, and exhibited the lowest total soluble solids (9.50%) on the 12th day of storage, much lower than the control group (12.20%) (62). The rot rate of strawberries was reduced by 14.29% since the carrageenan film inhibited the metabolic activity and reduced water loss (62).
Figure 7. Schematic diagram of the application of the carrageenan-ZnO nanocomposite coating for mango preservation.
Carrageenan film can play a similar role during vegetable storage. Tomatoes coated with a naturally biodegradable edible film (arrowroot starch and ι-carrageenan) have been demonstrated to be more firm than unwrapped tomatoes (57). Konjac glucomannan-SiO2-carrageenan composite nano films can also reduce the weight loss and oxygen permeability of white mushrooms, thereby slowing down the respiration of mushrooms and reducing their browning index, extending the shelf life of mushrooms stored at 4 °C by 5-12 days (124).
Fish and meat products are favored for their high protein and unsaturated fatty acid content (65). However, fish and meat products are prone to spoilage. The spoilage of fish and meat products is affected by microbial contamination, fat oxidation, protein degradation and endogenous enzymes (126). In addition, environmental factors such as temperature, oxygen, moisture and light, can easily cause changes in the color, smell, texture and flavor of fish and meat products, which are difficult to detect with the naked eyes (127, 128). Low temperature preservation is commonly used for preservation of fish and meat products, including refrigeration and freezing. However, frozen fish and meat products do not taste good, and cold fish and meat products are more popular. Therefore, in order to extend the shelf life of the cold fish and meat products, the development of carrageenan-based composite films has received tremendous attention. For example, compared with unpackaged beef, the application of copper sulfide nanoparticle-carrageenan films to beef can reduce effectively the amounts of Escherichia coli and Staphylococcus aureus by 52.6% and 69.8%, respectively (129). Similarly, it was found that the use of carrageenan/camellia oil films for chicken preservation reduced the amounts of cryophilic bacteria (3.86 Log CFU/g) sharply, compared with that of the control group (5.35 Log CFU/g) (130). In addition, due to the antioxidant and antibacterial activities of curcumin, the carrageenan/curcumin films can inhibit the growth of Staphylococcus aureus and Serratia marcescens, thus being applied for shrimp packaging (98).
Spoilage of fish and meat products is often accompanied by the production of nitrogenous substances such as ammonia, dimethylammonium and trimethylamine, which may alter pH (98). Therefore, the incorporation of pH-sensitive substances into carrageenan matrix allows the composite films to monitor the freshness of fish and meat products. For example, the curcumin-loaded carrageenan film has been developed for monitoring pork freshness, since the phenolic hydroxyl groups of curcumin readily reacted with OH- to form phenolate anions (98). It was found that the color of the film changed from yellow to red, as the TVBN value of the pork increased from 4.91 to 31.11 mg/100 g (108). The κ-carrageenan/anthocyanins films can also be used as an indicator film for monitoring pork freshness. Studies have demonstrated that the color of the indicator film turned from purple to green, with the TVBN value increasing from 8.23 to 14.63 mg/100 g (131). In lard packaging, the color of the carrageenan/carboxymethylcellulose/plum sap composite film changed from deep red to blue-gray, with the pH increasing from 3 to 13 (132). The natural dye-loaded carrageenan composite films are also applied for fish (112) and shrimp (98) preservation.
Oxidation and microorganisms are important factors affecting the spoilage of dairy products (121). Currently, carrageenan-based composite films have been gradually popularized in the application of dairy product preservation. Studies have shown that the addition of mulberry polyphenolic extract into carrageenan matrix can be applied for monitoring milk freshness (96, 133). It was found that the color of the carrageenan composite film changed from gray, purple to dark pink, when the film was covered on milk stored at 40 °C for 0–6 h (96, 133). Because the lactic acid produced by microbial metabolism increased, the acidity increased, the pH decreased, and thus leading to the color change of the composite film (96).
Furthermore, due to whipping, the oxidation of lipids and the reproduction of microorganisms are accelerated during the production of ice cream, it is imperative to fabricate carrageenan-based composite films for ice cream preservation (134). The aloe vera gel/carrageenan composite film was found to inhibit the reproduction of various microbial pathogens such as Staphylococcus aureus, Escherichia coli, Streptococcus agalactiae, and Klebsiella pneumoniae in ice cream (135). The carrageenan-based composite film has also been used in cheese preservation. It was proven that the carrageenan-black bean extract film exhibited excellent antioxidant, mechanical and water barrier properties and was coated on the cheese. Results showed that the peroxide value of the coated sample after 20 days storage was 1.25 mEq O2 /Kg, while that of the uncoated cheese sample was 4.2 mEq O2 /Kg (136, 137).
An increasing attention in carrageenan-based films for food packaging has been drawn, due to abundant resource, biodegradability and excellent compatibility of carrageenan. This review provided an overview of the carrageenan (source, extraction method, and property), film-forming methods (direct coating, solvent casting and electrospinning), the property of the carrageenan-based composite film and applications. We focused on the driving forces for the formation of carrageenan-based films and how to enhance the properties of carrageenan-based films. In summary, most of researches focused on the applications of carrageenan-based films in the laboratory, further studies concentrating on industrial scale productions requires to be done. Moreover, the future trend of carrageenan-based films for food packaging applications is to fabricate excellent multifunctional films, the interaction mechanism of carrageenan with other components therefore requires further discussed.
CC and SC contributed to the writing. MZhu, MZhou, and TC reviewed and revised the manuscript. YH designed the study and wrote the manuscript. All authors contributed to the article and approved the submitted version.
This work was supported by Hubei Provincial Natural Science Foundation of China (2020CFB314), China Postdoctoral Science Foundation (2019M662659), the Postdoctoral Innovative Research Position of Hubei Province, the Fundamental Research Funds for the Central Universities (2042021kf0042), the fund of the Beijing Engineering and Technology Research Center of Food Additives, Beijing Technology; Business University (BTBU), China Postdoctoral Science Foundation (2022M712473), the Fundamental Research Funds for the Central Universities (11041910103), and the Students Research Fund (2022264).
The authors declare that the research was conducted in the absence of any commercial or financial relationships that could be construed as a potential conflict of interest.
All claims expressed in this article are solely those of the authors and do not necessarily represent those of their affiliated organizations, or those of the publisher, the editors and the reviewers. Any product that may be evaluated in this article, or claim that may be made by its manufacturer, is not guaranteed or endorsed by the publisher.
1. Kan M, Miller SA. Environmental impacts of plastic packaging of food products. Resour, Conserv Recycl. (2022) 180:106156–66. doi: 10.1016/j.resconrec.2022.106156
2. An L, Hu X, Perkins P, Ren T. A sustainable and antimicrobial food packaging film for potential application in fresh produce packaging. Front Nutr. (2022) 9:924304–13. doi: 10.3389/fnut.2022.924304
3. Tas CE, Unal H. Thermally buffering polyethylene/halloysite/phase change material nanocomposite packaging films for cold storage of foods. J Food Eng. (2021) 292:110351–58. doi: 10.1016/j.jfoodeng.2020.110351
4. Vera P, Canellas E, Nerín C. Compounds responsible for off-odors in several samples composed by polypropylene, polyethylene, paper and cardboard used as food packaging materials. Food Chem. (2020) 309:125792–00. doi: 10.1016/j.foodchem.2019.125792
5. Tran TH, Hao LT, Jeon H, Koo JM, Shin G, et al. Biorenewable, transparent, and oxygen/moisture barrier nanocellulose/nanochitin-based coating on polypropylene for food packaging applications. Carbohydr Polym. (2021) 271:118421–32. doi: 10.1016/j.carbpol.2021.118421
6. Khalaj M, Ahmadi H, Lesankhosh R, Khalaj G. Study of physical and mechanical properties of polypropylene nanocomposites for food packaging application: nano-clay modified with iron nanoparticles. Trends Food Sci Technol. (2016) 51:41–8. doi: 10.1016/j.tifs.2016.03.007
7. Pilevar Z, Bahrami A, Beikzadeh S, Hosseini H, Jafari SM. Migration of styrene monomer from polystyrene packaging materials into foods: Characterization and safety evaluation. Trends Food Sci Technol. (2019) 91:248–61. doi: 10.1016/j.tifs.2019.07.020
8. Mostafavi FS, Zaeim D. Agar-based edible films for food packaging applications-A review. Int J Biol Macromol. (2020) 159:1165–76. doi: 10.1016/j.ijbiomac.2020.05.123
9. Hitchcock JN. Microplastics can alter phytoplankton community composition. Sci Total Environ. (2022) 819:153074–80. doi: 10.1016/j.scitotenv.2022.153074
10. Molina E, Benedé S. Is there evidence of health risks from exposure to micro- and nanoplastics in foods? Front Nutr. (2022) 9:910094–01. doi: 10.3389/fnut.2022.910094
11. Manzoor MF, Hussain A, Naumovski N, Ranjha MMAN, Ahmad N, Karrar E, et al. A narrative review of recent advances in rapid assessment of anthocyanins in agricultural and food products. Front Nutr. (2022) 9:901342–55. doi: 10.3389/fnut.2022.901342
12. Islam MS, Islam MM, Islam KN, Sobuz MHR. Biodegradable and bio-based environmentally friendly polymers. Mater. Sci. Eng.. (2022) 2:820–36. doi: 10.1016/B978-0-12-820352-1.00271-6
13. Sharanagat VS, Mor RS, Kumar K. Active and intelligent biodegradable packaging films using food and food waste-derived bioactive compounds: a review Trends. Food Sci Technol. (2020) 105:385–01. doi: 10.1016/j.tifs.2020.09.015
14. Wang B, Yan S, Qiu L, Gao W, Kang X, Yu B, et al. Antimicrobial activity, microstructure, mechanical, and barrier properties of cassava starch composite films supplemented with geranium essential oil. Front Nutr. (2022) 9:882742–49. doi: 10.3389/fnut.2022.882742
15. Zhu F. Polysaccharide based films and coatings for food packaging: effect of added polyphenols. Food Chem. (2021) 359:129871–91. doi: 10.1016/j.foodchem.2021.129871
16. Yao X, Liu J, Hu H, Yun D, Liu J. Development and comparison of different polysaccharide/PVA-based active/intelligent packaging films containing red pitaya betacyanins. Food Hydrocol. (2022) 124:107305–15. doi: 10.1016/j.foodhyd.2021.107305
17. Nobili MD, Bernhardt DC. Basanta MF, Rojas AM. Sunflower (Helianthus annuus L) seed hull waste: composition, antioxidant activity, and filler performance in pectin-based film composites. Front Nutr. (2021) 8:777214–27. doi: 10.3389/fnut.2021.777214
18. Wang C, Gong C, Qin Y, Hu Y, Jiao A, Jin Z, et al. Bioactive and functional biodegradable packaging films reinforced with nanoparticles. J Food Eng. (2022) 312:110752–65. doi: 10.1016/j.jfoodeng.2021.110752
19. Monção ÉDC, Grisi CVB, de Moura Fernandes J, Souza PS, de Souza AL. Active packaging for lipid foods and development challenges for marketing. Food Biosci. (2022) 45:101370–83. doi: 10.1016/j.fbio.2021.101370
20. McDaniel A, Tonyali B, Yucel U, Trinetta V. Formulation and development of lipid nanoparticle antifungal packaging films to control postharvest disease. J Agri Food Res. (2019) 1:100013–19. doi: 10.1016/j.jafr.2019.100013
21. Hadidi M, Jafarzadeh S, Forough M, Garavand F, Alizadeh S, Salehabadi A, et al. Plant protein-based food packaging films; recent advances in fabrication, characterization, and applications. Trends Food Sci Technol. (2022) 120:154–73. doi: 10.1016/j.tifs.2022.01.013
22. Azaza YB, Hamdi M, Charmette C, Jridi M, Li S, Nasri M, et al. Development and characterization of active packaging films based on chitosan and sardinella protein isolate: effects on the quality and the shelf life of shrimps. Food Packag Shelf Life. (2022) 31:100796–06. doi: 10.1016/j.fpsl.2021.100796
23. Lu J, Li T, Ma L, Li S, Jiang W, Qin W, et al. Optimization of heat-sealing properties for antimicrobial soybean protein isolate film incorporating diatomite/thymol complex and its application on blueberry packaging. Food Packag Shelf Life. (2021) 29:100690–99. doi: 10.1016/j.fpsl.2021.100690
24. Zhang R, Han Y, Xie W, Liu F, Chen S. Advances in protein-based nanocarriers of bioactive compounds: From microscopic molecular principles to macroscopical structural and functional attributes. J Agri Food Chem. (2022) 70:6354–67. doi: 10.1021/acs.jafc.2c01936
25. Crepaldi MI, Junior ODOS, de Oliveira AC, Martins AF, Garcia PS, et al. Biodegradable films based on commercial κ-carrageenan and cassava starch to achieve low production costs. Inter J Biol Macromol. (2020) 165:582–90. doi: 10.1016/j.ijbiomac.2020.09.150
26. Zia KM, Tabasum S, Nasif M, Sultan N, Aslam N, Noreen A, et al. A review on synthesis, properties and applications of natural polymer based carrageenan blends and composites. Inter J Biol Macromol. (2017) 96:282–01. doi: 10.1016/j.ijbiomac.2016.11.095
27. Jiang J, Zhang W, Ni W, Shao J. Insight on structure-property relationships of carrageenan from marine red algal: a review. Carbohydr Polym. (2021) 257:117642–53. doi: 10.1016/j.carbpol.2021.117642
28. Aga MB, Dar AH, Nayik GA, Panesar PS, Allai F, Khan SA, et al. Recent insights into carrageenan-based bio-nanocomposite polymers in food applications: a review. Inter J Biol Macromol. (2021) 192:197–209. doi: 10.1016/j.ijbiomac.2021.09.212
29. Nouri A, Tavakkoli Yaraki M, Lajevardi A, Rahimi T, Tanzifi M, Ghorbanpour M. An investigation of the role of fabrication process in the physicochemical properties of κ-carrageenan-based films incorporated with Zataria multiflora extract and nanoclay. Food Packag Shelf Life. (2020) 23:100435–43. doi: 10.1016/j.fpsl.2019.100435
30. Sedayu BB, Cran MJ. Bigger SW. A review of property enhancement techniques for carrageenan-based films and coatings. Carbohydr Polym. (2019) 216:287–02. doi: 10.1016/j.carbpol.2019.04.021
31. Eha K, Pehk T, Heinmaa I, Kaleda A, Laos K. Impact of short-term heat treatment on the structure and functional properties of commercial furcellaran compared to commercial carrageenans. Heliyon. (2021) 7:e6640–48. doi: 10.1016/j.heliyon.2021.e06640
32. ion i. purification, structure, biological activities and applications. Algal Res. (2022) 61:102593–06. doi: 10.1016/j.algal.2021.102593
33. Nguyen BT, Nicolai T, Benyahia L, Chassenieux C. Synergistic effects of mixed salt on the gelation of κ-carrageenan. Carbohydr Polym. (2014) 112:10–5. doi: 10.1016/j.carbpol.2014.05.048
34. Dong Y, Wei Z, Xue C. Recent advances in carrageenan-based delivery systems for bioactive ingredients: a review. Trends Food Sci Technol. (2021) 112:348–61. doi: 10.1016/j.tifs.2021.04.012
35. Ili Balqis AM, Nor Khaizura MAR. Russly AR, Nur Hanani ZA. Effects of plasticizers on the physicochemical properties of kappa-carrageenan films extracted from Eucheuma cottonii. Inter J Biol Macromol. (2017) 103:721–32. doi: 10.1016/j.ijbiomac.2017.05.105
36. Torres MD, Chenlo F, Moreira R. Thermal reversibility of kappa/iota-hybrid carrageenan gels extracted from Mastocarpus stellatus at different ionic strengths. J Taiwan Inst Chem Eng. (2017) 71:414–20. doi: 10.1016/j.jtice.2016.11.028
37. Rafiquzzaman SM, Ahmed R, Lee JM, Noh G, Jo G, Kong I. Improved methods for isolation of carrageenan from Hypnea musciformis and its antioxidant activity. J Appl Phycol. (2016) 28:1265–74. doi: 10.1007/s10811-015-0605-6
38. Youssouf L, Lallemand L, Giraud P, Soulé F, Bhaw-Luximon A, Meilhac O, et al. Ultrasound-assisted extraction and structural characterization by NMR of alginates and carrageenans from seaweeds. Carbohydr Polym. (2017) 166:55–63. doi: 10.1016/j.carbpol.2017.01.041
39. Nie J, Chen D, Ye J, Lu Y, Dai Z. Optimization and kinetic modeling of ultrasonic-assisted extraction of fucoxanthin from edible brown algae Sargassum fusiforme using green solvents. Ultrason Sonochem. (2021) 77:105671–78. doi: 10.1016/j.ultsonch.2021.105671
40. Sharayei P, Azarpazhooh E, Zomorodi S, Einafshar S, Ramaswamy HS. Optimization of ultrasonic-assisted extraction of astaxanthin from green tiger (Penaeus semisulcatus) shrimp shell. Ultrason Sonochem. (2021) 76:105666–74. doi: 10.1016/j.ultsonch.2021.105666
41. Zhang R, Han Y, McClements DJ, Xu D, Chen S. Production, characterization, delivery, and cholesterol-lowering mechanism of phytosterols: a review. J Agri Food Chem. (2022) 70:2483–94. doi: 10.1021/acs.jafc.1c07390
42. Vázquez-Delfín E, Robledo D, Freile-Pelegrín Y. Microwave-assisted extraction of the Carrageenan from Hypnea musciformis (cystocloniaceae, rhodophyta). J Appl Phycol. (2014) 26:901–07. doi: 10.1007/s10811-013-0090-8
43. Boulho R, Marty C, Freile-Pelegrín Y, Robledo D, Bourgougnon N, Bedoux G. Antiherpetic (HSV-1) activity of carrageenans from the red seaweed Solieria chordalis (Rhodophyta, Gigartinales) extracted by microwave-assisted extraction (MAE). J Appl Phycol. (2017) 29:2219–28. doi: 10.1007/s10811-017-1192-5
44. Yuguchi Y, Thu Thuy TT, Urakawa H, Kajiwara K. Structural characteristics of carrageenan gels: temperature and concentration dependence. Food Hydrocol. (2002) 16:515–22. doi: 10.1016/S0268-005X(01)00131-X
45. Tao H, Guo L, Qin Z, Yu B, Wang Y, Li J, et al. Textural characteristics of mixed gels improved by structural recombination and the formation of hydrogen bonds between curdlan and carrageenan. Food Hydrocol. (2022) 129:107678–85. doi: 10.1016/j.foodhyd.2022.107678
46. Elfaruk MS, Wen C, Chi C, Li X, Janaswamy S. Effect of salt addition on iota-carrageenan solution properties. Food Hydrocol. (2021) 113:106491–97. doi: 10.1016/j.foodhyd.2020.106491
47. Yuguchi Y, Urakawa H, Kajiwara K. Structural characteristics of carrageenan gels: various types of counter ions. Food Hydrocol. (2003) 17:481–85. doi: 10.1016/S0268-005X(03)00021-3
48. Iijima M, Hatakeyama T, Takahashi M, Hatakeyama H. Effect of thermal history on kappa-carrageenan hydrogelation by differential scanning calorimetry. Thermochim Acta. (2007) 452:53–8. doi: 10.1016/j.tca.2006.10.019
49. de Alcântara MG, de Freitas Ortega N, Souza CJF, Garcia-Rojas EE. Electrostatic hydrogels formed by gelatin and carrageenan induced by acidification: rheological and structural characterization. Food Struct. (2020) 24:100137–46. doi: 10.1016/j.foostr.2020.100137
50. Liu Y, Zhao K, Ma Q. Insight into the glucose cosolvent induced gelation of κ-carrageenan by broadband dielectric spectroscopy. J Mol Liq. (2020) 318:114268–75. doi: 10.1016/j.molliq.2020.114268
51. Huang M, Mao Y, Li H, Yang H. Kappa-carrageenan enhances the gelation and structural changes of egg yolk via electrostatic interactions with yolk protein. Food Chem. (2021) 360:129972–80. doi: 10.1016/j.foodchem.2021.129972
52. Wang L, Cao Y, Zhang K, Fang Y, Nishinari K, Phillips GO. Hydrogen bonding enhances the electrostatic complex coacervation between κ-carrageenan and gelatin. Colloids Surf A: Physicochem Eng Asp. (2015) 482:604–10. doi: 10.1016/j.colsurfa.2015.07.011
53. Fabra MJ, Talens P, Chiralt A. Effect of alginate and λ-carrageenan on tensile properties and water vapour permeability of sodium caseinate–lipid based films. Carbohydr Polym. (2008) 74:419–26. doi: 10.1016/j.carbpol.2008.03.010
54. Yang Z, Yang H, Yang H. Effects of sucrose addition on the rheology and microstructure of κ-carrageenan gel. Food Hydrocol. (2018) 75:164–73. doi: 10.1016/j.foodhyd.2017.08.032
55. Stenner R, Matubayasi N, Shimizu S. Gelation of carrageenan: effects of sugars and polyols. Food Hydrocol. (2016) 54:284–92. doi: 10.1016/j.foodhyd.2015.10.007
56. Zhao J, Sun C, Li H, Dong X, Zhang X. Studies on the physicochemical properties, gelling behavior and drug release performance of agar/κ-carrageenan mixed hydrogels. Int J Biol Macromol. (2020) 154:878–87. doi: 10.1016/j.ijbiomac.2020.03.087
57. Abdillah AA, Charles AL. Characterization of a natural biodegradable edible film obtained from arrowroot starch and iota-carrageenan and application in food packaging. Int J Biol Macromol. (2021) 191:618–26. doi: 10.1016/j.ijbiomac.2021.09.141
58. Zhang X, Ismail BB, Cheng H, Jin TZ, Qian M, Arabi SA, et al. Emerging chitosan-essential oil films and coatings for food preservation-a review of advances and applications. Carbohydr Polym. (2021) 273:118616–31. doi: 10.1016/j.carbpol.2021.118616
59. Qu B, Luo Y. A review on the preparation and characterization of chitosan-clay nanocomposite films and coatings for food packaging applications. Carbohydr Polym Technol Appl. (2021) 2:100102–11. doi: 10.1016/j.carpta.2021.100102
60. Muxika A, Etxabide A, Uranga J, Guerrero P. de la Caba K. Chitosan as a bioactive polymer: Processing, properties and applications. Int J Biol Macromol. (2017) 105:1358–68. doi: 10.1016/j.ijbiomac.2017.07.087
61. Moller A, Leone C, Kataria J, Sidhu G, Rama EN, Kroft B, et al. Effect of a carrageenan/chitosan coating with allyl isothiocyanate on microbial load in chicken breast. LWT. (2022) 161:113397–02. doi: 10.1016/j.lwt.2022.113397
62. Malik AR, Ganaie TA, Masoodi FA, Gani A, Wani SM, Gull A, et al. Effect of gum Arabic, xanthan and carrageenan coatings containing antimicrobial agent on postharvest quality of strawberry: assessing the physicochemical, enzyme activity and bioactive properties. Int J Biol Macromol. (2021) 183:2100–08. doi: 10.1016/j.ijbiomac.2021.06.008
63. Leceta I, Molinaro S, Guerrero P, Kerry JP, de la Caba K. Quality attributes of map packaged ready-to-eat baby carrots by using chitosan-based coatings Postharvest. Biol Technol. (2015) 100:142–50. doi: 10.1016/j.postharvbio.2014.09.022
64. Tavassoli-Kafrani E, Shekarchizadeh H, Masoudpour-Behabadi M. Development of edible films and coatings from alginates and carrageenans. Carbohydr Polym. (2016) 137:360–74. doi: 10.1016/j.carbpol.2015.10.074
65. He F, Kong Q, Jin Z, Mou H. Developing a unidirectionally permeable edible film based on κ-carrageenan and gelatin for visually detecting the freshness of grass carp fillets. Carbohydr Polym. (2020) 241:116336–47. doi: 10.1016/j.carbpol.2020.116336
66. Shahbazi M, Majzoobi M, Farahnaky A. Physical modification of starch by high-pressure homogenization for improving functional properties of κ-carrageenan/starch blend film. Food Hydrocol. (2018) 85:204–14. doi: 10.1016/j.foodhyd.2018.07.017
67. Saurabh CK, Tye YY, Suriani AB, Mohamed A, Banerjee A, Rizal S, et al. Biodegradable films for fruits and vegetables packaging application: preparation and properties. Food Eng Rev. (2018) 10:139–53. doi: 10.1007/s12393-018-9180-3
68. Bagheri F, Radi M, Amiri S. Drying conditions highly influence the characteristics of glycerol-plasticized alginate films. Food Hydrocol. (2019) 90:162–71. doi: 10.1016/j.foodhyd.2018.12.001
69. Alvarado S, Sandoval G, Palos I, Tellez S, Aguirre-Loredo Y, Velazquez G. The effect of relative humidity on tensile strength and water vapor permeability in chitosan, fish gelatin and transglutaminase edible films. Food Sci Technol. (2015) 35:690–95. doi: 10.1590/1678-457X.6797
70. Alam J, Alhoshan M, Shukla AK, Aldalbahi A, Ali FAA. κ-Carrageenan – A versatile biopolymer for the preparation of a hydrophilic PVDF composite membrane. Eur Polym J. (2019) 120:109219–27. doi: 10.1016/j.eurpolymj.2019.109219
71. Moreno MA, Orqueda ME, Gómez-Mascaraque LG, Isla MI, López-Rubio A. Crosslinked electrospun zein-based food packaging coatings containing bioactive chilto fruit extracts. Food Hydrocol. (2019) 95:496–05. doi: 10.1016/j.foodhyd.2019.05.001
72. Mendes AC, Stephansen K, Chronakis IS. Electrospinning of food proteins and polysaccharides. Food Hydrocol. (2017) 68:53–68. doi: 10.1016/j.foodhyd.2016.10.022
73. Amjadi S, Almasi H, Ghorbani M, Ramazani S. Reinforced ZnONPs/ rosemary essential oil-incorporated zein electrospun nanofibers by κ-carrageenan. Carbohydr Polym. (2020) 232:115800–09. doi: 10.1016/j.carbpol.2019.115800
74. Fabra MJ, Lopez-Rubio A, Lagaron JM. High barrier polyhydroxyalcanoate food packaging film by means of nanostructured electrospun interlayers of zein. Food Hydrocol. (2013) 32:106–14. doi: 10.1016/j.foodhyd.2012.12.007
75. Zhijiang C, Cong Z, Ping X, Yunming Q. Preparation, characterization and antibacterial activity of biodegradable polyindole/bacterial cellulose conductive nanocomposite fiber membrane. Mater Let. (2018) 222:146–49. doi: 10.1016/j.matlet.2018.03.203
76. Aydogdu A, Sumnu G, Sahin S. A novel electrospun hydroxypropyl methylcellulose/polyethylene oxide blend nanofibers: morphology and physicochemical properties. Carbohydr Polym. (2018) 181:234–46. doi: 10.1016/j.carbpol.2017.10.071
77. Prabha K, Ghosh PSA, Joseph RM, Krishnan R, Rana SS, Pradhan RC. Recent development, challenges, and prospects of extrusion technology. Future Foods. (2021) 3:100019–33. doi: 10.1016/j.fufo.2021.100019
78. Nur Hanani ZA, Beatty E, Roos YH, Morris MA, Kerry JP. Manufacture and characterization of gelatin films derived from beef, pork and fish sources using twin screw extrusion. J Food Eng. (2012) 113:606–14. doi: 10.1016/j.jfoodeng.2012.07.002
79. Nur Hanani ZA, O'Mahony JA, Roos YH, Oliveira PM, Kerry JP. Extrusion of gelatin-based composite films: Effects of processing temperature and pH of film forming solution on mechanical and barrier properties of manufactured films. Food Packag Shelf Life. (2014) 2:91–01. doi: 10.1016/j.fpsl.2014.09.001
80. Huang J, Hu Z, Hu L, Li G, Yao Q, Hu Y. Pectin-based active packaging: a critical review on preparation, physical properties and novel application in food preservation. Trends Food Sci Technol. (2021) 118:167–78. doi: 10.1016/j.tifs.2021.09.026
81. Gouveia TIA, Biernacki K, Castro MCR, Gonçalves MP, Souza HKS. A new approach to develop biodegradable films based on thermoplastic pectin. Food Hydrocol. (2019) 97:105175–84. doi: 10.1016/j.foodhyd.2019.105175
82. Gómez-Mascaraque LG, Martínez-Sanz M, Fabra MJ, López-Rubio A. Development of gelatin-coated ι-carrageenan hydrogel capsules by electric field-aided extrusion. Impact of phenolic compounds on their performance. Food Hydrocol. (2019) 90:523–33. doi: 10.1016/j.foodhyd.2018.12.017
83. Jade PB, Sonawane RO, Patil SD, Ige PP, Pardeshi CV. Co-processed κ-carrageenan-pectin as pelletizing aid for immediate-release pellets. Part Sci Technol. (2017) 35:192–00. doi: 10.1080/02726351.2016.1146811
84. Balasubramanian R, Kim SS, Lee J. Novel synergistic transparent κ-Carrageenan/Xanthan gum/Gellan gum hydrogel film: mechanical, thermal and water barrier properties. Int J Biol Macromol. (2018) 118:561–68. doi: 10.1016/j.ijbiomac.2018.06.110
85. Paula GA, Benevides NMB, Cunha AP, de Oliveira AV, Pinto AMB, Morais JPS, et al. Development and characterization of edible films from mixtures of κ-carrageenan, ι-carrageenan, and alginate. Food Hydrocol. (2015) 47:140–45. doi: 10.1016/j.foodhyd.2015.01.004
86. Zhou X, Zong X, Wang S, Yin C, Gao X, Xiong G, et al. Emulsified blend film based on konjac glucomannan/carrageenan/camellia oil: physical, structural, and water barrier properties. Carbohydr Polym. (2021) 251:117100–09. doi: 10.1016/j.carbpol.2020.117100
87. Larotonda FDS, Torres MD, Gonçalves MP, Sereno AM, Hilliou L.. Hybrid carrageenan-based formulations for edible film preparation: Benchmarking with kappa carrageenan. J Appl Polym Sci. (2016) 133:42253–63. doi: 10.1002/app.42263
88. Praseptiangga D, Mufida N, Panatarani C, Joni IM. Enhanced multi functionality of semi-refined iota carrageenan as food packaging material by incorporating SiO2 and ZnO nanoparticles. Heliyon. (2021) 7:e6963–71. doi: 10.1016/j.heliyon.2021.e06963
89. Kassab Z, Aziz F, Hannache H, Ben Youcef H, El Achaby M. Improved mechanical properties of κ-carrageenan-based nanocomposite films reinforced with cellulose nanocrystals. Int J Biol Macromol. (2019) 123:1248–56. doi: 10.1016/j.ijbiomac.2018.12.030
90. Duan N, Li Q, Meng X, Wang Z, Wu S. Preparation and characterization of κ-carrageenan/konjac glucomannan/TiO2 nanocomposite film with efficient anti-fungal activity and its application in strawberry preservation. Food Chem. (2021) 364:130441–49. doi: 10.1016/j.foodchem.2021.130441
91. Rukmanikrishnan B, Rajasekharan SK, Lee J, Ramalingam S, Lee J. κ-Carrageenan/lignin composite films: Biofilm inhibition, antioxidant activity, cytocompatibility, UV and water barrier properties. Mater Today Commun. (2020) 24:101346–55. doi: 10.1016/j.mtcomm.2020.101346
92. Nur Hanani ZA, Aelma Husna AB. Effect of different types and concentrations of emulsifier on the characteristics of kappa-carrageenan films. Int J Biol Macromol. (2018) 114:710–16. doi: 10.1016/j.ijbiomac.2018.03.163
93. Golding JB, Stathopoulos CE, Scarlett CJ, Bowyer M, Singh SP, Vuong QV, et al. Amylose-lipid complex as a measure of variations in physical, mechanical and barrier attributes of rice starch- ι -carrageenan biodegradable edible film. Food Packag Shelf Life. (2017) 14:108–15. doi: 10.1016/j.fpsl.2017.10.002
94. Zhou X, Yu X, Xie F, Fan Y, Xu X, Qi J, et al. pH-responsive double-layer indicator films based on konjac glucomannan/camellia oil and carrageenan/anthocyanin/curcumin for monitoring meat freshness. Food Hydrocol. (2021) 118:106695–05. doi: 10.1016/j.foodhyd.2021.106695
95. Ili Balqis AM, Nur Hanani ZA. Seydim AC. The properties of κ-carrageenan and whey protein isolate blended films containing pomegranate seed oil. Polym Test. (2019) 77:105886–93. doi: 10.1016/j.polymertesting.2019.05.002
96. Liu Y, Qin Y, Bai R, Zhang X, Yuan L, Liu J. Preparation of pH-sensitive and antioxidant packaging films based on κ-carrageenan and mulberry polyphenolic extract. Int J Biol Macromol. (2019) 134:993–01. doi: 10.1016/j.ijbiomac.2019.05.175
97. Sun G, Chi W, Xu S, Wang L. Developing a simultaneously antioxidant and pH-responsive κ-carrageenan/hydroxypropyl methylcellulose film blended with Prunus maackii extract. Int J Biol Macromol. (2020) 155:1393–00. doi: 10.1016/j.ijbiomac.2019.11.114
98. Liu J, Wang H, Wang P, Guo M, Jiang S, Li X, et al. Films based on κ-carrageenan incorporated with curcumin for freshness monitoring. Food Hydrocol. (2018) 83:134–42. doi: 10.1016/j.foodhyd.2018.05.012
99. Huang X, Luo X, Liu L, Dong K, Yang R, Lin C, et al. Formation mechanism of egg white protein/κ-carrageenan composite film and its application to oil packaging. Food Hydrocol. (2020) 105:105780–90. doi: 10.1016/j.foodhyd.2020.105780
100. Balasubramanian R, Kim SS, Lee J, Lee J. Effect of TiO2 on highly elastic, stretchable UV protective nanocomposite films formed by using a combination of κ-carrageenan, xanthan gum and gellan gum. Int J Biol Macromol. (2019) 123:1020–27. doi: 10.1016/j.ijbiomac.2018.11.151
101. Sedayu BB, Cran MJ. Bigger SW. Effects of surface photocrosslinking on the properties of semi-refined carrageenan film. Food Hydrocol. (2021) 111:106196–05. doi: 10.1016/j.foodhyd.2020.106196
102. Shojaee-Aliabadi S, Mohammadifar MA, Hosseini H, Mohammadi A, Ghasemlou M, Hosseini SM, et al. Characterization of nanobiocomposite kappa-carrageenan film with Zataria multiflora essential oil and nanoclay. Int J Biol Macromol. (2014) 69:282–89. doi: 10.1016/j.ijbiomac.2014.05.015
103. Sandhu KS, Sharma L, Kaur M, Kaur R. Physical, structural and thermal properties of composite edible films prepared from pearl millet starch and carrageenan gum: process optimization using response surface methodology. Int J Biol Macromol. (2020) 143:704–13. doi: 10.1016/j.ijbiomac.2019.09.111
104. Varela P, Fiszman SM. Hydrocolloids in fried foods. a review. Food Hydrocol. (2011) 25:1801–12. doi: 10.1016/j.foodhyd.2011.01.016
105. Yavari Maroufi L, Ghorbani M, Tabibiazar M, Mohammadi M, Pezeshki A. Advanced properties of gelatin film by incorporating modified kappa-carrageenan and zein nanoparticles for active food packaging. Int J Biol Macromol. (2021) 183:753–59. doi: 10.1016/j.ijbiomac.2021.04.163
106. Ellis AL, Mills TB, Norton IT, Norton-Welch AB. The hydrophobic modification of kappa carrageenan microgel particles for the stabilisation of foams. J Colloid Interface Sci. (2019) 538:165–73. doi: 10.1016/j.jcis.2018.11.091
107. Hou Y, Liu H, Zhu D, Liu J, Zhang C, Li C, et al. Influence of soybean dietary fiber on the properties of konjac glucomannan/κ-carrageenan corn oil composite gel. Food Hydrocol. (2022) 129:107602–10. doi: 10.1016/j.foodhyd.2022.107602
108. Farhan A, Hani NM. Active edible films based on semi-refined κ-carrageenan: antioxidant and color properties and application in chicken breast packaging. Food Packag Shelf Life. (2020) 24:100476–83. doi: 10.1016/j.fpsl.2020.100476
109. Abdelhedi O, Nasri R, Jridi M, Kchaou H, Nasreddine B, Karbowiak T, et al. Composite bioactive films based on smooth-hound viscera proteins and gelatin: physicochemical characterization and antioxidant properties. Food Hydrocol. (2018) 74:176–86. doi: 10.1016/j.foodhyd.2017.08.006
110. Liu Y, Zhang X, Li C, Qin Y, Xiao L, Liu J. Comparison of the structural, physical and functional properties of κ-carrageenan films incorporated with pomegranate flesh and peel extracts. Int J Biol Macromol. (2020) 147:1076–88. doi: 10.1016/j.ijbiomac.2019.10.075
111. Chen S, Ma Y, Dai L, Liao W, Zhang L, Liu J, et al. Fabrication, characterization, stability and re-dispersibility of curcumin-loaded gliadin-rhamnolipid composite nanoparticles using pH-driven method. Food Hydrocol. (2021) 118:106758–68. doi: 10.1016/j.foodhyd.2021.106758
112. Alizadeh Sani M, Tavassoli M, Salim SA. Azizi-lalabadi M, McClements DJ. Development of green halochromic smart and active packaging materials: TiO2 nanoparticle- and anthocyanin-loaded gelatin/κ-carrageenan films. Food Hydrocol. (2022) 124:107324–35. doi: 10.1016/j.foodhyd.2021.107324
113. Shojaee-Aliabadi S, Hosseini H, Mohammadifar MA, Mohammadi A, Ghasemlou M, Hosseini SM, et al. Characterization of κ-carrageenan films incorporated plant essential oils with improved antimicrobial activity. Carbohydr Polym. (2014) 101:582–91. doi: 10.1016/j.carbpol.2013.09.070
114. Daniel-da-Silva AL, Ferreira L, Gil AM, Trindade T. Synthesis and swelling behavior of temperature responsive κ-carrageenan nanogels. J Colloid Interface Sci. (2011) 355:512–17. doi: 10.1016/j.jcis.2010.12.071
115. Abdorreza MN, Cheng LH. Karim AA. Effects of plasticizers on thermal properties and heat sealability of sago starch films. Food Hydrocol. (2011) 25:56–60. doi: 10.1016/j.foodhyd.2010.05.005
116. Sun G, Liang T, Tan W, Wang L. Rheological behaviors and physical properties of plasticized hydrogel films developed from κ-carrageenan incorporating hydroxypropyl methylcellulose. Food Hydrocol. (2018) 85:61–8. doi: 10.1016/j.foodhyd.2018.07.002
117. Chen H, Wu D, Ma W, Wu C, Liu J, Du M. Strong fish gelatin hydrogels double crosslinked by transglutaminase and carrageenan. Food Chem. (2022) 376:131873–81. doi: 10.1016/j.foodchem.2021.131873
118. Li T, Wen C, Dong Y, Li D, Liu M, Wang Z, et al. Effect of ε-polylysine addition on κ-carrageenan gel properties: rheology, water mobility, thermal stability and microstructure. Food Hydrocol. (2019) 95:212–18. doi: 10.1016/j.foodhyd.2019.04.027
119. Mostafidi M, Sanjabi MR, Shirkhan F, Zahedi MT. A review of recent trends in the development of the microbial safety of fruits and vegetables. Trends Food Sci Technol. (2020) 103:321–32. doi: 10.1016/j.tifs.2020.07.009
120. Otoni CG, Avena-Bustillos RJ, Azeredo HMC, Lorevice MV, Moura MR, Mattoso LHC, et al. Recent advances on edible films based on fruits and vegetables—a review. Compr Rev Food Sci Food Saf. (2017) 16:1151–69. doi: 10.1111/1541-4337.12281
121. Zhang C, Chi W, Meng F, Wang L. Fabricating an anti-shrinking κ-carrageenan/sodium carboxymethyl starch film by incorporating carboxylated cellulose nanofibrils for fruit preservation. Int J Biol Macromol. (2021) 191:706–13. doi: 10.1016/j.ijbiomac.2021.09.134
122. Meindrawan B, Suyatma NE, Wardana AA, Pamela VY. Nanocomposite coating based on carrageenan and ZnO nanoparticles to maintain the storage quality of mango. Food Packag Shelf Life. (2018) 18:140–46. doi: 10.1016/j.fpsl.2018.10.006
123. Golding JB, Stathopoulos CE, Scarlett CJ, Bowyer M, et al. Development and application of rice starch based edible coating to improve the postharvest storage potential and quality of plum fruit (Prunus salicina). Sci Hortic. (2018) 237:59–66. doi: 10.1016/j.scienta.2018.04.005
124. Zhang R, Wang X, Li L, Cheng M, Zhang L. Optimization of konjac glucomannan/carrageenan/nano-SiO2 coatings for extending the shelf-life of Agaricus bisporus. Int J Biol Macromol. (2019) 122:857–65. doi: 10.1016/j.ijbiomac.2018.10.165
125. Resende N, Gonçalves G, Reis K, Tonoli GHD, Boas E. Chitosan/Cellulose Nanofibril nanocomposite and its effect on quality of coated strawberries. J Food Qual. (2018) 2018:1–13. doi: 10.1155/2018/1727426
126. Ghimire A, Paudel N, Poudel R. Effect of pomegranate peel extract on the storage stability of ground buffalo (Bubalus bubalis) meat. LWT. (2022) 154:112690–98. doi: 10.1016/j.lwt.2021.112690
127. Faustman C, Cassens RG. The biochemical basis for discoloration in fresh meat: a review. J Muscle Foods. (2007) 1:217–43. doi: 10.1111/j.1745-4573.1990.tb00366.x
128. Zhang C, Sun G, Cao L, Wang L. Accurately intelligent film made from sodium carboxymethyl starch/κ-carrageenan reinforced by mulberry anthocyanins as an indicator. Food Hydrocol. (2020) 108:106012–19. doi: 10.1016/j.foodhyd.2020.106012
129. Li F, Liu Y, Cao Y, Zhang Y, Zhe T, Guo Z, et al. Copper sulfide nanoparticle-carrageenan films for packaging application. Food Hydrocol. (2020) 109:106094–03. doi: 10.1016/j.foodhyd.2020.106094
130. Zhou X, Zong X, Zhang M, Ge Q, Qi J, Liang J, et al. Effect of konjac glucomannan/carrageenan-based edible emulsion coatings with camellia oil on quality and shelf-life of chicken meat. Int J Biol Macromol. (2021) 183:331–39. doi: 10.1016/j.ijbiomac.2021.04.165
131. Chi W, Cao L, Sun G, Meng F, Zhang C, Li J, et al. Developing a highly pH-sensitive κ-carrageenan-based intelligent film incorporating grape skin powder via a cleaner process. J Clean Prod. (2020) 244:118862–70. doi: 10.1016/j.jclepro.2019.118862
132. Sun G, Chi W, Zhang C, Xu S, Li J, Wang L. Developing a green film with pH-sensitivity and antioxidant activity based on κ-carrageenan and hydroxypropyl methylcellulose incorporating Prunus maackii juice. Food Hydrocol. (2019) 94:345–53. doi: 10.1016/j.foodhyd.2019.03.039
133. Liu J, Wang H, Guo M, Li L, Chen M, Jiang S, et al. Extract from Lycium ruthenicum Murr. Incorporating κ-carrageenan colorimetric film with a wide pH–sensing range for food freshness monitoring. Food Hydrocol. (2019) 94:1–10. doi: 10.1016/j.foodhyd.2019.03.008
134. Ullah R, Nadeem M. Imran M. Omega-3 fatty acids and oxidative stability of ice cream supplemented with olein fraction of chia (Salvia hispanica L) oil. Lipids Health Dis. (2017) 16:34–41. doi: 10.1186/s12944-017-0420-y
135. Mahajan K, Kumar S, Bhat ZF, Naqvi Z, Mungure TE, Bekhit AEA. Functionalization of carrageenan based edible film using Aloe vera for improved lipid oxidative and microbial stability of frozen dairy products. Food Biosci. (2021) 43:101336–45. doi: 10.1016/j.fbio.2021.101336
136. Pérez MJ, Moreno MA, Martínez-Abad A, Cattaneo F, Zampini C, Isla MI, et al. Interest of black carob extract for the development of active biopolymer films for cheese preservation. Food Hydrocol. (2021) 113:106436–44. doi: 10.1016/j.foodhyd.2020.106436
Keywords: carrageenan, food packaging, film-forming methods, film properties, formation mechanism
Citation: Cheng C, Chen S, Su J, Zhu M, Zhou M, Chen T and Han Y (2022) Recent advances in carrageenan-based films for food packaging applications. Front. Nutr. 9:1004588. doi: 10.3389/fnut.2022.1004588
Received: 27 July 2022; Accepted: 16 August 2022;
Published: 09 September 2022.
Edited by:
Long Chen, Jiangnan University, ChinaReviewed by:
Taotao Dai, Guangxi Academy of Agricultural Science, ChinaCopyright © 2022 Cheng, Chen, Su, Zhu, Zhou, Chen and Han. This is an open-access article distributed under the terms of the Creative Commons Attribution License (CC BY). The use, distribution or reproduction in other forums is permitted, provided the original author(s) and the copyright owner(s) are credited and that the original publication in this journal is cited, in accordance with accepted academic practice. No use, distribution or reproduction is permitted which does not comply with these terms.
*Correspondence: Yahong Han, eWFob25naGFuMjAxOUBob3RtYWlsLmNvbQ==
†These authors have contributed equally to this work and share first authorship
Disclaimer: All claims expressed in this article are solely those of the authors and do not necessarily represent those of their affiliated organizations, or those of the publisher, the editors and the reviewers. Any product that may be evaluated in this article or claim that may be made by its manufacturer is not guaranteed or endorsed by the publisher.
Research integrity at Frontiers
Learn more about the work of our research integrity team to safeguard the quality of each article we publish.