- 1Department of Gastroenterology, The Second Affiliated Hospital and Yuying Children's Hospital of Wenzhou Medical University, Wenzhou, China
- 2Institute of Ischemia/Reperfusion Injury, Wenzhou Medical University, Wenzhou, China
- 3Department of Pathophysiology, School of Basic Medicine Science, Wenzhou Medical University, Wenzhou, China
- 4Department of Gastrointestinal Oncology, The Second Affiliated Hospital and Yuying Children's Hospital of Wenzhou Medical University, Wenzhou, China
Background and aims: Metabolic syndrome (MetS), accompanied with significant intestinal dysbiosis, causes a great public health burden to human society. Here, we carried out a meta-analysis to qualify randomized controlled trials (RCTs) and to systematically evaluate the effect of microbial therapy on MetS.
Methods and results: Forty-two RCTs were eligible for this meta-analysis after searching the PubMed, Cochrane, and Embase databases. Pooled estimates demonstrated that treatment with microbial therapy significantly reduced the waist circumference (WC) (SMD = −0.26, 95% CI −0.49, −0.03), fasting blood glucose (FBG) (SMD = −0.35, 95% CI −0.52, −0.18), total cholesterol (TC) (SMD = −0.36, 95% CI −0.55, −0.17), low-density lipoprotein cholesterol (LDL-C) (SMD = −0.42, 95% CI −0.61, −0.22), and triacylglycerol (TG)(SMD = −0.38, 95% CI −0.55, −0.20), but increased the high-density lipoprotein cholesterol (HDL-C) (SMD = 0.28, 95% CI.03, 0.52). Sensitivity analysis indicated that after eliminating one study utilizing Bifidobacteriumlactis, results became statistically significant in diastolic blood pressure (DBP) (SMD = −0.24, 95% CI −0.41, −0.07) and in Homeostatic Model Assessment of Insulin Resistance (HOMA-IR) (SMD = −0.28, 95% CI −0.54, −0.03), while the body mass index (BMI) showed significant difference after eliminating one study utilizing oat bran (SMD = −0.16, 95% CI −0.31, −0.01). There was still no significant effect in systolic blood pressure (SBP) and in hemoglobin A1c (HbA1c%).
Conclusion: In patients with MetS, the conditioning with microbial therapy notably improves FBG, TC, TG, HDL-C, LDL-C, WC, BMI (except for the study using oat bran), HOMA-IR, and DBP (except for the Study using Bifidobacteriumlactis), however, with no effect in SBP and in HbA1c%.
Introduction
Metabolic syndrome (MetS) is prevalent in the whole world and holds the largest burden of non-communicable diseases worldwide. It is a metabolic intertwined condition composed mainly of morbidities such as glucose intolerance, dyslipidemia, abdominal obesity, and high blood pressure (1). The development of this metabolic perturbation could double the risk of developing type 2 diabetes mellitus, obesity, cardiovascular disease, non-alcoholic steatohepatitis, and cancer (2, 3). According to the National Health and Nutrition Examination Survey in the U.S., the weighted MetS prevalence has steadily increased from 32.5% in 2011–2012, 34.6% in 2013–2014, and to 36.9% in 2015–2016, respectively (4). Therefore, it is urgent to prevent and control the development of MetS.
Notably, the sedentary lifestyles and the preference for nutrient-depleted, energy-dense, and highly refined foods have been considered as the main etiological factors. However, the corresponding prevention measures did not obtain anticipative results in practices. As the microbiota become the center of systematic diseases, published studies in the last decades have shown that the underlying mechanisms of MetS might have originated from flora disturbance. According to different fiber types (5, 6), fat composition (7, 8), food additives (9, 10), and microbiome could establish different sensitivity, and the individuals with MetS had a lower gut microbiota diversity than the healthy ones (11). Proteobacteria and Firmicutes (other than Ruminococcaceae) were reported to be positively associated with MetS, whereas the Bacteroidetes and Ruminococcaceae have a negative association (12).
Therefore, nowadays, microbial therapy that includes microbial agents and fecal microbiota transplantation (FMT), which could modulate intertwined microbiota, has emerged gradually as the new candidate to MetS treatment due to the recently published observations in both animal and human studies of its beneficial effects. In animal experiments, it has been demonstrated that oligofructosein, Lactobacillus fermentum TS1 and S2, pasteurized A. muciniphila, and a combination of Lactobacillus and Bacillus subtilis have shown tremendous potential, especially in lipid metabolism in treating MetS (13–16). In addition, microbial metabolites, such as short-chain fatty acids (SCFAs) contributing to improved glucose homeostasis and insulin sensitivity, were also identified as a therapeutic target for MetS (17, 18).
In the last few decades, FMTs ranging from the healthy to the target-therapy subjects, with the aim of correcting microbiota perturbation, have shown promising metabolic improvements. To begin with, FMT was broadly researched in Clostridioides difficile infection (19, 20). Considering that altered gut microbiome may be one of the factors contributing to inflammatory bowel disease (IBD), FMT later became of increasing importance in IBD remission (21–23). More recently, emerging evidence has indicated that MetS is another potential target for FMT therapy. One of the randomized controlled trials enrolled 68 bariatric patients with MetS who were randomly allocated to FMT or placebo group (24). Improvements were seen in Homeostatic Model Assessment of Insulin Resistance (HOMA-IR), insulin sensitivity, and diastolic blood pressure (DBP). Another pilot FMT trial reported that patients in the FMT arm had a decrease in both glucose and insulin level compared to baseline, suggesting a protective role of FMT in MetS (25).
These data suggested that microbial therapy could exert a remarkable benefit to a host with MetS risk factors. However, due to the variety in microbial therapy type and dosage, the interplay between microbial therapy and MetS has not yet been systematically expounded. We decoupled the risk factors for analyses and investigated whether there was a microbial therapy link to hyperglycemia, dyslipidemia, hypertension, and anthropometric parameters; thus, systematically addressing the compelling published studies regarding the effect of microbial therapy on specific risk factors.
Methods
Search Strategy
The Preferred Reporting Items for Systematic Reviews and Meta-Analysis (PRISMA) guidelines were followed in this meta-analysis (26). A search of the electronic literature up to May 2021 was conducted using the Pubmed database, the Cochrane Library, and the Embase database. The search strategy was developed with the following keywords and synonyms for related terms: intervention (“prebiotics” OR “probiotics” OR “synbiotics” OR “short-chain fatty acids” OR “niacin” OR “bile acids” OR “bacterial metabolites” OR “fecal microbiota transplantation) AND disease (“metabolic syndrome”). The RCTs examining the effect of microbial therapy on MetS were eligible for this analysis. There was no language restriction. The initial search after importing the located results from the database into the EndNote was derived from the titles and abstracts evaluation in accordance to the appropriateness of our selection criteria. Sequentially, full texts examination was conducted for a better choice to our study question. Two reviewers independently carried it out and then recorded the concrete inclusion or exclusion felts. Any disagreement was resolved by conversation. The bibliographies of all identified related papers were carefully checked to perform a recursive search. We also contacted authors of studies that have incomplete information in available databases to complete the partial texts, which will then maximize our chances to get eligible research.
This measure was also applied for fully published studies that randomized MetS patients to receive microbial therapy or placebo, but did not refer to data concerning subsequent available intervention results, so as to get the data at the most recent point of follow-up.
Selection Criteria
Randomized controlled trials (RCTs) conducted in MetS human subjects with the intervention of microbial therapy were considered as our inclusion criteria. The MetS diagnosis must meet at least three of the following five criteria in accordance with the International Diabetes Federation Guidelines: (1) Increased waist circumference (WC) with ethnic-specific WC cut-points (White and all other ethnic groups—men ≥ 94 cm; women ≥ 80 cm. South Asians, Chinese, and Japanese—men ≥ 90 cm; women ≥ 80 cm); (2) Triglyceride (TG) ≥ 150 mg/dl (1.7 mmol/L) or treatment for elevated triglycerides; (3) High-density lipoprotein cholesterol (HDL-C) <40 mg/dl (1.03 mmol/l) in men or <50 mg/dl (1.29 mmol/L) in women, or treatment for low HDL; (4) Systolic blood pressure (SBP) ≥ 130, diastolic blood pressure (DBP) ≥ 85, or treatment for hypertension; and (5) Fasting blood-glucose (FBG) ≥ 100 mg/dl (5.6 mmol/L) or after 2 h glucose loading blood glucose was ≥7.8 mmol/L or was previously diagnosed with type 2 diabetes. Availability in data for quantitative calculation was the final eligible criteria. Animal experiments, in vitro studies, reviews and meta-analysis, letters, and comments were excluded for this analysis.
Outcome Assessment
The overriding outcome assessment was the effect of the microbial therapy on MetS included BMI(kg/m2), body weight (kg), WC (cm), hip circumference (cm), waist-to-hip ratio, body fat mass (BFM), body fat percentage (BFP) (%), blood pressure (BP) including SBP and DBP (mmHg), FBG (mmol/L), insulin resistance (HOMA-IR) or S (%), TC (mmol/L), HDL-C (mmol/L), low density lipoprotein cholesterol (LDL-C) (mmol/L), TG(mmol/L), and/or HbA1c%. These were some of the commonly used indicators related to our topic.
Data Extraction
All relevant data from each article were independently examined and extracted by the two authors as dichotomous outcomes to estimate reliability, and some of the concrete information needed were as follows: (1) characteristics of the studies (i.e., the first author, publication year, and number of included participants), (2)' characteristics of the participants (i.e., age, sex, and BMI), (3) information on interventions (i.e., route of administration, dosage, duration of treatment, length of follow-up, and set of control group), and (4) outcome variables (i.e., anthropometric parameters, lipid profile, and glucose metabolism). Disagreements were resolved by consensus and no divergence required adjudication.
Quality Assessment
One author critically appraised all eligible studies to determine the risk of bias, while a second author critically appraised a random sample of included studies to check for consistency. Conflicts in the quality assessment were resolved by a mutual discussion via reference to the original paper. The methodological quality of RCTs was independently assessed by two reviewers using the Cochrane Risk Assessment Scale mentioned in the Cochrane handbook where six items, including selection bias (random sequence generation and allocation concealment), performance bias (blinding of participants and personnel), detection bias (blinding of outcome assessment), attrition bias (incomplete outcome data), reporting bias (selective reporting), and other biases, were evaluated. Reviewers appraising each criterion demonstrated if the included study has conformed to each bias minimization item by recording “high risk,” “low risk,” or “unclear.”
Statistical Analysis
RevMan 5.3 was used for calculation. SMD with 95% CIs at end-point data from intervention and control groups were measured for continuous variables through DerSimonian and Laird random effects meta-analysis, therefore reflecting the efficacy of microbial therapy treatment. The heterogeneity between the study-specific estimates was qualitatively assessed with Cochran's Q test and further quantified by the I2 statistics, while the former demonstrated the inconsistency among results and the latter indicated the proportion attributed to the heterogeneity rather than sampling error of total variation in the study estimates. In this, value of p < 0.10 or a value of >50% was considered suggestive of significant heterogeneity. When noted heterogeneity existed, possible explanations were investigated via subgroup analyses according to some variables, such as the type of microbial therapy. Sensitivity analysis was also conducted by removing one study in turn to estimate the weight of each study in heterogeneity. These measures may partly explain the observed variability so the final conclusion should be made with caution. Publication bias was conducted using Begg's and Egger's tests. If there were ≥10 eligible studies in our eventual analysis, funnel plots would be employed for evidence of asymmetry and, hence, would be a possible publication bias. Review Manager Version 5.3 was used for generating these analyses. A value p ≤ 0.05, except for heterogeneity, was considered to have statistical significance.
Results
Identification of Eligible Studies
A flow diagram outlining the overall search strategy and selection procedure in this meta-analysis is shown in Figure 1. Among the 9,986 records identified in our initial search, there were 3,840 duplications removed. After being screened for titles and abstracts, 6,024 studies were excluded since they delivered inconformity of information to our subject. For the remaining 122 papers correlated to the topic, 80 studies were ineligible due to its dissociation to the topic (n = 41), irrelevant intervention (n = 12), null outcome of interest to review (n = 8), overlapping data (n = 4), reviews and meta-analysis (n = 11), and conference abstract (n = 4). Finally, the search strategy has returned 42 studies for qualitative synthesis in this meta-analysis.
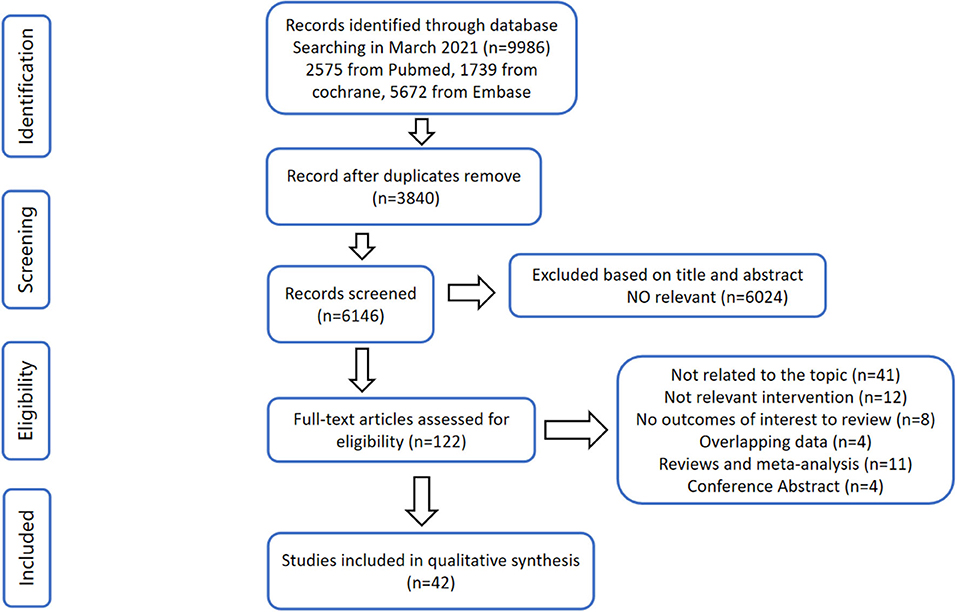
Figure 1. Flow diagram according to the preferred reporting items for systematic reviews and meta-analyses (PRISMA) protocol.
Characteristics of Included Studies
The characteristics of included studies are shown in Table 1. Among 42 eligible studies, 14 studies intervened with prebiotics (24, 29–39, 46, 47), 10 with probiotics (40, 41, 43, 48–54), 6 with synbiotics (24, 44, 55–58), 10 with microbial metabolites (27, 28, 59–66), and the remaining 4 with fecal microbiota transplantation (FMT) (24, 25, 45, 67). Among these studies, Mocanu et al. (24) not only explored the respective but also the synergetic efficiency of FMT and the prebiotics on MetS. The earliest paper was published in 2007, while the latest was in 2021. Most studies included were carried out in the Western countries, except for 10 studies that were mainly focused on Asian populations (two in China, two in Korea, one in Palestine, five in Iran) (29, 31, 37, 40, 44, 53, 55, 56, 58, 66). Exceptionally, other researchers such as Bernini et al. (52) utilized Lactobacillus as probiotic intervention and chose Bifidobacteriumlactis, while Leila et al. (53) used Lactobacillus and Bifidobacterium for observation (52). For microbial metabolites, one study used whey protein (27), another study utilized Lactobacillus plantarum fermented barley (66), which is abundant of biologically active ingredients, and other studies employed niacin as bacterial metabolites. Prebiotics were implicated in researches, such as isomaltulose (46), glucose polysaccharide (37), and resistant starch (24, 32). For FMT intervention, the participants were randomized in receiving the intervention from single lean vegan-donors.
Quality of Included Studies
As shown in Table 2, the allocation concealment, blinding of participants and personnel, and incomplete data outcome were the main fields that are reaching a high risk of bias. Nevertheless, most studies were at low risk of bias and of high methodological quality. In 42 trials that reported the effect of microbial therapy on MetS, 20 were judged as fully marked by authors, whereby 12 studies scored 6 points, 6 studies scored 5 points, 3 studies scored 4 points, and only 1 study scored 3 points.
Effect of Microbial Therapy on Blood Glucose Control
Twenty-two studies enrolling overall 1,454 participants have investigated the effect of microbial therapy on FBG (Figure 2), and an intervention group established more pronounced decline in FBG (SMD = −0.35, 95% CI −0.52, −0.18, P < 0.0001) with moderate heterogeneity (I2 = 57%). Publication bias was not reported in Begg's test (p = 0.141) but was reported in Egger's test (p = 0.026). The studies led by Abutair (29) and Allegretti et al. (25) have a high risk of bias. Even so, concomitant with the statistical decline in FBG, fasting insulin (Supplementary Figure 1) (42), which determines the ability of insulin resistance, did not show statistical difference (SMD = −0.22, 95% CI −0.49, 0.05, P = 0.10), similar to HOMA-IR (SMD = −0.23, 95% CI −0.49, 0.02, P = 0.08) (Supplementary Figure 2) (42). Sensitivity analysis indicated that when dropped one study from Luciana et al. used Bifidobacterium lactis for probiotic intervention, rather than Lactobacillus mainly in the other studies, the pooled outcome of HOMA-IR was −0.28 (95% CI −0.54, −0.03). Additionally, the result of HbA1c% (Supplementary Figure 3) (42), which reveals the level of blood glucose control in the last 3 months, was not statistically different to the control group (SMD = −0.11, 95% CI −0.50, 0.29, P = 0.60). This may be attributed to the short intervention duration in most studies.
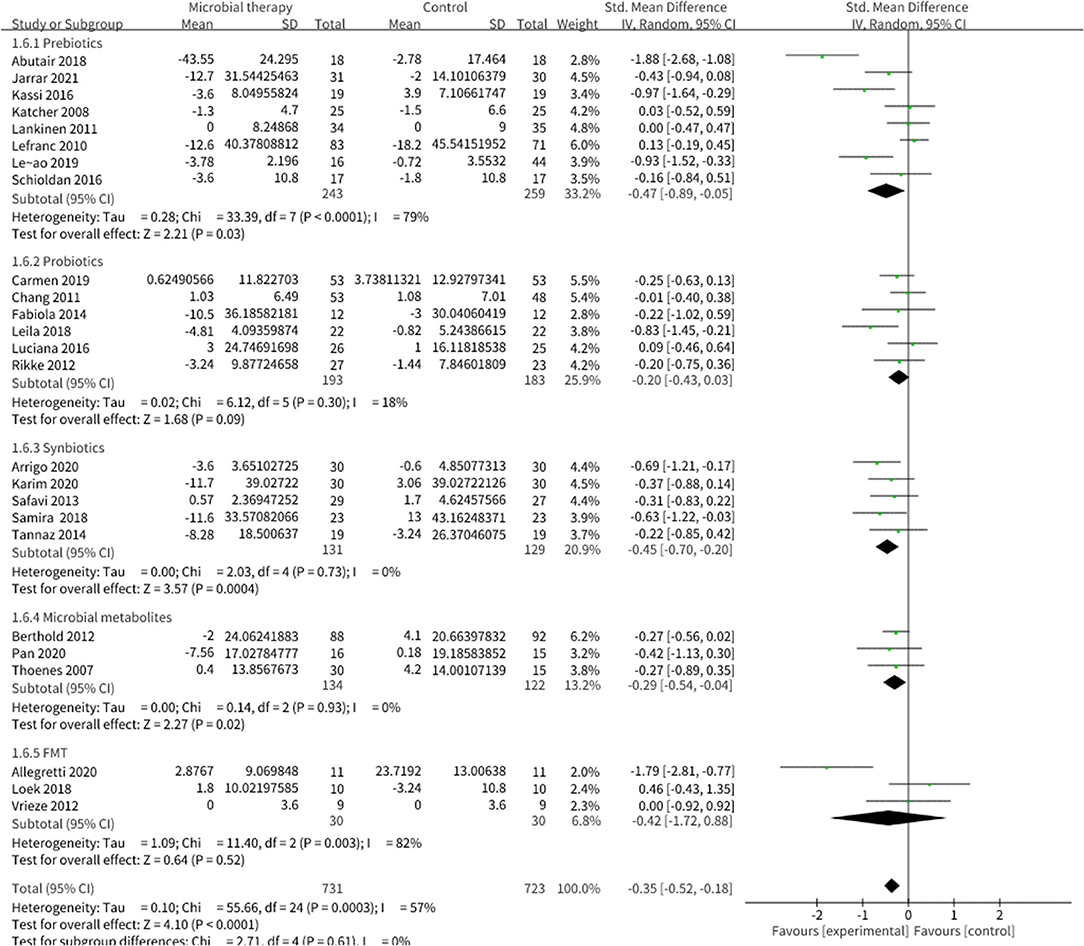
Figure 2. Comparison of standard mean difference (SMD) of fasting blood glucose (FBG) control between intervention groups and control groups Tau2 = 0.10, I2 = 57%, 95% CI −0.52 to −0.18, Z = 4.10, p < 0.0001. Significant difference was shown in FBG.
Effect of Microbial Therapy on BP Control
Eighteen studies explored the effect of microbial therapy on BP (Supplementary Figures 4, 5) (42), leading to a non-statistical difference to the placebo in SBP (SMD = −0.11, 95% CI −0.32, 0.10, P = 0.29) and in DBP (SMD = −0.18, 95% CI −0.39, 0.02, P = 0.08). Sensitivity analysis showed that removing one study led by Bernini et al. (52) could make the DBP outcome significant (SMD = −0.24, 95% CI −0.41, −0.07), whereby no study could exert excessive contribution to the SBP outcome.
Effect of Microbial Therapy on Serum Lipoproteins Control
Microbial therapy could regulate hyperlipemia to some extent, as indicated by more dampened level of TC (SMD = −0.36, 95% CI −0.55, −0.17, P < 0.0001) (Figure 3), TG (SMD = −0.38, 95% CI −0.55,−0.20, P < 0.0001) (Figure 4), LDL-C (SMD = −0.42, 95% CI −0.61, −0.22, P < 0.0001) (Figure 5), and more strong elevation in HDL-C (SMD = 0.28, 95% CI.03, 0.52, P = 03) (Figure 6) with significant heterogeneity. No publication bias was uncovered in the TC outcome by Begg's test (p = 0.771) and Egger's test (p = 0.136), similar to the TG outcome (Begg's test p = 0.508, Egger's test p = 0.069). In the HDL-C outcome, there was no hint of publication bias by Begg's test (p = 0.072) unlike in Egger's test (p = 0.001), which is similar to the LDL-C outcome (Begg's test p = 0.182, Egger's test p = 0.022).
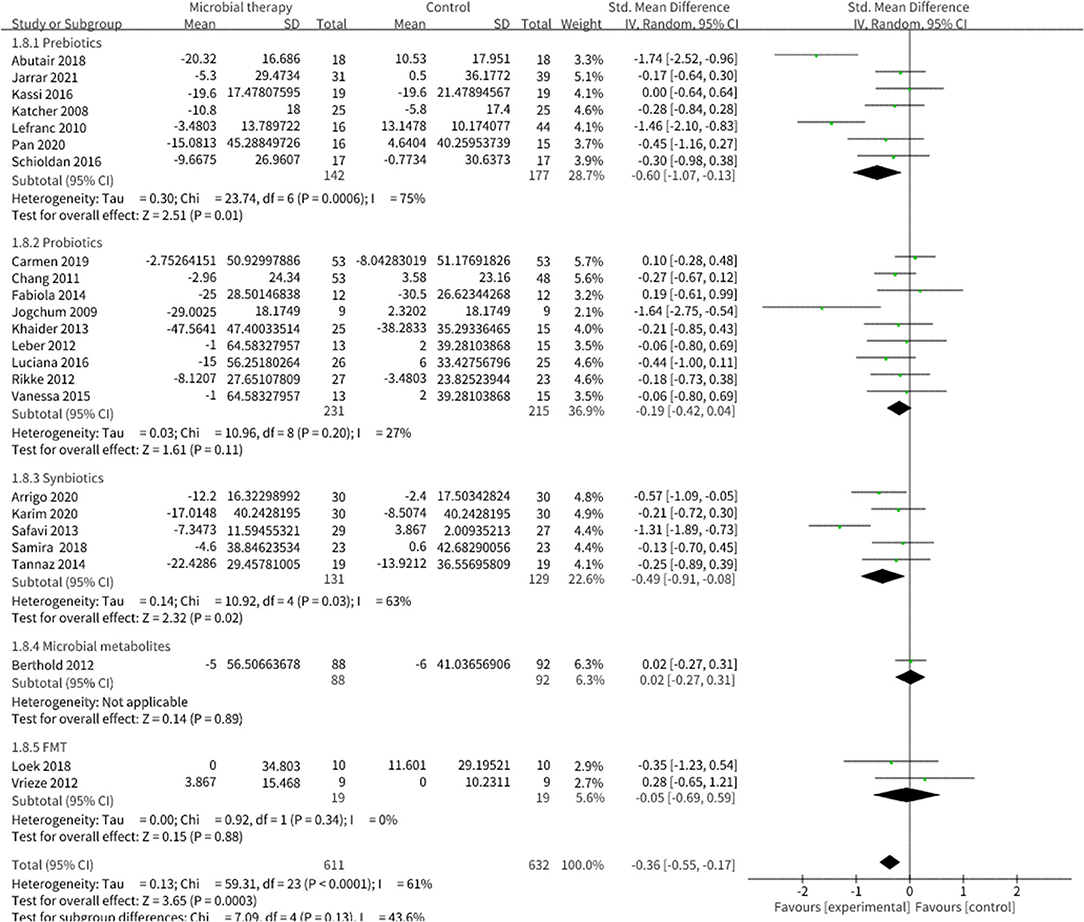
Figure 3. Comparison of SMD of total cholesterol (TC) control between intervention groups and control groups Tau2 = 0.13, I2 = 61%, 95% CI −0.55 to −0.17, Z = 3.65, p = 0.0003. Significant difference was shown in TC.
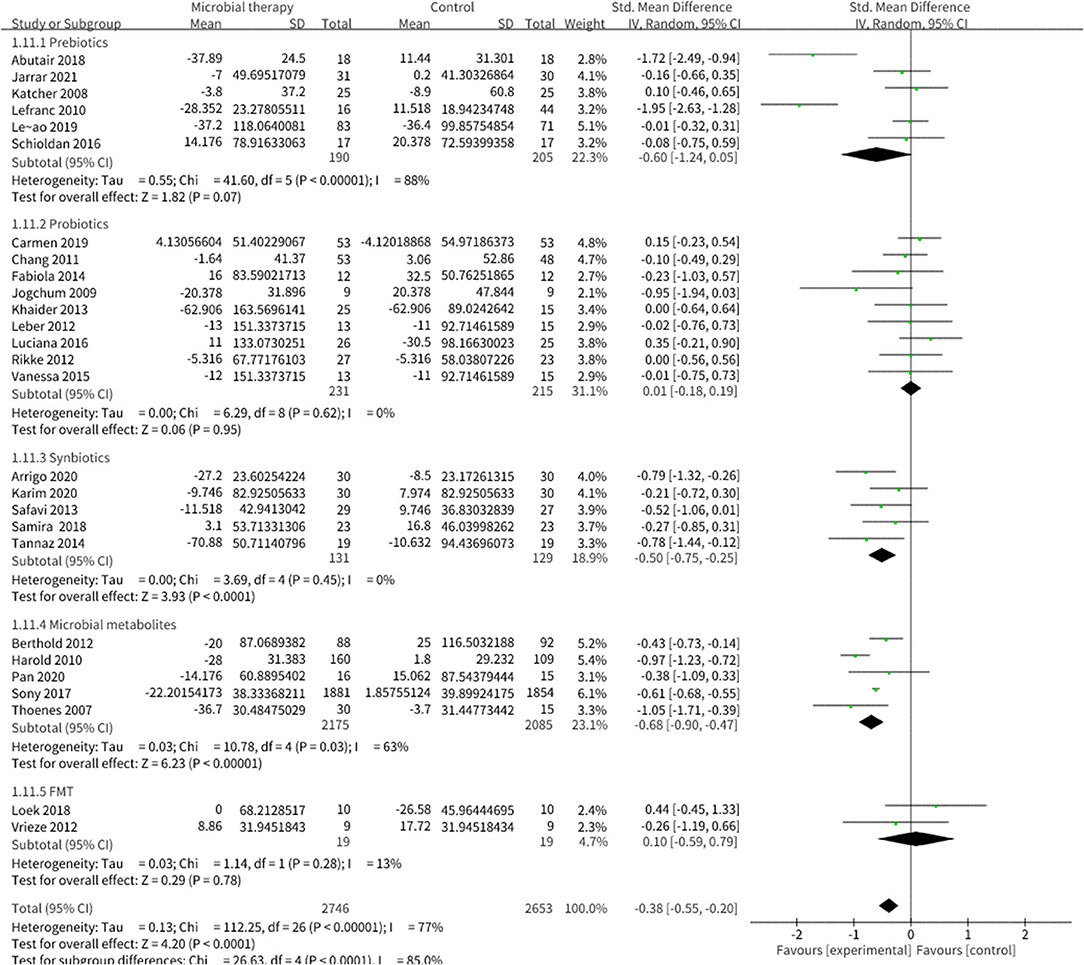
Figure 4. Comparison of SMD of triacylglycerol (TG) control between intervention groups and control groups Tau2 = 0.13, I2 = 77%, 95% CI −0.55 to −0.20, Z = 4.20, p < 0.0001. Significant difference was shown in TG.
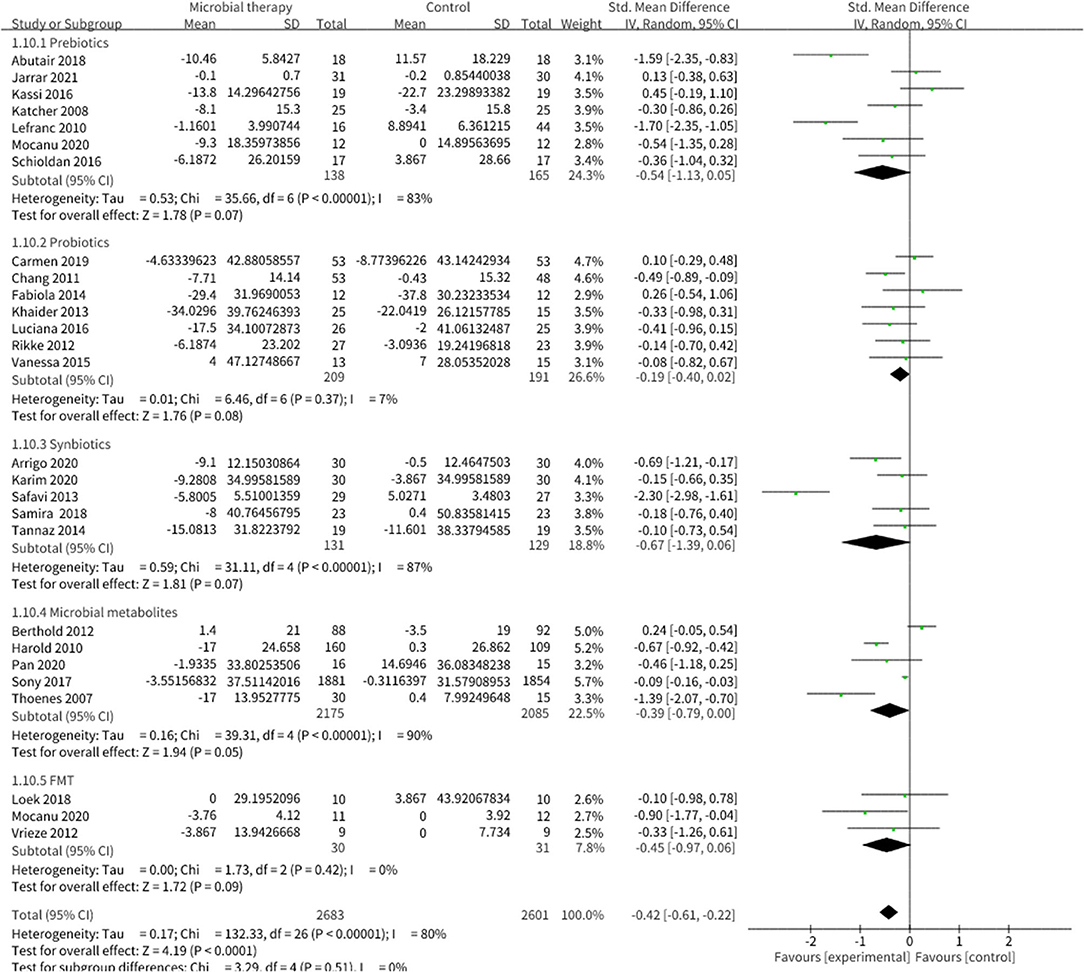
Figure 5. Comparison of SMD of low-density lipoprotein cholesterol (LDL-C) control between intervention groups and control groups Tau2 = 0.17, I2 = 80%, 95% CI −0.61 to −0.22, Z = 4.19, p < 0.0001. Significant differences were shown in LDL-C.
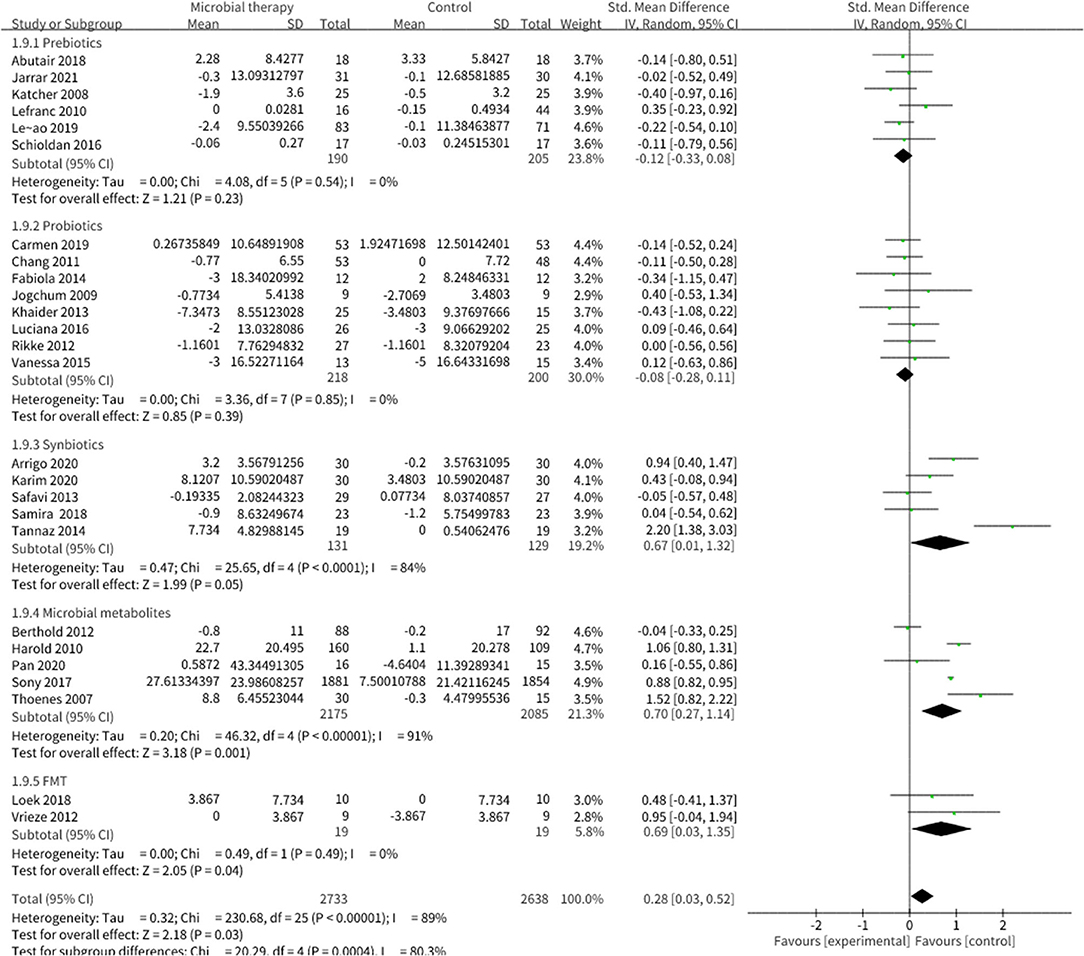
Figure 6. Comparison of SMD of high-density lipoprotein cholesterol (HDL-C) control between intervention groups and control groups Tau2 = 0.32, I2 = 89%, 95% CI 0.03 to 0.52, Z = 2.18, p = 0.03. Significant differences were shown in HDL-C.
Effect of Microbial Therapy on Anthropometric Parameters
Twenty studies reported the effect of microbial therapy on WC (Figure 7). A more pronounced decline was displayed to the placebo (SMD = −0.26, 95% CI −0.49, −0.03, P = 0.03) with moderate heterogeneity (I2 = 57 %, p = 0.007). No publication bias was assessed by Begg's test (p = 0.731) and Egger's test (p = 0.231). No significant difference was displayed compared with the placebo in BMI (SMD = −0.13, 95% CI −0.27, 0.00, P = 0.05) (Supplementary Figure 6) (42). Sensitivity analysis indicated that when we removed the study conducted by Leão et al. (36), which utilized oat bran as prebiotic intervention, the pooled result BMI could be significant (SMD = −0.16, 95% CI −0.31, −0.01).
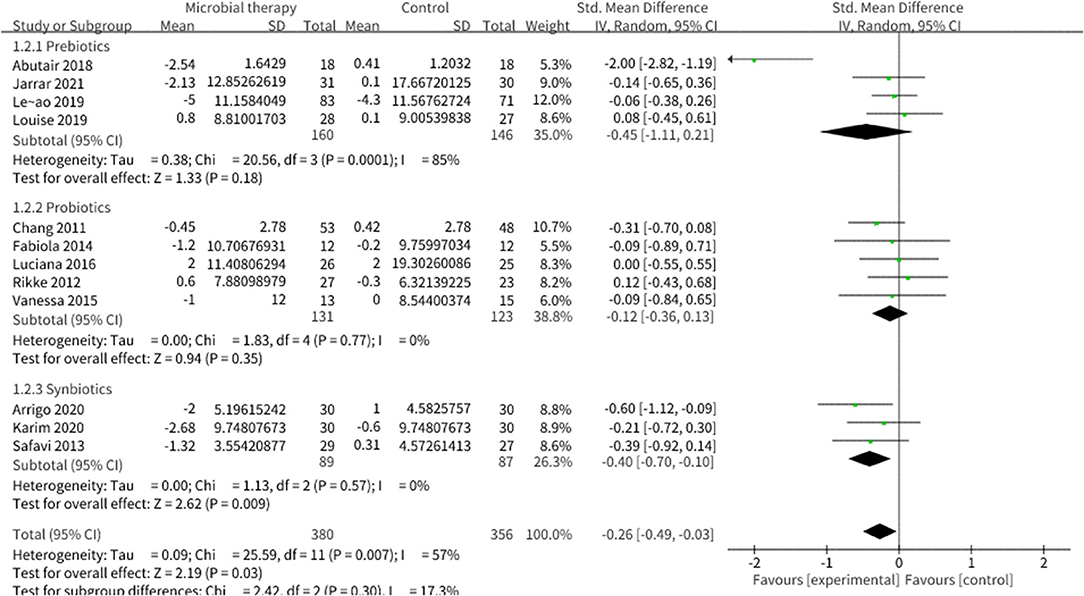
Figure 7. Comparison of SMD of anthropometric parameters between intervention groups and control groupsTau2 = 0.09, I2 = 57%, 95% CI −0.49 to −0.03, Z = 2.19, p = 0.03. Significant difference was shown in WC.
Adverse Events
Treatment-related adverse experiences could be attributed to the nature of the interventions. In microbial metabolites, niacin-induced flushing was reported in three studies (28, 60, 61), as well as the slight gastrointestinal (27)and hepatic (60) disorders. For probiotics and prebiotics, gastrointestinal symptoms, including increased bowel movements, diarrhea, flatulence, temporary constipation, and decreased appetite were mentioned (30, 36, 41, 49). The study led by Louise et al. (36) in 2019 also reflected seasonal diseases such as sore throat, common cold, and influenza. Seven serious events with no specific indication were even recorded in Gouni-Berthold's trial (27).
Discussion
Our analysis showed that microbial therapy is essential for mounting an effective response against intertwined metabolism in MetS. Building on the pooled outcomes, we provided strong evidence that microbial therapy application significantly dampens the risk indicators in MetS, including FBG, TC, TG, HDL-C, LDL-C, and WC. Further showing the straight benefit of microbial therapy in MetS is the improvement of DBP, HOMA-IR, and BMI in a sensitivity analysis. After omitting one study using Bifidobacteriumlactis as probiotic intervention, DBP and HOMA-IR improvements showed statistical significance, whereby BMI decreased significantly after neglecting one study that employed oat bran as prebiotic intervention. No obvious publication bias was detected in most of the bias test that we performed.
About 100 trillion micro-organisms inhabit the human gastrointestinal tract, providing unique metabolic functions to the host and giving fundamental importance to health and disease (68, 69). Early in 2007, animal studies demonstrated that a high-fat diet could chronically increase the proportion of lipopolysaccharide (LPS) contained in the gut together with the elevation of inflammation markers, liver triglyceride content, and liver insulin resistance (70), thereby contributing to the emergence of gut-centric theory in MetS. Evidence suggested that ingestion of a high-fat and low-fiber diet could induce the dysbiosis of gut microbiome, which contributed to the aberrant blooms or loss of bacteria (71). Of these intertwined bacteria, the proportion of gram-negative microbiota (mainly Bacteroidetes and Proteobacteria) (72) was notably elevated, while the relative proportions of gram-positive microbiome including Lactobacillus and Bifidobacterium were notably decreased. As consequence, aberrant metabolites from maladjusted bacteria, such as lipopolysaccharide (LPS) and trimethylamine (TMA), could disrupt intestinal barrier integrity, which should have been maintained by homeostatic metabolites such as glucagon-like peptide 1 (GLP-1) and GLP-2 (71). When these metabolites circulated into the liver, adipose, and other tissues, endoplasmic reticulum stress in lipid-overloaded adipocytes (73), and/or innate immune Toll-like receptors (TLRs) that signal activation (70) would be invited, leading to the chronic low—grade systematic inflammation (74). Consequently, this chronic inflammation would ultimately bring about metabolism perturbation (75), introducing the occurrence of MetS. The essential role of gut barrier integrity in chronic systematic inflammation attributes microbiome to the core in the inflammation-induced metabolic defects.
However, this ensuing chronic systematic inflammation and dysmetabolism could be mediated by microbiome modulation. Probiotics or FMT are conductive to restore disordered microbial function in alleviating obesity, blood lipids, and even inflammation in patients (50, 52). Through our systematic retrieval, we discovered that Lactobacillus and Bifidobacterium are the most commonly utilized probiotic interventions and displayed anticipated benefits. As mentioned above, patients with MetS showed a sharp decline of gram-positive bacteria but also an increase in gram-negative bacteria. Specific gram-positive bacteria, like bile salt-hydrolyzing Lactobacillus reuteri strain, can inhibit lipoprotein lipase, the enzyme responsible for TG hydrolysis, and, therefore, against the calorie's uptake from gut and storage in adipose tissue (72). Moreover, Lactobacillus also inhibit angiotensin I-converting enzyme (ACE) activities via casein degradation (76, 77), thus, controlling the increase of BP. Additionally, gram-positive microbiota (mainly Lactobacillus and Bifidobacterium) could degrade complex plant-derived polysaccharides (78) to SCFAs. Subjects that were assigned to be given the small intestinal infusions of allogenic microbiota have showed elevated levels of butyrate-producing intestinal microbiota, along with the increased insulin sensitivity of recipients (45).
In terms of microbial metabolism, the SCFAs of metabolites and nicotinic acid have received great attention. SCFAs serve microbial cross-feeding communities and satisfy some of our daily energy requirements (79). Moreover, they could regulate the immune system through the free fatty acid receptor FFA2R activation (80) and nuclear factor (NF)-kB inhibition (81). In addition, they suppress the lipopolysaccharide-stimulated tumor necrosis factor (TNF)α production from neutrophils (82) and the proinflammatory cytokines formation in human adipose tissue (83). Acetate, propionate, and butyrate represent the most capable SCFAs, and among them, propionate is mainly a substrate for gluconeogenesis, whereas acetate and butyrate are primarily ready for lipogenesis (84). Butyrate, as the principal fuel for intestinal epithelial cells (85), establishes a strong ability to restore gut permeability through activating peroxisomal proliferator-activated receptor (86) and upregulates mucin-associated genes (MUC1-4) expression in intestinal epithelial goblet cells (87). Eventually, the abnormally increased intestinal permeability could be alleviated. Moreover, SCFAs could influence appetite and satiety signals. The intestine expressed some proteins involved in food intake, including peptide YY (PYY), GLP-1, glucose-dependent insulinotropic polypeptide (GIP), the expression of which were induced by SCFAs and mediated by G protein-coupled receptors (Gpr) 43 and Gpr41 (72). SCFAs supplementation could foster the homeostasis of these peptides, sequentially increasing satiety levels and ultimately reducing food and energy intake (88). In line with the results, recent work demonstrated that colonic infusions of SCFAs mixtures in concentrations and ratios reached after fiber intake can increase fat oxidation, energy expenditure, and PYY, and can decrease lipolysis in overweight/obese men (89).
It is worth noting that in our analysis, most studies, including performance evaluation of microbial metabolites, used niacin as an intervention. Niacin supplementation was sufficient to significantly modulate FBG, TG, and HDL-C. According to existing work, niacin could decrease free fatty acids (FFA) concentrations in humans (65); the raise of which could cause a release of inflammatory cytokines and impairment in brachial artery flow-mediated dilation (90). This process targeted the nicotinamide adenine dinucleotide axis via stimulation of the salvage pathway and also supported a microenvironment for beneficial expansion of adipocytes and activation state of the resident and recruited macrophages in white adipose tissue. Therefore, this is against the low-grade inflammatory state in the high-fat-diet-induced MetS as introduced by dysfunctional white adipose tissue (91–93).
Prebiotics are non-viable food components that can be fermented by commensal organisms. They could be converted into SCFAs and other beneficial microbial metabolites through bacteria fermentation. Supplementation of prebiotics could create an acidic milieu in the gut, suppressing the growth of pathogenic or opportunistic pathogenic bacteria such as Clostridium perfringens and Escherichia coli (94), however, preferentially stimulating the growth of specific bacteria strains like Lactobacillus and Bifidobacterium (95, 96). Different prebiotics exhibit variant metabolism-regulating effects. From existing pieces of research, the relative solubility of different oligosaccharides or polysaccharides related with cell wall material shared variable digestion rate by bacteria desorbed from the biofilms, and followed by the discrepant SCFAs generation (85). SCFAs serve as initial substrates for hepatic gluconeogenesis and de novo lipogenesis (72), thereby affecting the metabolic results. Therefore, we attributed it to be responsible for our sensitivity analysis result; our study utilized oat bran as a prebiotic intervention, thus setting this as the main factor that influenced the significance of the pooled BMI result.
Our observation that the pooled HOMA-IR and DBP became significant after eliminating the study led by Luciana using Bifidobacteriumlactis as probiotic intervention seems to be attributed to the differential ability in carbohydrate metabolism (97). The characteristic types of glycosyl hydrolases in these two bacteria reflected the different types of oligosaccharides that can be fermented (Lactobacillus and Bifidobacterium digest plant and animal-oriented sugars, respectively) (97). In addition, a high diversity impacting on glucose control by specific species of microbiome from Lactobacillus (98, 99) and Bifidobacterium (100, 101) was also reported. Admittedly, some of the variances were accounted for by a different approach of an outcome data presentation in Luciana's article, which was manifested as median (25–75%), while the other works mainly utilized mean (SD) or mean (SE).
There are other similar integration studies focused on this subject. In 2016, Sáez-Lara reviewed the effects of probiotics and synbiotics on metabolism-related diseases including MetS, and have reported decreased plasma lipid levels (102). In the same year, Chen et al. (103) suggested an inverse association between dietary fiber intake and the risk of MetS. However, Dong et al. (104) denoted that probiotic treatment alone could not reduce overall health risks in MetS. A similar conclusion was recapitulated with the study by Snelsonet al. (105) through resistant starch intervention. Collectively, existing articles mostly studied the specific species of microbial therapy on MetS with inconsistent conclusions. Hence, this analysis summarized the relevant treatment of MetS and outlined the importance of microbial therapy to improve risk factors for patients affected by MetS. Therefore, this study provided further evidence to the causes of MetS and the core role of microbiome in systematic diseases.
Conclusion and Limitations
From this analysis, conditioning with microbial therapy presented a favorable effect in controlling BG, blood lipid, and BP. The effect of attenuation in dysmetabolism may be beneficial in the long term for the improvement of MetS or other metabolism-related diseases like diabetes and even other diseases. Due to the relatively single microbial metabolites intervention and the existence of variables like experimental design, the data should be extrapolated more prudently, and further RCTs in various microbial therapy are urgently needed before clinical application.
Data Availability Statement
The original contributions presented in the study are included in the article/Supplementary Materials, further inquiries can be directed to the corresponding author/s.
Author Contributions
XL and ZX: study design and literature search strategy. FW and JS: initial manuscript screening. JS and YC: data extraction and verification. DS and GR: risk-of-bias assessment. BP: statistical analysis. BP and XL: writing of first draft of the manuscript YH and CX: manuscript revision. All authors contributed to the article and approved the submitted version.
Funding
This work was supported by grants from the Zhejiang Provincial Natural Science Foundation of China [LY20H180010], the Wenzhou Science and Technology Bureau [Y20180142], the Wenzhou Science and Technology Bureau [Y2020214], the Zhejiang Provincial Medical and Health Science and Technology Project General Project [No. 2019KY461], the Wenzhou Science and Technology Bureau [Y20190060], and the Zhejiang Provincial Public Welfare Technology Research Plan/Social Development Project [LGF20H070003].
Conflict of Interest
The authors declare that the research was conducted in the absence of any commercial or financial relationships that could be construed as a potential conflict of interest.
Publisher's Note
All claims expressed in this article are solely those of the authors and do not necessarily represent those of their affiliated organizations, or those of the publisher, the editors and the reviewers. Any product that may be evaluated in this article, or claim that may be made by its manufacturer, is not guaranteed or endorsed by the publisher.
Acknowledgments
We thank Xiangyang Xue for assisting and guiding in the revision of the article.
Supplementary Material
The Supplementary Material for this article can be found online at: https://www.frontiersin.org/articles/10.3389/fnut.2021.775216/full#supplementary-material
Abbreviations
MetS, metabolic syndrome; RCTs, randomized controlled trials; WC, waist circumference; SMD, standard mean difference; FBG, fasting blood glucose; TC, total cholesterol; LDL—C, low—density lipoprotein cholesterol; TG, triacylglycerol; HDL—C, high—density lipoprotein cholesterol; DBP, diastolic blood pressure; HOMA—IR, Homeostatic Model Assessment of Insulin Resistance; BMI, body mass index; SBP, systolic blood pressure; HbA1c%, hemoglobin A1c; FMT, fecal microbiota transplantation; SCFAs, short-chain fatty acids.
References
1. Bakris G, Stockert J, Molitch M, Zhou Q, Champion A, Bacher P, et al. Reduced risk of new-onset diabetes with trandolapril/verapamil-SR in patients with the metabolic syndrome. Cardiovasc J Afr. (2007) 18:190. doi: 10.1109/ICICTA.2011.226
2. Scholze J, Alegria E, Ferri C, Langham S, Stevens W, Jeffries D. et al. Epidemiological and economic burden of metabolic syndrome and its consequences in patients with hypertension in Germany, Spain and Italy; a prevalence-based model. BMC Public Health. (2010) 10:529. doi: 10.1186/1471-2458-10-529
3. Pothiwala P, Jain SK, Yaturu S. Metabolic syndrome and cancer. Metab Syndr Relat Disord. (2009) 7:279–88. doi: 10.1089/met.2008.0065
4. Hirode G, Wong RJ. Trends in the prevalence of metabolic syndrome in the United States, 2011-2016. JAMA. (2020) 323:2526–28. doi: 10.1001/jama.2020.4501
5. Sonnenburg ED, Smits SA, Tikhonov M, Higginbottom SK, Wingreen NS, Sonnenburg JL. Diet-induced extinctions in the gut microbiota compound over generations. Nature. (2016) 529:212–5. doi: 10.1038/nature16504
6. Desai MS, Seekatz AM, Koropatkin NM, Kamada N, Hickey CA, Wolter M. et al. A dietary fiber-deprived gut microbiota degrades the colonic mucus barrier and enhances pathogen susceptibility. Cell. (2016) 167:1339–53. doi: 10.1016/j.cell.2016.10.043
7. Devkota S, Wang Y, Musch MW, Leone V, Fehlner-Peach H, Nadimpalli A. et al. Dietary-fat-induced taurocholic acid promotes pathobiont expansion and colitis in Il10-/- mice. Nature. (2012) 487:104–8. doi: 10.1038/nature11225
8. Wan Y, Wang F, Yuan J, Li J, Jiang D, Zhang J, et al. Effects of dietary fat on gut microbiota and faecal metabolites, and their relationship with cardiometabolic risk factors: a 6-month randomised controlled-feeding trial. Gut. (2019) 68:1417–29. doi: 10.1136/gutjnl-2018-317609
9. Chassaing B T. Van de Wiele, De Bodt J, Marzorati M, Gewirtz AT. Dietary emulsifiers directly alter human microbiota composition and gene expression ex vivo potentiating intestinal inflammation. Gut. (2017) 66:1414–27. doi: 10.1136/gutjnl-2016-313099
10. Suez J, Korem T, Zeevi D, Zilberman-Schapira G, Thaiss CA, Maza O, et al. Artificial sweeteners induce glucose intolerance by altering the gut microbiota. Nature. (2014) 514:181–6. doi: 10.1038/nature13793
11. Lim MY, You HJ, Yoon HS, Kwon B, Lee JY, Lee S, et al. The effect of heritability and host genetics on the gut microbiota and metabolic syndrome. Gut. (2017) 66:1031–38. doi: 10.1136/gutjnl-2015-311326
12. He Y, Wu W, Wu S, Zheng HM, Li P, Sheng HF, et al. Linking gut microbiota, metabolic syndrome and economic status based on a population-level analysis. Microbiome. (2018) 6:172. doi: 10.1186/s40168-018-0557-6
13. Cani PD, Neyrinck AM, Maton N, Delzenne NM. Oligofructose promotes satiety in rats fed a high-fat diet: involvement of glucagon-like Peptide-1. Obes Res. (2005) 13:1000–7. doi: 10.1038/oby.2005.117
14. Cho WY, Hong GE, Lee HJ, Yeon SJ, Paik HD, Hosaka YZ, et al. Effect of Yogurt Fermented by Lactobacillus Fermentum TSI and L. Fermentum S2 derived from a mongolian traditional dairy product on rats with high-fat-diet-induced obesity. Foods. (2020) 9(5). doi: 10.3390/foods9050594
15. Depommier C, Everard A, Druart C, Plovier H, Van Hul M, Vieira-Silva S, et al. Supplementation with Akkermansia muciniphila in overweight and obese human volunteers: a proof-of-concept exploratory study. Nat Med. (2019) 25:1096–1103. doi: 10.1038/s41591-019-0495-2
16. Zhao C, Zhu Y, Kong B, Huang Y, Yan D, Tan H, et al. Dual-Core prebiotic microcapsule encapsulating probiotics for metabolic syndrome. ACS Appl Mater Interfaces. (2020) 12:42586–94. doi: 10.1021/acsami.0c13518
17. Canfora EE, Jocken JW, Blaak EE. Short-chain fatty acids in control of body weight and insulin sensitivity. Nat Rev Endocrinol. (2015) 11:577–91. doi: 10.1038/nrendo.2015.128
18. Bridgeman SC, Northrop W, Melton PE, Ellison GC, Newsholme P, Mamotte CDS. Butyrate generated by gut microbiota and its therapeutic role in metabolic syndrome. Pharmacol Res. (2020) 160:105174. doi: 10.1016/j.phrs.2020.105174
19. Li YT, Cai HF, Wang ZH, Xu J, Fang JY. Systematic review with meta-analysis: long-term outcomes of faecal microbiota transplantation for clostridium difficile infection. Aliment Pharmacol Ther. (2016) 43:445–57. doi: 10.1111/apt.13492
20. Hui W, Li T, Liu W, Zhou C, Gao F. Fecal microbiota transplantation for treatment of recurrent C. difficile infection: An updated randomized controlled trial meta-analysis. PLoS ONE. (2019) 14:e0210016. doi: 10.1371/journal.pone.0210016
21. Rossen NG, Fuentes S, van der Spek MJ, Tijssen JG, Hartman JH, Duflou A, et al. Findings from a randomized controlled trial of fecal transplantation for patients with ulcerative colitis. Gastroenterology. (2015) 149:110–118 e4. doi: 10.1053/j.gastro.2015.03.045
22. Costello SP, Hughes PA, Waters O, Bryant RV, Vincent AD, Blatchford P, et al. Effect of fecal microbiota transplantation on 8-week remission in patients with ulcerative colitis: a randomized clinical trial. JAMA. (2019) 321:156–64. doi: 10.1001/jama.2018.20046
23. Paramsothy S, Kamm MA, Kaakoush NO, Walsh AJ, van den Bogaerde J, Samuel D, et al. Multidonor intensive faecal microbiota transplantation for active ulcerative colitis: a randomised placebo-controlled trial. Lancet. (2017) 389:1218–28. doi: 10.1016/S0140-6736(17)30182-4
24. Mocanu V, Deehan E, Samarasinghe KK, Hotte N, Dang J, Zhang Z, et al. Fermentable vs. non-fermentable dietary fibers differentially modulate responses to fecal microbial transplantation in bariatric patients with metabolic syndrome: a single-center, randomized, double-blind, placebo-controlled pilot trial. Gastroenterology. (2020). 158:S-36-S-37. doi: 10.1016/S0016-5085(20)30775-7
25. Allegretti JR, Kassam Z, Hurtado J, Carrellas M, Marchesi J, Mullish BH, et al. Impact of fecal microbiota transplantation on prevention of metabolic syndrome among patients with obesity. Gastroenterology. (2020) 158: S-1214-S-1215. doi: 10.1016/S0016-5085(20)33696-9
26. Hutton B, Salanti G, Caldwell DM, Chaimani A, Schmid CH, Cameron C, et al. The PRISMA extension statement for reporting of systematic reviews incorporating network meta-analyses of health care interventions: checklist and explanations. Ann Intern Med. (2015) 162:777–84. doi: 10.7326/M14-2385
27. Gouni-Berthold I, Schulte DM, Krone W, Lapointe JF, Lemieux P, Predel HG, et al. The whey fermentation product malleable protein matrix decreases TAG concentrations in patients with the metabolic syndrome: a randomised placebo-controlled trial. Br J Nutr. (2012) 107:1694–706. doi: 10.1017/S0007114511004843
28. Linke A, Sonnabend M, Fasshauer M, Höllriegel R, Schuler G, Niebauer J, et al. Effects of extended-release niacin on lipid profile and adipocyte biology in patients with impaired glucose tolerance. Atherosclerosis. (2009) 205:207-213. doi: 10.1016/j.atherosclerosis.2008.11.026
29. Abutair AS. The Effect of soluble fiber supplementation on metabolic syndrome profile among newly diagnosed type 2 diabetes patients. Clin Nutr Res. (2018) 7:31-39. doi: 10.7762/cnr.2018.7.1.31
30. Dall'Alba V, Silva FM, Antonio JP, Steemburgo T, Royer CP, Almeida JC, et al. Improvement of the metabolic syndrome profile by soluble fibre—guar gum—in patients with type 2 diabetes: a randomised clinical trial. Br J Nutr. (2013) 110:1601–10. doi: 10.1017/S0007114513001025
31. Jarrar AH, Stojanovska L, Apostolopoulos V, Feehan J, Bataineh MF, Ismail LC, et al. The effect of gum arabic (Acacia senegal) on cardiovascular risk factors and gastrointestinal symptoms in adults at risk of metabolic syndrome: A randomized clinical trial. Nutrients. (2021) 13:1–10. doi: 10.3390/nu13010194
32. Johnston KL, Thomas EL, Bell JD, Frost GS, Robertson MD. Resistant starch improves insulin sensitivity in metabolic syndrome. Diabetic Medicine. (2010) 27:391–7. doi: 10.1111/j.1464-5491.2010.02923.x
33. Kassi E. Long-term effects of Stevia rebaudiana on glucose and lipid profile, adipocytokines, markers of inflammation and oxidation status in patients with metabolic syndrome. Diabetes therapy. (2016) 545-EP. doi: 10.1530/endoabs.41.EP545
34. Katcher, H.I. The effects of a whole grain– enriched hypocaloric diet on cardiovascular disease risk factors in men and women with metabolic syndrome. Am J Clin Nutr. (2008) 87:79–90. doi: 10.1093/ajcn/87.1.79
35. Lankinen M, Schwab U, Kolehmainen M, Paananen J, Poutanen K, Mykkänen H, et al. Whole grain products, fish and bilberries alter glucose and lipid metabolism in a randomized, controlled trial: the sysdimet study. PLoS ONE. (2011) 6:8. doi: 10.1371/journal.pone.0022646
36. Leão L, Aquino LA, Dias JF, Koifman RJ. Addition of oat bran reduces HDL-C and does not potentialize effect of a low-calorie diet on remission of metabolic syndrome: a pragmatic, randomized, controlled, open-label nutritional trial. Nutrition. (2019) 65:126-130. doi: 10.1016/j.nut.2019.03.007
37. Lefranc-Millot, C. Effects of a soluble dietary fiber supplementation with NUTRIOSE® on risk factors of the metabolic syndrome in Chinese male adults. Obesity Rev. (2010) 10:438.
38. Robertson MD, Wright JW, Loizon E, Debard C, Vidal H, Shojaee-Moradie F, et al. Insulin-sensitizing effects on muscle and adipose tissue after dietary fiber intake in men and women with metabolic syndrome. J Clin Endocrinol Metab. (2012) 97:3326–32. doi: 10.1210/jc.2012-1513
39. Schioldan AG, Gregersen S, Hald S, Bjørnshave A, Bohl M, Hartmann B, et al. Effects of a diet rich in arabinoxylan and resistant starch compared with a diet rich in refined carbohydrates on postprandial metabolism and features of the metabolic syndrome. Eur J Nutr. (2018) 57:795–807. doi: 10.1007/s00394-016-1369-8
40. Chang BJ, Park SU, Jang YS, Ko SH, Joo NM, Kim SI, et al. Effect of functional yogurt NY-YP901 in improving the trait of metabolic syndrome. Eur J Clin Nutr. (2011) 65:1250–5. doi: 10.1038/ejcn.2011.115
41. Leber B, Tripolt NJ, Blattl D, Eder M, Wascher TC, Pieber TR, et al. The influence of probiotic supplementation on gut permeability in patients with metabolic syndrome: An open label, randomized pilot study. Euro J Clinic Nutri. (2012) 66:1110–15. doi: 10.1038/ejcn.2012.103
42. Pan BH, Liu XJ, Shi JM, Chen YX, Xu ZH, Shi DB, et al. A meta-analysis of microbial therapy against metabolic syndrome: evidence from randomized controlled trials Dataset. (2021) 5:21. doi: 10.6084/m9.figshare.16571289
43. Tripolt NJ, Leber B, Blattl D, Eder M, Wonisch W, Scharnagl H, et al. Short communication: effect of supplementation with lactobacillus casei shirota on insulin sensitivity, β-cell function, and markers of endothelial function and inflammation in subjects with metabolic syndrome–a pilot study. J Dairy Sci. (2013) 96:89–95. doi: 10.3168/jds.2012-5863
44. Safavi M, Farajian S, Kelishadi R, Mirlohi M, Hashemipour M. The effects of synbiotic supplementation on some cardio-metabolic risk factors in overweight and obese children: a randomized triple-masked controlled trial. Int J Food Sci Nutr. (2013) 64:687–93. doi: 10.3109/09637486.2013.775224
45. Vrieze A. Transfer intestinal microbiota from lean donors increases insulin sitivity in individuals with metabolic syndrome. Gastroenterology. (2012) 143:913–6. doi: 10.1053/j.gastro.2012.06.031
46. König D, Theis S, Kozianowski G, Berg A. Postprandial substrate use in overweight subjects with the metabolic syndrome after isomaltulose (Palatinose™) ingestion. Nutrition. (2011) 28:651–6. doi: 10.1016/j.nut.2011.09.019
47. Kjølbæk L, Benítez-Páez A, Gómez del Pulgar EM, Brahe LK, Liebisch G, Matysik S, et al. Arabinoxylan oligosaccharides and polyunsaturated fatty acid effects on gut microbiota and metabolic markers in overweight individuals with signs of metabolic syndrome: a randomized cross-over trial. Clinic Nutri. (2020) 39:67–79. doi: 10.1016/j.clnu.2019.01.012
48. Gøbel RJ, Larsen N, Jakobsen M, Mølgaard C, Michaelsen KF. Probiotics to adolescents with obesity: effects on inflammation and metabolic syndrome. J Pediatr Gastroenterol Nutr. (2012) 55:673–8. doi: 10.1097/MPG.0b013e318263066c
49. Sharafedtinov KK. Hypocaloric diet supplemented with probiotic cheese improves body mass index and blood pressure indices of obese hypertensive patients—a randomized double-blind placebo-controlled pilot study. Nutri J. (2013) 12:138. doi: 10.1186/1475-2891-12-138
50. Barreto FM, Colado Simão AN, Morimoto HK, Batisti Lozovoy MA, Dichi I, Helena da Silva Miglioranza L. Beneficial effects of Lactobacillus plantarum on glycemia and homocysteine levels in postmenopausal women with metabolic syndrome. Nutrition. (2014) 30(7-8):939-942. doi: 10.1016/j.nut.2013.12.004
51. Stadlbauer V, Leber B, Lemesch S, Trajanoski S, Bashir M, Horvath A, et al. Lactobacillus casei shirota supplementation does not restore gut microbiota composition and gut barrier in metabolic syndrome: a randomized pilot study. PLoS ONE. (2015) 10:e0141399. doi: 10.1371/journal.pone.0141399
52. Bernini LJ, Simão AN, Alfieri DF, Lozovoy MA, Mari NL, de Souza CH, et al. Beneficial effects of Bifidobacterium lactis on lipid profile and cytokines in patients with metabolic syndrome: A randomized trial. effects of probiotics on metabolic syndrome. Nutrition. (2016) 32:716–9. doi: 10.1016/j.nut.2015.11.001
53. Rezazadeh L, Gargari BP, Jafarabadi MA, Alipour B. Effects of probiotic yogurt on glycemic indexes and endothelial dysfunction markers in patients with metabolic syndrome. Nutrition. (2018) 62:162–8. doi: 10.1016/j.nut.2018.12.011
54. Tenorio-Jiménez C, Martínez-Ramírez MJ, Del Castillo-Codes I, Arraiza-Irigoyen C, Tercero-Lozano M, Camacho J, et al. Lactobacillus reuteri V3401 reduces inflammatory biomarkers and modifies the gastrointestinal microbiome in adults with metabolic syndrome: the prosir study. Nutrients. (2019) 11(8). doi: 10.3390/nu11081761
55. Eslamparast T, Zamani F, Hekmatdoost A, Sharafkhah M, Eghtesad S, Malekzadeh R, et al. Effects of synbiotic supplementation on insulin resistance in subjects with the metabolic syndrome: a randomised, double-blind, placebo-controlled pilot study. Br J Nutr. (2014) 112:438–45. doi: 10.1017/S0007114514000919
56. Rabiei S, Hedayati M, Rashidkhani B, Saadat N, Shakerhossini R. The effects of synbiotic supplementation on body mass index, metabolic and inflammatory biomarkers, and appetite in patients with metabolic syndrome: a triple-blind randomized controlled trial. J Diet Suppl. (2019) 16:294–306. doi: 10.1080/19390211.2018.1455788
57. Cicero AFG, Fogacci F, Bove M, Giovannini M, Borghi C. Impact of a short-term synbiotic supplementation on metabolic syndrome and systemic inflammation in elderly patients: a randomized placebo-controlled clinical trial. Euro J Nutri. (2020) 1:8. doi: 10.1007/s00394-020-02271-8
58. Parastouei K, Saeidipoor S, Sepandi M, Abbaszadeh S, Taghdir M. Effects of synbiotic supplementation on the components of metabolic syndrome in military personnel: a double-blind randomised controlled trial. BMJ Military Health. (2020). doi: 10.1136/bmjmilitary-2020-001459
59. Thoenes M, Oguchi A, Nagamia S, Vaccari CS, Hammoud R, Umpierrez GE, et al. The effects of extended-release niacin on carotid intimal media thickness, endothelial function and inflammatory markers in patients with the metabolic syndrome. Int J Clin Pract. (2007) 61:1942–8. doi: 10.1111/j.1742-1241.2007.01597.x
60. Plaisance EP. Postprandial triglyceride responses to aerobic exercise and extended-release niacin. Am J Clin Nutr. (2008) 88:30–7. doi: 10.1093/ajcn/88.1.30
61. Bays HE, Shah A, Lin J, McCrary Sisk C, Paolini JF, Maccubbin D. Efficacy and tolerability of extended-release niacin/laropiprant in dyslipidemic patients with metabolic syndrome. J Clin Lipidol. (2010) 4:515–21. doi: 10.1016/j.jacl.2010.08.020
62. Shearer GC, Pottala JV, Hansen SN, Brandenburg V, Harris WS. Effects of prescription niacin and omega-3 fatty acids on lipids and vascular function in metabolic syndrome: a randomized controlled trial. J Lipid Res. (2012) 53:2429–35. doi: 10.1194/jlr.P022392
63. Tuteja S, Wang L, Dunbar RL, Chen J, DerOhannessian S, Marcovina SM, et al. Genetic coding variants in the niacin receptor, hydroxyl-carboxylic acid receptor 2, and response to niacin therapy. Pharmacogenet Genom. (2017) 27:285–93. doi: 10.1097/FPC.0000000000000289
64. Adiels M, Chapman MJ, Robillard P, Krempf M, Laville M, Borén J. Niacin action in the atherogenic mixed dyslipidemia of metabolic syndrome: Insights from metabolic biomarker profiling and network analysis. J Clin Lipidol. (2018) 12:810–821.e1. doi: 10.1016/j.jacl.2018.03.083
65. Aday AW, Goldfine AB, Gregory JM, Beckman JA. Impact of acipimox therapy on free fatty acid efflux and endothelial function in the metabolic syndrome: a randomized trial. Obesity. (2019) 27:1812–9. doi: 10.1002/oby.22602
66. Pan R, Xu T, Bai J, Xia S, Liu Q, Li J, et al. Effect of Lactobacillus plantarum fermented barley on plasma glycolipids and insulin sensitivity in subjects with metabolic syndrome J Food Biochem. (2020) 20:e13471. doi: 10.1111/jfbc.13471
67. Smits LP, Kootte RS, Levin E, Prodan A, Fuentes S, Zoetendal EG, et al. Effect of vegan fecal microbiota transplantation on carnitine- and choline-derived trimethylamine-n-oxide production and vascular inflammation in patients with metabolic syndrome. J Am Heart Assoc. (2018) 7:7. doi: 10.1161/JAHA.117.008342
68. Valdes AM, Walter J, Segal E. Spector role of the gut microbiota in nutrition and health. BMJ. (2018) 361:k2179. doi: 10.1136/bmj.k2179
69. Qin J, Li R, Raes J, Arumugam M, Burgdorf KS, Manichanh C, et al. A human gut microbial gene catalogue established by metagenomic sequencing. Nature. (2010) 464:59–65. doi: 10.1038/nature08821
70. Cani PD, Amar J, Iglesias MA, Poggi M, Knauf C, Bastelica D, et al. Metabolic endotoxemia initiates obesity and insulin resistance. Diabetes. (2007) 56:1761–72. doi: 10.2337/db06-1491
71. Dabke K, Hendrick G, Devkota S. The gut microbiome and metabolic syndrome. J Clin Invest. (2019) 129:4050–57. doi: 10.1172/JCI129194
72. Scheithauer TP, Dallinga-Thie GM, de Vos WM, Nieuwdorp M, van Raalte DH. Causality of small and large intestinal microbiota in weight regulation and insulin resistance. Mol Metab. (2016) 5:759–70. doi: 10.1016/j.molmet.2016.06.002
73. Kawasaki N, Asada R, Saito A, Kanemoto S, Imaizumi K. Obesity-induced endoplasmic reticulum stress causes chronic inflammation in adipose tissue. Sci Rep. (2012) 2:799. doi: 10.1038/srep00799
74. Hawkesworth S, Moore SE, Fulford AJ, Barclay GR, Darboe AA, Mark H, et al. Evidence for metabolic endotoxemia in obese and diabetic Gambian women. Nutr Diabetes. (2013) 3:e83. doi: 10.1038/nutd.2013.24
75. Cani PD, Bibiloni R, Knauf C, Waget A, Neyrinck AM, Delzenne NM, et al. Changes in gut microbiota control metabolic endotoxemia-induced inflammation in high-fat diet-induced obesity and diabetes in mice. Diabetes. (2008) 57:1470–81. doi: 10.2337/db07-1403
76. Minervini F, Algaron F, Rizzello CG, Fox PF, Monnet V, Gobbetti M. Angiotensin I-converting-enzyme-inhibitory and antibacterial peptides from Lactobacillus helveticus PR4 proteinase-hydrolyzed caseins of milk from six species. Appl Environ Microbiol. (2003) 69:5297–305. doi: 10.1128/AEM.69.9.5297-5305.2003
77. Hayes M, Stanton C, Slattery H, O'Sullivan O, Hill C, Fitzgerald GF, et al. Casein fermentate of Lactobacillus animalis DPC6134 contains a range of novel propeptide angiotensin-converting enzyme inhibitors. Appl Environ Microbiol. (2007) 73:4658–67. doi: 10.1128/AEM.00096-07
78. Flint HJ, Scott KP, Louis P, Duncan SH. The role of the gut microbiota in nutrition and health. Nat Rev Gastroenterol Hepatol. (2012) 9:577–89. doi: 10.1038/nrgastro.2012.156
79. Marchesi JR, Adams DH, Fava F, Hermes GD, Hirschfield GM, Hold G, et al. The gut microbiota and host health: a new clinical frontier. Gut. (2016) 65:330–9. doi: 10.1136/gutjnl-2015-309990
80. Nilsson NE, Kotarsky K, Owman C, Olde B. Identification of a free fatty acid receptor, FFA2R, expressed on leukocytes and activated by short-chain fatty acids. Biochem Biophys Res Commun. (2003) 303:1047–52. doi: 10.1016/S0006-291X(03)00488-1
81. Luhrs H, Gerke T, Muller JG, Melcher R, Schauber J, Boxberge F, et al. Butyrate inhibits NF-kappaB activation in lamina propria macrophages of patients with ulcerative colitis. Scand J Gastroenterol. (2002) 37:458–66. doi: 10.1080/003655202317316105
82. Tedelind S, Westberg F, Kjerrulf M, Vidal A. Anti-inflammatory properties of the short-chain fatty acids acetate and propionate: a study with relevance to inflammatory bowel disease. World J Gastroenterol. (2007) 13:2826–32. doi: 10.3748/wjg.v13.i20.2826
83. Al-Lahham SH, Roelofsen H, Priebe M, Weening D, Dijkstra M, Hoek A, et al. Regulation of adipokine production in human adipose tissue by propionic acid. Eur J Clin Invest. (2010) 40:401–7. doi: 10.1111/j.1365-2362.2010.02278.x
84. den Besten G, Lange K, Havinga R, van Dijk TH, Gerding A, van Eunen K, et al. Gut-derived short-chain fatty acids are vividly assimilated into host carbohydrates and lipids. Am J Physiol Gastrointest Liver Physiol. (2013) 305:G900–10. doi: 10.1152/ajpgi.00265.2013
85. Macfarlane GT, Macfarlane S. Fermentation in the human large intestine: its physiologic consequences and the potential contribution of prebiotics. J Clin Gastroenterol. (2011) 45:S120–7. doi: 10.1097/MCG.0b013e31822fecfe
86. Kinoshita M, Suzuki Y, Saito Y. Butyrate reduces colonic paracellular permeability by enhancing PPARgamma activation. Biochem Biophys Res Commun. (2002) 293:827–31. doi: 10.1016/S0006-291X(02)00294-2
87. Gaudier E, Jarry A, Blottiere HM, de Coppet P, Buisine MP, Aubert JP, et al. Butyrate specifically modulates MUC gene expression in intestinal epithelial goblet cells deprived of glucose. Am J Physiol Gastrointest Liver Physiol. (2004) 287:G1168–74. doi: 10.1152/ajpgi.00219.2004
88. Tolhurst G, Heffron H, Lam YS, Parker HE, Habib AM, Diakogiannaki E, et al. Short-chain fatty acids stimulate glucagon-like peptide-1 secretion via the G-protein-coupled receptor FFAR2. Diabetes. (2012) 61:364–71. doi: 10.2337/db11-1019
89. Canfora EE, van der Beek CM, Jocken JWE, Goossens GH, Holst JJ, Olde Damink SWM, et al. Colonic infusions of short-chain fatty acid mixtures promote energy metabolism in overweight/obese men: a randomized crossover trial. Sci Rep. (2017) 7: (2360). doi: 10.1038/s41598-017-02546-x
90. Tripathy D, Mohanty P, Dhindsa S, Syed T, Ghanim H, Aljada A, et al. Elevation of free fatty acids induces inflammation and impairs vascular reactivity in healthy subjects. Diabetes. (2003) 52:2882–7. doi: 10.2337/diabetes.52.12.2882
91. Ivanov S, Merlin J, Lee MKS, AJ, Murphy R, Guinamard R. Biology and function of adipose tissue macrophages, dendritic cells and B cells. Atherosclerosis. (2018) 271:102–10. doi: 10.1016/j.atherosclerosis.2018.01.018
92. Kumari M, Heeren J, Scheja L. Regulation of immunometabolism in adipose tissue. Semin Immunopathol. (2018) 40:189–202. doi: 10.1007/s00281-017-0668-3
93. Montserrat-de la Paz S, Naranjo MC, Millan-Linares MC, Lopez S, Abia R, Biessen EAL, et al. Monounsaturated fatty acids in a high-fat diet and niacin protect from white fat dysfunction in the metabolic syndrome. Mol Nutr Food Res. (2019) 63:e1900425. doi: 10.1002/mnfr.201900425
94. Kaur N, Gupta AK. Applications of inulin and oligofructose in health and nutrition. J Biosci. (2002) 27:703–14. doi: 10.1007/BF02708379
95. Roberfroid M, Gibson GR, Hoyles L, McCartney AL, Rastall R, Rowland I, et al. Prebiotic effects: metabolic and health benefits. Br J Nutr. (2010) 104 Suppl 2:S1–63. doi: 10.1017/S0007114510003363
96. Cani PD, Neyrinck AM, Fava F, Knauf C, Burcelin RG, Tuohy KM, et al. Selective increases of bifidobacteria in gut microflora improve high-fat-diet-induced diabetes in mice through a mechanism associated with endotoxaemia. Diabetologia. (2007) 50:2374–83. doi: 10.1007/s00125-007-0791-0
97. Satti M, Tanizawa Y, Endo A, Arita M. Comparative analysis of probiotic bacteria based on a new definition of core genome. J Bioinform Comput Biol. (2018) 16:1840012. doi: 10.1142/S0219720018400127
98. Karlsson FH, Tremaroli V, Nookaew I, Bergstrom G, Behre CJ, Fagerberg B, et al. Gut metagenome in European women with normal, impaired and diabetic glucose control. Nature. (2013) 498:99–103. doi: 10.1038/nature12198
99. Forslund K, Hildebrand F, Nielsen T, Falony G, Le Chatelier E, Sunagawa S, et al. Disentangling type 2 diabetes and metformin treatment signatures in the human gut microbiota. Nature. (2015) 528:262–6. doi: 10.1038/nature15766
100. Sasaki M, Ogasawara N, Funaki Y, Mizuno M, Iida A, Goto C, et al. Transglucosidase improves the gut microbiota profile of type 2 diabetes mellitus patients: a randomized double-blind, placebo-controlled study. BMC Gastroenterol. (2013) 13:81. doi: 10.1186/1471-230X-13-81
101. Gao R, Zhu C, Li H, Yin M, Pan C, Huang L, et al. Dysbiosis signatures of gut microbiota along the sequence from healthy, young patients to those with overweight and obesity. Obesity. (2018) 26:351–361. doi: 10.1002/oby.22088
102. Sáez-Lara MJ, Robles-Sanchez C, Ruiz-Ojeda FJ, Plaza-Diaz J, Gil A. Effects of probiotics and synbiotics on obesity, insulin resistance syndrome, type 2 diabetes and non-alcoholic fatty liver disease: a review of human clinical trials. Int J Mol Sci. (2016) 17:6. doi: 10.3390/ijms17060928
103. Chen JP, Chen GC, Wang XP, Qin L, Bai Y. Dietary fiber and metabolic syndrome: a meta-analysis and review of related mechanisms. Nutrients. (2017) 10(1). doi: 10.3390/nu10010024
104. Dong Y, Xu M, Chen L, Bhochhibhoya A. Probiotic foods and supplements interventions for metabolic syndromes: a systematic review and meta-analysis of recent clinical trials. Annals Nutri Metabol. (2019) 74:224–41. doi: 10.1159/000499028
Keywords: prebiotics, probiotics, synbiotics, microbial metabolites, metabolic syndrome, fecal microbiota transplantation
Citation: Pan B, Liu X, Shi J, Chen Y, Xu Z, Shi D, Ruan G, Wang F, Huang Y and Xu C (2021) A Meta-Analysis of Microbial Therapy Against Metabolic Syndrome: Evidence From Randomized Controlled Trials. Front. Nutr. 8:775216. doi: 10.3389/fnut.2021.775216
Received: 13 September 2021; Accepted: 10 November 2021;
Published: 15 December 2021.
Edited by:
Yang Liu, Shantou University, ChinaReviewed by:
Randa Salah Gomaa Mahmoud, Zagazig University, EgyptHugo Tadashi Kano, São Paulo State University, Brazil
Copyright © 2021 Pan, Liu, Shi, Chen, Xu, Shi, Ruan, Wang, Huang and Xu. This is an open-access article distributed under the terms of the Creative Commons Attribution License (CC BY). The use, distribution or reproduction in other forums is permitted, provided the original author(s) and the copyright owner(s) are credited and that the original publication in this journal is cited, in accordance with accepted academic practice. No use, distribution or reproduction is permitted which does not comply with these terms.
*Correspondence: Changlong Xu, xchlong@163.com; Yingpeng Huang, 171121477@qq.com
†These authors share first authorship