- 1State Key Laboratory of Animal Nutrition, College of Animal Science and Technology, China Agricultural University, Beijing, China
- 2Adaptation Physiology Group, Department of Animal Sciences, Wageningen University and Research, Wageningen, Netherlands
The aim of this study was to investigate the effects of the high level of xylooligosaccharides (XOS) on growth performance, antioxidant capability, immune function, and fecal microbiota in weaning piglets. The results showed that 28 d body weight exhibited linear and quadratic increases (P < 0.05) with increasing dietary XOS level, as well as average daily feed intake (ADFI) on d 15–28, average daily gain (ADG) on d 15–28 and 1–28. There was a linear decrease (P < 0.05) between XOS levels and feed conversion rate (FCR) on d 1–14 and 1–28. Additionally, glutathione peroxidase (GSH-Px) showed a linear increase (P < 0.05), while the malondialdehyde (MDA) level decreased linearly and quadratically (P < 0.05) with the increasing dietary level of XOS. Moreover, the XOS treatments markedly increased the levels of immunoglobulin A (Ig A) (linear, P < 0.05; quadratic, P < 0.05), IgM (quadratic, P < 0.05), IgG (linear, P < 0.05), and anti-inflammatory cytokine interleukin-10 (IL-10) (quadratic, P < 0.05) in serum, while the IL-1β (linear, P < 0.05; quadratic, P < 0.05) and IL-6 (linear, P < 0.05) decreased with increasing level of XOS. Microbiota analysis showed that dietary supplementation with 1.5% XOS decreased (P < 0.05) the α-diversity and enriched (P < 0.05) beneficial bacteria including Lactobacillus, Bifidobacterium, and Fusicatenibacter at the genus level, compared with the control group. Importantly, linearly increasing responses (P < 0.05) to fecal acetate, propionate, butyrate, and total short-chain fatty acids (SCFAs) were observed with increasing level of XOS. Spearman correlation analyses found that Lactobacillus abundance was positively correlated with ADG, acetate, propionate, and IgA (P < 0.05), but negatively correlated with IL-1β (P < 0.05). Bifidobacterium abundance was positively related with ADFI, total SCFAs, IgG, and IL-10 (P < 0.05), as well as g_Fusicatenibacter abundance with ADFI, total SCFAs, and IL-10. However, Bifidobacterium and Fusicatenibacter abundances were negatively associated with MDA levels (P < 0.05). In summary, dietary supplementation with XOS can improve the growth performance in weaning piglets by increasing antioxidant capability, enhancing immune function, and promoting beneficial bacteria counts.
Introduction
In modern swine production, weaning is a critical period for piglets to encounter multiple stressors including environmental, dietary, and social changes (1). Due to immunological and physiological immaturity, weaning results in stress syndromes in piglets including transient anorexia, unbalanced gut microbiota, increased diarrhea incidence, and decreased growth performance (2). China is the biggest porker producer and consumer in the world but about 24 million piglets die from diarrhea caused by bacterial infection after weaning each year (3, 4). Thus, antibiotics were widely applied to prevent pathogenic infection and promote growth in the postweaning period (5). However, the antibiotic growth promoters have been limited to being used in swine industry in China due to the adverse effects of antibiotics resistance and residue, which pose a great threat to human health and food security (3). To maintain piglet health during the weaning period and preserve public health, it is urgent to find and develop antibiotic alternatives.
The growing evidence suggested prebiotics as preferable alternatives to antibiotics (6). Prebiotics are non-digestible substrates that improve host health by selectively stimulating growth or activity of specific bacteria (7). At present, commercial prebiotics are mainly functional oligosaccharides, such as galactooligosaccharides (GOS), mannanoligosaccharides (MOS), fructooligosaccharides (FOS), and xylooligosaccharides (XOS) (5, 6). The XOS are produced from hydrolysis of xylan, which widely exist in plant cell wall and various agricultural waste or byproducts. The XOS are composed of 2–10 xylose monomers linked through β-(1,4) linkages with acidity and temperature stability, and non-toxicity (8–10). Many researches indicated that dietary supplementation of XOS improve the animal growth performance in weaning piglets and broiler chicken by enhancing immune functions, elevating antioxidant activity, increasing nutrient digestibility, and maintaining intestinal morphology and barrier (4, 11–14). In addition, some studies found that XOS are able to increase short-chain fatty acids (SCFAs) concentrations in broiler chicken or weaning piglets (4, 15), stimulate proliferation of Lactobacillus, and inhibit the growth of Escherichia coli in weaning piglets (4, 16). Christensen et al. (17) found that Bifidobacteriaceae varied approximately 10,000-fold from 0.001 to 10.7% in rats after consumption of 10% XOS. However, the abundance of Bifidobacterium had no remarkable increase at the low dosage of XOS in pigs (4, 11, 18).
Whether the high level of XOS can promote Bifidobacterium microbiota in weaning piglets remains unknown. Meanwhile, the effects of the high level of XOS on growth performance, antioxidant capability, and immune function have not been fully studied in weaning piglets. Therefore, the objective of this study was to investigate the high level of XOS effects on growth performance, antioxidant capability, and anti-inflammatory action, and whether the high level of XOS promote Bifidobacterium abundance of weaned piglets.
Materials and Methods
This work was approved by the Animal Ethics Committee of China Agricultural University.
Animals, Housing, and Experimental Design
A total of 192 piglets (Duroc × Landrace × Large White) weaned at 30 d of age with an average initial body weight (BW) of 7.50 ± 0.8 kg were randomly divided into four groups based on BW and sex. Each group had six replicate pens with eight piglets per pen. The control group piglets were fed with a control diet without the supplementation of XOS. The XOS-treated groups piglets were received dietary supplementation of 0.75% XOS, 1.5% XOS, and 3% XOS, respectively. The XOS (P70, 70%) were provided by Longlive Biotechnology Corporation (Shandong, China). The experimental period included two feeding phases: phase 1, from d 1 to d 14 postweaning; phase 2, from d 15 to d 28 postweaning. The dietary compositions and nutrient concentrations are presented in Table 1. All diets without antibiotics were formulated to meet the nutrient requirements of National Research Council (19). The pigs were housed in an environmentally controlled room with slatted plastic flooring. The environmental temperature was maintained at 26–28°C and relative humidity was controlled at 40–50%. The pigs were given ad libitum access to feed and water throughout the trial for 28 days.
Samples Collection
Individual pig BW was recorded at the initial of the weaning and on d 14 and d 28. Pen feed intake was also measured on d 14 and d 28 of this experiment. The average daily gain (ADG), average daily feed intake (ADFI), and feed conversion rate (FCR) were calculated. The feces of pigs were scored daily for diarrhea according to the following criteria: 1 = firm and well-form feces, 2 = soft and form feces, 3 = sloppy feces and mild diarrhea, and 4 = pasty and liquid diarrhea. The incidence of diarrhea was calculated for the first 7 d after weaning. The incidence of diarrhea was calculated as follows: Diarrhea incidence (%) = (total number of pigs per pen with diarrhea)/(number of pigs per pen × 7 d) × 100%.
On d 28, eight pigs of each group were randomly selected (at least one pig per pen) to collect blood samples from precaval vein into 10 mL sterile tubes. The blood samples were centrifuged at 3,000 × g at room temperature for 10 min to obtain serum, and stored at −80°C for further analysis. Fecal samples (one pig per pen) were collected into sterile tubes on d 28 and stored at −80°C for further analysis.
Biochemical and Immunological Parameters in Blood
Serum total protein (TP), albumin (ALB), albumin globulin ratio (A/G), alanine aminotransferase (ALT), aspartate aminotransferase (AST), blood urea nitrogen (BUN), and alkaline phosphatase (ALP) were measured using automatic biochemical analyzer (Hitachi 7020, Tokyo, Japan). The total superoxide dismutase (T-SOD) activity, catalase (CAT) activity, total antioxidant capacity (T-AOC), malondialdehyde (MDA) content, and glutathione peroxidase (GSH-Px) capacity in serum were measured using commercial kits (Jiancheng Co., Ltd, Nanjing, China). The activity of T-SOD was determined at 450 nm by the xanthine oxidase method. The CAT activity was determined at 405 nm by ammonium molybdate method. The T-AOC was determined at 405 nm by 2,2′-azinobis-(3-ethylbenzthiazoline-6-sulphonate) method. The MDA content was measured at 532 nm using the thiobarbituric acid method. The activity of GSH-Px was measured at 412 nm using 2,2′-dithiodibenzoic acid method. The levels of immunoglobulin A (IgA), IgG, IgM, interleukin-1β (IL-1β), IL-6, and IL-10 in serum were detected by commercial ELISA kits (Jiancheng Co., Ltd, Nanjing, China) according to manufacturer's instructions.
Analysis of Microbiota in Feces
The total bacterial DNA of the feces was extracted using the QIAamp Fast DNA Stool Mini Kit (Qiagen, Germany) following the manufacturer's protocol. The V3–V4 hypervariable regions of bacteria 16S rDNA were amplified with universal primers 338F (ACTCCTACGGGAGGCAGCAG) and 806R (GGACTACHVGGGTWTCTAAT). The purified PCR products were equimolarly combined and paired-end sequenced on the Illumina Miseq platform (Illumina, San Diego, CA, USA).
The raw reads were demultiplexed and quality filtered by Quantitative Insights Into Microbial Ecology (QIIME) (version 1.9) software with the following criteria: (i) the reads were truncated at any site receiving an average quality score of <20 over a 50 bp sliding window, and the truncated reads shorter than 50 bp were discarded, reads containing ambiguous characters were also discarded; (ii) only overlapping sequences longer than 10 bp and the reads without more than two nucleotide mismatches in the primer were assembled. The remaining sequences with 97% similarity were clustered into the same operational taxonomic units (OTUs) using UPARSE (version 7.1) and chimeric sequences were removed. The taxonomy of each OTU representative sequence was analyzed by Ribosomal Database Project (RDP) classifier against the SILVA 16S rRNA gene database using confidence threshold of 70%. The analysis of the α-diversity was calculated by the MOTHUR program. For the β-diversity, principal coordinate analysis (PCoA) was performed based on Bray-Curtis distances. The data were analyzed on the Majorbio I-Sanger cloud platform (www.i-sanger.com).
Quantification of Specific Bacteria in Feces
The total bacterial DNA of feces was isolated as described above. The absolute copy numbers of specific bacteria were calculated as previously described (22). The primers were listed in Table 2.
Determination of SCFAs in Feces
The concentrations of SCFAs, including lactate, acetate, propionate, and butyrate in the feces were determined using Ion Chromatograph. Briefly, 0.5 g of feces was weighed and dissolved in 10 mL distilled water, homogenized using ultrasound for 30 min at room temperature, and centrifuged at 5,000 × g for 10 min to obtain the supernatants. The supernatants were diluted 10 × and filtered using 0.22 μm membranes before being detected by high-performance ion chromatography system (DIONEX ICS-3000; Thermo Fisher, USA). The injection volume was 25 μL and the flow rate was 1.0 mL/min (23).
Statistical Analysis
All the data were performed using Statistics Analysis System (SAS) 9.4 version (Cary, NC, United States). Data statistical significances were analyzed by one-way ANOVA for multiple groups. Statistical differences among the treatments were separated by Duncan's multiple tests method. The data of two groups were analyzed by unpaired Student's t-test. The incidence of diarrhea was analyzed by χ2 test. The differential bacteria were identified by using linear discriminant analysis (LDA) effect size (LEfSe) analysis. The level of statistical significance was P ≤ 0.05, whereas 0.05 < P < 0.1 was considered as a trend of significant difference. Data are presented as means ± SEM.
Results
Effects of XOS Supplementation on Growth Performance in Weaning Piglets
The dietary supplementation with the high level of XOS had no effects (P > 0.05) on 14 d BW, ADG on d 1–14, ADFI on d 1–14, and FCR on d 15–28 and 1–28 (Table 3). However, supplementation with XOS significantly increased ADG on d 15–28 and 1–28 (P < 0.05). Similarly, 28 d BW in 1.5% and 3% XOS groups had higher than the control group (P < 0.05), as well as ADFI on d 15–28. Notably, 1.5% XOS group markedly increased (P < 0.05) ADFI on d 1–28 compared with other groups, but there were no differences among the other groups (P > 0.05). Compared with the control group, the FCR on d 1–14 was markedly decreased in the 3% XOS group (P < 0.05). In addition, linear and quadratic increases (P < 0.05) were observed between different XOS levels and 28 d BW, as well as ADG on d 15–28 and 1–28, and ADFI on d 15–28 (Table 3), with the highest (P < 0.05) at the supplemental level of 1.5% XOS. ADFI on d 1–14 and 1–28 exhibited quadratic increases (P < 0.05) with the increasing dietary level of XOS. There was a linear decrease (P < 0.05) between the dietary XOS levels and FCR on d 1–14 and 1–28, with the lowest FCR (P < 0.05) on d 1–14 at the level of 3% XOS (Table 3).
Effects of XOS Supplementation on Diarrhea Incidence of Weaning Piglets
There was a decreasing trend for the incidence of diarrhea between the pigs fed with the control diet and the 3% XOS diet. However, dietary supplementation with 0.75% XOS and 1.5% XOS significantly decreased (P < 0.05) the diarrhea incidence compared with the control group in weaning piglets (Table 3).
Effects of XOS Supplementation on Serum Biochemical Parameters and Antioxidant Indictors in Weaning Piglets
The serum biochemical parameters on d 28 are presented in Table 4. The XOS treatments had no effects on serum biochemical parameters on d 28 in weaning pigs (Table 4).
The serum T-SOD, CAT, T-AOC, GSH-PX, and MDA were measured to evaluate the effects of XOS on antioxidant capability (Table 5). The results showed that dietary supplementation with XOS failed to affect T-SOD, CAT, and T-AOC activities (P > 0.05) except for GSH-Px and MDA (P < 0.05). Compared with the control group, both 1.5 and 3% XOS groups significantly increased the GSH-Px level. However, the XOS treatments significantly declined (P < 0.05) the MDA level compared with the control group, and the diet supplemented with 1.5% XOS (P < 0.05) had the lowest MDA concentration among the four groups. Besides, the GSH-Px showed a linear increase (P < 0.05) with the increasing dietary level of XOS, but MDA levels decreased linearly and quadratically (P < 0.05).
Effects of XOS Supplementation on Immunological Function and Inflammatory Cytokines in Weaning Piglets
The serum levels of IgM, IgG, IgA, IL-1β, IL-6, and IL-10 were determined to evaluate the effects of XOS on immune status. As shown in Table 6, quadratic responses (P < 0.05) in serum levels of IgM and IL-10 were observed with the increasing supplemental XOS level. Addition of 0.75% XOS and 1.5% XOS had higher (P < 0.05) level of IgM than the control group. 1.5% XOS group also significantly increased IL-10 level compared with control group (P < 0.05). There were linear responses in serum levels of IgG and IL-6 (P < 0.05) with the increasing supplemental XOS level in the diets. Compared with the control group, 1.5 and 3% XOS groups significantly increased (P < 0.05) the IgG concentration in serum. However, the XOS-treated pigs remarkedly decreased (P < 0.05) serum level of IL-6, while no differences (P > 0.05) were found in XOS treatments. Additionally, IL-1β showed linear and quadratic (P < 0.05) responses with the increasing level of XOS in the diets, with the lowest (P < 0.05) serum concentration of IL-1β at the supplemental level of 1.5% XOS. Moreover, the XOS treatments significantly increased (P < 0.05) the level of IgA compared with control groups. The different levels of XOS showed linear and quadratic (P < 0.05) effects on IgA.
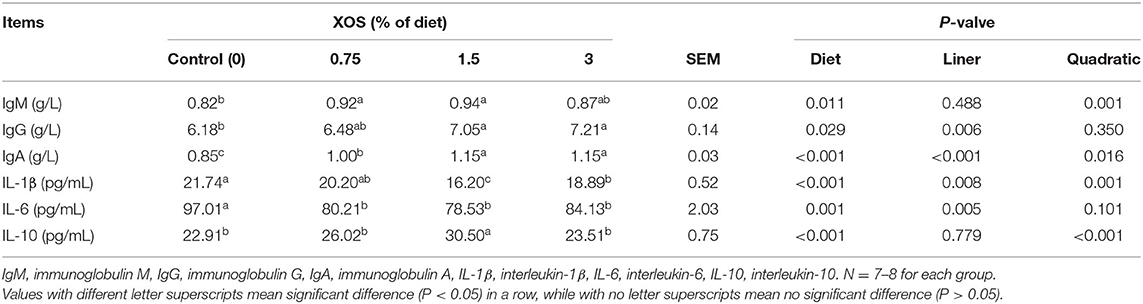
Table 6. The effects of XOS supplementation on immunological function and inflammatory cytokines of weaning piglets.
Effects of XOS Supplementation on Fecal Microbiota in Weaning Piglets
Next, the microbiota of fecal samples in the control group and 1.5% XOS group was analyzed. As shown in Table 7, the alpha diversity was evaluated. The Shannon index had a significant decrease (P < 0.05) in the 1.5% XOS group compared with the control group, whereas the Simpson index had a significant increase in the 1.5% XOS group (P < 0.05). However, there were no impacts of 1.5% XOS on Sobs (P > 0.05), Ace, and Chao indices compared with the control group.
Bata-diversity was assessed using PCoA plots based on Bray-Curtis distances. The fecal microbiota composition in the 1.5% XOS group could obviously separate from the control group (P < 0.05, Figure 1A). At the phylum level, Firmicutes, Bacteroidota, and Actinobacteriota predominated in feces (Figure 1B). At the genus level, Lactobacillus was dominant in both groups (Figure 1C). The relative abundances of Lactobacillus and Bifidobacterium were remarkedly increased (P < 0.05) in the 1.5% XOS group (Figure 1D), while the relative abundances of g_Clostridia_UCG-014, g__Ruminococcus, g__norank_f__Ruminococcaceae, and g__norank_f__Eubacterium_coprostanoligenes_group in the 1.5% XOS group were markedly lower (P < 0.05) than the control group (Figure 1D). The differential bacteria were identified by using linear discriminant analysis (LDA) effect size (LEfSe). A dietary supplemented with 1.5% XOS was associated with increased relative abundances of Lactobacillus, Bifidobacterium, and Fusicatenibacter at the genus level in weaning piglets (Figure 1E, LDA score >2).
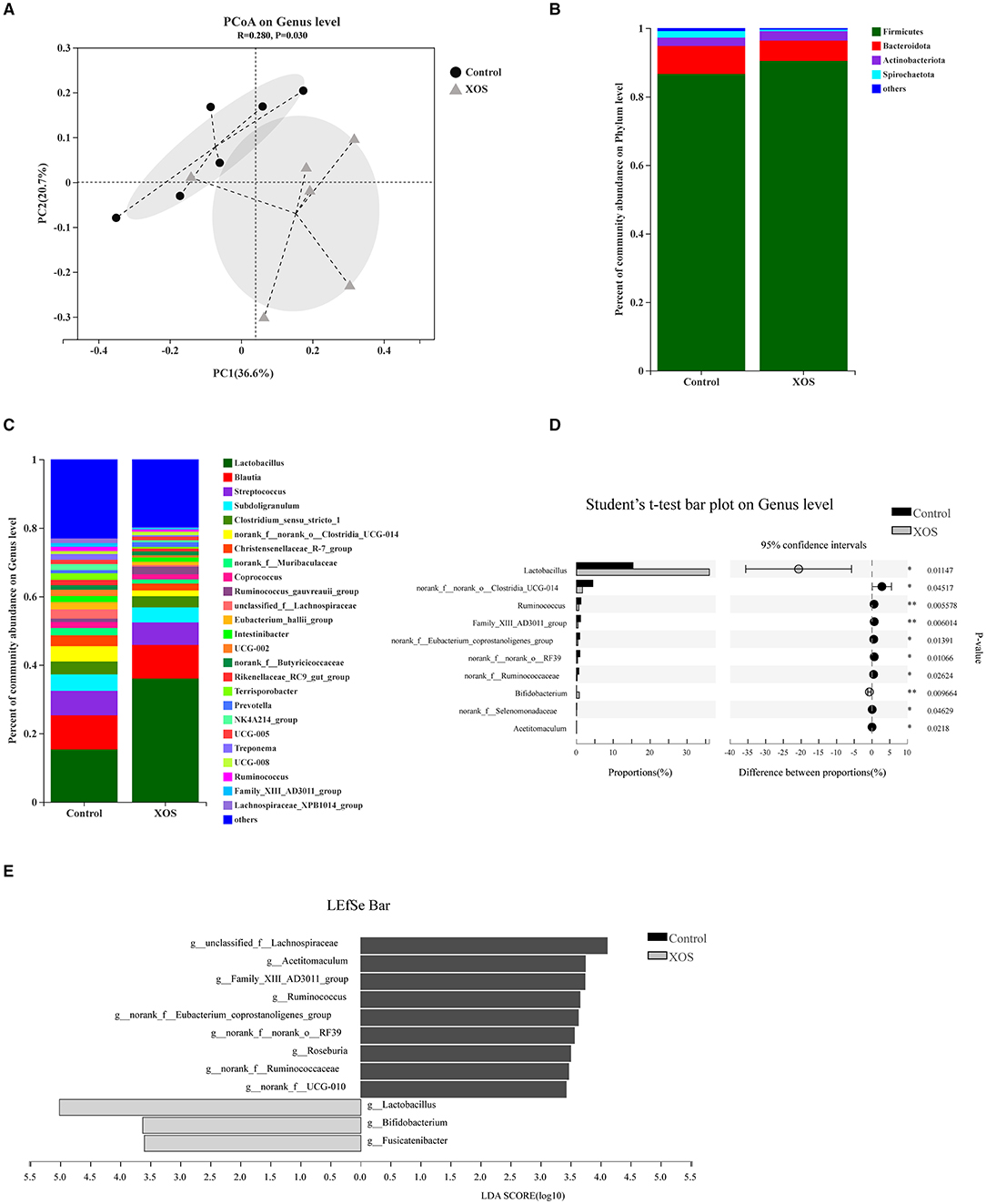
Figure 1. Dietary supplementation with xylooligosaccharides (XOS) modulates the composition of fecal microbiota. (A) Principal coordinate analysis (PCoA) on genus level based on Bray-Curtis distances. Composition of bacterial communities (B) at the phylum level and (C) at the genus level. (D) Differential bacteria at the genus level. (E) Linear discriminant analysis (LDA) effect size (LEfSe) analysis screened biomarker of microbial community after addition of XOS. *P < 0.05 and **P < 0.01, n = 6 for each group.
Quantification of Specific Bacteria in Feces
Compared with the control group, the pigs fed with a diet addition of 1.5% XOS markedly increased (P < 0.05) the copy numbers of Bifidobacterium and Lactobacillus in feces (Figure 2). However, 1.5% XOS did not differ (P > 0.05) the total bacteria, Salmonella, and E. coli (Figure 2).
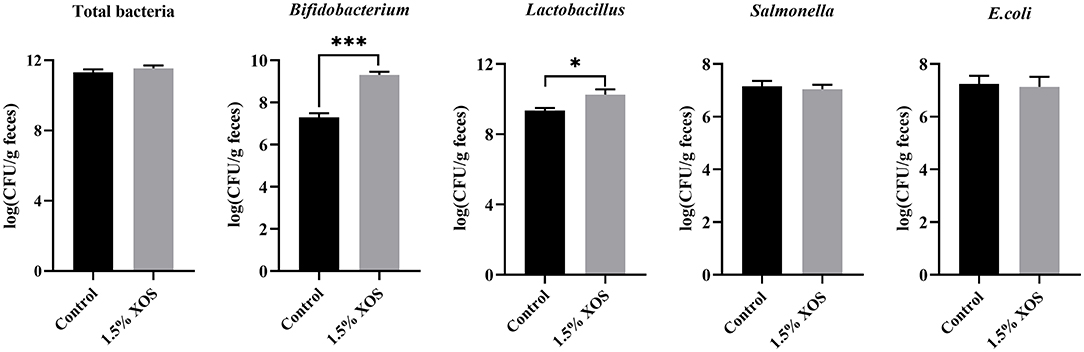
Figure 2. Effect of XOS on specific bacteria in feces. Data are presented as means + SEM. *P < 0.05 and ***P < 0.001, n = 6 for each group.
Effects of XOS Supplementation on Fecal SCFAs
The fecal SCFAs concentrations in response to different dosages of XOS supplementation on d 28 are shown in Table 8. Linearly increasing responses (P < 0.05) to the fecal acetate, propionate, butyrate, and total SCFAs were observed with the increasing supplemental XOS level. A dietary supplemented with 3% XOS had higher (P < 0.05) concentrations of acetate and propionate in feces than the control group, while there were no differences among in control, 0.75%, and 1.5% XOS groups or among the XOS treatments. Besides that, the supplementation of XOS markedly increased (P < 0.05) concentrations of butyrate and total SCFAs in feces compared with the control group, but no differences were noted in the XOS-treated groups (P > 0.05).
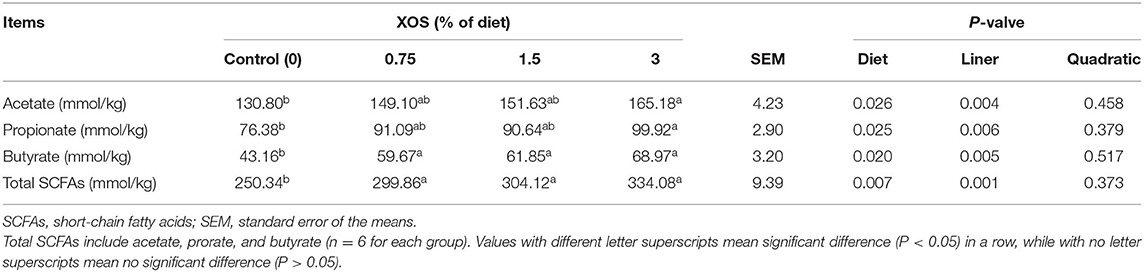
Table 8. The effects of dietary supplementation with XOS on SCFAs concentration in feces of weaning piglets.
Correlation of Fecal Microbiota With Serum Parameters, SCFAs, and Growth Performance
As shown in Figure 3, the genus Lactobacillus abundance was positively correlated with ADG, acetate, propionate, and IgA (P < 0.05), but negatively correlated with IL-1β (P < 0.05). The genus Bifidobacterium was positively related with ADFI, total SCFAs, IgG, and IL-10 (P < 0.05), as well as g_Fusicatenibacter abundance with ADFI, total SCFAs, and IL-10 (P < 0.05). However, the genus Bifidobacterium and Fusicatenibacter abundances were negatively associated with MDA (P < 0.05). Meanwhile, the genus Bifidobacterium abundance was negatively associated with IL-1β and IL-6 (P < 0.05). The genus g_Ruminococcus, g__Family_XIII_AD3011_group, and g__norank_f__norank_o__RF39 were negatively correlated with ADG, acetate, propionate, and butyrate. In addition, there was a positive correlation between g_Ruminococcus and MDA level (P < 0.05), while a remarkable negative correlation was found between g_Ruminococcus and total SCFAs, and IL-10 levels (P < 0.05). There were similar results for g__Family_XIII_AD3011_group, g__norank_f__norank_o__RF39, and g__norank_f__Eubacterium_coprostanoligenes_group. Moreover, a positive correlation was observed between g__norank_f__norank_o__RF39 abundance and IL-6 (P < 0.05), as well as Clostridia_UCG-014, g__Family_XIII_AD3011_group, g__norank_f__norank_o__RF39 abundance, and IL-1β level.
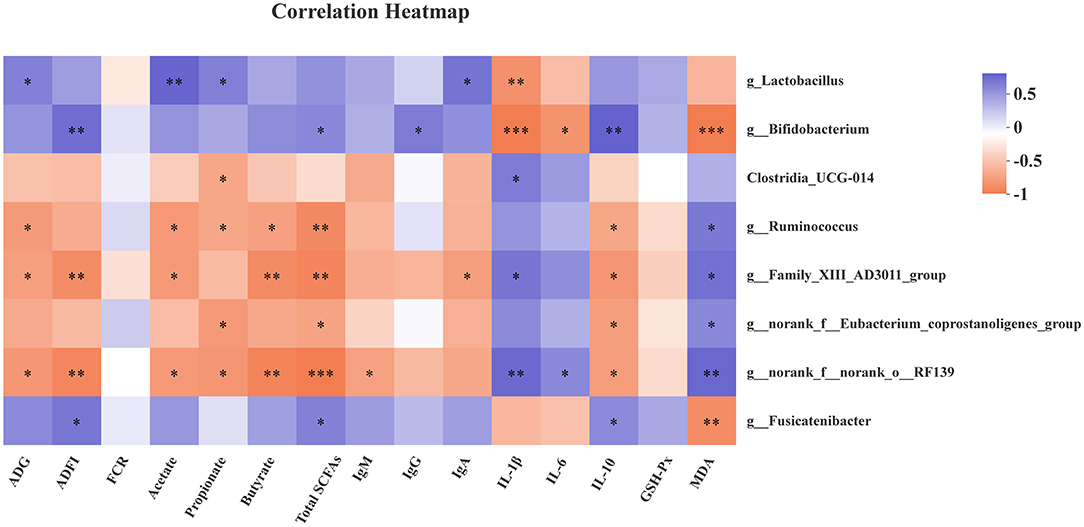
Figure 3. Correlation heatmap between microbiota and antioxidant capability, immune function, growth performance, and fecal SCFAs. Spearman correlations were applied. ADG, average daily gain; ADFI, average daily feed intake; FCR, feed conversion rate; Total SCFAs, total short-chain fatty acids; GSH-Px, glutathione peroxidase; MDA, malondialdehyde. Total SCFAs = acetate + propionate + butyrate. *P < 0.05, **P < 0.01, and ***P < 0.001.
Discussions
Recently, the XOS as functional food are widely used in animal diets for improving animal growth performance, enhancing the quality of animal products, and regulating intestinal health (24). However, the abundance of Bifidobacterium did not show a remarkable increase at the low dosage of XOS in weaning pigs or growing pigs (4, 11, 18). In the present study, we investigated the effects of the high level of XOS on growth performance, antioxidant capability, and anti-inflammatory action, and whether the high level of XOS promotes Bifidobacterium abundance of weaning piglets. We found that the diet supplemented with 1.5% XOS significantly increased final BW, ADG, and ADFI during d 15–28 and the whole study period. Moreover, 28 d BW exhibited linear and quadratic responses (P < 0.05) with the increasing dietary XOS level, as well as ADFI on d 15–28, ADG on d 15–28 and 1–28, with the highest at the level of 1.5% XOS. As the dietary XOS level increased, ADFI on d 1–14 and 1–28 increased quadratically. Besides, the FCR on d 1–14 and 1–28 decreased linearly with increasing level of XOS in the diets. The 3% XOS group piglets had a lower ADFI and ADG than the 1.5% XOS group, which due to an excessive level of XOS may affect the dietary palatability. The previous studies showed that supplementation of XOS improved animal growth performance. Chen et al. (4, 13) found that an addition of 500 mg/kg XOS significantly increased BW, ADG, and decreased FCR in weaning piglets. Similarly, a dietary supplementation with 400 mg/kg XOS also increased final BW and ADG in nursery pigs, while ADFI and FCR were not affected (12). Additionally, a dietary supplementation of 250 mg/kg XOS markedly increased ADG and ADFI for weaning piglets (25). The difference between our findings and previous reports might be attributable to the dosage of XOS.
It has been reported that oligosaccharides may influence serum biochemical parameters (26). Our results showed that either the high level or the low level of XOS failed to affect serum biochemical parameters in weaning pigs. Consistently, the previous studies indicated that serum biochemical parameters were not altered in XOS-treated weaning pigs or limited effect in XOS-treated nursery pigs (11, 12, 27).
The CAT, SOD, and GSH-Px as the main antioxidant enzymes in the antioxidant protection system play a vital role in scavenging free radicals, reducing and eliminating oxidative damage. The serum T-AOC is an important indicator for evaluating the overall function of antioxidant capability in vivo. The MDA is considered as a biomarker for lipid peroxidation under the oxidative stress status. In this study, serum GSH-Px concentration increased linearly with increasing supplemental XOS level in weaning pigs. In addition, there were linear and quadratic responses between serum MAD level and XOS, with the lowest at the 1.5% XOS. The XOS had no impact on serum T-SOD, CAT, and T-AOC in our findings. Consistently, dietary addition of 400 mg/kg XOS markedly declined the serum MDA level and increased a trend of GSH-Px (12). Chen et al. (13) also reported that piglets fed with the diet of 500 mg/kg XOS had higher T-SOD and CAT levels on day 28, whereas the MDA level was lower than the control group. Moreover, T-SOD, T-AOC, and CAT levels exhibited quadratic increases with different dietary XOS levels on day 28, but the level of MDA was decreased quadratically. A recent report also found that maternal supplementation of 500 mg/kg XOS in diet had a lower level of MDA in sucking pigs, while did not affect the SOD, T-AOC, and CAT activity (28). These results indicated that XOS improvement of growth performance of weaned piglets might be related to increasing the antioxidant capability.
The immunoglobulins, including IgG, IgM, and IgA involve host immune response and protect against pathogens and virus infection. The previous studies showed that XOS can elevate the serum level of IgG in nursery pigs and IgM in broilers (12, 29). Notably, with the level of XOS increased, the serum IgA had a linear increase and IgM showed linear and quadratic responses in laying hens (30). Besides, 500 mg/kg XOS increased the concentration of serum IgG on day 28 in weaning piglets, and serum IgG concentration showed a quadratic effect with increasing XOS level in diet (13). Similarly, our results found that a dietary supplementation of 0.75 and 1.5% XOS significantly increased the serum IgM concentration in weaning piglets compared with the control group. Additionally, 1.5% and 3% XOS had higher levels of IgG than the control group. Moreover, the serum level of IgA was increased later in XOS-treated pigs. Numerous studies indicated that XOS can alleviate inflammatory status in animals. Sow supplementation of 500 mg/kg XOS decreased serum IL-1β, IL-6, IL-2, and Tumor Necrosis Factor (TNF)-α levels in sucking piglets (28). In the present study, 1.5 and 3% XOS significantly decreased the serum IL-1β compared with the control group. The XOS-treated piglets had a lower serum level of IL-6 than the vehicle group. Furthermore, anti-inflammatory cytokine IL-10 was markedly increased in the 1.5% XOS group. Notably, the effects of XOS supplementation could depend on the dosage, since Yin et al. (11) found XOS at 100 mg/kg only decreased the serum IFN-γ in weaning piglets, but had no impacts on IL-1β, IL-6, and IL-10. Hansen et al. (31) reported that a diet supplemented with 10% XOS downregulated the IFN-γ and IL-1β in mice. Importantly, XOS suppressed the pro-inflammatory cytokines, IL-1β, IL-6, and TNF-α secretion from RAW265.7 macrophages stimulated with lipopolysaccharide in the pretreatment model (32). The possible mechanism responsible for the beneficial effects is that XOS can enhance immune function and decrease the inflammatory status in weaning piglets.
It has been widely demonstrated that XOS can modulate gut microbiota composition by selectively stimulating beneficial bacteria. Thus, the microbiota composition of fecal samples was analyzed by 16S rRNA gene sequencing. The result found that 1.5% XOS significantly decreased the Shannon index and increased the Simpson index, indicating dietary supplementation of XOS decreased the diversity of gut microbiota community. Similarly, the addition of 250 mg/kg XOS significantly decreased the Shannon index during growing fatty pigs (16). The XOS decreased α-diversity Chao1 and Shannon indices in high-fat diet-induced mice as well (33). The α-diversity decrease might result from increasing the relative abundances of Lactobacillus and Bifidobacterium in XOS-treated pigs. The PCoA plots based on Bray-Curtis distances were a clear separation between XOS and control groups, which is similar to that reported by Pan et al. (16). It has been reported that XOS can promote counts of Lactobacillus in weaning piglets (4, 16), while the low level of XOS failed to stimulate the abundance of Bifidobacterium (4, 11, 18, 34). In the current study, the abundances of Lactobacillus and Bifidobacterium were markedly increased in 1.5% XOS-treated pigs using l6S rRNA gene sequencing, as well as by qPCR. Notably, 1.5% XOS elevated the relative Bifidobacterium abundance approximately 35-fold from 0.021 to 0.74%. The high level of XOS did not markedly vary Bifidobacterium abundance in weaning piglets compared to rodents (17), probably due to the differences of microbial stability and physiological backgrounds between animal species. Holman et al. (35) analysis of core microbiota in the swine gut revealed that Clostridium, Blautia, Lactobacillus, Prevotella, Ruminococcus, Roseburia, the RC9 gut group, and Subdoligranulum were considered as core microbiota but the relative abundance of Bifidobacterium in fecal samples remained low (<0.35%). Moreover, the XOS treatments linearly increased the fecal acetate, propionate, butyrate, and total SCFAs levels in weaning piglets. Chen et al. (4) also found that the dietary supplementation of 500 mg/kg XOS elevated the acetate, propionate, butyrate, and total SCFAs concentrations in the cecum of piglets. Furthermore, the Lactobacillus, Bifidobacterium, and Fusicatenibacter were regarded as biomarkers in XOS-treated pigs identified by LEfSe analysis (LDA > 2). Collectively, the high level of XOS can alter the fecal microbiota composition and increase the concentrations of SCFAs by promoting the beneficial bacteria.
The growing evidence has shown that Lactobacillus and Bifidobacterium are associated with SCFAs production, antioxidant activity, and host inflammatory status (36–39). Thus, spearman correlations between bacteria and antioxidant, immune function, growth performance, and fecal SCFAs were performed. The Lactobacillus exhibited positive correlations with ADG, acetate, propionate, and serum IgA level, but a negative correlation with serum IL-1β level. The Bifidobacterium was positively associated with ADFI, total SCFAs, IgG, and IL-10, while negatively associated with levels of IL-1β, IL-6, and MDA in serum. The recent reports indicated that Fusicatenibacter is a SCFAs-producing bacteria, which closely related to host health, such as Parkinson's disease, ulcerative colitis (40–44). The Fusicatenibacter suppresses intestinal inflammation and is positively associated with SCFAs production (41). Consistently, there were positive correlations between Fusicatenibacter and ADFI, total SCFAs, and IL-10 level, while a negative correlation was observed between Fusicatenibacter and MDA in our findings. However, the bacteria with higher abundances in the control group, like g_Ruminococcus, g__Family_XIII_AD3011_group, and g__norank_f__norank_o__RF39, were in negative relations with ADG, ADFI, SCFAs, and IL-10, and in positive relations with IL-1β and MDA.
Conclusions
In conclusion, our results indicated that XOS supplementation improved the growth performance, increased antioxidant capability, enhanced immune function, and decreased body inflammatory status and incidence of diarrhea in weaning piglets, which could be contributed to the modulation of gut microbiota community, especially by increasing the abundances of Lactobacillus, Bifidobacterium, and Fusicatenibacter. However, the high level of XOS did not sharply the relative abundance of Bifidobacterium in feces. The optimum level of XOS supplementation was 1.5% for weaning piglets in this study.
Data Availability Statement
The datasets presented in this study can be found in online repositories. The names of the repository/repositories and accession number(s) can be found below: NCBI SRA; PRJNA762151.
Ethics Statement
The animal study was reviewed and approved by Animal Ethics Committee of China Agricultural University.
Author Contributions
JP wrote the manuscript. JP, XZ, YW, and DL performed the experiments. HY revised the manuscript. ZW analyzed the data. JW obtained financial support and oversaw this study. JP, DH, and JW designed the research. All authors contributed to the article and approved the submitted version.
Funding
This research was funded by the National Natural Science Foundation of China (31972596, 31630074, 31902170), the Beijing Municipal Natural Science Foundation (S170001), the China Agriculture Research System (CARS-35), and the Fundamental Research Funds for the Central Universities (2021TC089).
Conflict of Interest
The authors declare that the research was conducted in the absence of any commercial or financial relationships that could be construed as a potential conflict of interest.
Publisher's Note
All claims expressed in this article are solely those of the authors and do not necessarily represent those of their affiliated organizations, or those of the publisher, the editors and the reviewers. Any product that may be evaluated in this article, or claim that may be made by its manufacturer, is not guaranteed or endorsed by the publisher.
Acknowledgments
The experiments were conducted at the swine research unit of China Agricultural University (Chengde, China).
References
1. Campbell JM, Crenshaw JD, Polo J. The biological stress of early weaned piglets. J Anim Sci Biotechnol. (2013) 4:19. doi: 10.1186/2049-1891-4-19
2. Gresse R, Chaucheyras-Durand F, Fleury MA, Van De Wiele T, Forano E, et al. Gut microbiota dysbiosis in postweaning piglets: Understanding the keys to health. Trends Microbiol. (2017) 25:851–73. doi: 10.1016/j.tim.2017.05.004
3. Collignon P, Voss A. China, what antibiotics and what volumes are used in food production animals? Antimicrob Resist Infect Control. (2015) 4:16. doi: 10.1186/s13756-015-0056-5
4. Chen Y, Xie Y, Zhong R, Liu L, Lin C, Xiao L, et al. Effects of xylo-oligosaccharides on growth and gut microbiota as potential replacements for antibiotic in weaning piglets. Front Microbiol. (2021) 12:641172. doi: 10.3389/fmicb.2021.641172
5. Barton MD. Impact of antibiotic use in the swine industry. Curr Opin Microbiol. (2014) 19:9–15. doi: 10.1016/j.mib.2014.05.017
6. Cunningham M, Azcarate-Peril MA, Barnard A, Benoit V, Grimaldi R, Guyonnet D, et al. Shaping the future of probiotics and prebiotics. Trends Microbiol. (2021) 29:667–85. doi: 10.1016/j.tim.2021.01.003
7. Gibson GR, Roberfroid MB. Dietary modulation of the human colonic microbiota: introducing the concept of prebiotics. J Nutr. (1995) 125:1401–12. doi: 10.1093/jn/125.6.1401
8. Gao Y, Zhang S, Li C, Xiao L, Shen J, Yin J. Acute and subchronic toxicity of xylo-oligosaccharide in mice and rats. Toxicol Mech Methods. (2012) 22:605–10. doi: 10.3109/15376516.2012.706837
9. Gao Y, Wang Y, Li Y, Han R, Li C, Xiao L, et al. Repeated sub-chronic oral toxicity study of xylooligosaccharides (XOS) in dogs. Regul Toxicol Pharmacol. (2017) 86:379–85. doi: 10.1016/j.yrtph.2017.04.009
10. Amorim C, Silverio SC, Prather KLJ, Rodrigues LR. From lignocellulosic residues to market: Production and commercial potential of xylooligosaccharides. Biotechnol Adv. (2019) 37:107397. doi: 10.1016/j.biotechadv.2019.05.003
11. Yin J, Li F, Kong X, Wen C, Guo Q, Zhang L, et al. Dietary xylo-oligosaccharide improves intestinal functions in weaned piglets. Food Funct. (2019) 10:2701–9. doi: 10.1039/C8FO02485E
12. Hou Z, Wu D, Dai Q. Effects of dietary xylo-oligosaccharide on growth performance, serum biochemical parameters, antioxidant function, and immunological function of nursery piglets. Rev Bras Zootecn. (2020) 49:e20190170. doi: 10.37496./rbz4920190170
13. Chen Y, Xie Y, Zhong R, Han H, Liu L, Chen L, et al. Effects of graded levels of xylo-oligosaccharides on growth performance, serum parameters, intestinal morphology, and intestinal barrier function in weaned piglets. J Anim Sci. (2021) 99:1–8. doi: 10.1093./jas/skab183
14. Wang Q, Wang XF, Xing T, Li JL, Zhu XD, Zhang L, et al. The combined impact of xylo-oligosaccharides and gamma-irradiated Astragalus polysaccharides on growth performance and intestinal mucosal barrier function of broilers. Poult Sci. (2021) 100:100909. doi: 10.1016/j.psj.2020.11.075
15. Craig AD, Khattak F, Hastie P, Bedford MR, Olukosi OA. Xylanase and xylo-oligosaccharide prebiotic improve the growth performance and concentration of potentially prebiotic oligosaccharides in the ileum of broiler chickens. Br Poult Sci. (2020) 61:70–8. doi: 10.1080/00071668.2019.1673318
16. Pan J, Yin J, Zhang K, Xie P, Ding H, Huang X, et al. Dietary xylo-oligosaccharide supplementation alters gut microbial composition and activity in pigs according to age and dose. AMB Express. (2019) 9:134. doi: 10.1186/s13568-019-0858-6
17. Christensen EG, Licht TR, Leser TD, Bahl MI. Dietary xylo-oligosaccharide stimulates intestinal Bifidobacteria and Lactobacilli but has limited effect on intestinal integrity in rats. BMC Res Notes. (2014) 7:660. doi: 10.1186/1756-0500-7-660
18. Sutton TA, O'neill HVM, Bedford MR, Mcdermott K, Miller HM. Effect of xylanase and xylo-oligosaccharide supplementation on growth performance and faecal bacterial community composition in growing pigs. Anim Feed Sci Tech. (2021) 274:114822–33. doi: 10.1016./j.anifeedsci.2021.114822
19. National Research Council (NRC). (2012). Nutrient Requirements of Swine. 11th rev. ed. Washington DC: National Academies Press.
20. Chen H, Mao X, He J, Yu B, Huang Z, Yu J, et al. Dietary fibre affects intestinal mucosal barrier function and regulates intestinal bacteria in weaning piglets. Br J Nutr. (2013) 110:1837–48. doi: 10.1017/S0007114513001293
21. Cohen HJ, Mechanda SM, Lin W. PCR amplification of the fimA gene sequence of Salmonella Typhimurium, a specific method for detection of Salmonella spp. Appl Environ Microbiol. (1996) 62:4303–8. doi: 10.1128/aem.62.12.4303-4308.1996
22. Li N, Huang S, Jiang L, Dai Z, Li T, Han D, et al. Characterization of the early life microbiota development and predominant Lactobacillus species at distinct gut segments of low- and normal-birth-weight piglets. Front Microbiol. (2019) 10:797. doi: 10.3389/fmicb.2019.00797
23. He B, Li T, Wang W, Gao H, Bai Y, Zhang S, et al. Metabolic characteristics and nutrient utilization in high-feed-efficiency pigs selected using different feed conversion ratio models. Sci China Life Sci. (2019) 62:959–70. doi: 10.1007/s11427-018-9372-6
24. Baker JT, Duarte ME, Holanda DM, Kim SW. Friend or Foe? Impacts of dietary xylans, xylooligosaccharides, and xylanases on intestinal health and growth performance of monogastric animals. Animals (Basel). (2021) 11:609. doi: 10.3390/ani11030609
25. Ding H, Zhao X, Azad MaK, Ma C, Gao Q, et al. Dietary supplementation with Bacillus subtilis and xylo-oligosaccharides improves growth performance and intestinal morphology and alters intestinal microbiota and metabolites in weaned piglets. Food Funct. (2021) 12:5837–49. doi: 10.1039/D1FO00208B
26. Chen Q, Liu M, Zhang P, Fan S, Huang J, Yu S, et al. Fucoidan and galactooligosaccharides ameliorate high-fat diet-induced dyslipidemia in rats by modulating the gut microbiota and bile acid metabolism. Nutrition. (2019) 65:50–9. doi: 10.1016/j.nut.2019.03.001
27. Liu JB, Cao SC, Liu J, Xie YN, Zhang HF. Effect of probiotics and xylo-oligosaccharide supplementation on nutrient digestibility, intestinal health and noxious gas emission in weanling pigs. Asian-Australas J Anim Sci. (2018) 31:1660–9. doi: 10.5713/ajas.17.0908
28. Ma C, Gao Q, Zhang W, Zhu Q, Tang W, Blachier F, et al. Supplementing synbiotic in sows' diets modifies beneficially blood parameters and colonic microbiota composition and metabolic activity in suckling piglets. Front Vet Sci. (2020) 7:575685. doi: 10.3389/fvets.2020.575685
29. Luo D, Li J, Xing T, Zhang L, Gao F. Combined effects of xylo-oligosaccharides and coated sodium butyrate on growth performance, immune function, and intestinal physical barrier function of broilers. Anim Sci J. (2021) 92:e13545. doi: 10.1111/asj.13545
30. Ding XM Li DD, Bai SP, Wang JP, Zeng QF, Su ZW, et al. Effect of dietary xylooligosaccharides on intestinal characteristics, gut microbiota, cecal short-chain fatty acids, and plasma immune parameters of laying hens. Poult Sci. (2018) 97:874–81. doi: 10.3382/ps/pex372
31. Hansen CH, Frøkiær H, Christensen AG, Bergström A, Licht TR, Hansen AK, et al. Dietary xylooligosaccharide downregulates IFN-γ and the low-grade inflammatory cytokine IL-1β systemically in mice. J Nutr. (2013) 143:533–40. doi: 10.3945/jn.112.172361
32. Chen HH, Chen YK, Chang HC, Lin SY. Immunomodulatory effects of xylooligosaccharides. Food Sci Technol Res. (2012) 18:195–9. doi: 10.3136/fstr.18.195
33. Long J, Yang J, Henning SM, Woo SL, Hsu M, Chan B, et al. Xylooligosaccharide supplementation decreases visceral fat accumulation and modulates cecum microbiome in mice. J Funct Foods. (2019) 52:138–46. doi: 10.1016/j.jff.2018.10.035
34. Zhou J, Wu S, Qi G, Fu Y, Wang W, Zhang H, et al. Dietary supplemental xylooligosaccharide modulates nutrient digestibility, intestinal morphology, and gut microbiota in laying hens. Anim Nutr. (2021) 7:152–62. doi: 10.1016/j.aninu.2020.05.010
35. Holman DB, Brunelle BW, Trachsel J, Allen HK. Meta-analysis to define a core microbiota in the swine gut. mSystems. (2017) 2:e00004–17. doi: 10.1128./mSystems.00004-17
36. Hidalgo-Cantabrana C, Delgado S, Ruiz L, Ruas-Madiedo P, Sánchez B, Margolles A. Bifidobacteria and their health-promoting effects. Microbiol Spectr. (2017) 5:BAD-0010-2016. doi: 10.1128./microbiolspec.BAD-0010-2016
37. Zhang Z, Lv J, Pan L, Zhang Y. Roles and applications of probiotic Lactobacillus strains. Appl Microbiol Biotechnol. (2018) 102:8135–43. doi: 10.1007/s00253-018-9217-9
38. Nowak A, Paliwoda A, Błasiak J. Anti-proliferative, pro-apoptotic and anti-oxidative activity of Lactobacillus and Bifidobacterium strains: a review of mechanisms and therapeutic perspectives. Crit Rev Food Sci Nutr. (2019) 59:3456–67. doi: 10.1080/10408398.2018.1494539
39. Markowiak-Kopeć P, Slizewska K. The effect of probiotics on the production of short-chain fatty acids by human intestinal microbiome. Nutrients. (2020) 12:1107–29. doi: 10.3390./nu12041107
40. Takada T, Kurakawa T, Tsuji H, Nomoto K. Fusicatenibacter saccharivorans gen. nov, sp nov, isolated from human faeces. Int J Syst Evol Microbiol. (2013) 63:3691–6. doi: 10.1099/ijs.0.045823-0
41. Takeshita K, Mizuno S, Mikami Y, Sujino T, Saigusa K, Matsuoka K, et al. A single species of Clostridium subcluster XIVa decreased in ulcerative colitis patients. Inflamm Bowel Dis. (2016) 22:2802–10. doi: 10.1097/MIB.0000000000000972
42. Jin M, Kalainy S, Baskota N, Chiang D, Deehan EC, Mcdougall C, et al. Faecal microbiota from patients with cirrhosis has a low capacity to ferment non-digestible carbohydrates into short-chain fatty acids. Liver Int. (2019) 39:1437–47. doi: 10.1111/liv.14106
43. Yuan Y, Zhao G, Ji H, Peng B, Huang Z, Jin W, et al. Changes in the gut microbiota during and after commercial helium-oxygen saturation diving in China. Occup Environ Med. (2019) 76:801–7. doi: 10.1136/oemed-2019-106031
Keywords: Xylooligosaccharides, weaning piglets, growth performance, antioxidant, immune function, fecal microbiota, short-chain fatty acids
Citation: Pang J, Zhou X, Ye H, Wu Y, Wang Z, Lu D, Wang J and Han D (2021) The High Level of Xylooligosaccharides Improves Growth Performance in Weaned Piglets by Increasing Antioxidant Activity, Enhancing Immune Function, and Modulating Gut Microbiota. Front. Nutr. 8:764556. doi: 10.3389/fnut.2021.764556
Received: 25 August 2021; Accepted: 26 October 2021;
Published: 06 December 2021.
Edited by:
Fengjiao Xin, Institute of Food Science and Technology, Chinese Academy of Agricultural Science (CAAS), ChinaReviewed by:
Jiashun Chen, Hunan Agricultural University, ChinaFang Chen, Allegheny Veterinary Emergency Trauma & Specialty, United States
Copyright © 2021 Pang, Zhou, Ye, Wu, Wang, Lu, Wang and Han. This is an open-access article distributed under the terms of the Creative Commons Attribution License (CC BY). The use, distribution or reproduction in other forums is permitted, provided the original author(s) and the copyright owner(s) are credited and that the original publication in this journal is cited, in accordance with accepted academic practice. No use, distribution or reproduction is permitted which does not comply with these terms.
*Correspondence: Dandan Han, handandan@cau.edu.cn