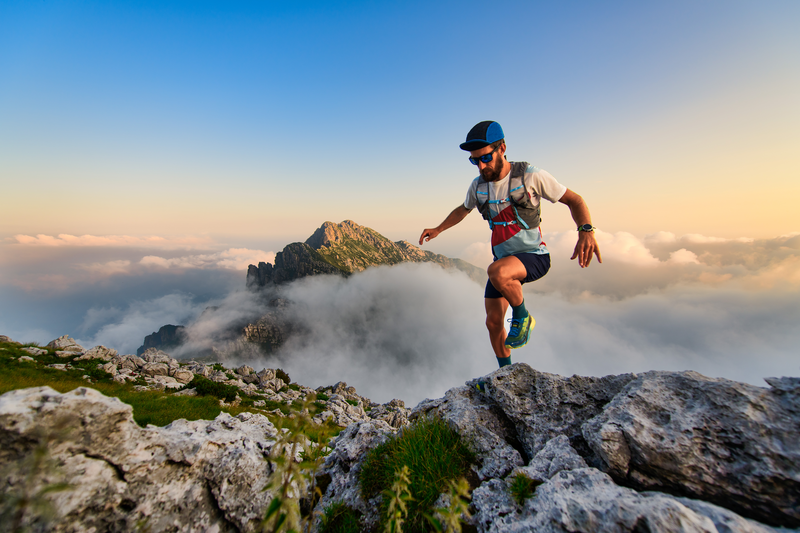
94% of researchers rate our articles as excellent or good
Learn more about the work of our research integrity team to safeguard the quality of each article we publish.
Find out more
ORIGINAL RESEARCH article
Front. Nutr. , 24 November 2021
Sec. Nutrition and Food Science Technology
Volume 8 - 2021 | https://doi.org/10.3389/fnut.2021.757728
The study evaluates the effects of genotype, maturity, and growing location on the functional and pasting properties of freshly harvested orange maize hybrids and open-pollinated varieties (OPVs). Eight fresh orange maize hybrid and eight fresh maize OPV, including the control, were harvested at three stages: 20, 27, and 34 days after planting (DAP). The freshly harvested maize samples were lyophilized and characterized for the pasting and functional properties using standard laboratory methods. The peak viscosity, final viscosity, and swelling power of the OPVs increased between 20 and 27 DAP. Additionally, the water absorption capacity increased between 20 and 27 DAP for the maize hybrids, with a decreasing trend between 27 and 34 DAP. However, genotypes 2, from the orange maize hybrid, and 5, amongst the OPV, were outstanding with the highest peak viscosities, indicating good final product quality. The combined ANOVA for the fresh orange maize hybrid and OPV showed a highly significant effect (p ≤ 0.01 and p ≤ 0.001) for the maturity and location on the pasting and functional properties except for the pasting temperature, final viscosity, and pasting time which showed no significant effect. In contrast, the location by genotypes by maturity interactions had no significant effects on the pasting and functional properties of the fresh maize hybrid and the orange OPV except only for the setback, which was highly significant at p ≤ 0.001. Nutritionists, food scientists, and maize breeders could use the information from this study to select the best maize genotypes at the appropriate harvesting period suitable for the production of the preferred maize-based products of consumers.
Maize (Zea mays L.) is a popular crop having a wide adaptability to different agroclimatic conditions. It is one of the most important annual cereal crops in the world (1). Maize is globally known as the queen of cereals because of its high yield potential amongst other cereal crops. It is cultivated on about 150,000,000 hectares in over 160 countries with a broader diversity of climate, soil, biodiversity, and management practices contributing to 36% of the global grain production. Maize is an important carbohydrate source for human diets in developing countries and animal feed in developed countries (2). The USA is the global leader in maize production, with 377,500,000 metric tons of maize (3) and 36% of the world total in 2014 (4). Maize can be successfully cultivated in a variety of soils ranging from loamy sand to clay loam. However, soils with good organic matter content and high water-holding capacity with neutral pH are considered suitable for higher productivity (5). Maize is processed and consumed in various forms, varying from region to region or from one ethnic group to another. For instance, maize grains are prepared by boiling, roasting, or as paste in Nigeria and Ghana, or as popcorn consumed all over West Africa (6). Maize provides about 1,400 Kcal/100 g of energy on a dry weight basis (7). Its common forms of consumption as an energy source for breakfast meals are cornflakes, tortillas, corn starch, tapioca, etc. In addition to being a source of carbohydrates, maize is a booster for fats, protein, and insoluble fibers, which helps in providing sufficient energy to meet the human daily dietary requirement (8).
The most cultivated maize are the white, yellow, and red maize varieties. Most people prefer the white and yellow varieties depending on the region. About 14 countries consume 85–95% of white maize as their staple food in sub-Saharan Africa. White maize also represents the leading staple food in Southern Africa, while most parts of South America and the Caribbean prefer yellow maize for animal feed (https://www.iita.org/cropsnew/maize). White maize, however, has limited micronutrients such as Vitamin A, Zinc, and Fe. The deficiencies of these essential micronutrients pose serious health concerns, especially in developing countries. Biofortification is a recent public health intervention that seeks to improve the micronutrient content of staple foods such as maize consumed by most poor people using conventional plant-breeding techniques to make a quantifiable impact on the extent of micronutrient malnutrition. Recently, plant breeders have developed biofortified varieties of maize that contain higher concentrations of beta-carotene, which are usually orange in color. Orange maize has been grown commercially in African countries like Nigeria, Ghana, and Zambia since 2013 (9).
Open-pollinated maize varieties are genetically diverse and developed by selecting open-pollinated corn ears that are desirable to the breeders or farmers. This causes a variation within plant populations that allow the varieties to adapt to local growing conditions and climates. Open-pollinated variety (OPV) seeds can be saved for future planting without losing vigor or yield (10). Hybrid varieties are the first generation of the cross that involves two or more inbred lines. They are genetically similar. Hybrid maize exhibits heterosis: the performance of the progeny of a cross between two pure-breeding lines superior to the parents. When a single-cross hybrid is allowed to open-pollinate, approximately half the hybrid vigor is lost. The crop produced from open-pollinated seeds harvested from a single-cross hybrid will not be as productive as the original single cross; therefore, seeds cannot be saved for the next planting (11).
Maize flour is frequently used in various pharmaceutical and food formulations globally. This flour is used either in its pure form or blended with other legumes such as groundnut and soybean (12). The industrial and food applications of this flour significantly depend on its functional characteristics. Functional properties such as water absorption, oil absorption, gelation, foaming, and emulsifying capacities are the inherent physicochemical properties that illustrate the structural behavior of food systems (13). The effect of harvesting time and processing method on the qualitative, physical, and chemical properties of OPV and hybrid maize has been previously reported (14–17). Mehrshad et al. (15) studied the effect of harvest time using moisture content (MC) to determine the best grain yield and reported that grains are best harvested when they have 20% MC. Maize yield increases with delayed harvesting time, but quality parameters such as crude fiber content decrease with the harvest period (17). Similar research by Panison et al. (18) on the effect of harvest time on the performance of maize hybrid reported that the delay in harvest time after physiological maturity increases the percentage of lodged and broken plants, reducing the actual grain yield. The authors also reported that the delay in harvest time does not affect grain mass in regions with high altitudes and latitudes. The most recent studies on maize are focused on the effects of harvesting time on its physicochemical properties (1, 14). However, similar studies on the effect of harvesting time on the pasting and functional properties of maize hybrid and OPV are scanty. Maize breeding efforts have improved agronomic traits, but the efforts have altered the functional and biophysical properties that determine the final processing and product quality (19). Considering the great importance of the functional properties of maize to its consumption pattern and in the food industry, it is also imperative to study the changes in the pasting properties of maize flour as affected by the period of harvesting and growing location. Thus, this study aimed to evaluate the effects of harvest time and growing location on the pasting and functional properties of OPVs and orange maize hybrids.
Freshly harvested cobs from eight orange maize hybrids and OPVs were obtained from the experimental field of the International Institute of Tropical Agriculture (IITA) for the study. The viable seeds from these cobs were planted in two separate trials at Ibadan (7°22'N, 3°58'E, altitude 150 m) and Ikenne (10°40'N, 8°77'E, altitude 730 m) with different and known meteorological information, in the early seasons of April–August 2010 and 2011. The hybrids and OPV were arranged in a randomized complete block design (RCBD) with three replications. The cobs of each hybrid were self-pollinated to minimize contamination from other pollen sources. The description of the genetic materials, including the control used for the study, is provided in Supplementary Tables 1, 2.
Plants were pre-labeled randomly on the field at the three harvest maturity stages of 20, 27, and 34 days after pollination (DAP) for each hybrid (the day after pollination started from 50% anthesis or 50% silk emergence, 57 days after planting). They were harvested at 08:00 on the relevant dates. Twenty selected cobs of each hybrid were harvested from each plot and pooled to have 60 cobs/hybrid/harvest. A total of 192 samples were packed and taken to the laboratory as soon as possible (20). The samples were shelved and loaded into a glass beaker in the LABCONCO freeze dryer (South Kansas City, Missouri, USA) and freeze-dried for 72 h. The freeze-dried maize samples were pulverized into fine flour using a laboratory mill to a <0.8 micron particle size and then transferred into a Whirl park for further analysis.
The swelling power and solubility of the maize flour were determined by heating the flour-water slurry (0.35 g flour in 12.5 ml of distilled water) in a water bath at 60°C for 30 min, with constant stirring (21). The slurries were centrifuged for 15 min using a Super-speed centrifuge (Model No. L-708-2, Phillips Drucker, Oregon, USA) at 168 × g. The supernatant was decanted into a weighed evaporating dish and dried at 100°C for 20 min. The flour solubility was calculated by the difference in the weight of the evaporating dish. The swelling power was obtained by weighing the residue after centrifugation and dividing it by the original flour weight on a dry weight basis.
The WAC was determined using the method described by Beuchat et al. (23). One gram of the samples was weighed into graduated 25 ml conical centrifuge tubes. Ten ml of distilled water was added and allowed to stand at 30 ± 2°C for 1 h. The suspension was centrifuged (Model No. L-708-2, Phillips Drucker, Oregon, USA) at 200 × g for 30 min. The supernatant was decanted, and the sample was reweighed. The weight change was expressed as the percent of the water absorption based on the original sample weight.
The pasting properties of the flour were determined in duplicate for each sample using a Rapid Visco Analyzer (RVA) (Model RVA-4; Newport Scientific Pty. Ltd., Warriewood, Australia). Three grams of the flour samples were weighed into a 25 ml canister, and 25 ml of distilled water was added. After thoroughly stirring the mixture, the canister was fitted into the RVA according to the instructions of the manufacturer. The slurry was heated from 50 to 95°C with a holding time of 2 min followed by cooling to 50°C with a 2 min holding time. The heating and cooling rate was constantly at 11.25°C/min. The pasting profile was determined with the aid of Thermocline for Windows Software (Newport Scientific Pty Ltd., Warriewood) connected to a computer.
The starch damage was determined using the standard method by the Association of Official Analytical Chemists (AOAC). The sample solution was made by dissolving 0.5 g of the flour samples with 20 mL of extractant. The flour samples were extracted for 15 min by shaking each flask for 10 s every 3 min at 30°C and filtered. Two milliliters of the filtrate were pipetted into a 25 ml volumetric flask containing 15 ml of distilled water at 21°C and 1 ml iodine solution. The mixture was left to stand for 10 min. The absorbance was 600 nm against a blank and was measured using a spectrophotometer (NOVA SPEC II, England). The starch damage was determined using the regression equation of Farrand (22). The analyses were in triplicate.
The analysis of the results of the functional and pasting properties of the maize hybrid and OPV were at different stages of maturity were subjected to statistical analyses using the XLSTAT (Addinsoft, NY, USA) tools (24). An ANOVA was used to calculate the least squares means used to estimate the differences among the means of the pasting and functional properties for each genotype at 5% of the probability level. The mean, SD, coefficient of variation (CV), and SE values were also calculated.
Table 1 shows the mean squares (MS) from the ANOVA for the pasting and functional properties of the fresh orange maize hybrid and OPV maize evaluated at two growing locations. The combined ANOVA for the fresh orange maize hybrid and OPV showed a highly significant effect (p ≤ 0.01 and p ≤ 0.001) for maturity and location on the pasting and functional properties except for pasting temperature, final viscosity, and pasting time which showed no significant effect. Still, location and genotype had no significant effect (p > 0.05) on the pasting and functional properties except for the DSD of the fresh orange maize hybrid which showed a significant effect at p < 0.05. The effect of genotype and maturity was highly significant (p ≤ 0.001) on the solubility, peak time (p ≤ 0.01), pasting time, and DSD (p ≤ 0.05) of the fresh orange hybrid maize. Peak time and DSD had significant (p ≤ 0.001) genotype and maturity effects on the orange OPV. Location by genotypes by maturity interactions had no significant effects on the pasting and functional properties of the fresh maize hybrid and the orange OPV, except for the setback, which was highly significant at p ≤ 0.001.
Table 1. Mean squares from the ANOVA for the pasting and functional properties of the fresh orange maize hybrid.
The descriptive statistics for the fresh orange maize hybrid and orange OPV maize are presented in Tables 2, 3. The mean values of the peak viscosity, trough viscosity, breakdown viscosity, final viscosity, and swelling power increased between 20 and 27 DAP for the fresh orange maize hybrid. However, they decreased between 27 and 34 DAP. The reverse of this trend was observed for the mean values of the peak time, DSD, and WAC, where the values decreased between 20 and 27 DAP but increased between 27 and 34 DAP. Additionally, the mean values for setback viscosity increased and decreased significantly for solubility (P < 0.05) across the three maturity stages, whereas the mean value of the pasting temperature remained unaffected between 20 and 27 DAP but increased slightly at the last maturity stage. However, the mean values for peak viscosity, breakdown viscosity, peak time, final viscosity, setback viscosity, DSD, solubility, and WAC were significantly (P < 0.05) different between 20 and 27 DAP.
Table 2. Descriptive statistics of the pasting and functional properties of the fresh orange maize hybrid by maturity stages (N = 192).
Table 3. Descriptive statistics of the pasting and functional properties of the fresh orange OPV maize by maturity stages (N = 192).
In contrast, the swelling power showed a mean value that is significantly different across the three maturity periods. Also, the pasting temperature shows no significant difference between 20 and 27 DAP, but there was a significant (p < 0.05) difference at 34 DAP. For the fresh orange OPV maize samples, at p > 0.05, the mean values of peak viscosity, trough viscosity, breakdown viscosity, and pasting temperature have no significant differences across the maturity periods. No significant difference exists between the mean values of the final viscosity and set back viscosity at maturity periods of 20 and 27 DAP but significantly different from 34 DAP. The swelling power and solubility have mean values significantly different from 20 to 27 to 34 DAP. In contrast, the peak time, DSD, and WAC have mean values that are not significantly different between 20 and 34 DAP.
Table 4 shows the pasting and functional properties of the fresh orange hybrid maize by genotypes and maturity stages. The results showed that the MC, trough, and pasting temperature for all the maize genotypes were not significantly different at 20, 27, and 34 DAP. In comparison, the WAC is significantly (p < 0.05) different at the different maturity periods for all the maize hybrid except for genotype 8, where the WAC at 27 and 34 DAP were not significantly different. The swelling power for genotypes 2, 3, 6, 7, and 8 are not significantly different, but genotypes 1 and 5 have significant differences (p < 0.05) in their swelling power at 20 and 27 DAP. The DSD differs significantly at 20, 27, and 34 DAP for genotypes 1, 2, 4, 6, 7, and 8, while solubility differs significantly at all the maturity stages for only genotypes 6 and 8. At all stages of maturity, the peak viscosity for genotype 4 had no significant difference, while other genotypes, including the control (genotype 8), had significant differences (p < 0.05) in their peak viscosity, mostly at 27 to 34 DAP. The final peak viscosity had no significant difference at 20, 27, and 34 DAP for all the maize hybrid except for genotypes 2 and 8. Also, genotypes 2, 5, and 6 had no significant difference in their setback viscosity.
Table 4. The pasting and functional properties of the fresh orange maize hybrid by genotypes and maturity stages (N = 192).
Table 5 shows the pasting and functional properties of the fresh orange OPV maize by genotypes and maturity stages. The pasting properties, including peak viscosity, trough, breakdown, final viscosity, peak time, and pasting temperature for genotypes 1 to 8 had no significant differences at 20, 27, and 34 DAP except for the setback viscosity, which was significantly different (p < 0.05) across the different stages of maturity. Also, for the functional properties, the swelling power and solubility for all the OPV genotypes were significantly different at 20, 27, and 34 DAP, although the solubility of genotype 2 had no significant difference between 20 and 27 DAP. In contrast, genotype 8 shows no significant difference at 20 and 34 DAP, respectively. The setback of genotypes 3, 7, and 6 shows a significant (p < 0.05) difference at all the stages of maturity. In comparison, genotypes 5 and 2 had no significant (P > 0.05) difference in setback viscosity between 20 and 27 DAP, and no significant difference in the setback viscosity for genotypes 1 and 4 between 27 and 34 DAP.
Table 5. The pasting and functional properties of the unprocessed fresh orange OPV maize by genotypes and maturity stages (N = 192).
Tables 6, 7 show the cluster analysis of the fresh maize hybrid and OPV using the pasting and functional properties. Genotypes 1 to 7, including genotype 8, which was used as control, were clustered into groups using their pasting and functional properties for the OPV and maize hybrid separately. Figure 1 shows the dendrograms for the orange OPV and maize hybrid. For the fresh orange maize hybrid, genotypes 1, 5, and 6 belong to cluster 1, while genotypes 2, 3, 4, 7, and 8 (control) were grouped into cluster 2. The pasting and functional properties in cluster 1 were slightly higher than in cluster 2, except for the DSD and solubility in cluster 2, which were slightly higher than those in cluster 1. Also, for the orange OPV genotypes, cluster 1 comprises genotypes 1 and 4, while genotypes 2, 3, 5, 6, 7, and 8 were contained in cluster 2 (Table 7). These genotypes have similarities in their functional and pasting properties with the control, making them a choice for selection over genotypes 1 and 4.
The maturity stages affected the pasting and functional properties of the fresh orange OPV and maize hybrid. Most of the functional properties of carbohydrate foods are explained based on the structure and amount of starch and protein in the different cultivars (25, 26). The peak viscosity of the fresh orange maize hybrid 3, 5, 6, and 7 at 20 DAP was higher than the grand mean value of 134 rapid Visco units (RVU), while varieties 5 and 6 had the highest value of 150 RVU for peak viscosity. However, the peak viscosity of varieties 1, 6, and 8 increased above the grand mean of 138 RVU. Also, at 34 DAP, varieties 1, 5, 6, and 7 showed a higher peak viscosity than the grand mean value of 138 RVU, but variety 6 had the highest, having 151 RVU. The peak viscosity in this study is higher than the range of 57.9–114.9 RVU reported by Abdulazeez et al. (27). The peak viscosity indicates the strength of the pastes formed from gelatinization during processing in food applications. The pasting properties of starch are affected by the amylose content, amylose/amylopectin ratio, lipids, and protein content (28). The starch concentration also influences the pasting properties in a solution, and high starch content requires a shorter time and low temperature to swell, thereby increasing the peak viscosity compared with solutions with very low starch content (29).
The study shows that variety 6 showed high peak viscosity at all stages of maturity while varieties 5 and 7 showed higher peak viscosity at 20 and 34 DAP. An increase in the peak viscosity could be attributed to an increased water absorption rate and swollen starch granules during heating (30). Additionally, for the fresh maize hybrid, it was observed that at 20 DAP, varieties 2, 3, 5, 6, and 7 had a higher peak viscosity than the grand mean of 160 RVU, while variety 5 had the highest value of 184 RVU. At 27 DAP, a higher peak viscosity than the grand mean of 160 RVU was observed for varieties 2, 5, 7, and 8. The differences in the pasting properties of the various genotypes might be related to the starch composition and structures (31). Also, high peak viscosity could be due to low protein and lipid content. During pasting, the swelling of the starch increases due to low protein and lipid content, which might increase its viscosity (32).
Meanwhile, at 34 DAP, varieties 1, 2, 3, 5, and 6 showed higher peak viscosity than the grand mean of 148 RVU, and variety 5 had the highest value of 152 RVU. These values were lower than the findings of Adedeji and Tadawus (33), where the average peak viscosity of maize hybrid was reported to be 473.83 RVU. Peak viscosity has also been reported to be associated closely with the degree of starch damage, and high starch damage results in high peak viscosity (34). Also, the mean values for setback viscosity increase across the three maturity stages, whereas the mean value of the pasting temperature remained unaffected between 20 and 27 DAP but increased slightly at the third maturity stage. The pasting temperature measures the minimum temperature required to cook foodstuff (35). The low pasting temperature of the maize flours suggests that they quickly formed pastes, hence, more suitable in most food and non-food industrial processes. A high pasting temperature indicates a higher resistance toward the swelling of the maize starch granules (36). The setback viscosity of the fresh orange hybrid maize at 20 DAP was 88.10 and at 34 DAP 109 RVU, while the OPV was at 91.80 and 135 RVU. The setback viscosity of OPV and hybrid increases as the maize matures. The setback is referred to as the ability to retrograde, which indicates the re-association of the amylose molecules released during gelatinization (37). Onabanjo et al. (38) reported a setback viscosity ranging from 60.70–74.80 RVU for the maize wheat blend. This range is lower than the values observed in this current study for OPV and maize hybrid. Variations in the paste properties of the starches of the different maize genotypes are essential in producing commercially resistant starches (39), among other uses.
The functional properties of the orange OPV maize genotypes and maize hybrid at different stages of maturity are presented in Tables 3, 4, respectively. The WAC was affected by maturity stages for the maize hybrid, including genotype 8 (control). The differences in WAC amongst the genotypes and maturity stages could result from many factors, including the protein (40) and crude fiber content (41). The degree of starch damage could also contribute to changes in the WAC. This is evident in the significant difference in the DSD for all the maize hybrids at all stages of maturity. However, the DSD for the orange OPV was not affected by the maturity stages for all the genotypes, including the control. The maize genotype 7 had the highest WAC (167.35%) at 34 DAP, higher than genotype 8 (control) that had 118.96% at 34 DAP for the maize hybrid. The WAC for the OPV ranged from 117% for genotype 7 at 27 DAP to 235% for genotype 3 at 27 DAP, which is in close agreement with the 173–235% reported by Oladapo et al. (42) for white and yellow maize flour, respectively. The swelling power for genotypes 1 and 5 is significantly different at 20 and 27 DAP, while genotypes 2, 3, 4, 6, 7, and control had no significant changes in swelling power across the maturity periods. Genotype 3 at 34 DAP had the highest swelling power of 11.89%, which is different significantly from the control (6.96%) at 34 DAP and higher than the value of 6.13% reported for yellow maize flour (42). However, a similar trend was established as swelling power mainly increases with the period of maturity. Swelling power reflects the hydration capacity of the insoluble fraction of the maize starch. The higher the swelling power, the greater the digestibility and utilization of the flour for dietary applications (33, 43, 44). Genotypes 2 and 3 had 7.44 and 7.86% solubilities, respectively, which have similarities with the control (genotype 8) with a solubility of 7.96 at 20 DAP as there were no significant differences in the solubilities. The solubility index for all maize hybrids in the present study is higher than 5.28% reported by Makanjuola and Makanjuola (45) for yellow maize starch. In this study, genotype 5 had the highest solubility of 12.13% at 27 DAP, which is consistent with the findings of Alamu et al. (46) for a solubility index of 12.69% in 100% maize flour.
The combined ANOVA for the pasting and functional properties of the fresh orange hybrid and OPV maize (Table 1) shows that location and maturity significantly impact all the pasting properties except for breakdown viscosity, final viscosity, and pasting time. There was no significant location × genotype interaction MS on the pasting and functional properties for the orange OPV. However, DSD showed significant location × genotypes interaction for the orange maize hybrid. Also, setback viscosity showed significant location × genotype × maturity interactions for both the OPV and orange maize hybrid. Alamu et al. (14) also reported significant location × maturity interactions on specific physical properties of maize hybrids.
Similarly, the peak time and DSD of the orange OPV had significant genotype and maturity interactions. The present study results revealed that location and genotype interaction did not significantly affect the pasting and functional properties of the maize hybrid and OPV. However, most of the properties were affected chiefly by the interaction of location and stages of maturity.
The present study has shown that the stages of maturity affect the pasting and functional properties of the orange OPV and maize hybrids. Higher peak viscosity was observed with maize hybrids 2, 3, 5, and 6. Variety 2 had an outstanding peak viscosity at all stages of maturity, though the highest peak viscosity for genotype 2 was at the 34 DAP maturity stage. This makes maize hybrid 2 the best choice based on its peak viscosity, indicating the quality of the final products. Cluster analysis also indicates that genotype 2 had similarities with genotype 8 (control). Also, genotypes 2, 5, and 7 are best amongst the open-pollinated genotypes with high peak viscosity. Genotype 5 was most preferred, with the highest peak viscosity at 20 DAP. Similarly, genotype 5 was grouped with the control in the cluster analysis for the orange OPV maize. The OPV varieties had higher setback viscosity than the maize hybrid; this indicates an unlikely tendency for maize hybrid to retrograde and better product stability.
The raw data supporting the conclusions of this article will be made available by the authors, without undue reservation.
EA and BM-D designed the study. EA and AM conducted the experiments. EA and MA analyzed and interpreted the datasets and prepared the manuscript. OO, BM-D, and AM supervised the study and edited the manuscript. All authors contributed to the article and approved the submitted version.
This research was supported by the International Institute of Tropical Agriculture (IITA) Ibadan, Nigeria, and the Bill & Melinda Gates Foundation (BMGF) through a grant OPP1178942. This work was also supported by Crop (CRP) Maize under Consultative Group on International Agricultural Research (CGIAR), a global partnership for a food-secure future.
The authors declare that the research was conducted in the absence of any commercial or financial relationships that could be construed as a potential conflict of interest.
All claims expressed in this article are solely those of the authors and do not necessarily represent those of their affiliated organizations, or those of the publisher, the editors and the reviewers. Any product that may be evaluated in this article, or claim that may be made by its manufacturer, is not guaranteed or endorsed by the publisher.
The Supplementary Material for this article can be found online at: https://www.frontiersin.org/articles/10.3389/fnut.2021.757728/full#supplementary-material
1. Tandzi LN, Mutengwa CS. Estimation of maize (Zea mays L.) yield per harvest area: appropriate methods. Agronomy. (2019) 10:1–18. doi: 10.3390/agronomy10010029
2. Undie UL, Uwah DF, Attoe EE. Effect of intercropping and crop arrangement on yield and productivity of late season maize/soybean mixtures in the humid environment of South Southern Nigeria. J Agri Sci. (2012) 4:37–50. doi: 10.5539/jas.v4n4p37
3. World Atlas. World Leaders in Corn (Maize) Production, By Country. (2016). Available online at: https://www.worldatlas.com/articles/world-leaders-in-corn-maize-production-by-country.html (accessed July 24, 2021).
4. Statista. Distribution of Global Corn Production in 2015 by Country. (2016). Available online at: www.statista.com/market/ (accessed July 20, 2021).
5. Annual Progress Report Kharif Maize. All India Coordinated Research Project on Maize. Directorate of Maize Research, Pusa Campus. New Delhi (2012).
6. Abdulrahman AA, Kolawole OM. Traditional preparations and uses of maize in Nigeria. Ethnobot Leaflets. (2006) 10:219–27.
7. Shikha B, Manpreet J, Ramanjit K. Nutritive Value, Maize-Production and Use, Akbar Hossain. IntechOpen. (2019). doi: 10.5772/intechopen.88963
8. Nuss ET, Tanumihardjo SA. Maize: A paramount staple crop in the context of global nutrition. Comp Rev Food Sci Food Saf. (2010) 9:417–36. doi: 10.1111/j.1541-4337.2010.00117.x
9. Smith GN, Sarah C, Alinafe M, Peter M. Biofortification of maize and sweetpotatoes with provitamin A carotenoids and implication on eradicating vitamin A deficiency in developing countries. J Agric Food Res. (2020) 2:100068. doi: 10.1016/j.jafr.2020.100068
11. van Heerwaarden J, Hellin J, Visser R, Van Eeuwijk F. Estimating maize genetic erosion in modernized smallholder agriculture. Theor Appl Genet. (2009) 119:875–88. doi: 10.1007/s00122-009-1096-0
12. Akubor PI, Onimawo IA. Functional properties and performance of soybean and maize flour blends in cookies. Plant Foods Human Nutr. (2003) 58:1–12. doi: 10.1023/B:QUAL.0000040342.75650.5c
13. Awuchi CG, Igwe VS, Echeta CK. The functional properties of foods and flours. Int J Adv Acad Res. (2019) 5:139–60.
14. Alamu OE, Menkir A, Maziya-Dixon B, Olorunfemi OO. Effects of husk and harvest time on carotenoid content and acceptability of roasted fresh cobs of orange maize hybrids. Food Sci Nutr. (2014) 2014:811–20. doi: 10.1002/fsn3.179
15. Mehrshad B, Sajad K, Masoud R, Ali AM. Effect of harvesting time on grain yield, yield components, and some qualitative properties of four maize hybrids. Int J Agri Food Res. (2014) 3:1–7. doi: 10.24102/ijafr.v3i4.354
16. Akinjide AO, Idowu MA, Karim O, Segilola VO, Obisesan DO. Effect of processing method II: anti-nutritional, microbial, and sensory quality of maize-millet-soybean complementary food. Annals Food Sci Technol. (2017) 18:686–95.
17. Shehzad MA, Maqsood M, Bhatti MA, Ahmad W, Shahid MR. Effects of nitrogen fertilization rate and harvest time on maize (Zea mays L.) fodder yield and its quality attributes. AJPBR. (2012) 2–19. doi: 10.2298/BAH1803345M
18. Panison F, Sangoi L, Casa RT, Durli MM. Harvest time, stem and grain sensibility of maize hybrids with contrasting growth cycles. Afr J Agric Res. (2016) 11:2403–11. doi: 10.5897/AJAR2016.11232
19. Goredema-Matongera N, Ndhlela T, Magorokosho C, Kamutando CN, van Biljon A, Labuschagne M. Multinutrient biofortification of maize (Zea mays L.) in Africa: current status, opportunities, and limitations. Nutrients. (2021) 13:1039. doi: 10.3390/nu13031039
20. Osanyintola OJ, Marek JH, Akingbala JO. Effect of time of harvest and variety on the quality of boiled green field maize [Zea mays Linn]. Trop Sci. (1992) 32:369–76.
21. Crosbie GB. The relationship between starch swelling properties, paste viscosity and boiled noodle quality in wheat flours. J Cereal Sci. (1991) 13:145–50. doi: 10.1016/S0733-5210(09)80031-3
22. Farrand EA. Flour properties in relation to the modern bread processes in the United Kingdom, with special reference to alpha-amylase and starch damage. Cereal Chem. (1964) 41:98–110.
23. Beuchat LR, Cherry JP, Quinn MR. Physicochemical properties of peanut flour as affected by proteolysis. J Agric Food Chem. (1975) 23:616–20. doi: 10.1021/jf60200a045
24. Addinsoft. XLSTAT Statistical and Data Analysis Solution. New York, NY (2021). Available online at: https://www.xlstat.com/en
25. Bean SR, Zhu L, Smith BM, Wilson JD, Ioerger BP, Tilley M. (2019). Starch and Protein Chemistry and Functional Properties. Sorghum Millets 2019:131–70. doi: 10.1016/B978-0-12-811527-5.00006-X
26. Pomeranz Y, Hall GE, Czuchajowska Z, Lai FS. Test weight, hardness and breakage susceptibility of yellow dent corn hybrids. Cereal Chem. (1986) 63:349–51.
27. Abdulazeez EO, Irondi EA, Alamu EO, Ajani EO, Maziya-Dixon B, Menkir A. Characterization of striga-resistant yellow-orange maize hybrids for bioactive, carbohydrate, pasting properties. Front Sustain Food Syst. (2020) 4:585865. doi: 10.3389/fsufs.2020.585865
28. Shabir AM, Sowriappan JD, Mudasir B, Manzoor AS, Mohammad M. Physicochemical and structural properties of starches isolated from corn cultivars grown in Indian temperate climate. Int J Food Propert. (2017) 4:821–32. doi: 10.1080/10942912.2016.1184274
29. Acosta-Osorio AA, Herrera-Ruiz G, Pineda-Gómez P, Cornejo-Villegas M, de los A, Martínez-Bustos F, et al. Analysis of the apparent viscosity of starch in aqueous suspension within agitation and temperature by using rapid visco analyzer system. Mech Eng Res. (2011) 1:1. doi: 10.5539/mer.v1n1p110
30. Ragaee S, Abdel-Aal ESM. Pasting properties of starch and protein in selected cereals and quality of their food products. Food Chem. (2006) 95:9–18. doi: 10.1016/j.foodchem.2004.12.012
31. Yaeel IC, Oliviert M, Carmen LD, Francisco JW, Jesús B, Francisco JC. The structural characteristics of starches and their functional properties. J Food. (2018) 16:1003–17. doi: 10.1080/19476337.2018.1518343
32. Uarrota VG, Amante ER, Demiate IM, Vieira ID, Maraschin M. Physicochemical, thermal, and pasting properties of flours and starches of eight brazilian maize landraces (Zea Mays L.). Food Hydrocoll. (2013) 30:614–24. doi: 10.1016/j.foodhyd.2012.08.005
33. Adedeji OE, Tadawus N. Functional properties of maize flour (Zea mays) and stability of its paste (tuwo) as influenced by processing methods and baobab (Adansonia digitata) pulp inclusion. Ukrainian J Food Sci. (2019) 7:49–60. doi: 10.24263/2310-1008-2019-7-1-7
34. Sanni LO, Ikuomola DP, Sanni SA. Effect of Length of Fermentation and Varieties on the Qualities of Sweet Potato Gari. In: Akoroda MO, editor. Proceeding 8th triennial Symposium of the International Society for Tropical Root Crops. Ibadan: IITA (2001). p. 208–211.
35. Newport Scientific. Applications Manual for the Rapid Visco Analyzer Using Thermocline for Windows. Warriewood: Newport Scientific Pty Ltd.
36. Sandhu KS, Singh N. Some properties of corn starches II: Physicochemical, gelatinization, retrogradation, pasting and gel textural properties. Food Chem. (2007) 101:1499–507. doi: 10.1016/j.foodchem.2006.01.060
37. Lawal O. Composition, physicochemical properties and retrogradation characteristics of native, oxidised, acetylated and acid-thinned new cocoyam (Xanthosomas agittifolium) starch. Food Chem. (2004) 87:205–18. doi: 10.1016/j.foodchem.2003.11.013
38. Onabanjo OO, Olayiwola IO, Adegunwa MO, Ighere DA, Dave-Omoregie AO, Abaku NS. Functional and pasting characteristic of wheat, yellow maize and beniseed composite flour. Trends Appl Sci Res. (2020) 15:187–92. doi: 10.3923/tasr.2020.187.192
39. Shinde SV, Nelson JE, Huber KC. Soft wheat starch pasting behavior in relation to a- and b-type granule content and composition. Cereal Chem. (2003) 80:91–8. doi: 10.1094/CCHEM.2003.80.1.91
40. Celik I, Isik F, Gursoy O. Couscous, a traditional Turkish food product: production method and some applications for enrichment of nutritional value. Int J Food Sci Technol. (2004) 39:263–9. doi: 10.1111/j.1365-2621.2004.00780.x
41. Keihireddipalli P, Hung YC, McWatters KH, Phillips RD. Effect of milling method (wet and dry) on the functional properties of cowpea (Vigna unguiculata) pastes and end-product (akara) quality. J Food Sci. (2002) 67:48–52. doi: 10.1111/j.1365-2621.2002.tb11357.x
42. Oladapo AS, Adepeju AB, Akinyele AA, Adepeju DM. The proximate, functional and anti-nutritional properties of three selected varieties of maize (Yellow, White and Pop Corn) flour. Int J Sci Eng Sci. (2017) 1:23–6.
43. Suresh C, Samsher S. Assessment of functional properties of different flours. Afr J Agric Res. (2013) 8:4849–52.
44. Craig SAS, Stark JR. The effect of physical damage on the molecular structure of wheat starch. Carbohydr Res. (1984) 125:117–25. doi: 10.1016/0008-6215(84)85147-2
45. Makanjuola OM, Makanjuola JO. Evaluation of functional and pasting properties of different corn starch flours. Int J Food Sci Nutr. (2018) 3:95–9.
Keywords: maize hybrid, open-pollinated varieties, maturity, genotypes, pasting properties, functional properties
Citation: Alamu EO, Maziya-Dixon B, Menkir A, Adesokan M and Olaofe O (2021) Assessment of Functional and Pasting Properties of Fresh Orange Maize Hybrids and Open-Pollinated Varieties as Influenced by Genotype, Harvesting Time, and Growing Location. Front. Nutr. 8:757728. doi: 10.3389/fnut.2021.757728
Received: 12 August 2021; Accepted: 08 October 2021;
Published: 24 November 2021.
Edited by:
Akinbode A. Adedeji, University of Kentucky, United StatesReviewed by:
Olufunke Ezekiel, University of Ibadan, NigeriaCopyright © 2021 Alamu, Maziya-Dixon, Menkir, Adesokan and Olaofe. This is an open-access article distributed under the terms of the Creative Commons Attribution License (CC BY). The use, distribution or reproduction in other forums is permitted, provided the original author(s) and the copyright owner(s) are credited and that the original publication in this journal is cited, in accordance with accepted academic practice. No use, distribution or reproduction is permitted which does not comply with these terms.
*Correspondence: Emmanuel Oladeji Alamu, by5hbGFtdUBjZ2lhci5vcmc=
Disclaimer: All claims expressed in this article are solely those of the authors and do not necessarily represent those of their affiliated organizations, or those of the publisher, the editors and the reviewers. Any product that may be evaluated in this article or claim that may be made by its manufacturer is not guaranteed or endorsed by the publisher.
Research integrity at Frontiers
Learn more about the work of our research integrity team to safeguard the quality of each article we publish.