- 1State Key Laboratory of Grassland Agro-Ecosystem, School of Life Sciences, Lanzhou University, Lanzhou, China
- 2Qinghai Provincial Key Laboratory of Adaptive Management on Alpine Grassland, Qinghai University, Xining, China
- 3Gansu Devotion Biotechnology Co., Ltd., Zhangye, China
- 4Qinghai Qilian Yida Meat Co., Ltd., Qinghai, China
- 5Desert Animal Adaptations and Husbandry, Wyler Department of Dryland Agriculture, Blaustein Institutes for Desert Research, Ben-Gurion University of the Negev, Beer Sheva, Israel
Traditionally, yaks graze only natural grassland, even in harsh winters. Meat from grazing yaks is considered very healthy; however, feedlot fattening, which includes concentrate, has been introduced. We questioned whether this change in management and diet would have an impact on the rumen and meat quality of yaks. This study examined the morphology, fermentation, and microbiota of the rumen and the quality of meat of three groups of bovines: (1) grazing yaks (GYs, 4-year olds), without dietary supplements; (2) yaks (FYs, 2.5-year olds) feedlot-fattened for 5 months after grazing natural pasture; and (3) feedlot-fattened cattle (FC, Simmental, 2-year olds). This design allowed us to determine the role of diet (with and without concentrate) and genotype (yaks vs. cattle) on variables measured. Ruminal papillae surface area was greater in the FYs than in the GYs (P = 0.02), and ruminal microbial diversity was greater but richness was lesser in the GYs than in the FC and FYs. Concentrations of ruminal volatile fatty acids were greater in the yaks than in the cattle. In addition, both yak groups had higher protein and lower fat contents in meat than the FC. Meat of GY had a lower n6:n3 ratio than FY and FC, and was the only group with a ratio below r, which is recommended for healthy food. Essential amino acids (EAA), as a proportion of total AA and of non-essential AA of yak meat, met WHO criteria for healthy food; whereas FC did not.
Introduction
The yak (Poephagus grunniens) is the sole bovine species that has adapted well to the Qinghai-Tibetan plateau. This ruminant is crucial for the livelihood of residents, providing meat, milk, dung, and fiber. Traditionally, yaks grazed natural pasture all year, without supplementary feed, even in long, harsh winters. Because of the clean environment and year-round grazing of natural pasture, yak meat has been regarded as healthy and organic, and is in high demand (1). However, with increased number of yaks in recent years and the widespread degradation of grasslands, the natural pasture cannot sustain the stocking rate of yaks. Different options are being examined to raise yaks, and feedlots are being established. Weaned calves are being bought from herders and fattened on concentrate feed.
Dietary intake affects rumen fermentation and development (2), and meat properties. For example, supplementary concentrate increased rumen microbial abundance and enhanced epithelium development in Tibetan sheep (3), while a high forage diet increased intramuscular fat in steers (4). We questioned how feedlot fattening with concentrate feed would affect the rumen and meat quality of yaks. To answer this query, we examined rumen morphology, fermentation, and microbiota, and meat quality in yaks grazing only natural pasture, without supplements, yaks fattened with concentrate in a feedlot for 5 months after only grazing natural pasture for 2 years, and Simmental cattle fattened in a feedlot. We also questioned whether dietary intake (concentrate vs. no concentrate) or genotype (yaks vs. cattle) would have greater influence on the measurements.
Materials and Methods
Animals and Sample Collection
Three groups of bovines were included in the study: (1) four, 4-year-old male yaks that only grazed natural pasture with no supplements (GYs); (2) three 2.5-year-old male yaks that grazed natural pasture for 2 years but were fattened in a feedlot for the last 5 months (FYs); and (3) three 2-year-old male Simmental cattle that were fattened in a feedlot (FC). Simmental is the popular beef breed in the area and is raised only in feedlots. Attempts to graze this breed have been unsuccessful, because they are not adapted to harsh pasture conditions. The GYs and FYs were of the same genetic background and grazed in Qilian County (3,200 m. a. s. l.), Qinghai province; The FYs and FC were fattened in a feedlot in Zhangye city (2,000 m. a. s. l.), Gansu province, adjacent to Qilian County, on a total mixed ration (Table 1). Because the growing rate of GYs was slower than that of FYs and FC, they were slaughtered at an older age than the other two groups. The aim of the study was to follow the natural management system for yaks and Simmental cattle in the area, so that the results would be relevant for the meat sold to consumers. The grazing yaks are slaughtered at 4 years of age and older; whereas the feedlot yaks are fattened between 2 and 2.5 years and slaughtered at 2.5 years of age. We wanted the GYs and FYs to be from the same genetic background to eliminate genetic differences from the comparison between the yak groups. This was accomplished but also limited the number of yaks available for the study.
The FYs and FC were slaughtered in an abattoir on November 21 and 22, 2019, and the GYs on December 12, 2019, at 05:00 after fasting 18 h, but they had free access to water for up to 2 h before slaughter. Total rumen contents were collected immediately after slaughter, mixed thoroughly, which also dislodged much of the microbiota adhering to the solid particles, and strained through four layers of cheesecloth, as described by Hu et al. (5). Fifty ml rumen fluid was transferred immediately to the laboratory and stored at −80°C until analysis. Tissues (~1 cm2) were collected from the ventral region of the rumen. The left muscularis longissimus (12–13th rib levels) was collected from each yak, cooled for 24 h at 4°C, and then stored at −20°C until analysis.
Ruminal Histology
The ruminal tissues were rinsed with physiological saline, fixed in 10% buffered formalin solution for 48 h, dehydrated in a graded series of absolute ethanol (30, 50, 70, and 90%), cleared with benzene, and then saturated with and embedded in paraffin wax. Blocks were cut into 5-μm sections using a rotary microtome (RM2235; Leica, Wetzlar, Germany), and sections were stained by hematoxylin-eosin (6). The morphology of the tissues was examined by microscopy and photographed (Smartzoom 5; Zeiss, Jena, Germany) and images were analyzed for length and width of papillae with the Motic imaging software (Motic, Kowloon, Hong Kong). The number of papillae was estimated following the method described by Shen (7). In brief, the number of papillae per cm2 of mucosa was counted by video camera imaging using the Motic imaging software (Motic, Kowloon, Hong Kong). The total surface area of papillae per cm2 was calculated as length × width × 2, times the number of papillae per cm2.
Rumen Microbial DNA Extraction and Sequencing
The frozen rumen fluid was thawed on ice for DNA extraction. Microbial DNA was extracted with TIANamp Stool DNA Kit (Tiangen Biotech Co., Ltd, Beijing, China), following the protocol of the manufacturer. After checking the quality and quantity of DNA with a DNA spectrophotometer (ND-1000; Nano Drop, Wilmington, DE, United States), the DNA pellet was freeze-dried and dissolved in Tris-EDTA (TE) buffer (pH 8) containing DNase-free RNase (100 μg/ml), and stored at −20°C. The primers used were designed according to the conserved region, and the end of the primers was added with a sequencing connector, which was amplified by PCR. The products were purified, quantified, and homogenized to form a sequencing library. The targets in the V3-V4 region of the bacterial 16S rRNA gene were amplified using 338F (5′-ACTCCTACGGGAGGCAGCA-3′) and 806R (5′-GGACTACHVGGGTWTCTAAT-3′), as reported by Wei et al. (8). Phusion High-Fidelity PCR Master Mix and GC Buffer were used for PCR reaction. The PCR temperature regime was as follows: denaturing at 94°C for 1 min, annealing at 51°C for 1 min, and elongating at 72°C for 1.5 min. At the end of 30 cycles, a final extension was dwelled at 72°C for 8 min. The PCR products were purified by 2% agarose gel electrophoresis. Finally, the obtained amplicons were sequenced using an Illumina HiSeq platform to generate paired 250-bp reads.
Microbial diversity analysis was assessed with an Illumina HiSeq sequencing platform, using the paired-end (PE) sequencing method to construct a small fragment library for sequencing. The FLASH v1.2.7 software was used to splice the PE reads of each sample through overlap, and the resulting spliced sequence was the original Tags data (Raw Tags). The Trimmomatic v0.33 software filtered the Raw Tags obtained by splicing for Clean Tags, and the UCHIME v4.2 software identified and removed chimera sequences to obtain the final Effective Tags. UCLUST in the QIIME 1.9.1 software was used to cluster Tags at 97% similarity level, obtain operational taxonomic units (OTUs), and annotate the OTUs based on the Silva and UNITE taxonomy databases.
Determination of Ruminal Volatile Fatty Acids
Ruminal volatile fatty acids (VFAs) were determined as described by Stewart and Duncan (9). In brief, after thawing at 4°C for 2 h, 2 ml of the rumen fluid was centrifuged at 4,830 × g at 4°C for 10 min, and then 0.15 ml metaphosphoric acid was added to 1.5 ml of supernatant and homogenized. The mixed solution was centrifuged at 5,670 × g at 4°C for 15 min, and the supernatant was used to determine the VFAs (acetate, propionate, and butyrate) using a gas chromatograph (GC, SP-3420A; Beijing Beifen-Ruili Analytical Instrument Co., Ltd., Beijing, China) equipped with an AT-FFAP type capillary column (30 m × 0.32 mm × 0.5 μm) and a flame ionization detector, with the temperature regime as: the column temperature was maintained at 90°C for 1 min, increased to 120°C at 10°C /min for 1 min, then increased from 120 to 150°C at 10°C/min, and maintained at 150°C for 3 min.
Meat Composition
Meat samples were dried and milled (Wiley Mill No 1; Arthur Thomas Co, Philadelphia, PA, United States) through a 2-mm screen. Protein content was determined by the Dumas method (combusting samples, AACC method 46-30.01) using an elemental analyzer (Elementar Vario MACRO Cube; Elementar, Hanau, Germany), and water and intramuscular fat (IMF) contents were determined using a SMART Trac rapid fat analyzer (CEM Corporation, Matthews, NC, United States) (Methods 985.14 and 985.26, AOAC, 1990). Ash was determined by burning the samples at 550°C for 4 h in a furnace (TM-0912p; ICHCM, Beijing, China). Amino acids were determined by an amino acid analyzer (Biochrom 30+; Biochrom, Cambridge, United Kingdom). Meat fatty acid (FA) concentrations were determined as described by Sukhija et al. (10). Briefly, the total fat in a 0.2-g sample was extracted with 2 ml benzene containing an internal standard (0.5 mg/ml C19) and 3 ml of 5% methanolic HC1 (10 ml of acetyl chloride to 100 ml of anhydrous methanol) in culture tubes. The tube was vortexed for 1 min and heated for 2 h in a water bath at 70°C. After cooling to room temperature, 5 ml of 6% K2CO3 was added, and the tube was vortexed again for 0.5 min and centrifuged for 5 min at 75 × g at 4°C. The clear upper benzene layer was transferred to another tube for determination of fatty acids using a gas chromatograph mass spectrometer (GC-MS, QP2020, Shimadzu, Kyoto, Japan) fitted with a fused-silica capillary column (100 m × 0.25 mm × 0.2 μm, sp-2560; Supelco, Belfonte, PA, USA), following Ding et al. (11).
The atherogenic index (AI) and thrombogenicity index (TI) of meat were calculated based on the FA concentrations (12):
AI = (C12:0+4×C14:0+C16:0)/(MUFA + PUFA);
TI = (C14:0+C16:0+C18:0)/[(0.5 × MUFA) + (0.5 × n6 PUFA) + (3 × n3 PUFA) + *n3/n6 PUFA]
where C12:0, C14:0, C16:0, and C18:0 are lauric, myritoleic, palmitoleic, and stearic acids; MUFA is monounsaturated fatty acids, and PUFA is polyunsaturated fatty acids (g/100 g total fatty acids).
Statistical Analysis
Ruminal VFAs and meat chemical, fatty acid, and amino acid compositions were analyzed by one-way ANOVA (SPSS v26, Chicago, IL, United States). The microbial alpha diversity index, which included Shannon and Chao1 indices, was evaluated using the Mothur (version v.1.30) software. The relative abundance of rumen bacteria (phyla and genera), operational taxonomic units (OTUs), Kyoto Encyclopedia of Genes and Genomes (KEGGs), and principal coordinates analysis (PCoA) were determined using the vegan package in R 3.4.1.
Following log transformations, microbial data deviated from normality (Shapiro–Wilk's tests, P < 0.05). Non-parametric factorial Kruskal–Wallis sum-rank tests were performed to test for differences among groups at the bacterial phylum and genus levels, and Dunn's test (13) was performed to separate means where significance was found. Measurements are reported as their non-transformed values. Correlations among the top 10 most abundant bacterial genera with bovine genotype (FY vs. FC) and feed (GY vs. FY), were tested using Spearman rank correlation analysis of R software. Statistical significance was accepted at P < 0.05 and as a tendency at 0.05 ≤ P < 0.1. When significant differences were found, means were separated by Tukey's test.
Results
Rumen Histology
Diets affected the morphology of ruminal papillae of the three bovine groups. The papillae surface area in FY was larger than that in GY, but did not differ from that in FC. GY had a thicker stratum corneum and wider connective tissue of ruminal papilla (Figure 1, Table 2), and wider but shorter ruminal papillae than FY and FC (P < 0.05) (Table 2). Of the three groups, GY had the highest papillae density, while FC had the lowest.
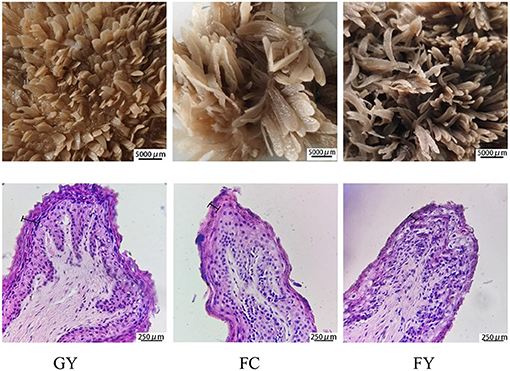
Figure 1. Morphology structure of rumen papillae of grazing yaks (GYs), feedlot-fattened cattle (FC), and feedlot-fattened yaks (FYs) (The 250-μm scale denotes stratum corneum thickness).

Table 2. Rumen morphology of grazing yaks (GYs), feedlot-fattened cattle (FC), and feedlot-fattened yaks (FYs).
Rumen Fermentation
Ruminal acetate and total volatile fatty acid concentrations were greater (P < 0.05) in GY and FY than in FC, but concentrations of ruminal propionate, butyrate, and valerate did not differ among the three bovine groups (Table 3).
Ruminal Microbiota
A total of 160,598 raw bacterial 16S rRNA gene sequences were obtained after quality control and filtering, and 73,332 valid sequences were analyzed. The ruminal bacteria contained 1,134 OTUs by 16S rRNA gene sequencing. The three groups of bovines shared 675 OTUs of bacteria (Figure 2), while there were 123 non-shared OTUs in GY, 11 OTUs in FY, and 3 OTUs in FC. The ruminal microbial diversity (Shannon index) of GY was greater, but species richness (Chao 1 index) was lesser (P < 0.05) than in FY and FC (Figure 3). The rumen microbial community was separated clearly among GY, FY, and FC (Figure 4).
There were 10 bacterial phyla identified in the rumen in the three groups, with Bacteroidetes and Firmicutes being dominant (Table 4). The relative abundance of Bacteroidetes in GY was greater (P = 0.03) than in FC, but did not differ from that in FY; whereas, that of Firmicutes did not differ among groups. The relative abundance of Proteobacteria in FC tended to be greater (P = 0.09) than in GY or FY, while there was no difference in Kiritimatiellaeota among the three groups. The relative abundances of Fusobacteria and Actinobacteria were lesser (P < 0.01) in GY than in FC and FY; uncultured_bacterium_k_Bacteria were greater (P = 0.02) in FC than in GY and FC; and Synergistetes and Patescibacteria were greater (P < 0.05) in GY than in FY and FC. The relative abundance of Chloroflexi was greater (P = 0.04) in FY than FC, but not different from that in GY.
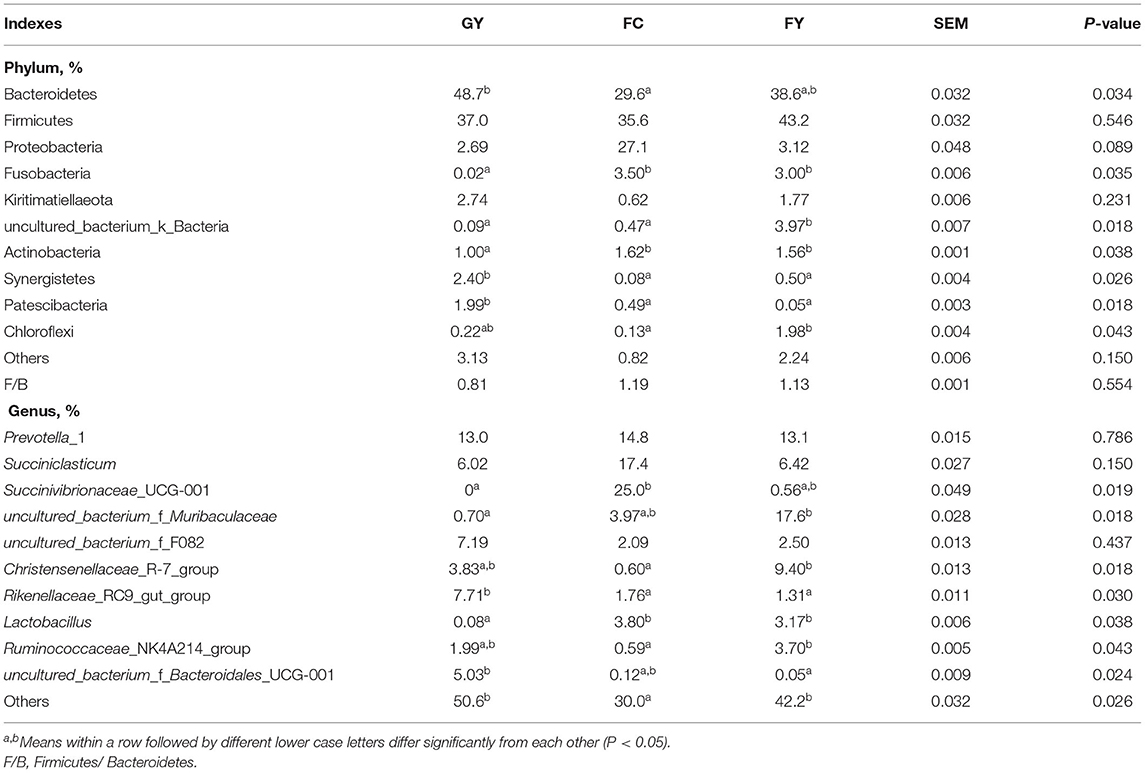
Table 4. Relative abundance of ruminal bacterial communities at phylum and genus levels of GYs, FC, and FYs.
There was no difference in the relative abundances of Prevotella_1, Succiniclasticum, and uncultured_bacterium_f_F082 among the three bovine groups; Succinivibrionaceae_UCG-001 was greater (P = 0.02) in FC than in GY (not detected), but not different than in FY; uncultured_bacterium_f_Muribaculaceae was greater (P = 0.02) in FY than in GY, but not different from that in FC; the Christensenellaceae_R-7_ and Ruminococcaceae_NK4A214_groups were greater (P < 0.01) in FY than in FC, but not different from those in GY. In addition, the Rikenellaceae_RC9_gut_group was greater (P = 0.03) in GY than in FC and FY; uncultured_bacterium_f_Bacteroidales_UCG-001 was greater (P = 0.02) in GY than in in FY, but not different from that in FC; Lactobacillus was lesser (P < 0.05) in GY than in FC and FY. More than 50% in GY and 40% in FY of the ruminal bacteria genera were not identified, which was higher (P < 0.05) than the 30% in FC (Table 4).
Spearman correlation analysis showed that the relative abundance of uncultured_bacterium_f_Muribaculaceae and Rikenellaceae_RC9_gut_group was correlated significantly (P < 0.01) with bovine genotype (FY vs. FC); the relative abundance of Prevotella_1, uncultured_bacterium_f_Muribaculaceae, and unculcured_bacterium_f_F082 was correlated significantly (P < 0.01) with feed (GY vs. FY) (Figure 5A). There were significant (P < 0.05) positive correlations between Lactobacillus and Prevotella_1, Lactobacillus and Succinivibrionaceae_UCG-001, the Ruminococcaceae_NK4A214_ and Christensenellaceae_R-7_groups, and uncultured_bacterium_f_Bacteroidales_UCG-001 and the Rikenellaceae_RC9_gut_group; and negative correlations (P < 0.05) between the Rikenellaceae_RC9_gut_group and uncultured_bacterium_f_Muribaculaceae, the Ruminococcaceae_NK4A214_group and Succiniclasticum, and uncultured_bacterium_f_Bacteroidales_UCG-001 and uncultured_bacterium_f_Muribaculaceae (Figure 5B).
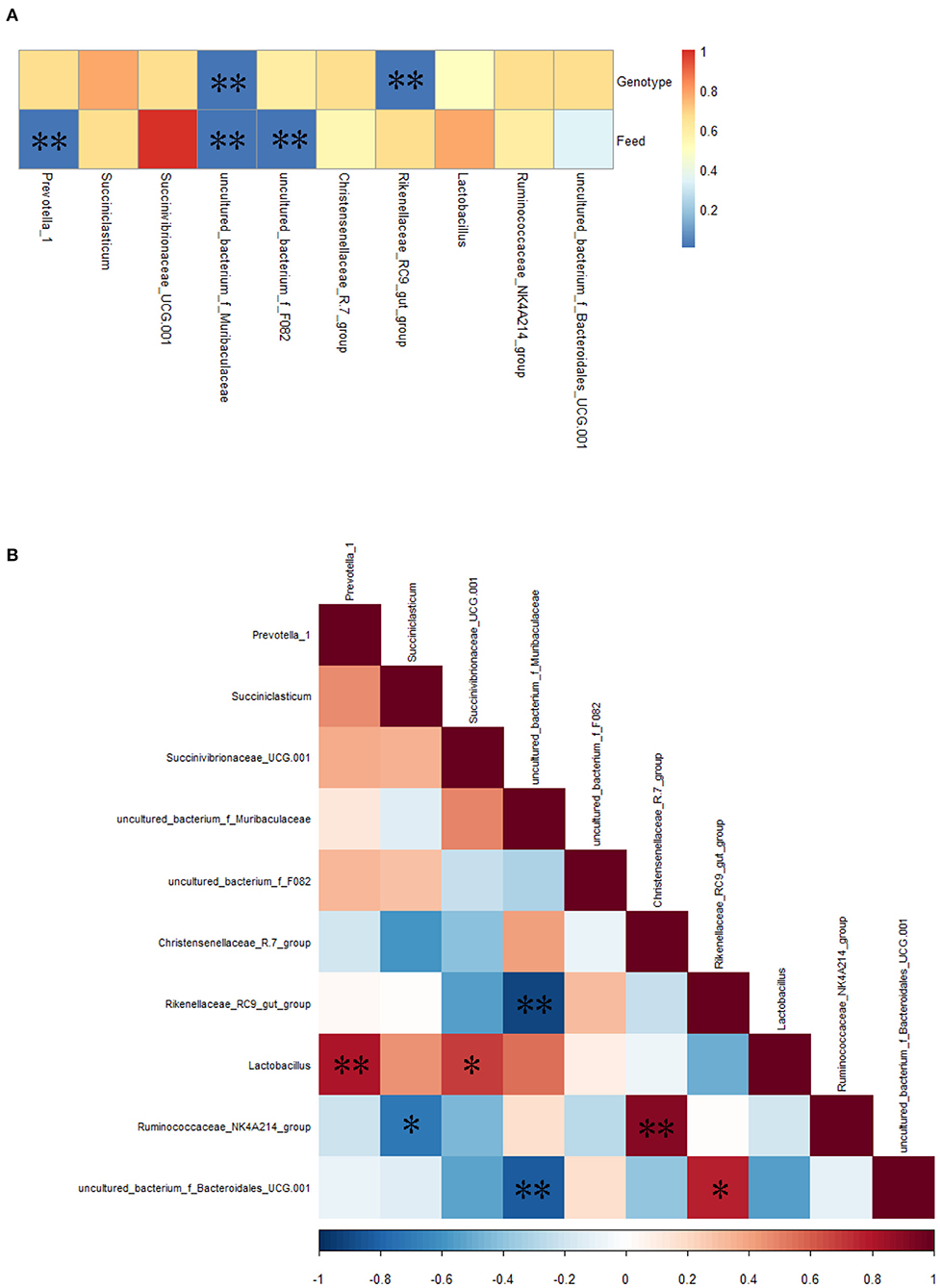
Figure 5. (A) Spearman correlations between the top 10 most abundant genera of rumen bacteria and bovine genotype and feed, (B) mutual-correlation among the top 10 genera. Color indicates (A) P-values and (B) correlation index (r); (B) Red represents a positive correlation, and blue represents a negative correlation. *P < 0.05, **P < 0.01.
Eleven significant rumen microbial metabolic pathways were identified between GY and FC by the Kyoto Encyclopedia of Genes and Genomes (KEGG) analysis (Figure 6). The rumen microbial functions of metabolism of co-factors and vitamins, nucleotide metabolism, replication and repair, transcription, and membrane transport were greater in FC than in GY. However, the metabolism of terpenoids and polyketides, biosynthesis of other secondary metabolites, lipid metabolism, energy metabolism, signal transduction, and carbohydrate metabolism were greater in GY than in FC.
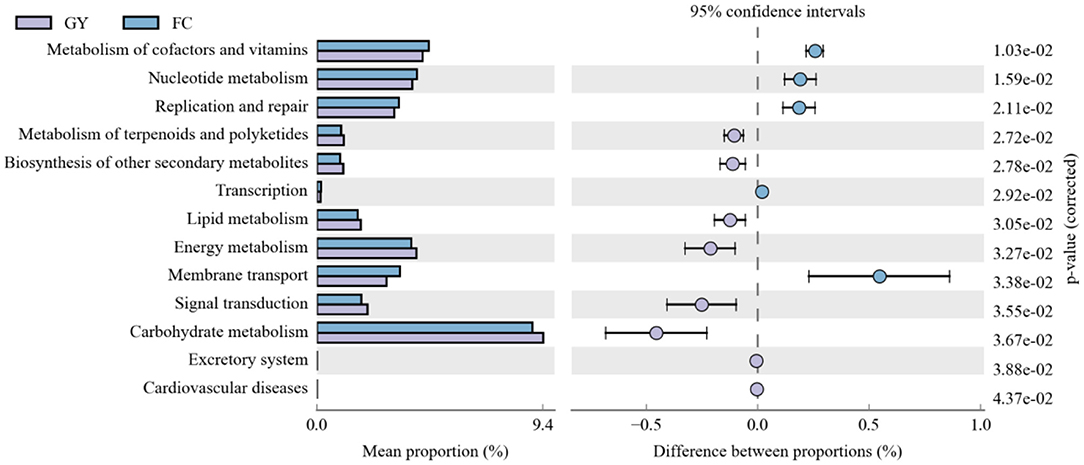
Figure 6. Kyoto Encyclopedia of Genes and Genomes (KEGG) analysis of rumen bacteria between GYs and FC.
Meat Quality
The moisture content of meat did not differ among the three bovine groups. The crude protein content was higher (P < 0.01), but the fat content was lower (P < 0.05), in meat of the two yak groups (GY and FY) than in FC (Table 5).
Myristic acid (C14:0) and myristoleic acid (C14:1) concentrations were higher (P < 0.05) in FC than in GY and FY (Table 6). Concentrations of palmitoleic (C16:1) and heptadecenoic acids (C17:1) tended to be lower (P = 0.06) in FY than in GY and FC; whereas the concentration of stearic acid (C18:0) tended to be lower (P = 0.06) in FC than in GY and FY. The concentration of oleic acid (C18:1n9c) tended to be higher (P = 0.051) in FC than in the two yak groups; whereas the concentrations of linolelaidic acid (C18:2n6t) and linoleic acid (C18:2n6c) were lower (P < 0.05) in GY than in FC and FY. The concentration of α-linolenic acid (C18:3n3) was higher (P < 0.01) in GY than in FC and FY, the concentration of γ-linolenic acid (C18:3n6) was not detected in GY, and the concentration of tricosanoic acid (C23:0) was not detected in FC. Concentrations of henicosanoic acid (C21:0) and tricosanoic acid (C23:0) tended to be greater (P < 0.1) in GY than in FC and FY. Fatty acid cis-11,14,17-eicosatrienoic acid (C20:3n3) was detected only in GY, arachidonic acid (C20:4n6) was detected only in FC, and erucic acid (C22:1n9) was detected in GY and FC but not in FY. Fatty acids cis-13,16-docosadienoic acid (C22:2) and cis-4,7,10,13,16,19-docosahexaenoic acid (C22:6n3) were detected only in GY. There was a tendency (P = 0.06) of the total saturated fatty acid (SFA) content to be lower in FC than in GY and FY. The concentration of monounsaturated fatty acids (MUFAs) was higher in FC than in FY, but did not differ from that in GY. There was no significant difference in polyunsaturated fatty acids (PUFAs), medium-chain fatty acids (MCFAs), long-chain fatty acids (LCFAs), PUFA:SFA ratio, n3 fatty acids, antherogenic index (AI), and thrombogenicity index (TI) among the three groups. The concentration of n6 fatty acids was lower in GY than in FY, and the n6:n3 ratio of meat was 2.3 in GY, which was lower (P = 0.002) than the 8.1 in FC and 11.4 in FY.
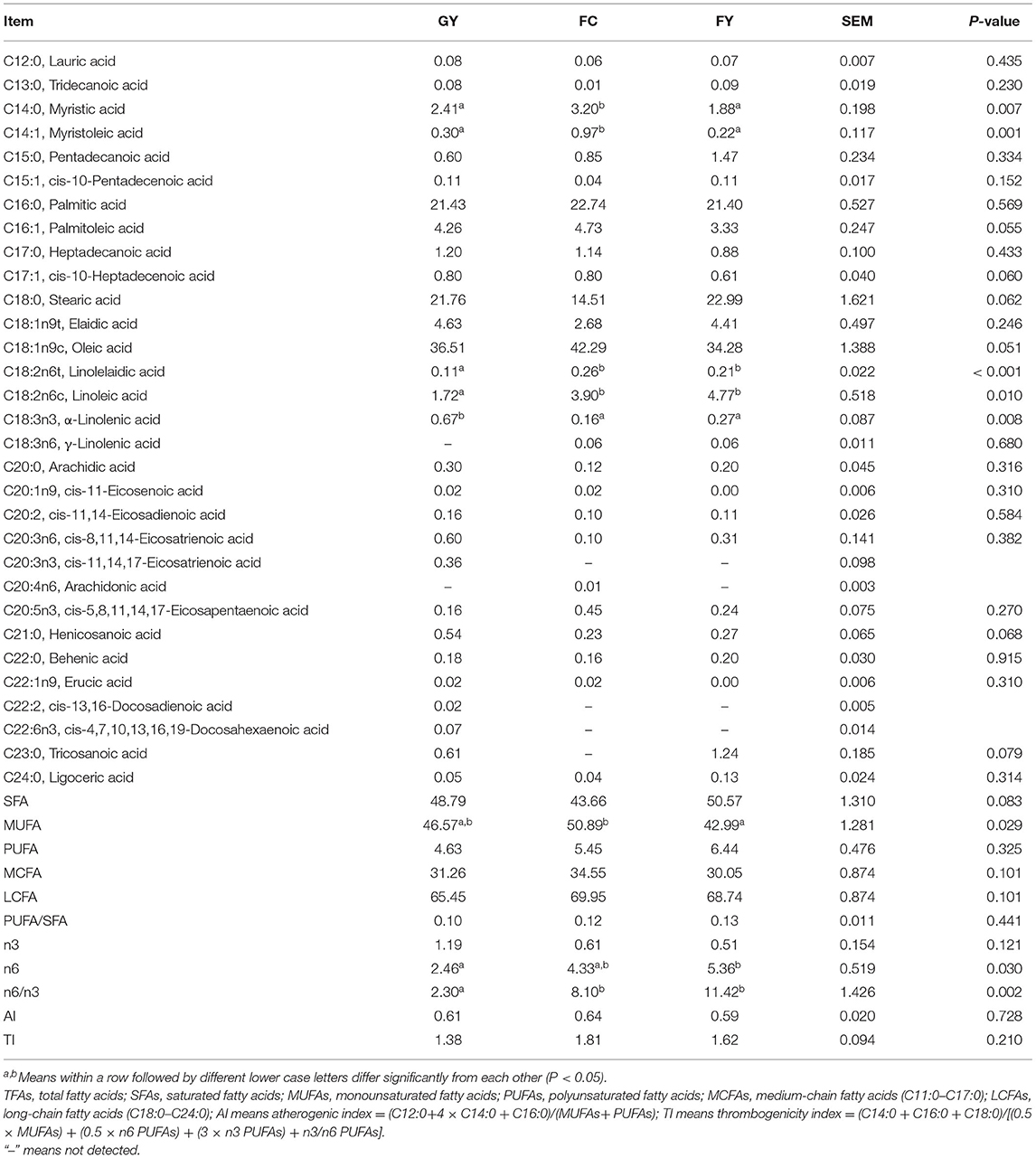
Table 6. Fatty acid composition (g/100 g fatty acid methyl) of Longissimus thoracis from GYs, FC, and FYs.
The meat of GY contained higher (P < 0.05) concentrations of isoleucine, tryptophan, tyrosine, leucine, and essential amino acids (EAAs) than the meat of FC, and the concentrations in FY did not differ from those in either group. Glutamic acid concentration in FC was lower than in GY and FY (P = 0.03); whereas the concentrations of methionine (P = 0.008) and phenylalanine (P = 0.001) were higher in GY than in FY and FC. The ratios of EAA acids to total amino acids (TAAs) and to non-essential amino acids (NEAAs) were higher (P < 0.001) in GY than in FY and FC, and in FY than in FC (Table 7).
Discussion
Rumen Morphology
Concentrate feed has been reported to stimulate the proliferation and growth of rumen epithelium (14, 15). For example, goats supplemented with dietary concentrate feed increased the length of rumen papillae when compared with grazing goats (16). This could explain the increased rumen papillae length of the two groups consuming concentrate in this study, especially for the feedlot yaks (FYs), when compared with the grazing yaks (GYs). These results also indicated that the yak rumen can adapt to a high-grain diet. High-grain diet can enhance the production of short-chain fatty acids (volatile fatty acids, VFAs) in the rumen, which are absorbed mainly by the rumen epithelium (17). The rumen epithelium absorbs 50–80% of VFAs, and depends mainly on the papillae surface area (3, 18). Therefore, the higher papillae surface area of FY facilitated the absorption of VFAs and nutrients (6) when compared with GY. It was also reported that the stratum corneum, which is formed in layers, can have four times the number of layers in a ruminant consuming a high concentrate diet than a high forage diet (19, 20). However, in this study, in contrast to these reports, the GY group had a thicker stratum corneum than the two feedlot bovines (FY and FC). The stratum corneum is in direct contact with the rumen milieu, and was observed to slough off throughout the epithelial surface when feeding high grain diet (15). Further research is required to explain this difference in stratum corneum thickness among groups and between feeds.
Ruminal Microbiota and Fermentation
Several methods have been employed to collect rumen samples for determining relative abundances of microbiota, namely, via oro-ruminal tube (21–24) and ruminal fistula (25, 26), and collection from a slaughtered animal (5, 27). The contents are often strained through cheesecloth and snap-frozen at −80°C until analyzed. It has been reported that the solid part of rumen contents contains ~70% of microbial mass, the liquid part ~25%, and the rumen epithelium and protozoa approximately 5% (28). Comparative studies have shown that there are differences in the relative abundances of bacteria among the methods of collection and among rumen samples (fluid, solid, or whole contents) analyzed (29, 30). For example, in the study of Cunha et al. (30) on Holstein cows, “mean relative abundance of Fibrobacteres was greater in solid and mixed liquid-solid than in liquid and oral samples,” whereas “no difference in mean relative abundance was detected in the 30 most prevalent genera among sample types.” Hendersen et al. (29), in their study on cows and sheep, stated that “the liquid fraction appeared to contain a higher relative abundance of the family Prevotellaceae (1.4-fold increase, 25.1–36.1%) and a lower abundance of the family Lachnospiraceae (1.6-fold decrease, 12.7–20.8%) when compared with total (and solid) rumen sample fraction. These findings generally agree with those of others” (three references are provided). Therefore, this study indicates that the same relation in relative abundance of bacteria exists across diets when comparing the different rumen samples, and that comparisons among studies on rumen bacteria cannot be made unless the same method of measurement is used. However, it also indicates that comparisons within a study can be made if the same method of measurement is used for all animals. In this study, the handling of rumen contents and preparation of the samples were performed in the same manner for all animals and were performed by the same person and, consequently, comparisons among the three bovine groups could be made.
The ruminal bacteria OTU data indicated that diet affected the bacterial community, more so than genotype. FY had more common communities with FC than with GY by more than a 4-fold difference. In addition, GY had 11-fold and 41-fold more unique OTUs when compared with FY and FC, respectively, which also explained the greater bacterial community diversity in GY than in FY and FC. The total OTUs of GY, which was lower than that of FY and FC, was also reflected by the Chao1 index. The greater diversity was a consequence of the high number of plant species in the natural pasture consumed by GY when compared with FY and FC. Natural forage diets provide a greater range of carbohydrate substrates (e.g., cellulose, semi-cellulose) and enhance the growth of microorganisms (31). In addition, high grain diets reduced rumen microbial richness and diversity (4, 32), which is related to higher feed efficiency (33). Nutrient-rich diets do not supply optimal fermenting substrate for all rumen microbes, especially for fiber-digesting bacteria (34, 35). The enhanced ruminal diversity with forage, when compared with high starch and lipid diets, is a consequence of the long-term co-evolution of microbes and yaks grazing on Alpine pasture. The higher Shannon index and lower Chao1 index of GY, compared with those of FY and FC, indicated greater evenness of OTUs in the GY group. Forage consumed by GY could be the reason for the high OTU evenness, as forages displayed more uniform OTU distributions than corn in denaturing gradient gel electrophoresis (DGGE) libraries (36).
Bacteroidetes and Firmicutes were the dominant phyla in the three bovine groups, which is consistent with previous studies (37–39). It was reported that a high concentrate diet decreased the ruminal abundance of Bacteroidetes, and increased the abundance of Firmicutes in cattle (31, 40, 41). This was also observed, at least partially, in this study, where the ruminal abundance of Bacteroidetes was lower in FC than in GY. The abundance in FY was intermediate between FC and GY but did not differ from that in either group. Firmicutes were not affected by the concentrate intake in this study, which could be, at least in part, because of the 18-h post-feeding sampling time. Previous studies have reported that Firmicutes were highest just before feeding and lowest ~12 h post feeding (42). It was reported that the ratio of F:B increased with a high energy diet (5, 43). This trend occurred in this study, albeit the differences were not significant, as GY had a ratio of 0.81, whereas FC had a ratio of 1.19, and FY had a ratio of 1.13. In this study, the relative abundance of Proteobacteria, which has little, if any, and cellulolytic activity (44) tended to be greater in FC than in GY and FY. Sheep breeds were reported to affect the abundance of Proteobacteria, which consists mostly of pathogenic bacteria (45). Therefore, the low abundance of Proteobacteria in the two yak groups indicates that this bacterial phylum is affected by genotype, as it was in sheep, and that yaks have a stronger ability to resist pathogens than cattle. The relative abundance of ruminal Fusobacteria was greater in FC and FY than in GY. Fusobacterial infections can cause liver abscesses in cattle (46), which indicates that high-concentrate feeding has the risk of causing liver damage. Studies demonstrated that a dietary shift from concentrate to pasture increased the abundance of ruminal Actinobacteria in sheep (23), but that its role is still unknown (47). High protein diets increased the relative abundance of ruminal Synergistetes that degrade amino acids rather than carbohydrates (48, 49). The high digestible carbohydrate proportion in the diet of FC and FY could be the reason for the inhibition of growth of Synergistetes. Few reports are available on ruminal Patescibacteria; however, the current results demonstrated that high-concentrate diets reduced the relative abundance of this phylum. In this study, the relative abundance of Chloroflexi increased with concentrate intake, as was reported earlier (50), but the function of this phylum is still uncertain.
Prevotella_1 and Succiniclasticum are the dominant ruminal genera in this study, as has been reported earlier in bovines (51). Prevotella_1, which uses mainly proteins as a substrate for growth (52), was correlated significantly with feed; whereas Succiniclasticum specializes in fermenting succinate to produce propionate (53). Ruminococcaceae, which was observed in different ovine breeds (54, 55), is a cellulolytic family of bacteria that plays a role in biohydrogenation (56). Hydrogen can be incorporated into succinate to increase Christensenellaceae, which is involved in carbohydrate digestion, and increases at the mid-fattening period of cattle (57, 58). However, in this study, the Ruminococcaceae_NK4A214_group was not correlated with genotype. Among genera, the Ruminococcaceae_NK4A214_group was correlated positively with the Christensenellaceae-R-7_group but negatively with Succiniclasticum. The competition to use succinate could explain the latter negative correlation. The higher relative abundance of the Christensenellaceae-R-7_group in FY than in FC was related with the fattening period of yaks.
Succinivibrionaceae_UCG-001 was a dominant genus in FC, present in FY but not detected in GY. It was reported that ruminal Succinivibrionaceae reduced methane emission (59), as occurs with a high-concentrate diet (60). However, FY had a low relative abundance of Succinivibrionaceae_UCG-001, which suggests that, presumably, a longer time period was required for colonization of the bacteria. The proliferation of Lactobacillus contributes to rumen lactate production when fed with a high-concentrate diet (61), which could be the reason for the high relative abundance in FC and FY when compared with GY. Concentrate promoted the relative abundance of both Succinivibrionaceae_UCG-001 and Lactobacillus, which also showed a positive correlation between them.
Rumen Muribaculaceae was correlated positively with feed nutrient digestibility in yaks (62). The concentrate intake in FY explained the higher relative abundance of uncultured _bacterium_f_Muribaculaceae in these yaks than in grazing yaks. The Rikenellaceae_RC9_gut_group degrades cellulose and hemicellulose (63, 64), while Bacteroidales enhances fiber digestion and is more abundant in high-forage than low-forage diets (65). Therefore, the high fiber content in forage could explain the increased growth of the Rikenellaceae_RC9_gut_group and uncultured_bacterium_f_Bacteroidales_UCG-001 in GY. Bacteroidales promotes ruminal acetate production (31), which was consistent with the higher acetate concentration in GY than in FC. The high forage fiber increased the abundance of the Rikenellaceae_RC9_gut_group and uncultured_bacterium_f_Bacteroidales_UCG-001, but decreased the abundance of uncultured -_bacterium_f_Muribaculaceae. The uncultured _bacterium_f_Muribaculaceae and the Rikenellaceae_RC9_gut_group were correlated significantly with genotype (yaks vs. cattle) in this study. Except for the top 10 genera, the higher relative abundances of other ruminal genera in the yak groups (GY and FY) than in FC indicate the greater rumen microbial diversity in yaks than in cattle.
Concentrations of ruminal total VFAs and acetate were greater in the two yak groups than in FC, while propionate and butyrate displayed the same pattern albeit were not significantly higher. This would indicate that yaks are more efficient in producing VFAs than cattle, that is, VFA production is dependent mainly on genotype and less so on dietary intake. This premise was supported in the study of Zhou et al., who reported that yaks produced greater concentrations of ruminal total VFAs than Qaidam Yellow cattle when consuming the same diet (66).
The KEGG data revealed that the rumen microbiota from feedlot-fattened cattle (FC) had an enhanced capacity to influence the metabolism of co-factors and vitamins, for nucleotide metabolism, replication and repair, transcription, and membrane transport. These metabolite changes indicate that the rumen microbiota in the FC group exhibited relatively high fluctuations and enhanced turnover rates (32), which were consistent with the reduced diversity and richness in FC when compared with GY.
Compared with FC, GY displayed enhanced metabolism of terpenoids and polyketides, and biosynthesis of other secondary metabolites. These responses were due to the large variety of secondary metabolites, such as polysaccharides, flavonoids and saponins, provided by natural pasture on the Qinghai-Tibetan Plateau (67). The metabolism of lipids, carbohydrates, and energy, all related with feed energy utilization and storage, was enhanced in grazing yaks when compared with the feedlot cattle in this study. Through long-term adaptations to energy stress, yaks have evolved traits of slow fat mobilization and strategies to conserve energy in the harsh alpine environment (68).
Fatty Acids and Meat Quality
Meat water content reflects meat texture, tenderness, and juiciness (69). The proportion of dietary forage intake did not affect meat water content in steers (4), which is in agreement with this study, as there was no difference among the three groups. The meat of FC had lower crude protein and higher fat contents than that of both yak groups, which did not differ between them, and which is in agreement with the study of Zi et al. (70). This indicates that genotype was the main factor affecting these components, as was stated in an earlier study (71), and that feed had a lesser effect, at least in yaks.
Microbial activity in the rumen is responsible for the hydrolysis of esterified plant lipids and liberation of unsaturated fatty acids, which determines the fatty acid composition of the meat (72). However, diet plays an important role in determining the tissue fatty acid content (73, 74). Myristic acid (C14:0) is regarded as an undesirable fatty acid in food because of its linkage with high blood cholesterol level in humans (75). However, it was reported that C14:0 could enhance intramuscular fat deposition in pork and increase the accumulation of myristic and myristoleic acids (C14:1) in meat tissues, which may have beneficial effects on human health (76). The higher concentrations of C14:0 and C14:1 in FC than in both yak groups could be due mainly to the effect of genotype, as Simmental was found to have a higher carcass C14:1 concentration than Red Angus (77). Palmitoleic acid (C16:1) and oleic acid (C18:1) were converted from palmitic acid (C16:0) and stearic acid (C18:0) by delta-9 desaturase (78). The tendency of higher C18:1n9c and lower C18:0 in FC than in the two yak groups indicates the high activity of delta-9 desaturase and high fat anabolism (79), which is consistent with the higher fat content in FC. It is also consistent with the report of Lobo et al. that genotype plays an important role in body fat anabolism (80). Lower concentrations of linoleic and linolelaidic acids (C18:2n6c and n6t) were observed in the meat of GY than in FC and FY, which was due to the lower content of C18:2 in grass than in concentrate feed (81), as C18:2n6c in meat is derived wholly from dietary intake (82). High levels of C18:2 can have adverse effect on odors and flavors of beef (83). The higher concentration of α-linoleic acid (C18:3n3) in forage than in concentrate feed is the reason for the higher concentration in the meat of GY than in the meat of FC and FY (84, 85), with no effect because of genotype (79). High ingestion of C18:3n3 could result in the increase of DHA (C22:6n3) in meat, which is the elongation product of C18:3n3 (74, 85). This can explain why DHA in meat was detected only in GY in this study. DHA has been reported to possess many healthy properties for humans, such as anti-obesity and improving cognitive function and neuronal development (86).
Particular attention has been paid to n-3 PUFAs, as they are reputed to possess the ability to prevent and treat cancer, coronary artery disease, hypertension, diabetes, and inflammatory and autoimmune disorders (87, 88). Food with a high n6:n3 ratio, however, can cause a number of diseases, such as cardiovascular problems, cancer, and inflammatory and autoimmune disorders (89). The high proportion of α-linoleic acid (n3 fatty acid) in GY led to a lower n6:n3 ratio (2.3) in these yaks than in FC (8.1) and FY (11.4). Most foods have am n6:n3 ratio ranging between 10:1 and 30:1 (88, 90); however, a ratio of <4 was recommended by the British Department of Health (91) to be healthy for humans, and only GY meat met this criterion. Consequently, from the point of DHA content and n6:n3 ratio, grazing yaks provide healthier meat than feedlot-fattened livestock.
Eicosatrienoic acid (C20:3n3) was detected only in GY, and arachidonic acid (C20:4n6) was detected only in FC, which indicates that grazing animals increased n3 fatty acid deposition in meat to a greater extent than feedlot animals fattened on a high concentrate diet. Furthermore, the intake of C18:3n3 increased the contents of n3 PUFAs and decreased n6 PUFAs in the meat of lambs (92), which also explains the lower n6:n3 ratio in GY than in FY and FC. Tricosanoic acid (C23:0), which is involved in the synthesis of ceramide and reduces the risk of diabetes (93, 94), was not detected in FC, but was present in the yaks (GY and FY). The tendency of lower saturated fatty acids (SFAs) in FC than in the two yak groups was attributed mainly to C18:0, which amounted to 30–45% of SFAs. Other studies found no difference in SFAs between confined and grazing cattle meat (95, 96). The higher total concentrations of monounsaturated fatty acids (MUFAs) in FC than in FY was related mainly to oleic acid. The insignificance of MUFAs between GY and FY indicates that feed did not affect the delt-9 desaturase activity to convert SFAs in yaks (97). No difference in PUFA and PUFA:SFA ratio was observed among the three bovine groups in this study. The PUFA:SFA ratios were all below the ratio (0.4) recommended by the World Health Organization (98), which is consistent with the reported values for cattle (~0.1) (54, 99), but lower than values reported for yaks (0.4–0.6) (100) and bison (0.4–0.6) (101). The atherogenic index (AI) and thrombogenicity index (TI) are related to the incidence of coronary heart disease (80, 102); there was no difference in these indices among GY, FC, and FY in this study.
The amino acids in meat affect the taste, at least, of beef (103). Glutamic acid is regarded as an important amino acid that adds flavor in meat (104), and FC had a higher content of glutamic acid than GY and FY. Other amino acids that add flavor to the meat, namely, glycine, alanine, and proline (105), did not differ among the three groups. The yak meat (GY and FY) had higher concentrations of phenylalanine than FC, and GY had higher concentrations than FC and FY of the essential or semi-essential amino acids for humans, namely, leucine, isoleucine, methionine, tryptophan, and tyrosine. According to FAO/WHO (106), healthy food composition should have an EAA:TAA ratio of at least 0.4 and an EAA:NEAA ratio of at least 0.6. Yak meat (GY and FY) obtained these ratios, with GY higher than FY, but FC did not.
Conclusions
Yaks fattened in a feedlot (FY) had a greater rumen papillae surface area and ruminal microbial diversity, but lesser microbial richness when compared with grazing yaks (GY) and cattle fattened in a feedlot (FC). Both the grazing and feedlot-fattened yaks had lower abundance of ruminal Proteobacteria and higher concentrations of volatile fatty acids than the feedlot-fattened cattle. The yaks, both grazing and feedlot-fattened, produced greater concentrations of ruminal VFAs and had meat with higher protein and lower fat contents than cattle. Meat from the grazing yaks had greater α-linolenic acid (C18:3n3) and lesser linolelaidic acid (C18:2n6t) concentrations, and n6:n3 ratios of fatty acids (<4) than the meat from feedlot-fattened meat (yak or Simmental). Yak meat had healthier ratios of EAA:TAA and EAA:NEAA than cattle meat, but the cattle meat had greater concentrations of flavor amino acids than the meat of the yaks.
Data Availability Statement
The datasets presented in this study can be found in online repositories. The names of the repository/repositories and accession number(s) can be found at: https://www.ncbi.nlm.nih.gov/sra/?term=PRJNA761282.
Ethics Statement
The animal study was reviewed and approved by Animal Care and Use Committee of Lanzhou University.
Author Contributions
LD designed the experiment. CH, CJ, and CM conducted the experiment. CH, LD, and AD wrote and revised the manuscript. BL and DL collected the meat samples. CH did the data analysis. All authors reviewed the manuscript.
Funding
This research was supported by Qinghai Provincial Science and Technology Major Project (2018-NK-A2), Platform of Adaptive Management on Alpine Grassland-livestock System (2020-ZJ-T07), STS grant from the Chinese Academy of Sciences (KFJ-STS-QYZD-113), Qinghai Key Research and Development Project (2017-NK-114), Key Lab Project of Qinghai Province (2013-Z-Y03), and China Agriculture Research System: National Modern Agriculture (Beef and Yak) Industrial Technology System Project (CARS-37).
Conflict of Interest
BL is employed by Gansu Devotion Biotechnology Co. Ltd. DL is employed by Qinghai Qilian Yida Meat Co. Ltd.
The remaining authors declare that the research was conducted in the absence of any commercial or financial relationships that could be construed as a potential conflict of interest.
Publisher's Note
All claims expressed in this article are solely those of the authors and do not necessarily represent those of their affiliated organizations, or those of the publisher, the editors and the reviewers. Any product that may be evaluated in this article, or claim that may be made by its manufacturer, is not guaranteed or endorsed by the publisher.
Acknowledgments
The authors would like to thank the Central Laboratory of the School of Life Sciences, Lanzhou University, for providing the instruments and helping in the laboratory analysis.
Supplementary Material
The Supplementary Material for this article can be found online at: https://www.frontiersin.org/articles/10.3389/fnut.2021.755255/full#supplementary-material
References
1. Bai WL, Yin RH, Zhao SJ, Li C, Ma ZJ, Yin RL, et al. A PCR assay for sex determination of yak (Bos grunniens) meat by amplification of the male-specific SRY gene. Food Control. (2010) 21:726–31. doi: 10.1016/j.foodcont.2009.10.016
3. Jing XP, Peng QH, Hu R, Zou HW, Wang HZ, Yu XQ, et al. Dietary supplements during the cold season increase rumen microbial abundance and improve rumen epithelium development in Tibetan sheep. J Anim Sci. (2018) 96:293–305. doi: 10.1093/jas/skx032
4. Ku MJ, Mamuad L, Nam KC, Cho YI, Kim SH, Choi YS, et al. The effects of total mixed ration feeding with high roughage content on growth performance, carcass characteristics, and meat quality of Hanwoo steers. Food Sci Anim Resour. (2021) 41:45–58. doi: 10.5851/kosfa.2020.e73
5. Hu R, Zou HW, Wang HZ, Wang ZS, Wang XY, Ma J, et al. Dietary energy levels affect rumen bacterial populations that influence the intramuscular fat fatty acids of fattening Yaks (Bos grunniens). Animls. (2020) 10:1474. doi: 10.3390/ani10091474
6. Diaz TG, Branco AF, Jacovaci FA, Jobim CC, Bolson DC, Daniel JLP. Inclusion of live yeast and mannan-oligosaccharides in high grain-based diets for sheep: ruminal parameters, inflammatory response and rumen morphology. PLoS ONE. (2018) 13:e0193313. doi: 10.1371/journal.pone.0193313
7. Shen ZM, Seyfert HM, Lohrke B, Schneider F, Zitnan R, Chudy A, et al. An energy-rich diet causes rumen papillae proliferation associated with more IGF type 1 receptors and increased plasma IGF-1 concentrations in young goats. J Nutr. (2004) 134:11–7. doi: 10.1093/jn/134.1.11
8. Wei HY, Ding LM, Wang XJ, Yan Q, Jiang CX, Hu CS, et al. Astragalus root extract improved average daily gain, immunity, antioxidant status and ruminal microbiota of early weaned yak calves. J Sci Food Agric. (2021) 101:82–90. doi: 10.1002/jsfa.10617
9. Stewart CS, Duncan SH. The effect of avoparcin on cellulolytic bacteria of the ovine rumen. J Gener Microb. (1985) 131:427–35. doi: 10.1099/00221287-131-3-427
10. Sukhija PS, Palmquist DL. Rapid method for determination of total fatty acid content and composition of feedstuffs and feces. J Agric Food Chem. (1988) 36:1202–6. doi: 10.1021/jf00084a019
11. Ding LM, Wang YP, Kreuzer M, Guo XS, Mi JD, Gou YJ, et al. Seasonal variations in the fatty acid profile of milk from yaks grazing on the Qinghai-Tibetan plateau. J Dairy Res. (2013) 80:410–7. doi: 10.1017/S0022029913000496
12. Ulbricht TLV, Southgate DAT. Coronary heart disease: 7 dietary factors. Lancet. (1991) 338:985–92. doi: 10.1016/0140-6736(91)91846-M
13. Dunn QJ. Multiple comparisons using rank sums. Technometrics. (1964) 6:241–52. doi: 10.1080/00401706.1964.10490181
14. Odongo NE, AlZahal O, Lindinger MI, Duffield TF, Valdes EV, Terrell SP, et al. Effects of mild heat stress and grain challenge on acid-base balance and rumen tissue histology in lambs. J Anim Sci. (2006) 84:447–55. doi: 10.2527/2006.842447x
15. Steele MA, Croom J, Kahler M, AlZahal O, Hook SE, Plaizier K, et al. Bovine rumen epithelium undergoes rapid structural adaptations during grain-induced subacute ruminal acidosis. Am J Physiol-Regul Integr Comp Physiol. (2011) 300:R1515–23. doi: 10.1152/ajpregu.00120.2010
16. Jiao JZ, Li XP, Beauchemin KA, Tang SX, Zhou CS. Rumen development process in goats as affected by supplemental feeding vs. grazing: age-related anatomic development, functional achievement and microbial colonisation. Brit J Nutr. (2015) 113:888–900. doi: 10.1017/S0007114514004413
17. Sato S. Pathophysiological evaluation of subacute ruminal acidosis (SARA) by continuous ruminal pH monitoring. Anim Sci J. (2016) 87:168–77. doi: 10.1111/asj.12415
18. Aschenbach JR, Penner GB, Stumpff F, Gaebel G, Ruminant Nutrition Symposium. Role of fermentation acid absorption in the regulation of ruminal pH. J Anim Sci. (2011) 89:1092–107. doi: 10.2527/jas.2010-3301
19. Gaebel G, Martens H, Suendermann M, Galfi P. The effect of diet, intraruminal pH and osmolarity on sodium, chloride and magnesium absorption from the temporarily isolated and washed reticulo-rumen of sheep. Q J Exp Physiol. (1987) 72:501–11. doi: 10.1113/expphysiol.1987.sp003092
20. Baldwin RL, Connor EE. Rumen function and development. Vet Clin N Am-Food Anim Pract. (2017) 33:427. doi: 10.1016/j.cvfa.2017.06.001
21. Schären M, Drong C, Kiri K, Riede S, Gardener M, Meyer U, et al. Differential effects of monensin and a blend of essential oils on rumen microbiota composition of transition dairy cows. J Dairy Sci. (2017) 100:2765–83. doi: 10.3168/jds.2016-11994
22. Schären M, Frahm J, Kersten S, Meyer U, Hummel J, Breves G, et al. Interrelations between the rumen microbiota and production, behavioral, rumen fermentation, metabolic, and immunological attributes of dairy cows. J Dairy Sci. (2018) 101:4615–37. doi: 10.3168/jds.2017-13736
23. Belanche B, Kingston-Smith AH, Griffith GW, Newbold CJ. A multi-kingdom study reveals the plasticity of the rumen microbiota in response to a shift from non-grazing to grazing diets in sheep. Front Microbiol. (2019) 10:122. doi: 10.3389/fmicb.2019.00122
24. Li FY, Li CX, Chen YH, Liu JH, Zhang CY, Irving B, et al. Host genetics influence the rumen microbiota and heritable rumen microbial features associate with feed efficiency in cattle. Microbiome. (2019) 7:92. doi: 10.1186/s40168-019-0699-1
25. Chen XD, Su XD, Li JL, Yang YT, Wang PY, Yan F, et al. Real-time monitoring of ruminal microbiota reveals their roles in dairy goats during subacute ruminal acidosis. Npj Biofilms Microbiomes. (2021) 7:45. doi: 10.1038/s41522-021-00215-6
26. Castillo C, Hernández J. Ruminal fistulation and cannulation: a necessary procedure for the advancement of biotechnological research in ruminants. Animals. (2021) 11:1870. doi: 10.3390/ani11071870
27. Han XP, Liu HJ, Hu LY, Zhao N, Xu SX, Lin ZJ, et al. Bacterial community characteristics in the gastrointestinal tract of yak (Bos grunniens) fully grazed on pasture of the Qinghai-Tibetan Plateau of China. Animals. (2021) 11:2243. doi: 10.3390/ani11082243
28. Matthews C, Crispie F, Lewis E, Reid M, O'Toole PW, Cotter PD. The rumen microbiome: a crucial consideration when optimising milk and meat production and nitrogen utilisation efficiency. Gut Microbes. (2019) 10:115–32. doi: 10.1080/19490976.2018.1505176
29. Henderson G, Cox F, Kittelmann S, Miri VH, Zethof M, Noel SJ, et al. Effect of DNA Extraction methods and sampling techniques on the apparent structure of cow and sheep rumen microbial communities. PLoS ONE. (2013) 8:e74787. doi: 10.1371/journal.pone.0074787
30. Cunha L, Peixoto PMG, Elolimy AA, Canisso IF, Cardoso FC, Bicalho RC, et al. Comparison of rumen microbiome structure in samples collected using an oro-esophageal probe, and solid, liquid and combined solid-liquid fractions collected using a rumen fistula in Holstein dairy cows. Available online at: https://www.researchgate.net/publication/336855132 (accessed July 25, 2021).
31. Liu HJ, Xu TW, Xu SX, Ma L, Han XP, Wang XG, et al. Effect of dietary concentrate to forage ratio on growth performance, rumen fermentation and bacterial diversity of Tibetan sheep under barn feeding on the Qinghai-Tibetan plateau. PeerJ. (2019) 7:e7462. doi: 10.7717/peerj.7462
32. Zhang RY, Liu YJ, Yin YY, Jin W, Mao SY, Liu JH. Response of rumen microbiota, and metabolic profiles of rumen fluid, liver and serum of goats to high-grain diets. Animal. (2019) 13:1855–64. doi: 10.1017/S1751731118003671
33. Shabat SKB, Sasson G, Doron-Faigenboim A, Durman T, Yaacoby S, Berg Miller ME, et al. Specific microbiome-dependent mechanismsunderlie the energy harvest efficiency of ruminants. ISME J. (2016) 10:2958–72. doi: 10.1038/ismej.2016.62
34. De Filippo C, Cavalieri D, Di Paola M, Ramazzotti M, Poullet JB, Massart S, et al. Impact of diet in shaping gut microbiota revealed by a comparative study in children from Europe and rural Africa. Proc Natl Acad Sci. (2010) 14691–6. doi: 10.1073/pnas.1005963107
35. Costa-Roura S, Balcells J, de la Fuente G, Mora-Gil J, Llanes N, Villalba D. Nutrient utilization efficiency, ruminal fermentation and microbial community in Holstein bulls fed concentrate-based diets with different forage source. Anim Feed Sci Technol. (2020) 269:114662. doi: 10.1016/j.anifeedsci.2020.114662
36. Kocherginskaya SA, Aminov RI, White BA. Analysis of the rumen bacterial diversity under two different diet conditions using denaturing gradient gel electrophoresis, random sequencing, and statistical ecology approaches. Anaerobe. (2001) 7:119–34. doi: 10.1006/anae.2001.0378
37. Xin JW, Chai ZX, Zhang CF, Zhang Q, Zhu Y, Cao HW, et al. Comparing the microbial community in four stomach of dairy cattle, yellow cattle and three Yak herds in Qinghai-Tibetan Plateau. Front Microbiol. (2019) 10:157. doi: 10.3389/fmicb.2019.01547
38. Leng J, Xie LJ, Zhu RJ, Yang SL, Gou X, Li S, et al. Dominant bacterial communities in the rumen of Gayals (Bos frontalis), Yaks (Bos grunniens) and Yunnan Yellow Cattle (Bos taurs) revealed by denaturing gradient gel electrophoresis. Mol Biol Rep. (2011) 38:4863–72. doi: 10.1007/s11033-010-0627-8
39. Huang XD, Tan HY, Long RJ, Liang JB, Wright ADG. Comparison of methanogen diversity of yak (Bos grunniens) and cattle (Bos taurus) from the Qinghai-Tibetan plateau, China. BMC Microbiol. (2012) 12:237. doi: 10.1186/1471-2180-12-237
40. Petri RM, Schwaiger T, Penner GB, Beauchemin KA, Forster RJ, McKinnon JJ, et al. Characterization of the core rumen microbiome in cattle during transition from forage to concentrate as well as during and after an acidotic challenge. PLoS ONE. (2013) 8:e83424. doi: 10.1371/journal.pone.0083424
41. Mao SY, Zhang RY, Wang DS, Zhu WY. Impact of subacute ruminal acidosis (SARA) adaptation on rumen microbiota in dairy cattle using pyrosequencing. Anaerobe. (2013) 24:12–9. doi: 10.1016/j.anaerobe.2013.08.003
42. Wang LJ, Li Y, Zhang YG, Wang LH. The effects of different concentrate-to-forage ratio diets on rumen bacterial microbiota and the structures of Holstein cows during the feeding cycle. Animals. (2020) 10:957. doi: 10.3390/ani10060957
43. Liu H, Jiang H, Hao LZ, Cao XL, Degen AA, Zhou JW, et al. Rumen bacterial community of grazing lactating yaks (Poephagus grunniens) supplemented with concentrate feed and/or rumen-protected lysine and methionine. Animals. (2021) 11:2425. doi: 10.3390/ani11082425
44. Mao SY, Zhang ML, Liu JH, Zhu WY. Characterising the bacterial microbiota across the gastrointestinal tracts of dairy cattle: membership and potential function. Sci Rep. (2015) 5:16116. doi: 10.1038/srep16116
45. Mani S, Aiyegoro OA, Adeleke MA. Characterization of rumen microbiota of two sheep breeds supplemented with direct-fed lactic acid bacteria. Front Vet Sci. (2021) 7:570074. doi: 10.3389/fvets.2020.570074
46. Tadepalli S, Narayanan SK, Stewart GC, Chengappa MM, Nagaraja TG. Fusobacterium necrophorum: a ruminal bacterium that invades liver to cause abscesses in cattle. Anaerobe. (2009) 15:36–43. doi: 10.1016/j.anaerobe.2008.05.005
47. Trabi EB, Seddik HE, Xie F, Wang XF, Liu JH, Mao S. Effect of pelleted high-grain total mixed ration on rumen morphology, epithelium-associated microbiota and gene expression of proinflammatory cytokines and tight junction proteins in Hu sheep. Anim Feed Sci Technol. (2020) 263:114453. doi: 10.1016/j.anifeedsci.2020.114453
48. Hugenholtz P, Hooper SD, Kyrpides NC. Focus: synergistetes. Environ Microbiol. (2009) 11:1327–9. doi: 10.1111/j.1462-2920.2009.01949.x
49. Wetzels SU, Mann E, Pourazad P, Qumar M, Pinior B, Metzler-Zebeli BU, et al. Epimural bacterial community structure in the rumen of Holstein cows with different responses to a long-term subacute ruminal acidosis diet challenge. J Dairy Sci. (2017) 100:1829–44. doi: 10.3168/jds.2016-11620
50. Mamuad LL, Kim SH, Lee SS, Dae C. Adaptation period during diet of dairy changes rumen fermentation characteristics and microbial qualities and communities. J Anim Sci. (2017) 95:372–372. doi: 10.2527/asasann.2017.869
51. Xue D, Chen H, Zhao XQ, Xu SX, Hu LY, Xu TW, et al. Rumen prokaryotic communities of ruminants under different feeding paradigms on the Qinghai-Tibetan Plateau. Syst Appl Microbiol. (2017) 40:227–36. doi: 10.1016/j.syapm.2017.03.006
52. Cui XX, Wang ZF, Tan YH, Chang SH, Zheng HR, Wang HY, et al. Selenium yeast dietary supplement affects rumen bacterial population dynamics and fermentation parameters of tibetan sheep (Ovis aries) in alpine meadow. Front Microbiol. (2021) 12:663945. doi: 10.3389/fmicb.2021.663945
53. Van Gylswyk NO. Succiniclasticum ruminis gen. nov, sp nov, a rumen bacterium converting succinate to propionate as sole energy yielding mechanism. Int J Syst Bacteriol. (1995) 45:297–300. doi: 10.1099/00207713-45-2-297
54. Manzano HAP, Kononoff PJ, Fernando SC. Evaluation of rumen bactyerial composition in Holstein and Jersey cows under simillar dietary conditions using different sampling methods. J Anim Sci. (2016) 94:170. doi: 10.2527/msasas2016-363
55. Dai QD, Ma J, Cao G, Hu R, Zhu YX, Li GY, et al. Comparative study of growth performance, nutrient digestibility, and ruminal and fecal bacterial community between yaks and cattle-yaks raised by stall-feeding. AMB Express. (2021) 11:98. doi: 10.1186/s13568-021-01259-9
56. Huws SA, Kim EJ, Lee MRF, Scott MB, Tweed JKS, Pinloche E, et al. As yet uncultured bacteria phylogenetically classified as Prevotella, Lachnospiraceae incertae sedis and unclassified Bacteroidales, Clostridiales and Ruminococcaceae may play a predominant role in ruminal biohydrogenation. Environ Microbiol. (2011) 13:1500–12. doi: 10.1111/j.1462-2920.2011.02452.x
57. Greening C, Geier R, Wang C, Woods LC, Morales SE, McDonald MJ, et al. Diverse hydrogen production and consumption pathways influence methane production in ruminants. ISME J. (2019) 13:2617–32. doi: 10.1038/s41396-019-0464-2
58. Miura H, Hashimoto T, Kawanishi Y, Kawauchi H, Inoue R, Shoji N, et al. Identification of the core rumen bacterial taxa and their dynamics during the fattening period in Janpanese Black cattle. Anim Sci J. (2021) 92:e13601. doi: 10.1111/asj.13601
59. Pope PB, Smith W, Denman SE, Tringe SG, Barry K, Hugenholtz P, et al. Isolation of Succinivibrionaceae implicated in low methane emissions from Tammar Wallabies. Science. (2011) 333:646–8. doi: 10.1126/science.1205760
60. Johnson KA, Johnson DE. Methane emissions from cattle. J Anim Sci. (1995) 73:2483–92. doi: 10.2527/1995.7382483x
61. Zhang RY, Liu JH, Jiang LS, Mao SY. Effect of high-concentrate diets on microbial composition, function, and the VFAs formation process in the rumen of dairy cows. Anim Feed Sci Technol. (2020) 269:114619. doi: 10.1016/j.anifeedsci.2020.114619
62. Ma H, Zhu YX, Wang ZS, Yu X, Hu R, Wang XY, et al. Glutamine supplementation affected the gut bacterial community and fermentation leading to improved nutrient digestibility in growth-retarded yaks. FEMS Microbiol Ecol. (2021) 97:fiab084. doi: 10.1093/femsec/fiab084
63. Asma Z, Sylvie C, Laurent C, Jérôme M, Christophe K, Olivier B, et al. Francis Microbial ecology of the rumen evaluated by 454 GS FLX pyrosequencing is affected by starch and oil supplementation of diets. FEMS Microbiol Ecol. (2013) 83:504–14. doi: 10.1111/1574-6941.12011
64. Sha YZ, Hu J, Shi BG, Dingkao RQ, Wang JQ, Li SB, et al. Characteristics and functions of the rumen microbial community of Cattle-Yak at different ages. Biomed Res Int. (2020) 2020:3482692. doi: 10.1155/2020/3482692
65. Henderson G, Cox F, Ganesh S, Jonker A, Young W, Janssen PH. Rumen microbial community composition varies with diet and host, but a core microbiome is found across a wide geographical range. Sci Rep. (2015) 5:14567. doi: 10.1038/srep14567
66. Zhou JW, Liu H, Zhong CL, Degen AA, Yang G, Zhang Y, et al. Apparent digestibility, rumen fermentation, digestive enzymes and urinary purine derivatives in yaks and Qaidam cattle offered forage-concentrate diets differing in nitrogen concentration. Livest Sci. (2018) 208:14–21. doi: 10.1016/j.livsci.2017.11.020
67. Qiao YM, Wang QJ, Wang WY. Yak (Bos grunnies L) grazing effects on vegetation of alpine meadow with Potentilla fruticosa L (Rosaceae) Shrub in Qinghai-Tibet Plateau. Pol J Ecol. (2009) 57:769–77.
68. Xiong L, Pei J, Wu XY, Kalwar Q, Liang CN, Guo X, et al. The study of the response of fat metabolism to long-term energy stress based on serum, fatty acid and transcriptome profiles in yaks. Animals. (2020) 10:1150. doi: 10.3390/ani10071150
69. Wu FY, Smith SB. Ionic strength and myofibrillar protein solubilization. J Anim Sci. (1987) 65:597–608. doi: 10.2527/jas1987.652597x
70. Zi XD, Zhong GH, Wen YL, Zhong JC, Liu CL, Ni YA, et al. Growth performance, carcass composition and meat quality of Jiulong-Yak (Bos grunniens). Asian Australas J Anim Sci. (2004) 17:410–4. doi: 10.5713/ajas.2004.410
71. Xie XX, Meng QX, Cui ZL, Ren LP. Effect of cattle breed on meat quality, muscle fiber characteristics, lipid oxidation and fatty acids in China. Asian Australas J Anim Sci. (2012) 25:824–31. doi: 10.5713/ajas.2011.11462
72. Harfoot CG. Lipid metabolism in the rumen. Prog in Lipid Res. (1978) 17:21–54. doi: 10.1016/0079-6832(78)90004-6
73. Scollan ND, Dhanoa MS, Choi NJ, Maeng WJ, Enser M, Wood JD. Biohydrogenation and digestion of long chain fatty acids in steers fed on different sources of lipid. J Agrc Sci. (2001) 136:345–55. doi: 10.1017/S0021859601008796
74. Vatansever L, Kurt E, Enser M, Nute GR, Scollan ND, Wood JD, et al. Shelf life and eating quality of beef from cattle of different breeds given diets differing in n-3 polyunsaturated fatty acid composition. Anim Sci. (2000) 71:471–82. doi: 10.1017/S135772980005548X
75. French P, O'Riordan EG, Monahan F, Caffrey PJ, Moloney AP. Fatty acid composition of intra-muscular triacylglycerols of steers fed autumn grass and concentrates. Livest Prod Sci. (2003) 81:307–17. doi: 10.1016/S0301-6226(02)00253-1
76. Lu NS, Shu G, Xie QP, Zhu XT, Gao P, Zhou GX, et al. Myristic acid (MA) promotes adipogenic gene expression and the differentiation of porcine intramuscular adipocyte precursor cells. J Integr Agric. (2014) 13:2488–99. doi: 10.1016/S2095-3119(13)60664-4
77. Laborde FL, Mandell IB, Tosh JJ, Wilton JW, Buchanan-Smith JG. Breed effects on growth performance, carcass characteristics, fatty acid composition, and palatability attributes in finishing steers. J Anim Sci. (2001) 79:355–65. doi: 10.2527/2001.792355x
78. Smith SB, Lunt DK, Chung KY, Choi CB, Tume RK, Zembayashi M. Adiposity, fatty acid composition, and delta-9 desaturase activity during growth in beef cattle. Anim Sci J. (2006) 77:478–86. doi: 10.1111/j.1740-0929.2006.00375.x
79. Wood JD, Enser M, Fisher AV, Nute GR, Sheard PR, Richardson RI, et al. Fat deposition, fatty acid composition and meat quality: a review. Meat Sci. (2008) 78:343–58. doi: 10.1016/j.meatsci.2007.07.019
80. Bezerra Oliveira Lobo AM, Delmondes Bomfim MA, Faco O, Fernandes Junior GA, Ponciano MF, Braga Lobo RN. Intramuscular fat and fatty acid profile of muscle of lambs finished in irrigated pasture. J Appl Anim Res. (2014) 42:110–7. doi: 10.1080/09712119.2013.822810
81. Nascimento CO, Pina DS, Cirne LGA, Santos SA, Araújo MLGML, Rodrigues TCGC, et al. Effects of whole corn germ, a source of linoleic acid, on carcass characteristics and meat quality of feedlot lambs. Animals. (2021) 11:267. doi: 10.3390/ani11020267
82. Romero-Bernal J, Almaraz EM, Ortega OAC, Salas NP, Gonzalez-Ronquillo M. Chemical composition and fatty acid profile in meat from grazing lamb diets supplemented with ryegrass hay, fishmeal and soya bean meal as PUFA sources. Cienc Rural. (2017) 47:e20160533. doi: 10.1590/0103-8478cr20160533
83. Wood JD, Enser M, Fisher AV, Nute GR, Sheard PR. Manipulating meat quality and composition. Proc Nutr Soc. (1999) 58:363–70. doi: 10.1017/S0029665199000488
84. Fisher AV, Enser M, Richardson RI, Wood JD, Nute GR, Kurt E, et al. Fatty acid composition and eating quality of lamb types derived from four diverse breed × production systems. Meat Sci. (2000) 55:141–7. doi: 10.1016/S0309-1740(99)00136-9
85. Wang X, Yan SM, Shi BL, Zhang Y, Guo XY. Effects of concentrate supplementation on fatty acid composition and expression of lipogenic genes of meat and adipose tissues in grazing lambs. Ital J Anim Sci. (2019) 18:554–63. doi: 10.1080/1828051X.2018.1547666
86. Jia W, Shi QY, Shi L. Effect of irradiation treatment on the lipid composition and nutritional quality of goat meat. Food Chem. (2021) 351:129295. doi: 10.1016/j.foodchem.2021.129295
87. Simopoulos AP. The importance of the omega-6/omega-3 fatty acid ratio in cardiovascular disease and other chronic diseases. Exp Biol Med. (2008) 233:674–88. doi: 10.3181/0711-MR-311
88. Simopoulos AP. Evolutionary aspects of diet: the omega-6/omega-3 ratio and the brain. Mol Neurobiol. (2011) 44:203–15. doi: 10.1007/s12035-010-8162-0
89. Simopoulos AP. The importance of the ratio of omega-6/omega-3 essential fatty acids. Biomed Pharmacother. (2002) 56:365–79. doi: 10.1016/S0753-3322(02)00253-6
90. Valencak TG, Gamsjäger L, Ohrnberger S, Culbert NJ, Ruf T. Healthy n-6/n-3 fatty acid composition from five European game meat species remains after cooking. BMC Res Notes. (2015) 8:273. doi: 10.1186/s13104-015-1254-1
91. Department of Health. Report on Health and Social subjects. Nutritional aspects of cardiovascular disease. Report of the Cardiovascular Review Group Committee on Medical Aspects of Food Policy. London, UK: HMSO. (1994). p. 46.
92. Ebrahimi M, Rajion MA, Goh YM. Effects of oils rich in linoleic and alpha-Linolenic acids on fatty acid profile and gene expression in goat meat. Nutrients. (2014) 6:3913–28. doi: 10.3390/nu6093913
93. Forouhi NG, Koulman A, Sharp SJ, Imamura F, Kroger J, Schulze MB, et al. Differences in the prospective association between individual plasma phospholipid saturated fatty acids and incident type 2 diabetes: the EPIC-InterAct case-cohort study. Lancet Diabetes Endocrinol. (2014) 2:810–8. doi: 10.1016/S2213-8587(14)70146-9
94. Otto MCD, Lemaitre RN, Sun Q, King IB, Wu JHY, Manichaikul A, et al. Genome-wide association meta-analysis of circulating odd-numbered chain saturated fatty acids: results from the CHARGE consortium. PLoS ONE. (2018) 13:e0196951. doi: 10.1371/journal.pone.0196951
95. Blanco M, Casasús I, Ripoll G, Panea B, Albertí P, Joy M. Lucerne grazing compared with concentrate-feeding slightly modifies carcase and meat quality of young bulls. Meat Sci. (2010) 84:545–52. doi: 10.1016/j.meatsci.2009.10.010
96. Duckett SK, Neel JPS, Lewis RM, Fontenot JP, Clapham WM. Effects of forage species or concentrate finishing on animal performance, carcass and meat quality. J Anim Sci. (2013) 91:1454–67. doi: 10.2527/jas.2012-5914
97. Dinh TTN, Blanton JR, Riley DG, Chase CC, Coleman SW, Phillips WA, et al. Intramuscular fat and fatty acid composition of longissimus muscle from divergent pure breeds of cattle. J Anim Sci. (2010) 88:756–66. doi: 10.2527/jas.2009-1951
98. World Health Organization. Diet, Nutrition and the Prevention of Chronic Diseases. Geneva: WHO (2003).
99. He L, Yang J, Chen W, Zhou Z, Wu H, Meng Q. Growth performance, carcass trait, meat quality and oxidative stability of beef cattle offered alternative silages in a finishing ration. Animal. (2018) 12:657–66. doi: 10.1017/S1751731117001902
100. Hao LZ, Xiang Y, Degen A, Huang YY, Ni JZ, Sun L, et al. Adding heat-treated rapeseed to the diet of yak improves growth performance and tenderness and nutritional quality of the meat. Anim Sci J. (2019) 90:1177–84. doi: 10.1111/asj.13266
101. Janssen J, Cammack K, Legako J, Cox R, Grubbs JK, Underwood K, et al. Influence of grain- and grass-finishing systems on carcass characteristics, meat quality, nutritional composition, and consumer sensory attributes of Bison. Foods. (2021) 10:1060. doi: 10.3390/foods10051060
102. Szyndler-Nedza M, Swiatkiewicz M, Migdal L, Migdal W. The quality and health-promoting value of meat from pigs of the native breed as the effect of extensive feeding with acorns. Animals. (2021) 11:789. doi: 10.3390/ani11030789
103. Macleod G. The Flavour of Beef. New York, NY: Springer, US (1994). doi: 10.1007/978-1-4615-2177-8_2
104. Watkins PJ, Frank D, Singh TK, Young OA, Warner RD. Sheepmeat flavor and the effect of different feeding systems: a review. J Agric Food Chem. (2013) 61:3561–79. doi: 10.1021/jf303768e
105. Chen GS, Cai Y, Su YY, Wang D, Pan XL, Zhi XJ. Study of meat quality and flavour in different cuts of Duroc-Bamei binary hybrid pigs. Vet Med Sci. (2020) 7:724–34. doi: 10.1002/vms3.409
Keywords: grazing, feedlot, yak, cattle, rumen microbiota, meat quality
Citation: Hu CS, Ding LM, Jiang CX, Ma CF, Liu BT, Li DL and Degen AA (2021) Effects of Management, Dietary Intake, and Genotype on Rumen Morphology, Fermentation, and Microbiota, and on Meat Quality in Yaks and Cattle. Front. Nutr. 8:755255. doi: 10.3389/fnut.2021.755255
Received: 08 August 2021; Accepted: 11 October 2021;
Published: 11 November 2021.
Edited by:
Satyanarayan R. S. Dev, Florida Agricultural and Mechanical University, United StatesReviewed by:
Yury Tatiana Granja-Salcedo, Colombian Corporation for Agricultural Research (AGROSAVIA), ColombiaKeawin Sarjeant, Florida A&M University, United States
Copyright © 2021 Hu, Ding, Jiang, Ma, Liu, Li and Degen. This is an open-access article distributed under the terms of the Creative Commons Attribution License (CC BY). The use, distribution or reproduction in other forums is permitted, provided the original author(s) and the copyright owner(s) are credited and that the original publication in this journal is cited, in accordance with accepted academic practice. No use, distribution or reproduction is permitted which does not comply with these terms.
*Correspondence: Luming Ding, ZGluZ2xtJiN4MDAwNDA7bHp1LmVkdS5jbg==