- 1Key Laboratory of Molecular Animal Nutrition of the Ministry of Education, Key Laboratory of Animal Nutrition and Feed Science (Eastern of China) of the Ministry of Agriculture, Key Laboratory of Animal Feed and Nutrition of Zhejiang, College of Animal Sciences, Institute of Animal Nutrition and Feed Sciences, Zhejiang University, Hangzhou, China
- 2School of Life Science and Engineering, Foshan University, Foshan, China
Clostridium perfringens is an important zoonotic pathogen associated with food contamination and poisoning, gas gangrene, necrotizing enterocolitis or necrotic enteritis in humans and animals. Dysbacteriosis is supposedly associated with the development of C. perfringens infection induced necrotic enteritis, but the detailed relationship between intestinal health, microbiome, and C. perfringens infection-induced necrotic enteritis remains poorly understood. This research investigated the effect of probiotics on the growth performance and intestinal health of broilers, and the involved roles of intestinal microbiota and microbial metabolic functions under C. perfringens infection. Results showed that subclinical necrotic enteritis was successfully induced as evidenced by the significant lower body weight (BW), suppressed feed conversion ratio (FCR), decreased ileal villus height and mucosal barrier function, and increased ileal histopathological score and bursal weight index. Lactobacillus plantarum or Paenibacillus polymyxa significantly attenuated C. perfringens-induced compromise of growth performance (BW, FCR) and ileal mucosa damage as illustrated by the increased ileal villus height and villus/crypt ratio, the decreased ileal histopathological score and the enhanced ileal mucosal barrier function. L. plantarum also significantly alleviated C. perfringens-induced enlarged bursa of fabricius and the decreased levels of ileal total SCFAs, acetate, lactate, and butyrate. Furthermore, dietary L. plantarum improved C. perfringens infection-induced intestinal dysbiosis as evidenced by significantly enriched short-chain fatty acids-producing bacteria (Lachnospiraceae, Ruminococcaceae, Oscillospira, Faecalibacterium, Blautia), reduced drug-resistant bacteria (Bacteroides, Alistipes) and enteric pathogens (Escherichia coli, Bacteroides fragilis) and bacterial metabolic dysfunctions as illustrated by significantly increased bacterial fatty acid biosynthesis, decreased bacterial lipopolysaccharide biosynthesis, and antibiotic biosynthesis (streptomycin and vancomycin). Additionally, the BW and intestinal SCFAs were the principal factors affecting the bacterial communities and microbial metabolic functions. The above findings indicate that dietary with L. plantarum attenuates C. perfringens-induced compromise of growth performance and intestinal dysbiosis by increasing SCFAs and improving intestinal health in broilers.
Introduction
Clostridium perfringens (C. perfringens) is a widely distributed anaerobic spore-forming zoonotic pathogen, which causes foodborne illnesses in humans and necrotic enteritis in animals (1, 2). Foodborne illness caused by C. perfringens-contaminated food in the United States is estimated to be nearly 1 million cases per year (2). Necrotic enteritis induced by C. perfringens is a widespread avian intestinal necrotic disease, which is estimated to cause the total global economic loss in poultry industry to be over US$6 billion annually (3). Clinical form of necrotic enteritis is characterized by sudden death and increased mortality of chickens, with a mortality rate from 2 to 50% (4). Subclinical infection leads to disrupted villus-crypt micro-architecture and reduced nutrient digestion and absorption, which adversely decreases feed conversion and impairs growth performance (4, 5). Infeed antibiotics used to be the main strategy for preventing or controlling necrotic enteritis in poultry production. However, with the increasing public concerns about antimicrobial resistance and antibiotic residues in food animal products, infeed antimicrobial growth promoters have been widely removed from the animal feed by increasing global countries (6, 7). Subsequently, outbreaks of necrotic enteritis have become a significant economic concern for poultry farmers, especially in subclinical form, which shows unobvious pathological symptoms and thereby compromises the growth performance (8). The withdrawal of infeed prophylactic antibiotics and outbreaks of necrotic enteritis in the commercial poultry industry inspires an interest in seeking effective alternative antimicrobial strategies to prevent or control necrotic enteritis outbreaks. In recent years, multiple dietary alternatives to prophylactic antibiotics, such as probiotics, prebiotics, plant extracts, enzymes, and organic acids, have been proved to be effective in reducing or abolishing C. perfringens-induced necrotic enteritis (5, 9).
Intestinal microbes play crucial roles in the development of the intestinal defense system (immune function and barrier function) and in regulating the processes of inflammation and maintaining homeostasis (10–12). It is reported that the anomalous intestinal microbiota is associated with the development of necrotic enteritis in animals or necrotizing enterocolitis in humans, but interactive roles of intestinal microbes in this relationship remain poorly understood (13, 14). As a potential advanced alternative to antibiotic growth promoters, many studies have shown that probiotics and commensal microbes exert beneficial effects on inhibiting growth and toxin secretion of pathogens, modulating gastrointestinal immune systems against adhesion and invasion of pathogens, restoring altered intestinal microbes, maintaining gastrointestinal homeostasis, and promoting tissue healing (15–17). Many studies have proved that L. plantarum exerts antibacterial activities by secreting lactic acid (18) and plantaricin (19, 20), and P. polymyxa exerts antibacterial activities by secreting polymyxin and lantibiotic (21, 22). Although numerous studies have demonstrated that probiotics, such as lactobacillus, bacillus, and yeast, could alleviate or abolish C. perfringens infection-induced necrotic enteritis (23–26), the involved interactive roles of intestinal microbiota, microbial metabolic functions, and short-chain fatty acids (SCFAs) under C. perfringens infection-induced necrotic enteritis remain poorly understood. Our previous works found that two probiotics, Lactobacillus plantarum (Lac16) and Paenibacillus polymyxa (BSC10), had in vitro anti-C. perfringens activities and Lac16 protected-Caenorhabditis elegans against C. perfringens infection (Supplementary Figure 1). The present study further evaluated the effect of the two probiotics on growth performance and intestinal health of broilers challenged with C. perfringens, and the interactive roles of intestinal microbial communities, bacterial metabolic functions, and SCFAs under C. perfringens infection condition.
Experimental Procedures
Bacteria Preparation
L. plantarum (Lac16) deposited in China Center for Type Culture Collection (CCTCC M2016259) was isolated from fermented vegetables, and P. polymyxa (BSC10) was purchased from China General Microbiological Culture Collection Center (CGMCC1.10711). The Lac16 and BSC10 were separately cultured in DeMan-Rogosa-Sharpe and Luria-Bertani broth at 37°C for overnight under aerobic conditions. Clostridium perfringens type A (ATCC13124, Cp) was cultured in reinforced clostridium medium at 37°C for 20 h in anaerobic gas-generating packs (Mitsubishi Gas Chemical Company Inc., Tokyo, Japan). After centrifugation at 3,500 × g for 10 min at 4°C, the BSC10, Lac16, and Cp pellets were collected and then washed three times with sterile phosphate-buffered saline (PBS, pH 7.2), respectively. Finally, the concentration of the bacteria was constantly checked by spreading the plate method (27).
Chicken Experiment
Seven hundred and twenty hatched 1-day-old Cobb 500 broilers with similar body weight were randomly allocated into four treatments with six pens per group and 30 birds per pen: (1) Control group: birds were fed a basal diet (Supplementary Table 1) formulated to meet the nutritional requirements of broilers (28), (2) Cp group: birds were fed a basal diet and then orally challenged with C. perfringens type A cultures [1. × 108 colony-forming units (CFU)/bird] on day 1 and during day 14–20 of age, (3) BSC10+Cp group: birds were fed a basal diet supplemented with P. polymyxa (1. × 108 CFU/kg feed) and orally challenged with C. perfringens A cultures (1. × 108 CFU/bird) on day 1 and during day 14–20 of age, (4) Lac16 + Cp group: birds were fed a basal diet supplemented with L. plantarum (1. × 108 CFU/kg feed) and orally challenged with C. perfringens A cultures (1. × 108 CFU/bird) on day 1 and during day 14–20 of age. The animal experiment lasted for 21 days. Birds were allowed ad libitum access to mashed diets and fresh water. Feed consumption and body weight were recorded every week. Mortality was recorded every day, and dead birds were weighed to adjust estimates of body weight gain, feed intake, and feed conversion ratio.
Sample Collection
At day 21 of age, six birds from each group were randomly selected, weighed, and euthanized by electrical stun after deprivation of feed for 6 h (05:00–11:00 A.M.). After being removed and weighed, spleen and bursa of fabricius indexes were calculated as a percentage relative to body weight. The ileal segments were fixed in 4% paraformaldehyde for hematoxylin and eosin (H&E) staining or in 2.5% buffered glutaraldehyde for transmission electron microscopy (TEM). The ileal contents and whole caecum of birds were sampled, snap frozen in liquid nitrogen, and stored at −80°C for short-chain fatty acids (SCFAs) and microbial analysis.
Ileal Morphological Analysis
After being fixed in 4% paraformaldehyde, ileal samples were embedded in paraffin, sliced, dehydrated, and stained with hematoxylin and eosin. Images were observed by Olympus microsystem (Tokyo, Japan). Histopathological scores of the ileal samples were examined by three independent observers as previously described (29).
For TEM observation, after being fixed in 2.5% buffered glutaraldehyde, ileal segments were washed three times by a cold 100-mM phosphate buffer, and then postfixed in cold 0.1% buffered osmium tetroxide (OsO4) for 2 h. After being washed by a phosphate buffer, the ileal segments were rapidly dehydrated in ascending grades of ethanol (30, 50, 70, 95, and 100%), and then transferred into a 1:1 mixture of propylene oxide and epoxy araldite. The ultrathin ileal sections were embedded and cut by an LKB Nova ultramicrotome (Leica Microsystems, Buffalo Grove, IL) and then stained with uranyl acetate. Transmission electron micrographs of the ileal samples were then observed and captured by the transmission electron microscope (JEOL, Tokyo, Japan).
Lactate and SCFAs Analysis
Lactate levels in ileal digesta were determined by the Lactic Acid assay kit (NanJingJianCheng Bioengineering Institute, Nanjing, China) according to the instructions of the manufacturer. SCFAs contents in ileal digesta were measured as follows: 1 g of digesta was mixed with 2. ml of distilled water. After being homogenized and centrifuged at 5,000 × g for 10 min at 4°C, 500 μl of the supernatant were mixed with 0.2 ml 25% (w/v) phosphoric acid and then stored at −20°C for overnight. After thawing, the mixtures were centrifuged (15,000 × g for 10 min at 4°C), filtered by 0.22-μm membrane filter and then analyzed by gas chromatography (Varian CP-3800, USA). Quantification of SCFAs was carried out by using the external calibration standard curves method and expressed as μmol/g of wet ileal digesta.
Microbial Analysis
The bacterial genomic DNA from cecal contents was extracted under sterile conditions using the TIANamp Stool DNA Kit (Tiangen, Beijing, China) and was stored at −80°C for PCR amplification and sequencing. The V3-V4 hypervariable region of the 16S rRNA gene was amplified by using the 341F/805R primer pairs, and the amplicon sequencing was performed on an Illumina MiSeq platform (Illumina Inc., San Diego, CA, USA). The Quantitative Insights into Microbial Ecology (QIIME) software (version 1.9.1) was used for the quality filter of raw sequences and a cluster of filtered sequences into operational taxonomic unit (OTU) at 97% similarity (30). Bacterial OTU representative sequences were assigned to a taxonomic lineage by a Ribosomal Database Project (RDP) classifier based on the Greengenes 13.8 database.
Alpha and beta diversities of the microbial community were analyzed based on a subsample of a minimum number of sequences (12981) by QIIME software. Significant differences in microbial beta diversity and metagenome predicational functions among different groups (based on the Bray-Curtis distance matrices) were calculated by ANOSIM (analysis of similarities), PERMANOVA (permutational multivariate analysis of variance), and MRPP (multi-response permutation procedure) analyses using a “vegan” package and were visualized by Principal coordinates analysis (PCoA) using the “ggplot2” package of R software (v4.1.0). Canonical correspondence analysis (CCA) and variation partitioning analysis (VPA) were performed using the R package “vegan.” The linear discriminant analysis (LDA) effect size (LEfSe) analysis (https://huttenhower.sph.harvard.edu/galaxy/) was performed to analyze and characterize the bacterial differences and microbial predicted pathway functions among different groups.
The metagenome functional predictions based on 16S rRNA gene sequencing of bacterial communities were analyzed by the Phylogenetic Investigation of Communities by the Reconstruction of Unobserved States 1 (PICRUSt 1) method (31). The OTU table and representative sequences subsampled at a minimum number of sequences (12981) were selected for the functional annotation to KEGG ortholog groups (KO) based on KEGG databases. Significant differential predicted pathway abundances were then analyzed and visualized by statistical analysis of taxonomic and functional profiles (STAMP) software with a two-sided Welch's t-test (32). Pearson correlation between phenotypic variables was analyzed and visualized by R software (v4.1.0) using the “corrplot” package. Mantel test was performed to examine the linkage between phenotypic variables and microbial communities or microbial predicted pathways (33).
The co-occurrence patterns were constructed to visualize the correlations between bacterial communities and microbial predicted pathways. Firstly, only those bacterial OTUs and microbial predicted KOs pathways with an average relative abundance >0.1% across all samples were retained according to Hartman et al. (34). We then normalized the filtered bacterial OTUs and microbial predicted KOs pathways separately by the “trimmed means of M” (TMM) method, and the normalized counts were expressed as relative abundance counts per million (CPM) using the R package “edgeR.” The indicator species of the filtered bacterial OTUs and microbial predicted KOs pathways were analyzed using the R package “indicspecies.” Differential OTUs and KOs abundances among all the groups were also analyzed by likelihood ratio tests (LRT) using the R package “edgeR.” The differential OTUs and KOs at a value of p < 0.05 were defined as OTUs and KOs responsive. Treatment-sensitive OTUs and KOs (hereafter: tsNodes) were then confirmed by both indicator species analysis and LRT. The TMM-normalized CPM counts of bacterial OTUs and microbial-predicted KOs pathways were then combined and further calculated the Spearman rank correlations by the R package “Hmisc.” Significant correlations (ρ > 0.7 and FDR-adjusted p < 0.01) were kept as the edges of the co-occurrence networks. Then, the co-occurrence networks were visualized using the Fruchterman-Reingold layout by the R package “igraph.” The network topological properties and modules were also calculated and identified to describe the complex patterns of the interrelationships. Keystone OTUs and KOs were identified as those nodes within top 1% of node degree values in the networks.
Statistical Analysis
Data on growth performance and ileal histomorphology analysis were assessed using SPSS™ software (SPSS Inc., Chicago, IL, USA) by one-way ANOVA, and the contrast of means was evaluated by Tukey's multiple range tests. The data on SCFAs analysis were analyzed by two-tailed Student's T-test using SPSS™ software.
Results
Growth Performance
Performance results showed that, compared with the control group, C. perfringens infection significantly (p < 0.05) decreased the body weight and feed conversion and significantly (p < 0.05) increased bursal weight index of broilers, whereas dietary with P. polymyxa or L. plantarum significantly (p < 0.05) ameliorated C. perfringens-induced side effects of growth performance (body weight and feed conversion) (Table 1). Additionally, L. plantarum treatment also attenuated C. perfringens-induced enlarged bursa of fabricius (p < 0.05).
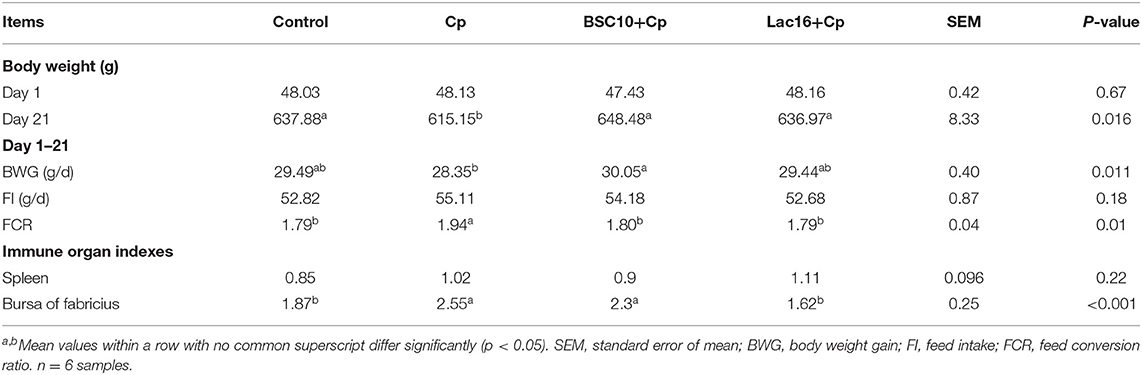
Table 1. Effect of probiotics on growth performance and immune organ indexes of broilers infected with C. perfringens.
Morphological Observation
As presented in Table 2, C. perfringens infection significantly (p < 0.05) decreased the villus height and significantly (p < 0.05) increased the histopathological score of the ileum compared with those of the uninfected birds. Dietary with P. polymyxa or L. plantarum significantly (p < 0.05) ameliorated C. perfringens-induced ileal mucosa injury, as evidenced by the significantly (p < 0.05) increased villus height and villus height to crypt depth ratio, and decreased crypt depth and the histopathological score of the ileum.
TEM results showed that, compared with the control group, the ileum of the C. perfringens-infected birds showed sparse microvilli, disrupted and shorter tight junction, adherens junction, and desmosomes (Figure 1). Compared with the C. perfringens-infected group, the improved ileal intercellular junctional complexes of broilers in BSC10+Cp and Lac16+Cp groups were observed as evidenced by higher and ordered microvillus, longer tight junction and adherens junction, and darker desmosomes.
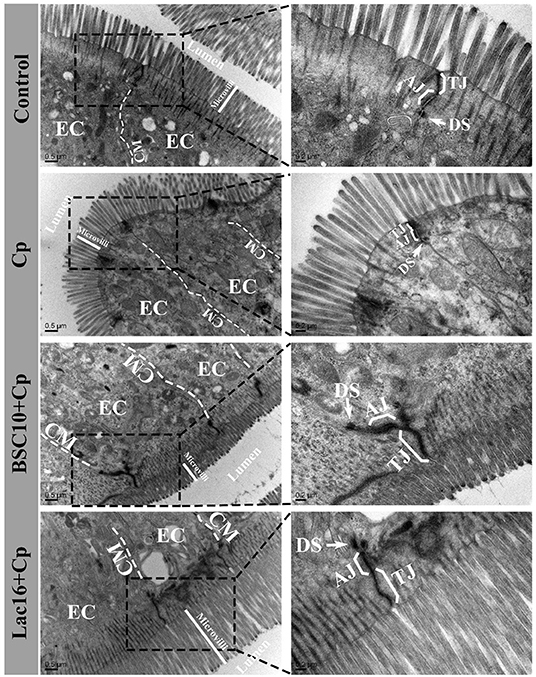
Figure 1. Transmission electron micrographs of the ileal microvilli in broilers at day 21 of age. EC, epithelial cell; CM, cell membrane; TJ, tight junction; AJ, adherens junction. The white arrow indicates desmosomes (DS).
SCFAs in Ileal Digesta
The levels of total SCFAs, acetate, lactate, and butyrate in ileal digesta of the C. perfringens-infected broilers were significantly (p < 0.01) decreased compared with the uninfected broilers (Figure 2). Compared with the C. perfringens-infected group, P. polymyxa treatment increased the concentrations of total SCFAs, acetate, lactate, and butyrate in ileal digesta of the C. perfringens-infected broilers but had no significant differences (p > 0.05). Dietary with L. plantarum significantly (p < 0.05) increased the contents of total SCFAs, acetate, lactate, and butyrate in ileal digesta of the C. perfringens-infected broilers.
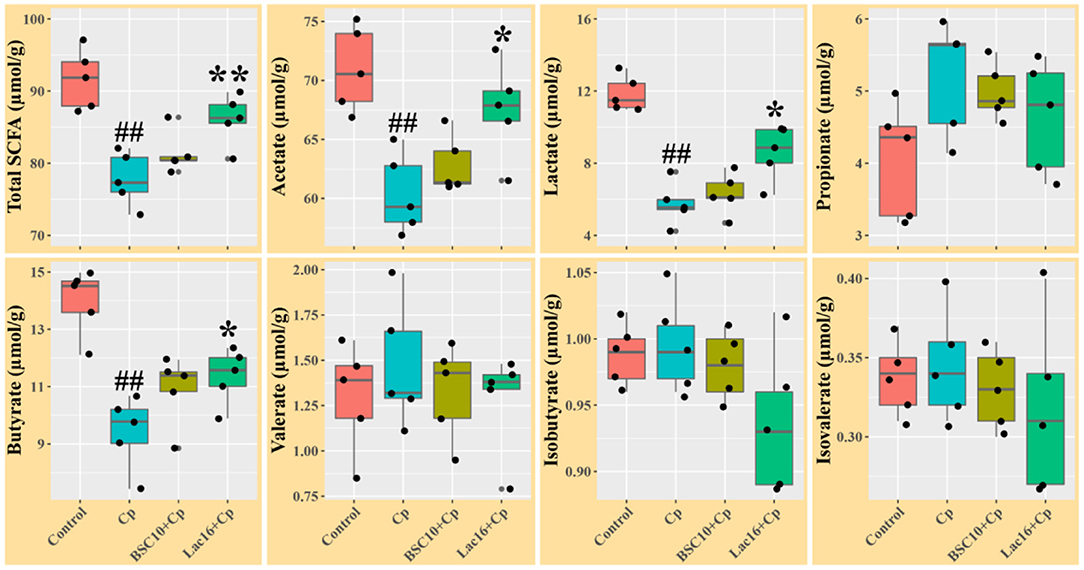
Figure 2. Short chain fatty acids levels in ileal digesta of broilers at day 21 of age. Significant differences vs. the control group: ##p < 0.01. Significant differences vs. the Cp group: *p < 0.05; **p < 0.01. n = five samples.
Microbial Composition
Alpha diversity analysis showed that no significant difference was observed among the four groups (p > 0.05, Figure 3A). PCoA based on Bray-Curtis distance showed that the microbial communities were clustered into two different types of communities (Figure 3B). The microbial communities of broilers in control and Lac16+Cp groups formed a cluster and formed another cluster in the Cp and BSC10+Cp groups. Significant differences in beta diversity of microbial communities among all treatments were further confirmed by ANOSIM, PERMANOVA, and MRPP analyses (Table 3).
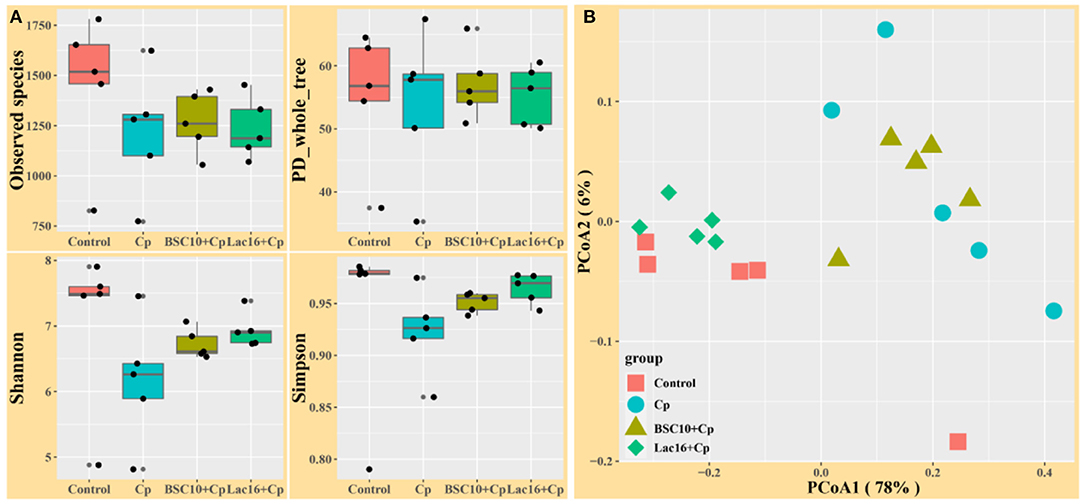
Figure 3. Diversity analyses of microbial communities among groups. (A) Alpha diversity analysis (B) Principal coordinates analysis (PCoA) based on Bray-Curtis distance. n = five samples.
LEfSe analysis was employed to explore the specific microbial features among the four groups (Figure 4). It was found that 36 taxa biomarkers in four groups were identified with LDA score >3, which mainly belong to the phyla of Firmicutes, Bacteroidetes, Proteobacteria, Tenericutes, and Cyanobacteria. Differential analysis results (Figure 5) further showed that, compared with the uninfected group, C. perfringens infection significantly (p < 0.05 or p < 0.01) reduced the relative abundances of Firmicutes, Clostridia, Clostridiales, Lachnospiraceae, Ruminococcaceae, [Ruminococcus], Oscillospira, Blautia, and Clostridium citroniae in the cecum, while markedly (p < 0.05 or p < 0.01) increased the relative abundances of Bacteroidetes, Rikenellaceae, Alistipes, Alistipesmassiliensis, Clostridium, and Clostridium methylpentosum. Compared with the C. perfringens-infected birds, the significantly (p < 0.05 or p < 0.01) increased Oscillospira abundance and decreased relative abundances of Faecalibacterium, and Escherichia coli were observed in the broilers of the BSC10+Cp group. Furthermore, L. plantarum significantly (p < 0.05 or p < 0.01) improved the anomalous microbial composition induced by C. perfringens infection, as evidenced by the increased relative abundances of Firmicutes, Clostridia, Clostridiales, Lachnospiraceae, Ruminococcaceae, [Ruminococcus], Oscillospira, Faecalibacterium, and Blautia and the reduced relative abundances of Bacteroidetes, Rikenellaceae, Bacteroides, Alistipes, Alistipesmassiliensis, Clostridium, Escherichia coli, Bacteroides fragilis, Bacteroides acidifaciens, Clostridium methylpentosum (Figure 5).
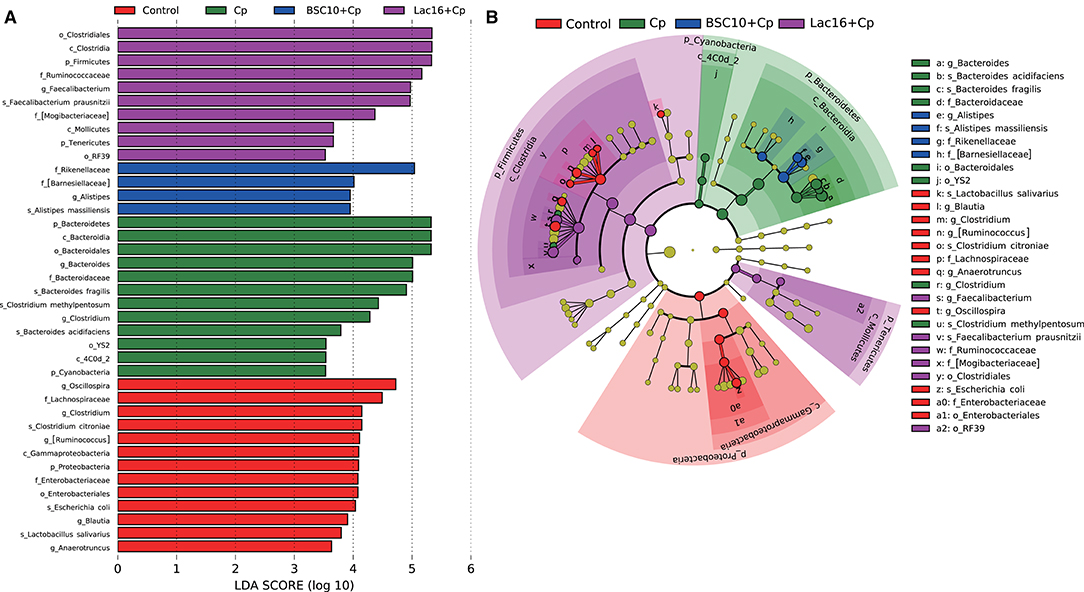
Figure 4. Linear discriminant analysis (LDA) effect size (LEfSe) analysis (LDA > 3., p < 0.05) of the intestinal microbes. Histogram (A) and cladogram (B) of LEfSe analysis among the four groups. The prefixes “p_,” “c_,” “o_,” “f_,” “g_,” and “s_” represent the annotated levels of phylum, class, order, family, genus, and species.
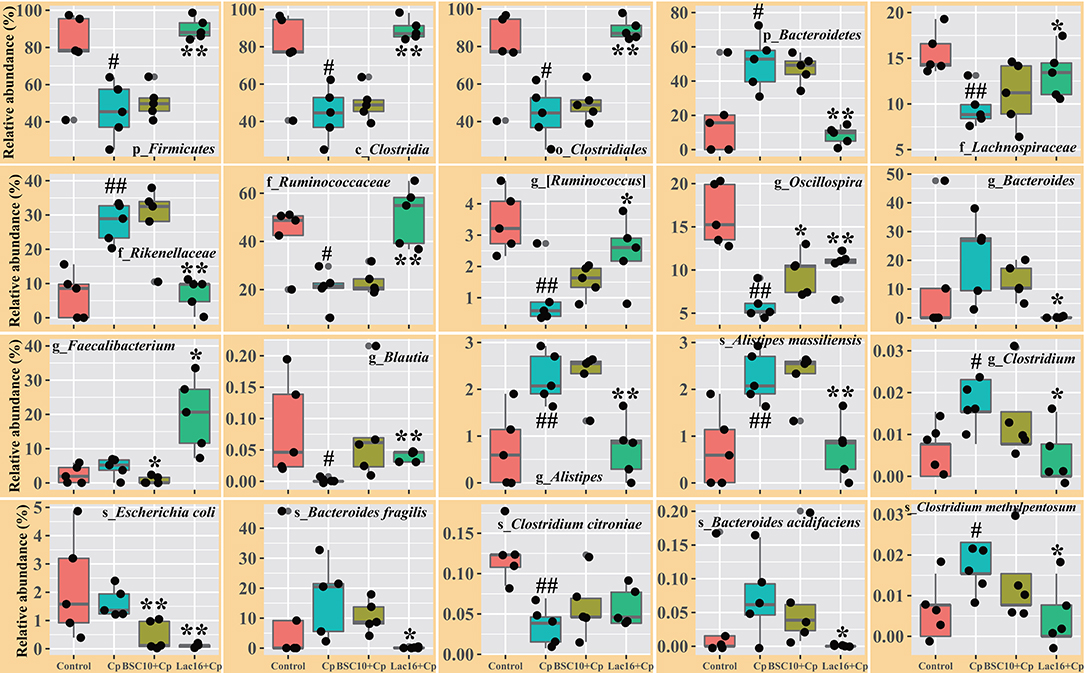
Figure 5. Comparison of the intestinal microbiota among the groups. Significant differences vs. the control group: #p < 0.05; ##p < 0.01. Significant differences vs. the Cp group: *p < 0.05; **p < 0.01. The prefixes “p_,” “c_,” “o_,” “f_,” “g_,” and “s_” represent the annotated level of phylum, class, order, family, genus, and species. n = five samples.
Metagenome Functional Predictions
PICRUSt analysis was performed to explore the metagenome functions based on 16S rRNA marker gene sequences. PCoA results based on Bray-Curtis distance showed that the microbial metabolic functions were significantly distinct among the groups (Figure 6). Consisted with PCoA results for microbial communities, C. perfringens infection also altered the bacterial metabolic functions, whereas dietary with L. plantarum significantly (R2 = 0.781, P = 0.009) ameliorated the shifts of bacterial metabolic functions induced by C. perfringens infection. The STAMP and LEfSe analysis based on level 1 and level 3 of the microbial-predicted pathway functions further verified the differences in metabolic functions (Figures 7, 8 and Supplementary Figure 2). Specifically, L. plantarum treatment significantly (p < 0.05) inversed the shifts of bacterial metabolic functions induced by C. perfringens infection, such as metabolism, lipopolysaccharide (LPS) biosynthesis, LPS biosynthesis proteins, fatty acid biosynthesis, streptomycin biosynthesis, biosynthesis of vancomycin group antibiotics, cysteine and methionine metabolism, lysine biosynthesis, thiamine metabolism, histidine metabolism, and cell division (Figure 8 and Supplementary Figure 2). Dietary with P. polymyxa had less effects on microbial metabolic functions than L. plantarum in C. perfringens-infected broilers (Figure 8 and Supplementary Figure 2).
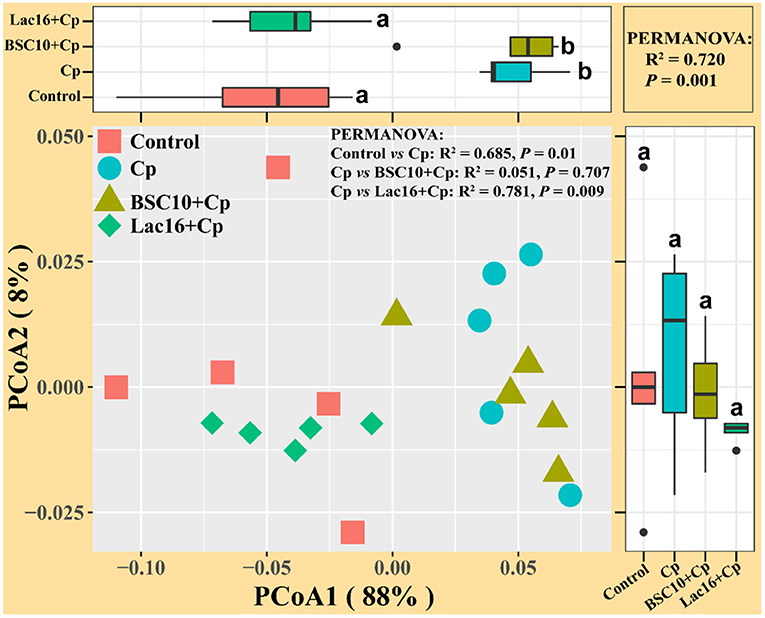
Figure 6. Principal coordinates analysis (PCoA) of predicated metabolic functions among groups based on Bray-Curtis distance. a,bindicate a significant difference (p < 0.05).
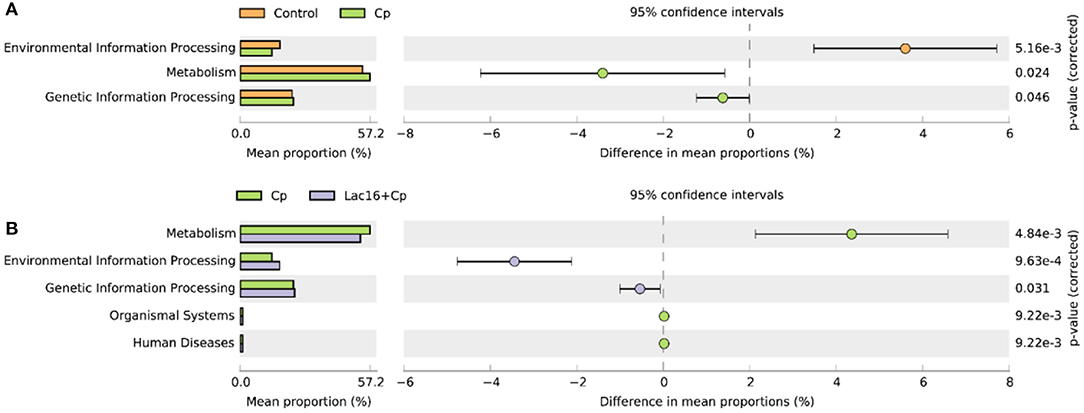
Figure 7. Comparison of predicted metabolic pathway abundances between the groups by statistical analysis of taxonomic and functional profiles (STAMP) at Level 1. (A) Control vs. Cp; (B) Cp vs. Lac16+Cp.
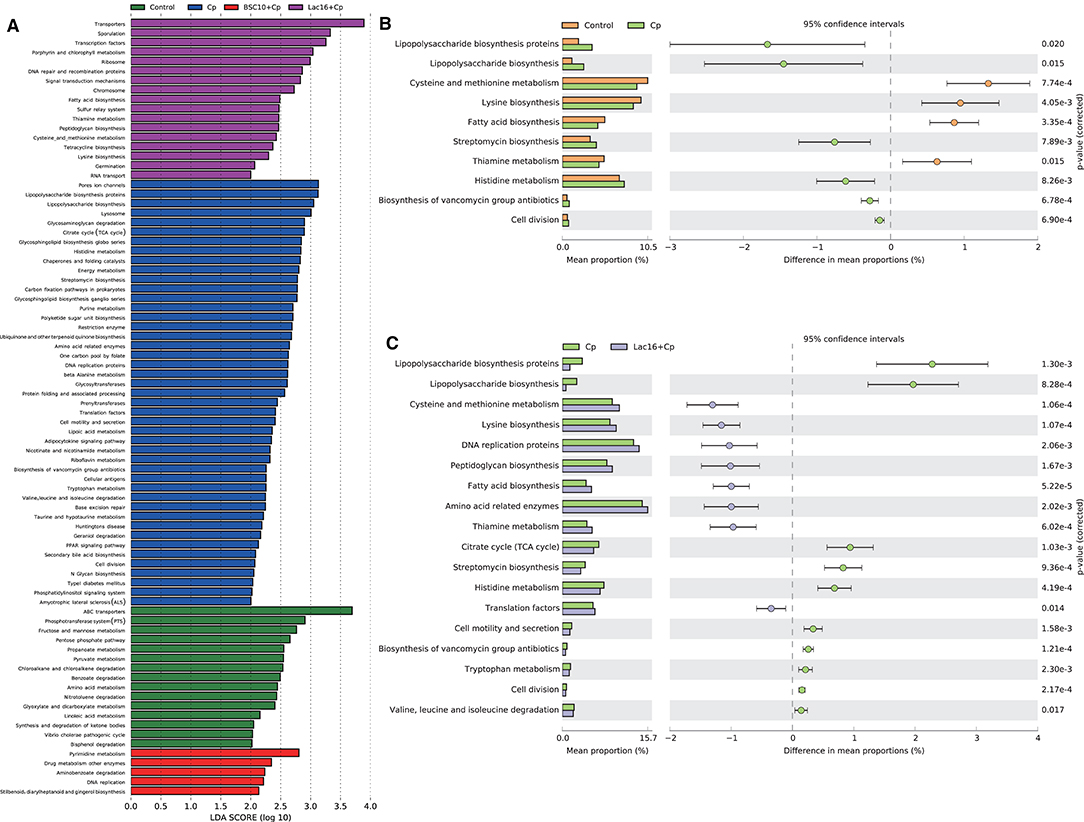
Figure 8. (A) LEfSe analysis (LDA > 2., p < 0.01) of the microbial-predicted metabolic pathway functions at Level 3. (B,C) Comparison of predicted pathway abundances between the groups by statistical analysis of taxonomic and functional profiles (STAMP) at Level 3. (B) Control vs. Cp; (C) Cp vs. Lac16+Cp.
Relationships Between Phenotypic Variables, Bacterial Communities and Microbial Metabolic Functions
The shifts in microbial community and metabolic functional composition induced by C. perfringens infection and dietary with probiotics were tightly linked to phenotypic variables and ileal SCFAs as revealed by the Mantel test (Figure 9), CCA and VPA analyses (Supplementary Figure 3). Pearson correlation analysis (Figure 9) showed that the ileal SCFAs (including total SCFAs, acetate, lactate and butyrate) were positively (p < 0.001) correlated with the final body weight, whereas were negatively (p < 0.05 or p < 0.01) correlated with immune organ indexes (spleen and bursa of Fabricius). The bursa of Fabricius index was positively (p < 0.05) correlated with propionate and isobutyrate. Additionally, the spleen index was negatively (p < 0.05) correlated with valerate, isobutyrate, and isovalerate. Mantel correlation analysis (Figure 9) showed that taxonomic composition was significantly correlated with bursa of Fabricius index (0.25 < r < 0.5, 0.001 < p < 0.01), total SCFAs (0.25 < r < 0.5, 0.01 < p < 0.05), acetate (r < 0.25, 0.01 < p < 0.05), lactate (r < 0.25, 0.01 < p < 0.05), and butyrate (r < 0.25, 0.01 < p < 0.05). The microbial functional compositions were significantly correlated with the final body weight (r < 0.25, 0.01 < p < 0.05), bursa of Fabricius index (r > 0.5, 0.001 < p < 0.01), total SCFAs (r > 0.5, 0.001 < p < 0.01), acetate (r > 0.5, 0.001 < p < 0.01), lactate (r > 0.5, 0.001 < p < 0.01), and butyrate (0.25 < r < 0.5, 0.001 < p < 0.01). CCA analysis showed that bursa of Fabricius index (R2 = 0.56, P = 0.004; R2 = 0.55, P = 0.004), ileal total SCFAs (R2 = 0.58, P = 0.002; R2 = 0.68, P = 0.001), acetate (R2 = 0.53, P = 0.002; R2 = 0.67, P = 0.001), lactate (R2 = 0.66, P = 0.002; R2 = 0.65, P = 0.001), and butyrate (R2 = 0.51, P = 0.005; R2 = 0.72, P = 0.001) were significantly correlated with the bacterial (Supplementary Figure 3A) and microbial functional (Supplementary Figure 3B) community structures. The final body weight (R2 = 0.36, P = 0.026) and isobutyrate (R2 = 0.48, P = 0.002) were also significantly correlated with the microbial functional community structure (Supplementary Figure 3B). The VPA analysis showed that the phenotypic variables and ileal SCFAs explained 27.21 and 28.73% of the variations in the bacterial communities, and their interaction explained 10.72% of the variation (Supplementary Figure 3C). For the microbial predicted pathway functions, phenotypic variables and ileal SCFAs explained 17.07 and 25.26% of the variations, respectively, and the interaction explained 24.00% of the variation (Supplementary Figure 3D).
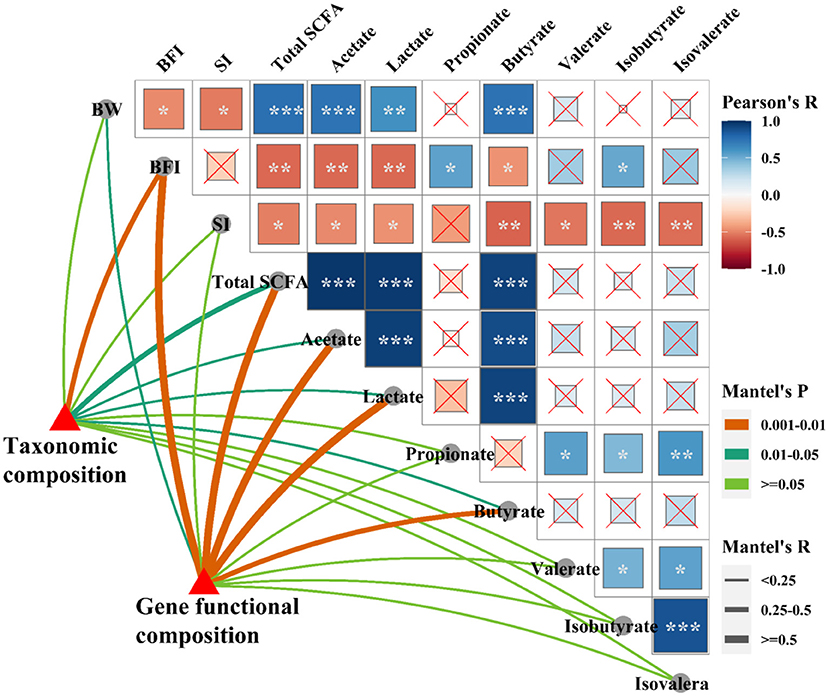
Figure 9. Relationships among phenotypic variables, the microbial community, and metabolic functional composition. Pairwise comparisons of phenotypic variables with a color gradient denoting Pearson correlation coefficient. Taxonomic and functional community structures were related to each phenotypic variable by Mantel correlation (based on Bray-Curtis dissimilarity). The edge width represents the Mantel's r statistic for the corresponding distance correlations, and edge color denotes the statistical significance. *p < 0.05; **p < 0.01; ***p < 0.001.
To further investigate the specific relationships between phenotypic variables and the microbial community or microbial metabolic functional composition, Pearson's correlation analysis was performed (Figure 10). As shown in Figure 10A, we found that the final body weight was positively (p < 0.05, p < 0.01, or p < 0.001) correlated with cecal Firmicutes, Blautia, Dorea, Oscillospira, cc_115, Lactobacillus, Lactobacillus salivarius, and Butyricicoccus pullicaecorum but negatively correlated with Bacteroides and Citrobacter. The bursa of Fabricius index was positively (p < 0.05, p < 0.01, or p < 0.001) correlated with cecal Cyanobacteria, Bacteroidetes, Alistipes, Alistipes massiliensis, and Clostridium methylpentosum, whereas negatively correlated with Firmicutes, Dehalobacterium, [Ruminococcus], Oscillospira, and Ruminococcus. The ileal SCFAs (such as total SCFAs, acetate, lactate, and butyrate) were positively (p < 0.05, p < 0.01, or p < 0.001) correlated with cecal Firmicutes, Blautia, [Ruminococcus], Anaerotruncus, Oscillospira, Ruminococcus, Coprobacillus, cc_115, Lactobacillus, Butyricicoccus, Clostridium citroniae, Lactobacillus salivarius, and Butyricicoccus pullicaecorum, whereas negatively (p < 0.05, p < 0.01, or p < 0.001) correlated with Bacteroidetes, Alistipes, Citrobacter, and Alistipes massiliensis. The ileal propionate and valerate were positively (p < 0.05, p < 0.01, or p < 0.001) correlated with cecal Proteobacteria, Escherichia, Shigella, Escherichia coli, and Shigella sonnei. As shown in Figure 10B, the results showed that the final body weight and ileal SCFAs (including total SCFAs, acetate, lactate, and butyrate) were positively (p < 0.05, p < 0.01, or p < 0.001) correlated with cecal microbial carbohydrate metabolism, amino acid metabolism, peptidoglycan biosynthesis, fatty acid biosynthesis, and tetracycline biosynthesis, whereas negatively (p < 0.05, p < 0.01, or p < 0.001) correlated with glycan biosynthesis and metabolism (such as LPS biosynthesis and LPS biosynthesis proteins) and antibiotic biosynthesis (streptomycin biosynthesis and biosynthesis of vancomycin group antibiotics). The bursa of Fabricius index exerts opposite effect on the correlation with the cecal microbial metabolic functions compared with that of final body weight.
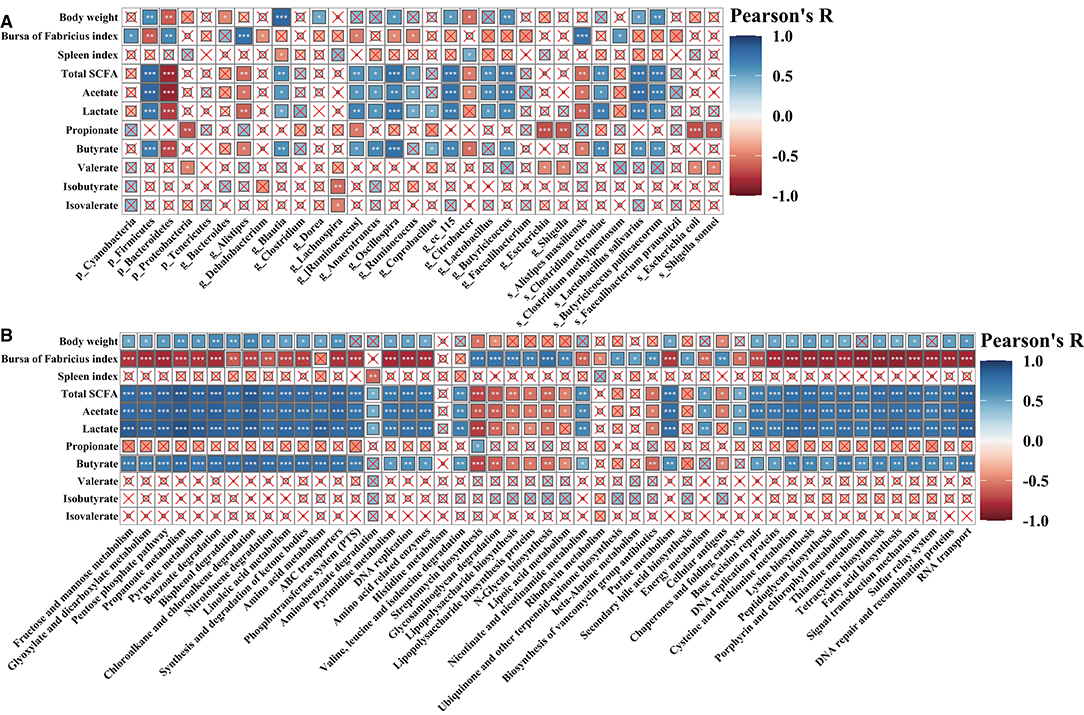
Figure 10. Pearson's correlation analysis. Correlation of phenotypic variables and microbial communities (A) and microbial-predicted metabolic pathway functions (B). The color and the dot size represent the correlation coefficient. *p < 0.05; **p < 0.01; ***p < 0.001.
Microbial Co-occurrence Patterns
Next, we explored the distribution patterns of sensitive OTUs and KOs in the co-occurrence patterns of bacterial communities and microbial metabolic functions in four groups (Figure 11 and Table 4). The co-occurrence network consisted of 1,633 nodes and 7,309 edges (with a mean of 8.95 edges per node) (Figure 11A). In the network, the bacteria shared 1,368 nodes with 2,204 edges (with a mean of 3.22 edges per node), and the microbial metabolic functions shared 265 nodes with 4,027 edges (with a mean of 30.39 edges per node). Among the 1,633 nodes, 387 nodes (235 tsOTUs and 150 tsKOs) were identified as treatment-sensitive nodes, and 17 nodes (three OTUs and 14 KOs) were defined as keystones (Table 4 and Supplementary Tables 2, 3). Consistent with the results showed in Figures 5, 8, compared with the uninfected group, C. perfringens infection significantly (p < 0.05 or p < 0.01) increased the relative abundance of 15 keystones, such as Rikenellaceae and microbial metabolisms (LPS biosynthesis, LPS biosynthesis proteins, streptomycin biosynthesis, etc.) (Figure 4). Compared with the C. perfringens-infected group, dietary with L. plantarum significantly (p < 0.05 or p < 0.01) decreased C. perfringens-induced relative abundance of 17 keystones.
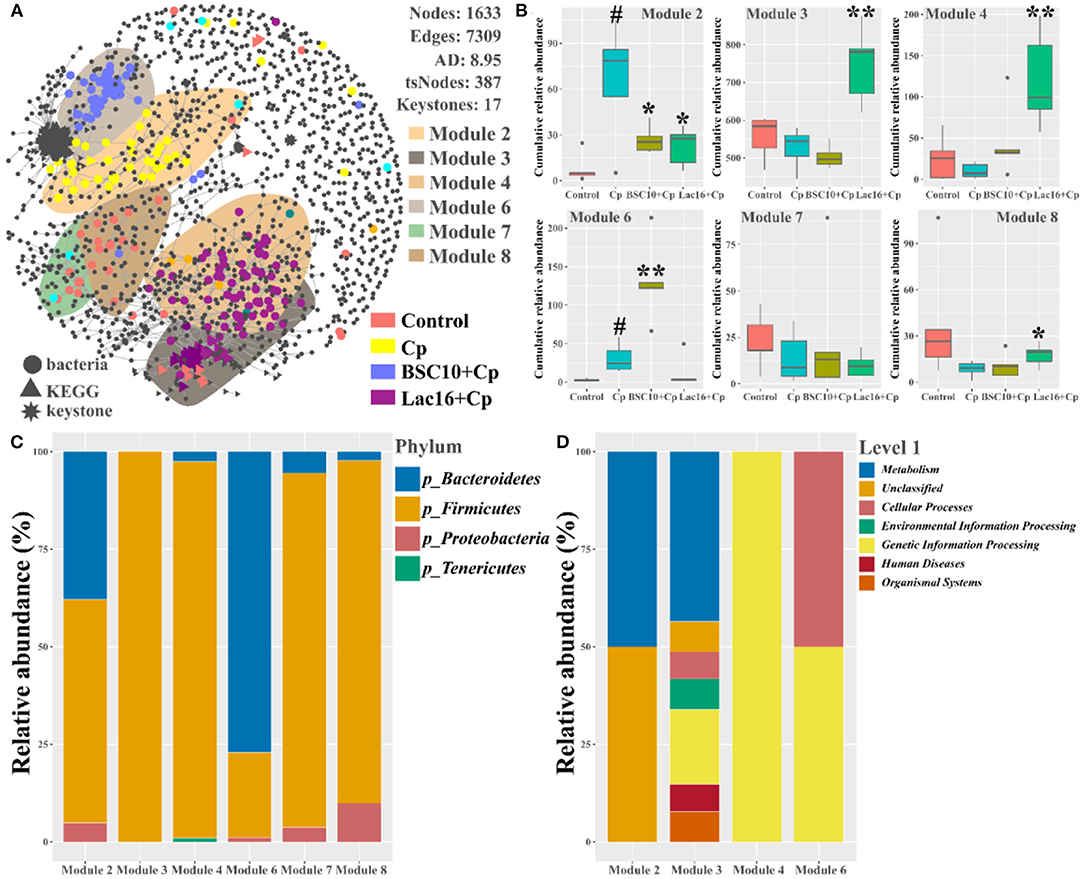
Figure 11. Co-occurrence network of bacterial and metabolic functional communities. (A) Significant and close (|ρ| >0.8, p < 0.05) correlations among bacterial OTUs and metabolic KOs are visualized in the network with gray lines. The bacterial, metabolic functional, and keystone nodes are indicated by circles, triangles, and stars, respectively. Sensitive nodes are colored by their association with the treatments, and gray nodes are insensitive to the treatments. Shaded areas represent the top six modules with high proportions. (B) Cumulative-relative abundance (as counts per million, CPM; y-axis in × 1,000) of the bacterial OTUs metabolic KOs belonging to the responsive modules. (C) The bacterial phyla and metabolic functional compositions for each module. Significant differences versus Control group: #p < 0.05. Significant differences versus Cp group: *p < 0.05; **p < 0.01.
In addition, we noticed that the distribution patterns of microbial and microbial functional associations also responded to treatments. Six modules with relatively high proportions in the network were identified and visualized (Figures 11A,B). We found that the type of sensitivity of these modules to the specific-treated groups (Figures 11A,B), and their distribution in the network partially reflected the community similarity showed in Figures 3B, 6. For example, the effect of C. perfringens infection in the cecal microbial and functional communities was apparent with discrete modules (Modules 2 and 6) in the network (Figure 11B). Module 2 and Module 6 were separated from other modules that primarily contained sensitive OTUs and KOs specific to the control (Modules 7 and 8) and Lac16+Cp (Module 3 and Module 4) groups. The cumulative-relative abundances of the sensitive nodes of Module 2 in the BSC10+Cp and Lac16+Cp groups were significantly (p < 0.05) lower than those in the C. perfringens-infected group. The cumulative-relative abundances of the sensitive nodes of three modules (Modules 3, 4, and 8) in the Lac16+Cp group and Module 6 in the BSC10+Cp group were significantly (p < 0.05 or p < 0.01) higher than those in the C. perfringens-infected group. Furthermore, we found that the six responsive modules comprised a broad set of bacteria and microbial metabolic functions (Figure 11C), indicating that the different treatments may be not target-specific microbial lineages.
Discussion
Necrotic enteritis caused by C. perfringens in poultry exists in two forms: clinical (acute) or subclinical (chronic) infections. Subclinical necrotic enteritis infection accompanies with continuously chronic damage of the gastrointestinal mucosa, which leads to poor growth performance (decreased digestion and absorption, reduced body weight and the feed-conversion ratio) of broilers with or without mortality (9, 35). Subclinical necrotic enteritis without mortality in the present study was successfully induced by C. perfringens infection as observed by decreased body weight and feed conversion, and increased bursal weight index of broilers. The result was consistent with previous studies that subclinical necrotic enteritis infection impaired broiler growth performance with no mortality (36, 37). Our previous study reported that Saccharomyces boulardii attenuates C. perfringens-induced inflammatory response via the TLR4/TLR15-MyD88-signaling pathway in HD11 avian macrophages (23). Bacillus amyloliquefaciens could alleviate necrotic enteritis-induced undesirable effects by modulation of genes related to gut integrity, apoptosis, and immunity, hence further improve performance (38). Although many studies have reported that Lactobacillus or Bacillus could attenuate C. perfringens-induced necrotic enteritis (24–26), but the potential protective mechanism of probiotics against C. perfringens infection remains poorly understood, which needs to be further investigated. This study shows that dietary with L. plantarum or P. polymyxa significantly attenuates C. perfringens infection-induced compromise of growth performance. Previous studies showed that C. perfringens infection compromised the growth performance of broilers by impairing intestinal health and inducing intestinal dysbacteriosis (24, 39). The ameliorated growth performance of C. perfringens-infected broilers in L. plantarum or P. polymyxa-fed groups in this study may be related to the improved intestinal health and intestinal microbiota.
Healthy intestinal morphology and balance of intestinal microbes are important indicators of gastrointestinal tract homeostasis (40, 41). Due to the continuously chronic intestinal mucosa damage with low or no mortality, subclinical necrotic enteritis imposes a huge economic burden on global poultry industry (9). In this study, the increased ileal crypt depth and the histopathological score and the decreased ileal villus height and villus height to the crypt-depth ratio were observed in C. perfringens-infected broilers, which was the main reason to impair the growth performance mentioned above and also indicated that subclinical necrotic enteritis was successfully induced (35). It was found that C. perfringens-induced ileal mucosa damage was alleviated by dietary with P. polymyxa or L. plantarum, as illustrated by increased ileal villus height and villus height to the crypt-depth ratio and decreased ileal crypt depth and the histopathological score. Previous studies also reported that dietary with probiotics had positive effects on reducing subclinical necrotic enteritis occurrence by ameliorating C. perfringens-induced damage of intestinal mucosa (24, 42). The intact intestinal epithelium serves as a physical intestinal mucosal barrier function against invasion of zoonotic enteric pathogens and is responsible for proper nutrient absorption and utilization and waste secretion (43). This physical mucosal barrier is composed of junctional complexes, which provide different types of intercellular connections (44). It is reported that the junctional complexes are binding sites of C. perfringens toxins and enterotoxins (45, 46). In the present study, C. perfringens-infected broilers showed shorter and sparse ileal microvilli, disrupted and shorter tight junction, adherens junction, and desmosomes, consistent with previous studies (47, 48). The intestinal epithelial junction was enhanced in the increased ileal villus of broilers in BSC10+Cp and Lac16+Cp groups, as observed by higher and ordered microvillus, longer tight junction, enhanced adherens junction, and darker desmosomes. The improved intestinal morphology and mucosal barrier function in probiotics treatments may contribute to greater nutrient absorption and utilization from the intestinal digesta (25) and thereby attenuates impairment of growth performance in C. perfringens-infected broilers mentioned above.
SCFAs (mainly including acetate, propionate, and butyrate) are fermentative products metabolized by the gastrointestinal commensal microbiota from dietary carbohydrates (49, 50). As one of the major microbial metabolites, SCFAs play critical roles in maintaining or improving the integrity of intestinal epithelium and tissue repair after mucosal damage (50, 51). C. perfringens infection in this study decreased the levels of ileal total SCFAs, acetate, lactate, and butyrate of broilers, which might impair the energy supply for intestinal enterocytes to repair mucosal damage. Partially consistent with our findings, a previous study reported that subclinical necrotic enteritis infection decreased concentrations of cecal acetate and butyrate of broilers, but increased levels of cecal lactate and propionate partly because of the increased relative abundance of Lactobacillus (52). SCFAs exert not only as an energy source for the host but also as main regulators of the physiological function of intestinal epithelial cells and immune cells (50, 53). SCFAs, especially butyrate and lactate, are also important bacterial metabolites that exert antibacterial and anti-inflammatory activities (53–55). The altered concentrations of the ileal SCFAs (total SCFAs, acetate, lactate, and butyrate) induced by C. perfringens infection were significantly reversed in the Lac16+Cp group, but not significantly reversed in the BSC10+Cp group, indicating that the improved intestinal morphology and mucosal barrier function of C. perfringens-infected broilers in the L. plantarum-treated group may be partly due to the increased intestinal SCFAs levels.
The enteric pathogen infection-induced intestinal dysbiosis could aggravate the gastrointestinal mucosal injury and then compromise the intestinal health and growth performance (56). Many studies reported that dysbiosis is allegedly correlated with C. perfringens infection (14, 57, 58), but it remains unclear about the causal relationship between the intestinal dysbiosis and gastrointestinal infectious diseases (59). In this study, L. plantarum supplementation significantly improved C. perfringens infection-induced intestinal dysbiosis and bacterial metabolic dysfunctions. PCoA results clearly indicated that dietary L. plantarum significantly reversed C. perfringens infection induced shifts of structures of microbial communities (Figure 3B and Table 3) and bacterial metabolic functions (Figure 6), which is consistent with previous studies (60, 61). C. perfringens infection significantly induced a shift of broiler intestinal microbiota structure (61), which could be reversed by dietary Bacillus direct-fed microbial (DFM) in feed (60). The structural shifts may be related with the changes of microbial and microbial metabolic compositions. Dietary L. plantarum improved C. perfringens infection-induced anomalous microbial composition as evidenced by enriched Firmicutes, Ruminococcaceae, SCFAs-producing bacteria (Lachnospiraceae, Ruminococcaceae, Oscillospira, Faecalibacterium, and Blautia) (62–65), which may be partly related to the increase of bacterial fatty acid biosynthesis and intestinal SCFAs levels observed in this study. L. plantarum supplementation significantly reduced Bacteroidetes, drug-resistant bacteria (Bacteroides, Alistipes) (66, 67), and enteric pathogens (Escherichia coli, Bacteroides fragilis) (66), which may partly contribute to the inhibition of bacterial LPS biosynthesis and antibiotic biosynthesis (streptomycin and vancomycin). These findings indicated that L. plantarum-mediated-ameliorated compromise of growth performance in C. perfringens-infected broilers may be related to the restored gut microbial communities and bacterial metabolic functions, which should be further confirmed by whole shotgun metagenomic sequencing because of the limited taxonomical and functional attributes offered by 16S rRNA gene sequencing.
Strong correlations among the final body weight, ileal SCFAs (including total SCFAs, acetate, lactate, and butyrate), microbial communities, and microbial metabolic functions were observed. Consistent with previous reports (68, 69), the final body weight had a strong positive correlation with the ileal SCFAs (including total SCFAs, acetate, lactate, and butyrate), further indicating that the increased intestinal SCFAs levels were beneficial for ameliorating C. perfringens infection-induced compromise of broiler growth performance. Furthermore, the final body weight and ileal SCFAs (including total SCFAs, acetate, lactate, and butyrate) were positively correlated with SCFAs-producing bacteria (Blautia, Oscillospira, Lactobacillus, Lactobacillus salivarius, and Butyricicoccus pullicaecorum) and bacterial fatty acid biosynthesis, whereas negatively correlated with drug-resistant bacteria (Bacteroides and Citrobacter), which was partly consistent with previous findings (70–72). These results indicated that the body weight and intestinal SCFAs might be principal factors affecting the bacterial communities and microbial metabolic functions.
Co-occurrence patterns of gut microbiota were further performed to investigate the treatment-sensitive species, keystones, and the microbial interactions. The results found that 235 bacteria and 150 microbial metabolic functions were sensitive to the four treatments, and most of the tsNodes significantly grouped in six distinct modules that reflected the different treatments. The uninfected treatment-sensitive nodes grouped in Modules 7 and 8; C. perfringens-infected-treatment-sensitive nodes grouped in Modules 2 and 6; P. polymyxa pretreatment-sensitive nodes grouped in Module 6; L. plantarum pretreatment-sensitive nodes grouped in Modules 3 and 4, indicating that dietary L. plantarum significantly modulated the co-occurrence interactions of intestinal microbes. By frequently interacting with many other microbes, keystone species are thought to play crucial roles in modulating microbial communities and functions (34, 73). The present study found that three bacteria (Rikenellaceae, Bacteroides, Bacteroides_unidentified) and 14 bacterial metabolic functions (LPS biosynthesis, LPS biosynthesis proteins, streptomycin biosynthesis etc.) were defined as keystones, in which dietary L. plantarum significantly decreased C. perfringens infection induced all the 17 keystones. Bacteroides species are multiple drug-resistant and significant clinical anaerobic pathogenic bacteria that can cause life-threatening infection with mortality of more than 19% (66). As the major component of the outer membrane of gram-negative microbes, LPS acts as a key pathogenic stimulator for the dysfunctions and plays major role in pathogens-mediated toxicity and pathogenicity (74). These results indicate that the shifts of these keystones maybe the driving factors involved in L. plantarum-mediated-ameliorated growth performance and improved mucosal damage of the C. perfringens-infected broilers.
Conclusion
The current results indicate that dietary L. plantarum-mediated amelioration of growth performance and improvement of intestinal mucosal damage of broilers under subclinical NE condition are associated with the increased intestinal SCFAs levels, enhanced intestinal epithelial barriers, and improved intestinal dysbiosis. Dietary P. polymyxa-mediated amelioration of broiler growth performance under subclinical NE condition may partly be due to the improved mucosal structure and intestinal epithelial barriers. These findings provide a potential preventive approach against avian necrotic enteritis caused by C. perfringens infection. However, the detailed preventive mechanism of L. plantarum against subclinical necrotic enteritis should be further investigated.
Data Availability Statement
Raw sequences have been deposited in the Genome Sequence Archive (GSA) of the BIG Data Center (https://bigd.big.ac.cn/gsa/) under accession number PRJCA004271/CRA003760.
Ethics Statement
All the procedures of this project were conducted according to the Chinese guidelines for animal welfare and were approved by the Zhejiang University Institutional Animal Care and Use Committee (Permission number: ZJU20160416).
Author Contributions
WL and HZ conceptualization and supervision. BW, YM, and YZ data curation, microbial analysis, writing—original draft, review, and editing. BW, QG, and YZ conducted the animal experiments. LG, XL, SX, and FW assisted with the experiments. XL and SX assisted in the manuscript preparation. All authors contributed to the article and approved the submitted version.
Funding
This study was supported by the National Natural Science Foundation of China (Grant Nos. 31472128 and 31672460), the Natural Science Foundation of Zhejiang province (Grants Nos. LZ20C170002 and 2006C12086), and National High-Tech R&D Program (863) of China (Grant No. 2013AA102803D).
Conflict of Interest
The authors declare that the research was conducted in the absence of any commercial or financial relationships that could be construed as a potential conflict of interest.
Publisher's Note
All claims expressed in this article are solely those of the authors and do not necessarily represent those of their affiliated organizations, or those of the publisher, the editors and the reviewers. Any product that may be evaluated in this article, or claim that may be made by its manufacturer, is not guaranteed or endorsed by the publisher.
Supplementary Material
The Supplementary Material for this article can be found online at: https://www.frontiersin.org/articles/10.3389/fnut.2021.706148/full#supplementary-material
Figure S1. Probiotics exerts anti-C. perfringens activity. (A) Fermented supernatant of P. polymyxa (BSC10) and L. plantarum (Lac16) significantly inhibited the expression of virulence genes (α and β toxins) of C. perfringens. Significant differences versus control group: *p < 0.05. (B) BSC10 and Lac16 cultures significantly inhibited C. perfringens growth. (C) Live Lac16 significantly protect C. elegans against C. perfringens infection.
Figure S2. Comparison of predicted pathway abundances between the groups by statistical analysis of taxonomic and functional profiles (STAMP). (A) Control versus Cp; (A) Cp versus BSC10+Cp; (B) Cp versus Lac16+Cp.
Figure S3. Canonical correspondence analysis and variation partitioning analysis of the phenotypic variables (including body weight, bursa of fabricius index and spleen index) and ileal SCFAs for the bacterial community (A,C) and microbial predicted pathway functions (B,D).
Figure S4. Relative abundances of the keystone species. Significant differences versus Control group: #p < 0.05; ##p < 0.01. Significant differences versus Cp group: *p < 0.05; **p < 0.01. The prefixes “b_” and “k_” represent the bacteria and KEGG. n = 5 samples.
References
1. Prescott JF, Parreira VR, Gohari IM, Lepp D, Gong J. The pathogenesis of necrotic enteritis in chickens: what we know and what we need to know: a review. Avian Pathol. (2016) 45:288–94. doi: 10.1080/03079457.2016.1139688
2. Grass JE, Gould LH, Mahon BE. Epidemiology of foodborne disease outbreaks caused by clostridium perfringens, United States, 1998-2010. Foodborne Pathog Dis. (2013) 10:131–6. doi: 10.1089/fpd.2012.1316
3. Wade B, Keyburn A. The true cost of necrotic enteritis. World Poult. (2015) 31:16–7. Available online at: https://www.poultryworld.net/Meat/Articles/2015/10/The-true-cost-of-necrotic-enteritis-2699819W/
4. Lee KW, Lillehoj HS, Jeong W, Jeoung HY, An DJ. Avian necrotic enteritis: Experimental models, host immunity, pathogenesis, risk factors, vaccine development. Poultry Sci. (2011) 90:1381–90. doi: 10.3382/ps.2010-01319
5. Caly DL, D'Inca R, Auclair E, Drider D. Alternatives to antibiotics to prevent necrotic enteritis in broiler chickens: a microbiologist's perspective. Front Microbiol. (2015) 6:1336. doi: 10.3389/fmicb.2015.01336
6. Castanon JIR. History of the use of antibiotic as growth promoters in European poultry feeds. Poultry Sci. (2007) 86:2466–71. doi: 10.3382/ps.2007-00249
7. China. Announcement of the Ministry of Agriculture and Rural People's Republic of China. (2019). Available online at: http://www.moa.gov.cn/nybgb/2017/dqq/201801/t20180103_6133925.htm (accessed July 20, 2017).
8. Hofacre CL, Smith JA, Mathis GF. An optimist's view on limiting necrotic enteritis and maintaining broiler gut health and performance in today's marketing, food safety, regulatory climate. Poult Sci. (2018) 97:1929–33. doi: 10.3382/ps/pey082
9. M'Sadeq SA, Wu S, Swick RA, Choct M. Towards the control of necrotic enteritis in broiler chickens with in-feed antibiotics phasing-out worldwide. Anim Nutr. (2015) 1:1–11. doi: 10.1016/j.aninu.2015.02.004
10. Caballero S, Pamer EG. Microbiota-mediated inflammation and antimicrobial defense in the intestine. Annu Rev Immunol. (2015) 33:227–56. doi: 10.1146/annurev-immunol-032713-120238
11. Hooper LV, Littman DR, Macpherson AJ. Interactions between the microbiota and the immune system. Science. (2012) 336:1268–73. doi: 10.1126/science.1223490
12. Kurashima Y, Kiyono H. Mucosal ecological network of epithelium and immune cells for gut homeostasis and tissue healing. Ann Rev Immunol. (2017) 35:119–47. doi: 10.1146/annurev-immunol-051116-052424
13. Heida FH, van Zoonen AGJF, Hulscher JBF, te Kiefte BJC, Wessels R, Kooi EMW, et al. A necrotizing enterocolitis-associated gut microbiota is present in the meconium: results of a prospective study. Clin Infect Dis. (2016) 62:863–70. doi: 10.1093/cid/ciw016
14. Bortoluzzi C, Vieira BS, Hofacre C, Applegate TJ. Effect of different challenge models to induce necrotic enteritis on the growth performance and intestinal microbiota of broiler chickens. Poultry Sci. (2019) 98:2800–12. doi: 10.3382/ps/pez084
15. Yeoman CJ, White BA. Gastrointestinal tract microbiota and probiotics in production animals. Ann Rev Animal Biosci. (2014) 2:469–86. doi: 10.1146/annurev-animal-022513-114149
16. Buntyn JO, Schmidt TB, Nisbet DJ, Callaway TR. The role of direct-fed microbials in conventional livestock production. Annu Rev Anim Biosci. (2016) 4:335–55. doi: 10.1146/annurev-animal-022114-111123
17. Sanchez B, Delgado S, Blanco-Miguez A, Lourenco A, Gueimonde M, Margolles A. Probiotics, gut microbiota, and their influence on host health and disease. Mol Nutr Food Res. (2017) 61:1600240. doi: 10.1002/mnfr.201600240
18. Todorov SD, Franco BDGD. Lactobacillus plantarum: characterization of the species and application in food production. Food Rev Int. (2010) 26:205–29. doi: 10.1080/87559129.2010.484113
19. Kareem RA, Razavi SH. Plantaricin bacteriocins: As safe alternative antimicrobial peptides in food preservation-A review. J Food Safety. (2020) 40:12735. doi: 10.1111/jfs.12735
20. Sabo SD, Vitolo M, Gonzalez JMD, Oliveira RPD. Overview of Lactobacillus plantarum as a promising bacteriocin producer among lactic acid bacteria. Food Res Int. (2014) 64:527–36. doi: 10.1016/j.foodres.2014.07.041
21. He ZG, Kisla D, Zhang LW, Yuan CH, Green-Church KB, Yousef AE. Isolation and identification of a Paenibacillus polymyxa strain that coproduces a novel lantibiotic and polymyxin. Appl Environ Microb. (2007) 73:168–78. doi: 10.1128/Aem.02023-06
22. Ma MC, Wang CC, Ding YQ, Li L, Shen DL, Jiang X, et al. Complete genome sequence of paenibacillus polymyxa SC2, a strain of plant growth-promoting rhizobacterium with broad-spectrum antimicrobial activity. J Bacteriol. (2011) 193:311–2. doi: 10.1128/Jb.01234-10
23. Wang B, Hussain A, Zhou Y, Zeng Z, Wang Q, Zou P, et al. Saccharomyces boulardii attenuates inflammatory response induced by Clostridium perfringens via TLR4/TLR15-MyD8 pathway in HD11 avian macrophages. Poult Sci. (2020) 99:5356–65. doi: 10.1016/j.psj.2020.07.045
24. Li Z, Wang W, Liu D, Guo Y. Effects of Lactobacillus acidophilus on the growth performance and intestinal health of broilers challenged with Clostridium perfringens. J Anim Sci Biotechnol. (2018) 9:25. doi: 10.1186/s40104-018-0243-3
25. Ramlucken U, Ramchuran SO, Moonsamy G, Lalloo R, Thantsha MS, van Rensburg CJ. A novel Bacillus based multi-strain probiotic improves growth performance and intestinal properties of Clostridium perfringens challenged broilers. Poultry Sci. (2020) 99:331–41. doi: 10.3382/ps/pez496
26. Guo SS, Xi Y, Xia Y, Wu T, Zhao D, Zhang ZF, et al. Dietary Lactobacillus fermentum and Bacillus coagulans supplementation modulates intestinal immunity and microbiota of broiler chickens challenged by Clostridium perfringens. Front Vet Sci. (2021) 8:e680742. doi: 10.3389/fvets.2021.680742
27. Wang B, Zhou Y, Tang L, Zeng Z, Gong L, Wu Y, et al. Effects of Bacillus amyloliquefaciens instead of antibiotics on growth performance, intestinal health and intestinal microbiota of broilers. Front Vet Sci. (2021) 8:679368. doi: 10.3389/fvets.2021.679368
28. Council NN. Nutrient Requirements of Poultry (9th rev. ed.). Washington, DC: National Academies Press (1994).
29. Jerzsele A, Szeker K, Csizinszky R, Gere E, Jakab C, Mallo JJ, et al. Efficacy of protected sodium butyrate, a protected blend of essential oils, their combination, and Bacillus amyloliquefaciens spore suspension against artificially induced necrotic enteritis in broilers. Poultry Sci. (2012) 91:837–43. doi: 10.3382/ps.2011-01853
30. Caporaso JG, Kuczynski J, Stombaugh J, Bittinger K, Bushman FD, Costello EK, et al. QIIME allows analysis of high-throughput community sequencing data. Nat Methods. (2010) 7:335–6. doi: 10.1038/nmeth.f.303
31. Langille MGI, Zaneveld J, Caporaso JG, McDonald D, Knights D, Reyes JA, et al. Predictive functional profiling of microbial communities using 16S rRNA marker gene sequences. Nat Biotechnol. (2013) 31:814–21. doi: 10.1038/nbt.2676
32. Parks DH, Tyson GW, Hugenholtz P, Beiko RG. STAMP: statistical analysis of taxonomic and functional profiles. Bioinformatics. (2014) 30:3123–4. doi: 10.1093/bioinformatics/btu494
33. Guo X, Gao Q, Yuan MT, Wang GS, Zhou XS, Feng JJ, et al. Gene-informed decomposition model predicts lower soil carbon loss due to persistent microbial adaptation to warming. Nat Commun. (2020) 11:1. doi: 10.1038/s41467-020-18706-z
34. Hartman K, van der Heijden MGA, Wittwer RA, Banerjee S, Walser JC, Schlaeppi K. Cropping practices manipulate abundance patterns of root and soil microbiome members paving the way to smart farming. Microbiome. (2018) 6:1–14. doi: 10.1186/s40168-017-0389-9
35. Van Immerseel F, Rood JI, Moore RJ, Titball RW. Rethinking our understanding of the pathogenesis of necrotic enteritis in chickens. Trends Microbiol. (2009) 17:32–6. doi: 10.1016/j.tim.2008.09.005
36. Bansal M, Fu Y, Alrubaye B, Abraha M, Almansour A, Gupta A, et al. A secondary bile acid from microbiota metabolism attenuates ileitis and bile acid reduction in subclinical necrotic enteritis in chickens. J Anim Sci Biotechno. (2020) 11:1–10. doi: 10.1186/s40104-020-00441-6
37. Xue GD, Wu SB, Choct M, Swick RA. The role of supplemental glycine in establishing a subclinical necrotic enteritis challenge model in broiler chickens. Anim Nutr. (2017) 3:266–70. doi: 10.1016/j.aninu.2017.05.004
38. Gharib-Naseri K, de Paula Dorigam JC, Doranalli K, Kheravii S, Swick RA, Choct M, et al. Modulations of genes related to gut integrity, apoptosis, and immunity underlie the beneficial effects of Bacillus amyloliquefaciens CECT 5940 in broilers fed diets with different protein levels in a necrotic enteritis challenge model. J Anim Sci Biotechnol. (2020) 11:104. doi: 10.1186/s40104-020-00508-4
39. Kheravii SK, Swick RA, Choct M, Wu SB. Effect of oat hulls as a free choice feeding on broiler performance, short chain fatty acids and microflora under a mild necrotic enteritis challenge. Anim Nutr. (2018) 4:65–72. doi: 10.1016/j.aninu.2017.11.003
40. Ohland CL, Jobin C. Microbial activities and intestinal homeostasis: a delicate balance between health and disease. Cell Mol Gastroenter. (2015) 1:28–40. doi: 10.1016/j.jcmgh.2014.11.004
41. Pham VH, Kan LG, Huang JY, Geng YQ, Zhen WR, Guo YM, et al. Dietary encapsulated essential oils and organic acids mixture improves gut health in broiler chickens challenged with necrotic enteritis. J Anim Sci Biotechno. (2020) 11:1–18. doi: 10.1186/s40104-019-0421-y
42. Wu YY, Shao YJ, Song BC, Zhen WR, Wang Z, Guo YM, et al. Effects of Bacillus coagulans supplementation on the growth performance and gut health of broiler chickens with Clostridium perfringens-induced necrotic enteritis. J Anim Sci Biotechno. (2018) 9:1–14. doi: 10.1186/s40104-017-0220-2
43. Turner JR. Intestinal mucosal barrier function in health and disease. Nat Rev Immunol. (2009) 9:799–809. doi: 10.1038/nri2653
44. Shen L, Weber CR, Raleigh DR, Yu D, Tumer JR. Tight, junction pore and leak pathways: a dynamic duo. Annu Rev Physiol. (2011) 73:283–309. doi: 10.1146/annurev-physiol-012110-142150
45. Eichner M, Protze J, Piontek A, Krause G, Piontek J. Targeting and alteration of tight junctions by bacteria and their virulence factors such as Clostridium perfringens enterotoxin. Pflug Arch Eur J Phy. (2017) 469:77–90. doi: 10.1007/s00424-016-1902-x
46. Petit L, Gibert M, Popoff MR. Clostridium perfringens: toxinotype and genotype. Trends Microbiol. (1999) 7:104–10. doi: 10.1016/S0966-842x(98)01430-9
47. Cao L, Wu XH, Bai YL, Wu XY, Gu SB. Anti-inflammatory and antioxidant activities of probiotic powder containing Lactobacillus plantarum 1.2567 in necrotic enteritis model of broiler chickens. Livest Sci. (2019) 223:157–63. doi: 10.1016/j.livsci.2019.03.009
48. Olkowski AA, Wojnarowicz C, Chirino-Trejo M, Laarveld B, Sawicki G. Sub-clinical necrotic enteritis in broiler chickens: Novel etiological consideration based on ultra-structural and molecular changes in the intestinal tissue. Res Vet Sci. (2008) 85:543–53. doi: 10.1016/j.rvsc.2008.02.007
49. Dalile B, Van Oudenhove L, Vervliet B, Verbeke K. The role of short-chain fatty acids in microbiota-gut-brain communication. Nat Rev Gastro Hepat. (2019) 16:461–78. doi: 10.1038/s41575-019-0157-3
50. Kayama H, Okumura R, Takeda K. Interaction between the microbiota, epithelia, and immune cells in the intestine. Ann Rev Immunol. (2020) 38:23–48. doi: 10.1146/annurev-immunol-070119-115104
51. Goncalves P, Araujo JR, Di Santo JP. A cross-talk between microbiota-derived short-chain fatty acids and the host mucosal immune system regulates intestinal homeostasis and inflammatory bowel disease. Inflamm Bowel Dis. (2018) 24:558–72. doi: 10.1093/ibd/izx029
52. Gharib-Naseri K, Kheravii SK, Keerqin C, Morgan N, Swick RA, Choct M, et al. Two different Clostridium perfringens strains produce different levels of necrotic enteritis in broiler chickens. Poultry Sci. (2019) 98:6422–32. doi: 10.3382/ps/pez480
53. McCarville JL, Chen GY, Cuevas VD, Troha K, Ayres JS. Microbiota metabolites in health and disease. Ann Rev Immunol. (2020) 38:147–70. doi: 10.1146/annurev-immunol-071219-125715
54. Koh A, De Vadder F, Kovatcheva-Datchary P, Backhed F. From dietary fiber to host physiology: short-chain fatty acids as key bacterial metabolites. Cell. (2016) 165:1332–45. doi: 10.1016/j.cell.2016.05.041
55. Lamas A, Regal P, Vazquez B, Cepeda A, Franco CM. Short chain fatty acids commonly produced by gut microbiota influence salmonella enterica motility, biofilm formation, gene expression. Antibiotics-Basel. (2019) 8:265. doi: 10.3390/antibiotics8040265
56. Wang C, Li Q, Ren J. Microbiota-immune interaction in the pathogenesis of gut-derived infection. Front Immunol. (2019) 10:1873. doi: 10.3389/fimmu.2019.01873
57. Minamoto Y, Dhanani N, Markel ME, Steiner JM, Suchodolski JS. Prevalence of Clostridium perfringens, Clostridium perfringens enterotoxin and dysbiosis in fecal samples of dogs with diarrhea. Vet Microbiol. (2014) 174:463–73. doi: 10.1016/j.vetmic.2014.10.005
58. Lacey JA, Stanley D, Keyburn AL, Ford M, Chen H, Johanesen P, et al. Clostridium perfringens-mediated necrotic enteritis is not influenced by the pre-existing microbiota but is promoted by large changes in the post-challenge microbiota. Vet Microbiol. (2018) 227:119–26. doi: 10.1016/j.vetmic.2018.10.022
59. Bien J, Palagani V, Bozko P. The intestinal microbiota dysbiosis and Clostridium difficile infection: is there a relationship with inflammatory bowel disease? Ther Adv Gastroenter. (2013) 6:53–68. doi: 10.1177/1756283x12454590
60. Hernandez-Patlan D, Solis-Cruz B, Pontin KP, Hernandez-Velasco X, Merino-Guzman R, Adhikari B, et al. Impact of a bacillus direct-fed microbial on growth performance, intestinal barrier integrity, necrotic enteritis lesions, and ileal microbiota in broiler chickens using a laboratory challenge model. Front Vet Sci. (2019) 6:108–19. doi: 10.3389/fvets.2019.00108
61. Latorre JD, Adhikari B, Park SH, Teague KD, Graham LE, Mahaffey BD, et al. Evaluation of the epithelial barrier function and ileal microbiome in an established necrotic enteritis challenge model in broiler chickens. Front Vet Sci. (2018) 5:199. doi: 10.3389/fvets.2018.00199
62. Bai S, Wang W, Wang T, Li J, Zhang S, Chen Z, et al. CD36 deficiency affects depressive-like behaviors possibly by modifying gut microbiota and the inflammasome pathway in mice. Transl Psychiatry. (2021) 11:16. doi: 10.1038/s41398-020-01130-8
63. Konikoff T, Gophna U. Oscillospira: a central, enigmatic component of the human gut microbiota. Trends Microbiol. (2016) 24:523–4. doi: 10.1016/j.tim.2016.02.015
64. Ozato N, Saito S, Yamaguchi T, Katashima M, Tokuda I, Sawada K, et al. Blautia genus associated with visceral fat accumulation in adults 20-76 years of age. Npj Biofilms Microbi. (2019) 5:1–9. doi: 10.1038/s41522-019-0101-x
65. Reichardt N, Duncan SH, Young P, Belenguer A, Leitch CM, Scott KP, et al. Phylogenetic distribution of three pathways for propionate production within the human gut microbiota. Isme J. (2014) 8:1323–35. doi: 10.1038/ismej.2014.14
66. Wexler HM. Bacteroides: the good, the bad, the nitty-gritty. Clin Microbiol Rev. (2007) 20:593–621. doi: 10.1128/Cmr.00008-07
67. Parker BJ, Wearsch PA, Veloo ACM, Rodriguez-Palacios A. The genus alistipes: gut bacteria with emerging implications to inflammation, cancer, mental health. Front Immunol. (2020) 11:906. doi: 10.3389/fimmu.2020.00906
68. Kumar A, Toghyani M, Kheravii SK, Pineda L, Han Y, Swick RA, et al. Potential of blended organic acids to improve performance and health of broilers infected with necrotic enteritis. Anim Nutr. (2021) 7:440–9. doi: 10.1016/j.aninu.2020.11.006
69. Yu M, Li ZM, Chen WD, Wang G, Cui YY, Ma XY. Dietary supplementation with citrus extract altered the intestinal microbiota and microbial metabolite profiles and enhanced the mucosal immune homeostasis in yellow-feathered broilers. Front Microbiol. (2019) 10:2662. doi: 10.3389/fmicb.2019.02662
70. Guo F, Wang F, Ma H, Ren Z, Yang X, Yang X. Study on the interactive effect of deoxynivalenol and Clostridium perfringens on the jejunal health of broiler chickens. Poultry Sci. (2020) 100:1–13. doi: 10.1016/j.psj.2020.10.061
71. Liao XD, Shao YX, Sun GM, Yang YF, Zhang LY, Guo YL, et al. The relationship among gut microbiota, short-chain fatty acids, and intestinal morphology of growing and healthy broilers. Poultry Sci. (2020) 99:5883–95. doi: 10.1016/j.psj.2020.08.033
72. Liu J, Stewart SN, Robinson K, Yang Q, Lyu W, Whitmore MA, et al. Linkage between the intestinal microbiota and residual feed intake in broiler chickens. J Anim Sci Biotechno. (2021) 12:22. doi: 10.1186/s40104-020-00542-2
73. Zhang XP, Bian FY, Zhong ZK, Gai X, Yang CB. Deciphering the rhizosphere microbiome of a bamboo plant in response to different chromium contamination levels. J Hazard Mater. (2020) 399:123107. doi: 10.1016/j.jhazmat.2020.123107
Keywords: Lactobacillus plantarum, Paenibacillus polymyxa, Clostridium perfringens, necrotic enteritis, growth performance, short-chain fatty acids, intestinal health, intestinal dysbiosis
Citation: Wang B, Zhou Y, Mao Y, Gong L, Li X, Xu S, Wang F, Guo Q, Zhang H and Li W (2021) Dietary Supplementation With Lactobacillus plantarum Ameliorates Compromise of Growth Performance by Modulating Short-Chain Fatty Acids and Intestinal Dysbiosis in Broilers Under Clostridium perfringens Challenge. Front. Nutr. 8:706148. doi: 10.3389/fnut.2021.706148
Received: 06 May 2021; Accepted: 17 September 2021;
Published: 14 October 2021.
Edited by:
Hui Han, Chinese Academy of Sciences (CAS), ChinaReviewed by:
Yuan Gao, Zhejiang Academy of Agricultural Sciences, ChinaSéverine Zirah, Muséum National d'Histoire Naturelle, France
Xianyong Ma, Guangdong Academy of Agricultural Sciences (GDAAS), China
Copyright © 2021 Wang, Zhou, Mao, Gong, Li, Xu, Wang, Guo, Zhang and Li. This is an open-access article distributed under the terms of the Creative Commons Attribution License (CC BY). The use, distribution or reproduction in other forums is permitted, provided the original author(s) and the copyright owner(s) are credited and that the original publication in this journal is cited, in accordance with accepted academic practice. No use, distribution or reproduction is permitted which does not comply with these terms.
*Correspondence: Weifen Li, wfli@zju.edu.cn; Huihua Zhang, hhzhang2@163.com
†ORCID: Baikui Wang orcid.org/0000-0001-5283-7337
Weifen Li orcid.org/0000-0001-8159-9876