- 1International Centre of Insect Physiology and Ecology (icipe), Nairobi, Kenya
- 2Department of Food Science and Technology, Jomo Kenyatta University of Agriculture and Technology, Nairobi, Kenya
- 3Department of Environmental Health, University of Kabianga, Kericho, Kenya
- 4Department of Animal Science, Jomo Kenyatta University of Agriculture and Technology, Nairobi, Kenya
- 5Department of Food Technology and Nutrition, School of Food Technology, Nutrition and Bioengineering, Makerere University, Kampala, Uganda
Globally, there is growing interest to integrate cricket-based ingredients (flour) into food products to combat food and nutrition insecurity. However, there is lack of information on in-depth nutrient profile of the two cricket species (Scapsipedus icipe and Gryllus bimaculatus), which are the most widely consumed in Africa. Here we determined the nutrient composition of two cricket species and compared them with published records of key animal and plant sources. Our results revealed that the crude protein contents of S. icipe and G. bimaculatus were similar (56.8 and 56.9%, respectively) and comparable to those of animal protein sources. Both cricket species had balanced amino acid profiles that are superior to that of animal and plant sources, except for histidine and cysteine. The protein digestibility of S. icipe and G. bimaculatus ranged between 80 and 88%, which is comparable to that of common plant foods but slightly lower than that of animal proteins. The iron, Zinc, and potassium contents were considerably higher in both cricket species compared to that of plant and animal sources. The calcium contents of both crickets (S. icipe and G. bimaculatus) was superior to that of plant and animal origin except for kidney beans and eggs, respectively. Riboflavin, thiamine, and folic acid concentrations of S. icipe and G. bimaculatus were superior to that of the conventional sources. Vitamin A levels were significantly higher in S. icipe compared to G. bimaculatus. This implies that S. icipe and G. bimaculatus can adequately contribute to our daily required nutrient intake. Thus, integrating cricket flours into ready-to-eat food products would address some of the most pressing nutritional deficiency challenges that many developing countries have to grapple with, particularly high risk to serious health problems such as anemia, poor pregnancy outcomes, hypertension, increased risk of morbidity and mortality, stunted growth and impaired physical and cognitive development. We conclude that edible crickets present unique opportunities for improving food and nutritional insecurity status of both resource-poor and Western populations.
Introduction
The world's population is expected to reach 9.2 billion in 2050, with most increases occurring in less developed regions (1, 2). Therefore, the worldwide demand for food and meat is likely to increase by 50 and 85%, respectively (3). The vast majority of the hungry people−827 million—live in developing regions and Africa remains the region with the highest prevalence of undernourishment with sub-Saharan Africa (SSA) accounting for a prevalence of 24.8% (4). Among the undernourished populations, over 2 billion people are affected by micronutrient deficiencies globally (5). In terms of global public health significance, iron, zinc, and vitamins are the most important micronutrients (6, 7), which play key roles in preventing malnutrition and early stunting (7). The deficiency of these nutrients is prevalent in areas where there is high cereal and low animal products consumption (8), especially resource-poor areas where the situation is exacerbated by infectious diseases (5). Besides the traditional nutrient deficiency diseases, there is a rising prevalence of non-communicable diseases in both developing and developed countries. Thus, deficiencies of these important nutrients have negative effects on many physiological systems and health, leading to high economic burden for many countries with increasing risk of morbidity and impaired physical and cognitive development (9) as well as poor pregnancy outcome (10, 11).
In SSA, the situation is projected to worsen over the next decades unless drastic measures are taken to reverse food insecurity (5). Efforts to tackle this problem have largely focused on enhancing crop and livestock productivity, which are becoming unsustainable due to dwindling arable land, and water scarcity caused by climate change. Livestock and crop production has been blamed for its significant contribution to negative environmental impact, particularly responsible for huge greenhouse gas emissions (high ecological blueprints), which could possibly contribute to global warming and severe environmental degradation (7).
Thus, there is an urgent need for alternative nutrient sources, and edible insects are promising and potential choice (12). Globally, over 2,000 insect species are consumed by approximately 2 billion people as delicacy with well-documented evidence that they can significantly provide the amount of daily nutrient requirements for human nutrition, especially for children and women of reproductive age (8), thus a potential solution to malnutrition and growing food insecurity globally (13, 14). This explains why Food and Agriculture Organization of the United Nations (FAO) and other stakeholders have embarked on the promotion of edible insects for food (15, 16). Globally, insects from the order Orthoptera such as crickets are one of the most widely farmed and nutrient-rich edible insect group, generating income for women and youths, who represents more than 60% of all the medium- or large-scale enterprises (12). In many cases the nutrient profile of these cricket species is comparable to conventional livestock meat and crops in terms of quality and quantity (17). It has been postulated that crickets generally contain high-quality nutrients, which are easily digestible and more bio-available than those available from plant and animal food sources (18).
Thus, cricket consumption could be immediate solutions to many of the nutrient deficiency issues and can be both inexpensive and effective option. According to several researchers, multisectoral approaches that are tailored to the sustainable utilization of local materials such as insects and that consider the specific conditions of the people would be easily favored by policy makers in view of their sustainability. This explains why the FAO and WHO have recommended dietary diversification, food fortification and supplementation to simultaneously control infections and prevention of other nutritional deficiencies (19). So far, little attention has been paid to crickets as source of essential nutrients in human diets. This study seeks to provide a comprehensive dietary benefits of the newly described cricket Scapsipedus icipe Hugel & Tanga (20) and the two-spotted cricket Gryllus bimaculatus De Geer (21) in Africa, which are most widely consumed in terms of their richness in minerals, protein, fat, amino acids, and vitamins. Next, we discussed bioavailability of amino acids, vitamins, and minerals in relation to Recommended Dietary Allowance (RDA) published for animal and plant-based sources and outline future directions for research.
Materials and Methods
Sample Collection and Preparation
Adult crickets were obtained from subsistence and commercial farms in Western part of Kenya. Samples of each species were collected separately in 1 kg Ziplock bags, frozen alive at −20°C in a Hisense chest freezer (model number: 1159Q61, China) and transported frozen in cooler boxes lined with ice packs to the International Centre of Insect Physiology and Ecology (icipe) laboratories for analysis. The raw cricket samples were thawed and blended into a paste using a domestic blender (Signature SG-201, China). The insect paste was subjected to various chemical analysis as described below.
Proximate Analysis
Proximate components were determined using Association of Official Analytical Chemists (22) methods. The moisture content was determined by oven drying method at 135°C for 2 h (Method No. 930.15) (22). Ash content was determined by ignition of samples at 550°C in a muffle furnace until the weight remained constant (Method No. 930.05) (22). The crude fat content was determined by diethyl ether extraction in a fat extraction unit (SER 148/6; Velp Scientific, Usmate, Italy) following the Randall technique (Method No. 2003.05) (22). The crude protein content of the cricket powder was determined following the Kjeldahl method and the values multiplied by a conversion factor of 6.25 (Method No. 2001.11) (22). Crude fiber was determined by loss of ignition on weight of residue after hydrolysis with acid and alkali solutions (Method number 978.10) (23).
Determination of Amino Acid Composition
The amino acid composition was determined as previously described by Cheseto et al. (24). The cricket powder (100 mg) was transferred into a 5 mL micro-reaction vial into which 2 mL of 6N HCl was added and closed after careful introduction of nitrogen gas. The sample was hydrolyzed for 24 h at 110°C. After the hydrolysis, the mixtures were evaporated to dryness under vacuum. The hydrolysates were reconstituted in 1 mL 0.01% formic acid/acetonitrile (95: 5), vortexed for 30 s, sonicated for 30 min, and then centrifuged at 14,000 rpm and the supernatant analyzed by LC-MS. The same procedure was performed to determine basic amino acid by substituting 6N HCl with 6N NaOH.
The chromatographic separation was achieved on an Agilent system 1100 series (MA, USA) using ZORBAX SB-C18, 4.6 × 250 mm, 3.5 μm column, operated at 40°C. Mobile phases used were made up water (A) and 0.01% formic acid in acetonitrile (B). The following gradient was used: 0–8 min, 10% B; 8–14 min, 10–100% B; 14–19 min, 100% B; 19–21 min, 100-10% B; 21–25 min, 10% B. The flow rate was held constant at 0.5 ml min−1 and the injection volume was 3 μL. The LC was interfaced to a quadruple mass spectrometer. The mass spectrometer was operated on ESI-positive mode at a mass range of m/z 50–600 at 70 eV cone voltage.
Serial dilutions of the authentic standard containing 18 amino acids (1–105 μg/μl, Sigma–Aldrich, St. Louis, MO, USA) was also similarly analyzed by LC-MS to generate linear calibration curves (peak area vs. concentration) used for external quantification. Amino acid analysis was repeated three times using different batch of samples.
Determination of in-vitro Protein Digestibility
Protein digestibility in the insect samples was analyzed by the method described by Mertz et al. (25). Initial protein content of the samples was determined using micro-Kjeldahl nitrogen determination method. This was followed by pepsin digestion, where 0.2 g of the sample was weighed into 50 mL centrifuge tubes. Then 20 mL buffered pepsin was added and mixed. Similarly, a blank was prepared but without a sample. The tubes were placed in a water bath at 37°C for 2 h with gentle shaking after every 20 min. The tubes were then centrifuged at 6,000 rpm for 15 min at 4-degree celcius. The supernatant was discarded, and 10 mL of buffer solution added, then shaking and centrifugation was done again. The supernatant was discarded, and the residue filtered using a Whatman filter paper No. 4. The filter paper was rolled and inserted into a Kjeldahl flask and dried for 15 min in the oven at 100 degrees celcius. Ten (10) mL of Concentrated sulphuric acid, 1 g potassium sulfate and 1 mL of 10% copper sulfate solution were added to the Kjeldahl flask containing the dried filter paper and sample. Then digestion, distillation and titration were done according to the micro-Kjeldahl nitrogen determination.
Protein digestibility (%) = (A - B)/A
Where A = % protein content in the sample before pepsin digestion
B = % protein in the sample after pepsin digestion.
Determination of Mineral Composition
The cricket powder was ashed and digested in 6N HCl and the content of the various minerals (Iron, Zinc, Calcium, magnesium, sodium, potassium, manganese, copper and cobalt) determined using atomic absorption spectrometry (AAS) (Shimadzu, AA-6300, Tokyo, Japan) according to AOAC methods (23).
Determination of Vitamin Content
The vitamin content of cricket samples was determined for selected fat-soluble (A, E, pro D) and water-soluble (B1, B2, B3, B6, B9) vitamins. We adopted Cheseto et al. (26) and Jermacz et al. (27) methods for the analysis of fat-soluble vitamins, briefly, each cricket sample (300 mg), was transferred into a 10 mL glass vial containing a mixture of hexane, methanol and distilled deionized water (2:1:2, 5 mL), vortexed for 30 s, sonicated for 30 min and centrifuged at 14,000 rpm for 5 min. The supernatant was dried over anhydrous Na2SO4, evaporated to dryness under a gentle stream of N2(g) before derivatizing any residual fatty acids to fatty acid methyl esters to limit the matrix interference following the protocol described elsewhere (28), analyzed (1.0 μL) by GC-MS on a 7890 A gas chromatograph linked to a 5,975 C mass selective detector (Agilent Technologies Inc., Santa Clara, CA, USA). The GC was fitted with a (5%-phenyl)-methylpolysiloxane (HP5 MS) low bleed capillary column (30 m × 0.25 mm i.d., 0.25 μm; J&W, Folsom, CA, USA). Helium at a flow rate of 1.25 mL min−1 served as the carrier gas. The oven temperature was programmed from 35°C to 285°C, with the initial temperature maintained for 5 min, with a rise at 10°C min−1 to 280°C, and then held at this temperature for 20.4 min. The mass selective detector was maintained at ion source temperature of 230°C and a quadrupole temperature of 180°C. Electron impact (EI) mass spectra were obtained at the acceleration energy of 70 eV. Fragment ions were analyzed over 40–550 m/z mass range in the full scan mode. The filament delay time was set at 3.3 min. Serial dilutions of the authentic standard α-tocopherol (≥ 95.5% purity) (0.1–100 ng/μL, Sigma- Aldrich, St. Louis, MO) was analyzed by GC-MS in full scan mode to generate a linear calibration curves (peak area vs. concentration) which gave coefficient of determinations R2 = 0.9999. The regression equation was used for the external quantification of the different selected fat- soluble vitamins (Retinol, γ-tocopherol, α-tocopherol, and Pro vitamin D).
These compounds were identified by comparison of mass spectral data and retention times with those of authentic standards and reference spectra published by library–MS databases: National Institute of Standards and Technology (NIST) 05, 08, and 11. The samples were analyzed in triplicate, with each replicate collected from a different batch of respective samples.
Determination of water–soluble vitamins was carried out according to previously described method (28, 29). Briefly, 100 mg of each cricket sample was transferred in to a 50 mL falcon tube containing 25 mL distilled deionized waster (25 mL), vortexed for 20 s, sonicated for 15 min and the mixture filtered through 0.2 μm filters into Ultra Performance Liquid Chromatography (UPLC) vials and analyzed by Shimadzu UPLC-DAD. The chromatographic analysis was performed on (LC-30AC with Nexera column oven CTO-30A, Shimadzu, Tokyo, Japan) fitted with a Phenomenex C18 Column Synergi 100 mm × 3.00 mm, 2.6 μm polar (Phenomenex, Torrance, CA, USA) at 30°C. The mobile phase consisted of two phases, A: 25 mM phosphate buffer. B: 7:3 v/v Acetonitrile-Mobile phase A. Total run time was 12 min with a flow rate of 0.4 mL/min. Stock solutions of 1.0 mg/ml were prepared by dissolving the individual water-soluble vitamin standards in distilled water except for Vitamin B2 in (5 mM potassium hydroxide) and Vitamin B9 in (20 mM potassium hydrogen carbonate). Serial dilutions of the stock solution (2–15 μg/mL) for the 5-water-soluble vitamins were also analyzed by UPLC-DAD giving R2 of 0.996 or greater. These regression equations were used for the external quantification of the different water-soluble vitamins. All determinations were carried out in triplicates from different batch of respective samples.
Data Analysis
Data were checked for normality using the Shapiro-Wilk test. To determine the differences in nutritional value of the two cricket species, unpaired t-test was used for normally distributed data with equal variances while Welch's t-test was used to analyse data that did not fulfill the two assumptions. All the statistical analyses were conducted using R software version 3.6.0 (30).
Results and Discussion
Proximate Composition Scapsipedus icipe and Gryllus bimaculatus
The proximate composition (on dry matter basis-DM) of S. icipe and G. bimaculatus are presented in Figure 1. The crude protein (CP) content of S. icipe and G. bimaculatus was comparable to that reported previously for field cricket (32, 33). However, the CP values in the present is relative lower compared to reported for Acheta domesticus Linnaeus (73.63%) (34). The variation can be attributed to inter-species differences as well as the type of substrates fed to cricket during rearing (35). The crude fat recorded for Gryllus bimaculatus was significantly (P = 0.0005) higher compared to that S. icipe. These results are consistent to that reported for A. domesticus (32.6 %) (36) but contrary to that documented in other study for the same species (18.55–22.80%) (37). Based on the crude fat content, it implies that the consumption of about 200 g of crickets would potentially contribute the daily requirement of energy from fat for human nutrition, which ranges between 10 and 30% (38).
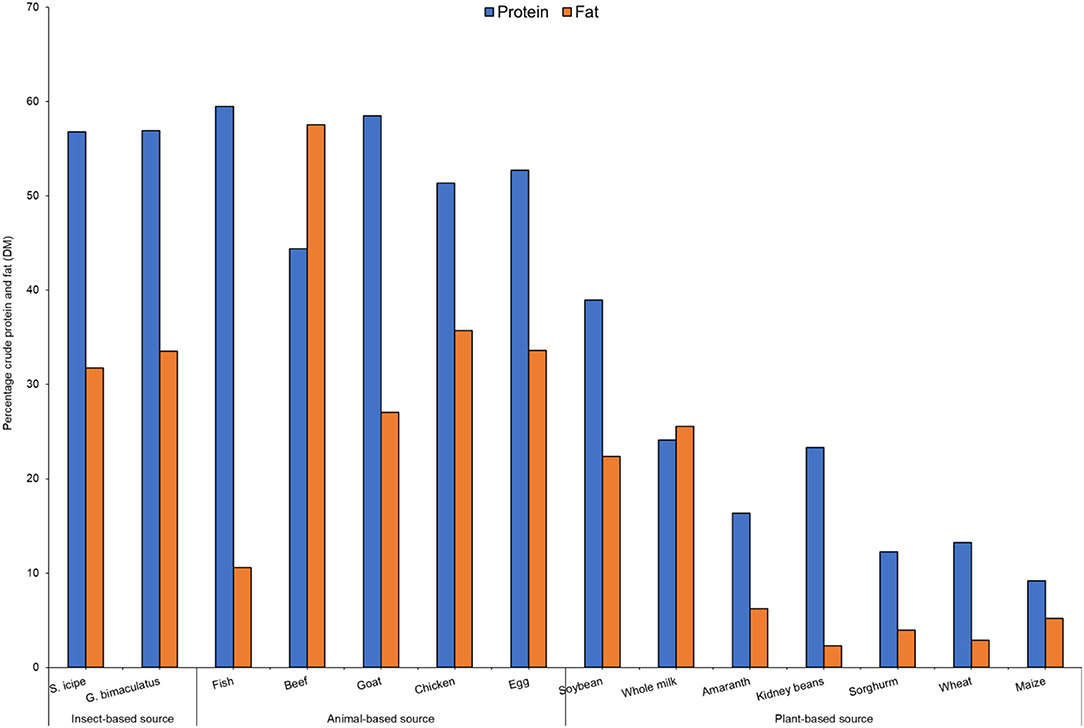
Figure 1. Comparative analysis of percentage crude protein and fat content [on dry matter basis—DM] of crickets (Scapsipedus icipe and Gryllus bimaculatus), and selected plants and animal sources. Data for plant and animal sources was extracted from FAO/GoK (31).
The values of crude ash reported in this study (Table 1) were comparable to that reported for A. domesticus (3.57%) (39). This aligns with results reported for adult cricket under rearing conditions (3.57–5.10% crude ash) in the United States of America (USA) (37). The crude fiber content of S. icipe and Gryllus bimaculatus are comparable to the values (5.95 and 8.7%) reported by Finke (40) and Moreki et al. (32) for crickets, respectively. Previous studies have demonstrated that the amount of crude fiber in an insect is a direct reflection of the chitin on the basis of the chemical structure (39, 40). In literature it is reported people of the African origin have high activity of chitinase enzyme, thus this opens new opportunities for the promotion and commercialization of edible insects chitin (41). Recently, chitin and chitosan have attracted considerable attention due to their biological activities (antifungal, antibacterial, antitumor, immunoadjuvant, antithrombogenic, anti-cholesteremic agent) and bio-adhesivity (42). Thus, chitin is widely used as absorption promoters and hydrating agents, as well as for film production and wound healing (43). The immunity-enhancing effects, promotion of beneficial bacterial growth and inhibition of pathogenic microorganisms have been reported, thus demonstrating clear health benefits after consumption (44–48). The application of chitin/chitosan for extension of shelf life of various foods from agriculture, poultry, and seafood origin by inhibiting microbial growth have been documented (49).
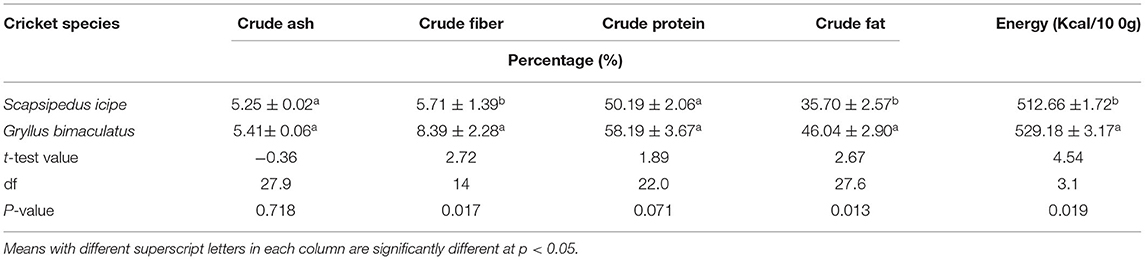
Table 1. Crude ash, crude fiber, crude protein, crude fat, and energy levels of Scapsipedus icipe and Gryllus bimaculatus on dry matter basis (mean ± SE).
The protein and fat content of the cricket species studied was comparable to that of common animal foods and higher than most plant sources (Figure 1). These results imply that 100 g of either cricket species consumed per day would provide at least twice as much protein as any of the common plant sources. Studies carried out in South Korea found that protein and fat content of five insect species including G. bimaculatus surpassed that of conventional livestock sources (50), which is slightly different from the current results. Therefore, consumption of S. icipe and G. bimaculatus could provide the much-needed fat and protein in communities where access to traditional animal sources are limited (51).
In general, edible insects in the order Orthoptera have been reported to contain significantly higher amounts of crude proteins compared to other insects (37, 52). However, quantifying bioavailable insect protein has been of great concern given that crude protein analysis using Kjeldahl method often includes nitrogen embedded in the exoskeleton of these insects which largely comprises the polysaccharide chitin, a phenomenon that tends to overestimate digestible protein of edible insects (52–54). In vitro protein digestibility has been shown as a reliable predictor of protein bioavailability in vivo based on Protein Efficiency Ratio (PER) and Net Protein Ratio (NPR) (29, 55). In this study, there was significant difference in digestibility of protein from both species were observed to be different (Figure 2). The values for S. icipe (87.8%) and Gryllus bimaculatus (79.5%) are within the range reported for other insects (76–96%) by Kourimská and Adámková (57). Other studies have also reported significantly higher protein digestibility values for the cricket species Gryllus assimilis (Fabricius) (73%) when compared to that of grasshopper, moth caterpillar and termite. The protein digestibility corrected for amino acid score of the newly described cricket species in Kenya (S. icipe) with 87.77%, is similar to that of egg (97%) and beef (98%) (58). Insect protein digestibility has been considered higher than most plant proteins in previous studies (59).
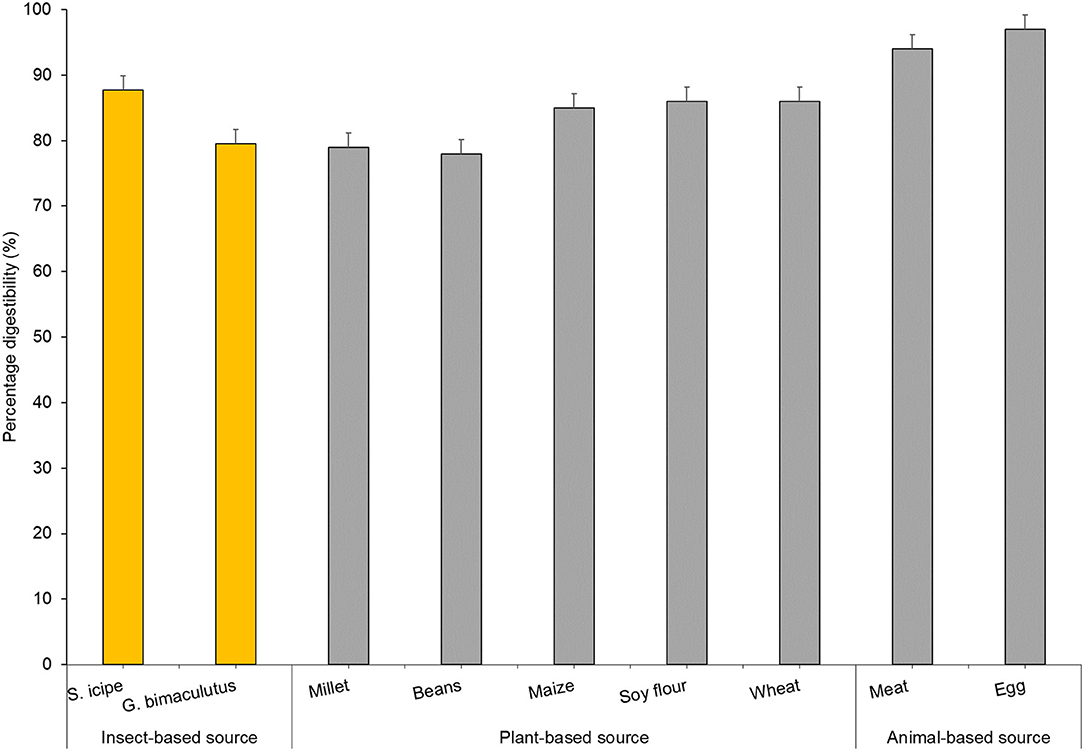
Figure 2. Comparative analyses of in vitro protein digestibility of crickets (Scapsipedus icipe and Gryllus bimaculatus), plants and animal food sources. Data for plant and animal sources was extracted from FAO/WHO/UNU (56).
Amino Acid Composition
Both S. icipe and Gryllus bimaculatus were found to have seven essential amino acids as shown in Figure 3. Five of these essential amino acids content varied significantly between S. icipe and G. bimaculatus. Non-essential amino acids detected S. icipe and G. bimaculatus are presented in Table 2. Leucine was the most abundant amino acid in S. icipe and Gryllus bimaculatus. The highest amount of glutamine, glutamic acid, proline, valine, methionine, leucine, and phenylalanine were recorded for G. bimaculatus. Histidine and lysine levels were more abundant in S. icipe than in Gryllus bimaculatus. No significant difference was observed for arginine (P = 0.0584), lysine (P = 0.2742), tyrosine (P = 0.7285), and isoleucine (P = 0.9412) between S. icipe and G. bimaculatus. The high levels of isoleucine and leucine recorded for icipe and G. bimaculatus are similar to that reported for other edible insects (60). The methionine content in S. icipe and G. bimaculatus were comparable to that reported for eggs, which is well-known as an excellent source of methionine. Methionine and lysine are the most limiting amino acid in legume and legume products such as bean, peas, lentils, chickpeas, and soybean as well as cereals, which is in line to that reported in other studies (61). Methionine is a classical sulfur amino acid, a limiting amino acid in plant proteins of legume origin and aids availability of cysteine which is a metabolic product of methionine catabolism. The values of methionine, leucine and phenylalanine recorded for Gryllus bimaculatus are similar to that reported for A. domesticus by Ramos-Elorduy et al. (33). Similarly, methionine and phenylalanine of the field cricket Gryllus testaceus Walker are comparable to the results observed in the present study, although, histidine, lysine, glutamic acid, proline, valine, tyrosine were higher except for much lower values for isoleucine and leucine (62). According to a report by FAO/WHO, daily amino acid requirements for human nutrition ranged between 0.010 and 0.039 mg/g (63), thus lower than the value reported for S. icipe and G. bimaculatus (31, 56, 64). This implies that both cricket species could be an excellent alternative and sustainable source of alternative amino acids than current animal and plant-based sources. Lysine deficient diets are common in African countries, where maize is a staple food, thus supplementing these diets with edible cricket protein would be a sustainable step toward dietary diversification (50) and ensuring nutritional security. However, the protein quality of the edible insects can further be improved with the removal of chitin which binds some amino acids (37).
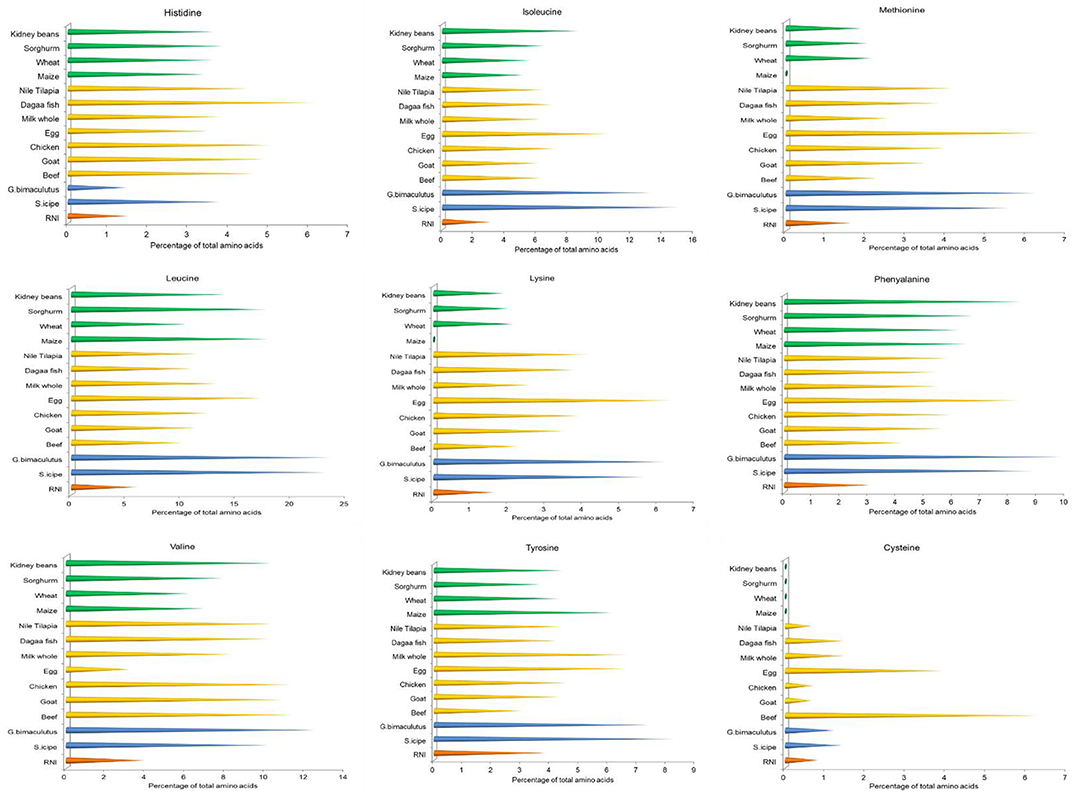
Figure 3. Comparison of selected essential amino acids content of crickets (Scapsipedus icipe and Gryllus bimaculatus), animal and plant-based sources. Amino acid data for plant and animal sources was extracted from FAO/GoK (31), while that of the recommended nutrient intake (RNI) was obtained from FAO/WHO/UNU (56).
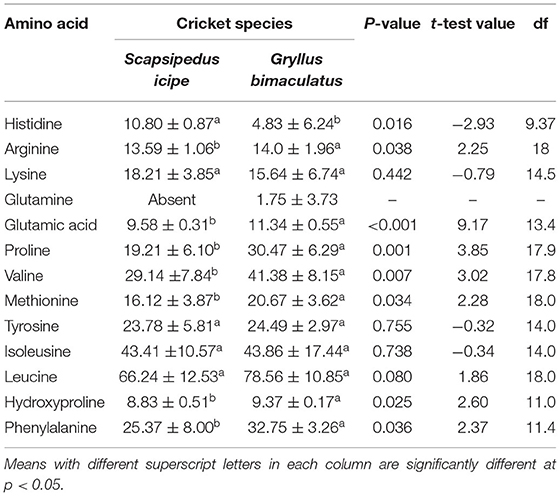
Table 2. Amino acid profile (μg/100 mg) of Scapsipedus icipe and Gryllus bimaculatus on dry matter basis (mean ± SE).
Histidine is an indispensable amino acid whose dietary deficiency has been shown to cause deleterious effects on hemoglobin concentrations in humans (65, 66), thus consumption of these cricket species could be a quick fix to such health complications. Amino acids such as Leucine, isoleucine, and valine, which are branched chain amino acids (BCAA) were recorded notably in higher amounts in both crickets than in the animal and plant-based sources. Several studies have documented the potential role of the BCAA in brain function and maintenance of muscle mass during weight loss (67–69). Other studies have cited leucine and histidine as essential in growth of children suggesting that both cricket species can be applied to supplemental diets to back up their protein requirements (70, 71). Leucine has a wide range of metabolic and regulatory influences in the body and has been shown to have a potential role in the treatment of obesity and metabolic syndrome due to its influence on insulin secretion and sensitivity as well as dietary macronutrient disposal (72, 73). Therefore, leucine can play a potential role in the prevention of type II diabetes (71, 73). Further, leucine has been shown to play a critical role in the reversal of adverse influences of high fat diet thus facilitating healthy weight maintenance in humans (72, 74, 75). Both cricket species in the present study were observed to have at least twice the amount of the recommended lysine and methionine for children at different age groups as well as adolescents (65). Lysine and arginine are important factors in the release of growth hormone in young children (76). High quality rich foods have been linked to reduced risk of stunting in young children aged 2–13 years (77, 78) and hence incorporating cricket-based protein in diets of young children and complementary or supplementary food products targeted at this most vulnerable segment of the resource poor communities in developing countries would contribute to easy access of diversified and well-balanced diets.
Mineral Composition
The importance of edible insects as a source of minerals has been documented in several studies (37, 79, 80). Based on the current study, the iron content of the two edible crickets ranged between 10.70 and 12.33 mg/100 g, which is similar to that reported for A. domesticus (11.23 mg/100 g) (81). Thus, the consumption of 100 g of S. icipe or G. bimaculatus will contribute to at least 54 and 100% of the recommended dietary allowance (RDA) of iron for women of reproductive age and children below 5 years of age, respectively (Table 3). Globally, iron deficiency is the commonest nutritional disorder affecting both children and women of reproductive age (82). For example, the anemia prevalence statistics for developing countries show that one in every two pregnant women and 40% of school going children are affected (82). Anemia which causes 20% of maternal deaths, also leads to irreversible health consequences including pre-term babies, poor physical and cognitive development, and increased risk of morbidity in children (82). It is thus, evident that integrating edible crickets into the regular diets of women and children might have the potential to address the anemia problem (61). Recent studies have also shown that acceptability of common staples that are fortified with edible insect meals is high (13) and should be encouraged.
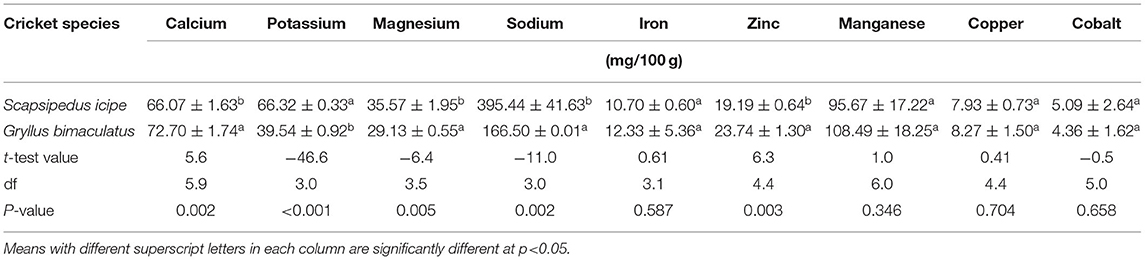
Table 3. Mineral composition of edible cricket species (Scapsipedus icipe and Gryllus bimaculatus) in milligrams/100 grams on dry matter basis (mean ± SE).
Zinc is another mineral of public health importance and the values obtained for S. icipe or G. bimaculatus in the present study are comparable to that of A. domesticus (18.64 mg/100 g) (81) but higher than that reported for A. domesticus (13 mg/100 g) (61) and Gryllodes Sigillatus (13.9 mg/g) (58). Consumption of 100 g of S. icipe or G. bimaculatus per day would potentially contribute enough nutrient to meet 100% of the recommended daily allowance for zinc (2–11 mg/100 g) for all age groups. As shown in Figure 4, the two cricket species are clearly superior in zinc content than common animal and plant foods. Thus, they may play a great role in alleviating this most common deficiency, which is associated with stunting and hypertension (83).
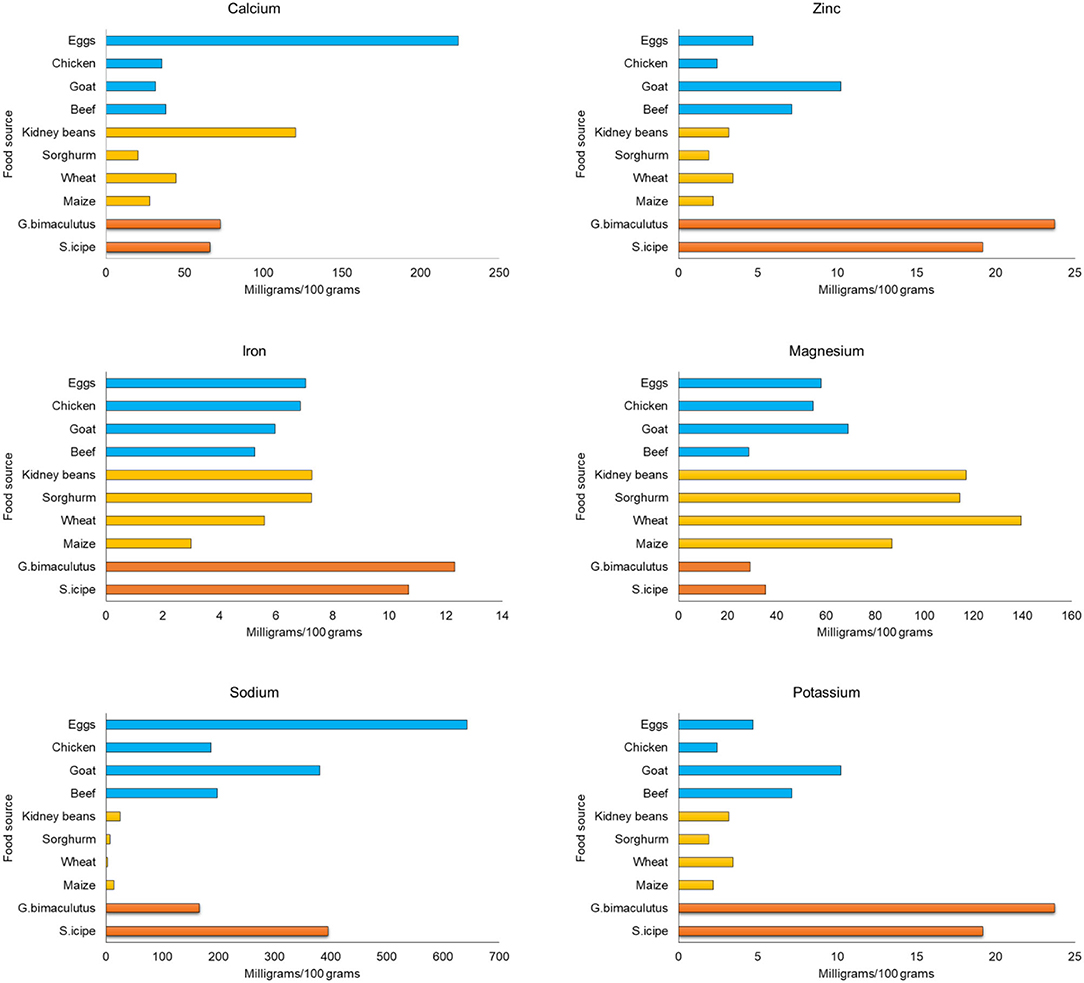
Figure 4. Comparison of mineral content of crickets (Scapsipedus icipe and Gryllus bimaculatus), animal and plant-based sources. Mineral content data for plant and animal food sources was extracted from FAO/GoK (31), while that of the recommended nutrient intake (RNI) was obtained from FAO/WHO/UNU (56).
Calcium content was higher in Gryllus bimaculatus (72.70%) than in S. icipe (66.07%). Values obtained in this study are higher than those recorded by Rumpold and Schlüter (36), Mousavi et al. (84) for A. domesticus. However, the calcium contents in this study are lower than 130 mg/100 g reported by Rumpold and Schlüter (36) for the edible cricket A. domesticus. This wide variation observed between the various studies can be attributed to the choice of diet fed to the crickets (35). It is anticipated that 100 g of either S. icipe and G. bimaculatus would contribute an estimated 16.5–18% of RDA for children (Table 3). Thus, these edible cricket species could potentially contribute significantly in alleviating calcium deficiencies in many highly vulnerable communities in sub-Saharan African countries, if incorporated in their diet regularly.
Magnesium content was higher in S. icipe (35.57 mg/100 g) than in Gryllus bimaculatus (29.13 mg/100 g) (Table 3). These values are close to 33.7% and higher than 22.6% previously reported for adult house cricket (A. domesticus) (39, 85). Therefore, intake of either S. icipe or G. bimaculatus food products could potentially contribute to ~50% of RDA of magnesium requirements for young children. The role played by magnesium in the body as a cofactor of enzymes involved in metabolism, synthesis of protein, RNA and DNA and the maintenance of electrical potential of nervous tissues and cell membranes has been well-documented (82).
The potassium content of S. icipe or G. bimaculatus ranged between 39.54 and 66.32 mg/100 g (Table 4), which is higher than value reported for other studies (37.4 mg/100g) (86). The potassium content of S. icipe was close to 74.6 mg/100 g, which is consistent to that reported for the giant African cricket (87). However, other studies have reported higher potassium content in cricket compared to values obtained in this study (39). The variation in potassium content could be attributed to differences in diets and age, as reported in crickets such as Brachytrypes membranaceus L. (88). No significant variation of copper content was observed between S. icipe and G. bimaculatus but the values recorded are higher than those reported for adult A. domesticus (0.62–0.85 mg/100 g) (39, 81). The sodium content of S. icipe and G. bimaculatus varied considerably, though the values were higher in the former than in the later. The sodium content of Gryllus bimaculatus was comparable to that reported for adult A. domesticus (134 mg/100 g) (39), while that of S. icipe was similar to that documented for the same cricket (430 mg/100 g) species (89). A higher potassium: sodium ratio in the two cricket species than common plant and animal foods (Figure 4) implies that they could be suitable source for people with or at risk of hypertension and the metabolic syndrome (90). The manganese content of S. icipe and G. bimaculatus were comparable. Comparative studies shows that the consumption of these crickets might be capable of contributing adequately to the amount of the RDA of manganese required for children, adolescents, adults, and even lactating mothers (82). However, the values obtained in this study are considerably higher than that reported for other adult crickets which ranged between 2.37 and 3.73 mg/100g (37). Similarly, the manganese content of the two cricket species in this study is significantly higher than the 1.15 mg/100 g reported in cricket species (39). However, further research to confirm this extreme high value of manganese is crucial. Finally, cobalt which is one of the trace minerals and whose main role is being a component of vitamin B12 (cyanocobalamin) (91) was recorded in S. icipe and G. bimaculatus. The value of cobalt in both crickets ranged between 4.36 and 5.09 mg/100 g, which is consistent to that reported in the Snout beetle (4.76 mg/100 g), though higher than those reported for termite (92, 93). This implies that cricket could potentially be a good source of minerals of public health importance such as iron and zinc with values that are superior to those of plant and animal-based food sources (Figure 4).
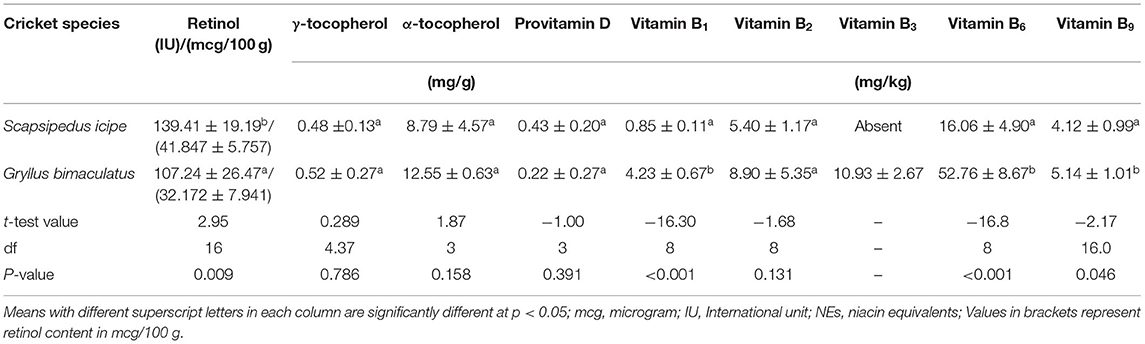
Table 4. Vitamin composition of edible cricket species (Scapsipedus icipe and Gryllus bimaculatus) on dry matter basis (mean ± SE).
Vitamins
There is insufficient data published on vitamin composition of edible insects and particularly on crickets such as A. domestica (57). In this study, the vitamin A (retinol) content of S. icipe (42 mcg/100 g) and Gryllus bimaculatus (32 mcg/100 g) varied considerably (Table 4). These values of vitamin A are relatively higher than what has been reported from adult domestic house cricket A. domesticus (24.33 mcg/100 g) (84), Brachytrypes spp. (0 mcg/100 g), adult grasshopper Cytacanthacris aeruginosus unicolor (1 mcg/100 g), short horned grasshoppers (6.82 mcg/100 g), Analeptes trifasciata (12.54 mcg/100 g), Anaphe infracta (2.95 mcg/100g), Anaphe recticulata (3.40 mcg/100 g), Anaphe spp. (2.78 mcg/100 g), Anaphe venata (3.12 mcg/100 g), Cirina forda (2.99 mcg/100 g), Apis mellifera (12.44 mcg/100 g), Oryctes boas (8.58 mcg/100 g), and Rhynchophorus phoenicis (11.25 mcg/100 mg) (88). Therefore, consumption of about 100 g of S. icipe (newly described species in science) can contribute to approximately 22% RDA of Vitamin A (retinol) among young children (Table 4). Children in sub-Sahara Africa and other low-income countries are particularly at risk of vitamin A deficiency (VAD), thus S. icipe and G. bimaculatus can be excellent promising and sustainable source of this vitamin (82).
The content of γ-tocopherol (P = 0.7823), alpha-tocopherol (P = 0.1536), and provitamin D observed in S. icipe and G. bimaculatus did not vary significantly. The values reported in this study for γ- and α-tocopherol were considerably higher than that of vitamin E content (0.072 mg/g) reported in reared cricket species such as A. domesticus (37). The high level of discrepancy in results of tocopherol concentrations in edible crickets could be attributed to the type of feeding substrate used (94).
The provitamin D concentration of the edible crickets ranged between 0.22 to 0.43 mg/g. However, there was no statistical significance difference (p = 0.2611) in provitamin D concentration between the two cricket species. The provitamin D concentration recorded for S. icipe and G. bimaculatus (0.22–0.43 mg/g) is much higher than 0.0064 mg/g reported for A. domesticus (39). This implies that the consumption of S. icipe and G. bimaculatus could also potentially contribute to the RDA for children, adolescents, adults, and lactating mothers.
However, the concentration of thiamine (vitamin B1) in Gryllus bimaculatus (4.23 mg/kg) was significantly higher when compared to that of S. icipe (0.85 mg/kg) fed on the same diet. The vitamin B1 content recorded for Gryllus bimaculatus is comparable to that reported for A. domesticus (3.6 mg/kg) (53). On the contrary the vitamin B1 of the two cricket species studied were considerably lower than 15.2 mg/kg reported for A. domesticus reared in Kenya (95). Contrarily, the Thiamine concentrations in S. icipe and G. bimaculatus are higher comparatively to that reported for other cricket species (0.4 mg/kg) (39). These differences observed could be associated to species difference and variation in rearing diet formulation (96).
For vitamin B2 (Riboflavin), no significant differences were observed (p = 0.0728) between S. icipe and G. bimaculatus. However, our values are comparable to that reported for the long-horned grasshopper Ruspolia differens (12.8 mg/kg) (97) and crickets (19.1 mg/kg) (98). The vitamin B2 content of the two cricket species reported in the present study is higher than what has been documented for other Orthopteran species (0.3–0.8 mg/kg) (99) and other commonly eaten insects in Southwestern Nigeria (0.03–3.24 mg/100 g). The variation in vitamin B2 content between literature and the findings of this study could be attributed to differences in species, age and feeding regime (96). Notably, niacin was not detected in S. icipe but the value recorded for G. bimaculatus (10.93 mg/kg) are lower compared to previous studies on crickets with values ranging between 31.0 and 38.4 mg/kg (39, 53).
The concentrations of vitamin B6 (pyridoxine) among the two cricket species differed significantly (p < 0.0001) with Gryllus bimaculatus recording the highest value (52.76 mg/kg) compared to S. icipe (16.06 mg/kg). The vitamin B6 concentrations observed in both crickets are lower than values (74.7 mg/kg) reported for other edible crickets (37).
The folic acid content of S. icipe (4.12 mg/kg) and Gryllus bimaculatus (5.14 mg/kg) were comparable but much higher than values reported for other edible crickets (1.5 mg/kg) in literature (39). Similarly, the folic acid content of the two cricket species is higher than what has been reported in Tenebrio molitor (1.37 mg/kg) (100). These levels of folic acid are also remarkably higher than that in some common foods (Figure 5) of animal and plant origin (Figure 5). Folic acid is a vitamin of public health significance whose deficiency is being addressed through folic acid supplementation and food fortification, especially during pregnancy to reduce cases of birth defects and consequently morbidity and mortality rates among children (100). Thus, S. icipe and Gryllus bimaculatus could be promising and cheaper alternative to commercial folic acid supplements. Similarly, the riboflavin levels in S. icipe and G. bimaculatus are significantly higher when compared to that of animal and plant-based food sources. It remarkable to note that S. icipe and Gryllus bimaculatus can significantly contribute to the recommended nutrient intake (RNI) of many vitamins such as riboflavin, thiamine, folic acids, and niacin, which are very critical for males, females, children, pregnancy, and lactating mothers as shown in Table 4 and Figure 5.
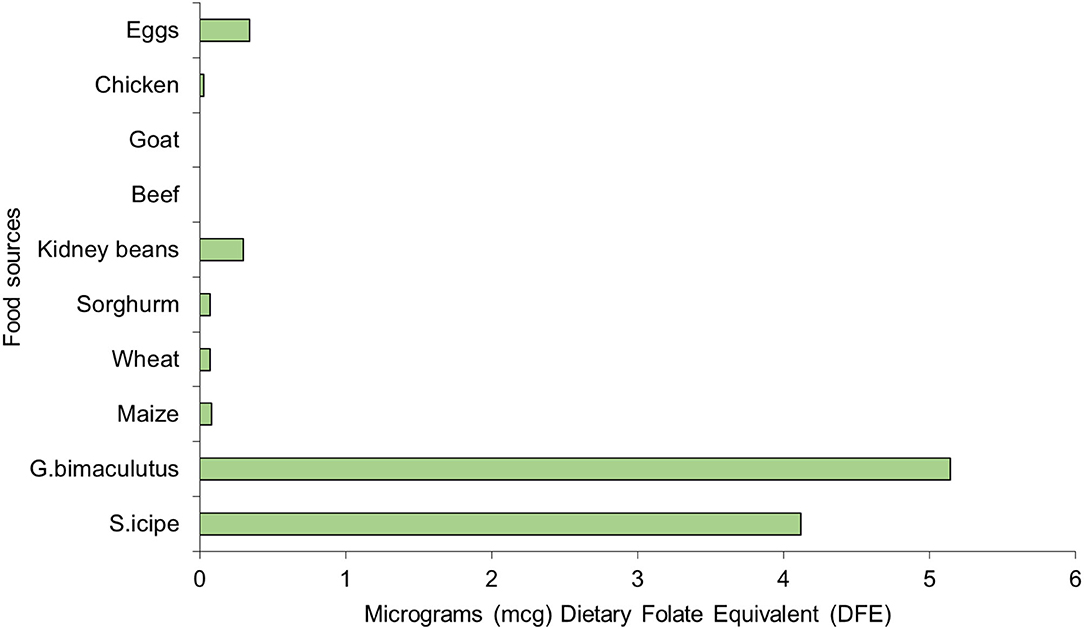
Figure 5. Folic acid content [microgram Dietary Folate Equivalent (mcg DFE)] of Scapsipedus icipe and Gryllus bimaculatus, animal and plant-based sources. Data for plant and animal food sources was extracted from FAO/GoK (31).
Conclusion
Scapsipedus icipe and G. bimaculatus present unique opportunities for improving the nutritional status of resource-poor and affluence populations toward addressing macronutrient and micronutrient malnutrition globally. Our results have demonstrated that the described cricket species can significantly contribute to the daily nutrient requirements of children and adults and children, particularly minerals (iron, zinc, calcium and others), protein, vitamins, and essential amino acids. It is important to note that crickets have featured in human diets around the world for many decades with their consumption widely practiced in parts of Africa, Asia, and Latin America. This partly explains why these insects are receiving increasing attention for their potential to alleviate the projected food and protein demand by the rapidly growing global population. Although, the sustainable utilization of crickets to contribute to our daily nutrient intake has received inadequate research attention globally, our results generated demonstrate the urgent need for their inclusion (whole, in-part, or processed flour) into human diet. This will play an important role toward the achievement of the “zero hunger” sustainable development goal target, given that 98% of the 795 million individuals suffering from hidden hunger live in low- and middle-income countries, where cricket consumption are widely accepted. Thus, there is urgent need for innovative community-based strategies for scaling up the coverage of cricket consumption. However, further studies on the bioavailability of Fe, Zn and folic acids from cricket-derived food products are required to quantify their overall dietary and therapeutic benefits. Also, factors that may interfere with nutrient intake or absorption such as processing, preservation, preparation, and incorporation into other foods needs to be addressed.
Data Availability Statement
The original contributions presented in the study are included in the article/supplementary material, further inquiries can be directed to the corresponding author/s.
Author Contributions
DM, AO, AN, IO, CX, DN, and CT: conceptualization, investigation, writing—review, and editing and final approval. DM and CT: data curation and formal analysis. CT: funding acquisition, project administration, and resources. DM, CX, and CT: methodology. AO, IO, and CT: supervision, validation, and visualization. DM, AO, IO, and CT: writing—original draft. All authors contributed to the article and approved the submitted version.
Funding
Financial support for this research was provided by the BioInnovate Africa Programme (INSBIZ—Contribution ID No. 51050076), Japan International Cooperation Agency (JICA) through the African Union – African innovation – JKUAT AND PAUSTI Network Project (AFRICA – ai – JAPAN Project – Phase 1), Federal Ministry for Economic Cooperation and Development (BMZ) (ENTONUTRI – 81194993), Canadian International Development Research Centre (IDRC) and the Australian Centre for International Agricultural Research (ACIAR) (INSFEED – Phase 2: Cultivate Grant No: 108866–001) and Norwegian Agency for Development Cooperation, the Section for research, innovation, and higher education grant number RAF−3058 KEN−18/0005 (CAP–Africa). We also gratefully acknowledge the support of International Centre of Insect Physiology and Ecology (icipe) core funding provided by United Kingdom's Foreign, Commonwealth and Development Office (FCDO); the Swedish International Development Cooperation Agency (Sida); the Swiss Agency for Development and Cooperation (SDC); the Federal Democratic Republic of Ethiopia; and the Government of the Republic of Kenya.
Author Disclaimer
The views expressed herein do not necessarily reflect the official opinion of the donors.
Conflict of Interest
The authors declare that the research was conducted in the absence of any commercial or financial relationships that could be construed as a potential conflict of interest.
Publisher's Note
All claims expressed in this article are solely those of the authors and do not necessarily represent those of their affiliated organizations, or those of the publisher, the editors and the reviewers. Any product that may be evaluated in this article, or claim that may be made by its manufacturer, is not guaranteed or endorsed by the publisher.
Acknowledgments
The authors wish to thank Jackton Ooko Ongere, Joshua Wambua, Shem Ondiaka, Rachami Isaiah E, and Faith Nyamu Wamurango for their substantial contribution in providing technical support during data collection.
References
1. United Nations Population Fund (UNFPA). State of World Population 2009: Facing a Changing World: Women, Population and Climate. UNFPA (2009).
2. Food and Agriculture Organization (FAO) United Nations World Food Programme (WFP) and International Fund for Agricultural Development (IFAD). The State of Food Insecurity in the World 2012. Economic Growth Is Necessary but Not Sufficient to Accelerate Reduction of Hunger and Malnutrition. FAO, Rome (2012).
3. Evans A. The Feeding of the Nine Billion: Global Food Security for the 21st Century. (2009). Available online at: https://www.wfp.org/stories/feeding-ten-billion-global-food-security-21st-century (accessed May 23, 2021).
4. Food and Agriculture Organization (FAO). The State of Food and Agriculture and Food Systems for Better Nutrition. FAO Corporate Document Repository. FAO, Rome (2013).
5. World Health Organization (WHO). Micronutrient Deficiencies. (2016). Available online at: http://www.who.int/nutrition/topics/ida/en/ (accessed January 13, 2021).
6. World Health Organization (WHO). Micronutrients. (2018). Available online at: http://www.who.int/nutrition/topics/micronutrients/en/ (accessed April 22, 2021).
7. Bhutta ZA, Das JK, Rizvi A, Gaffey MF, Walker N, Horton S, et al. Evidence-based interventions for improvement of maternal and child nutrition: what can be done and at what cost? Lancet. (2013) 382:452–77. doi: 10.1016/S0140-6736(13)60996-4
8. Roohani N, Hurrell R, Kelishadi R, Schulin R. Zinc, and its importance for human health: an integrative review. J Res Med Sci. (2013) 18:144–57.
9. Longo DL, Camaschella C. Iron-deficiency anemia. N Engl J Med. (2015) 372:1832–43. doi: 10.1056/NEJMra1401038
10. Mwangi MN, Roth JM, Smit MR, Trijsburg L, Mwangi AM, Demir AY, et al. Effect of daily antenatal iron supplementation on Plasmodium infection in Kenyan women: a randomized clinical trial. JAMA. (2015) 314:1009–20. doi: 10.1001/jama.2015.9496
11. International Food Policy Research Institute. Global Nutrition Report 2016: From Promise to Impact: Ending Malnutrition by 2030. Washington, DC: International Food Policy Research Institute (2016).
12. van Huis A. Potential of insects as food and feed in assuring food security. Annu Rev Entomol. (2013) 58:563–83. doi: 10.1146/annurev-ento-120811-153704
13. Kumar R. Anti-nutritional factors, the potential risks of toxicity and methods to alleviate them. In: Speedy A, Pugliese P, editors. Legume Trees and Other Fodder Trees as Protein Sources for Livestock. Rome: FAO (1992). p. 145–60.
14. Wieser S, Plessow R, Eichler K, Malek O, Capanzana MV, Agdeppa I, et al. Burden of micronutrient deficiencies by socio-economic strata in children aged 6 months to 5 years in the Philippines. BMC Public Health. (2013) 13:1167. doi: 10.1186/1471-2458-13-1167
15. World Health Organization (WHO). Global Health Estimates 2015: Deaths by Cause, Age, Sex and Country. (2016). Available online at: http://www.who.int/healthinfo/global_burden_disease/estimates/en/index1.html (accessed May 7, 2021).
16. Kassebaum NJ, Arora M, Barber RM, Bhutta ZA, Brown J, Carter A, et al. Global, regional, and national disability-adjusted life-years (DALYs) for 315 diseases and injuries and healthy life expectancy (HALE) 1990–2015: a systematic analysis for the Global Burden of Disease Study 2015. Lancet. (2016) 388:1603–58. doi: 10.1016/S0140-6736(16)31460-X
17. Verkerk MC, Tramper J, van Trijp JCM, Martens DE. Insect cells for human food. Biotechnol Adv. (2007) 25:198–202. doi: 10.1016/j.biotechadv.2006.11.004
18. Omotoso OT. The nutrient profile of the developmental stages of palm beetle, Oryctes rhinoceros L. Br J Environ Sci. (2018) 6:1–11.
19. Food and Agriculture Organization (FAO)/World Health Organization (WHO). Iron Deficiency Anaemia Assessment, Prevention, and Control A Guide for Programme Managers. (2001). p. 1–114.
20. Tanga CM, Magara HJO, Monica AA, Copeland RS, Khamis FM, Mohamed SA, et al. A new edible cricket species from Africa of the genus Scapsipedus. Zootaxa. (2018) 4486:383–92. doi: 10.11646/zootaxa.4486.3.9
21. International Centre of Insect Physiology and Ecology (icipe). An Overlooked Food Source. (2017). Available online at: http://www.icipe.org/news/insects-overlooked-food-source
22. Association of Official Analytical Chemists (AOAC). Official Methods of Analysis of the Association of Official Analytical Chemists. Vol. 2. 15th ed. Washington, DC (2009).
23. Association of Official Analytical Chemists (AOAC). Official Method of Analysis. 18th ed, Association of Officiating Analytical Chemists. Washington DC, Method. (2005). p. 935.14–992.24.
24. Cheseto X, Kachigamba DL, Ekesi S, Ndung'u M, Teal PEA, Beck JJ, et al. Identification of the ubiquitous antioxidant tripeptide glutathione as a fruit fly semiochemical. J Agric Food Chem. (2017) 65:8560–8. doi: 10.1021/acs.jafc.7b03164
25. Mertz ET, Hassen MM, Cairns-Whittern C, Kirleis AW, Tu L, Axtell JD. Pepsin digestibility of proteins in sorghum and other major cereals. Proc Natl Acad Sci. (1984) 81:1–2. doi: 10.1073/pnas.81.1.1
26. Cheseto X, Baleba SBS, Tanga CM, Kelemu S, Torto B. Chemistry, and sensory characterization of a bakery product prepared with oils from African edible insects. Foods. (2020) 9:800. doi: 10.3390/foods9060800
27. Jermacz I, Maj J, Morzycki JW, Wojtkielewicz A. GC-MS analysis of β-carotene ethenolysis products and their synthesis as potentially active vitamin A analogues. Toxicol Mech Methods. (2008) 18469–71. doi: 10.1080/15376510701623656
28. Thermo Fisher Scientific. Determination of Water – and Fat – Soluble Vitamins by HPLC, Knowledge Creation Diffusion Utilization. (2010). Available online at: http://www.dionex.com/en-us/webdocs/88784-TN89-HPLC-WaterFatSolubleVitamins-27Oct2010-LPN2598.pdf
29. Ramos-Elorduy J, Moreno JMP, Prado EE, Perez MA, Otero JL, de Guevara OL. Nutritional value of edible insects from the state of Oaxaca, Mexico. J Food Compos Anal. (1997) 10:142–57. doi: 10.1006/jfca.1997.0530
30. R Core Team. R: A Language and Environment for Statistical Computing. R Foundation for Statistical Computing, Vienna (2019). Available online at: http://www.R-project.org.
31. Ghosh S, Lee SM, Jung C, Meyer-Rochow VB. Nutritional composition of five commercial edible insects in South Korea. J Asia Pac Entomol. (2017) 20:686–94. doi: 10.1016/j.aspen.2017.04.003
32. Moreki JC, Tiroesele B, Chiripasi SC. Prospects of utilizing insects as alternative sources of protein in poultry diets in Botswana: a review. J Anim Sci Biotechnol. (2012) 2:649–58.
33. Yi L, Lakemond CMM, Sagis LMC, Eisner-Schadler V, van Huis A, van Boekel MAJS. Extraction and characterisation of protein fractions from five insect species. Food Chem. (2013) 141:3341–8. doi: 10.1016/j.foodchem.2013.05.115
34. Ramos-Elorduy J, González EA, Hernández AR, Pino JM. Use of Tenebrio molitor (Coleoptera: Tenebrionidae) to recycle organic wastes and as feed for broiler chickens. J. Econ. Entomol. (2002) 95:214–20. doi: 10.1603/0022-0493-95.1.214
35. Ramos-Bueno RP, González-Fernández MJ, Sánchez-Muros-Lozano MJ, García-Barroso F, Guil-Guerrero JL. Fatty acid profiles and cholesterol content of seven insect species assessed by several extraction systems. Eur Food Res Technol. (2016) 242:1471–7. doi: 10.1007/s00217-016-2647-7
36. Rumpold BA, Schlüter OK. Nutritional composition and safety aspects of edible insects. Mol Nutr Food Res. (2013) 57:802–23. doi: 10.1002/mnfr.201200735
37. Food and Agricultural Organization (FAO) and World Health Organization (WHO). Human Vitamin and Mineral Requirements. Report of a joint FAO/WHO expert consultation, Bangkok, Thailand. Rome: Food and Nutrition Division; FAO (2001). p. 223–4.
38. Finke MD. Complete nutrient composition of commercially raised invertebrates used as food for insectivores. Zoo Biol. (2002) 21:269–85. doi: 10.1002/zoo.10031
39. Finke MD. Estimate of chitin in raw whole insects. Zoo Biol. (2007) 26:93–104. doi: 10.1002/zoo.20122
40. Food and Agricultural Organization (FAO). Edible Insects. Future Prospects for Food and Feed Security. Food and Agriculture Organization of the United Nations 171 (2013).
41. Venter JP, Kotze AF, Auzely-Velty R, Rinaudo M. Synthesis, and evaluation of the mucoadhesivity of a CD-chitosan derivative. Int J Pharm. (2006) 313:36–42. doi: 10.1016/j.ijpharm.2006.01.016
42. Khor E. Chitin: a biomaterial in waiting. Curr Opin Solid State Mater Sci. (2002) 6:313–7. doi: 10.1016/S1359-0286(02)00002-5
43. Lee CG, Da Silva CA, Lee JY, Hartl D, Elias JA. Chitin regulation of immune responses: an old molecule with new roles. Curr Opin Immunol. (2008) 20:684–9. doi: 10.1016/j.coi.2008.10.002
44. Muzzarelli RAA. Chitins and chitosans as immunoadjuvants and non-allergenic drug carriers. Mar Drugs. (2010) 8:292–312. doi: 10.3390/md8020292
45. Reese TA, Liang HE, Tager AM, Luster AD, Van Rooijen N, Voehringer D, et al. Chitin induces accumulation in tissue of innate immune cells associated with allergy. Nat Faune. (2007) 3:92–6. doi: 10.1038/nature05746
46. Xia W, Liu P, Zhang J, Chen J. Biological activities of chitosan and chitooligosaccharides. Food Hydrocoll. (2011) 25:170–9. doi: 10.1016/j.foodhyd.2010.03.003
47. Liu D, Cai J, Xie CC, Liu C, Chen YH. Purification and partial characterization of a 36-kDa chitinase from Bacillus thuringiensis subsp. colmeri and its biocontrol potential. Enzyme Microb Technol. (2010) 46:252–6. doi: 10.1016/j.enzmictec.2009.10.007
48. No HK, Meyers SP, Prinyawiwatkul W, Xu Z. Application of chitosan for improvement of quality and shelf life of foods: a review. J Food Sci. (2007) 72:100–87. doi: 10.1111/j.1750-3841.2007.00383.x
49. Food and Agricultural Organization (FAO)/Government of Kenya. Kenya Food Composition Tables. Nairobi (2018). Available online at: http://www.fao.org/3/i8897en/I8897EN.pdf (accessed April 30, 2021).
50. Publishers WA. African edible insects for food and feed : inventory, diversity, commonalities and contribution to food security. J Insects Food Feed. (2015) 1:103–19. doi: 10.3920/JIFF2014.0016
51. Bukkens SGF. The nutritional value of edible insects. Ecol Food Nutr. (1997) 36:287–319. doi: 10.1080/03670244.1997.9991521
52. Jonas-Levi A, Martinez JJI. The high level of protein content reported in insects for food and feed is overestimated. J Food Compos Anal. (2017) 62:184–8. doi: 10.1016/j.jfca.2017.06.004
53. Van Huis A. Edible insects are the future? Proc Nutr Soc. (2016) 75:294–305. doi: 10.1017/S0029665116000069
54. Hur SJ, Lim BO, Decker EA, McClements DJ. In vitro human digestion models for food applications. Food Chem. (2011) 125:1–12. doi: 10.1016/j.foodchem.2010.08.036
55. Hsu HW, Vavak DL, Satterlee LD, Miller GA. A multienzyme technique for estimating protein digestibility. J Food Sci. (1977) 42:1269–73. doi: 10.1111/j.1365-2621.1977.tb14476.x
56. Chakravorty J, Ghosh S, Megu K, Jung C, Meyer-Rochow VB. Nutritional and anti-nutritional composition of Oecophylla smaragdina (Hymenoptera: Formicidae) and Odontotermes sp. (Isoptera: Termitidae): Two preferred edible insects of Arunachal Pradesh, India. J Asia Pac Entomol. (2016) 19:711–20. doi: 10.1016/j.aspen.2016.07.001
57. Kourimská L, Adámková A. Nutritional and sensory quality of edible insects. NFS J. (2016) 4:22–6. doi: 10.1016/j.nfs.2016.07.001
58. Finke MD. Nutrient content of insects. In: Capinera JL, editors. Encyclopedia of Entomology, Dordrecht; London: Kluwer Academic (2004). p. 1562–75.
59. Food and Agricultural Organization (FAO)/World Health Organization (WHO)/United Nations University (UNU). Protein and Amino Acid Requirements. Report of a Joint FAO/WHO/UNU Expert Consultation: Protein and Amino Acid Requirements in Human Nutrition (2007).
60. Tomé D, Bos C. Lysine requirement through the human life cycle. J Nutr. (2007) 137:1642S–5S. doi: 10.1093/jn/137.6.1642S
61. Rumpold BA, Schlüter OK. Potential and challenges of insects as an innovative source for food and feed production. Innov Food Sci Emerg Technol. (2013) 17:1–11. doi: 10.1016/j.ifset.2012.11.005
62. Wang D, Zhai SW, Zhang CX, Bai YY, An SH, Xu YN. Evaluation on nutritional value of field crickets as a poultry feedstuff. Asian Australasian J Anim Sci. (2005) 18:667–70. doi: 10.5713/ajas.2005.667
63. Food and Agricultural Organization (FAO)/World Health Organization (WHO)/United Nations University (UNU). Protein and Amino Acid Requirements. Report of a Joint FAO/WHO/UNU Expert Consultation: Protein and Amino Acid Requirements in Human Nutrition (2008).
65. Kurpad AV, Raj T, El-Khoury A, Beaumier L, Kuriyan R, Srivatsa A, et al. Lysine requirements of healthy adult Indian subjects, measured by an indicator amino acid balance technique. Am J Clin Nutr. (2001) 1–3:900–7. doi: 10.1093/ajcn/73.5.900
66. Fernstrom JD. 4th Amino Acid Assessment Workshop Branched-Chain Amino Acids and Brain Function. J Nutr. (2018) 135:1539–46. doi: 10.1093/jn/135.6.1539S
67. Cano N, Fougue D, Leverve X. Branched-chain amino acids : metabolism, physiological function, and application. Application of branched-chain amino acids in human pathological states: renal failure. J Nutr. (2006) 136:331–2. doi: 10.1093/jn/136.1.299S
68. Layman DK, Walker DA. Branched-chain amino acids : metabolism, physiological function, and application. Potential importance of leucine in treatment of obesity and the metabolic syndrome. J. Nutr. (2018) 136:319–23. doi: 10.1093/jn/136.1.319S
69. Chakravorty J, Ghosh S, Meyer-rochow VB. Practices of entomophagy and entomotherapy by members of the Nyishi and Galo tribes, two ethnic groups of the state of Arunachal Pradesh. J Ethnobiol Ethnomed. (2011) 7:5. doi: 10.1186/1746-4269-7-5
70. Millward DJ. Knowledge gained from studies of leucine consumption in animals and humans. J Nutr. (2012) 142:2212–2219S. doi: 10.3945/jn.111.157370
71. Zhang Y, Guo K, LeBlanc RE, Loh D, Schwartz GJ, Yu Y-H. Increasing dietary leucine intake reduces diet-induced obesity and improves glucose and cholesterol metabolism in mice via multimechanisms. Diabetes. (2007) 56:1647–54. doi: 10.2337/db07-0123
72. Yang J, Wong RK, Park M, Wu J, Cook JR, York DA, et al. Leucine regulation of glucokinase and ATP synthase sensitizes glucose-induced insulin secretion in pancreatic beta-cells. Diabetes. (2006) 55:193–201. doi: 10.2337/diabetes.55.01.06.db05-0938
73. Truby H, Baic S, deLooy A, Fox KR, Livingstone MBE, Logan CM, et al. Randomised controlled trial of four commercial weight loss programmes in the UK: initial findings from the BBC “diet trials”. BMJ. (2007) 332:80. doi: 10.1136/bmj.38833.411204.80
74. Macotela Y, Emanuelli B, Bang AM, Espinoza DO, Boucher J, Beebe K, et al. Dietary leucine - an environmental modifier of insulin resistance acting on multiple levels of metabolism. PLoS ONE. (2011) 6:e21187. doi: 10.1371/journal.pone.0021187
75. Uauy R, Kurpad A, Tano-Debrah K, Otoo GE, Aaron GA, Toride Y, et al. Role of protein and amino acids in infant and young child nutrition : protein and amino acid needs and relationship with child growth. J Nutr Sci Vitaminil. (2015) 61:192–4. doi: 10.3177/jnsv.61.S192
76. Ghosh S, Suri D, Uauy R. Assessment of protein adequacy in developing countries : quality matters. Br J Nutr. (2012) 108(Suppl. 2):S77–87. doi: 10.1017/S0007114512002577
77. Neumann CG, Bwibo NO, Murphy SP, Sigman M, Whaley S, Allen LH, et al. Animal source foods to improve dietary quality, micronutrient status, growth and cognitive function in Kenya school children: background, study design and baseline findings. J Nutr. (2003) 133:3957–64. doi: 10.1093/jn/133.11.3941S
78. Christensen DL, Orech FO, Mungai MN, Larsen T, Friis H, Aagaard-Hansen J. Entomophagy among the Luo of Kenya: a potential mineral source? Int J Food Sci Nutr. (2006) 57:198–203. doi: 10.1080/09637480600738252
79. Zielińska E, Baraniak B, Karaś M, Rybczyńska K, Jakubczyk A. Selected species of edible insects as a source of nutrient composition. Food Res Int. (2015) 77:460–6. doi: 10.1016/j.foodres.2015.09.008
80. Barker D, Fitzpatrick MP, Dierenfeld ES. Nutrient composition of selected whole invertebrates. Zoo Biol. (1998) 134:123–34.
81. Food and Agricultural Organization (FAO) and World Health Organization (WHO). Human vitamin and mineral requirements. Hum Vitam Miner Requir. (2001) 223–224.
82. Martin NM, Oonincx DGAB, Stouten T, Veenenbos M, Melse-Boonstra A, Dicke M, et al. Insects as sources of iron and zinc in human nutrition. Nutr Res Rev. (2018) 31:248–55. doi: 10.1017/S0954422418000094
83. Collavo A, Glew RH, Huang Y-S, Chuang LT, Bosse R, Paoletti MG. House cricket small-scale farming. In: Paoletti MG, editor. Ecological Implications of Minilivestock: Potential of Insects, Rodents, Frogs, and Snails. Enfield, NH: Science Publishers (2005). p. 519–44.
84. Mousavi SM, Mofrad MD, do Nascimento IJB, Milajerdi A, Mokhtari T, Esmaillzadeh A. The effect of zinc supplementation on blood pressure: a systematic review and dose–response meta-analysis of randomized-controlled trials. Eur J Nutr. (2020) 59:1815–27. doi: 10.1007/s00394-020-02204-5
85. Akhtar Y, Isman MB. Insects as an alternative protein source. In: Isman MB, editor. Proteins in Food Processing. 2nd ed., Sawston Cambridge: Woodhead Publishing Series in Food Science, Technology and Nutrition. (2018). p. 263–88.
86. Adeyeye EI, Awokunmi EE. Chemical composition of female and male giant African cricket, brachytrypes membranaceus L. Int J Pharm Bio Sci. (2010) 1:125–36.
87. Banjo AD, Lawal OA, Songonuga EA. The nutritional value of fourteen species of edible insects in southwestern Nigeria. African J Biotechnol. (2006) 5:298–301. doi: 10.5897/AJB05.250
88. Bernard JB, Allen ME, Ullrey DE. Feeding captive insectivorous animals: nutritional aspects of insects as food. Nutr Advis Gr Handb. (1997) 25:7.
89. Li X, Guo B, Jin D, Wang Y, Jiang Y, Zhu B, et al. Association of dietary sodium: potassium ratio with the metabolic syndrome in Chinese adults. Br J Nutr. (2018) 120:612–18. doi: 10.1017/S0007114518001496
90. Farrell JJ. Digestion and absorption of nutrients and vitamins. In: Feldman M, Friedman L, Brandt L, editors. Sleisenger and Fordtran's Gastrointestinal and Liver Disease. 9th ed. Philadelphia, PA: Saunders (2010). p.1695.
91. Adeyeye EI, Olaleye AA. Nutrient content of five species of edible insects consumed in South-West Nigeria. EC Nutr. (2016) 56:1285–97.
92. World Health Organization (WHO). Guideline: Potassium Intake for Adults and Children. Geneva: World Health Organization (2012). Available online at: https://apps.who.int/iris/bitstream/handle/10665/77986/9789241504829_eng.pdf
93. Chen X, Feng Y, Chen Z. Common edible insects and their utilization in China: invited Review. Entomol. Res. (2009) 39:299–303. doi: 10.1111/j.1748-5967.2009.00237.x
94. Ayieko MA, Ogola HJ, Ayieko IA. Introducing rearing crickets (gryllids) at household levels: adoption, processing and nutritional values. JIFF. (2016) 2:203–11. doi: 10.3920/JIFF2015.0080
95. Payne CLR, Scarborough P, Rayner M, Nonaka K. A systematic review of nutrient composition data available for twelve commercially available edible insects, and comparison with reference values. Trends Food Sci. Technol. (2015) 47:69–77. doi: 10.1016/j.tifs.2015.10.012
96. Kinyuru JN, Kenji GM, SN Muhoho, Ayieko MA. Nutritional potential of longhorn grasshopper (Ruspolia differens) consumed in Siaya District, Kenya. J Agric Sci Technol. (2010) 1:32–46.
97. Yhoung-Aree J, Puwastien P, Attig GA. Edible insects in Thailand: an unconventional protein source? Ecol Food Nutr. (1997) 36:133–149. doi: 10.1080/03670244.1997.9991511
98. Alamu OT, Amao AO, Nwokedi CI, Oke OA, Lawa IO. Diversity and nutritional status of edible insects in Nigeria: a review. Int J Biodivers Conserv. (2013) 5:215–22. doi: 10.5897/IJBC12.121
99. Nowak V, Persijn D, Rittenschober D, Charrondiere UR. Review of food composition data for edible insects. Food Chem. (2016) 193:39–46. doi: 10.1016/j.foodchem.2014.10.114
Keywords: edible crickets, Scapsipedus icipe, Gryllus bimaculatus, nutrient quality, recommended nutrient intake, human food
Citation: Murugu DK, Onyango AN, Ndiritu AK, Osuga IM, Xavier C, Nakimbugwe D and Tanga CM (2021) From Farm to Fork: Crickets as Alternative Source of Protein, Minerals, and Vitamins. Front. Nutr. 8:704002. doi: 10.3389/fnut.2021.704002
Received: 01 May 2021; Accepted: 13 July 2021;
Published: 10 August 2021.
Edited by:
Mauro Serafini, University of Teramo, ItalyReviewed by:
Karen Aulrich, Thuenen Institute of Organic Farming, GermanySaptawati Bardosono, University of Indonesia, Indonesia
Su Peng Loh, Universiti Putra Malaysia, Malaysia
Copyright © 2021 Murugu, Onyango, Ndiritu, Osuga, Xavier, Nakimbugwe and Tanga. This is an open-access article distributed under the terms of the Creative Commons Attribution License (CC BY). The use, distribution or reproduction in other forums is permitted, provided the original author(s) and the copyright owner(s) are credited and that the original publication in this journal is cited, in accordance with accepted academic practice. No use, distribution or reproduction is permitted which does not comply with these terms.
*Correspondence: Chrysantus M. Tanga, Y3RhbmdhQGljaXBlLm9yZw==; Dorothy K. Murugu, ZG9yb3RoeW11Z2FuZUB5YWhvby5jb20=