- 1Department for Food Chemistry and Toxicology, Faculty of Chemistry, University of Vienna, Vienna, Austria
- 2Laboratory of Toxicology, Department of Health Sciences and Technology, Eidgenoessische Technische Hochschule (ETH) Zurich, Zurich, Switzerland
Some prenylated polyphenols originating from hops, which are thus natural constituents of beer, have been discussed critically for their agonistic potential toward estrogen receptors. So far, little attention has been attributed to the fact that humans are typically not exposed to isolated compounds, but to mixtures which for example might comprise in addition to hop flavonoids further xenoestrogens, e.g., certain pesticides used for plant protection of hops and barley. Thus, we used the alkaline phosphatase assay to assess combinatory estrogenic effects of three signature compounds – xanthohumol, 8-prenylnaringenin and iso-xanthohumol–on Ishikawa cells in a combination that resembled the concentration ratios observable in beer. Moreover, we added this natural flavonoid pattern to a mixture of representative estrogenic pesticides to assess their combined effects. Using state-of-the-art statistical tools, we observed cumulative to slightly synergistic effects between isolated flavonoids as well as the flavonoid and the pesticide mixture. Of potential importance, these effects were found at low nanomolar hop polyphenol concentrations that one can reasonably expect to occur in vivo after the consumption of strongly hopped beer. Taken together, our results imply that cumulative/synergistic estrogenicity should be explored in detail and urgently be incorporated into risk assessment of prenylated chalcones.
Introduction
Endocrine disruptive chemicals (EDCs) are in the spotlight of an ongoing public debate. Particularly, the impact of exposure to so-called “xenoestrogens,” exogenous compounds that target the human estrogen receptor (ER), on human health is extensively discussed (1, 2). The public debate has contributed to the advance of regulative limits for food-contaminating chemicals such as bisphenol A, phthalates, and several pesticides. Among the latter, the 1970's scandal around the insecticide and endocrine disruptor dichlorodiphenyltrichlorethane (DDT) has cumulated in its world-wide ban with the Stockholm convention of 2001 (3). While the European Union has since forbidden the new registration of hormonally active compounds for agricultural use (4), such chemicals are still applied on fields in other parts of the world, including the USA and Canada. Without a doubt, the regulations issued within the EU have improved consumer safety. However, recent research makes way for the suspicion that risk assessment for xenoestrogens is still incomplete, as it (a) yet lacks the incorporation of mixture effects and (b) might thus seriously underestimate the impact of xenoestrogens occurring in food as natural constituents.
“Mixture effects” or “combinatory effects” are defined as the biological impact caused by two or more chemicals, and can be divided in two groups: A “cumulative” or “additive” effect describes the event when several chemicals cause a combinatory effect that can be explained by addition of their effects as single compounds (5). If this is not the case, a mixture effect is referred to as “interaction,” which can in turn be either antagonistic (the combinatory effect is lower than the additive effect of the single compounds) or synergistic (vice versa).
Concerning pesticides, the European Food Safety Authority (EFSA) is working on a cumulative risk assessment program (6), from which two pilot studies are published, focusing on pesticides affecting the thyroid (7) and the nervous system (8). However, important this initiative is for consumer safety, it primarily focuses on cumulative effects rather than synergisms, and moreover, does not consider combinatory effects with potentially co-occurring bioactives from other sources than plant protection agents. Particularly in the case of estrogenicity, this might lead to an underestimation of the chemical hazard.
Fueled by the comparably unspecific ligand binding of ERs, the sheer amount of known xenoestrogens from a wide range of different - including natural - sources makes their co-occurrence in food the rule rather than the exception (2). Therefore, it seems reasonable to expect combinatory effects on the endocrine system. This is also reflected in recent literature. Vejdovszky et al. (9) described synergistic estrogenic effects of co-occurring mycotoxins as well as a synergistic estrogenic interaction of the soy flavonoid genistein with single representatives of those natural food contaminants (10). Furthermore, we recently described cumulative estrogenic effects of mycotoxins with the controversially discussed endocrine disruptor bisphenol A (11) and an antagonistic interaction between regulated Fusarium toxins and a mixture of hop polyphenols (12).
The latter seem a particularly intriguing compound class from a food toxicologist's point of view. With beer as the main dietary source, prenylated chalcones in general – and the signature compound xanthohumol (XN, Figure 1A) in particular – have been linked with beneficial effects on human health due to their strong anti-oxidative capacity (13). This has also inspired efforts of breweries to develop hop polyphenol-enriched beers (14).
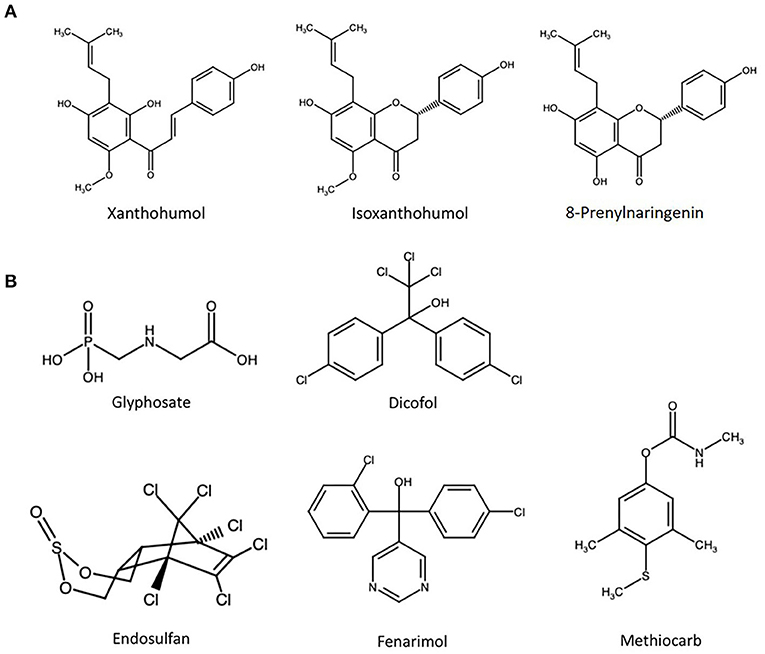
Figure 1. Chemical structure of (A) the three most prevalent hop polyphenols in beer and (B) selected pesticides with at least one report of estrogenicity.
On the other hand, and of potential concern, several metabolites of XN have been described to act as endocrine disruptors (15, 16). Among those compounds, 8-prenylnarginenin (8PN, Figure 1A) stands out as an extremely potent ER agonist and is referred to as the strongest phytoestrogen known today (17). It is formed from the comparably weak xenoestrogen iso-xanthohumol (iX, Figure 1A) in planta, but also in the brewing process and during digestion by the gut microbiome (18, 19). Accordingly, hops is widely used in folk and herbal medicine as a treatment for menopausal discomfort (20). It seems somewhat contradictory that prenylated chalcones are on the one hand openly marketed due to their effects on the endocrine system, but on the other hand considered as safe – and even beneficial – in beer. Obviously, this assumption could be valid for toxicokinetic reasons, i.e., when the systemic concentrations of estrogenic hop polyphenols that are achievable from beer consumption are below the point of departure for endocrine disruptive effects. However, this potential issue seems to be not very well-elucidated in a field that has so far largely focused on potentially beneficial properties of flavonoids in beer (13, 21). Moreover, the contribution of hop flavonoids to potential combinatory effects with other xenoestrogens is largely unexplored. In the only study published on this issue, we previously described a mixture of beer polyphenols to antagonize the estrogenic effects of the well-described natural xenoestrogen zearalenone in human endometrial cancer cells, and attributed this effect to anti-estrogenic properties of XN (12).
In the present study, five exemplary pesticides (Figure 1B) were selected based on at least one published report of them acting as ER agonists, among them the publicly discussed herbicide glyphosate (GLP). Alkaline phosphatase (ALP) expression in Ishikawa cells was used as a natural reporter gene system for estrogenicity. On the contrary to some other in vitro test systems, this human endometrial cancer cell line expresses both, ER-α and ER-β, and thus facilitates the observation of interactions between chemicals acting as agonists to different receptor isoforms (22). Measuring the ALP-catalyzed conversion rate of 4-nitrophenyl phosphate to 4-nitrophenol as a marker for enzyme expression and thus ER activation, the test compounds were tested individually and in combination to generally address the concern of cumulative effects within this class of pesticides. To enable a direct comparison of effect strength, the same test system was used to determine the estrogenicity of a mixture of the most important hop polyphenols, resembling their respective concentration ratios in beer. Lastly, the two mixtures were combined in different ratios to assess potential combinatory effects toward ER activation.
Materials and Methods
Materials
17β-estradiol (E2), 4-nitrophenyl phosphate, diethanolamine, fulvestrant (ICI 182,780), 8-penylnaringenin (8PN), dicofol (Dic), endosulfan (End), fenarimol (Fen), glyphosate (Glp) and methiocarb (Met) were purchased from Sigma-Aldrich (Schnelldorf, Germany), xanthohumol (XN) and iso-xanthohumol (iX) from Extrasynthese (Genay, France). Concerning cell culture, flasks and dishes were obtained from Sarstedt (Nümbrecht, Germany), media (DMEM, DMEM/F-12) as well as fetal bovine serum (FBS) from GIBCO / Thermo Fisher Scientific (Karlsruhe, Germany) and penicillin/streptomycin (P/S) as well as carcoal dextrane-stripped FBS (CD-FBS) from Sigma-Aldrich (Schnelldorf, Germany).
Cell Culture
The human endometrial adenocarcinoma cell line Ishikawa was obtained from EACC (Salisbury, UK) and cultured in Dulbecco's Modified Eagle Medium (DMEM) supplemented with 5% FBS and 1% P/S, at 37°C, 5% CO2 and under humidified conditions. Cells were subcultivated twice a week at a confluence of ~85% and used for experiments at a passage number between 5 and 20 to ensure reproducibility of results.
Alkaline Phosphatase (ALP) Assay
ALP assays were performed as recently described (23). Briefly, 10,000 Ishikawa cells per well were seeded in 96-well plates and allowed to attach for 48 h in assay medium (phenol red - free DMEM/F-12 supplemented with 1% (v/v) CD-FBS and 1% (v/v) P/S). Stock solutions were prepared by solving test compounds in DMSO, which were then diluted to the test concentrations in assay medium, resulting in a final DMSO concentration of 0.1–0.2% (v/v) (depending on the number of mixed compounds in incubation solutions, but consistently reflected by the same concentration in the respective solvent control). Cells were incubated with incubation solutions containing the test compounds or combinations thereof for another 48 h. Subsequently, cells were washed with PBS and lyzed by shock-freezing. ALP activity was determined by monitoring the conversion of 4-nitrophenyl phosphate to 4-nitrophenol photometrically at 405 nm with a PerkinElmer Victor 3VTM plate reader. The slope of the obtained dose-response curves was taken as a measure for the ER-dependent induction of ALP expression.
Sulforhodamine B (SRB) Assay
To ensure that effects observed in ALP assays were not caused by cytotoxicity, SRB cell viability assays were carried out in parallel. As with estrogenicity assays, 10,000 cells/well were seeded in 96-well plates, allowed to grow for 48 h and incubated with test solutions for another 48 h. Afterwards, cells were fixed by addition of 50% (v/v) trichloroacetic acid and 1 h incubation at 4°C. After 2 times washing with tab water and 2 times of washing with 1% (v/v) acetic acid, plates were dried overnight, incubated with SRB reagent for 45 min, washed twice with water, twice with 1% acetic acid and again dried overnight. Lastly, 10 mM TRIS base buffer was added to dissolve protein-bound SRB and the absorbance of each well was measured at 570 nm with a PerkinElmer Victor 3VTM plate reader.
Dose Rationale
Representative pesticides (Dic, End, Fen, Glp, Met) were tested in a mixture ratio that was based on the maximum residue levels (MRL) for beer (corresponding to the MRLs in hops or in barley, whichever is set lower) as required by EU law (Table 1). Thus, one unit “MRL eq.” refers to a mixture of pesticides (Mix P), with the dose of each rounded down to meet a round lot nanomolar concentration (i.e., 50 nM Dic, 100 nM End, 50 nM Fen, 500 nM Glp and 200 nM Met). For hop flavonoids, we estimated average concentrations in beer based on a survey (Table 2) by Stevens et al. (24), with one unit “beer eq.” of referring to a flavonoid mixture (Mix F) of 200 nM XN, 2 μM iX and 100 nM 8PN. The estrogenicity of the two mixtures was assessed by dilution of the mixture in parallel to the contained single compounds.
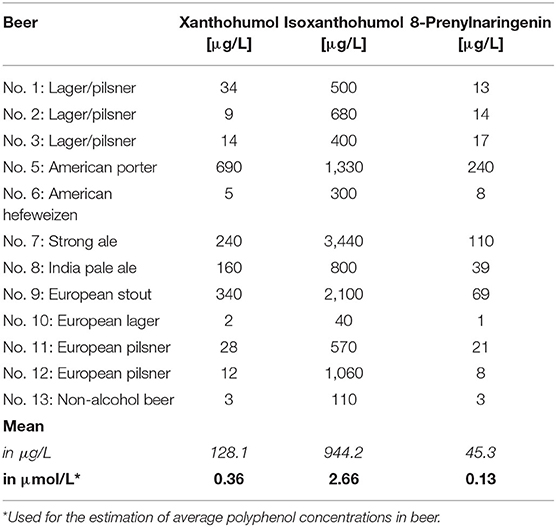
Table 2. Prenylflavonoid and -chalconoid contents in hops and beer measured by LC–MS/MS, adapted from Stevens et al. (24).
While originally planning to combine the two mixtures at a 1:1 ratio to simulate a worst-case scenario of pesticide contamination in beer, we quickly discovered that the hop polyphenol mixture at 1 beer eq. was too potent in activating our test system to be included in combinatory experiments. Thus, we followed two separate approaches to screen for combinatory effects: In an activity-based approach, the pesticide mixture and the polyphenol mixture were combined in a ratio of 1:500, ensuring an induction of ER-mediated gene expression by both mixtures in the tested concentration range. In a wider screening approach, we combined the mixtures in an array of varying concentrations.
Combination Index (CI) and Statistical Analysis
To assess potential interactions between compounds in the measured combinations, the Combination Index (CI) model of Chou and Talalay (25) was applied with the CISNE curve fitting adjustments as suggested by García-Fuente et al. (26). In brief, this method uses the mass-action law-derived median-effect equation (MDEE) to linearize dose-response curves of single compounds and combinations thereof. Subsequently, it facilitates the calculation of a parameter (the CI) which is indicative for the type and strength of a combinatory effect. In line with the suggestions by Chou (5), CI < 0.9 was considered to indicate a synergistic interaction, CI > 1.1 an antagonism and 0.9 < CI < 1.1 a nearly additive combinatory effect. To apply the model to ALP assay data, the latter were normalized to both, the solvent control and the positive control (1 nM E2, 100% effect). MDEE fitting and CI calculations were carried out using the CISNE tool (26) and MS Excel. Corresponding graphs and all further statistical calculations (normal distribution, one-way ANOVA, etc.) were carried out in Origin® 2020.
Results
Cell Viability
To ensure non-cytotoxicity and thus to exclude false reporting anti-estrogenic effects, SRB cell viability assays were carried out in parallel (Ishikawa cells, 48 h incubation) to ALP assays. The single pesticides Dic, End, Fen and Met caused cytotoxic effects at high concentrations, with the lowest observed effect doses reported in Table 3. iX lead to a loss of cellular protein at the highest applied dose of 100 μM, a concentration which was however not applied in ALP assays. Of note, no cytotoxicity was observed for the highest applied concentrations of the herbicide Glp (up to 500 μM), the polyphenol 8PN (500 nM), or the pesticide and polyphenol mixture (and the combination thereof). The non-cytotoxicity of XN in this experimental setup was previously demonstrated (12).

Table 3. Onset of cytotoxicity (lowest observed effect level) of toxic single compounds, as observed in at least three independent SRB assays.
Estrogenicity of Single Compounds
In line with previously published data (12), 8PN was found to induce ALP expression starting from as low as 0.5 nM, with an ED50 of 2.44 ± 1.60 nM (Figure 2). The structurally related iX was found to act as a considerably weaker estrogen agonist, with significant ALP induction starting from 100 nM and an ED50 of 342 ± 125 nM (Figure 2). Both reached a plateau at about 75% enzymatic activity, thus being confirmed not to fully agonize ER-mediated signaling, in contrast to 1 nM of the natural estrogen E2. XN was previously confirmed not to act estrogenic in this test system (12).
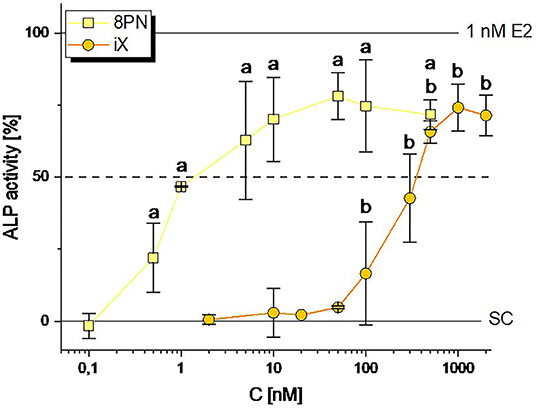
Figure 2. Induction of ALP expression by the hop polyphenols 8-prenylnaringenin (8PN) and isoxanthohumol (iX). Values were scaled to the solvent control (0; 0.1 % DMSO) and the positive control (1 nM E2, 100%) and are expressed as means ± SD of at least 4 independent experiments. Significant differences to the no-effect-level were calculated by one-way ANOVA, followed by Fisher LSD post-hoc testing, and are indicated by “a” or “b” (p < 0.05). SC, solvent control.
The pesticides End, Fen, Dic and Met were found to induce ALP expression in Ishikawa cells starting from 5, 2.5, 10, and 10 nM, respectively (Figure 3). For all of those, cytotoxic effects quenched estrogenicity at high concentrations (Table 3). Thus, only End was observed to reach the ED50 (12.3 ± 2.9 μM, calculated with the CISNE tool). Glp caused neither ER-mediated gene expression nor cytotoxicity in concentrations up to 300 μM (Figure 3). The mediation of all observed effects via ER activation was confirmed by co-incubating active concentrations of the compounds with the selective ER antagonist ICI 182,780 and monitoring the suppression of estrogenicity (Supplementary Figure 1).
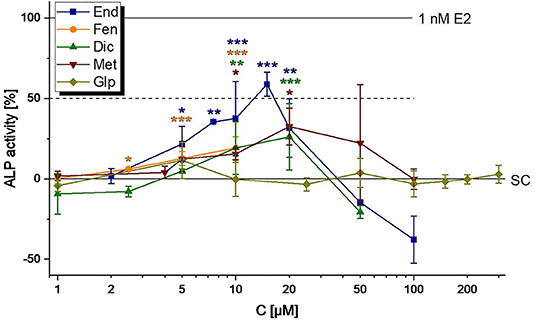
Figure 3. Estrogenicity of representative pesticides (endosulfan, End; fenarimol, FEN; dicofol, Dic; Methiocarb, Met; glyphosate, Glp), as measured in ALP assays. Values were scaled to the solvent control (0%) and the positive control (1 nM E2, 100%) and are expressed as means ± SD of at least three independent experiments. Significant differences to the solvent control (SC) were calculated by an one-way ANOVA test for each compound, followed by Fisher LSD post-hoc testing, and are indicated by “*” (p <0.05), “**” (p <0.01) or “***” (p < 0.001).
Estrogenicity of Independent Mixtures
The mixture of 8-PN, iX and XN was found to cause ER-mediated ALP expression starting at concentrations as low as 0.005 F. eq. (corresponding to 0.5% of the estimated average dose in beer), and reached a plateau with 0.1 F. eq. (Figure 4A), at about 80% of the activity caused by 1 nM of E2 in line with the testing of single compounds. A strong decline in estrogenicity was observed at concentrations above average beer doses, which could not be linked to cytotoxicity. CISNE/CI analysis revealed a nearly additive cumulative activity of 8-PN and iX at ED50 levels, with a CI50 of 0.94 (Figure 4B). Of note, the model predicted a slightly synergistic interaction in the higher effect range (~60-80% effect, Figure 4C).
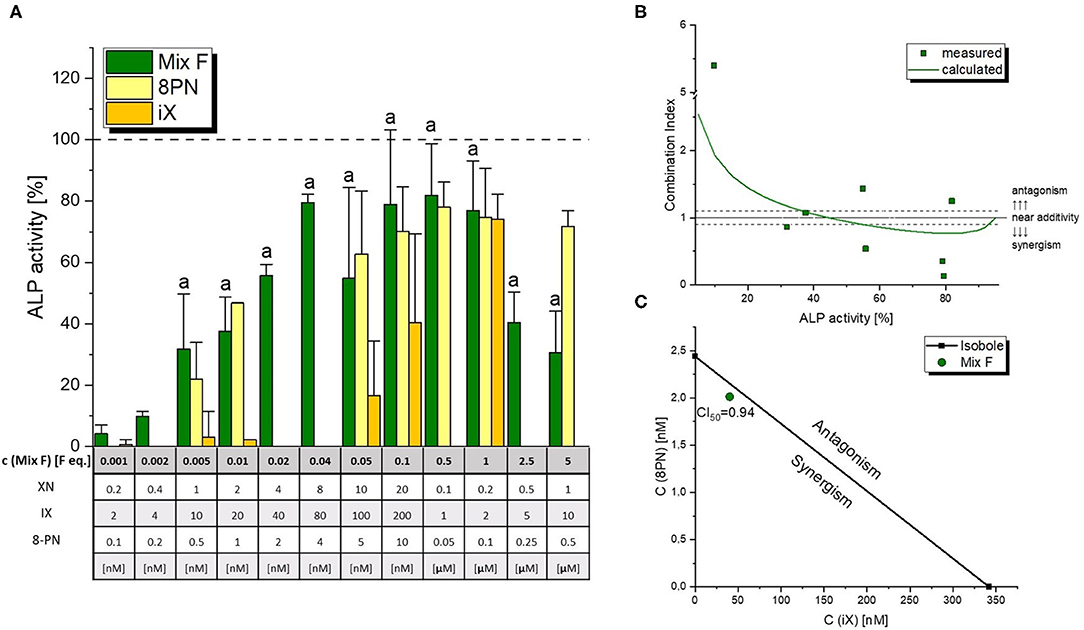
Figure 4. Estrogen receptor dependent induction of ALP expression by a polyphenol mixture. Graph (A) depicts ALP assay results for the flavonoid mixture in comparison to contained concentrations of the active constituents 8-prenylnaringenin (8PN) and isoxanthohumol (iX). Values were scaled to the solvent control (0%) and the positive control (1 nM E2, 100%) and are expressed as means ± SD of at least four independent experiments. Significant differences to the solvent control were calculated by one-way ANOVA, followed by Fisher LSD post-hoc testing, and are indicated by “a” (p < 0.05). The effect-CI plot in graph (B) shows the dependency of the combination index on estrogenicity levels, with the line indicating the calculated CI based on the whole dose-response curves and squares representing CI values calculated for actually measured data points. The isobologram in graph (C) depicts the strenght of interaction at ED50 levels.
The incubation of Ishikawa cells with a mixture of the five selected pesticides at their MRL did not lead to significant estrogenic effects in the ALP assay (Figure 5), which were however observed at higher concentrations, starting from 10 MRL eq. Of note, as most of the single compounds did not reach the ED50 activity level, CISNE/CI analysis was not applicable for this mixture.
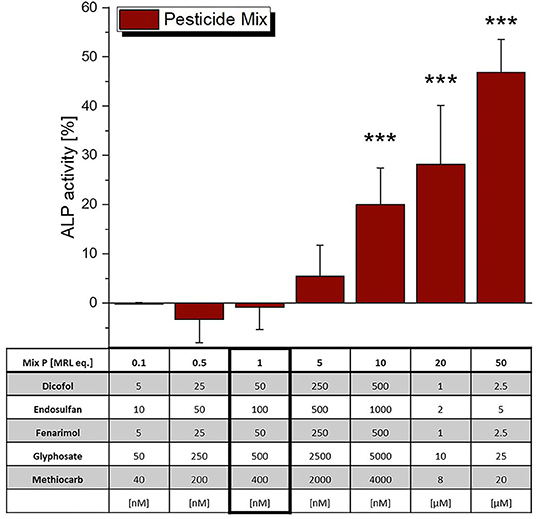
Figure 5. Estrogenicity of a mixture of selected pesticides, as measured in ALP assays. Values were scaled to the solvent control (0%) and the positive control (1 nM E2, 100%) and are expressed as means ± SD of at least 3 independent experiments. Significant differences to the solvent control were calculated by an one-way ANOVA test for each compound, followed by Fisher LSD post-hoc testing, and are indicated by “***” (p < 0.001).
Combinations of Pesticides and Hop Polyphenols
As mentioned in the dose rationale section, the pesticide and flavonoid mixture were combined in two distinct ways, an activity-based and a wider screening approach. In the former, we applied a constant concentration ratio of 0.002 Beer eq. to 1 MRL eq. Significant induction of ALP expression was observed at concentrations of 0.01/5 eq. and higher (Figure 6). Of note, the combination was slightly – albeit not significantly–more active than the flavonoid mixture even at concentrations where Mix P was still inactive. This was reflected in combination index analysis (Figure 7A), where a CI50 of 0.81 was calculated, indicating a slightly synergistic interaction of the two mixture toward ER activation. Moreover, effect-CI plotting (Figure 7B) revealed a synergistic interaction over most of the observable effect range.
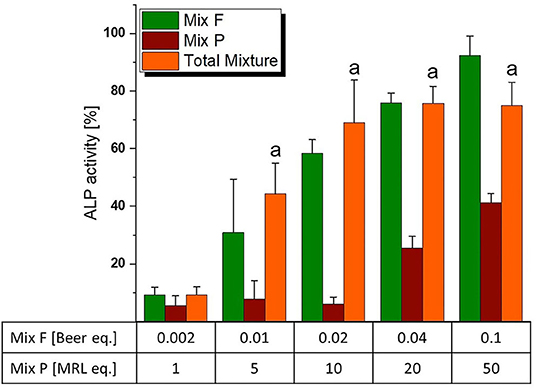
Figure 6. Estrogenicity of the total mixture (comprised of “Mix F” – hop flavonoid mixture and “Mix P” – pesticide mixture), as measured with ALP assays. Values were scaled to the solvent control (0%) and the positive control (1 nM E2, 100%) and are expressed as means ± SD of at least five independent experiments. Significant differences of the total mixture to the solvent control were calculated by one-way ANOVA, followed by Fisher LSD post-hoc testing, and are indicated by “a” (p < 0.05).
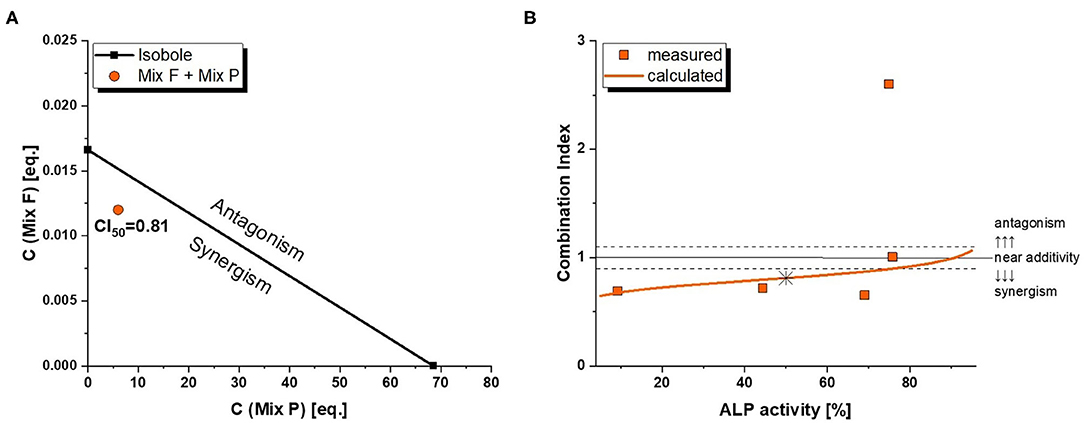
Figure 7. Combination Index (CI) analysis of total mixture estrogenicity. Graph (A) depicts the isobologram indicating a slight synergism at ED50 levels. The effect-CI plot in graph (B) depicts the course of interactive strength in dependence of effect strength.
In the screening approach, we combined the two mixtures in an array of different concentrations to investigate a potential influence of combination ratios more broadly (Figure 8). Here, no synergistic interaction was apparent, apart from a few combinatory incubations that gave higher signals than the corresponding concentration of the single mixture (e.g., the combination of 10 eq. Mix P with 0.0002 or 0.001 eq. of Mix F).
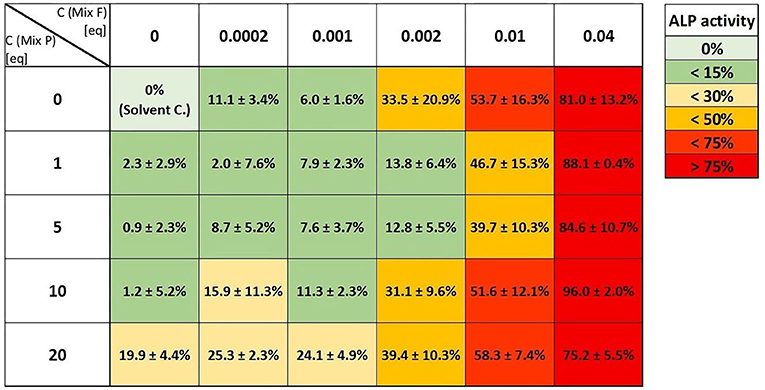
Figure 8. Heat map analysis of the screening approach to assess the combinatory estrogenic effects of combination of the flavonoid mixture (“Mix F”) and the pesticide mixture (“Mix P”) in an array of different concentrations. Values (normalized to both, solvent and positive control) are given as means ± SD of at least three independent experiments.
Discussion
Reports that constituents from hops are able to cause endocrine disruption date back to the middle ages, where German clergy added the plant to beer not only for conservation, but also to lower the libido (27). Later, menstrual disturbances of hops-harvesting women were commonly described to occur, and folk and herbal medicine use hop extracts to treat menopausal disorders. Milligan et al. (15) offered an explanation for these phenomena, as they identified the prenylated chalcone 8PN as an extremely potent phytoestrogen. Keeping all this in mind, it seems odd that the occurrence of 8PN and other estrogenic metabolites of the parent flavonoid XN in beer has not drawn more concern, particularly in a time where the hazard of xenoestrogens from man-made sources like pesticides or bisphenol A are publicly discussed. On the opposite, it seems that the scientific discourse is largely focused on potential beneficial effects of hop polyphenols, particularly their anti-oxidative properties (13) and their potential as anti-diabetic agents (28) while neglecting their potential as endocrine disruptive chemicals. This has even inspired breweries to strive to enrich prenylated chalcones in beers (14).
An often-used justification for discarding endocrine disrupting phytoestrogens in beer as a health concern is that the low bioavailability of hop polyphenols would limit systemically achieved doses to non-hazardous concentrations. At first sight, this notion seems to be supported by pharmacokinetic studies. In a human intervention study administering 20 mg of XN, a peak plasma concentration (CMax) of 33 ± 7 μg/L and an area under the curve (AUC) value of 92 ± 68 h×μg/L was reported (29), from which one could roughly estimate a low bioavailability of ~2% by assuming standard physiological parameters. However, the bioavailability of 8PN might be much higher as compared to XN, with van Breemen et al. (30) reporting an AUC of 24.3 ± 8.9 h×μg/L and a CMax of 1.4 ± 0.3 μg/L (= 3.8 nM) in humans receiving an extract from hops containing just 0.25 mg 8PN, which would corresponds to the concentration of 8PN per liter in strongly hopped beers. This might partially result from the additional formation of 8PN from the naturally co-occurring iX, the most prevalent prenylflavonoid in beer (24), within the human gut (18) and liver (31). This reaction should be regarded as a metabolic activation due to the much higher estrogenicity of the reaction product. Assuming linear kinetics, the consumption of 0.5–1 L of average-hopped beer (Table 2) could result in systemic 8PN concentrations around 0.5 nM, a dose sufficient to cause significant estrogenicity in our in vitro test system (Figure 2).
Additionally, we hereby report a cumulative to slightly synergistic activity of iX and 8PN toward the activation of ERs (Figure 4). In fact, the mixture of iX, 8PN and XN was able to significantly induce estrogenicity at a concentration of 0.005 beer eq., corresponding to a 200 times lower concentration of those compounds than estimated to occur in an average beer. At 0.04 beer eq., the ALP assay system was fully induced. These findings suggest cumulative/synergistic combinatory effects likely to occur under realistic exposure scenarios. Additional metabolites, e.g., 6-prenylnaringenin, that were not included in our study but were described to act estrogenic (16), should also be expected to cumulatively enhance the effects of 8PN as a leading compound in a similar way than demonstrated by our results. Thus, we suggest that risk assessment should avoid focusing on isolated compounds but should rather account for the simultaneous uptake of several endocrine active prenylated chalcones.
Of note, estrogenicity declined at high concentrations of the hop polyphenol mixture (Figure 4), an effect that could not be attributed to cytotoxicity (Table 3) and further confirms an anti-estrogenic activity that was previously described for XN (12, 32). As an antagonism toward the ER can also be considered a pathway of endocrine disruption, one might consider including XN in respective toxicological studies.
Another issue might arise from the proposed pharmaceutical use of hop flavonoids in the treatment of menopausal complaints (33) that could lead to the circulation of much higher doses of 8PN and related phytoestrogens as compared to nutritional exposure. Potentially adverse effects arising from prenylated flavonoids should be fully elucidated before pursuing these approaches. As reviewed by Bolton et al. (34), several intriguing biological pathways have been described to be targeted by those polyphenols, e.g., the activation of AhR receptors that is also discussed as a mechanism for potential endocrine effects of other natural compounds (35), or the activation of the Nrf2 pathway, a cellular defense system against oxidative stress. However, a complete toxicological characterization of the activities in vivo is still lacking.
On the opposite to hop flavonoids, the representative pesticides tested in this study exhibited much less estrogenic activity. At concentrations corresponding to the MRL level, none of the compounds induced the in vitro test system (Figure 3). In line with previous studies (36–38), the compounds Dic, End, Fen and Met caused estrogenicity at higher micromolar levels. Of note, Glp did not act estrogenic in Ishikawa cells up to a concentration of 300 μM, a result which is contradictory to the work of Mesnage et al. (39) who described an estrogen-like growth stimulation in MCF-7 breast cancer cells at concentrations ≥ 59 μM. This discrepancy could hint at a tissue-specific response to the herbicide, which should encourage further investigations, particularly on the in vivo situation, to clarify a potential endocrine impact of the widely-used herbicide.
The mixture of the above stated pesticides did not cause ER-mediated ALP expression at a level corresponding to the MRL (1 MRL eq.), but only at 10 times elevated concentrations (Figure 5). Thus, potential synergistic interactions that could put effective MRL levels into question were not observed, which seems to confirm their toxicological soundness.
Despite the pesticide mixture not being active at 1 MRL eq., it interacted in a slightly synergistic way with the hop flavonoid mixture (Figure 7). As humans are typically not exposed to isolated xenoestrogens, but to a mixture of vastly different chemicals from different (both natural and man-made) sources, this further underlines the importance of considering mixture effects in toxicological risk assessment. Given that the endocrine activity of hop phytoestrogens occurs at concentrations that could potentially be achieved by common nutritional exposure - the average beer consumption per capita is above 100 L/year in some regions of Europe (40) – synergistic estrogenic interactions with these compounds seem to be of high relevance and should be explored with further co-occurring substances.
Conclusion
Taken together, this study points at the possible underestimation of the endocrine activity of prenylated chalcones found in beer due to cumulative and synergistic mixture effects, and demonstrates the high potential for naturally occurring EDCs to synergistically interact with co-occurring compounds from artificial sources, a topic which should be increasingly considered in risk assessment.
Data Availability Statement
The raw data supporting the conclusions of this article will be made available by the authors, without undue reservation.
Author Contributions
GA was involved in study design, statistical analysis, supervised experiments, and wrote the manuscript. GB carried out experimental work and refined the manuscript. DM was involved in study design, supervised the project, and refined the manuscript. All authors contributed to the article and approved the submitted version.
Funding
This study was funded by the University of Vienna.
Conflict of Interest
The authors declare that the research was conducted in the absence of any commercial or financial relationships that could be construed as a potential conflict of interest.
Acknowledgments
We thank Amador Garcia Fuente (Universidad de Oviedo, Spain) for his advice on applying the CISNE tool.
Supplementary Material
The Supplementary Material for this article can be found online at: https://www.frontiersin.org/articles/10.3389/fnut.2021.691872/full#supplementary-material
References
1. Diamanti-Kandarakis E, Bourguignon JP, Giudice LC, Hauser R, Prins GS, Soto AM, et al. Endocrine-disrupting chemicals: an endocrine society scientific statement. Endocr Rev. (2009) 30:293–342. doi: 10.1210/er.2009-0002
2. Paterni I, Granchi C, Minutolo F. Risks and benefits related to alimentary exposure to xenoestrogens. Crit Rev Food Sci Nutr. (2017) 57:3384–404. doi: 10.1080/10408398.2015.1126547
3. Lallas PL. The stockholm convention on persistent organic pollutants. Am J Int'l L. (2001) 95:692–708. doi: 10.2307/2668517
4. The European Parliament and the Council of the European Union. Regulation (EC) No 1107/2009 of the European Parliament and of the Council of 21 October 2009 concerning the placing of plant protection products on the market and repealing Council Directives 79/117/EEC and 91/414/EEC (2009).
5. Chou TC. Theoretical basis, experimental design, and computerized simulation of synergism and antagonism in drug combination studies. Pharmacol Rev. (2006) 58:621–81. doi: 10.1124/pr.58.3.10
6. EFSA. Opinion of the scientific panel on plant protection products and their residues to evaluate the suitability of existing methodologies and, if appropriate, the identification of new approaches to assess cumulative and synergistic risks from pesticides to human health with a view to set MRLs for those pesticides in the frame of regulation (EC) 396/2005. EFSA J. (2008) 6:705. doi: 10.2903/j.efsa.2008.705
7. EFSA. Cumulative dietary risk characterisation of pesticides that have chronic effects on the thyroid. EFSA J. (2020) 18:e06088. doi: 10.2903/j.efsa.2020.6088
8. EFSA. Cumulative dietary risk characterisation of pesticides that have acute effects on the nervous system. EFSA J. (2020) 18:e06087. doi: 10.2903/j.efsa.2020.6087
9. Vejdovszky K, Hahn K, Braun D, Warth B, Marko D. Synergistic estrogenic effects of fusarium and alternaria mycotoxins in vitro. Arch Toxicol. (2017) 91:1447–60. doi: 10.1007/s00204-016-1795-7
10. Vejdovszky K, Schmidt V, Warth B, Marko D. Combinatory estrogenic effects between the isoflavone genistein and the mycotoxins zearalenone and alternariol in vitro. Mol Nutr Food Res. (2017) 61. doi: 10.1002/mnfr.201600526
11. Aichinger G, Pantazi F, Marko D. Combinatory estrogenic effects of bisphenol a in mixtures with alternariol and zearalenone in human endometrial cells. Tox Lett. (2020) 319:242–9. doi: 10.1016/j.toxlet.2019.10.025
12. Aichinger G, Beisl J, Marko D. The hop polyphenols xanthohumol and 8-prenyl-naringenin antagonize the estrogenic effects of fusarium mycotoxins in human endometrial cancer cells. Front Nutr. (2018) 5:85. doi: 10.3389/fnut.2018.00085
13. Stevens JF, Page JE. Xanthohumol and related prenylflavonoids from hops and beer: to your good health! Phytochemistry. (2004) 65:1317–30. doi: 10.1016/j.phytochem.2004.04.025
14. Karabín M, Jelínek L, Kinčl T, Hudcová T, Kotlíková B, Dostálek P. New approach to the production of xanthohumol-enriched beers. J Inst Brew. (2013) 119:98–102. doi: 10.1002/jib.71
15. Milligan SR, Kalita JC, Heyerick A, Rong H, De Cooman L, De Keukeleire D. Identification of a potent phytoestrogen in hops (Humulus lupulus L.) and beer. J Clin Endocrinol Metab. (1999) 84:2249–52. doi: 10.1210/jcem.84.6.5887
16. Milligan SR, Kalita JC, Pocock V, Van De Kauter V, Stevens JF, Deinzer ML, et al. The endocrine activities of 8-prenylnaringenin and related hop (Humulus lupulus L.) flavonoids. J Clin Endocrinol Metab. (2000) 85:4912–5. doi: 10.1210/jcem.85.12.7168
17. Cady N, Peterson SR, Freedman SN, Mangalam AK. Beyond metabolism: the complex interplay between dietary phytoestrogens, gut bacteria, and cells of nervous and immune systems. Front Neurol. (2020) 11:150. doi: 10.3389/fneur.2020.00150
18. Possemiers S, Bolca S, Grootaert C, Heyerick A, Decroos K, Dhooge W, et al. The prenylflavonoid isoxanthohumol from hops (Humulus lupulus L.) is activated into the potent phytoestrogen 8-Prenylnaringenin in vitro and in the human intestine. J Nutr. (2006) 136:1862–7. doi: 10.1093/jn/136.7.1862
19. Ban Z, Qin H, Mitchell AJ, Liu B, Zhang F, Weng J-K, et al. Noncatalytic chalcone isomerase-fold proteins in humulus lupulus are auxiliary components in prenylated flavonoid biosynthesis. PNAS. (2018) 115:E5223–32. doi: 10.1073/pnas.1802223115
20. Chadwick LR, Pauli GF, Farnsworth NR. The pharmacognosy of Humulus lupulus L. (hops) with an emphasis on estrogenic properties. Phytomedicine. (2006) 13:119–31. doi: 10.1016/j.phymed.2004.07.006
21. Wannenmacher J, Gastl M, Becker T. Phenolic substances in beer: structural diversity, reactive potential and relevance for brewing process and beer quality. Compr Rev Food Sci Food Saf. (2018) 17:953–88. doi: 10.1111/1541-4337.12352
22. Johnson SM, Maleki-Dizaji M, Styles JA, White INH. Ishikawa cells exhibit differential gene expression profiles in response to oestradiol or 4-hydroxytamoxifen. Endocr Relat Cancer. (2007) 14:337. doi: 10.1677/ERC-06-0085
23. Aichinger G, Kruger F, Puntscher H, Preindl K, Warth B, Marko D. Naturally occurring mixtures of alternaria toxins: anti-estrogenic and genotoxic effects in vitro. Arch Toxicol. (2019) 93:3021–31. doi: 10.1007/s00204-019-02545-z
24. Stevens JF, Taylor AW, Deinzer ML. Quantitative analysis of xanthohumol and related prenylflavonoids in hops and beer by liquid chromatography–tandem mass spectrometry. J Chromatogr A. (1999) 832:97–107. doi: 10.1016/S0021-9673(98)01001-2
25. Chou TC, Talalay P. Quantitative analysis of dose-effect relationships: the combined effects of multiple drugs or enzyme inhibitors. Adv Enzym Regul. (1984) 22:27–55. doi: 10.1016/0065-2571(84)90007-4
26. García-Fuente A, Vázquez F, Viéitez JM, García Alonso FJ, Martín JI, Ferrer J. CISNE: an accurate description of dose-effect and synergism in combination therapies. Sci Rep. (2018) 8:4964. doi: 10.1038/s41598-018-23321-6
27. Murkies AL, Wilcox G, Davis SR. Phytoestrogens. J Clin Endocrinol Metab. (1998) 83:297–303. doi: 10.1210/jcem.83.2.4577
28. Barrea L, Annunziata G, Muscogiuri G, Arnone A, Tenore GC, Colao A, et al. Could hop-derived bitter compounds improve glucose homeostasis by stimulating the secretion of GLP-1? Crit Rev Food Sci Nutr. (2019) 59:528–35. doi: 10.1080/10408398.2017.1378168
29. Legette L, Karnpracha C, Reed RL, Choi J, Bobe G, Christensen JM, et al. Human pharmacokinetics of xanthohumol, an antihyperglycemic flavonoid from hops. Mol Nutr Food Res. (2014) 58:248–55. doi: 10.1002/mnfr.201300333
30. van Breemen RB, Yuan Y, Banuvar S, Shulman LP, Qiu X, Alvarenga RF, et al. Pharmacokinetics of prenylated hop phenols in women following oral administration of a standardized extract of hops. Mol Nutr Food Res. (2014) 58:1962–9. doi: 10.1002/mnfr.201400245
31. Guo J, Nikolic D, Chadwick LR, Pauli GF, van Breemen RB. Identification of human hepatic cytochrome p450 enzymes involved in the metabolism of 8-prenylnaringenin and isoxanthohumol from hops (Humulus lupulus l.). Drug Metab Dispos. (2006) 34:1152–9. doi: 10.1124/dmd.105.008250
32. Gerhauser C, Alt A, Heiss E, Gamal-Eldeen A, Klimo K, Knauft J, et al. Cancer chemopreventive activity of xanthohumol, a natural product derived from hop. Mol Cancer Ther. (2002) 1:959–69. doi: 10.1023/A:1026082325529
33. Keiler AM, Zierau O, Kretzschmar G. Hop extracts and hop substances in treatment of menopausal complaints. Planta Med. (2013) 79:576–9. doi: 10.1055/s-0032-1328330
34. Bolton JL, Dunlap TL, Hajirahimkhan A, Mbachu O, Chen SN, Chadwick L, et al. The multiple biological targets of hops and bioactive compounds. Chem Res Tox. (2019) 32:222–33. doi: 10.1021/acs.chemrestox.8b00345
35. Hohenbichler J, Aichinger G, Rychlik M, Del Favero G, Marko D. Alternaria alternata toxins synergistically activate the aryl hydrocarbon receptor pathway in vitro. Biomolecules. (2020) 10:1018. doi: 10.3390/biom10071018
36. Andersen HR, Vinggaard AM, Rasmussen TH, Gjermandsen IM, Bonefeld-Jørgensen EC. Effects of currently used pesticides in assays for estrogenicity, androgenicity, and aromatase activity in vitro. Toxico. Appl Pharmacol. (2002) 179:1–12. doi: 10.1006/taap.2001.9347
37. Andersen HR, Bonefeld-Jørgensen EC, Nielsen F, Jarfeldt K, Jayatissa MN, Vinggaard AM. Estrogenic effects in vitro and in vivo of the fungicide fenarimol. Tox Lett. (2006) 163:142–52. doi: 10.1016/j.toxlet.2005.10.004
38. Okubo T, Yokoyama Y, Kano K, Soya Y, Kano I. Estimation of estrogenic and antiestrogenic activities of selected pesticides by mCF-7 cell proliferation assay. Arch Environ Contam Toxicol. (2004) 46:445–53. doi: 10.1007/s00244-003-3017-6
39. Mesnage R, Phedonos A, Biserni M, Arno M, Balu S, Corton JC, et al. Evaluation of estrogen receptor alpha activation by glyphosate-based herbicide constituents. Food Chem Toxicol. (2017) 108:30–42. doi: 10.1016/j.fct.2017.07.025
Keywords: endocrine disruptors, phytoestrogen, mixture effect, antioxidant, synergism, xenoestrogen, functional food, beer
Citation: Aichinger G, Bliem G and Marko D (2021) Systemically Achievable Doses of Beer Flavonoids Induce Estrogenicity in Human Endometrial Cells and Cause Synergistic Effects With Selected Pesticides. Front. Nutr. 8:691872. doi: 10.3389/fnut.2021.691872
Received: 07 April 2021; Accepted: 11 May 2021;
Published: 07 June 2021.
Edited by:
Daniel Cozzolino, University of Queensland, AustraliaReviewed by:
Shiyi Ou, Jinan University, ChinaGiuseppe Annunziata, University of Naples Federico II, Italy
Copyright © 2021 Aichinger, Bliem and Marko. This is an open-access article distributed under the terms of the Creative Commons Attribution License (CC BY). The use, distribution or reproduction in other forums is permitted, provided the original author(s) and the copyright owner(s) are credited and that the original publication in this journal is cited, in accordance with accepted academic practice. No use, distribution or reproduction is permitted which does not comply with these terms.
*Correspondence: Doris Marko, ZG9yaXMubWFya29AdW5pdmllLmFjLmF0
†ORCID: Georg Aichinger orcid.org/0000-0003-1691-7609
Doris Marko orcid.org/0000-0001-6568-2944