- 1Agricultural and Food Engineering Department, Indian Institute of Technology Kharagpur, Kharagpur, India
- 2Department of Food Engineering and Technology, Sant Longowal Institute of Engineering and Technology, Longowal, India
- 3Division of Dairy and Food Microbiology, Mansinhbhai Institute of Dairy and Food Technology, Mehsana, India
- 4Department of Life Sciences (Food Technology), Graphic Era (Deemed to Be) University, Dehradun, India
- 5Bioprocesses Research Group, Food Research Department, School of Chemistry, Universidad Autonoma de Coahuila, Unidad Saltillo, Saltillo, Mexico
In December 2019, the severe acute respiratory syndrome-related coronavirus 2 (SARS-CoV-2)—a novel coronavirus was identified which was quickly distributed to more than 100 countries around the world. There are currently no approved treatments available but only a few preventive measures are available. Among them, maintaining strong immunity through the intake of functional foods is a sustainable solution to resist the virus attack. For this, bioactive compounds (BACs) are delivered safely inside the body through encapsulated food items. Encapsulated food products have benefits such as high stability and bioavailability, sustained release of functional compounds; inhibit the undesired interaction, and high antimicrobial and antioxidant activity. Several BACs such as ω-3 fatty acid, curcumin, vitamins, essential oils, antimicrobials, and probiotic bacteria can be encapsulated which exhibit immunological activity through different mechanisms. These encapsulated compounds can be recommended for use by various researchers, scientists, and industrial peoples to develop functional foods that can improve immunity to withstand the coronavirus disease 2019 (COVID-19) outbreak in the future. Encapsulated BACs, upon incorporation into food, offer increased functionality and facilitate their potential use as an immunity booster. This review paper aims to target various encapsulated food products and their role in improving the immunity system. The bioactive components like antioxidants, minerals, vitamins, polyphenols, omega (ω)-3 fatty acids, lycopene, probiotics, etc. which boost the immunity and may be a potential measure to prevent COVID-19 outbreak were comprehensively discussed. This article also highlights the potential mechanisms; a BAC undergoes, to improve the immune system.
Introduction
The increased understanding of the close relationship between food and human health in developed societies has dramatically altered food choice, leading consumers to select one particular foodstuff over another to achieve certain desired health effects (1–3). In this context, functional foods are excellent food choices because they seek to enhance the quality of life by preventing diseases associated with diet (3). Functional foods may be described as foods that offer health benefits beyond their nutritional value such as probiotics, prebiotic compounds, dietary fibers, vitamins, essential oils, etc. As per the CLYMBOL project (the first European Commission-funded cross-country analysis to investigate the positions of public health claims and symbols and to compare food and beverage claims status quo in Europe), these foods that have health associated claims are considered to be marginally safer than other foods without any message (2–4).
The food industry and consumers are very involved in the use of functional food as they are believed to improve human health (5). The global functioning food market in 2018 was estimated at USD 161.49 billion and is expected for the next 5 years to rise at a cumulative annual growth rate of 7.9% (6). But the lack of absorption of functional foods limits its health benefits. Water solubility and low stability are mostly attributed to poor absorption in the human gastrointestinal (GI) tract (2). Functional food ingredients must pass through the human GI tract and absorb enteric epithelial cells as they are supplied orally. Low water solubility in the human lumen contributes to low dispersion, resulting in limiting absorption. The extremely low pH (~2.0), on the other hand, of gastric fluid and digestion enzyme can degrade functional food ingredients in the human stomach (7–9). Consequently, many usable food products have very low bioavailability. The carriers based on nanoparticles are therefore very promising to provide bioactive compounds (BACs), as their nanoscale improves enteric epithelial cell absorption (6, 10). A complete pathway and mechanism for the delivery of BACs in intestinal epithelial cells have been shown in Figure 1. The passive transcellular route, paracellular pathway, and endocytosis can be capable of absorbing BAC-loaded nanoparticles (12, 13). Thus, the bioavailability of functionally produced foodstuffs and the benefiting effect of the encapsulating BAC have therefore been expected to increase substantially.
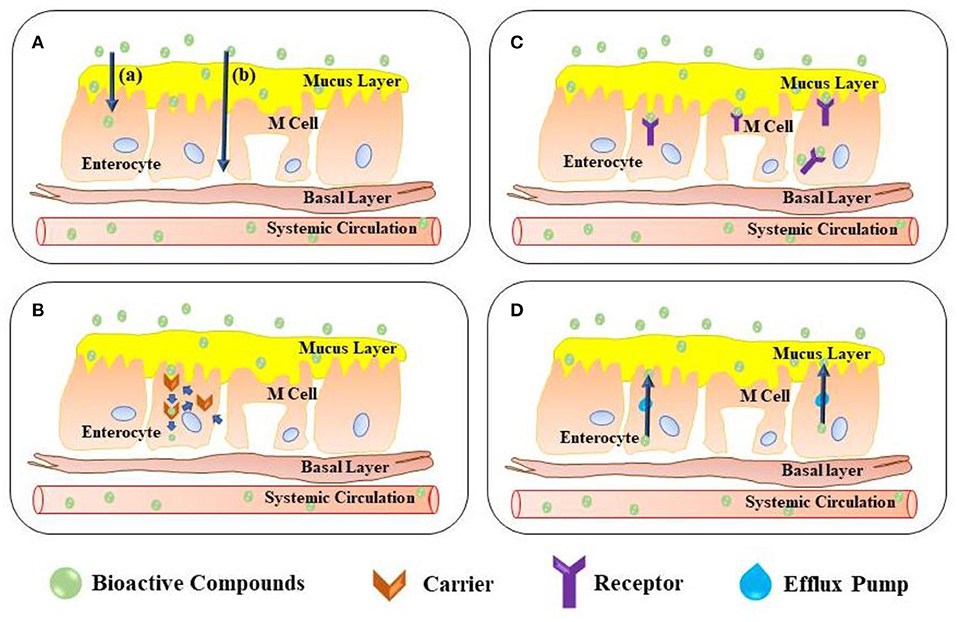
Figure 1. Transport mechanisms for bioactive components through intestinal absorption cells. (A) Passive diffusion in transport mechanisms provides an energy-independent route due to osmotic pressure, either by paracellular or transcellular pathway (11). Both pathways defining the mechanism, (a) the pathway of transcellular diffusion in a process where small hydrophobic molecules combine and are then transported into the membrane, while (b) another pathway of paracellular diffusion happens when the junctions of the intestinal epithelium cells are carried by small hydrophilic molecules; (B) Transportation mediated by carrier permits the introduction or expulsion of BACs from cells due to osmotic pressure gradients used by cellular protein transporters. These carriers include energy-dependent active transportation and easier diffusion; (C) Transportation mediated by the receptor, in which BACs are directly bound and internalized by cells to cell-surface receptors; and (D) In the mechanisms of efflux pumps, the most important ATP-based efflux pump of which is a p-glycoprotein (p-GlyP) in the intestinal epithelium cells. The use of a p-GlyP pathway to pump out BACs from enterocytes, leading to limited biological supply. This process has been reported to specifically affect the poor bioavailability of polyphenols.
The severe acute respiratory syndrome-related coronavirus 2 (SARS-CoV-2), a new coronavirus appeared in December 2019 and spread rapidly through more than 100 countries worldwide triggering an international outbreak of serious acute respiratory syndrome. On 21 January 2020, coronavirus disease 2019 (COVID-19) was treated by the Chinese Government as infectious diseases of class B and prevention and control as infectious diseases of class A. On 11 February 2020, the World Health Organization (WHO) named the novel coronavirus pneumonia COVID-19. In early August 2020, there were more than 20 million COVID-19 confirmed cases worldwide and more than 728,000 deaths (14). This isn't the first time that coronavirus is causing a major infectious disease. The Coronaviridae family comprised both SARS-CoV in 2003 and the Middle East respiratory syndrome-related coronavirus (MERS-CoV) in 2012 (15). Because of the infectivity of SARS-CoV, SARS-CoV-2, and MERS-CoV, a high fatality rate, and lack of advanced medicine, every single outbreak of coronavirus has been a major burden to society (16). This pandemic of COVID-19 posed several questions about how we can improve the body's immunity through food. SARS- CoV-2 is associated with the invasion and pathogenesis of the host immune system. The first line of defense against invasion is inherent immunity. In innate immunity, mammalian viral infection activates intracellular recognition pattern receptors that sense pathogens of molecular patterns, such as two-strand ribonucleic acid (RNA) or uncapped messenger RNA (mRNA) (17). Several approaches for immunotherapy were previously used in patients with COVID-19 to treat or avoid virus infection (18). Such procedures have been introduced with varying efficiencies in COVID-19, including plasma-recovery therapy, IL-6 monoclonal antibodies, and the C5 protein supplement, cytokine therapy, mesenchymal stem cell therapy, and intravenous immunoglobular disease (15, 19, 20). The virus's interaction with the immune system mediators triggers an immune response that can decide the viral infection outcome (21).
Recent research has shown that dietary supplementation may be effective in patients with COVID-19. Higher than the daily doses of recommended nutritional components such as vitamins (C, D, and E), zinc (Zn), and omega (ω)-3 fatty acids may benefit from reducing the infection rate of SARS-CoV-2 and hospitalization time (22–24). The antioxidant and immunomodulant effects of these nutrients are well-known. Such nutrient deficiencies may lead to immune dysfunction and increase susceptibility to pathological infection. We also analyzed some recently published reports and found that the published reports have described a lack of such nutrition (especially vitamins and minerals) in a diet that makes a significant contribution to the human immune system and therefore undermines immunity. As a result, it may develop morbidity and mortality for a group of elderly people (such as COVID-19 patients) who are at high risk (25–27). However, the elderly are considered to be more likely to be nutrient deficient and less immune, which increases their likelihood of bad COVID-19 results significantly, making proper nutrition double as critical (24). Further, it is essential to take protective measures and keep oneself secure and safe before obtaining a cure and novel coronavirus vaccine. This needs a range of functional food products that boost the immune system and assist in the reduction and alleviation of mortality rates in COVID-19 patients.
Considering such viewpoints, this review explores the importance of encapsulated food products to boost immunity and reports studies about improvement in human health due to encapsulated BACs in the past decades. The present work discusses the overview of existing functional components in different food products and how they can play a role against COVID 19. It also deals with novel encapsulated BACs that have the potential to introduce in food systems to impart immunological activity and develop potential functional foods. Such compounds promote an active immune system and can provide protection against COVID 19. This manuscript is, therefore, an attempt to review the potential of encapsulated functional components to fight against COVID-19 which may support researchers and scientists to develop novel food-based formulation as a preventive measure for COVID-19.
Human Immunity System, COVID-19 and Functional Foods
Our entire body contains the immune system organ for the defense of disease. Health and pathogenesis have a key role to play. It also protects our bodies from infectious chemicals, germs, and cell changes (neoplasm). White blood cells (WBCs) will pass through the blood vesicles around the body and are the main players in the immune system (28). Our body shares blood and lymph vessels with cells and liquids and helps the lymph system to check for microbial invasion (29). Lymph is stored in the lymph vessels. Specialized compartments for each lymph node can be found in which antigens may be present. The immune cells and foreign particles enter the lymph nodes through the inbound lymphatic vessels (28). They are processed into tissues in the body while they are in the bloodstream. They start throughout the process by targeting foreign antigens and then heading back slowly to the lymphatic system. In the lymph nodes and spleen cells, the immune cells absorb, function, and help to counter antigens (28).
COVID-19 is an approximately 60–140 nm diameter crown-like RNA virus (30). It is spread by coughing and sneezing through respiratory droplets. It reaches our nasal system by inhalation and begins replicating (31). The principal COVID-19 receptor is angiotensin-converting enzyme-2 (ACE2). In the host cell, the spike protein located on the COVID-19 surface is pinched within the ACE2 receiver binding (18). Then, the virus spreads and enters the respiratory tract from the airway. There is the immune response faces a ruggeder innate. The disease is clinically expressed at this level and cytokine may be an unconscious reaction to the subsequent clinical path (32). For 80% of infected patients, the disease is mild and is primarily limited to the upper and leading airways (33). Such individuals can be monitored and controlled at home using conservative symptomatic therapy. Roughly 20 percent of infected patients experience lung infiltration, some of which are very severe (34). Most COVD-19 patients were elderly patients with essential diseases in the extreme category (35, 36). The extreme category was more likely than the moderate to have obstructive pulmonary disorders, asthma, malignant tumor, coronary artery disease, and chronic kidney disease (16, 25, 37). Of those 145 critical patients, 51 were killed; representing 34.69 and 90.2% were dead over the age of 60. Of 51 deaths, 40 patients (78.43%) have a specific illness. Recent studies show patients over 60 years of age with comorbidities, especially hypertension, as risk factors for serious diseases and death of infection with SARS-CoV-2 (16, 36, 37).
The innate immunity mechanism of the human body helps to protect against infectious agents including viruses, bacteria, fungi, protozoa, and worms that resist infections (16, 17). In this scenario, as with other viral infections, our body's immune system can provide us with the best defense against coronavirus since there is no registered COVID-19 medication. We can't note infections like COVID-19 as long as the immune system is strong or active with regard to the development of antibodies and specific immune mechanisms to fight against a specific infectious agent (18). It can be divided into three groups of our immune systems which include (1) innate (or natural) immunity, (2) passive immunity, and (3) adaptive (or active) immunity (as depicted in Figure 2). We are all born with inherent immunity, which serves as a form of general defense. The skin, for example, serves as a shield to prevent black germs from penetrating the body. Two forms of passive immunity are again normal, that is, one in which we obtain artificial immunity from our mother and the second we obtained from drugs or food. The third type, adaptive immunity develops throughout our lives, that is, when we're exposed to diseases or when we're immunized against them with vaccines we develop adaptive immunity. When our body is affected, it starts with skin and inflammatory reactions (21, 22, 28). But the immune system cannot act properly when our body experiences viruses or germs for the first time, and we get sick (16–18). In the case of COVID-19, the same thing happened.
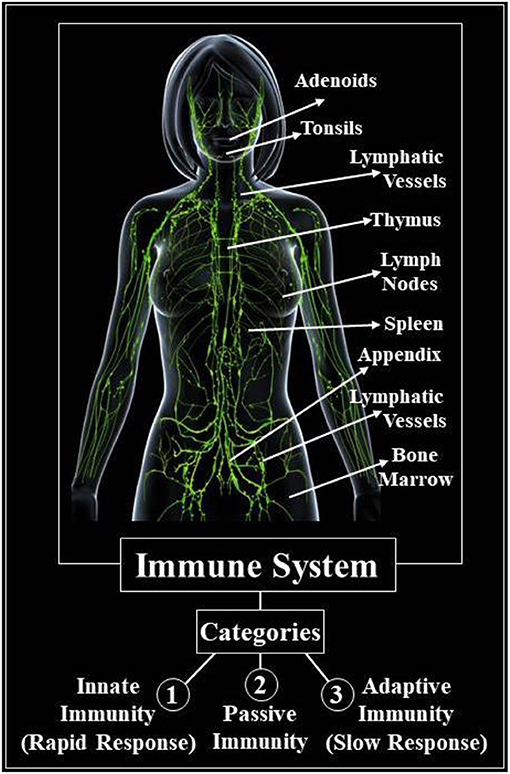
Figure 2. Different categories of the human immune system (28).
Recently, the protective phase that is further divided into two phases in immune defense has been identified as important for COVID-19 treatment and its damaging phase division due to inflammation (38). The damaging phase means when the virus attempts to infiltrate the body's tissues and organs, resulting in inflammation of the particular organ. In the first protective phase, doctors will try to increase immune response and suppress it in the second phase. The improvement in immunity can be accomplished with nutrition supplements containing encapsulated BACs in patients (Table 1) during mild corona infections or by routine intaking of these usable nutrients (25, 35, 39). The WHO has recommended in this regard that balanced foods and hydration are important. People who eat a healthy diet have a good immune system and are at reduced risk of chronic disease and infectious diseases (82). Vitamin and minerals are of essential importance. For example, vitamin-B protects against infection while vitamin-C protects us from signs of flu. In the case of coronavirus infection, it has been observed that the incorporation of half tea spoon turmeric (curcumin) powder in hot milk cures sour throat and boosts immunity, and hence it is also recommended by The Ministry of AYUSH. Similarly, use of various herbs like Tulsi (Basil), Kalimirch (Black pepper), Dalchini (Cinnamon), Giloy (Tinospora cordifolia), and Shunthi (Dry Ginger) in hot water as kadha as well as lemon juice (a rich source of vitamin-C) had also shown to improve the immunity against COVID-19 virus (83–86). The active compounds from such foods can be extracted, encapsulated, and used to treat COVID-19 (24, 66, 87). However, currently, all these work is at a nascent stage and it may further demand proper systematic approaches to prove the positive effects on the immune system. Vitamin-D or vitamin-E deficiency may contribute to coronavirus infection. But we can get these vitamins from their natural sources to overcome the problem (24, 88). For example, sunlight can provide vitamin-D, while oil, seeds, and fruits can provide vitamin-E. Failure to produce iron (Fe) and excess of Fe may lead to risk (89). While, the maintenance of our immune system requires Zn (24, 90). Protein-rich food should be at the forefront because the food has immune properties (production of immunoglobulin) and possible antiviral activity. Therefore, whole cereal grains, legumes, vegetables, fruits, nuts, and animal food should be consumed on daily meals. However, these biologically active compounds are very sensitive to the low pH of the belly and are not of great health benefit to our body because of their poor bioavailability in our GI tract (11, 91, 92). As a result, the encapsulation of a biological compound with immunological activity increases the safety of BACs in low pH conditions and increases their bioavailability in the GI tract. Figure 3 showed the effect of the pH of the mouth, stomach, and intestine on the encapsulated particle and described how the encapsulated particle of the BAC can protect at lower pH of the stomach (11). The use of these encapsulated compounds in the preparation of functional food is a novel method for improving the functionality of food as well as for enhancing human immunity to various microorganisms, such as the novel coronavirus. According to a recent survey of the Food Safety and Standards Authority of India (FSSAI), sales of immunity-boosting foods have increased by about 20–40% since the country went into lockdown due to the COVID-19 pandemic (93).
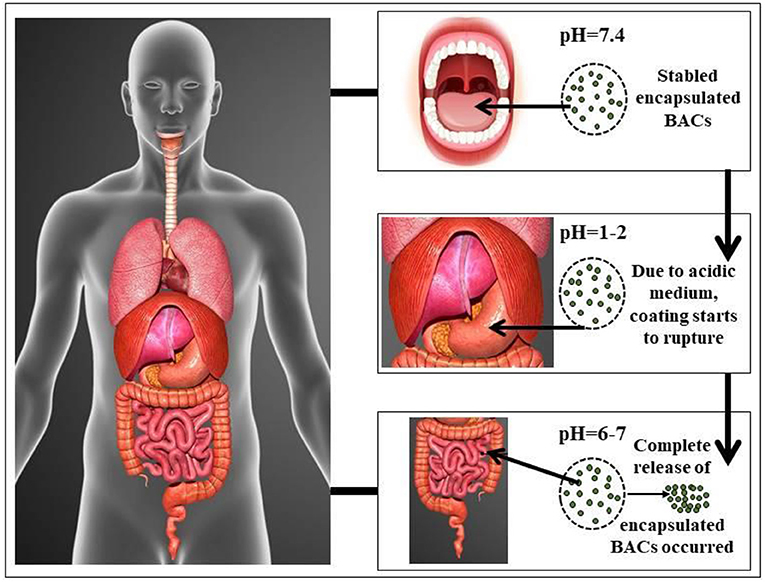
Figure 3. Effect of different pH on the encapsulated bioactive compounds (BACs) in different parts of the human body (11).
Encapsulated Food Products as a Potent Immunity Booster
The demand of consumers for healthier natural food products has increased in recent years and has shown scientific effectiveness in finding nutrients in alternative food sources (1, 3, 94). Inadequate nutrition, caused by eating, led to a widespread incidence of diseases such as obesity, cancer, cardiovascular disorders, or immunological diseases. This created an ideology of the modern consumer who wants food that has the ability to protect your health and avoid disease (95). The food industry is therefore centered on the production of functional foods with natural compounds that provide health benefits, such as antioxidants, anti-inflammatory activity, or immunological behavior. A schematic diagram for developing various functional foods is illustrated in Figure 4 that describes the encapsulation of the immunologically active BACs. These functional foods are known as nutraceuticals as such foods can help to cure diseases caused by imbalanced feeding or avoid them. The word “nutraceutical” is a nutrient-pharmaceutical fusion, first introduced in 1989. It is considered a good diet capable of preventing illness which removes the obstacle between food and medicines and which clearly demonstrates that food of this sort provides an incredible potential to enhance human health (2, 3, 95).
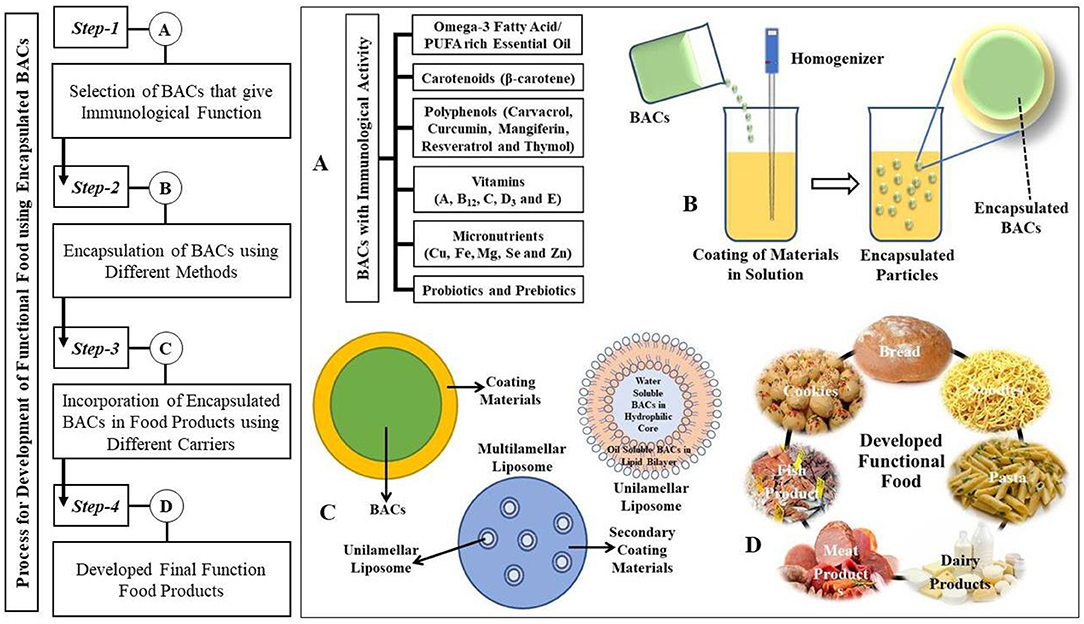
Figure 4. A general flow schematic diagram for the development of functional food using encapsulated bioactive compounds (BACs) of immunological activity (96). (A) First, food-grade BACs that are supposed to substantially increase immunological function are chosen. (B) Second, nanoparticle-based carriers are used to encapsulate the BACs since they have shown promise in delivering BACs. Since functional food ingredients are delivered orally, they pass through the human gastrointestinal (GI) tract and are absorbed by enteric epithelial cells, which is why nanoparticle-based carriers are used to deliver BACs. However, low water solubility in human lumen fluid, results in low dispersal, reducing absorption. On the other hand, certain functional food additives may be degraded by an extremely low pH (~2.0) of gastric fluid and digestion enzymes in the human stomach. (C) Following that, these encapsulated BACs are incorporated into food formulations through various carriers to develop formulated functional food. (D) Thus, by using these evolved final encapsulated functional foods, we may be able to improve the immunity of the human body against the novel Covid-19 virus.
Cereal-Based Products
Bioactive compound encapsulation retains the compound's structure and functional quality and has a limited effect on the organoleptic perception of food products, including bread in food matrix form (97, 98). The encapsulated material and the BAC contain the capsular wall material of the sample matrices, which provides a barrier between the BAC and the bread dough (98). The encapsulating cell wall materials typically are starch, starch derivatives, lipids, proteins, gums, and all combinations thereof. Spraying and freeze-drying techniques for encapsulation of BACs for bread making are the most common and relatively simple (97). Table 2 shows the wall materials used to encapsulate the functional ingredients used in the formulation of bread. These materials have been used by many people for the favorable effects of bread quality.
Different compounds such as ω-3 fatty acid, n-3 polyunsaturated fatty acids (PUFAs), curcumin, and probiotic bacteria have been encapsulated and used for the production of cereal-based functional and/or fortified food (Table 3A). Omega (ω)-3 fatty acids, however, are a class of long-chain acids that have demonstrated various beneficial health effects (Figure 5) (157, 158). Omega (ω)-3 fatty acids have recently been encapsulated in soybean phospholipids by liposome technique in order to mask the flavor of fish and used in the making of bread to provide ω-3 fatty acids to the health beneficiary (104, 105). Baking time and temperature were ranged between 13–26 min and 200–260°C, respectively. The sensory assessment showed that enriched bread had a higher amount of nano-liposomal ω-3 fatty acids than other samples. Additions of nano-liposomal ω-3 fatty acids had no detrimental effects on the texture property and sensory acceptability of bread (104, 105). Omega (ω)-3 fatty acids have helped to increase the immunity of the human body by affecting T-cells, macrophages, and neutrophils of the human body (40). T-cells are lymphocytes originating from the thymus that recognize the T-cell receptor (TCR) presented antigens. The association of a TCR with antigen-presenting cells (APCs) such as macrophage or dendritic cells (DCs) is the first time and the T-cells are activated. Alterations in APCs activation using ω-3 fatty acids are also the first pathways for modulating T-cell activation in-vivo through the use of ω-3 fatty acids (160). The principal adaptive branch lymphocytes of the immune response are B-cells, along with T-cells. As part of the innate immune system, macrophages play an important role. Omega (ω)-3 fatty acids induce important changes to macrophage gene regulation. The treatment of docosahexaenoic acid (DHA) or eicosapentaenoic acid (EPA) macrophages results in significant changes in the gene expression of lipopolysaccharide (LPS)-activated macrophages derived from T-helper type (THP) 1 (159). However, DHA and EPA do not have the same results. In addition to the cell culture medium for macrophages, ω-3 fatty acids (DHA and EPA) induce essential global changes in the microRNA (miRNA) profile. Neutrophils are the first cells in which inflammation occurs and play a significant role in removing pathogens (161). Neutrophils can communicate with the adaptive immune system, however, through the incorporation of naïve T-cells into THP-1 cells, and can have B-cell antigens in the spleen. After absorption of the ω-3 fatty acids into the phospholipids, they may be metabolized by neutrophils into leukotrienes, maresins, prostaglandins, protectins, resolvins, and thromboxanes (40). The neutrophil role of ω-3 fatty acids and their metabolites is modulated in many ways, they are as follows: phagocytic capacity, cytokines, neutrophil migration, and reactive oxygen species (ROS) production. Omega (ω)-3 fatty acids successfully integrate and control all immune cells into the cell membrane by in vitro stimulation or dietary supplementation (40). Successful encapsulation of ω-3 fatty acids has been described in various reports on the development of different food products, such as breads (103), cookies (106, 107) and pasta (114). As ω-3 fatty acid has an immunity-enhancing function, these food items based on cereals can be eaten to improve the body's immunity to foreign compounds that can help to fight against the novel coronavirus (24).
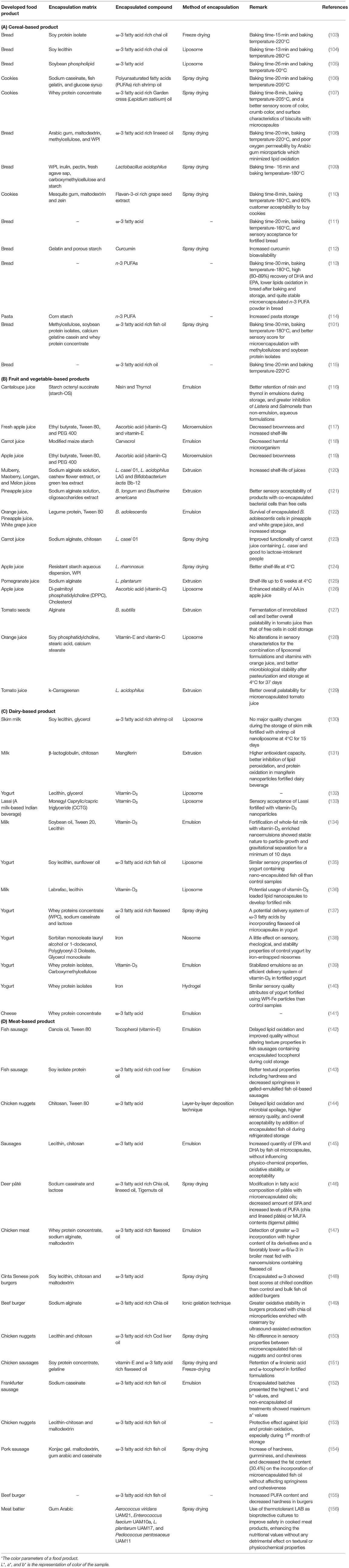
Table 3. Food product developed by the encapsulation of bioactive compounds (BACs) containing immunological activity.
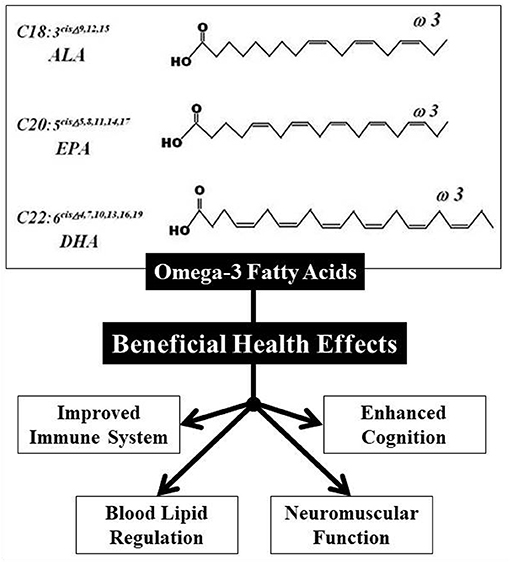
Figure 5. Different health benefits of omega (ω)-3 fatty acids in the human immune system (157–159).
Besides ω-3 fatty acids, the probiotic microorganisms are being encapsulated for developing novel functional foods with improved functionalities (162, 163). Recently, Muzzafar and Sharma (164) developed the biscuits containing microencapsulated probiotics whereas functional bread was prepared by Seyedain-Ardabili et al. (165) which contained encapsulated strains of Lactobacillus casei 431 and L. acidophilus LA-5. Bacteria found in the intestines help to digest food and suppress harmful bacteria, increase nutrition, and promote immunity. Several studies have shown that probiotics activate innate and acquired immune responses by stimulating immunoglobulin A (IgA) secretion, encouraging phagocytosis, altering T-cell reactions, and preserving THP-1 and THP-2 homeostasis by improving THP-1 responses and attenuating Th2 responses (166, 167). The mechanism of improving immunity by probiotics in the human host is complex and not thoroughly explained. However, it is assumed that probiotics will facilitate the production of bacteriocins and short-chain fatty acids, reduce the intestinal pH, provide colon nutrients, colonize and participate in binding sites of intestinal epithelial cells, promote mucosal barrier function, and modulate the immune system (168, 169). Microencapsulation is necessary to enhance process efficiency, solubility, dispersibility, flowability, and shelf-life by preventing certain reactions (oxidation, dehydration), improves process protection and convenience, and protecting unstable sensitive compounds (108, 170, 171).
Therefore, it can be suggested to use nano-liposomal ω-3 fatty acids as well as encapsulated probiotics for the enhancement of the nutritional value of bread, biscuits, and other bakery items. Such encapsulated bakery products are the need of the hour to promote the immune system of the human body because the process of encapsulation enhances the viability and thermal resistance of probiotic bacteria and other heat-sensitive compounds.
Fruit and Vegetable-Based Products
The worldwide consumption of fruit and vegetable juices is widespread. There is a continued increase in world demand for high-quality fresh juices; in particular, as there is a growing awareness that healthy fluids and juices are included in the human diet instead of sweetened beverages and soft drinks. Fruit and vegetable juices are naturally rich in BACs that are major contributors to human nutrition and have health-promoting and disease prevention properties (172). These natural BACs offer a wide range of health benefits to consumers, including bone-protection, skin-protection, cardiovascular disease, normal blood pressure maintenance, nervous system, weight loss, etc. (173–175). However, no evidence has been found that fruit and vegetable juices have been immunologically active so far. The use of natural additives to enhance the immunological activity of fruit and vegetable juices has therefore been extensively made. Antimicrobial products such as essential oils and their components as well as vitamins and probiotics have been reported as natural additives for juice (Table 3B).
Vitamin-C is a water-soluble vitamin (also known as ascorbic acid) that cannot be synthesized in the human body. Vitamin-C is well-known to have protective value in infectious diseases. It is an essential co-factor to form the cartilage, blood vessels, muscle, and collagen in bone and is important for the healing process (176). As an anti-oxidant, vitamin-C protects the cells from damage by free-radicals and contributes to the prevention of stroke, cancer, and other diseases. In reality, supplements are known to aid mechanisms for respiratory defense, prevent virus infections, and reduce their duration and severity, as well as improve flu-like symptoms with anti-histamine properties. It is therefore an important element in COVID-19 because it has the main respiratory defense function (24). A recent study showed an increased high sensitivity C-reactive protein (hs-CRP), a marker of inflammation, and oxidative stress in 29 patients with COVID-19 pneumonia. Transcription factor, nuclear factor erythroid 2 (nfe2)-related factor 2 (Nrf2), is a major regulator of antioxidant response element (ARE)-driven cytoprotective protein expression (177). Activation of Nrf2 signaling is vital for preventing the cells and tissues from injury induced by oxidative stress. Vitamin-C is an essential part of the cellular antioxidant system. Cytokine storm rises as the disease progresses during COVID-19 infection, and vitamin-C against this has been suggested (23, 57). The levels of the pro-inflammatory cytokines, including tumor necrosis factor-α (TNF-α), are known to be reduced and anti-inflammatory cytokines (IL-10) increase (178). After 5 days of production of acute respiratory distress syndrome (ARDS), a study recently published by Khan et al. (23), which describes a patient treated with high-dose vitamin-C, was removed from ventilation and this was exceptionally early. However, they have obtained anti-viral drugs which should be noted (23). The preventive role of vitamin-C assists in scavenging activity of dead cell, vitamin-E regeneration, normal neutrophils function, activating signaling cascade and proinflammatory transcription factor NFκB (nuclear factor kappa B), modulating the signaling pathways, regulating inflammatory mediators, phagocytosis, gene regulation, and signaling pathways in T-cells, and improves the neutrophils motility to reach the infection site. Using such mechanisms, vitamin-C exhibits a promising role in immune modulation and proper immune functions (179–182). Epidemiological studies have also been indicated that vitamin-E deficiencies affect immune responses and infectious diseases (35, 183). Vitamin-E is used to increase the number of T cells, increase lymphocyte mitogenic reactions, increase the secretion of IL-2 cytokine, boost NK cell function and reduce the risk of infection (35, 183). It also shows the suppressing effect on the generation of prostaglandin E2, a T cell-suppressing lipid mediator, which increases with aging. Vitamin-E-induced improvement in the immune system is of important therapeutic effect, as is seen in the results of improved respiratory resistance in both older mice and older adults (183, 184). Vitamin-C and vitamin-E can be supplied to the body using fruit juice (particularly fortified apple juice) that has been prepared by Tao et al. (117). By adding encapsulated vitamin-C (0.05%) and vitamin-E (0.05%) to the juice, the authors created the enhanced apple juice. Vitamin encapsulation was performed in microemulsion methods Ethyl butyrate, Tween 80, and PEG 400. Adding vitamins also decreased the browning and the acceptability of apple juice (117). The frequent use of high vitamin-C and vitamin-E apple juice will improve our body's immunity to viral infections such as COVID-19.
A potential intervention to improve outcomes, particularly for six ventilation-associated pneumonia, probiotics have been recommended in COVID-19-infected patients, in addition to vitamins (185). Lactobacilli spp. (120, 123, 124), Eleutherine spp. (121), and Bifidobacteria spp. (120–122) are the most commonly used probiotics in functional and other fermented foods (162). Thus, there is increasing interest in the development of fruit-juice-based probiotic products (186, 187). Fresh fruits and vegetables are known to be healthy matrices that may be suitable substrates for probiotics because of their antioxidants, fibers, minerals, and vitamins and their existence does not involve any dairy allergens which can prohibit their use by unique segments of the population (188). Fruit juices and beverages have an excellent flavor for all ages and are considered to be safe and healthy. A variety of fruit and vegetables like apples, banana, blackcurrant, oranges, blueberry, cashew apple, pineapple, raspberry, pomegranate juice, cantaloupe melon, pineapple, etc. and mixed vegetable juice are explored for the development of probiotic-containing juices (77, 186, 189–191).
It is well-known that probiotics improve the immunity of humans through the defense against GI pathogens. The mechanisms for action utilizing antimicrobial secretion, competitive exclusion from sites of adhesion and nutritional sources, improving the role of the intestinal barrier, and immunomodulation are thus used as beneficial effects on the host (167). Moreover, probiotic microbes produce microcin that works for cell entry by binding Fe-siderophore receptors or releases harmful materials after entry into the cell. These mechanisms contribute to the inhibition of intracellular enzymes like adenosine triphosphate (ATP) synthase, RNA polymerase, and deoxyribonucleic acid (DNA) gyrase, and their functions including translation into the mRNA and then induce the death of pathogenic cells (94). Several fruit juices were prepared by Wang et al. (122) such as white grape juice, pineapple juice, and orange juice, and fortified with encapsulated probiotic bacteria, Bifidobacterium adolescentis. The cells of B. adolescentis (ATCC 15703) were caught with protein-alginate capsules of faba, lentil, soy, and pea and subject to synthetic intestinal fluids (SIF, pH 6.5/37 °C) and gastric juice (SGJ pH 2.5/37°C). In cell counts 5.1, 5.5, 3.3, and 1.9 log cells, respectively, were B. adolescentis cells captured in faba, lentil, soy, and pea protein-alginate capsules after 2 h. Releases of the encapsulated cells of B. adolescentis in SIF over 3 h suggested that almost all cells, regardless of their wall content, were released within the first 10 min. In the pineapple and White grape juice, encapsulated B. adolescentis cells survived, but not in orange juice. Moreover, these fruit juices can be used as immunity boosters to avoid viral diseases such as COVID-19 (122). Recently, Mostafa et al. (192) developed the date juice containing L. sakei and L. acidophilus and examined the anti-proliferative activity against Hep-2 and Caco-2 cell lines. Total polyphenolic content and antioxidant activity were increased during storage in juice containing L. sakie strains. Probiotic date juice showed an anti-tumor response against the larynx Hep-2 cell linen with no response against Caco-2 cell linen (192).
Attributed to the biochemical composition, fruits and vegetables offer great advantages for improving the immune system and prevent several kinds of diseases. The encapsulation of vitamin-C and vitamin-E as well as probiotic strains not only improves the nutritional value of fruits and vegetables-based products but also boosts our immunity. Therefore, the intake of such products may protect the human body from being infected by the coronavirus. However, the blind use of traditional probiotics for COVID-19 shall not be suggested before we have a clearer idea of the pathogenesis of SARS-CoV-2 and its impact on gut microbiota.
Dairy-Based Products
A variety of functional and nutritious products from vitamin, mineral, and bioactive fortification to promote health benefits have been supplied with dairy products as the most common deliverables (131). As milk and dairy products are part of a regular daily diet, it is expected that any new product launched will gain market share (131). There are many studies in this link on various dairy products, such as milk (136, 193, 194), yogurt (195) and cheese (196). These dairy products have been fortified with different compounds to improve versatility and provide nutrients to human health. None of them, however, concentrated on its use as an immunity booster because they had fortified dairy products with some compounds of immunological activity.
Various compounds with immunological activity, such as ω-3 fatty acids, vitamin-D, probiotics, and Fe have been encapsulated for fortifying dairy products (Table 3C). Omega (ω)-3 fatty acids in soya lecithin shrimp oil have been encapsulated for the fortification of skim milk (130). Shrimp oil nanoliposomes (SONL) fortified by 10/100 ml of skim led to a high level of bitterness. β-glucan, with 0.1 g/100 mL, was almost fully extracted from SONL by adding fortified skim milk. In vitro digestion studies showed that almost half of EPA and DHA were bio-accessible for body adsorption following the release of shrimp oil into the GI tract from nanoliposomes, and the microbial load of fortified skimming milk was stable over 15 days at 4°C. Such foods, high in ω-3 fatty acids, are capable of improving the human immune system discussed in the previous section (see Section Cereal-Based Products), and the formulation of these products can be used as an immunity booster for further study and recommendations for patients with COVID-19. In addition, vitamin-D is also an important precursor to a fat-soluble steroid hormone that plays a role in various body functions, including innate and adaptive immune responses. Vitamin-D also facilitates differences between macrophages and monocytes, enhancing the production of superoxide, phagocytosis, and the destruction of bacteria (24). Furthermore, vitamin-D can modulate the adaptive immune response by suppressing the cell function THP-1 and reducing the development of IL-2 and interferon-gamma proinflammatory cytokines (INF-α). Vitamin-D also activates anti-inflammatory cell cytokines by suppressing Th1 cells indirectly, diverting pro-inflammatory cells to anti-inflammatory cells (197). The frequency and severity of the COVID-19 infection have also been proposed as a deficiency in vitamin-D (25, 26, 64). Low serum vitamin-D levels were related to acute respiratory tract infections, including epidemic influenza, in clinical trials (198). Some recent findings have shown that inadequate vitamin-D will affect the role of the respiratory immune system and increase the risk of severity and mortality in COVID-19 patients (25, 199). Few observational experiments have also been performed to establish the correlation between the vitamin-D levels and the incidence and mortality of the COVID−19 levels (26, 64, 88). Vitamin-D is therefore recommended to improve immunity and reduce human mortality from COVID-19. In this regard, fortified milk and lassi (milk-based Indian beverage) developed by Golfomitsou et al. (134) and Maurya and Aggarwal (133) respectively, with soya lecithin encapsulated vitamin-D3 and could serve as a proper human supplement to prevent the spread of COVID-19. In both products, the vitamin-D3 nanoparticles demonstrated stability in the milk system and the sensory evaluation revealed their overall acceptability to customers. Additional levels of vitamin-D have been proposed to help protect the respiratory epithelium and decrease the risk of infection against pathogens (26). Vitamin-D has a beneficial impact on the immune system, which is useful for COVID-19 ARDS patients during cytokine storms. Vitamin-D supplements have also been known to help minimize viral infection incidence and severity, with a reverse association between serum levels of 25-hydroxyvitamin-D and the upper respiratory tract infection (24).
Iron-fortified yogurt, developed by Gutiérrez et al. (138) by encapsulation in sorbitan monooleate lauryl alcohol and Glycerol, may also be used against coronavirus as Fe plays an important role in our immune system (66). Fe also plays a key role in immunosurveillance through its use in the growth and differentiation of immune cells, as well as in the control of cell-mediated immune response and cytokine production. Fe deficiency results in impaired cell immunity, leading in particular to defective T-cell maturation, discontinuation of macrophage differentiation, and impaired natural killer cells (NKCs) function. The link between Fe deficiency and reduced immune function has been established as iron deficiency anemia (IDA) patients have increased morbidity due to infectious disease. It is also necessary to maintain the amount of Fe in our body (66, 68). As a result, developed Fe-fortified yogurt showed almost identical rheological and sensory properties compared to the control sample (138). This fortified yogurt will serve as an effective product to increase the Fe content of the human body and eventually enhance the immunity of our body against various viral diseases such as COVID-19. A lower concentration of serum Fe was found as an independent risk factor for death in COVID-19 patients (200). Lower body Fe and high tissue Fe levels have been associated with lower lung function and severe pulmonary inflammation, respectively (201). Further, probiotics as discussed above can be encapsulated using dairy matrices and yogurt, fermented milk and cheese have long been used as probiotic carrier dairy foods (202). During the last two decades, probiotic yogurt has been a major consumer achievement, while the solid texture, high content of fat, and pH values of cheese better preserve probiotic cells (203). Yakult—a Japan-based fermented probiotic dairy beverage is prepared from skim milk or skimmed milk powder, sugar, and glucose which is fermented by L. casei Shirota. It contains 8 log cfu/mL of L casei Shirota strains and is effective in preventing digestive disorders as well as helping building immunity and reducing the risk of infections (204, 205). Most recently, dairy waste including buttermilk whey; cheese whey; and milk or cheese whey permeate has been shown to be ideal candidates for the production of functional beverages (206).
Curcumin, another BAC, is an important antioxidant, anti-carcinogenic and anti-inflammatory agent, but sensitive to heat, light, and oxygen, and also has very low water solubility (2.99 × 10−8 M) (207). Its encapsulation thus makes it possible to supply the required dose of curcuminoid to the colon, where they can be easily absorbed. Curcumin has been used as a traditional medicine to treat a spectrum of diseases like rheumatism, diarrhea, intermittent fevers, hepatic disorders, inflammations, leukoderma, amenorrhea, arthritis, colitis, hepatitis, etc. (208, 209). Curcumin's immunomodulating ability is the result of its association with different immunomodulators, not just cellular elements, such as macrophages, DCs, as well as lymphocytes (B and T), but also molecular components involved in inflammatory processes, including cytokines and different transcription factors with their downstream signals (210, 211). The immunostimulation feature of DCs and interferes with the myeloid DC maturation has been found to be inhibited by curcumin. These results have been correlated with the loss of the expression of CD80 and CD86, two co-working membrane proteins that provide the motivation signal required to stimulate T cells, and impairments in the development of proinflammatory cytokine (IL-12) due to NF-κB translocation and inhibition of MAPK (Mitogen-Activated Protein Kinase) activation (212, 213). In comparison, a slightly elevated serum level of immunoglobulin M (IgM) and immunoglobulin G (IgG) curcumin in the Rabbit Diet (2, 4, and 6 g/kg), indicating a possible enhancement of the immune system in curcumin (214). Milk is an important substrate for curcumin delivery in the gut. It possesses distinct structural and physicochemical features as the binding of ions and smaller compounds, self-assembly, and surface-active traits, gelling capacity, pH-based swelling behavior, ability to regulate the matrix rehydration kinetics, the release of BACs, generation of complexes and conjugates from macromolecules' interactions, biocompatibility, and biodegradability, potential to protect encapsulated compounds, and increasing the bioaccessibility and bioavailability of functional components (215–217). In this context, the Ministry of Ayush, Government of India has advised Golden Milk as an immunity-enhancing intervention for self-care during the COVID-19 crisis (218). This milk can be prepared with a half teaspoon of turmeric powder in 150 ml of hot milk. Curcumin can alter surface protein structure in viruses and obstruct the penetration of viruses. It also affects the membrane protein by altering host lipid bilayer functionality (12, 13). The systemic inflammatory reaction of type 1 and type 2 assist cells to activate the cardiovascular symptoms in patients with COVID-19 infection (36). In the myocardial ischemia-reperfusion study, curcumin has been shown to suppress inflammation and necrotic tissues in rats by hindering early-growth response-1 and TNF-α and interleukin-6 reductions (219). Curcumin has therefore demonstrated antiviral activity against many various viruses and may be a treatment choice for COVID-19 disease control (220).
Encapsulation of the above-discussed BACs and their intake would be an ideal solution for boosting immunity to manage the COVID-19 pandemic. Omega (ω)-3 fatty acids, Fe, probiotics, curcumin, etc. can be encapsulated successfully in milk and its products and help our body to resist any kind of undesired change. However, intake of such BACs must be standardized for their getting optimal health benefits.
Meat and Fish-Based Products
Muscle foods (like meat and fish) have been recognized as good sources of nutritional components, including high-quality proteins, essential amino acids (EAAs), vitamin-B complexes, minerals, and numerous other micronutrients (221–223). Numerous health advantages are linked to specific nutrients in muscle food (Figure 6). Muscle foods are known to spoil rapidly and reduce the quality and sustainability of food. Therefore, several researchers develop novel approaches to enhance muscle foods' health and shelf-life through the improvement of their nutrient profile or the addition of (natural) preservatives (221, 224, 225).
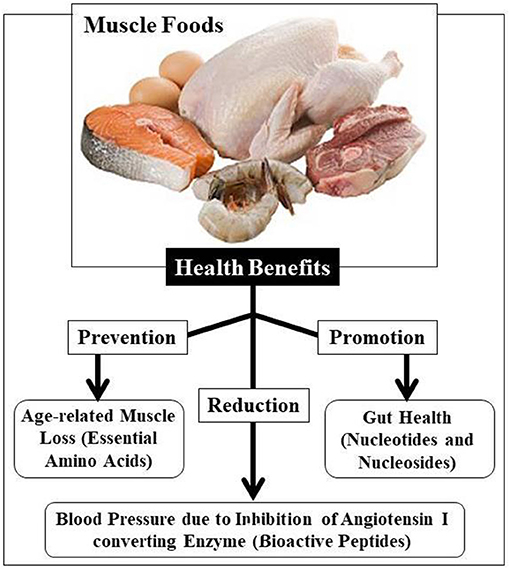
Figure 6. Health benefits of muscle food (221).
Modern nanotechnological techniques can also be applied to fortify meat and fish-based products with valuable preservatives, nutrients, or nutraceuticals (222). Generally, a wide variety of bioactive food-grade substances have been used in meat and fish-based food products for encapsulation with the application of nanotechnology-based delivery systems (6). These bioactive food-grade substances are antimicrobials, antioxidants, fat-soluble vitamins, essential oils, triglyceride oils, flavoring oils, and nutraceuticals (61, 142, 147). The integration into the meat and fish-based food products of food-grade substances by nanoemulsions affects their taste, texture, flavor, nutritional value, and shelf-life. For instance, ω-3 PUFAs have several beneficial effects on human health. The intake of fatty acids can also promote the growth and functioning of the immune system (40, 142, 222, 226). To increase ω-3 fatty acid content, Pourashouri et al. (143) introduced fish oil into fish sausage. There are some benefits to the use of fish oil-loaded nano-emulsions (as opposed to bulk oils) to enhance processed ω-3 fatty acid in meat and fish-based food products. For instance, the production of an even blend of fish oil in all meat and fish-based food products is simpler, which is clearer visually. Furthermore, due to the rapid and absolute digestion of small lipid droplets within the GI tract, the bioavailability of fish oil after ingestion may increase. The ω-3 fatty acid content of chicken meat, which contributes to improved biomarkers for human health, is enhanced by flaxseed oil-nanoemulsions, which are a rich source of plant α-linolenic acids (147). Different scientists have also confirmed that encapsulated ω-3 fatty acids have been used in nano-emulsions, for example, chicken sausage flaxseed oil (151); cod liver oil in chicken nuggets (150); Chia oil in the beef burger (149); and chia oil, linseed oil and tigernuts oil in Deer pâtés (146). The ω-3 PUFAs include docosahexaenoic and eicosapentaenoic fatty acids, considered to have beneficial effects on immunity and inflammation. Interestingly, ω-3 fatty acids prevent influenza virus replication by working against viruses. Accordingly, ω-3 fatty acids can improve the oxygenation of COVID-19 patients, similarly also said by experts of the European Society for Parenteral and Enteral Nutrition (ESPEN), although there is still no clear evidence (39, 227). However, a detailed discussion has already been made regarding the improved safety of ω-3 fatty acids (see Section Cereal-Based Products).
The use of nanoemulsions including fat-soluble vitamins (especially vitamin-E, called tocopherol), can also be integrated into the meat and fish-based food products. Feng et al. (142) encapsulated vitamin-E and incorporated it in fish-based sausages. They found that the nanoemulsions of vitamin-E retain below 500 nm in particle size when held at 4°C for 16 days, and coarse emulsions of vitamin-E increased from 4 to 6 μm by particle size. The reduced particulate size, even distribution, and tocopherol nanoemulsion stability can explain their better antioxidant activity in fish sausages. Interestingly, 250 mg/kg encapsulated nanoemulsions of vitamin-E can delay lipid oxidation and boost the consistency of fish-sausages without altering their texture characteristics during cold-stored conditions. Epidemiological studies have shown that vitamin-E deficiencies influence immune responses and infectious diseases. Vitamin-E is used to enhance T-cells, boost lymphocytes with Mitogenesis, increase IL-2 cytokine secretion, enhance NK cell function, and minimizes infection risks (35, 62, 63, 183).
Antioxidants refer to the molecules which can donate radical hydrogen (H•) radical in conjunction with other free radicals required to avoid the proliferation of oxidation reactions (228). Natural antioxidant compounds can be derived from plant matrices containing non-polar solvents, such as acetone, chloroform, ethanol, and methanol, and their combinations (229–231). The antioxidant potential of pomegranate by-products, grape seed extract, oregano, rosemary, and many different spices has been well-demonstrated in meat and poultry products (230, 232–234). The peels and seeds had more phenolic content than pulp and had the better antioxidant in-vitro ability. Peels and seeds are abundant in catechins, procyanidins, and hydroxycinnamic acids that encourage immunity from foreign pathogens (235, 236). Similarly, quercetin is a bioflavonoid naturally present in several vegetables and fruits quercetin supports antioxidant capacity and protects lung tissue that mainly gets damaged during infection. Laboratory and animal studies have observed that quercetin can inhibit a range of viral infections including a COVID-19-related coronavirus SARS-CoV (83). Various immune cells produce many forms of polyphenol receptors that accept and promote polyphenol cellular uptake that afterward activates signaling pathways for immune reactions (237). These compounds can (i) minimize SARS-CoV-2 viral infection by attaching to the ACE2 receptor and avoid viral infringement; and (ii) change the magnitude of COVID-19-related lung injury through ACE2 expression (238).
In the case of ginger, it has been reported that the immunity-boosting or protective activity of its components could be associated with antioxidant, antimicrobial, anti-inflammatory, and antimutagenic properties and other biological activities (239). Similarly, the major chemical constituents of tulsi are ursolic acid, oleanolic acid, eugenol, rosmarinic acid, linalool, carvacrol, and β-caryophyllene. Owing to the high concentration of eugenol, tulsi may be a COX-2 inhibitor, like many modern painkillers (240).
Thus, such encapsulated BACs will enhance our immune system. They protect us not only from influenza or bacterial infections but also from chronic or less treatable illnesses. Furthermore, eating a healthy diet cannot always be possible, therefore, integrating these bioactive encapsulated compounds into our food supply may be a potential tool for us to restore or preserve our health.
Prospective Future and Research Opportunities
Low-immunity individuals are more vulnerable to the COVID-19 global pandemic. In this regard, the encapsulated BACs play a key function by fostering healthy bacteria in the body, which support or improve immunity (Table 1). Further, the rising desire of consumers to make healthier food choices led to a fast-growing demand for functional foods and drinks. Microencapsulation can be useful for enhancing the nutritional and health promotion of food products by adding BACs. Microencapsulation can have huge benefits to increase the supply and protection of bioprotective ingredients in food; otherwise, it cannot be made feasible. There has been a tendency for many food products to enhance immune responses, including functional foods that control the immune system by increasing or inhibiting immune response, providing host protection against infection, and reducing allergies and inflammation. Several vitamins like C, D, and E are tested for essential immune enhancement facets in addition to BACs like ω-3 fatty acids, probiotics, antioxidants, etc (Table 1). These compounds have enough potential and possibilities to be employed for the prevention of COVID-19 by boosting immunity. The effect of micronutrients on immune function has undoubtedly become an essential component of immunity. Micronutrient homeostasis preservation is an important factor in maintaining a healthy immune system and it has also shown that a variety of vitamins and trace elements play a crucial part in immune function. However, micronutrients such as vitamin-A, vitamin-B, Zn, selenium (Se), copper (Cu), magnesium (Mg) have been encapsulated but not used to develop functional foods. Potential work can be done on these compounds to develop functional foods with immunological activity. Likewise, BACs based on plants such as β-carotene, resveratrol, and other flavonoids and polyphenols have been successfully used against other viral diseases such as human immunodeficiency virus (HIV), MERS-CoV. The effect of these BACs on immunity can also be studied and used to produce functional foods using various encapsulation techniques. In this regard, the probiotics that are living microbes can provide the host with health and well-being. They play a major role in improving immunity. However, their use in the beverage sector is very limited. Even its use in other foods like meat-based foods or cereal-based food items can be explored. On the other hand, prebiotics is used to encourage wellness as a novel food product and are mostly carbohydrates and some are non-carbohydrate. Nevertheless, no studies on the prebiotic interaction mechanism for human immunity have been released. The role of prebiotics in the production of functional foods with prebiotics to enhance the immune system of the person's body is therefore important.
The production of such functional foods should be increased and commercialized to improve the immunity of the human body to various infections including COVID-19. The application and use of some compounds have been reported in different studies due to their immunological activity (Table 1). However, several other compounds have an immunological activity and have been encapsulated but surprisingly, have not been used in the formulation of any functional food. These encapsulated BACs can be recommended for use by various researchers, scientists, and industrial peoples who are concerned with food and allied subjects to develop more and more functional foods that can improve immunity in the human body, ultimately to human health. Here are many important aspects for food researchers, food scientists, and the food industry that will give them a deeper understanding of their present work and future. The majority of the encapsulated BACs observed antioxidative, antimutagenic, anti-inflammatory, properties as well as other biological activities. However, the exact component responsible for the immunity-boosting effect of such components against COVID-19 may also be studied. These important points will also raise awareness among researchers about the future of functional food and its role in the control of viral diseases such as COVID-19.
Concluding Remarks
A healthy and strong immune system must be capable of recognizing and adapting to changes in the environment around it. Diverse micronutrients are important for immune-competence, including vitamins, trace elements, ω-3 fatty acids, probiotics, antioxidants, etc. The preservation of physical barriers of pathogens and two primary forms of immunity, both innate and adaptive immunity can contribute to varying degrees with food BACs and micronutrients. Encapsulation of these compounds with immunological activity and their use in food products can both increase the functionality and enhance human health's immunity to diseases such as COVID-19. However, far more compounds have not been investigated for their immunological effect. Therefore, researchers, scientists, and industry should concentrate on this research topic to detect and apply more immunity-enhancement compounds in the production of functional foods using encapsulation techniques to resolve the global war against COVID-19. More research with systematic approaches is needed to understand the behavior of coronaviruses and the role of food products in COVID-19 prevention.
Author Contributions
DV has conceptualized, interpreted, corrected, and technically sound final versions of the manuscript. ST has compiled literature for manuscripts. MT, SS, AP, and MC-G have interpreted the manuscript, corrected it, and made it scientifically sound for the final version. CA and PS have provided technical suggestions, corrections, and permissions for the finalization of the manuscript. All authors contributed to the article and approved the submitted version.
Conflict of Interest
The authors declare that the research was conducted in the absence of any commercial or financial relationships that could be construed as a potential conflict of interest.
Acknowledgments
ST thanks the Ministry of Education (formerly the Ministry of Human Resource Development), Government of India for an Institute Research Assistantship, and also thanks to the Agricultural and Food Engineering Department, Indian Institute of Technology Kharagpur for their assistance in this study.
Abbreviations
ACE2, angiotensin-converting enzyme-2; APCs, antigen presenting cells; ARDS, acute respiratory distress syndrome; ARE, antioxidant response element; ATP, adenosine triphosphate; BACs, bioactive compounds; CCTG, caprylic/capric triglyceride; CD, β-cyclodextrin; CGC, calcium-gelatin casein; COVID-19, coronavirus disease 2019; Cu, copper; DCs, dendritic cells; DHA, docosahexaenoic acid; DNA, deoxyribonucleic acid; DPPC, di-palmitoyl phosphatidylcholine; EAAs, essential amino acids; EPA, eicosapentaenoic acid; ESPEN, European Society for Parenteral and Enteral Nutrition; Fe, iron; FSSAI, Food Safety and Standards Authority of India; GI, gastrointestinal; HACS, high amylose corn starch; HIV, human immunodeficiency virus; hs-CRP, high sensitivity C-reactive protein; IDA, iron deficiency anemia; IgA, immunoglobulin A; IgG, immunoglobulin G; IgM, immunoglobulin M; INF-α, interferon-gamma proinflammatory cytokines; LPS, lipopolysaccharide; MAPK, mitogen-activated protein kinase; MC, methyl cellulose; MD, maltodextrin; MERS-CoV, middle east respiratory syndrome–related coronavirus; Mg, magnesium; miRNA, micro RNA; mRNA, messenger RNA; NFκB, nuclear factor kappa B; NKCs, natural killer cells; Nrf2, nuclear factor erythroid 2 (nfe2)-related factor 2; PUFAs, polyunsaturated fatty acids; RNA, ribonucleic acid; ROS, reactive oxygen species; SARS-CoV-2, severe acute respiratory syndrome–related coronavirus 2; Se, selenium; SIF, synthetic intestinal fluids; SPS, Soybean protein isolates; TCR, T-cell receptor; THP, T-helper type; TLR, toll-like receptor; TNF-α, tumor necrosis factor-α; WBCs, white blood cells; WHO, World Health Organization; WPC, whey protein concentrate; WPI, whey protein isolate; Zn, zinc.
References
1. Bogue J, Collins O, Troy AM. Market analysis and concept development of functional foods. In: Bagchi D, Nair S, editors. Developing New Functional Food and Nutraceutical Products. Cambridge: Elsevier Science Publishing Co Inc. (2017). p. 29–45.
2. Díaz DL, Fernández-Ruiz V, Cámara M. The frontier between nutrition and pharma: the international regulatory framework of functional foods, food supplements and nutraceuticals. Crit Rev Food Sci Nutr. (2020) 60:1738–46. doi: 10.1080/10408398.2019.1592107
3. Díaz DL, Fernández-Ruiz V, Cámara M. An international regulatory review of food health-related claims in functional food products labeling. J Funct Foods. (2020) 68:103896. doi: 10.1016/j.jff.2020.103896
4. Hieke S, Kuljanic N, Wills JM, Pravst I, Kaur A, Raats MM, et al. The role of health-related claims and health-related symbols in consumer behaviour: Design and conceptual framework of the CLYMBOL project and initial results. Nutrition Bulletin. (2015) 40:66–72. doi: 10.1111/nbu.12128
5. Verma DK, Patel AR, Sandhu KS, Baldi A, Garcia S. Biotechnical Processing in the Food Industry: New Methods, Techniques, and Applications. Florida, FL: Apple Academic Press (2021).
6. Xiong K, Zhou L, Wang J, Ma A, Fang D, Xiong L, et al. Construction of food-grade pH-sensitive nanoparticles for delivering functional food ingredients. Trends Food Sci Technol. (2020) 96:102–13. doi: 10.1016/j.tifs.2019.12.019
7. Huang YC, Kuo TH. O-carboxymethyl chitosan/fucoidan nanoparticles increase cellular curcumin uptake. Food Hydrocolloids. (2016) 53:261–9. doi: 10.1016/j.foodhyd.2015.02.006
8. McClements DJ. Encapsulation, protection, and delivery of bioactive proteins and peptides using nanoparticle and microparticle systems: a review. Adv Colloid Interface Sci. (2018) 253:1–22. doi: 10.1016/j.cis.2018.02.002
9. Martinez-Medina GA, Chávez-González ML, Verma DK, Prado-Barragán LA, Martínez-Hernández JL, Flores-Gallegos AC, et al. Bio-funcional components in mushrooms, a health opportunity: ergothionine and huitlacohe as recent trends. J Funct Foods. (2021) 77:104326. doi: 10.1016/j.jff.2020.104326
10. Verma DK, Goyal MR, Suleria HAR. Nanotechnology and Nanomaterial Applications in Food, Health and Biomedical Sciences. Florida, FL: Apple Academic Press, (2020).
11. Chai J, Jiang P, Wang P, Jiang Y, Li D, Bao W, et al. The intelligent delivery systems for bioactive compounds in foods: physicochemical and physiological conditions, absorption mechanisms, obstacles and responsive strategies. Trends Food Sci Technol. (2018) 78:144–54. doi: 10.1016/j.tifs.2018.06.003
12. Chen MC, Mi FL, Liao ZX, Hsiao CW, Sonaje K, Chung MF, et al. Recent advances in chitosan-based nanoparticles for oral delivery of macromolecules. Adv Drug Deliv Rev. (2013) 65:865–79 doi: 10.1016/j.addr.2012.10.010
13. Chen TY, Chen DY, Wen HW, Ou JL, Chiou SS, Chen JM, et al. Inhibition of enveloped viruses infectivity by curcumin. PLoS ONE. (2013) 8:e62482. doi: 10.1371/journal.pone.0062482
14. WHO (World Health Organization) (2020). Coronavirus Disease (COVID-19) Pandemic. Available online at: https://www.who.int/emergencies/diseases/novel-coronavirus-2019 (accessed August 29, 2020).
15. Saghazadeh A, Rezaei N. Towards treatment planning of COVID-19: Rationale and hypothesis for the use of multiple immunosuppressive agents: anti-antibodies, immunoglobulins, and corticosteroids. Int Immunopharmacol. (2020) 84:1–6. doi: 10.1016/j.intimp.2020.106560
16. Dongyuan W, Zigang L, Yihui L. An overview of the safety, clinical application and antiviral research of the COVID-19 therapeutics. J Infect Public Health. (2020) 13:1405–14. doi: 10.1016/j.jiph.2020.07.004
17. Zhong J, Tang J, Ye C, Dong L. The immunology of COVID-19: is immune modulation an option for treatment? Lancet Rheumatol. (2020) 2:E428–36. doi: 10.1016/S2665-9913(20)30120-X
18. Lotfi M, Hamblin MR, Rezaei N. COVID-19: transmission, prevention, and potential therapeutic opportunities. Clinica Chimica Acta. (2020) 508:254–66. doi: 10.1016/j.cca.2020.05.044
19. AminJafari A, Ghasemi S. The possible of immunotherapy for COVID-19: A systematic review. Int Immunopharmacol. (2020) 83:106455. doi: 10.1016/j.intimp.2020.106455
20. Klimek L, Jutel M, Akdis C, Bousquet J, Akdis M, Bachert C, et al. Handling of allergen immunotherapy in the COVID-19 pandemic: An ARIA-EAACI statement. Allergy. (2020) 75:1546–554. doi: 10.1111/all.14336
21. Yazdanpanah F, Hamblin MR, Rezaei N. The immune system and COVID-19: Friend or foe? Life Sci. (2020) 256:117900. doi: 10.1016/j.lfs.2020.117900
22. Calder PC. Nutrition, immunity and Covid-19. BMJ Nutr Prev Health. (2020) 3:74–92. doi: 10.1136/bmjnph-2020-000085
23. Khan HMW, Parikh N, Megala SM, Predeteanu GS. Unusual early recovery of a critical covid-19 patient after administration of intravenous vitamin C. Am J Case Rep. (2020) 21:e925521. doi: 10.12659/AJCR.925521
24. Shakoor H, Feehan J, Dhaheri ASA, Ali HI, Platat C, Ismail LC, et al. Immune-boosting role of vitamins D, C, E, zinc, selenium and omega-3 fatty acids: could they help against COVID-19? Maturitas. (2020) 43:1–9. doi: 10.1016/j.maturitas.2020.08.003
25. Grant WB, Lahore H, McDonnell SL, Baggerly CA, French CB, Aliano JL, et al. Evidence that vitamin D supplementation could reduce risk of influenza and COVID-19 infections and deaths. Nutrients. (2020) 12:988. doi: 10.3390/nu12040988
26. Merzon E, Tworowski D, Gorohovski A, Vinker S, Golan Cohen A, Green I, et al. Low plasma 25 (OH) vitamin D level is associated with increased risk of COVID-19 infection: an Israeli population-based study. FEBS J. (2020) 287:3693–702. doi: 10.1111/febs.15495
27. Xu L, Mao Y, Chen G. Risk factors for 2019 novel coronavirus disease (COVID-19) patients progressing to critical illness: a systematic review and meta-analysis. Aging. (2020) 12:12410. doi: 10.18632/aging.103383
28. Chowdhury MA, Hossain N, Kashem MA, Shahid MA, Alam A. Immune response in covid-19: a review. J Infect Public Health. (2020) 13:1619–29. doi: 10.1016/j.jiph.2020.07.001
29. Verma DK, Patel AR, Billoria S, Kaushik G, Kaur M. Microbial Biotechnology in Food Processing and Health: Advances, Challenges, and Potential. Florida, FL: Apple Academic Press (2021).
30. Shang J, Ye G, Shi K, Wan Y, Luo C, Aihara H, et al. Structural basis of receptor recognition by SARS-CoV-2. Nature. (2020) 581:221–4. doi: 10.1038/s41586-020-2179-y
31. Wan Y, Shang J, Graham R, Baric RS, Li F. Receptor recognition by the novel coronavirus from Wuhan: an analysis based on decade-long structural studies of SARS coronavirus. J Virol. (2020) 94: e00127–e00120. doi: 10.1128/JVI.00127-20
32. Tang NLS, Chan PKS, Wong CK, To KF, Wu AKL, Sung YM, et al. Early enhanced expression of interferon-inducible protein-10 (CXCL-10) and other chemokines predicts adverse outcome in severe acute respiratory syndrome. Clin Chem. (2005) 51:2333–2340. doi: 10.1373/clinchem.2005.054460
33. Wu Z, McGoogan JM. Characteristics of and important lessons from the coronavirus disease 2019 (COVID-19) outbreak in China: summary of a report of 72 314 cases from the Chinese Center for Disease Control and Prevention. JAMA. (2020) 323:1239–42. doi: 10.1001/jama.2020.2648
34. Wang D, Hu B, Hu C, Zhu F, Liu X, Zhang J, et al. Clinical characteristics of 138 hospitalized patients with 2019 novel coronavirus–infected pneumonia in Wuhan, China. JAMA. (2020) 323:1061–9. doi: 10.1001/jama.2020.1585
35. Kieliszek M, Lipinski B. Selenium supplementation in the prevention of coronavirus infections (COVID-19). Med Hypotheses. (2020) 143:109878. doi: 10.1016/j.mehy.2020.109878
36. Huang C, Wang Y, Li X, Ren L, Zhao J, Hu Y, et al. Clinical features of patients infected with 2019 novel coronavirus in Wuhan, China. Lancet. (2020) 395:497–506. doi: 10.1016/S0140-6736(20)30183-5
37. Chang MC, Park YK, Kim BO, et al. Risk factors for disease progression in COVID-19 patients. BMC Infect Dis. (2020). 20:1–6. doi: 10.1186/s12879-020-05144-x
38. Shi Y, Wang Y, Shao C, Huang J, Gan J, Huang X, et al. COVID-19 infection: the perspectives on immune responses. Cell Death Differ. (2020) 27:1451–4. doi: 10.1038/s41418-020-0530-3
39. Rogero MM, Leão MDC, Santana TM, de MB, Pimentel MV, Carlini GC, et al. Potential benefits and risks of omega-3 fatty acids supplementation to patients with COVID-19. Free Radic Biol Med. (2020) 156:190–9. doi: 10.1016/j.freeradbiomed.2020.07.005
40. Gutiérrez S, Svahn SL, Johansson ME. Effects of omega-3 fatty acids on immune cells. Int J Mol Sci. (2019) 20:5028. doi: 10.3390/ijms20205028
41. McDonnell RP, O'Doherty JV, Earley B, Clarke AM, Kenny DA. Effect of supplementation with n-3 polyunsaturated fatty acids and/or β-glucans on performance, feeding behaviour and immune status of Holstein Friesian bull calves during the pre-and post-weaning periods. J Anim Sci Biotechnol. (2019) 10:7. doi: 10.1186/s40104-019-0317-x
42. Chew BP, Park JS. Carotenoid action on the immune response. J Nutr. (2004) 134:257S−61S. doi: 10.1093/jn/134.1.257S
43. Milani A, Basirnejad M, Shahbazi S, Bolhassani A. Carotenoids: biochemistry, pharmacology and treatment. Br J Pharmacol. (2017) 174:1290–324. doi: 10.1111/bph.13625
44. Hekal AM, Saidahmed A, Easa F, Ali W, Mousa S. Effect of carvacrol on productive performance and some immunological parameters of growing rabbits. Egypt J Rabbit Sci. (2016) 26:281–99. doi: 10.21608/ejrs.2016.42112
45. Yilmaz E, Ergün S. Influence of carvacrol on the growth performance, hematological, non-specific immune and serum biochemistry parameters in rainbow trout (Oncorhynchus mykiss). Food Nutr Sci. (2015) 6:523. doi: 10.4236/fns.2015.65054
46. Nawab A, Tang S, Li G, An L, Wu J, Liu W, et al. Dietary curcumin supplementation effects on blood immunological profile and liver enzymatic activity of laying hens after exposure to high temperature conditions. J Therm Biol. (2020) 90:102573. doi: 10.1016/j.jtherbio.2020.102573
47. Muruganandan S, Lal J, Gupta PK. Immunotherapeutic effects of mangiferin mediated by the inhibition of oxidative stress to activated lymphocytes, neutrophils and macrophages. Toxicology. (2005) 215:57–68. doi: 10.1016/j.tox.2005.06.008
48. Rajendran P, Jayakumar T, Nishigaki I, Ekambaram G, Nishigaki Y, Vetriselvi J, et al. Immunomodulatory effect of mangiferin in experimental animals with benzo (a) pyrene-induced lung carcinogenesis. Int J Biomed Sci. (2013) 9:68.
49. Malaguarnera L. Influence of resveratrol on the immune response. Nutrients. (2019) 11:946. doi: 10.3390/nu11050946
50. Ognik K, Cholewińska E, Czech A. The effect of adding hesperidin, diosmin, quercetin and resveratrol extracts to feed for turkey hens on selected immunological and biochemical blood indices. Ann Anim Sci. (2016) 16:1101–14. doi: 10.1515/aoas-2016-0035
51. Shi Y, Zhou J, Jiang B, Miao M. Resveratrol and inflammatory bowel disease. Ann N Y Acad Sci. (2017) 1403:38–47. doi: 10.1111/nyas.13426
52. Gholijani N, Gharagozloo M, Kalantar F, Ramezani A, Amirghofran Z. Modulation of cytokine production and transcription factors activities in human Jurkat T cells by thymol and carvacrol. Adv Pharm Bull. (2015) 5:653. doi: 10.15171/apb.2015.089
53. Huang Z, Liu Y, Qi G, Brand D, Zheng SG. Role of vitamin A in the immune system. J Clin Med. (2018) 7:258. doi: 10.3390/jcm7090258
54. Trasino SE. A role for retinoids in the treatment of COVID-19? Clin Exp Pharmacol Physiol. (2020) 47:1765–7. doi: 10.1111/1440-1681.13354
55. Spinas E, Saggini A, Kritas SK, Cerulli G, Caraffa A, Antinolfi P, et al. Crosstalk between vitamin B and immunity. J Biol Regul Homeost Agents. (2015) 29:283–288.
56. Yoshii K, Hosomi K, Sawane K, Kunisawa J. Metabolism of dietary and microbial vitamin B family in the regulation of host immunity. Front Nutr. (2019) 6:48. doi: 10.3389/fnut.2019.00048
57. Hiedra R, Lo KB, Elbashabsheh M, Gul F, Wright RM, Albano J, et al. The use of IV vitamin C for patients with Covid-19: a single center observational study. Expert Rev Anti Infect Ther. (2020) 18:1–2. doi: 10.1080/14787210.2020.1794819
58. Khara H, Sayyadborani M, SayyadBorani M. Effects of α-tocopherol (vitamin E) and ascorbic acid (vitamin C) and their combination on growth, survival and some haematological and immunological parameters of Caspian brown trout, Salmo Trutta Caspius juveniles. Turk J Fish Aquat Sci. (2016) 16:385–393. doi: 10.4194/1303-2712-v16_2_18
59. Ang A, Pullar JM, Currie MJ, Vissers MC. Vitamin C and immune cell function in inflammation and cancer. Biochem Soc Trans. (2018) 46:1147–59. doi: 10.1042/BST20180169
60. Carr AC, Maggini S. Vitamin C and immune function. Nutrients. (2017) 9:1211. doi: 10.3390/nu9111211
61. Hedayati SA, Farsani HG, Naserabad SS, Hoseinifar SH, Van Doan H. Protective effect of dietary vitamin E on immunological and biochemical induction through silver nanoparticles (AgNPs) inclusion in diet and silver salt (AgNO3) exposure on Zebrafish (Danio rerio). Comp Biochem Physiol C Toxicol Pharmacol. (2019) 222:100–7. doi: 10.1016/j.cbpc.2019.04.004
62. Lee GY, Han SN. The role of vitamin E in immunity. Nutrients. (2018) 10:1614. doi: 10.3390/nu10111614
63. Coskun M, Kayis T, Gulsu E, Emel ALP. Effects of selenium and vitamin E on enzymatic, biochemical, and immunological biomarkers in Galleria mellonella L. Sci Rep. (2020) 10:1–7. doi: 10.1038/s41598-020-67072-9
64. Ali N. Role of vitamin D in preventing of COVID-19 infection, progression and severity. J Infect Public Health. (2020) 13:1373–80. doi: 10.1016/j.jiph.2020.06.021
65. Yamamoto E, Jørgensen TN. Immunological effects of vitamin D and their relations to autoimmunity. J Autoimmun. (2019) 100:7–16. doi: 10.1016/j.jaut.2019.03.002
66. Lange KW, Nakamura Y. Food bioactives, micronutrients, immune function and COVID-19. J Food Bioactives. (2020) 10:1–8. doi: 10.31665/JFB.2020.10222
67. Raha S, Mallick R, Basak S, Duttaroy AK. Is copper beneficial for COVID-19 patients? Med Hypotheses. (2020) 142:109814 doi: 10.1016/j.mehy.2020.109814
68. Phipps O, Brookes MJ, Al-Hassi HO. Iron deficiency, immunology, colorectal cancer. Nutr Rev. (2020) 79:88–97. doi: 10.1093/nutrit/nuaa040
69. Nairz M, Haschka D, Demetz E, Weiss G. Iron at the interface of immunity and infection. Front Pharmacol. (2014) 5:152. doi: 10.3389/fphar.2014.00152
70. Tam M, Gomez S, Gonzalez-Gross M, Marcos A. Possible roles of magnesium on the immune system. Eur J Clin Nutr. (2003) 57:1193–7. doi: 10.1038/sj.ejcn.1601689
72. Avery JC, Hoffmann PR. Selenium, selenoproteins, and immunity. Nutrients. (2018) 10:1203. doi: 10.3390/nu10091203
73. Hoffmann PR. Mechanisms by which selenium influences immune responses. Arch Immunol Ther Exp. (2007) 55:289–97. doi: 10.1007/s00005-007-0036-4
74. Bonaventura P, Benedetti G, Albarède F, Miossec P. Zinc and its role in immunity and inflammation. Autoimmun Rev. (2015) 14:277–85. doi: 10.1016/j.autrev.2014.11.008
75. Maywald M, Wessels I, Rink L. Zinc signals and immunity. Int J Mol Sci. (2017) 18:2222. doi: 10.3390/ijms18102222
76. Read SA, Obeid S, Ahlenstiel C, Ahlenstiel G. The role of zinc in antiviral immunity. Adv Nutr. (2019) 10:696–710. doi: 10.1093/advances/nmz013
77. Markowiak P, Slizewska K. Effects of probiotics, prebiotics, and synbiotics on human health. Nutrients. (2017) 9:1021. doi: 10.3390/nu9091021
78. Frei R, Akdis M, O'Mahony L. Prebiotics, probiotics, synbiotics, and the immune system: experimental data and clinical evidence. Curr Opin Gastroenterol. (2015) 31:153–8. doi: 10.1097/MOG.0000000000000151
79. Hao Q, Dong BR, Wu T. Probiotics for preventing acute upper respiratory tract infections. Cochrane Database Syst Rev. (2015) 2015:CD006895. doi: 10.1002/14651858.CD006895.pub3
80. Rinaldi E, Consonni A, Guidesi E, Elli M, Mantegazza R, Baggi F. Gut microbiota and probiotics: novel immune system modulators in myasthenia gravis?. Ann N Y Acad Sci. (2018) 1413:49–58. doi: 10.1111/nyas.13567
81. Vitetta L, Vitetta G, Hall S. Immunological tolerance and function: associations between intestinal bacteria, probiotics, prebiotics, and phages. Front Immunol. (2018) 9:2240. doi: 10.3389/fimmu.2018.02240
82. WHO (World Health Organization) (2020). Nutrition Advice for Adults During the COVID-19 Outbreak. Available online at: http://www.emro.who.int/nutrition/nutrition-infocus/nutrition-advice-for-adults-during-the-covid-19-outbreak.html. (accessed August 29, 2020).
83. Arshad MS, Khan U, Sadiq A, Khalid W, Hussain M, Yasmeen A, et al. Coronavirus disease (COVID-19) and immunity booster green foods: a mini review. Food Sci Nutr. (2020) 8:3971–6. doi: 10.1002/fsn3.1719
84. Mishra S, Patel M. Role of nutrition on immune system during COVID-19 pandemic. J Food Nutr Health. (2020) 3:1–6.
85. Verma DK, Goyal K, Kumar P, El-Shazly M. Unmasking the many faces of Giloy (Tinospora cordifolia L.): a fresh look on its phytochemical and medicinal properties. Curr Pharm Design. (2020) 26:1–11. doi: 10.2174/1381612826666200625111530
86. Kumar P, Verma DK, Kimmy G, Srivastav PP, Sandhu KS. Phytochemicals in Giloy (Tinospora cordifolia L.): structure, chemistry, health benefits. In: Verma DK, editors. Phytochemicals in Food and Health: Perspectives for Research and Technological Development. Florida, FL: Apple Academic Press (2021).
87. Verma DK, Thakur M. Phytochemicals in Food and Health: Perspectives for Research and Technological Development. Florida, FL: Apple Academic Press (2021).
88. Darling AL, Ahmadi KR, Ward KA, Harvey NC, Alves AC, Dunn-Waters DK, et al. Vitamin D status, body mass index, ethnicity and COVID-19: initial analysis of the first-reported UK Biobank COVID-19 positive cases (n 580) compared with negative controls (n 723). MedRxiv. (2020). doi: 10.1101/2020.04.29.20084277
89. Jayaweera JAAS, Reyes M, Joseph A. Childhood iron deficiency anemia leads to recurrent respiratory tract infections and gastroenteritis. Sci Rep. (2019) 9:1–8. doi: 10.1038/s41598-019-49122-z
90. Maares M, Haase H. Zinc and immunity: an essential interrelation. Arch Biochem Biophy. (2016) 611:58–65. doi: 10.1016/j.abb.2016.03.022
91. Ng TB, Cheung RCF, Wong JH, Wang Y, Ip DTM, Wan DCC, et al. Antiviral activities of whey proteins. Appl Microbiol Biotechnol. (2015) 99:6997–7008. doi: 10.1007/s00253-015-6818-4
92. Jin YH, Cai L, Cheng ZS, Cheng H, Deng T, Fan YP, et al. A rapid advice guideline for the diagnosis and treatment of 2019 novel coronavirus (2019-nCoV) infected pneumonia (standard version). Mil Med Res. (2020) 7:4. doi: 10.1186/s40779-020-0233-6
93. GLOBENEWSWIRE. Growing Demand For Immunity Boosting Functional Foods And Beverages With The Outbreak Of COVID-19. (2021). Available onlie at: https://www.globenewswire.com/news-release/2021/01/07/2155107/0/en/Growing-Demand-For-Immunity-Boosting-Functional-Foods-And-Beverages-With-The-Outbreak-Of-COVID-19.html (accessed April 23, 2021).
94. Ashaolu TJ. Immune boosting functional foods and their mechanisms: A critical evaluation of probiotics and prebiotics. Biomed Pharmacother. (2020) 130:110625. doi: 10.1016/j.biopha.2020.110625
95. Dullius A, Fassina P, Giroldi M, Goettert MI, de Souza CFV. A biotechnological approach for the production of branched chain amino acid containing bioactive peptides to improve human health: A review. Food Res Int. (2020) 131:109002. doi: 10.1016/j.foodres.2020.109002
96. Delshadi R, Bahrami A, Tafti AG, Barba FJ, Williams LL. Micro and nano-encapsulation of vegetable and essential oils to develop functional food products with improved nutritional profiles. Trends Food Sci Technol. (2020) 104:72–83. doi: 10.1016/j.tifs.2020.07.004
97. Ezhilarasi PN, Indrani D, Jena BS, Anandharamakrishnan C. Microencapsulation of Garcinia fruit extract by spray drying and its effect on bread quality. J Sci Food Agric. (2014) 94:1116–23. doi: 10.1002/jsfa.6378
98. Amoah I, Cairncross C, Sturny A, Rush E. Towards improving the nutrition and health of the aged: the role of sprouted grains and encapsulation of bioactive compounds in functional bread–a review. Int J Food Sci Technol. (2019) 54:1435–47. doi: 10.1111/ijfs.13934
99. Lu ZH, Donner E, Liu Q. Effect of roasted pea flour/ starch and encapsulated pea starch incorporation on the in vitro starch digestibility of pea breads. Food Chem. (2018) 245:71–8. doi: 10.1016/j.foodchem.2017.10.037
100. Pasrija D, Ezhilarasi PN, Indrani D, Anandharamakrishnan C. Microencapsulation of green tea polyphenols and its effect on incorporated bread quality. LWT—Food Sci Technol. (2015) 64:289–96. doi: 10.1016/j.lwt.2015.05.054
101. Davidov-Pardo G, Roccia P, Salgado D, León A, Pedroza-Islas R. Utilization of different wall materials to microencapsulate fish oil. Am J Food Technol. (2008) 3:384–93. doi: 10.3923/ajft.2008.384.393
102. Gökmen V, Mogol BA, Lumaga RB, Fogliano V, Kaplun Z, Shimoni E. Development of functional bread containing nanoencapsulated omega-3 fatty acids. J Food Eng. (2011) 105:585–91. doi: 10.1016/j.jfoodeng.2011.03.021
103. González A, Martínez ML, León AE, Ribotta PD. Effects on bread and oil quality after functionalization with microencapsulated chia oil. J Sci Food Agric. (2018) 98:4903–10. doi: 10.1002/jsfa.9022
104. Ojagh SM, Hasani S. Characteristics and oxidative stability of fish oil nano-liposomes and its application in functional bread. J Food Meas Characterization. (2018) 12:1084–92. doi: 10.1007/s11694-018-9724-5
105. Rasti B, Erfanian A, Selamat J. Novel nanoliposomal encapsulated omega-3 fatty acids and their applications in food. Food Chem. (2017) 230:690–6. doi: 10.1016/j.foodchem.2017.03.089
106. Takeungwongtrakul S, Benjakul S. Biscuits fortified with micro-encapsulated shrimp oil: characteristics and storage stability. J Food Sci Technol. (2017) 54:1126–36. doi: 10.1007/s13197-017-2545-4
107. Umesha SS, Manohar RS, Indiramma AR, Akshitha S, Naidu KA. Enrichment of biscuits with microencapsulated omega-3 fatty acid (Alpha-linolenic acid) rich Garden cress (Lepidium sativum) seed oil: Physical, sensory and storage quality characteristics of biscuits. LWT-Food Sci Technol. (2015) 62:654–61. doi: 10.1016/j.lwt.2014.02.018
108. Gallardo G, Guida L, Martinez V, López MC, Bernhardt D, Blasco R, et al. Microencapsulation of linseed oil by spray drying for functional food application. Food Res Int. (2013) 52:473–82. doi: 10.1016/j.foodres.2013.01.020
109. Altamirano-Fortoul R, Moreno-Terrazas R, Quezada-Gallo A, Rosell CM. Viability of some probiotic coatings in bread and its effect on the crust mechanical properties. Food Hydrocolloids. (2012) 29:166–74. doi: 10.1016/j.foodhyd.2012.02.015
110. Davidov-Pardo G, Moreno M, Arozarena I, Marín-Arroyo MR, Bleibaum RN, Bruhn CM. Sensory and consumer perception of the addition of grape seed extracts in cookies. J Food Sci. (2012) 77:S430–8. doi: 10.1111/j.1750-3841.2012.02991.x
111. de Conto LC, Oliveira RSP, Martin LGP, Chang YK, Steel CJ. Effects of the addition of microencapsulated omega-3 and rosemary extract on the technological and sensory quality of white pan bread. LWT-Food Sci Technol. (2012) 45:103–9. doi: 10.1016/j.lwt.2011.07.027
112. Wang YF, Shao JJ, Zhou CH, Zhang DL, Bie XM, Lv FX, et al. Food preservation effects of curcumin microcapsules. Food Control. (2012) 27:113–7. doi: 10.1016/j.foodcont.2012.03.008
113. Henna Lu FS, Norziah MH. Contribution of microencapsulated n-3 PUFA powder toward sensory and oxidative stability of bread. J Food Process Preserv. (2011) 35:596–604. doi: 10.1111/j.1745-4549.2010.00505.x
114. Verardo V, Ferioli F, Riciputi Y, Iafelice G, Marconi E, Caboni MF. Evaluation of lipid oxidation in spaghetti pasta enriched with long chain n– 3 polyunsaturated fatty acids under different storage conditions. Food Chem. (2009) 114:472–7. doi: 10.1016/j.foodchem.2008.09.074
115. Serna-Saldivar SO, Zorrilla R, De La Parra C, Stagnitti G, Abril R. Effect of DHA containing oils and powders on baking performance and quality of white pan bread. Plant Foods Hum Nutr. (2006) 61:121–9. doi: 10.1007/s11130-006-0009-5
116. Sarkar P, Bhunia AK, Yao Y. Impact of starch-based emulsions on the antibacterial efficacies of nisin and thymol in cantaloupe juice. Food Chem. (2017) 217:155–62. doi: 10.1016/j.foodchem.2016.08.071
117. Tao J, Zhu Q, Qin F, Wang M, Chen J, Zheng ZP. Preparation of steppogenin and ascorbic acid, vitamin E. butylated hydroxytoluene oil-in-water microemulsions: Characterization, stability, and antibrowning effects for fresh apple juice. Food Chem. (2017) 224:11–8. doi: 10.1016/j.foodchem.2016.12.045
118. Char C, Cisternas L, Pérez F, Guerrero S. Effect of emulsification on the antimicrobial activity of carvacrol. CyTA-J Food. (2016) 14:186–92. doi: 10.1080/19476337.2015.1079558
119. Dong X, Zhu Q, Dai Y, He J, Pan H, Chen J, et al. Encapsulation artocarpanone and ascorbic acid in O/W microemulsions: Preparation, characterization, and antibrowning effects in apple juice. Food Chem. (2016) 192:1033–40. doi: 10.1016/j.foodchem.2015.07.124
120. Chaikham P. Stability of probiotics encapsulated with Thai herbal extracts in fruit juices and yoghurt during refrigerated storage. Food Biosci. (2015) 12:61–6. doi: 10.1016/j.fbio.2015.07.006
121. Phoem AN, Chanthachum S, Voravuthikunchai SP. Applications of microencapsulated Bifidobacterium longum with Eleutherine americana in fresh milk tofu and pineapple juice. Nutrients. (2015) 7:2469–84. doi: 10.3390/nu7042469
122. Wang J, Korber DR, Low NH, Nickerson MT. Encapsulation of Bifidobacterium adolescentis cells with legume proteins and survival under stimulated gastric conditions and during storage in commercial fruit juices. Food Sci Biotechnol. (2015) 24:383–91. doi: 10.1007/s10068-015-0051-x
123. Petreska-Ivanovska T, Petrushevska-Tozi L, Grozdanov A, Petkovska R, Hadjieva J, Popovski E, et al. From optimization of synbiotic microparticles prepared by spray-drying to development of new functional carrot juice. Chem Ind Chem Eng Q. (2014) 20:549–64. doi: 10.2298/CICEQ130218036P
124. Ying D, Schwander S, Weerakkody R, Sanguansri L, Gantenbein-Demarchi C, Augustin MA. Microencapsulated Lactobacillus rhamnosus GG in whey protein and resistant starch matrices: Probiotic survival in fruit juice. J Funct Foods. (2013) 5:98–105. doi: 10.1016/j.jff.2012.08.009
125. Nualkaekul S, Lenton D, Cook MT, Khutoryanskiy VV, Charalampopoulos D. Chitosan coated alginate beads for the survival of microencapsulated Lactobacillus plantarum in pomegranate juice. Carbohydr Polym. (2012) 90:1281–7. doi: 10.1016/j.carbpol.2012.06.073
126. Wechtersbach L, Ulrih NP, Cigić B. Liposomal stabilization of ascorbic acid in model systems and in food matrices. LWT-Food Sci Technol. (2012) 45:43–9. doi: 10.1016/j.lwt.2011.07.025
127. Hernandez-Suarez M, Hernandez-Castillo FD, Gallegos-Morales G, Lira-Saldivar RH, Rodríguez-Herrera R, Aguilar CN. Biocontrol of soil fungi in tomato with microencapsulates containing Bacillus subtilis. Am J Agric Biol Sci. (2011) 6:189–95. doi: 10.3844/ajabssp.2011.189.195
128. Marsanasco M, Márquez AL, Wagner JR, Alonso SDV, Chiaramoni NS. Liposomes as vehicles for vitamins E and C: An alternative to fortify orange juice and offer vitamin C protection after heat treatment. Food Res Int. (2011) 44:3039–46. doi: 10.1016/j.foodres.2011.07.025
129. Tsen JH, Lin YP, Huang HY, King VAE. Studies on the fermentation of tomato juice by using κ-carrageenan immobilized Lactobacillus acidophilus. J Food Process Preserv. (2008) 32:178–89. doi: 10.1111/j.1745-4549.2008.00191.x
130. Gulzar S, Benjakul S, Hozzein WN. Impact of β-glucan on debittering, bioaccessibility and storage stability of skim milk fortified with shrimp oil nanoliposomes. Int J Food Sci Technol. (2020) 55:2092–103. doi: 10.1111/ijfs.14452
131. Samadarsi R, Mishra D, Dutta D. Mangiferin nanoparticles fortified dairy beverage as a low glycemic food product: its quality attributes and antioxidant properties. Int J Food Sci Technol. (2020) 55:589–600. doi: 10.1111/ijfs.14310
132. Jafari SM, Vakili S, Dehnad D. Production of a functional yogurt powder fortified with nanoliposomal vitamin D through spray drying. Food Bioprocess Technol. (2019) 12:1220–31. doi: 10.1007/s11947-019-02289-9
133. Maurya VK, Aggarwal M. Fabrication of nano-structured lipid carrier for encapsulation of vitamin D3 for fortification of ‘Lassi’; A milk based beverage. J Steroid Biochem Mol Biol. (2019) 193:105429. doi: 10.1016/j.jsbmb.2019.105429
134. Golfomitsou I, Mitsou E, Xenakis A, Papadimitriou V. Development of food grade O/W nanoemulsions as carriers of vitamin D for the fortification of emulsion based food matrices: a structural and activity study. J Mol Liquids. (2018) 268:734–42. doi: 10.1016/j.molliq.2018.07.109
135. Ghorbanzade T, Jafari SM, Akhavan S, Hadavi R. Nano-encapsulation of fish oil in nano-liposomes and its application in fortification of yogurt. Food Chem. (2017) 216:146–52. doi: 10.1016/j.foodchem.2016.08.022
136. Kiani A, Fathi M, Ghasemi SM. Production of novel vitamin D3 loaded lipid nanocapsules for milk fortification. Int J Food Properties. (2017) 20:2466–76. doi: 10.1080/10942912.2016.1240690
137. Goyal A, Sharma V, Sihag MK, Singh AK, Arora S, Sabikhi L. Fortification of dahi (Indian yoghurt) with omega-3 fatty acids using microencapsulated flaxseed oil microcapsules. J Food Sci Technol. (2016) 53:2422–33. doi: 10.1007/s13197-016-2220-1
138. Gutiérrez G, Matos M, Barrero P, Pando D, Iglesias O, Pazos C. Iron-entrapped niosomes and their potential application for yogurt fortification. LWT—Food Sci Technol. (2016) 74:550–6. doi: 10.1016/j.lwt.2016.08.025
139. Leskauskaite D, Jasutiene I, Malinauskyte E, Kersiene M, Matusevicius P. Fortification of dairy products with vitamin D3. Int J Dairy Technol. (2016) 69:177–83. doi: 10.1111/1471-0307.12242
140. Onsekizoglu Bagci P, Gunasekaran S. Iron-encapsulated cold-set whey protein isolate gel powder-Part 2: Effect of iron fortification on sensory and storage qualities of Yoghurt. Int J Dairy Technol. (2016) 69:601–8. doi: 10.1111/1471-0307.12316
141. Renuka V, Ramasamy D, Kumar DV. Fortification of omega-3 fatty acids in processed cheese spread. Int J Sci Environ Technol. (2016) 5:2557–65.
142. Feng X, Tjia JYY, Zhou Y, Liu Q, Fu C, Yang H. Effects of tocopherol nanoemulsion addition on fish sausage properties and fatty acid oxidation. LWT—Food Sci Technol. (2020) 118:108737. doi: 10.1016/j.lwt.2019.108737
143. Pourashouri P, Shabanpour B, Kordjazi M, Jamshidi A. Characteristic and shelf life of fish sausage: fortification with fish oil through emulsion and gelled emulsion incorporated with green tea extract. J Sci Food Agric. (2020) 100:4474–82. doi: 10.1002/jsfa.10488
144. Raeisi S, Ojagh SM, Pourashouri P, Salaun F, Quek SY. Shelf-life and quality of chicken nuggets fortified with encapsulated fish oil and garlic essential oil during refrigerated storage. J Food Sci Technol-Mysore. (2020) 58:121–8. doi: 10.1007/s13197-020-04521-3
145. Solomando JC, Antequera T, Perez-Palacios T. Evaluating the use of fish oil microcapsules as omega-3 vehicle in cooked and dry-cured sausages as affected by their processing, storage and cooking. Meat Sci. (2020) 162:108031. doi: 10.1016/j.meatsci.2019.108031
146. Vargas-Ramella M, Pateiro M, Barba FJ, Franco D, Campagnol PCB, Munekata PE, et al. Microencapsulation of healthier oils to enhance the physicochemical and nutritional properties of deer pâté. LWT-Food Sci Technol. (2020). 125:1–7. doi: 10.1016/j.lwt.2020.109223
147. Abbasi F, Samadi F, Jafari SM, Ramezanpour S, Shams-Shargh M. Production of omega-3 fatty acid-enriched broiler chicken meat by the application of nanoencapsultsed flaxseed oil prepared via ultrasonication. J Funct Foods. (2019) 57:373–81. doi: 10.1016/j.jff.2019.04.030
148. Aquilani C, Pérez-Palacios T, Sirtori F, Jiménez-Martín E, Antequera T, Franci O, et al. Enrichment of Cinta Senese burgers with omega-3 fatty acids. Effect of type of addition and storage conditions on quality characteristics. Grasas y Aceites. (2018) 69:e235. doi: 10.3989/gya.0671171
149. Heck RT, Lucas BN, Dos Santos DJP, Pinton MB, Fagundes MB, de Araújo Etchepare M, et al. Oxidative stability of burgers containing chia oil microparticles enriched with rosemary by green-extraction techniques. Meat Sci. (2018) 146:147–53. doi: 10.1016/j.meatsci.2018.08.009
150. Pérez-Palacios T, Ruiz-Carrascal J, Jiménez-Martín E, Solomando JC, Antequera T. Improving the lipid profile of ready-to-cook meat products by addition of omega-3 microcapsules: effect on oxidation and sensory analysis. J Sci Food Agric. (2018) 98:5302–12. doi: 10.1002/jsfa.9069
151. Bolger Z, Brunton NP, Monahan FJ. Effect of mode of addition of flaxseed oil on the quality characteristics of chicken sausage containing vitamin E and omega 3 fatty acids at levels to support a health claim. Food Funct. (2017) 8:3563–75. doi: 10.1039/C7FO00929A
152. Domínguez R, Pateiro M, Agregán R, Lorenzo JM. Effect of the partial replacement of pork backfat by microencapsulated fish oil or mixed fish and olive oil on the quality of frankfurter type sausage. J Food Sci Technol. (2017) 54:26–37. doi: 10.1007/s13197-016-2405-7
153. Jiménez-Martín E, Pérez-Palacios T, Carrascal JR, Rojas TA. Enrichment of chicken nuggets with microencapsulated omega-3 fish oil: effect of frozen storage time on oxidative stability and sensory quality. Food Bioprocess Technol. (2016) 9:285–97. doi: 10.1007/s11947-015-1621-x
154. Lorenzo JM, Munekata PES, Pateiro M, Campagnol PCB, Domínguez R. Healthy Spanish salchichón enriched with encapsulated n−3 long chain fatty acids in konjac glucomannan matrix. Food Res Int. (2016) 89:289–95. doi: 10.1016/j.foodres.2016.08.012
155. Keenan DF, Resconi VC, Smyth TJ, Botinestean C, Lefranc C, Kerry JP, et al. The effect of partial-fat substitutions with encapsulated and unencapsulated fish oils on the technological and eating quality of beef burgers over storage. Meat Sci. (2015) 107:75–85. doi: 10.1016/j.meatsci.2015.04.013
156. Pérez-Chabela ML, Lara-Labastida R, Rodriguez-Huezo E, Totosaus A. Effect of spray drying encapsulation of thermotolerant lactic acid bacteria on meat batters properties. Food Bioprocess Technol. (2013) 6:1505–15. doi: 10.1007/s11947-012-0865-y
157. Calder PC. Omega-3 fatty acids and inflammatory processes: from molecules to man. Biochem Soc Trans. (2017) 45:1105–15. doi: 10.1042/BST20160474
158. McGlory C, Calder P, Nunes EA. The influence of omega-3 fatty acids on skeletal muscle protein turnover in health and disease. Front Nutr. (2019) 6:144. doi: 10.3389/fnut.2019.00144
159. Allam-Ndoul B, Guenard F, Barbier O, Vohl MC. A study of the differential effects of eicosapentaenoic acid (EPA) and docosahexaenoic acid (DHA) on gene expression profiles of stimulated thp-1 macrophages. Nutrients. (2017) 9:424. doi: 10.3390/nu9050424
160. Carlsson JA, Wold AE, Sandberg AS, Ostman SM. The polyunsaturated fatty acids arachidonic acid and docosahexaenoic acid induce mouse dendritic cells maturation but reduce t-cell responses in vitro. PLoS ONE. (2015) 10:0143741. doi: 10.1371/journal.pone.0143741
161. Kolaczkowska E, Kubes P. Neutrophil recruitment and function in health and inflammation. Nat Rev Immunol. (2013) 13:159–75. doi: 10.1038/nri3399
162. Verma DK, Mahato DK, Billoria S, Kapri M, Prabhakar PK, Ajesh Kumar V, et al. Microbial approaches in fermentations for production and preservation of different food. In: Verma DK, Srivastav PP, editors. Microorganisms in Sustainable Agriculture, Food and the Environment. vol. 1. as part of book series on Innovations in AGRICULTURAL MICROBIOLOGY. Florida, FL: Apple Academic Press (2017). p. 105–42.
163. Verma DK, Patel A, Prajapati JB, Al-Manhel AJA, Srivastav PP, et al. Starter culture and probiotic bacteria in dairy food products. In: Verma DK, Patel AR, Srivastav PP, Mohapatra B, Niamah AK, editors. Microbiology for Food and Health Technological Developments and Advances. Florida, FL: Apple Academic Press (2020). p. 3–49.
164. Muzzafar A, Sharma V. Microencapsulation of probiotics for incorporation in cream biscuits. J Food Meas Characterization. (2018) 12:2193–201. doi: 10.1007/s11694-018-9835-z
165. Seyedain-Ardabili M, Sharifan A, Ghiassi Tarzi B. The production of synbiotic bread by microencapsulation. Food Technol Biotechnol. (2016) 54:52–9. doi: 10.17113/ftb.54.01.16.4234
166. Wu HJ, Wu E. The role of gut microbiota in immune homeostasis and autoimmunity. Gut Microbes. (2012) 3:4–14. doi: 10.4161/gmic.19320
167. Galdeano CM, Cazorla SI, Dumit JML, Vélez E, Perdigón G. Beneficial effects of probiotic consumption on the immune system. Ann Nutr Metab. (2019) 74:115–24. doi: 10.1159/000496426
168. Shah NP. Functional cultures and health benefits. Int Dairy J. (2007) 17:1262–77. doi: 10.1016/j.idairyj.2007.01.014
169. Zhang H, Yeh C, Jin Z, Ding L, Liu BY, Zhang L, et al. Prospective study of probiotic supplementation results in immune stimulation and improvement of upper respiratory infection rate. Synthetic Syst Biotechnol. (2018) 3:113–20. doi: 10.1016/j.synbio.2018.03.001
170. Anal AK, Singh H. Recent advances in microencapsulation of probiotics for industrial applications and targeted delivery. Trends Food Sci Technol. (2007) 18:240–51. doi: 10.1016/j.tifs.2007.01.004
171. Burgain J, Gaiani C, Linder M, Scher J. Encapsulation of probiotic living cells: From laboratory scale to industrial applications. J Food Eng. (2011) 104:467–83. doi: 10.1016/j.jfoodeng.2010.12.031
172. Ephrem E, Najjar A, Charcosset C, Greige-Gerges H. Encapsulation of natural active compounds, enzymes, and probiotics for fruit juice fortification, preservation, and processing: An overview. J Funct Foods. (2018) 48:65–84. doi: 10.1016/j.jff.2018.06.021
173. Zamora-Ros R, Béraud V, Franceschi S, Cayssials V, Tsilidis KK, Boutron-Ruault MC, et al. Consumption of fruits, vegetables and fruit juices and differentiated thyroid carcinoma risk in the European prospective investigation into cancer and nutrition (EPIC) study. Int J Cancer. (2018) 142:449–59. doi: 10.1002/ijc.30880
174. Dreher ML, Ford NA. A comprehensive critical assessment of increased fruit and vegetable intake on weight loss in Women. Nutrients. (2020) 12:1919. doi: 10.3390/nu12071919
175. Matute A, Tabart J, Cheramy-Bien JP, Pirotte B, Kevers C, Auger C, et al. Compared phenolic compound contents of 22 commercial fruit and vegetable juices: relationship to ex-vivo vascular reactivity and potential in vivo projection. Antioxidants. (2020) 9:92. doi: 10.3390/antiox9020092
177. Chen L, Liu HG, Liu W, Liu J, Liu K, Shang J, et al. Analysis of clinical features of 29 patients with 2019 novel coronavirus pneumonia. Chin J Tuberc Respir Dis. (2020) 43:E005. doi: 10.3760/cma.j.issn.1001-0939.2020.0005
178. Zhou L, Xu H. A clinical study for the efficacy and safety of adalimumab injection in the treatment of patients with severe novel coronavirus pneumonia (COVID-19). Chin Clin Trial Registry. (2020).
179. Li N, Karin M. Is NF-κB the sensor of oxidative stress?. FASEB J. (1999) 13:1137–43. doi: 10.1096/fasebj.13.10.1137
180. Tan PH, Sagoo P, Chan C, Yates JB, Campbell J, Beutelspacher SC, et al. Inhibition of NF-κB and oxidative pathways in human dendritic cells by antioxidative vitamins generates regulatory T cells. J Immunol. (2005) 174:7633–44. doi: 10.4049/jimmunol.174.12.7633
181. Winterbourn CC, Hampton MB. Thiol chemistry and specificity in redox signaling. Free Radic Biol Med. (2008) 45:549–61. doi: 10.1016/j.freeradbiomed.2008.05.004
182. Griffiths HR, Willetts RS, Grant MM, Mistry N, Lunec J, Bevan RJ. In vivo vitamin C supplementation increases phosphoinositol transfer protein expression in peripheral blood mononuclear cells from healthy individuals. Br J Nutr. (2009) 101:1432–9. doi: 10.1017/S0007114508079646
183. Wu D, Meydani SN. Vitamin E. Immune function, and protection against infection. In: Weber P, Birringer M, Blumberg J, Eggersdorfer M, Frank J, editors. Vitamin E in Human Health. Cham: Humana Press (2019). p. 371–84.
184. Moriguchi S, Muraga M. Vitamin E and immunity. Vitam Horm. (2000) 59:305–36. doi: 10.1016/S0083-6729(00)59011-6
185. Rozga M, Cheng FW, Handu D. Effects of probiotics in conditions or infections similar to covid-19 on health outcomes: an evidence analysis center scoping review. J Acad Nutr Diet. (2020). doi: 10.1016/j.jand.2020.07.016
186. Thakur M, Deshpande HW, Bhate MA. Isolation and identification of lactic acid bacteria and their exploration in non-dairy probiotic drink. Int J Curr Microbiol Appl Sci. (2017) 6:1023–30. doi: 10.20546/ijcmas.2017.604.127
187. Sridharan S, Das KMS. A study on suitable non dairy food matrix for probiotic bacteria–a systematic review. Curr Res Nutr Food Sci J. (2019) 7:5–16. doi: 10.12944/CRNFSJ.7.1.02
188. Bansal S, Mangal M, Sharma SK, Gupta RK. Non-dairy based probiotics: A healthy treat for intestine. Crit Rev Food Sci Nutr. (2016) 56:1856–67. doi: 10.1080/10408398.2013.790780
189. Fonteles TV, Costa MGM, de Jesus ALT, Fontes CPML, Fernandes FAN, et al. Stability and quality parameters of probiotic cantaloupe melon juice produced with sonicated juice. Food Bioprocess Technol. (2013) 6:2860–9. doi: 10.1007/s11947-012-0962-y
190. Nosrati R, Hashemiravan M, Talebi M. Fermentation of vegetables juice by probiotic bacteria. Int J Biosci. (2014) 4:171–80. doi: 10.12692/ijb/4.3.171-180
191. Nguyen BT, Bujna E, Fekete N, Tran A, Rezessy-Szabo JM, Prasad R, et al. Probiotic beverage from pineapple juice fermented with Lactobacillus and Bifidobacterium strains. Front Nutr. (2019) 6:54. doi: 10.3389/fnut.2019.00054
192. Mostafa HS, Ali MR, Mohamed RM. Production of a novel probiotic date juice with anti-proliferative activity against Hep-2 cancer cells. Food Sci Technol. (2021). 41:105–15. doi: 10.1590/fst.09920
193. Gupta C, Chawla P, Arora S. Development and evaluation of iron microencapsules for milk fortification. CyTA-J Food. (2015) 13:116–23. doi: 10.1080/19476337.2014.918179
194. Gupta C, Chawla P, Arora S, Tomar SK, Singh AK. Iron microencapsulation with blend of gum arabic, maltodextrin and modified starch using modified solvent evaporation method–milk fortification. Food Hydrocolloids. (2015) 43:622–8. doi: 10.1016/j.foodhyd.2014.07.021
195. Tamjidi F, Nasirpour A, Shahedi M. Rheological characteristics of yogurt enriched with microencapsulated fish oil. J Agric Sci Technol. (2014) 16:1073–82. Available online at: http://jast.modares.ac.ir/article-23-11239-en.html
196. Amine KM, Champagne CP, Raymond Y, St-Gelais D, Britten M, Fustier P, et al. Survival of microencapsulated bifidobacterium longum in cheddar cheese during production and storage. Food Control. (2014) 37:193–9. doi: 10.1016/j.foodcont.2013.09.030
197. Jeffery LE, Burke F, Mura M, Zheng Y, Qureshi OS, Hewison M, et al. 1, 25-Dihydroxyvitamin D3 and IL-2 combine to inhibit T cell production of inflammatory cytokines and promote development of regulatory T cells expressing CTLA-4 and FoxP3. J Immunol. (2009) 183:5458–67. doi: 10.4049/jimmunol.0803217
198. Ginde AA, Mansbach JM, Camargo CA. Association between serum 25-hydroxyvitamin D level and upper respiratory tract infection in the Third National Health and Nutrition Examination Survey. Arch Intern Med. (2009) 169:384–90. doi: 10.1001/archinternmed.2008.560
200. Zhao K, Huang J, Dai D, Feng Y, Liu L, Nie S. Serum iron level as a potential predictor of coronavirus disease 2019 severity and mortality: a retrospective study. Open Forum Infect Dis. (2020) 7:ofaa250. doi: 10.1093/ofid/ofaa250
201. Brigham EP, McCormack MC, Takemoto CM, Matsui EC. Iron status is associated with asthma and lung function in US women. PLoS ONE. (2015) 10:e0117545. doi: 10.1371/journal.pone.0117545
202. Granato D, Branco GF, Cruz AG, Faria JAF, Shah NP. Probiotic dairy foods as functional foods. Compr Rev Food Sci Food Safety. (2010) 9:455–70. doi: 10.1111/j.1541-4337.2010.00120.x
203. Özer B, Kirmaci HA. Technological and health aspects of probiotic cheese. In: Foster RD, editor. Cheese: Types, Nutrition and Consumption. New Yory, NY: Nova Science Publ (2011). p. 1–42.
204. de Preter V, Vanhoutte T, Huys G, Swings J, de Vuyst L, Rutgeerts P, et al. Effects of Lactobacillus casei Shirota, Bifidobacterium breve, and oligofructoseenriched inulin on colonic nitrogen-protein metabolism in healthy humans. Am J Physiol Gastrointest Liver Physiol. (2007) 292:G358–68. doi: 10.1152/ajpgi.00052.2006
205. Spanhaak S, Havenaar R, Schaafsma G. The effect of consumption of milk fermented by Lactobacillus casei strain Shirota on the intestinal microflora and immune parameters in humans. Eur J Clin Nutr. (1998) 52:899–907. doi: 10.1038/sj.ejcn.1600663
206. Turkmen N, Akal C, Özer B. Probiotic dairy-based beverages: A review. J Funct Foods. (2019) 53:62–75. doi: 10.1016/j.jff.2018.12.004
207. Joe B, Vijaykumar M, Lokesh BR. Biological properties of curcumin-cellular and molecular mechanisms of action. Crit Rev Food Sci Nutr. (2004) 44:97–111. doi: 10.1080/10408690490424702
208. Aggarwal BB, Sundaram C, Malani N, Ichikawa H. Curcumin: the Indian solid gold. In: Aggarwal BB, Surh YJ, Shishodia S, editors. The Molecular Targets and Therapeutic Uses of Curcumin in Health and Disease. Boston, MA: Springer (2007). p. 1–75.
209. Aggarwal BB, Harikumar KB. Potential therapeutic effects of curcumin, the anti-inflammatory agent, against neurodegenerative, cardiovascular, pulmonary, metabolic, autoimmune and neoplastic diseases. Int J Biochem Cell Biol. (2009) 41:40–59. doi: 10.1016/j.biocel.2008.06.010
210. Boroumand N, Samarghandian S, Hashemy SI. Immunomodulatory, anti-inflammatory, and antioxidant effects of curcumin. J Herbmed Pharmacol. (2018) 7:211–9. doi: 10.15171/jhp.2018.33
211. Momtazi-Borojeni AA, Haftcheshmeh SM, Esmaeili SA, Johnston TP, Abdollahi E, Sahebkar A. Curcumin: a natural modulator of immune cells in systemic lupus erythematosus. Autoimmunity Rev. (2018) 17:125–35. doi: 10.1016/j.autrev.2017.11.016
212. Kim GY, Kim KH, Lee SH, Yoon MS, Lee HJ, Moon DO, et al. Curcumin inhibits immunostimulatory function of dendritic cells: MAPKs and translocation of NF-κB as potential targets. J Immunol. (2005) 174:8116–24. doi: 10.4049/jimmunol.174.12.8116
213. Catanzaro M, Corsini E, Rosini M, Racchi M, Lanni C. Immunomodulators inspired by nature: a review on curcumin and echinacea. Molecules. (2018) 23:2778. doi: 10.3390/molecules23112778
214. Alagawany M, Ashour EA, Reda FM. Effect of Dietary Supplementation of garlic (Allium sativum) and Turmeric (Curcuma longa) on growth performance, carcass traits, blood profile and oxidative status in growing rabbits. Ann Anim Sci. (2016) 6:489–505. doi: 10.1515/aoas-2015-0079
215. Livney YD. Milk proteins as vehicles for bioactives. Curr Opin Colloid Interface Sci. (2010) 15:73–83. doi: 10.1016/j.cocis.2009.11.002
216. Yazdi SR, Bonomi F, Iametti S, Miriani M, Brutti A, Corredig M. Binding of curcumin to milk proteins increases after static high pressure treatment of skim milk. J Dairy Res. (2013) 80:152–8. doi: 10.1017/S0022029913000125
217. Solghi S, Emam-Djomeh Z, Fathi M, Farahani F. The encapsulation of curcumin by whey protein: Assessment of the stability and bioactivity. J Food Process Eng. (2020) 43:e13403. doi: 10.1111/jfpe.13403
218. MAGOI (Ministry of Ayush Ministry of Ayush Government of India) (2020). Ayurveda's immunity boosting measures for self-care during COVID 19 crisis. Ministry of Ayush, Government of India. Available online at: https://www.ayush.gov.in/docs/123.pdf (accessed September 12, 2020).
219. Salabei JK, Conklin DJ. Cardiovascular autophagy: crossroads of pathology, pharmacology and toxicology. Cardiovasc Toxicol. (2013) 13:220–9. doi: 10.1007/s12012-013-9200-8
220. Zahedipour F, Hosseini SA, Sathyapalan T, Majeed M, Jamialahmadi T, Al-Rasadi K, et al. Potential effects of curcumin in the treatment of COVID-19 infection. Phytother Res. (2020) 2020:1–10. doi: 10.1002/ptr.6738
221. Ramachandraiah K, Choi MJ, Hong GP. Micro- and nano-scaled materials for strategy-based applications in innovative livestock products: a review. Trends Food Sci Technol. (2018) 71:25–35. doi: 10.1016/j.tifs.2017.10.017
222. Das AK, Nanda PK, Bandyopadhyay S, Banerjee R, Biswas S, McClements DJ. Application of nanoemulsion-based approaches for improving the quality and safety of muscle foods: a comprehensive review. Compr Rev Food Sci Food Safety. (2020) 19:2677–700. doi: 10.1111/1541-4337.12604
223. Nölle N, Genschick S, Schwadorf K, Hrenn H, Brandner S, Biesalski HK. Fish as a source of (micro) nutrients to combat hidden hunger in Zambia. Food Security. (2020) 12:1385–406. doi: 10.1007/s12571-020-01060-9
224. Hayes JE, Stepanyan V, Allen P, O'Grady MN, Kerry JP. Evaluation of the effects of selected plant-derived nutraceuticals on the quality and shelf-life stability of raw and cooked pork sausages. LWT—Food Sci Technol. (2011) 44:164–72. doi: 10.1016/j.lwt.2010.05.020
225. Banerjee DK, Das AK, Banerjee R, Pateiro M, Nanda PK, Gadekar YP, et al. Application of enoki mushroom (Flammulina velutipes) stem wastes as functional ingredients in processed meat. Foods. (2020) 9:432. doi: 10.3390/foods9040432
226. Jin W, Xu W, Liang H, Li Y, Liu S, Li B. Nanoemulsions for food: properties, production, characterization, and applications. In: Grumezescu AM, editors. Emulsions. Cambridge, MA: Academic Press (2016). p. 1–36.
227. Barazzoni R, Bischoff SC, Breda J, Wickramasinghe K, Krznaric Z, Nitzan D, et al. ESPEN expert statements and practical guidance for nutritional management of individuals with SARS-CoV-2 infection. Clin Nutr. (2020) 39:1631–8. doi: 10.1016/j.clnu.2020.03.022
228. Saito S, Okamoto Y, Kawabata J. Effects of alcoholic solvents on antiradical abilities of protocatechuic acid and its alkyl esters. Biosci Biotechnol Biochem. (2004) 68:1221–7. doi: 10.1271/bbb.68.1221
229. Garrido MD, Auqui M, Martí N, Linares MB. Effect of two different red grape pomace extracts obtained under different extraction systems on meat quality of pork burgers. LWT-Food Sci Technol. (2011) 44:2238–43. doi: 10.1016/j.lwt.2011.07.003
230. Vaithiyanathan S, Naveena BM, Muthukumar M, Girish PS, Kondaiah N. Effect of dipping in pomegranate (Punica granatum) fruit juice phenolic solution on the shelf life of chicken meat under refrigerated storage (4 C). Meat Sci. (2011) 88:409–14. doi: 10.1016/j.meatsci.2011.01.019
231. Biswas AK, Chatli MK, Sahoo J. Antioxidant potential of curry (Murraya koenigii L.) and mint (Mentha spicata) leaf extracts and their effect on colour and oxidative stability of raw ground pork meat during refrigeration storage. Food Chem. (2012) 133:467–72. doi: 10.1016/j.foodchem.2012.01.073
232. Sebranek JG, Sewalt VJH, Robbins K, Houser TA. Comparison of a natural rosemary extract and BHA/BHT for relative antioxidant effectiveness in pork sausage. Meat Sci. (2005) 69:289–96. doi: 10.1016/j.meatsci.2004.07.010
233. Brannan RG. Effect of grape seed extract on physicochemical properties of ground, salted, chicken thigh meat during refrigerated storage at different relative humidity levels. J Food Sci. (2008) 73:C36–C40. doi: 10.1111/j.1750-3841.2007.00588.x
234. Keşkekoglu H, Üren A. Inhibitory effects of pomegranate seed extract on the formation of heterocyclic aromatic amines in beef and chicken meatballs after cooking by four different methods. Meat Sci. (2014) 96:1446–51. doi: 10.1016/j.meatsci.2013.12.004
235. Sonia NS, Mini C, Geethalekshmi PR. Vegetable peels as natural antioxidants for processed foods-a review. Agric Rev. (2016) 37:35–41. doi: 10.18805/ar.v37i1.9262
236. Rafiq S, Kaul R, Sofi SA, Bashir N, Nazir F, Nayik GA. Citrus peel as a source of functional ingredient: A review. J Saudi Soc Agric Sci. (2018) 17:351–8. doi: 10.1016/j.jssas.2016.07.006
237. Ding S, Jiang H, Fang J. Regulation of immune function by polyphenols. J Immunol Res. (2018) 2018:1264074. doi: 10.1155/2018/1264074
238. Paraiso IL, Revel JS, Stevens JF. Potential use of polyphenols in the battle against COVID-19. Curr Opin Food Sci. (2020) 32:149–55. doi: 10.1016/j.cofs.2020.08.004
239. Srinivasan K. Antioxidant potential of spices and their active constituents. Crit Rev Food Sci Nutr. (2014) 54:352–72. doi: 10.1080/10408398.2011.585525
Keywords: bioactive compounds, curcumin, probiotics, COVID-19, SARS-CoV-2, encapsulated food, human immune system, immunological activity
Citation: Tripathy S, Verma DK, Thakur M, Patel AR, Srivastav PP, Singh S, Chávez-González ML and Aguilar CN (2021) Encapsulated Food Products as a Strategy to Strengthen Immunity Against COVID-19. Front. Nutr. 8:673174. doi: 10.3389/fnut.2021.673174
Received: 26 February 2021; Accepted: 26 April 2021;
Published: 21 May 2021.
Edited by:
Brandt D. Pence, University of Memphis, United StatesReviewed by:
Shima Shahjouei, Geisinger Health System, United StatesJashbhai B. Prajapati, Anand Agricultural University, India
Copyright © 2021 Tripathy, Verma, Thakur, Patel, Srivastav, Singh, Chávez-González and Aguilar. This is an open-access article distributed under the terms of the Creative Commons Attribution License (CC BY). The use, distribution or reproduction in other forums is permitted, provided the original author(s) and the copyright owner(s) are credited and that the original publication in this journal is cited, in accordance with accepted academic practice. No use, distribution or reproduction is permitted which does not comply with these terms.
*Correspondence: Deepak Kumar Verma, cmFqYWRrdiYjeDAwMDQwO3JlZGlmZm1haWwuY29t; Cristobal N. Aguilar, Y3Jpc3RvYmFsLmFndWlsYXImI3gwMDA0MDt1YWRlYy5lZHUubXg=