- 1Guang'anmen Hospital, Chinese Academy of Chinese Medical Sciences, Beijing, China
- 2Beijing University of Chinese Medicine, Beijing, China
- 3Key Laboratory of Chinese Internal Medicine of the Ministry of Education, Dongzhimen Hospital Affiliated to Beijing University of Chinese Medicine, Beijing, China
Intermittent fasting (IF) has gained attention as a promising diet for weight loss and dysmetabolic diseases management. This systematic review aimed to investigate the effects of IF on cardiometabolic risk factors (CMRFs). A systematic literature search was carried out using three electronic databases, namely PubMed, Embase, and the Cochrane Library, until October 2020. Randomized controlled trials that compared the IF intervention with a control group diet were included. Fourteen effect sizes were expressed as weighted mean difference (WMD) using a fixed-effects model and 95% confidence intervals (CI). Compared to the ones within control groups, participants exposed to the IF intervention reduced their body weight (WMD, −1.78 kg; 95% CI, −2.21 to −1.35; p <0.05), waist circumference (WMD, −1.19 cm; 95% CI, −1.8 to −0.57; p <0.05), fat mass (WMD, −1.26 kg; 95% CI, −1.57 to −0.95; p <0.05), body mass index (WMD, −0.58 kg/m2; 95% CI, −0.8 to −0.37; p <0.05), systolic blood pressure (WMD, −2.14 mmHg; 95% CI: −3.54 to −0.73; p <0.05), diastolic blood pressure (WMD: −1.38 mmHg, 95% CI, −2.35 to −0.41, p <0.05), fasting blood glucose (WMD: −0.053 mmol/L; 95% CI: −0.105 to 0.001; p <0.05), fasting insulin (WMD, −0.8 mIU/L; 95% CI, −1.15 to −0.44; p <0.05), insulin resistance (WMD, −0.21; 95% CI, −0.36 to −0.05; p <0.05), total cholesterol (WMD, −0.10 mmol/L; 95% CI, −0.17 to −0.02; p <0.05), and triglycerides (WMD, −0.09 mmol/L; 95% CI, −0.13 to −0.04; p <0.05). No effects were observed for low-density lipoprotein cholesterol, high-density lipoprotein cholesterol, or glycosylated hemoglobin. This meta-analysis supports the role of IF in improving the component composition of CMRFs, including weight, waist circumference, fat mass, BMI, blood pressure, total cholesterol, triglycerides, fasting insulin, and insulin resistance, compared to a control group diet. Further research on IF interventions should take into account long-term and well-designed administration to draw definitive conclusions.
Introduction
The term “cardiometabolic risk” was first employed by the American Diabetes Association (1) as an umbrella term to include all risk factors for diabetes and cardiovascular disease (CVD) (2). Cardiometabolic risk factors (CMRFs), including obesity, hypertension, diabetes mellitus, and hyperlipidemia, are major contributors to atherosclerosis, ischemic heart disease, stroke, and certain cancers (3). The worldwide burden of cardiometabolic risk has expanded rapidly (4). Mortality from cardiometabolic diseases such as type 2 diabetes mellitus, CVD, and coronary heart disease has increased, and CVD is now the leading cause of death (5). Calorie restriction is an effective management strategy for CMRFs (6). Intermittent fasting (IF), an important measure of energy restriction, also significantly improves metabolic disease (7–10).
IF has gradually come into focus in our daily lives (11). At present, a large number of studies have shown that IF is beneficial in the treatment of metabolic diseases, as well as dietary intervention is easier to accept than drug treatment. Dietary restrictions (12) through IF have been shown to improve metabolic disease risk indicators. Further, IF reportedly plays a considerable role in regulating cardiovascular risk indicators (13), insulin resistance (HOMA-IR), and circulating blood glucose levels (14, 15). There are different types of IF that act as an energy-limiting diet for a specific period, including alternate-day fasting (ADF), alternate-modified-day fasting (AMDF), periodic fasting (PF), time-restricted feeding (TRF), and religious fasting (Table 1). Intermittent energy restriction (IER) is an alternative of IF in this study, and the control group is often a continuous energy restriction (CER).
Over the past three decades, original studies (16, 21) have investigated the impact of IF on a variety of health outcomes, including metabolic disease risk factors, such as weight, blood pressure (BP), waist circumference (WC), body fat, lipid distribution, and blood glucose. However, a recent study by Lowe et al. (22) demonstrated that in the absence of controlled food intake, IF will not play a significant role in weight loss. In turn, it will lead to a reduction in muscle mass. In some randomized crossover trials, IF had no effect on glucose and lipid metabolism (23, 24). These results indicate that the effects of IF on various metabolic factors are contradictory. Therefore, we need a comprehensive and systematic meta-analysis representing all included randomized controlled trials (RCTs), a large sample size, a variety of IF types, and multiple effect indicators to determine the effectiveness of IF interventions in improving health outcomes and modifiable risk factors for people with CMRFs.
Methods
This study used a systematic review and a meta-analysis of PRISMA's preferred reporting items as a guide for reporting research results (25, 26).
Data Source and Search Strategy
Articles were identified by searching through three electronic databases, i.e., PubMed, Embase, and the Cochrane Library until October 2020. Two reviewers (C.L. and X.L.) independently evaluated articles' eligibility, and the inconsistencies shall be made by the corresponding author (F.Y.). The search strategy is described in detail in the Supplementary Table 1.
Inclusion and Exclusion Criteria
The articles had the following characteristics: (1) Type study: RCTs; (2) Participants: participants >18 years; (3) Intervention: different types of IF, including PF, ADF, AMDF, TRF, and part of IER; and (4) Outcomes: data on at least one CMRFs component: body composition (weight, WC, fat mass [FM], and body mass index [BMI]), BP (systolic blood pressure [SBP], diastolic blood pressure [DBP]), lipid panel (total cholesterol [TC], triglycerides (TG), low-density lipoprotein cholesterol [LDL-C], and high-density lipoprotein cholesterol [HDL-C]), glycemic control (fasting blood glucose [FBG], fasting insulin [Fins], glycosylated hemoglobin [HbA1c], and HOMA-IR).
The exclusion criteria were as follows: (1) uncontrolled trials or other study designs; (2) studies lacking a control group; (3) studies without CMRFs component as an outcome and/or lacking sufficient information; (4) non-human samples, reviews, case studies, as well as unpublished abstracts; (5) studies with animal models; (6) pregnant or lactating women; (7) studies in languages other than English; (8) absence of time limits in IER and fasting.
Data Extraction and Study Quality Assessment
Two investigators independently extracted the relevant data from the eligible studies using predesigned forms. Data included study, publication year, country, study design, inclusion and exclusion criteria, total number of participants, participant details, study duration, intervention details and control groups, baseline patient characteristics (mean age, sex), body composition, BP, glycemic control, and lipid panel. Disagreements were resolved by consensus. When necessary, we emailed the corresponding author to acquire study details.
Quality Assessment and Publication Bias
The researchers used Cochrane Collaboration's bias risk tool to evaluate the quality of the methodology included in the studies. According to the criteria of the Cochrane handbook for systematic reviews, the bias risk of each item is classified as low, high, or unclear (27).
Data Statistical and Analysis
Effect estimates were expressed as weighted mean differences (WMD) with a 95% confidence interval (CI). Inter-study heterogeneity was tested using the Higgins I2 statistic, and I2 > 50% indicated significant statistical heterogeneity. The heterogeneity of the study and measurement of effect estimates were determined using the mean and standard deviation (SD) of the differences before and after IF intervention. Publication bias was evaluated using funnel plots; formal testing was conducted with Egger's test (28), and a sensitivity analysis was also performed. We used STATA 16 (StataCorp LLC, College Station, TX, USA) for the statistical analyses.
In order to determine the influence of IF on various effect indicators, it is necessary to change the mean value before and after intervention as well as the SD of the changes. Therefore, we used a method outlined in the Cochrane handbook (27, 29) to determine the SD of changes between time points. SDchange = √[SD SD- (2 × R × SDbaseline× SDfinal)]. In addition, we performed some conversion of data units through international calculation formulas to ensure that results are clinically significant.
Results
Characteristics of Included Studies
The PRISMA statement flow diagram is shown in Figure 1 (30). A total of 9,087 articles were part of the initial database search (PubMed: 6128, EMBASE: 40, Cochrane Library: 2919), after the removal of 1,540 duplicate articles. After filtering the titles and abstracts to exclude irrelevant articles, we found 106 articles that met the topic of interest. The full texts of the 106 records were reviewed. Of them, 60 records were excluded for the following reasons: data not available (n = 15), literature review, letter, or case report (n = 34), unrelated to relevant predictive factors (n = 2), related to protocol (n = 2), and meta-analyses (n = 7). Finally, 46 articles from the database searches were included in the meta-analysis (12, 22, 31–75). A total of 2,681 participants were randomized in the IF intervention group (n = 1,423) and the control group (n = 1,258). The characteristics of the eligible 46 articles with 55 arms are summarized in Supplementary Table 2. All the results calculated using Stata are shown in Table 2.
Risk of Bias and Quality Assessment of Studies
Figure 2 summarizes the risk of bias for RCTs. Twenty-six articles (56%) had a low risk of selection bias. This was because of the intervention type, as no RCT adequately performed blinding of the participants (blinding of dietary interventions is impossible); however, 22 articles (48%) were judged as having a low risk of bias for outcome assessment blinding, 38 articles (82%) were judged as having a low risk of bias for incomplete outcome data, 40 articles (86%) were judged as having a low risk of bias for selective reporting, and 43 articles (93%) showed a low risk of other biases. Overall, 17 articles (37%) were rated with a high risk of bias due to random sequence generation, allocation concealment, outcome assessment blinding, incomplete outcome data, and selective reporting.
Meta-Analysis Results
Effect of IF on Body Composition
Body composition was operationalized in weight, WC, FM, and BMI. Forty-five arms, with 2,225 participants (case = 1,136, control = 1,089), showed a consistent effect of IF on weight (Figure 3A). The fixed-effect analysis showed significant weight reduction (WMD, −1.78 kg, 95% CI: −2.21 to −1.35, p <0.05), thus, indicating significant weight loss. There was no evidence of effect heterogeneity (I2 = 0.0%, p = 0.96). Regarding funnel plot symmetry and Egger's test, p = 0.547 (Figure 4A).
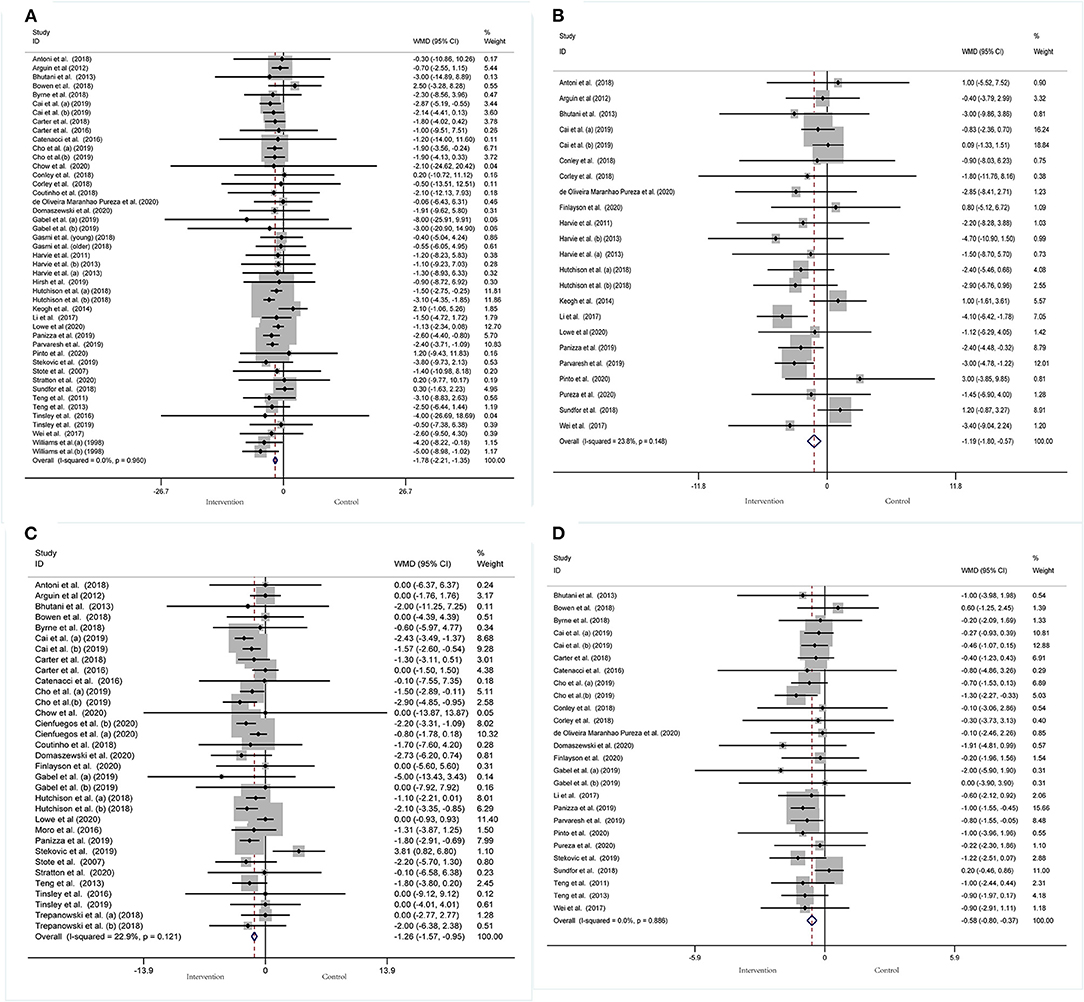
Figure 3. Forest plot of RCTs investigating the effects of intermittent fasting on body composition (A) Weight, (B) WC, (C) FM, (D) BMI.
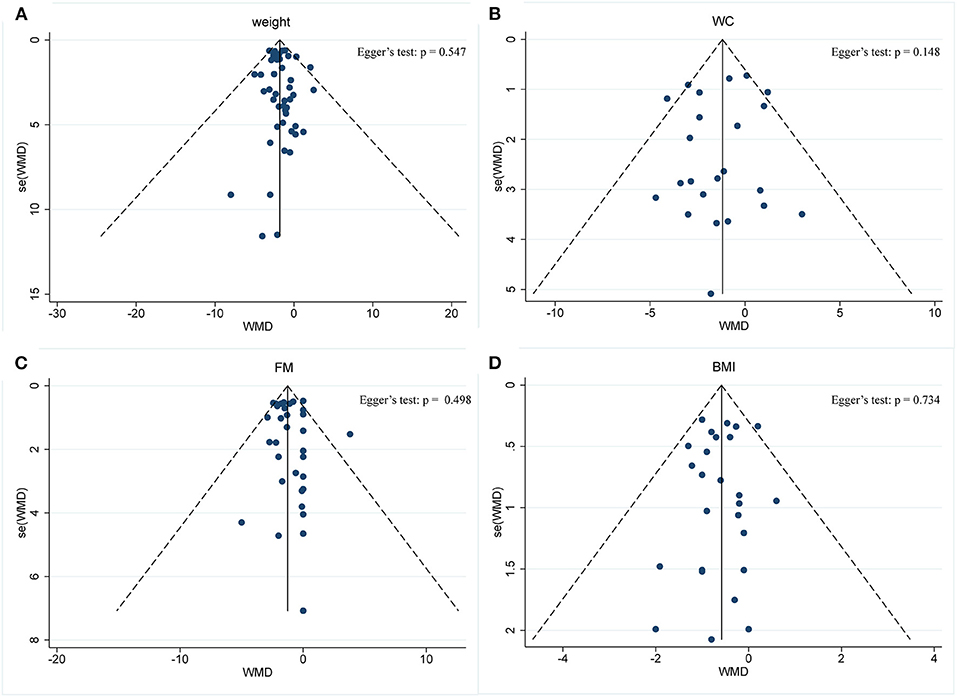
Figure 4. Funnel plot displaying no publication bias in the studies reporting the impact of intermittent fasting on body composition (A) Weight, (B) WC, (C) FM, (D) BMI.
Pooled data from 23 arms (1,385 participants: case = 714, control = 671) showed a consistent effect of IF on WC (Figure 3B). While the fixed-effect analysis showed a significant WC reduction (WMD: −1.19 cm, 95% CI: −1.8 to −0.57, p <0.05), there was also evidence of effect heterogeneity (I2 = 23.8%, p = 0.148). Regarding funnel plot symmetry and Egger's test, p = 0.576 (Figure 4B).
A pooled meta-analysis including 33 arms with 1,610 participants, found a significant effect of IF on FM when compared to placebo (WMD: −1.26 kg, 95% CI: −1.57 to −0.95, p <0.05) (Figure 3C). There was a slight effect heterogeneity (I2 = 22.9%, p = 0.121). Regarding funnel plot symmetry and Egger's test, p = 0.498 (Figure 4C).
The effects of IF on changes in BMI were assessed in 26 RCTs with 1,590 participants (case = 806, control = 784). The results showed a significant effect on the BMI (a fixed-effects model, WMD: −0.58 kg/m2, 95% CI: −0.8 to −0.37, p <0.05) (Figure 3D). There was no evidence of effect heterogeneity (I2 = 0.0%, p = 0.886). Regarding funnel plot symmetry and Egger's test, p = 0.734 (Figure 4D).
Effect of IF on Glycemic Control
A meta-analysis of the effect of IF on glycemic control was performed, including the relevant studies. A cumulative meta-analysis of 34 arms with 1,863 participants (case = 947, control = 916) evaluated changes in FBG during IF (Figure 5A). The WMD was −0.053 mmol/L (95% CI: −0.105 to −0.001, p <0.05, a fixed-effects model), which indicates significant FBG reduction. We observed a moderate effect heterogeneity (I2 = 44.4%, p = 0.003). Regarding funnel plot symmetry and Egger's test, p = 0.502 (Figure 6A).
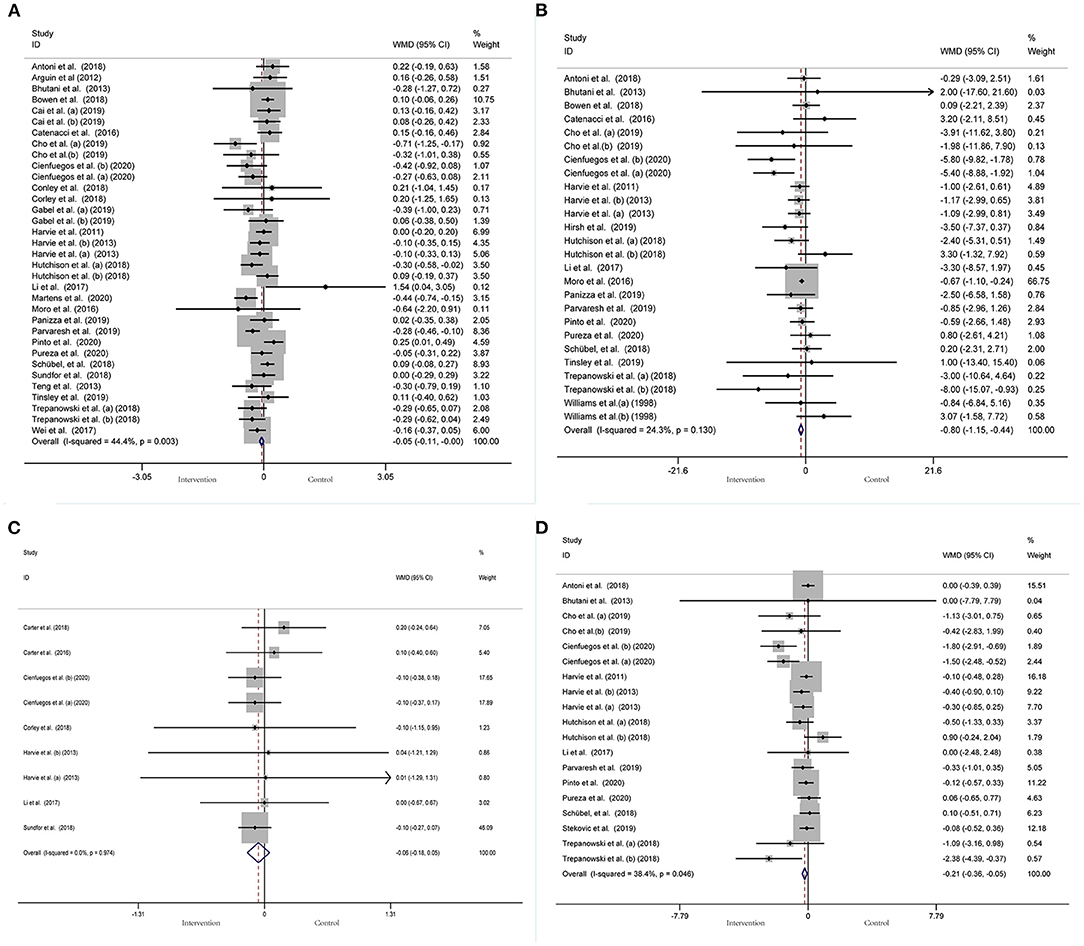
Figure 5. Forest plot of RCTs investigating the effects of intermittent fasting on glycemic control (A) FBG, (B) Fins, (C) HbA1c, (D) HOMA-IR.
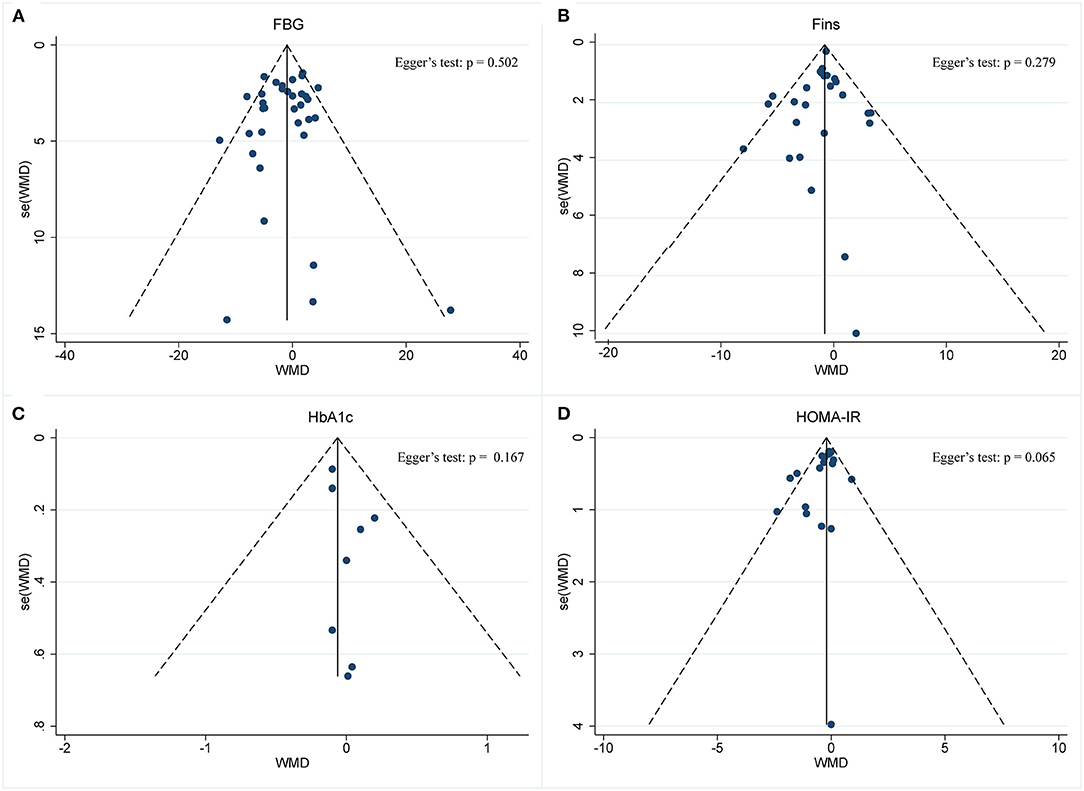
Figure 6. Funnel plot displaying no publication bias in the studies reporting the impact of intermittent fasting on glycemic control (A) FBG, (B) Fins, (C) HbA1c, (D) HOMA-IR.
A pooled meta-analysis including 26 arms with 1,160 participants (case = 584, control = 576) showed significant Fins reduction (WMD: −0.8 mIU/L, 95% CI: −1.15 to −0.44, p <0.05) (Figure 5B). There was evidence of effect heterogeneity (I2 = 24.3%, p = 0.13). Regarding funnel plot symmetry and Egger's test, p = 0.279 (Figure 6B).
A pooled meta-analysis including nine arms with 544 participants (case = 280, control = 264) reported changes in HbA1c during IF (Figure 5C). The WMD was −0.06% (95% CI: −0.18 to 0.05, p > 0.05, a fixed-effects model), which indicates no tangible effect in HbA1c. There was no evidence of effect heterogeneity (I2 = 0.0%, p = 0.974). Regarding funnel plot symmetry and Egger's test, p = 0.167 (Figure 6C).
Pooled data from 19 arms with 866 participants (case = 443, control = 423) reported the effect of IF on HOMA-IR (Figure 5D). The WMD using a fixed-effects model was −0.21 (95% CI: −0.36 to −0.05, p <0.05), which indicates significant HOMA-IR reduction. We observed a mild level of heterogeneity among the studies (I2 = 38.4%, p = 0.046). Regarding funnel plot symmetry and Egger's test, p = 0.065 (Figure 6D).
Effect of IF on BP
BP was operationalized in SBP and DBP. In a pooled meta-analysis including 29 arms with 1,393 participants, we found a tangible effect of IF on SBP level when compared to placebo (a fixed-effects model, WMD: −2.14 mmHg, 95% CI: −3.54 to −0.73, p <0.05) (Figure 7A). We found mild effect heterogeneity (I2 = 36.2%, p = 0.028). Regarding funnel plot symmetry and Egger's test, p = 0.111 (Figure 8A).
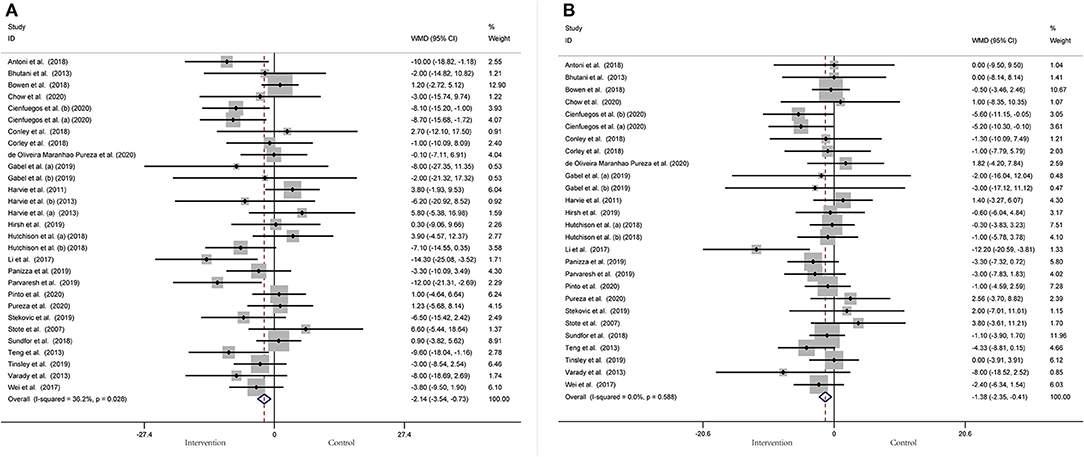
Figure 7. Forest plot of RCTs investigating the effects of intermittent fasting on blood pressure (A) SBP and (B) DBP.
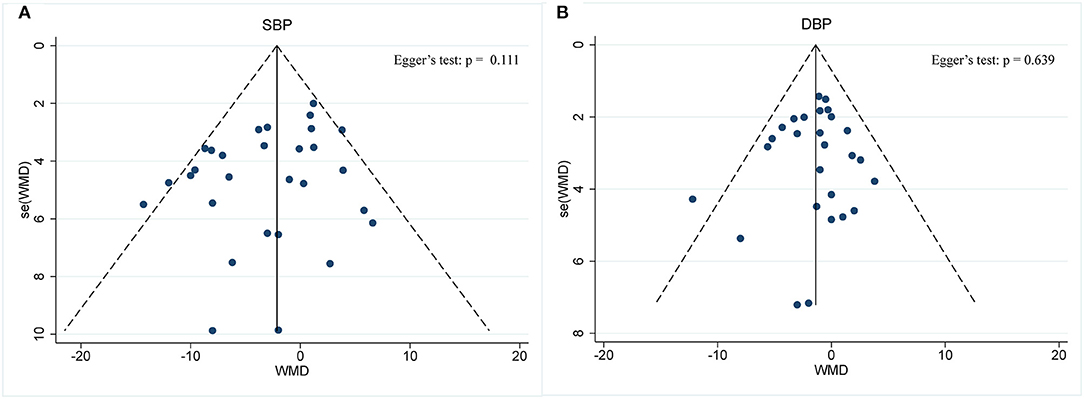
Figure 8. Funnel plot displaying no publication bias in the studies reporting the impact of intermittent fasting on blood pressure (A) SBP and (B) DBP.
Twenty-seven arms with 1,277 participants (case = 640, control = 637) indicated an IF effect on DBP (Figure 7B). The WMD using a fixed-effects model was −1.38 mmHg (95% CI: −2.35 to −0.41, p <0.05), which indicates significant DBP reduction. There was no evidence of effect heterogeneity among studies (I2 = 0.0%, p = 0.588). Regarding funnel plot symmetry and Egger's test, p = 0.639 (Figure 8B).
Effect of IF on Blood Lipid Panel
A meta-analysis of blood lipid levels was performed involving TC, TG, HDL-C, and LDL-C. In 33 arms with 1,766 participants (case = 896, control = 870), a significant reduction in TC concentration (WMD: −0.10 mmol/L, 95% CI: −0.17 to −0.02, p <0.05) (Figure 9A) was observed, with slight effect heterogeneity (I2 =14.6%, p = 0.233). Regarding funnel plot symmetry and Egger's test, p = 0.907 (Figure 10A).
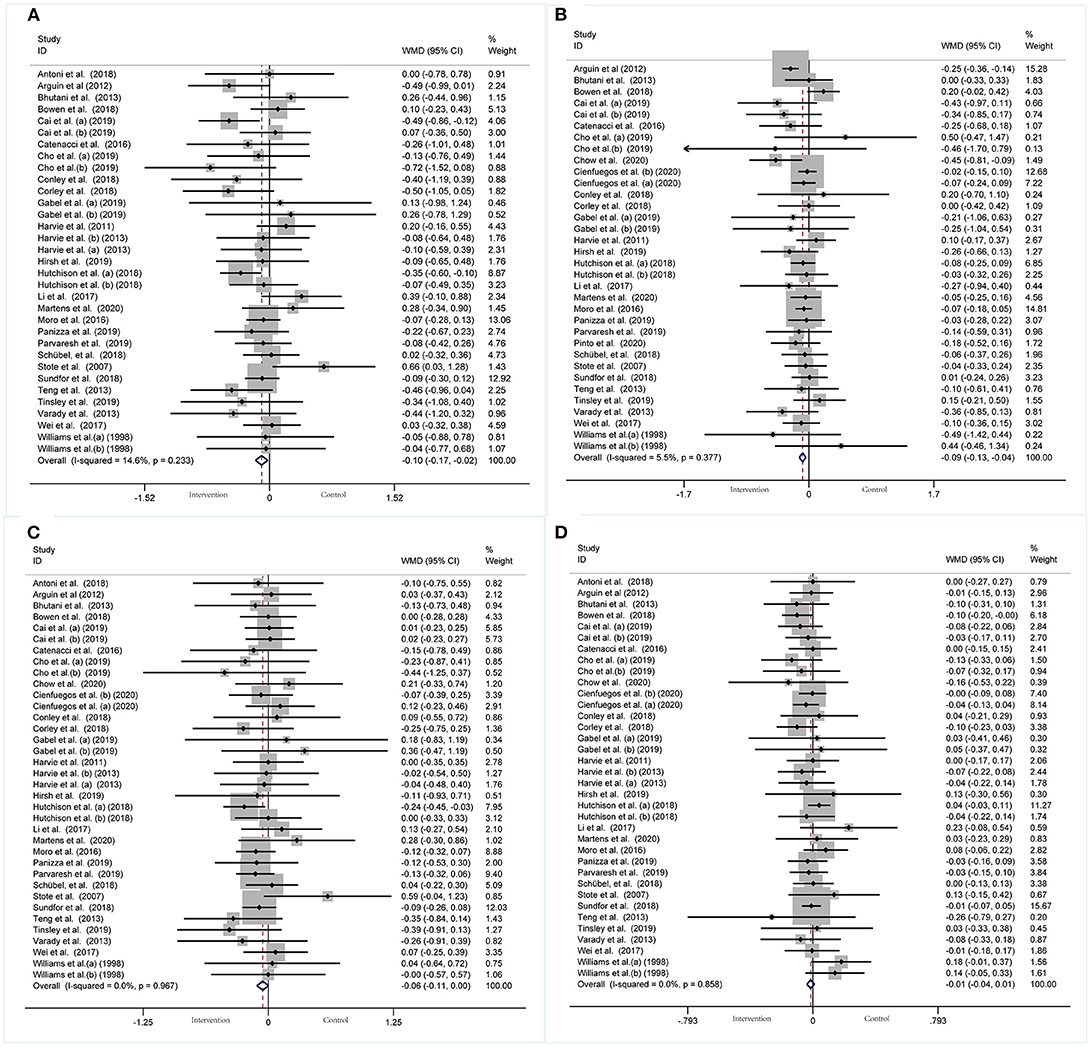
Figure 9. Forest plot of RCTs investigating the effects of intermittent fasting on lipid panel (A) TC, (B) TG, (C) LDL-C, (D) HDL-C.
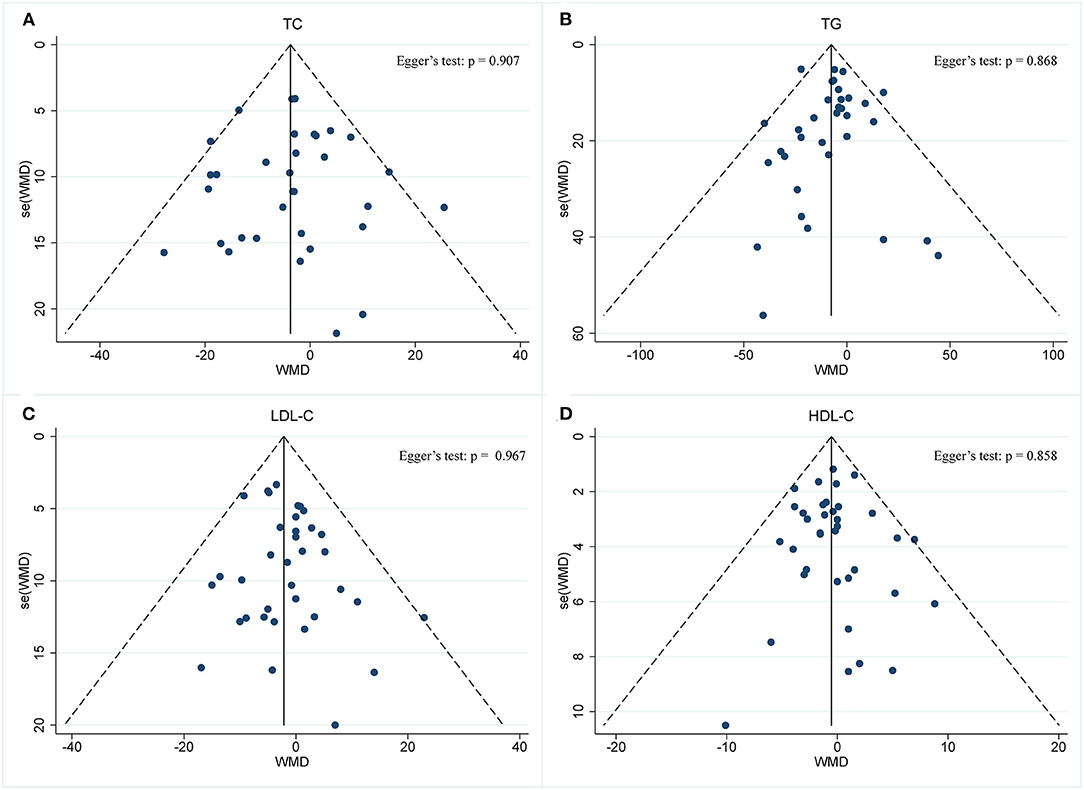
Figure 10. Funnel plot displaying no publication bias in the studies reporting the impact of intermittent fasting on lipid panel (A) TC, (B) TG, (C) LDL-C, (D) HDL-C.
A pooled meta-analysis including 34 arms with 1,750 participants (case = 887, control = 863) evaluated the effect of IF on TG level (Figure 9B). The WMD using a fixed-effects model was −0.09 mmol/L (95% CI: −0.13 to −0.04, p <0.05), which indicates significant TG reduction. There was no evidence of effect heterogeneity among the studies (I2 =5.5%, p = 0.377). Regarding funnel plot symmetry and Egger's test, p = 0.868 (Figure 10B).
A pooled meta-analysis including 36 arms with 1,850 participants (case = 943, control = 907) found no tangible effect of IF on LDL-C concentration (WMD: −0.056 mmol/L, 95% CI: −0.114 to 0.003, p > 0.05) (Figure 9C). There was also no evidence of effect heterogeneity among the studies (I2 =0.0%, p = 0.967). Regarding funnel plot symmetry and Egger's test, p = 0.214 (Figure 10C).
There were 36 RCTs involving 1,852 participants (case =943, control = 909) that evaluated the effect of IF on changes in HDL-C. Data pooling showed no significant changes in HDL-C level (WMD: −0.01 mmol/L, 95% CI: −0.04 to 0.01, p > 0.05) (Figure 9D), with no significant heterogeneity (I2 = 0.0%, p = 0.858). Regarding funnel plot symmetry and Egger's test, p = 0.711 (Figure 10D).
Sensitivity Analysis
In order to determine the impact of each individual study on the effect index, we used a sensitivity analysis in our meta-analysis. Finally, we did not observe the significant effects of any individual study (Supplementary Figures 1–4).
Discussion
In this study, 46 articles with 55 arms were systematically reviewed to evaluate the effects of IF on CMRFs. The pooled analysis showed that IF had significantly reduced body composition (weight, WC, FM, and BMI), BP (SBP, DBP), lipid panel (TC, TG), and improved glycemic control by reducing FBG, Fins, and HOMA-IR; however, it did not affect the HbA1c level and lipid profile (LDL-C and HDL-C).
Comparison With Other Systematic Reviews
Compared with the previous six systematic reviews of IF on cardiac metabolic risk-related indicators, the meta-analysis has obvious distinction and added novel value: First, there were three RCT (76–78) and three non-RCT articles (79–81) in the previous meta-analysis. We included only RCTs that represented the highest level of scientific evidence. Second, there were 30 (77), 19 (79), 28 (80), 10 (76), 15 (81), and 15 articles (78) included in the previous six meta-analyses on IF. We pooled the results of 46 articles to estimate the impact of IF on CMRFs. Third, some studies have focused on metabolic drivers, such as abnormal adipocytokine (81), dysglycemia (76, 77), blood pressure (79), blood lipids (80), and inflammatory factors (78). However, we provided the most comprehensive CMRF indicators, including body weight, blood sugar, blood lipids, and blood pressure. Finally, we also included studies with participants who had a range of subject types, including obese, sedentary, overweight, and healthy-weight adults, as well as those with type 2 diabetes and non-alcoholic liver disease. In addition, we included baseline levels of changes, rather than absolute levels of metabolic indicators, so as to assess the true impact of IF on these parameters, regardless of the health status of participants.
Effects of Body Composition
Overall, in terms of body composition, there was a significant positive correlation between BMI and weight loss during IF (i.e., the higher the starting BMI, the greater the weight loss during the fasting period). This suggests that IF may be more effective for people with a higher BMI. The results for the effect of IF on body composition were similar to those obtained in a previous meta-analysis, by (82), which involved 11 trials that found that TRF was effective in promoting weight loss and reducing FBG compared to not limiting meal times approaches. In addition, IER was more effective in reducing weight than a regular control diet. Moreover, it was also more effective in reducing FM level than CER (17). In a meta-analysis on religious fasting (83), it was found that overweight participants had a greater reduction in weight and percentage of fat than normal people. A recent meta-analysis of RCTs showed that ADF effectively lowered body composition (BMI, weight, and FM) in overweight adults within 6 months compared to the control group (84). However, in another meta-analysis of 12 RCTs, researchers confirmed that lean mass was relatively conserved in the IF group, and no significant weight reduction was identified (59). In addition, a recent study by Lowe et al. (22) on 16:8 time-restricted eating (TRE), an IF plan encouraging the consumption of all dietary intake within an 8-h eating window, demonstrated that IF does not play a significant role in weight loss in the absence of controlled food intake. In turn, it may lead to a reduction in muscle mass. There were no significant differences in FM, Fins, glucose level, HbA1C, or blood lipids between the TRE and control groups. The results of Lowe's study are contrary to the results of most studies related to fasting. This may have been brought about by the time window of fasting. Meantime, our meta-analysis is also included in this study, but a comprehensive assessment of IF in body composition is beneficial.
Effects of Glycemic Control
O'Keefe et al. (85) found that IF habits can improve glucose metabolism as well as reduce abdominal fat accumulation, free radical production, inflammation, and the risks of diabetes, CVD, cancer, and neurodegenerative diseases. After 12 h of fasting, insulin levels drop, liver glycogen reserves are depleted, and the body begins to absorb fatty acids from fat cells to replace glucose for combustion, which improves insulin sensitivity (12, 86). In reality, the effectiveness of IF and energy restriction on the levels of FBG, insulin level, and HOMA-IR in human participants remains inconsistent.
In a recent meta-analysis of RCTs, IF-induced FBG, Fins, and HOMA-IR were more effectively controlled than with a normal diet or CER, which was in keeping with the findings of our study. Moreover, there was also a decrease in HbA1c, but not statistically significant. Compared with our findings, this analysis has a significant effect on reducing FBG and fibrin levels. However, these analyses were based on only 35 arms, whereas we included 55 arms (77). In contrast, in some studies, no significant association was found between IF and FBG (8).
Furthermore, the impaired activity of the IGF-1 axis could be the basis of numerous metabolic, biochemical, and functional alterations that characterize aging and disease (87). In a recent meta-analysis of IF and energy-restricted diets on IGF-1 levels, Rahmani et al. revealed that only fasting regimens and energy restriction to ≤ 50% normal required daily energy intake resulted in significantly reduced levels of plasma IGF-1 in human participants (76).
Effects of Glycemic Control
In the lipid module analysis, the findings of a recent meta-analysis of the effects of IF and energy-limited diet on blood lipids were similar to those in our systematic review. The results showed that, compared with the control group, IF significantly reduced the concentration of TC and TG, but had no meaningful effects on HDL-C concentration (80). However, the two meta-analyses are contradictory in terms of LDL-C levels. The lack of standardization of blood lipid measurement time in individual studies may lead to changes in LDL-C concentrations in some studies. In healthy adults, the longer the week of fasting, the higher the TC, LDL-C, and other indicators (88). This meta-analysis contains the energy limitation of uninterrupted time, which may be the main reason for the difference. Although we did not observe a significant effect of IF on HDL-C concentration, some previous studies have demonstrated the effect of dietary intervention on this lipid marker. Moro et al. demonstrated a small increase in HDL-C concentrations in people who exercised for 8 weeks as part of a normal-calorie diet (43).
Previous studies have shown that IF is not only beneficial in reducing the production of free radicals or weight loss; it also has several health benefits (7, 89–91). IF can cause an evolutionarily conservative adaptive cellular response, improve blood glucose regulation, enhance anti-stress ability, and inhibit inflammation between and within organs. During fasting, cells activate pathways that enhance the body's defense against oxidation and metabolic stress, as well as remove or repair damaged molecules. IF improves the healthy physiological indexes related to metabolism, which lays the foundation for improving the metabolic characteristics of the animal (91, 92). Fat is the main energy source for cells and is stored in the adipose tissue in the form of TGs after meals. During fasting, TGs are broken down into fatty acids and glycerol, which are used to provide energy consumption by the organism (93).
Although not discussed in this study, we found further interesting indicators (leptin and adiponectin secreted by adipocytes) that were used in weight control studies. A meta-analysis by Varkaneh Kord et al. (81) revealed that there was a significant reduction in serum leptin and a non-significant reduction in serum adiponectin following IF. This provides more reference indicators for the effect of IF on metabolic risk.
Effects of Blood Pressure
Blood pressure is an important metabolic index of CVD; thus, any viable mechanism to reduce or manage vascular indices, such as blood pressure, warrants detailed investigation. The reduction of SBP (2.14 mmHg) was observed in our meta-analysis compared with the control group. In the current meta-analysis, IF was slightly more effective in reducing SBP (3.26 mmHg) than energy-restricting diets (1.09 mmHg). The reason for this difference was that, in our study, IF was mainly used in the intervention group, while CER and daily diet were used in the control group.
The available data show that the increase in vascular oxidative stress and vascular inflammation is not only the mechanism of endothelial dysfunction but also the key to the pathogenesis of hypertension (94, 95); and an empirical study showed that fasting reduces hypertension (12, 89, 96). The mechanism of the decreased blood pressure may be the increase in parasympathetic activity due to the brain-derived neurotrophic factor, increased norepinephrine excretion by the kidneys, and increased sensitivity of natriuretic peptides and insulin (97). Cardiovascular health benefits do not persist after termination of the IF diet. After cessation of the diet, the blood pressure values return to their initial values (98).
Although many studies have shown that IF is beneficial to human health and suitable for a wide range of metabolic diseases, this dietary model has rarely been promoted in practice. The main reason for this lack of implementation is that people are accustomed to eating three meals a day. When changing to an IF program, some people feel hungry, irritable, and lose concentration. In addition, IF has side effects in humans. Previous studies have shown that the exercise level of athletes during fasting is extremely decreased due to hypoglycemia (99); there are also disturbances in sleep architecture. A recent study by Lowe et al. (22) demonstrated that in the absence of controlled food intake, IF will lead to a reduction in muscle mass. Incorrect fasting time windows can lead to gastrointestinal diseases. In unhealthy people, using fasting therapy can lead to a decline in physical fitness. Finally, IF includes different fasting methods, time windows, and energy limitations, which require consultation with a dietitian or doctor to adopt an appropriate fasting schedule to ensure the patient's nutritional needs, while gradually reducing the time window for daily eating to prevent side effects, and simultaneously providing continuous counseling and education.
There are some limitations to this meta-analysis. As this is the case with some studies, some of the included ones have a small sample size, and there are several studies with a high risk of bias. Second, the number of long-term studies conducted is very limited, and larger long-term trials with a longer duration are needed to understand the effects of IF on weight loss and long-term weight management. Moreover, different types of IF have different characteristics in various metabolic diseases, and we did not analyze each of them individually. Finally, although IF has a variety of components, a comparison with other types of IF could not be conducted due to the lack of RCT research on religious fasting and lack of data on other kinds of fasting.
Conclusion
This systematic review has demonstrated that IF may improve body composition (weight, WC, FM, and BMI) and moderate BP, TC, TG, and blood glucose, but there may be no difference regarding the LDL-C, HDL-C, and HbA1c levels; components of CMRFs are also risk factors for the development of diabetes and CVDs. Therefore, high-quality and long-term RCTs are needed to provide data on the persistence of the effect and to strengthen the certainty of the evidence.
Data Availability Statement
The original contributions presented in the study are included in the article/Supplementary Material, further inquiries can be directed to the corresponding authors.
Author Contributions
YX and YG designed the manuscript. FY wrote the manuscript. FY, XLiu, and CL searched databases performed the selection of studies. YX and XP revised the manuscript. XLi, LT, and JS critically evaluated the review and commented on it. SY, RZ, NA, and XY contributed in revised version. All authors approved the manuscript for publication.
Funding
This work was supported by the National Key R&D Program of China (grants 2018YFC1704900 and 2018YFC1704901) and the CACMS Innovation Fund (grant number CI2021A00919).
Conflict of Interest
The authors declare that the research was conducted in the absence of any commercial or financial relationships that could be construed as a potential conflict of interest.
Publisher's Note
All claims expressed in this article are solely those of the authors and do not necessarily represent those of their affiliated organizations, or those of the publisher, the editors and the reviewers. Any product that may be evaluated in this article, or claim that may be made by its manufacturer, is not guaranteed or endorsed by the publisher.
Acknowledgments
We express our appreciation to the participants of this study.
Supplementary Material
The Supplementary Material for this article can be found online at: https://www.frontiersin.org/articles/10.3389/fnut.2021.669325/full#supplementary-material
Abbreviations
IF, Intermittent fasting; CMRFs, cardiometabolic risk factors; RCTs, randomized controlled trials; CVDs, cardiovascular diseases; PRISMA, Preferred Reporting Items for Systematic Reviews and Meta-Analyses; CI, confidence interval; WMD, weighted mean difference; ADF, Alternate-day fasting; AMDF, alternate-modified-day fasting; CR, Caloric restriction; TRF, time-restricted feeding; TRE, time-restricted eating; IER, Intermittent energy restriction; CER, Continuous energy restriction; WC, waist circumference; FM, fat mass; BMI, body mass index; SBP, systolic blood pressure; DBP, diastolic blood pressure; TC, total cholesterol; TG, triglyceride; HDL, high-density lipoprotein; LDL, low-density lipoprotein; FBG, fasting blood glucose; Fins, fasting insulin; HbA1c, glycosylated hemoglobin; HOMA-IR, insulin resistance; MetS, metabolic syndrome; VLCD, very low-calorie diet; IECR, Intermittent energy and carbohydrate restriction.
References
1. Eckel RH, Kahn R, Robertson RM, Rizza RA. Preventing cardiovascular disease and diabetes: a call to action from the American Diabetes Association and the American Heart Association. Circulation. (2006) 113:2943–6. doi: 10.1161/CIRCULATIONAHA.106.176583
2. An N, Gao Y, Si Z, Zhang H, Wang L, Tian C, et al. Regulatory Mechanisms of the NLRP3 Inflammasome, a Novel Immune-Inflammatory Marker in Cardiovascular Diseases. Front Immunol. (2019) 10:1592. doi: 10.3389/fimmu.2019.01592
3. Cannon CP. Cardiovascular disease and modifiable cardiometabolic risk factors. Clin Cornerstone. (2007) 8:11–28. doi: 10.1016/S1098-3597(07)80025-1
4. Nichols GA, Horberg M, Koebnick C, Young DR, Waitzfelder B, Sherwood NE, et al. Cardiometabolic risk factors among 1.3 million adults with overweight or obesity, but not diabetes, in 10 geographically diverse regions of the United States, 2012-2013. Prev Chronic Dis. (2017) 14:E22. doi: 10.5888/pcd14.160438
5. Danaei G, Lu Y, Singh GM, Carnahan E, Stevens GA, Cowan MJ, et al. Cardiovascular disease, chronic kidney disease, and diabetes mortality burden of cardiometabolic risk factors from 1980 to 2010: a comparative risk assessment. Lancet Diabetes Endocrinol. (2014) 2:634–47. doi: 10.1016/S2213-8587(14)70102-0
6. Rubin R. Modest calorie reduction can improve cardiometabolic health. JAMA. (2019) 322:1032–3. doi: 10.1001/jama.2019.12314
7. Grundy SM. Metabolic syndrome update. Trends Cardiovasc Med. (2016) 26:364–73. doi: 10.1016/j.tcm.2015.10.004
8. St-Onge MP, Ard J, Baskin ML, Chiuve SE, Johnson HM, Kris-Etherton P, et al. Meal timing and frequency: implications for cardiovascular disease prevention: a scientific statement from the American Heart Association. Circulation. (2017) 135:e96–121. doi: 10.1161/CIR.0000000000000476
9. Cioffi I, Evangelista A, Ponzo V, Ciccone G, Soldati L, Santarpia L, et al. Intermittent versus continuous energy restriction on weight loss and cardiometabolic outcomes: a systematic review and meta-analysis of randomized controlled trials. J Transl Med. (2018) 16:371. doi: 10.1186/s12967-018-1748-4
10. Guo Y, Luo S, Ye Y, Yin S, Fan J, Xia M. Intermittent fasting improves cardiometabolic risk factors and alters gut microbiota in metabolic syndrome patients. J Clin Endocrinol Metab. (2021) 106:64–79. doi: 10.1210/clinem/dgaa644
11. Collier R. Intermittent fasting: the next big weight loss fad. Can Med Assoc. (2013) 185:E321–2. doi: 10.1503/cmaj.109-4437
12. Sutton EF, Beyl R, Early KS, Cefalu WT, Ravussin E, Peterson CM. Early time-restricted feeding improves insulin sensitivity, blood pressure, and oxidative stress even without weight loss in men with prediabetes. Cell Metab. (2018) 27:1212–21.e1213. doi: 10.1016/j.cmet.2018.04.010
13. Kim K, Kim Y, Son J, Lee J, Kim S, Choe M, et al. Intermittent fasting promotes adipose thermogenesis and metabolic homeostasis via VEGF-mediated alternative activation of macrophage. Cell Res. (2017) 27:1309–26. doi: 10.1038/cr.2017.126
14. Haluzík M, Mráz M. Intermittent fasting and prevention of diabetic retinopathy: where do we go from here? Diabetes. (2018) 67:1745–7. doi: 10.2337/dbi18-0022
15. Lamos EM, Malek R, Munir KM. Effects of intermittent fasting on health, aging, and disease. N Engl J Med. (2020) 382:1771. doi: 10.1056/NEJMc2001176
16. Patterson RE, Sears DD. Metabolic effects of intermittent fasting. Annu Rev Nutr. (2017) 37:371–93. doi: 10.1146/annurev-nutr-071816-064634
17. Harris L, Hamilton S, Azevedo LB, Olajide J, De Brun C, Waller G, et al. Intermittent fasting interventions for treatment of overweight and obesity in adults: a systematic review and meta-analysis. JBI Database System Rev Implement Rep. (2018) 16:507–47. doi: 10.11124/JBISRIR-2016-003248
18. Allaf M, Elghazaly H, Mohamed OG, Fareen MFK, Zaman S, Salmasi M, et al. Intermittent fasting for the prevention of cardiovascular disease. Cochrane Database Syst Rev. (2019) 1:CD013496. doi: 10.1002/14651858.CD013496
19. Adler-Lazarovits C, Weintraub A. Physicians' attitudes and views regarding religious fasting during pregnancy and review of the literature. Europ J Obst Gynecol Reproduct Biol. (2019) 233:76–80. doi: 10.1016/j.ejogrb.2018.12.015
20. Trepanowski JF, Bloomer RJ. The impact of religious fasting on human health. Nutr J. (2010) 9:57. doi: 10.1186/1475-2891-9-57
21. Stekovic S, Hofer S, Tripolt N, Aon M, Royer P, Pein L, et al. Alternate day fasting improves physiological and molecular markers of aging in healthy, non-obese humans. Cell Metab. (2020) 31:878–81. doi: 10.1016/j.cmet.2020.02.011
22. Lowe DA, Wu N, Rohdin-Bibby L, Moore AH, Kelly N, Liu YE, et al. Effects of time-restricted eating on weight loss and other metabolic parameters in women and men with overweight and obesity: the TREAT randomized clinical trial. JAMA Intern Med. (2020) 180:1491–9. doi: 10.1001/jamainternmed.2020.4153
23. Carlson O, Martin B, Stote KS, Golden E, Maudsley S, Najjar SS, et al. Impact of reduced meal frequency without caloric restriction on glucose regulation in healthy, normal-weight middle-aged men and women. Metabolism. (2007) 56:1729–34. doi: 10.1016/j.metabol.2007.07.018
24. Soeters MR, Lammers NM, Dubbelhuis PF, Ackermans M, Jonkers-Schuitema CF, Fliers E, et al. Intermittent fasting does not affect whole-body glucose, lipid, or protein metabolism. Am J Clin Nutr. (2009) 90:1244–51. doi: 10.3945/ajcn.2008.27327
25. Guyatt GH, Oxman AD, Vist GE, Kunz R, Falck-Ytter Y, Alonso-Coello P, et al. GRADE: an emerging consensus on rating quality of evidence and strength of recommendations. BMJ. (2008) 336:924–6. doi: 10.1136/bmj.39489.470347.AD
26. McInnes MDF, Moher D, Thombs BD, McGrath TA, Bossuyt PM, Clifford T, et al. Preferred reporting items for a systematic review and meta-analysis of diagnostic test accuracy studies: the PRISMA-DTA statement. JAMA. (2018) 319:388–96. doi: 10.1001/jama.2017.19163
27. Higgins JPT, Thomas J, Chandler J, Cumpston M, Li T, Page MJ, Welch VA editors. Cochrane Handbook for Systematic Reviews of Interventions. 2nd ed. Chichester: John Wiley & Sons (2019).
28. Egger M, Davey Smith G, Schneider M, Minder C. Bias in meta-analysis detected by a simple, graphical test. BMJ. (1997) 315:629–34. doi: 10.1136/bmj.315.7109.629
29. Hozo SP, Djulbegovic B, Hozo I. Estimating the mean and variance from the median, range, and the size of a sample. BMC Med Res Methodol. (2005) 5:13. doi: 10.1186/1471-2288-5-13
30. Moher D, Liberati A, Tetzlaff J, Altman DG. Preferred reporting items for systematic reviews and meta-analyses: the PRISMA statement. PLoS Med. (2009) 6:e1000097. doi: 10.1371/journal.pmed.1000097
31. Williams KV, Mullen ML, Kelley DE, Wing RR. The effect of short periods of caloric restriction on weight loss and glycemic control in type 2 diabetes. Diabetes Care. (1998) 21:2–8. doi: 10.2337/diacare.21.1.2
32. Stote KS, Baer DJ, Spears K, Paul DR, Harris GK, Rumpler WV, et al. A controlled trial of reduced meal frequency without caloric restriction in healthy, normal-weight, middle-aged adults. Am J Clin Nutr. (2007) 85:981–88. doi: 10.1093/ajcn/85.4.981
33. Harvie MN, Pegington M, Mattson MP, Frystyk J, Dillon B, Evans G, et al. The effects of intermittent or continuous energy restriction on weight loss and metabolic disease risk markers: a randomized trial in young overweight women. Int J Obes. (2011) 35:714–27. doi: 10.1038/ijo.2010.171
34. Teng NI, Shahar S, Manaf ZA, Das SK, Taha CS, Ngah WZ. Efficacy of fasting calorie restriction on quality of life among aging men. Physiol Behav. (2011) 104:1059–64. doi: 10.1016/j.physbeh.2011.07.007
35. Arguin H, Dionne IJ, Sénéchal M, Bouchard DR, Carpentier AC, Ardilouze JL, et al. Short- and long-term effects of continuous versus intermittent restrictive diet approaches on body composition and the metabolic profile in overweight and obese postmenopausal women: a pilot study. Menopause. (2012) 19:870–6. doi: 10.1097/gme.0b013e318250a287
36. Bhutani S, Klempel MC, Kroeger CM, Trepanowski JF, Varady KA. Alternate day fasting and endurance exercise combine to reduce body weight and favorably alter plasma lipids in obese humans. Obesity. (2013) 21:1370–9. doi: 10.1002/oby.20353
37. Harvie M, Wright C, Pegington M, McMullan D, Mitchell E, Martin B, et al. The effect of intermittent energy and carbohydrate restrictionv. daily energy restriction on weight loss and metabolic disease risk markers in overweight women. Br J Nutr. (2013) 110:1534–47. doi: 10.1017/S0007114513000792
38. Teng NI, Shahar S, Rajab NF, Manaf ZA, Johari MH, Ngah WZ. Improvement of metabolic parameters in healthy older adult men following a fasting calorie restriction intervention. Aging Male. (2013) 16:177–83. doi: 10.3109/13685538.2013.832191
39. Varady K, Bhutani S, Klempel M, Kroeger C, Trepanowski J, Haus J, et al. Alternate day fasting for weight loss in normal weight and overweight subjects: a randomized controlled trial. Nutr J. (2013) 12:146. doi: 10.1186/1475-2891-12-146
40. Keogh JB, Pedersen E, Petersen KS, Clifton PM. Effects of intermittent compared to continuous energy restriction on short-term weight loss and long-term weight loss maintenance. Clin Obes. (2014) 4:150–56. doi: 10.1111/cob.12052
41. Carter S, Clifton PM, Keogh JB. The effects of intermittent compared to continuous energy restriction on glycaemic control in type 2 diabetes; a pragmatic pilot trial. Diabetes Res Clin Pract. (2016) 122:106–12. doi: 10.1016/j.diabres.2016.10.010
42. Catenacci VA, Pan Z, Ostendorf D, Brannon S, Gozansky WS, Mattson MP, et al. A randomized pilot study comparing zero-calorie alternate-day fasting to daily caloric restriction in adults with obesity. Obesity. (2016) 24:1874–83. doi: 10.1002/oby.21581
43. Moro T, Tinsley G, Bianco A, Marcolin G, Pacelli Q, Battaglia G, et al. Effects of eight weeks of time-restricted feeding (16/8) on basal metabolism, maximal strength, body composition, inflammation, and cardiovascular risk factors in resistance-trained males. J Transl Med. (2016) 14:290. doi: 10.1186/s12967-016-1044-0
44. Tinsley GM, Forsse JS, Butler NK, Paoli A, Bane AA, La Bounty PM, et al. Time-restricted feeding in young men performing resistance training: a randomized controlled trial. Europ J Sport Sci. (2016) 17:200–07. doi: 10.1080/17461391.2016.1223173
45. Li C, Sadraie B, Steckhan N, Kessler C, Stange R, Jeitler M, et al. Effects of a one-week fasting therapy in patients with Type-2 diabetes mellitus and metabolic syndrome – a randomized controlled explorative study. Exp Clin Endocrinol Diabetes. (2017) 125:618–24. doi: 10.1055/s-0043-101700
46. Wei M, Brandhorst S, Shelehchi M, Mirzaei H, Cheng C, Budniak J, et al. Fasting-mimicking diet and markers/risk factors for aging, diabetes, cancer, cardiovascular disease. Sci Transl Med. (2017) 9:eaai8700. doi: 10.1007/978-94-024-1045-7
47. Antoni R, Johnston KL, Collins AL, Robertson MD. Intermittent v. continuous energy restriction: differential effects on postprandial glucose and lipid metabolism following matched weight loss in overweight/obese participants. Br J Nutr. (2018) 119:507–16. doi: 10.1017/S0007114517003890
48. Bowen J, Brindal E, James-Martin G, Noakes M. Randomized trial of a high protein, partial meal replacement program with or without alternate day fasting: similar effects on weight loss, retention status, nutritional, metabolic, behavioral outcomes. Nutrients. (2018) 10:1145. doi: 10.3390/nu10091145
49. Byrne NM, Sainsbury A, King NA, Hills AP, Wood RE. Intermittent energy restriction improves weight loss efficiency in obese men: the MATADOR study. Int J Obes. (2018) 42:129–38. doi: 10.1038/ijo.2017.206
50. Carter S, Clifton PM, Keogh JB. Effect of intermittent compared with continuous energy restricted diet on glycemic control in patients with Type 2 diabetes: a randomized noninferiority trial. JAMA Netw Open. (2018) 1:e180756. doi: 10.1001/jamanetworkopen.2018.0756
51. Conley M, Le Fevre L, Haywood C, Proietto J. Is two days of intermittent energy restriction per week a feasible weight loss approach in obese males? A randomised pilot study. Nutr Diet. (2018) 75:65–72. doi: 10.1111/1747-0080.12372
52. Corley BT, Carroll RW, Hall RM, Weatherall M, Parry-Strong A, Krebs JD. Intermittent fasting in Type 2 diabetes mellitus and the risk of hypoglycaemia: a randomized controlled trial. Diabet Med. (2018) 35:588–94. doi: 10.1111/dme.13595
53. Coutinho SR, Halset EH, Gåsbakk S, Rehfeld JF, Kulseng B, Truby H, et al. Compensatory mechanisms activated with intermittent energy restriction: a randomized control trial. Clin Nutr. (2018) 37:815–23. doi: 10.1016/j.clnu.2017.04.002
54. Gasmi M, Sellami M, Denham J, Padulo J, Kuvacic G, Selmi W, et al. Time-restricted feeding influences immune responses without compromising muscle performance in older men. Nutrition. (2018) 51-52:29–37. doi: 10.1016/j.nut.2017.12.014
55. Schübel R, Nattenmüller J, Sookthai D, Nonnenmacher T, Graf ME, Riedl L, et al. Effects of intermittent and continuous calorie restriction on body weight and metabolism over 50 wk: a randomized controlled trial. Am J Clin Nutr. (2018) 108:933–45. doi: 10.1093/ajcn/nqy196
56. Sundfør TM, Svendsen M, Tonstad S. Effect of intermittent versus continuous energy restriction on weight loss, maintenance and cardiometabolic risk: a randomized 1-year trial. Nutr Metab Cardiovasc Dis. (2018) 28:698–706. doi: 10.1016/j.numecd.2018.03.009
57. Trepanowski JF, Kroeger CM, Barnosky A, Klempel M, Bhutani S, Hoddy KK, et al. Effects of alternate-day fasting or daily calorie restriction on body composition, fat distribution, and circulating adipokines: secondary analysis of a randomized controlled trial. Clin Nutr. (2018) 37:1871–8. doi: 10.1016/j.clnu.2017.11.018
58. Cai H, Qin YL, Shi ZY, Chen JH, Zeng MJ, Zhou W, et al. Effects of alternate-day fasting on body weight and dyslipidaemia in patients with non-alcoholic fatty liver disease: a randomised controlled trial. BMC Gastroenterol. (2019) 19:219. doi: 10.1186/s12876-019-1132-8
59. Cho AR, Moon JY, Kim S, An KY, Oh M, Jeon JY, et al. Effects of alternate day fasting and exercise on cholesterol metabolism in overweight or obese adults: a pilot randomized controlled trial. Metabolism. (2019) 93:52–60. doi: 10.1016/j.metabol.2019.01.002
60. Gabel K, Kroeger CM, Trepanowski JF, Hoddy KK, Cienfuegos S, Kalam F, et al. Differential effects of alternate-day fasting versus daily calorie restriction on insulin resistance. Obesity. (2019) 27:1443–50. doi: 10.1002/oby.22564
61. Hirsh SP, Pons M, Joyal SV, Swick AG. Avoiding holiday seasonal weight gain with nutrient-supported intermittent energy restriction: a pilot study. J Nutr Sci. (2019) 8:e11. doi: 10.1017/jns.2019.8
62. Hutchison AT, Liu B, Wood RE, Vincent AD, Thompson CH, O'Callaghan NJ, et al. Effects of intermittent versus continuous energy intakes on insulin sensitivity and metabolic risk in women with overweight. Obesity. (2019) 27:50–58. doi: 10.1002/oby.22345
63. Panizza CE, Lim U, Yonemori KM, Cassel KD, Wilkens LR, Harvie MN, et al. Effects of intermittent energy restriction combined with a mediterranean diet on reducing visceral adiposity: a randomized active comparator pilot study. Nutrients. (2019) 11:1386. doi: 10.3390/nu11061386
64. Parvaresh A, Razavi R, Abbasi B, Yaghoobloo K, Hassanzadeh A, Mohammadifard N, et al. Modified alternate-day fasting vs. calorie restriction in the treatment of patients with metabolic syndrome: A randomized clinical trial. Complement Therap Med. (2019) 47:102187. doi: 10.1016/j.ctim.2019.08.021
65. Stekovic S, Hofer SJ, Tripolt N, Aon MA, Royer P, Pein L, et al. Alternate day fasting improves physiological and molecular markers of aging in healthy, non-obese humans. Cell Meta. (2019) 30:462–76.e466. doi: 10.1016/j.cmet.2019.07.016
66. Tinsley GM, Moore ML, Graybeal AJ, Paoli A, Kim Y, Gonzales JU, et al. Time-restricted feeding plus resistance training in active females: a randomized trial. Am J Clin Nutr. (2019) 110:628–40. doi: 10.1093/ajcn/nqz126
67. Chow LS, Manoogian ENC, Alvear A, Fleischer JG, Thor H, Dietsche K, et al. Time-restricted eating effects on body composition and metabolic measures in humans who are overweight: a feasibility study. Obesity. (2020) 28:860–9. doi: 10.1002/oby.22756
68. Cienfuegos S, Gabel K, Kalam F, Ezpeleta M, Wiseman E, Pavlou V, et al. Effects of 4- and 6-h time-restricted feeding on weight and cardiometabolic health: a randomized controlled trial in adults with obesity. Cell Metab. (2020) 32:366–78 e363. doi: 10.1016/j.cmet.2020.06.018
69. Pureza I, Melo ISV, Macena ML, Praxedes DRS, Vasconcelos LGL, Silva-Júnior AE, et al. Acute effects of time-restricted feeding in low-income women with obesity placed on hypoenergetic diets: randomized trial. Nutrition. (2020) 77:110796. doi: 10.1016/j.nut.2020.110796
70. Domaszewski P, Konieczny M, Pakosz P, Baczkowicz D, Sadowska-Krepa E. Effect of a six-week intermittent fasting intervention program on the composition of the human body in women over 60 years of age. Int J Environ Res Public Health. (2020) 17:4138. doi: 10.3390/ijerph17114138
71. Finlayson G, Blundell J, Varady K, Hopkins M, Gibbons C, Turicchi J, et al. Matched weight loss through intermittent or continuous energy restriction does not lead to compensatory increases in appetite and eating behavior in a randomized controlled trial in women with overweight and obesity. J Nutr. (2020) 150:623–33. doi: 10.1093/jn/nxz296
72. Martens CR, Rossman MJ, Mazzo MR, Jankowski LR, Nagy EE, Denman BA, et al. Short-term time-restricted feeding is safe and feasible in non-obese healthy midlife and older adults. Geroscience. (2020) 42:667–86. doi: 10.1007/s11357-020-00156-6
73. Pinto AM, Bordoli C, Buckner LP, Kim C, Kaplan PC, Del Arenal IM, et al. Intermittent energy restriction is comparable to continuous energy restriction for cardiometabolic health in adults with central obesity: a randomized controlled trial; the Met-IER study. Clin Nutr. (2020) 39:1753–63. doi: 10.1016/j.clnu.2019.07.014
74. Stratton MT, Tinsley GM, Alesi MG, Hester GM, Olmos AA, Serafini PR, et al. Four weeks of time-restricted feeding combined with resistance training does not differentially influence measures of body composition, muscle performance, resting energy expenditure, blood biomarkers. Nutrients. (2020) 12:1126. doi: 10.3390/nu12041126
75. de Oliveira Maranhão Pureza IR da Silva Junior AE Silva Praxedes DR Lessa Vasconcelos LG de Lima Macena M Vieira de Melo IS . Effects of time-restricted feeding on body weight, body composition and vital signs in low-income women with obesity: a 12-month randomized clinical trial. Clin Nutr. (2020) 40:759–66. doi: 10.1016/j.clnu.2020.06.036
76. Rahmani J, Kord Varkaneh H, Clark C, Zand H, Bawadi H, Ryan PM, et al. The influence of fasting and energy restricting diets on IGF-1 levels in humans: a systematic review and meta-analysis. Ageing Res Rev. (2019) 53:100910. doi: 10.1016/j.arr.2019.100910
77. Fatahi S, Nazary-Vannani A, Sohouli MH, Mokhtari Z, Kord-Varkaneh H, Moodi V, et al. The effect of fasting and energy restricting diets on markers of glucose and insulin controls: a systematic review and meta-analysis of randomized controlled trials. Crit Rev Food Sci Nutr. (2020) 1–12. doi: 10.1080/10408398.2020.1798350
78. Wang X, Yang Q, Liao Q, Li M, Zhang P, Santos HO, et al. Effects of intermittent fasting diets on plasma concentrations of inflammatory biomarkers: a systematic review and meta-analysis of randomized controlled trials. Nutrition. (2020) 79–80:110974. doi: 10.1016/j.nut.2020.110974
79. Kord-Varkaneh H, Nazary-Vannani A, Mokhtari Z, Salehi-Sahlabadi A, Rahmani J, Clark CCT, et al. The influence of fasting and energy restricting diets on blood pressure in humans: a systematic review and meta-analysis. High Blood Press Cardiovasc Prev. (2020) 27:271–80. doi: 10.1007/s40292-020-00391-0
80. Meng H, Zhu L, Kord-Varkaneh H, H OS, Tinsley GM, Fu P. Effects of intermittent fasting and energy-restricted diets on lipid profile: a systematic review and meta-analysis. Nutrition. (2020) 77:110801. doi: 10.1016/j.nut.2020.110801
81. Varkaneh Kord H, M Tinsley G, O Santos H, Zand H, Nazary A, Fatahi S, et al. The influence of fasting and energy-restricted diets on leptin and adiponectin levels in humans: a systematic review and meta-analysis. Clin Nutr. (2021) 40:1811–21. doi: 10.1016/j.clnu.2020.10.034
82. Pellegrini M, Cioffi I, Evangelista A, Ponzo V, Goitre I, Ciccone G, et al. Effects of time-restricted feeding on body weight and metabolism. a systematic review and meta-analysis. Rev Endocr Metab Disord. (2020) 21:17–33. doi: 10.1007/s11154-019-09524-w
83. Fernando H, Zibellini J, Harris R, Seimon R, Sainsbury A. Effect of ramadan fasting on weight and body composition in healthy non-athlete adults: a systematic review and meta-analysis. Nutrients. (2019) 11:478. doi: 10.3390/nu11020478
84. Park J, Seo YG, Paek YJ, Song HJ, Park KH, Noh HM. Effect of alternate-day fasting on obesity and cardiometabolic risk: a systematic review and meta-analysis. Metabolism. (2020) 111:154336. doi: 10.1016/j.metabol.2020.154336
85. O'Keefe JH, Torres-Acosta N, O'Keefe EL, Saeed IM, Lavie CJ, Smith SE, et al. A pesco-mediterranean diet with intermittent fasting. J Am Coll Cardiol. (2020) 76:1484–93. doi: 10.1016/j.jacc.2020.07.049
86. Most J, Gilmore LA, Smith SR, Han H, Ravussin E, Redman LM. Significant improvement in cardiometabolic health in healthy nonobese individuals during caloric restriction-induced weight loss and weight loss maintenance. Am J Physiol Endocrinol Metab. (2018) 314:E396–405. doi: 10.1152/ajpendo.00261.2017
87. Junnila RK, List EO, Berryman DE, Murrey JW, Kopchick JJ. The GH/IGF-1 axis in ageing and longevity. Nat Rev Endocrinol. (2013) 9:366–76. doi: 10.1038/nrendo.2013.67
88. Sävendahl L, Underwood LE. Fasting increases serum total cholesterol, LDL cholesterol and apolipoprotein B in healthy, nonobese humans. J Nutr. (1999) 129:2005–8. doi: 10.1093/jn/129.11.2005
89. Longo VD, Mattson MP. Fasting: molecular mechanisms and clinical applications. Cell Metab. (2014) 19:181–92. doi: 10.1016/j.cmet.2013.12.008
90. Panda S. Circadian physiology of metabolism. Science. (2016) 354:1008–15. doi: 10.1126/science.aah4967
91. Mattson MP, Longo VD, Harvie M. Impact of intermittent fasting on health and disease processes. Ageing Res Rev. (2017) 39:46–58. doi: 10.1016/j.arr.2016.10.005
92. Weindruch R, Sohal RS. Caloric intake and aging. N Engl J Med. (1997) 337:986–94. doi: 10.1056/nejm199710023371407
93. de Cabo R, Mattson MP. Effects of intermittent fasting on health, aging, and disease. N Engl J Med. (2019) 381:2541–51. doi: 10.1056/NEJMra1905136
94. Widlansky ME, Gokce N, Keaney JF Jr, Vita JA. The clinical implications of endothelial dysfunction. J Am Coll Cardiol. (2003) 42:1149–60. doi: 10.1016/S0735-1097(03)00994-X
95. Guzik TJ, Touyz RM. Oxidative stress, inflammation, and vascular aging in hypertension. Hypertension. (2017) 70:660–7. doi: 10.1161/HYPERTENSIONAHA.117.07802
96. Lane MA, Ingram DK, Roth GS. Calorie restriction in nonhuman primates: effects on diabetes and cardiovascular disease risk. Toxicol Sci. (1999) 52:41–8. doi: 10.1093/toxsci/52.suppl_1.41
97. Wilhelmi de Toledo F, Grundler F, Bergouignan A, Drinda S, Michalsen A. Safety, health improvement and well-being during a 4 to 21-day fasting period in an observational study including 1422 subjects. PLoS ONE. (2019) 14:e0209353. doi: 10.1371/journal.pone.0209353
98. Mager DE, Wan R, Brown M, Cheng A, Wareski P, Abernethy DR, et al. Caloric restriction and intermittent fasting alter spectral measures of heart rate and blood pressure variability in rats. FASEB J. (2006) 20:631–7. doi: 10.1096/fj.05-5263com
Keywords: meta-analysis, intermittent fasting, weight loss, blood pressure, fasting blood glucose, blood lipid, cardiometabolic risk factors
Citation: Yang F, Liu C, Liu X, Pan X, Li X, Tian L, Sun J, Yang S, Zhao R, An N, Yang X, Gao Y and Xing Y (2021) Effect of Epidemic Intermittent Fasting on Cardiometabolic Risk Factors: A Systematic Review and Meta-Analysis of Randomized Controlled Trials. Front. Nutr. 8:669325. doi: 10.3389/fnut.2021.669325
Received: 18 February 2021; Accepted: 24 September 2021;
Published: 18 October 2021.
Edited by:
Maria Hassapidou, International Hellenic University, GreeceReviewed by:
Stephen F. Burns, Nanyang Technological University, SingaporeHamed Kord Varkaneh, Shahid Beheshti University of Medical Sciences, Iran
Copyright © 2021 Yang, Liu, Liu, Pan, Li, Tian, Sun, Yang, Zhao, An, Yang, Gao and Xing. This is an open-access article distributed under the terms of the Creative Commons Attribution License (CC BY). The use, distribution or reproduction in other forums is permitted, provided the original author(s) and the copyright owner(s) are credited and that the original publication in this journal is cited, in accordance with accepted academic practice. No use, distribution or reproduction is permitted which does not comply with these terms.
*Correspondence: Yonghong Gao, Z2FveWg3MDg4JiN4MDAwNDA7MTYzLmNvbQ==; Yanwei Xing, eGluZ3lhbndlaTEyMzQ1JiN4MDAwNDA7MTYzLmNvbQ==
†These authors have contributed equally to this work