- College of Plant Protection, Hainan University, Haikou, China
Background/Aim: Camellia oil from Hainan (SY) is a unique vegetable oil in Hainan, China, due to the geographical environment and oil extraction only through simple physical treatments. To compare SY with camellia oil from Guangxi (SC), olive oil (GL), and peanut oil (HS), this study analyzed the antioxidant and antibacterial activity of four vegetable oils.
Methods: Using Gallic acid, BHT as the control, Saccharomyces cerevisiae as the model organism, the antioxidant activities of vegetable oils were measured in vitro and in vivo, and the antibacterial activity was measured with the minimum inhibitory concentration (MIC) method.
Results: The major contents of SY, SC, and HS were oleic Acid; the major content of GL was squalene. The highest total flavonoids content of SY was 39.50 ± 0.41 mg RE/g DW; and the highest total phenolic content of SC was 47.05 ± 0.72 mg GAE/g DW. SY exhibited the strongest scavenging activity of hydroxyl radical (HO·) and superoxide anions (), the IC50 value were 2.06 mg/mL, 0.62 mg/mL, respectively; and SC showed the strongest DPPH· and ABTS· scavenging activity and the reducing abilities. SY showed excellent effect on survival rate, protection rate, flavonoids uptake of S. cerevisiae cells, decreased MDA content and ROS level, inhibited CAT, POD, and GR enzyme activity. The absorption of SC total phenols was the highest by cells. The activity showed GL had a broad-spectrum antibacterial activity.
Conclusion: Thus, SY shows potential antioxidant activity and provides an important reference value for people to choose edible vegetable oils.
Introduction
Camellia oleifera, known as the edible tea oil tree, is a subtropical evergreen tree distributed in China and Southeast Asian countries (1, 2). The camellia is the most well-known and largest genus in the Theaceae family, with more than 120 recognized tree species (3). It has been widely grown as an oil crop in many countries including China, the Philippines, India, Brazil, and South Korea (4). More than 90% of the world's production of Camellia oil comes from China (5, 6). The Compendium of Materia Medica recorded that Camellia oil was tasted sweet, lubricating the intestines (7), and clearing away heat and eliminate dampness, moisturizing the lungs and eliminating phlegm. Camellia oil also has good effects on reducing swelling, relieving itching, cuts, burns, and bruises (8). In addition, it was use for the treatment of skin diseases such as dermatitis and stretch marks (9).
Camellia oil is rich in unsaturated fatty acids (2, 10), such as oleic acid, linoleic acid; Sesamin (11), saturated acids (12), polyphenols (13) also are found in camellia oil. These compounds shows excellent antioxidant activities. So, Lee and Yen found methanol extract of camellia oil exhibited DPPH· scavenging activity (11); Wang et al. (10) and Wang et al. (13) reported Supercritical Carbon Dioxide extract of camellia oil also showed a stronger DPPH· and ABTS· scavenging activity; the antioxidant activities from the literatures were most limited in DPPH· and ABTS· scavenging activity in vitro, were not involved to treat with Saccharomyces cerevisiae in vivo.
The unsaturated and saturated fatty acids of camellia oil were similar to those in olive oil (14), olive oil also showed excellent activities, which could accelerate the elimination of ROS (15, 16), reduce the risk of cardiovascular disease, extend lifespan (17), improve memory and cognitive function in the elderly, and reduce the risk of Alzheimer's (18). And peanut oil is a widely used vegetable oil in China. It is rich in natural vitamin E, unsaturated fats, phytosterols. Its α-tocopherol and γ-tocopherol have antioxidation and anti-aging (19, 20). Consumption of phytosterols may be induced activity of antioxidant enzymes and reduced oxidative stress (21).
Camellia oil from Hainan Island is unique due to the unique geographical environment and oil extraction only through simple physical treatments such as precipitation and filtration. Therefore, the taste and quality of Camellia oil from Hainan are better than ordinary Camellia oil (22). So, we used Camellia oil from Hainan, camellia oil from Guangxi, olive oil from Guangdong and peanut oil from Shandong Province as research samples to compare the in vitro antioxidant activities, and evaluate the antioxidant capacity of wild-type S. cerevisiae, a good model organism in antioxidant research (23, 24), and genetically deficient S. cerevisiae in vivo, also, the antibacterial activity of the four vegetable oils was detected. We hope that these data can provide a reference for people to choose edible vegetable oils.
Materials and Methods
Test Materials
Vegetable Oils
The tea seeds from Wenchang and Qionghai of Hainan Province were picked and mixed together at December 16, 2018, and dried, shelled, crushed; then roasted, physically pressed at January 7, 2019, precipitated and filtered to yield Camellia oil from Hainan Province (SY); According to above method, camellia oil from Baise of Guangxi Province (SC) were yield at March 5, 2019. A bottle of SY and SC was randomly selected and stored at 25°C. Olive oil (GL) from Guangdong Province (Production date: 2018.10.16) and peanut oil (HS) from Shandong Province (Production date: 2018.06.22) were commercially available and randomly selected in the shelf.
Test Strain
The wild-type (WT) BY4741 of S. cerevisiae and its homologous gene-deficient strains Sod1 and Ctt1 were provided by the Laboratory of Biotechnology and Food Science, Tianjin University of Commerce (Sod1 encodes cytoplasmic superoxide dismutase, Carries the gene SOD 1 knocked out by the gene KanMX 4; Ctt1 carries the gene CAT 1 knocked out by the gene KanMX 4).
Test Agent
Folin-Ciocalteu reagent, gallic acid, rutin, 2,6-ditert-butyl-4-methylphenol (BHT), 1,1-diphenyl-2-trinitrophenylhydrazine (DPPH), 2,2-diazo-bis (3-ethyl-benzothiazole-6-sulfonic acid) diammonium salt (ABTS), tripyridyltriazine (TPTZ), isopropyl myristate (IPM), Span80, Tween 80, salicylic acid, trichloroacetic acid, ferric chloride, ferrous sulfate heptahydrate, potassium peroxodisulfate, potassium ferricyanide, etc. were all purchased In Shanghai Maclean Biochemical Technology Co., Ltd. Superoxide dismutase (SOD), peroxidase (POD), catalase (CAT), glutathione reductase (GR) and MDA content kits were purchased from Beijing Soleibao Technology Co., Ltd. All reagents used in this study either analytical or chemical grade were commercially available.
Preparation of Vegetable Oils
We prepared four different kinds of vegetable oils using Shah Method (25). Mixing vegetable oils (0.20 g) and 0.40 g IPM as oil phases; 0.58 g Tween 80, 0.14 g Span 80, and 0.18 g n-butanol as surfactant; the surfactant was mixed with oil phases in the ratio of 6:4, then were diluted in distilled water to yield the concentration 20 mg/mL.
GC-MS Analysis of Vegetable Oils
Take 0.1 g of vegetable oil separately; dilute n-hexane into a 10 mL volumetric flask to dissolve entirely. the vegetable oils components were analyzed by using GC-MS (Agilent 7890B-7000B, USA) technology. Gas chromatographic conditions: Agilent HP-5ms (30 m × 250 μm × 0.25 μm) chromatographic column, temperature program: 60°C (retention 1 min), 6°C/min to 300°C (retention 16 min); carrier gas: He, inlet temperature: 270°C, transfer line temperature: 280°C. Conditions of mass spectrometry: EI source; ionization voltage: 70 eV, ion source temperature: 230°C, scanning range: 20–500 aum, injection volume: 1.0 μL.
Determination of Total Phenols Content (TPC)
TPC of vegetable oils was determined according to the method (26). A mixture of 80 μL vegetable oil solution, 200 μL Folin-ciocalteu reagent, and 3,780 μL Na2CO3 (2% w/v) solution was incubated at 40°C for 60 min, and then measured the absorbance at 760 nm. Gallic acid was used as a positive control. According to the standard curve of gallic Acid, TPC was calculated (mg GAE/g DW, the total phenols equivalent per gram of vegetable oils sample).
Determination of Total Flavonoids Content (TFC)
TFC of vegetable oils was determined using the previously described method. We mixed 0.5 mL of vegetable oils, 2.5 mL of ultrapure water and 150 μL of 5%(w/v) sodium nitrite solution, and were kept for 6 min at room temperature, then were added into 300 μL of 10%(w/v) aluminum chloride aqueous solution, keeping at room temperature for 5 min again. We added 1 mL of 1 mol/L sodium hydroxide aqueous solution and 550 μL of ultrapure water, and then the absorbance was measured at 510 nm. Rutin was used as a positive control. According to Rutin's standard curve, TFC was calculated (mg RE/g DW, that is, the equivalent of total flavonoids per gram of vegetable oils).
Determination of Antioxidant Activity of Vegetable Oils in vitro
DPPH Radical Scavenging Activity
DPPH scavenging activity of vegetable oils was tested according to the reference (27), with slight modification. For the Preparation of 2 × 10−4 M DPPH 95% solution, we used 2 mL of DPPH methanol solution in a test tube, and were mixed with 2 mL of vegetable oils (10, 5, 2.5, 1.25, 0.625 mg/mL). Then were incubated at 25°C in the dark for 30 min. the absorbance A was measured at 517 nm. All experiment was replicated three times. The DPPH· scavenging rate was calculated using the following formula:
DPPH· scavenging rate /% = [1-(Ai-Aj)/A0] × 100
A0 was the absorbance of 2 mL DPPH methanol solution and 2 mL ultrapure water; Ai was the absorbance of 2 mL DPPH methanol solution and 2 mL vegetable oil; Aj was the absorbance of 2 mL ultrapure water and 2 ml vegetable oil.
ABTS Radical Scavenging Activity
ABTS· scavenging activity of vegetable oils was measured according to the reference. A mixture of 10 mL 7 mmol/L ABTS radical and 10 mL 2.45 mmol/L potassium persulfate, were stored in the dark for 12–16 h. Two mL vegetable oils (20, 10, 5, 2.5, 1.25 mg/mL) and 2 mL ABTS solution reacted for 6 min, then the absorbance A was measured at 734 nm. The calculation formula of the ABTS scavenging rate was as follows:
ABTS· scavenging rate/% = [1-(Ai-Aj)/A0] × 100
A0 was the absorbance of 2 mL ABTS solution and 2 mL ultrapure water; Ai was the absorbance of 2 mL ABTS solution and 2 mL vegetable oil; Aj was the absorbance of 2 mL ultrapure water and 2 ml vegetable oil.
Hydroxyl Radical Scavenging Activity
According to the reference (28), a mixture of 2 mL vegetable oils (20, 10, 5, 2.5, 1.25 mg/mL), 2 mL of 6 mM ferrous sulfate solution, and 2 mL of 6 mM H2O2 solution were kept at room temperature for 10 min. We added 2 mL of 6 mM salicylic acid, and were kept again for 30 min. The absorbance A of the mixture was measured at 510 nm. Each experiment was replicated three times. HO· scavenging rate was calculated using the formula:
HO· scavenging rate /% = [1-(Ai-Aj)/A0] × 100
Where: A0 was the absorbance of 2 mL ultrapure water, 2 mL ferrous sulfate solution, 2 mL H2O2 solution, and 2 mL salicylic acid; Ai was the absorbance of 2 mL vegetable oil, 2 mL ferrous sulfate solution, 2 mL H2O2 solution, and 2 mL salicylic acid; Aj was the absorbance of 2 mL vegetable oil, 2 mL ferrous sulfate solution, 2 mL H2O2 solution and 2 mL ultrapure water.
Superoxide Anion Free Radical Scavenging Activity
According to the reference (29), we mixed 4.5 mL of Tris-HCl buffer solution and 3 mL of ultrapure water. The mixture was incubated at 25°C for 20 min, then added 0.4 mL of 3 mM pyrogallol solution and 1 mL vegetable oils (20, 10, 5, 2.5, 1.25 mg/mL), again incubated at 25°C for 4 min, then immediately 0.1 mL of 8 M hydrochloric acid solution were added to stop the entire reaction. The absorbance A was measured at 325 nm. Each experiment was replicated three times. scavenging rate was calculated as follows:
scavenging rate /% = [1-(Ai-Aj)/A0] × 100
Where: A0 was the absorbance of 4.5 mL buffer solution, 3 mL ultrapure water, 0.4 mL pyrogallol solution, 1 mL buffer solution, and 0.1 mL hydrochloric acid solution; Ai was the absorbance of 4.5 mL buffer solution, 3 mL ultrapure water, 0.4 mL pyrogallol solution, 1 ml vegetable oil and 0.1 mL hydrochloric acid solution; Aj was the absorbance of 4.5 mL buffer solution, 3 mL ultrapure water, 0.4 mL ultrapure water, 1 mL vegetable oil and 0.1 mL hydrochloric acid solution.
FRAP Assay
We performed FRAP assay according to the described method (30), 0.2 mL vegetable oils were mixed with 3.8 mL of FRAP solution (31), incubated at 37°C for 5 min; the absorbance was measured at 593 nm. Ferrous sulfate heptahydrate was used as a positive control. According to the standard curve of ferrous sulfate heptahydrate, the reducing power of vegetable oils was calculated (μM Fe2+/g DW, that is, the reducing ability of high-valent iron ions per gram of vegetable oil).
Total Reduction Capacity (TRC)
According to the method (32), 1 mL of vegetable oils (20, 10, 5, 2.5, 1.25 mg/mL) was added to 2.5 mL of phosphate buffer with pH = 6.6 and 2.5 mL of 1% (w/v) Potassium ferricyanide solution, and were incubated at 50°C for 20 min, then we added 2.5 mL of 10% (w/v) trichloroacetic acid solution, and were mixed by hand slowly and centrifuged at 3,000 rpm/min for 10 min. After centrifuge 2.5 mL of supernatant was shifted to a new test tube, added 2.5 mL ultrapure water and 0.5 ml 0.1% (w/v) ferric chloride solution, then were incubated for 5 min at 25°C, at last the absorbance was measured at 700 nm.
Determination of Antioxidant Activity of Vegetable Oils in vivo
Determination of the Survival Rate of S. cerevisiae Cells
Cell survival is expressed as cell tolerance (33). According to the reference, the yeast cells suspension was prepared with YPD (Yeast extract peptone dextrose) medium (34, 35). Ten-milliliter cell suspension was mixed with 40 mL liquid YPD medium thoroughly, adding 200 μL H2O as the H2O treatment group, 200 μL vegetable oils as the oils group, 200 μL vegetable oil solvent as the solvent group, and no additive as the blank control group. After shaking and mixing thoroughly, the solution was incubated for 1 h at 28°C/180 rpm. Then, 20 μL of H2O2 (final concentration of 2.0 mM) was added into the above mixtures, set at 28°C/180 rpm for 1 h. The mixtures were diluted 1,500 times, 100 μL of the diluted solution was coated on the plate containing 2% agar YPD medium. The plate was incubated at 28°C for 72 h, and the cell numbers were counted, the cell viability was calculated.
Cell viability (%) =(A0/A) × 100
A0 represents the number of cell growth in the H2O treatment group, the oils group, and the solvent group; while A represents the number of cell growth in the blank control group.
Determination of Cell Uptake of Four Vegetable Oils by S. cerevisiae Cells
The changes in TPC and TFC were indirectly reflected the uptake of vegetable oils by yeast cells (36). The above mixture without adding H2O2 was centrifuged at 10,000 g for 5 min; the supernatant was filtered with the membrane (pore size: 0.45 μm), TPC and TFC of the filtrate were tested as C1; after adding H2O2, the treated method was the same as the method without adding H2O2, and TPC and TFC of the filtrate were tested as C2. The changes in C1 and C2 were used as the uptake of vegetable oils by yeast cells.
Determination of the Protective Effect of Four Vegetable Oils
The protective effect of vegetable oil on cells was measured on a plate containing 0.7% agar YPD medium by cell halo method (37). The cell suspension was diluted 100 times, and added 10 mL into 90 mL of 0.7% agar YPD medium, which was poured into three plates (Φ = 9 cm). After solidification, three filter papers (Φ = 0.5 cm) were placed on the medium's surface, arranged in an equilateral triangle. Adding 2 μL of 50 mg/mL vegetable oil to the top filter paper, and 2 μL of 50 mg/mL vegetable oil and 2 μL of vegetable oil solvent to the right and left filter papers, 1 h later, 2 μL of H2O2 were added to the right and left filter papers, which was incubated at 28°C for 3 days, and the cell halo was observed. The halo was measured by the cross method, the cell protection rate was calculated.
Cell protection rate (%) = [(B0-0.5)–(B-0.5)]/(B0-0.5) × 100,
Among them, B0 represents the vegetable oil solvent+H2O2 group, B represents the vegetable oil group+H2O2, and 0.5 represents the filter paper diameter.
Determination of MDA Content, ROS Level, the Enzyme Activity of Four Vegetable Oils
The MDA content of the cells was measured using the MDA kit (BC0020) method. The DCFH-DA kit was used to measure intracellular ROS level changes. A kit (SOD, BC0175; CAT, BC0205; POD, BC0090; GR, BC1160) was used to determine the corresponding enzyme activity.
Determination of the Antibacterial Activities of Vegetable Oils
Staphylococcus aureus, Escherichia coli, Pseudomonas aerugino, Bacillus cereus, and Bacillus subtilis were provided by the college of plant protection, Hainan University. The minimum inhibitory concentration (MIC) of four vegetable oils was measured against five pathogenic bacteria using the multiple dilution method (38).
Statistics Analysis
Statistical analysis were performed using (SAS 9.1.3; SPSS version 21), Origin pro 9.0 were used for data and image analysis. The mean ± standard deviation (SD) of each repeated experiment. When p < 0.05, it is a significant difference.
Results and Analysis
GC-MS Analysis of Vegetable Oils
GC-MS obtained the total ion flow charts of the four vegetable oils (Figure 1). Compared with the GC-MS database, the chemical components of vegetable oils were identified; the peak area normalization method was used to express the compound's content.
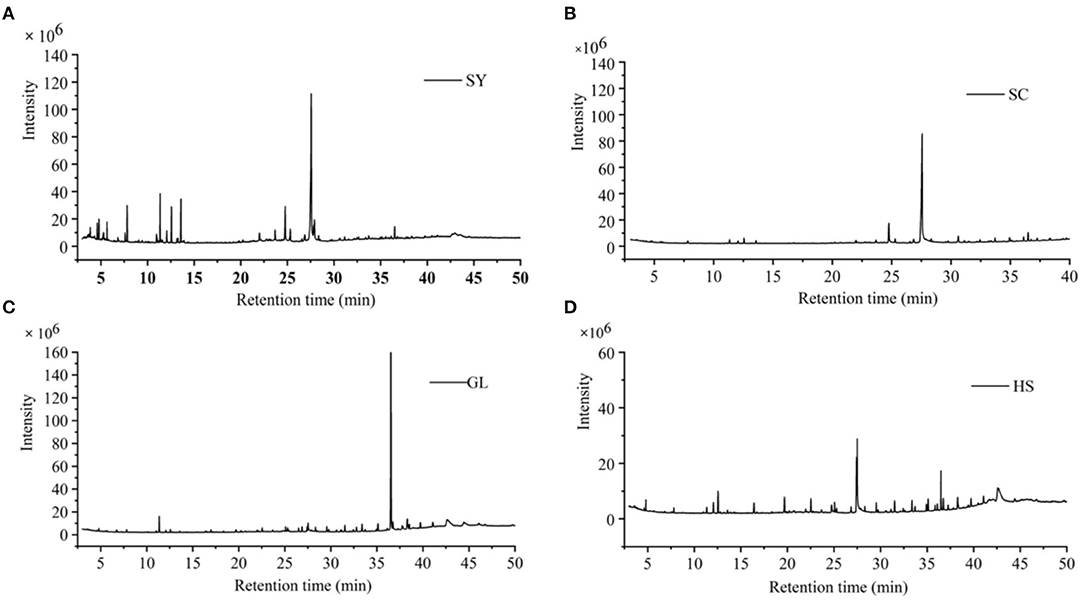
Figure 1. Total ion flow charts of four vegetable oils. (A) SY camellia oil from Hainan, (B) SC camellia oil from Hainan Guangxi, (C) GL olive oil, and (D) HS peanut oil.
Through GC-MS analysis, the major content of SY was Oleic Acid (51.63%), followed by n-hexadecanoic Acid (8.41%), methyl oleate (6.59%), a-Amyrin (6.13%), and so on (Table 1); the major content of SC was Oleic Acid (58.02%), followed by n-hexadecanoic Acid (6.86%), a-Amyrin (5.29%), Ethyl iso-allocholate (3.38%), etc. (Table 2); the major content of GL was squalene (52.60%), followed by β-sitosterol (9.15%), 2-(3-acetoxy-4,4,14-trimethylandrost-8-en-17-yl)-Propanoic Acid (4.77%), Oleic Acid (4.22%), etc. (Table 3); the major content of HS was Oleic Acid (26.46%), followed by β-sitosterol (14.24%), squalene (6.54%), Oleic acid, eicosyl ester (2.65%), etc. (Table 4).
TPC and TFC of Vegetable Oils
With gallic Acid and Rutin as references, the standard curves of TPC and TFC were established, and the fitted linear regression equations were y = 0.0011x + 0.0025, y = 0.0007x + 0.0078, respectively; the correlation coefficients were R2 = 0.9972, R2 = 0.9998, which showed that the correlation was excellent. According to the regression equations, the TPC and TFC of four vegetable oils were further calculated (Table 5). Among the vegetable oils, the TFC of SY was the highest (39.50 ± 0.41 mg RE/g DW), followed by GL (35.84 ± 0.08 mg RE/g DW). TPC of SC was the highest (47.05 ± 0.72 mg GAE/g DW), followed by SY (43.8 ± 0.28 mg GAE/g DW); there was no significant difference in TPC between HS and GL.
Antioxidant Effect of Vegetable Oil in vitro
The DPPH· scavenging activities were increased with the concentration of vegetable oils increases (Figure 2A). When the concentration was 0.625–2.5 mg/mL, the four vegetable oils' scavenging rate increased rapidly; the concentration was 5–10 mg/mL, the scavenging rate increased slows down. At the same concentration, SC's scavenging rate in the four vegetable oils was the highest; mostly, the scavenging rate was 98.32% at 10 mg/mL. The IC50 of each vegetable oil was shown in Table 6. The four vegetable oils' DPPH· scavenging activity was ranked from strong to weak: SC> SY> GL> HS.
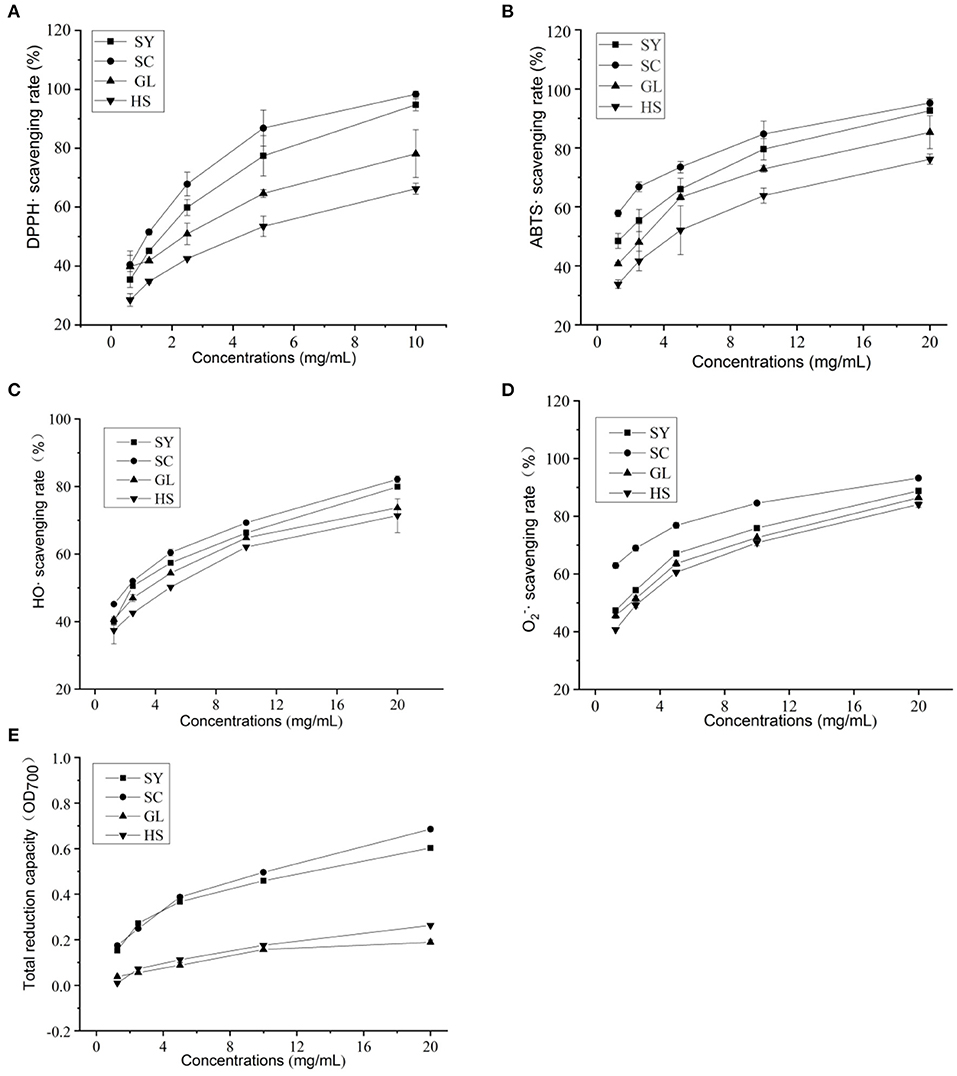
Figure 2. Antioxidant activities of four vegetable oils. (A) DPPH·, (B) ABTS·, (C) HO·, (D) , (E) total reduction capacity.
The ABTS· scavenging activities of four vegetable oils were also increased with the increase in vegetable oil concentration (Figure 2B). When the concentration was 1.25–2.5 mg/mL, the four vegetable oils' scavenging rate increased rapidly and then slowly increased. Among them, SC showed the highest scavenging rate. When the concentration was 20 mg/ml, the clearance rate was 95.22%, followed by SY, the scavenging rate was 92.64%. The IC50 of each vegetable oil was shown in Table 6. The ABTS· scavenging activity of the four vegetable oils was ranked from strong to weak: SC> SY> GL> HS.
The HO· scavenging activities of four vegetable oils were also increased with the increase in vegetable oil concentration (Figure 2C). When the concentration was 1.25–5 mg/mL, the scavenging rate of the four vegetable oils increased rapidly, and the scavenging rates of SY and SC were similar at 2.5 mg/mL; when the concentration was 20 mg/mL, the scavenging rate of SY was as high as 82.16%, followed by SC, the scavenging rate was 79.92%. The IC50 of each vegetable oil was shown in Table 6. The HO· scavenging activity of the four vegetable oils was ranked from strong to weak: SY> SC> GL> HS.
The scavenging activities of four vegetable oils were also increased with increased vegetable oil concentration (Figure 2D). When the concentration was 1.25–5 mg/mL, the four vegetable oils' scavenging rate increased rapidly. When the concentration was 20 mg/mL, the scavenging rate of SY was the highest (93.22%), followed by SC, GL, and HS The IC50 of each vegetable oil was shown in Table 6. The HO· scavenging activity of the four vegetable oils was ranked from strong to weak: SY> SC> GL> HS.
The reduction effect of four vegetable oils on FRAP high-valent iron ions was measured. It was found that the four vegetable oils had significant differences in the reduction effect (Table 7). Among them, SC had the most substantial reducing effect. One mg of SC sample was equivalent to the reducing ability of 197.68 ± 1.54 μM FeSO4; followed by SY (138.01 ± 1.99 μM FeSO4).
The total reducing capacity of vegetable oils increased with vegetable oil concentration (Figure 2E). In comparison, the total reducing capacity of SY and SC vegetable oils were relatively close, and the total reducing capacity of GL and HS vegetable oils were relatively close. Overall, SC showed the strongest total reducing capacity. The IC50 of each vegetable oil was shown in Table 6. The total reducing ability of the four vegetable oils is SC> SY> GL> HS.
Protective Effects of Vegetable Oils on Yeast Cells Under H2O2 Stress
The Protective Effect of Vegetable Oils on the Yeast Cells
Wild-type (WT) and genetic mutant (sod1Δ, cttlΔ) yeast cells were used to test cell viability. The oxidative stress of yeast cells stressed by H2O2 was alleviated by adding SY, SC, GL, and HS (Figure 3A). The survival rate of yeast cells was the lowest in the H2O treatment group, and was the highest in the solvent group without H2O2 stress, which showed that the solvent had little effect on the yeast cells. SY, SC, GL, and HS could better alleviate the oxidative damage caused by H2O2 in WT, sod1Δ, and ctt1Δ cells. In particular, the survival rate of SY was the highest in yeast cells under H2O2 stress, the increased value of the survival rate, in turn, was 17.19, 28.98, 33.54%, respectively. Also, the survival rates of sod1Δ and ctt1Δ cells were significantly higher than the other vegetable oils. Besides, SC also showed a better effect on the cell's survival under stress. In comparison, the impact on sod1Δ and ctt1Δ cells was higher than that of WT cells. Compared with the control, vegetable oils' protective effect on yeast cells was found treated with H2O2 (Figure 3B). SY showed the most obvious protective effect. The protective rates for WT, sod1Δ, and ctt1Δ were 21.07, 12.01, and 11.34%, respectively. Secondly, the protective rates of SC were also more obvious. It was 15.27, 9.51, 10.93%, respectively. Comparison between different yeast cells, the vegetable oils showed an excellent protective effect on WT cells.
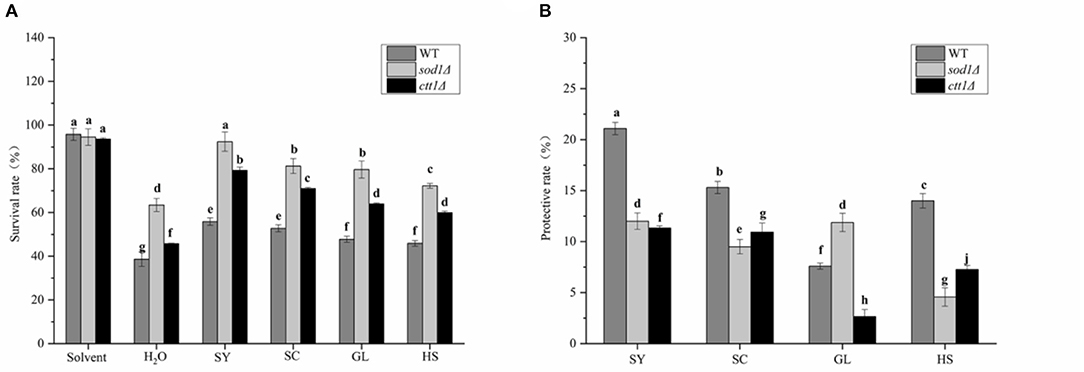
Figure 3. The protective effect of vegetable oils on yeast cells. (A) the survival rate of yeast cells; (B) the protective effect on yeast cells; Lower case letters have significant differences at p < 0.05.
Yeast Cells' Uptake to Total Phenols and Total Flavonoids of Vegetable Oils
WT, sod1Δ, and ctt1Δ yeast cells showed the highest uptake to total phenols of SC (Figure 4A), and the uptake content was 5.37 times, 7.38 times, and 7.12 times that of the blank, respectively; followed by SY, the uptake content was 5.16 times, 6.70 times and 6.43 times that of the blank. Compared with three yeast cells, the uptake to total phenols by sod1Δ cell was the highest, followed by ctt1Δ cell, and WT cell.
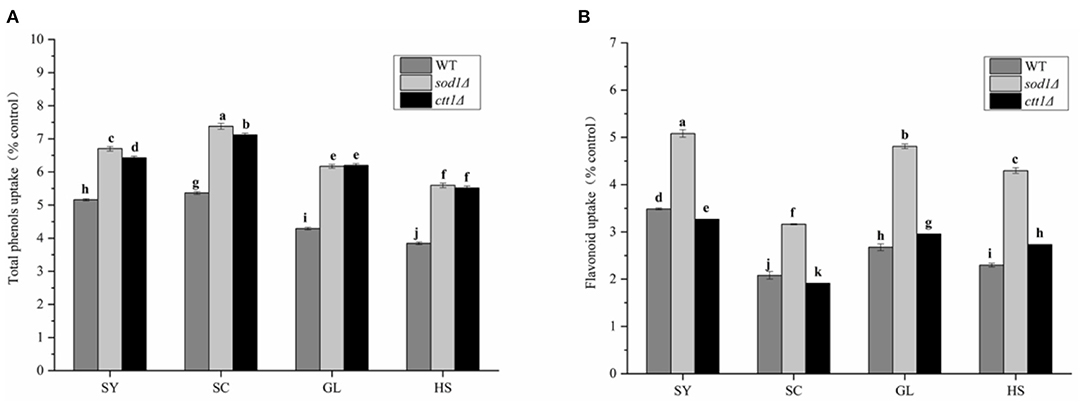
Figure 4. Total phenols and Flavonoid intake of yeast oil cells against four oils. (A) Total phenols (B) total Flavonoids; Lower case letters indicate the difference in entire phenolic content at p < 0.05.
Also, WT, sod1Δ, and ctt1Δ yeast cells showed the highest uptake to total flavonoids of SY (Figure 4B), and the uptake content was 3.48 times, 5.08 times, and 3.27 times that of the blank, respectively; The three kinds of yeast cells had the lowest intake to total flavonoids of SC The same as total phenols, sod1Δ cells showed the highest uptake of total flavonoids. These results were the same as the TPC and TFC of the oils.
Effect of Vegetable Oils on MDA Content of Yeast Cells
Under oxidative stress, the MDA content of the yeast cells will increase. So, treated yeast cells with H2O2, the MDA content of the cells increased dramatically in the H2O treatment group (Figure 5A), the MDA content of WT, sod1Δ, and ctt1Δ cells were 2.42, 1.23, and 1.78 times that of the cells without H2O2 treatment; the changes in MDA content under vegetable oils protection, the H2O treatment group showed a significant difference, especially, the SY was significantly different from the other three vegetable oils. The MDA content was decreased from 2.42 times to 1.43 times, and the MDA content of sod1Δ cells was reduced from 1.23 times to 0.96 times. The MDA content of ctt1Δ cells decreased from 1.78 times to 1.48 times. Compared with the effect of vegetable oils on the three yeasts, the MDA content in WT cells was the most obvious, especially, under SY and SC protection, the MDA content was decreased to 59.0% and 83.1% compared with the H2O treatment group.
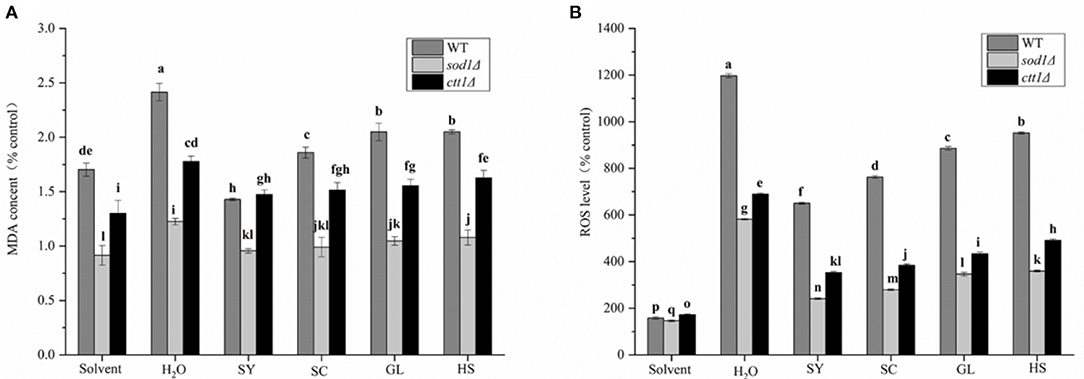
Figure 5. MDA content and ROS level of four oils in yeast cells. (A) MDA content, (B) ROS level. Lower case letters indicates the difference at p < 0.05.
Effect of Vegetable Oil on the ROS Level of Yeast Cells
When cells are subjected to external stimulation to produce oxidative stress, the intracellular ROS level increase significantly. The intracellular ROS level was significantly increased under H2O2 treatment (H2O treatment group), the ROS levels of WT, sod1Δ, ctt1Δ cells were 11.98 times, 5.82 times, 6.90 times compared with the blank, respectively (Figure 5B). Under the protection of the vegetable oils, the changes in ROS level in the cells were reduced. Mostly, the protective effect of SY was the most obvious. The ROS level of WT cells was 6.51 times compared with the blank, which was about 54.3% of the H2O treatment group; the ROS level of sod1Δ cells was 2.41 times of the blank, which was approximately 41.4% of the H2O treatment group; the ROS level of ctt1Δ cells was 3.53 times of the blank, which was about 51.1% of the H2O treatment group. Secondly, the protective effect of SC showed an excellent effect. Comparison with different yeast cells, the protective effect of the oils to sod1Δ cells was the best, followed by ctt1Δ cells.
Effect of Vegetable Oil on Enzyme Activity in Yeast Cells
It was found that the H2O2 treatment (H2O treatment group) could cause the intracellular SOD enzyme activity to be significantly lower than the solvent group (Figure 6A). The SOD enzyme activity of WT, sod1Δ, ctt1Δ cells was 16.99, 14.91, 10.38% of the control, respectively. Under the protection of the vegetable oils, the SOD enzyme activity was all increased. The protective effect of GL was excellent, SOD enzyme activity of WT, sod1Δ, ctt1Δ cells was 72.63, 60.00, and 46.87% of the control, respectively. Followed by HS, SOD enzyme activity of WT, sod1Δ, ctt1Δ cells was 40.68, 42.26, and 33.46% of the control, respectively. The protective effect of SY to ctt1Δ cells was the better than that of WT, sod1Δ cells.
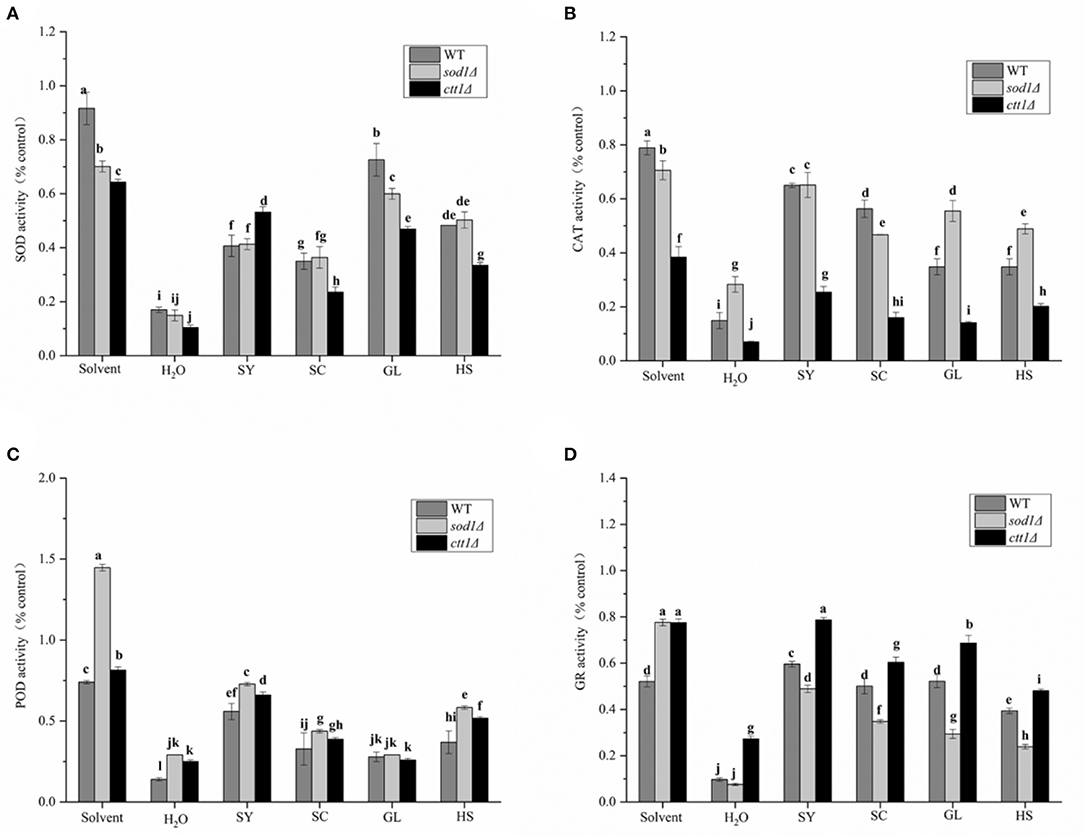
Figure 6. Effects of the oils on enzyme activity of yeast cells. (A) SOD, (B) CAT, (C) POD, (D) GR the results of enzyme activity are expressed as the ratio of cells treated with oil, H2O2 treated cells, and non-H2O2 treated cells. Lower case letters indicate the difference at p < 0.05.
The CAT enzyme activity of WT, sod1Δ, ctt1Δ cells was 14.93%, 28.33% while 7.05% of the control group (Figure 6B). Under the protection of the vegetable oils, the CAT enzyme activity of the yeast cells was increased. The CAT enzyme activity of SY in WT, sod1Δ, and ctt1Δ cells was 64.97, 65.14, and 25.39% of the control, respectively. Following SC treatment, the CAT enzyme activity of WT, sod1Δ, and ctt1Δ cells was 56.34, 46.74, and 16.00% of the control group. Different yeast cells, For example, SY, GL, and HS showed the most significant effect on sod1Δ cells, while SC showed the most significant effect on WT cells.
The POD activity of WT, sod1Δ, ctt1Δ cells was 13.97%, 29.13% while 24.99% of the control (Figure 6C). When the vegetable oils were added, the POD enzyme activity of the yeast cells was increased. Among them, the protective effect of SY was the most obvious. The POD enzyme activity in WT, sod1Δ, and ctt1Δ cells was 55.89, 72.83, and 65.97% of the control. After HS treatment, the POD enzyme activity of WT, sod1Δ, and ctt1Δ cells was 36.94, 58.26, and 51.75% of the control.
The GR enzyme activity of WT, sod1Δ, ctt1Δ cells was 9.65, 7.64, 27.27% of the control (Figure 6D). When the vegetable oils were added, the three yeast cells' GR enzyme activity was all increased. Among them, the protective effect of SY treatment was the most obvious. The GR enzyme activity in WT, sod1Δ, and ctt1Δ cells was 59.63, 48.90, and 78.75% of the control. The vegetable oils had the same order (ctt1Δ, WT, sod1Δ) to affect the GR enzyme activity.
Inhibitory Effect of the Vegetable Oils on Five Bacteria
At the tested concentration, SY had no inhibitory effect on the five bacteria (Table 8). SC had inhibitory effects on E. coli and B. cereus, and the MIC of both was 20 mg/mL. GL had an excellent inhibitory effect on five bacteria, the MIC was the same 5 mg/mL against E. coli and B. cereus; the MIC was 10 mg/mL against S. aureus, and the MIC was 20 mg/mL against B. subtilis. HS had an excellent inhibitory effect on B. cereus, and the MIC was 10 mg/mL. But, treated with Streptomycin (5 mg/mL), five bacteria did not grow. Thus, the inhibitory effect of the oils was not good.
Discussion
According to the GC-MS analysis results, the chemical composition of the vegetable oils was not the same. Oleic Acid, Squalene, and Alkane (19, 20-carbon chain) were detected in four vegetable oils. Oleic Acid was the major in SY, SC, and HS, but the content of oleic Acid was lower than that of Feás's and Zhou's reports (12, 39); β-sitosterol was detected in SY, GL, and HS The major content of GL was squalene, and squalene showed important bioactivities (40). This study showed that GL had the best antibacterial effect against five bacteria; also, HS's antibacterial effect was good. Maybe, the content of squalene was higher than other oils. Squalene was easily oxidized, so GL's protective effect was less than that of the other oils. And SC had inhibitory effects, MIC for B. cereus was 20 mg/mL, which was better than Feás's report, but the MIC of SC for E. coli was not good as Feás's report (12). There are differences in tea seeds from different sources, regions, and climatic conditions, as well as in the vegetable oils obtained by different pressing methods. Thus, this is a preliminary result because the four vegetable oils were selected at random and not sampled in large numbers.
TPC of SC and TFC of SY were the highest in the four vegetable oils. This result was consistent with the reference (41), and TPC of SC and SY was higher than that reported by Wang et al. (2.18 mg GAE/g oil) (13). It was possible that the tea seeds of our sample were physically pressed by shelling, while Wang's sample was extracted with supercritical carbon dioxide. The antioxidant capacity was also outstanding. Among them, SC exhibited the strongest scavenging ability for DPPH·, ABTS·, FRAP, and TRC. At the same time, the scavenging ability for HO· and was also prominent; then, SY showed the excellent scavenging ability for DPPH·, ABTS·, FRAP, and TRC. It was pointed out that free radical scavenging ability was often positively correlated with TPC (42). This study supported this view. And DPPH· scavenging activity of SC and SY was higher that reported by Wang et al. (10), which was due to the higher TPC of SC and SY. At the same time, this study's results proved that the free radical scavenging ability was positively correlated with the TFC of vegetable oils. To a certain extent, excessive H2O2 can induce oxidative stress and lead to membrane damage in cells (43). MDA content changes can indirectly prove membrane damage in the cell (44, 45). Treated yeast cells (wild-type and mutant) with H2O2, the MDA content and ROS level of SY, SC were significantly reduced, which was alleviated the oxidative damage induced by H2O2 and increased the survival rate of yeast cells. This conclusion was consistent with Li's reports, and verified the guess “the oil of tea seed may act as a prophylactic agent to prevent free radical related diseases” (11). Also, the tested yeast cells showed the highest intake of total phenols of SC and the highest intake of total flavonoids of SY, which was the same as the corresponding TPC and TFC of the these vegetable oils. We speculated that the content of antioxidants in vegetable oils could affect the uptake of yeast cells. At the same time, SY showed the best protective effect on yeast cells, and it was the most significant about the reduction of intracellular ROS levels and MDA content. Saccharomyces cerevisiae is a good model organism in antioxidant research. In this paper, for the first time, yeast was used to clarify the antioxidant effect of camellia oil, which was consistent with the results of antioxidant activity in vitro. Phenolic acids and flavonoids had strong antioxidant activity, and TPC and TFC of SC, SY were significantly higher than those of other oils, so, SC, SY showed excellent antioxidant activities in vitro and in vivo in this study.
On the whole, many indexes of SY in the antioxidant determination were significantly lower than those of SC Exogenous antioxidants can also alleviate the damage of intracellular peroxides; the cell's antioxidant system also plays a vital role, mainly including antioxidant enzymes and small molecules antioxidant substances. So, the enzyme activities of CAT, POD, GR treated with SY were higher than that of other oils.
Conclusion
By comparison, many components were the same as in SY and SC, such as oleic Acid, n-hexadecanoic Acid, a-Amyrin, only the content was not consistent; those were different from GL. Also, TPC and TFC of SY and SC were higher than that of the other oils. In vitro, SY showed the strongest HO· and scavenging activity, and SC exhibited excellent DPPH· and ABTS· scavenging activity and the reducing abilities. In vivo, SY showed excellent protective effect on S cerevisiae cells, decreased MDA content and ROS level, inhibited CAT, POD and GR enzyme activity, followed by SC. The antibacterial activity showed GL had a broad-spectrum inhibited activity. Thus, the results provided a reference for the selection of edible vegetable oils in the future.
Data Availability Statement
The raw data supporting the conclusions of this article will be made available by the authors, without undue reservation.
Author Contributions
LW and YL conceived and designed the experiments. LW, SA, and YL wrote the manuscript. All authors were contributed the experiments and analyzed the data.
Funding
This work was supported by the Natural Science Foundation of Hainan (2019RC121) and National NSFC (31860513, 31760526).
Conflict of Interest
The authors declare that the research was conducted in the absence of any commercial or financial relationships that could be construed as a potential conflict of interest.
References
1. Yang P, Li X, Liu YL, Xu QM, Li YQ, Yang SL. Two triterpenoid glycosides from the roots of Camellia oleifera and their cytotoxic activity. J Asian Nat Prod Res. (2015) 17:800–7. doi: 10.1080/10286020.2015.1004630
2. Luan F, Zeng J, Yang Y, He X, Zeng N. Recent advances in Camellia oleifera Abel: a review of nutritional constituents, biofunctional properties, and potential industrial applications. J Funct Foods. (2020) 75:104242. doi: 10.1016/j.jff.2020.104242
3. Nguyên DH, Luong VD, Lê TH, Trân QT, 0ô N, Lý NS. Camellia puhoatensis (Sect. Archecamellia - Theaceae), a new species from Vietnam. PhytoKeys. (2020) 153:1–11. doi: 10.3897/phytokeys.153.49388
4. Wang SL, Zhong C, Tong XJ, Liu YL, Xia L, Xu QM, et al. Triterpenoids from the roots of camellia oleifera c.abel and their cytotoxic activities. Helv Chim Acta. (2013) 96:1126–33. doi: 10.1002/hlca.201200333
5. Cheng X, Liu Z, Yang T, Wang Y, Yan L, Teng L, et al. New method for effective identification of adulterated Camellia oil basing on Camellia oleifera -specific DNA. Arab J Chem. (2017) 11:815–26. doi: 10.1016/j.arabjc.2017.12.025
6. Shi T, Wu G, Jin Q, Wang X. Camellia oil authentication: a comparative analysis and recent analytical techniques developed for its assessment. A review. Trends Food Sci Tech. (2020) 97:88–99. doi: 10.1016/j.tifs.2020.01.005
7. Wang R, Tung Y, Chen S, Lee Y, Yen G. Protective effects of camellia oil (Camellia brevistyla) against indomethacin-induced gastrointestinal mucosal damage in vitro and in vivo. J Funct Foods. (2019) 62:103539. doi: 10.1016/j.jff.2019.103539
8. Guo L, Guo Y, Wu P, Lu F, Zhang T. Camellia oil lowering blood pressure in spontaneously hypertension rats. J Funct Foods. (2020) 70:103915. doi: 10.1016/j.jff.2020.103915
9. Hoffmann J, Gendrisch F, Schempp CM, Wölfle U. New herbal biomedicines for the topical treatment of dermatological disorders. Biomedicines. (2020) 8:27. doi: 10.3390/biomedicines8020027
10. Wang Y, Sun D, Chen H, Qian L, Xu P. Fatty acid composition and antioxidant activity of tea (Camellia sinensis L.) seed oil extracted by optimized supercritical carbon dioxide. In J Mol Sci. (2011) 12:7708–7719. doi: 10.3390/ijms12117708
11. Lee CP, Yen GC. Antioxidant activity and bioactive compounds of tea seed (Camellia oleifera Abel.) Oil. J Agr Food Chem. (2006) 54:779–84. doi: 10.1021/jf052325a
12. Feás X, Estevinho L, Salinero C, Vela P, Sainz M, Vázquez-Tato M, et al. Triacylglyceride, antioxidant and antimicrobial features of virgin Camellia oleifera, C. reticulata and C. sasanqua oils. Molecules. (2013) 18:4573–87. doi: 10.3390/molecules18044573
13. Wang W, Han S, Jiao Z, Cheng J, Song J. Antioxidant activity and total polyphenols content of camellia oil extracted by optimized supercritical carbon dioxide. J Am Oil Chem Soc. (2019) 96:1275–89. doi: 10.1002/aocs.12285
14. Shyu SL, Huang JJ, Yen GC, Chang RL. Study on properties and oxidative stability of teaseed oil. Food Sci. (1990) 17:114–22.
15. Donato-Trancoso A, Monte-Alto-Costa A, Romana-Souza B. Olive oil-induced reduction of oxidative damage and inflammation promotes wound healing of pressure ulcers in mice. J Dermatol Sci. (2016) 83:60–9. doi: 10.1016/j.jdermsci.2016.03.012
16. Lin TK, Zhong L, Santiago JL. Anti-inflammatory and skin barrier repair effects of topical application of some plant oils. Int J Mol Sci. (2017) 19:70. doi: 10.3390/ijms19010070
17. Delgado-Lista J, Perez-Martinez P, Garcia-Rios A, Alcala-Diaz JF, Perez-Caballero AI, Gomez-Delgado F, et al. CORonary Diet Intervention with Olive oil and cardiovascular PREVention study (the CORDIOPREV study): rationale, methods, and baseline characteristics: A clinical trial comparing the efficacy of a Mediterranean diet rich in olive oil versus a low-fat diet on cardiovascular disease in coronary patients. Am heart j. (2016) 177:42–50. doi: 10.1016/j.ahj.2016.04.011
18. Li Y, Lv MR, Wei YJ, Sun L, Zhang JX, Zhang HG, et al. Dietary patterns and depression risk: a meta-analysis. Psychiatry Res. (2017) 253:373–82. doi: 10.1016/j.psychres.2017.04.020
19. La Fata G, Weber P, Mohajeri MH. Effects of vitamin E on cognitive performance during ageing and in Alzheimer's disease. Nutrients. (2014) 6:5453–72. doi: 10.3390/nu6125453
20. Matthäus B, Musazcan Özcan M. Oil content, fatty acid composition and distributions of vitamin-e-active compounds of some fruit seed oils. Antioxidants (Basel, Switzerland). (2015) 4:124–33. doi: 10.3390/antiox4010124
21. Greenwald P, Clifford CK, Milner JA. Diet and cancer prevention. Eur J Cancer. (2001) 37:948–65. doi: 10.1016/s0959-8049(01)00070-3
22. Zhang H, Gao H, Wang T, Zhao K, Luo S, Chen Y, et al. The study of controlling benzo(a)pyrene content in Hainan Shanyou oil by HACCP. Cereal Food Industry. (2016) 23:18-21:25.
23. Meng D, Zhang P, Li S, Ho CT, Zhao H. Antioxidant activity evaluation of dietary phytochemicals using Saccharomyces cerevisiae as a model. J Funct Foods. (2017) 38:36–44. doi: 10.1016/j.jff.2017.08.041
24. Gao Y, Fang L, Wang X, Lan R, Wang M, et al. Antioxidant activity evaluation of dietary flavonoid hyperoside using Saccharomyces cerevisiae as a model. Molecules. (2019) 24:788. doi: 10.3390/molecules24040788
25. Shah DO, Hamlin RM Jr. Structure of water in microemulsions: electrical, birefringence, and nuclear magnetic resonance studies. Science. (1971) 171:483–5. doi: 10.1126/science.171.3970.483
26. Brown A, Anderson D, Racicot K, Pilkenton SJ, Apostolidis E. Evaluation of phenolic phytochemical enriched commercial plant extracts on the in vitro inhibition of α-Glucosidase. Front Nutr. (2017) 4:56. doi: 10.3389/fnut.2017.00056
27. Rafique R, Khan KM, Arshia, Chigurupati S, Wadood A, Rehman AU, et al. Synthesis, in vitro α-amylase inhibitory, and radicals (DPPH & ABTS) scavenging potentials of new N-sulfonohydrazide substituted indazoles. Bioorg Chem. (2020) 94:103410. doi: 10.1016/j.bioorg.2019.103410
28. Karami A, Kavoosi G, Maggi F. The emulsion made with essential oil and aromatic water from Oliveria decumbens protects murine macrophages from LPS-induced oxidation and exerts relevant radical scavenging activities. Biocat Agr Biotech. (2019) 17:538–44. doi: 10.1016/j.bcab.2019.01.015
29. Lu C, Li H, Li C, Chen B, Shen Y. Chemical composition and radical scavenging activity of Amygdalus pedunculata Pall leaves' essential oil. Food Chem Toxicol. (2018) 119:368–74. doi: 10.1016/j.fct.2018.02.012
30. Nayaka N, Fidrianny I, Sukrasno, Hartati R, Singgih M. Antioxidant and antibacterial activities of multiflora honey extracts from the Indonesian Apis cerana bee. J Taibah Univ Med Sci. (2020) 15:211–7. doi: 10.1016/j.jtumed.2020.04.005
31. Benzie IF, Strain JJ. The ferric reducing ability of plasma (FRAP) as a measure of “antioxidant power”: the FRAP assay. Anal Biochem. (1996) 239:70–6. doi: 10.1006/abio.1996.0292
32. Zhu CH, Lei ZL, Luo YP. Studies on antioxidative activities of methanol extract from Murraya paniculata. Food Sci Hum Well. (2015) 4:108–14. doi: 10.1016/j.fshw.2015.07.001
33. Silva CG, Herdeiro RS, Mathias CJ, Panek AD, Silveira CS, Rodrigues VP, et al. Evaluation of antioxidant activity of Brazilian plants. Pharmacol Res. (2005) 52:229–33. doi: 10.1016/j.phrs.2005.03.008
34. Li H, Wang L, Luo Y. Composition Analysis by UPLC-PDA-ESI (-)-HRMS and antioxidant activity using saccharomyces cerevisiae model of herbal teas and green teas from hainan. Molecules. (2018) 23:2550. doi: 10.3390/molecules23102550
35. Wang M, Meng D, Zhang P, Wang X, Du G, Brennan C, et al. Antioxidant Protection of Nobiletin, 5-Demethylnobiletin, Tangeretin, and 5-Demethyltangeretin from Citrus Peel in Saccharomyces cerevisiae. J Agric Food Chem. (2018) 66:3155–60. doi: 10.1021/acs.jafc.8b00509
36. Slatnar A, Jakopic J, Stampar F, Veberic R, Jamnik P. The effect of bioactive compounds on in vitro and in vivo antioxidant activity of different berry juices. PLoS ONE. (2012) 7:e47880. doi: 10.1371/journal.pone.0047880
37. Foor F, Parent SA, Morin N, Dahl AM, Ramadan N, Chrebet G, et al. Calcineurin mediates inhibition by FK506 and cyclosporin of recovery from alpha-factor arrest in yeast. Nature. (1992) 360:682–4. doi: 10.1038/360682a0
38. Satoh M, Kato H, Takayama-Ito M, Ogawa M, Ando S, Saijo M. In vitro evaluation of minimum inhibitory concentration of several antibacterial agents against Rickettsia japonica using a plaque reduction assay system. J Infect Chemother. (2019) 25:917–9. doi: 10.1016/j.jiac.2019.05.019
39. Zhou X, Jiang S, Zhao D, Zhang J, Gu S, Pan Z, et al. Changes in physicochemical properties and protein structure of surimi enhanced with camellia tea oil. LWT - Food Sci Tech. (2017) 84:562–71. doi: 10.1016/j.lwt.2017.03.026
40. Tarhan I. A comparative study of ATR-FTIR, UV-visible and fluorescence spectroscopy combined with chemometrics for quantification of squalene in extra virgin olive oils. Spectrochimica Acta Part A, Mol Biomol Spectrosc. (2020) 241:118714. doi: 10.1016/j.saa.2020.118714
41. Sahari MA, Ataii D, Hamedi M. Characteristics of tea seed oil in comparison with sunflower and olive oils and its effect as a natural antioxidant. J Am Oil Chem Soc. (2004) 81:585–8. doi: 10.1007/s11746-006-0945-0
42. Kwon TH, Kim TW, Kim CG, Park NH. Antioxidant activity of various solvent fractions from edible brown alga, Eisenia bicyclis and its active compounds. J Food Sc. (2013) 78:C679–84. doi: 10.1111/1750-3841.12109
43. Oh SH, Vo TS, Ngo DH, Kim SY, Ngo DN, et al. Prevention of H2O2-induced oxidative stress in murine microglial BV-2 cells by chitin-oligomers. Process Biochem. (2016) doi: 10.1016/j.procbio.2016.08.015
44. Richards DM, Dean RT, Jessup W. Membrane proteins are critical targets in free radical mediated cytolysis. Biochim Biophys Acta. (1988) 946:281–8. doi: 10.1016/0005-2736(88)90403-8
Keywords: camellia oil from Hainan, camellia oil from Guangxi, olive oil, peanut oil, Saccharomyces cerevisiae
Citation: Wang L, Ahmad S, Wang X, Li H and Luo Y (2021) Comparison of Antioxidant and Antibacterial Activities of Camellia Oil From Hainan With Camellia Oil From Guangxi, Olive Oil, and Peanut Oil. Front. Nutr. 8:667744. doi: 10.3389/fnut.2021.667744
Received: 14 February 2021; Accepted: 09 April 2021;
Published: 03 May 2021.
Edited by:
Mohamed Fawzy Ramadan, Umm al-Qura University, Saudi ArabiaReviewed by:
Carmine Summo, University of Bari Aldo Moro, ItalyIbrahim Khalifa, Huazhong Agricultural University, China
Copyright © 2021 Wang, Ahmad, Wang, Li and Luo. This is an open-access article distributed under the terms of the Creative Commons Attribution License (CC BY). The use, distribution or reproduction in other forums is permitted, provided the original author(s) and the copyright owner(s) are credited and that the original publication in this journal is cited, in accordance with accepted academic practice. No use, distribution or reproduction is permitted which does not comply with these terms.
*Correspondence: Yanping Luo, yanpluo2012@hainanu.edu.cn
†These authors have contributed equally to this work