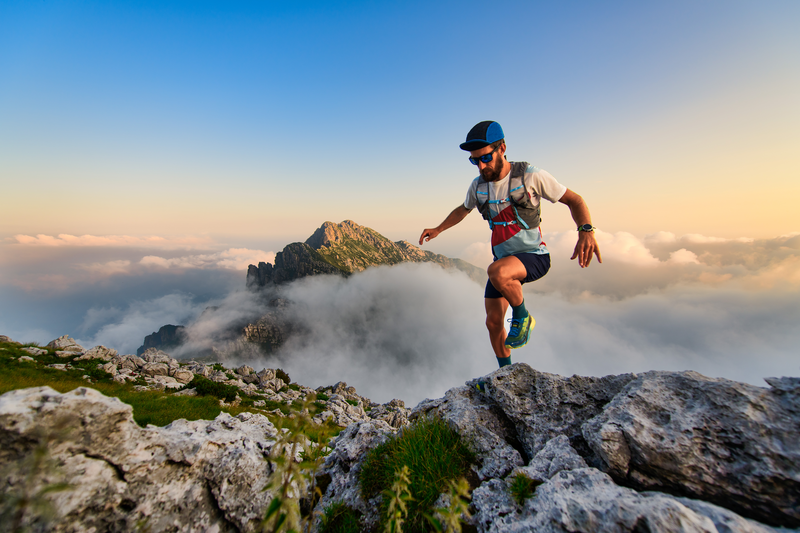
94% of researchers rate our articles as excellent or good
Learn more about the work of our research integrity team to safeguard the quality of each article we publish.
Find out more
REVIEW article
Front. Nutr. , 22 February 2021
Sec. Nutritional Epidemiology
Volume 8 - 2021 | https://doi.org/10.3389/fnut.2021.638740
This article is part of the Research Topic The Impact of Dietary Changes on Non-Communicable Diseases in Latin America View all 12 articles
In the last decades changes in the pattern of health and disease in Latin America and in the world has been observed, with an increase in cases of chronic non-communicable diseases. Changes in intestinal microbiota composition can contribute to the development of these diseases and be useful in their management. In this context, the consumption of fermented foods with probiotic properties, such as kefir, stands out due to its gut microbiota-modulating capacity. There is an increasing interest in the commercial use of kefir since it can be marketed as a natural beverage containing health-promoting bacteria and has been gaining international popularity in Latin America. Also the consumption of these drinks in Latin America seems to be even more relevant, given the socioeconomic situation of this population, which highlights the need for disease prevention at the expense of its treatment. In this narrative review, we discuss how kefir may work against obesity, diabetes mellitus, liver disease, cardiovascular disorders, immunity, and neurological disorders. Peptides, bioactive compounds and strains occurring in kefir, can modulate gut microbiota composition, low-grade inflammation and intestinal permeability, which consequently may generate health benefits. Kefir can also impact on the regulation of organism homeostasis, with a direct effect on the gut-brain axis, being a possible strategy for the prevention of metabolic diseases. Further studies are needed to standardize these bioactive compounds and better elucidate the mechanisms linking kefir and intestinal microbiota modulation. However, due to the benefits reported, low cost and ease of preparation, kefir seems to be a promising approach to prevent and manage microbiota-related diseases in Latin America and the rest of the world.
In the last decades, a major change in global health has been observed (1), with changes in the intestinal microbiota. The formation of intestinal microbiota during childhood is believed to be a key factor in the development of human diseases, such as allergies, neurological disorders and obesity (2–4), which indicates that the intestinal microbiota has a crucial role in the progress of new strategies for maintaining health.
The intestinal microbiota is a set of microorganisms that inhabit the human intestinal tract, and is composed by archaea, bacteria, fungi, helminths, and others (5). In a healthy adult individual, it is believed to be composed of more than 1014 microorganisms (6). According to the World Gastroenterology Organization stomach and duodenum have very low number of microorganisms, mainly lactobacilli and streptococci. Jejunum and ileum have an intermediate amount (104-107 cells per gram) (7) while large intestine has the largest amount with more than 1012 cells per gram, especially anaerobic microorganisms (7, 8). This microbial diversity also suggests the importance of the role that the intestinal microbiota plays in human health, indicating that—unlike what was thought in the past—microorganisms are not necessarily negative or the cause of diseases. On the contrary, it is increasingly clear that they co-evolved with human hosts and the presence of microorganisms can be important for maintaining health (1–4).
Knowledge on the importance of bacteria for human health dates back over 150 years (9), and fermented foods are important in this context. Through fermentation, food has contributed to the evolution of humanity due to its increased conservation capacity and shelf life, development of flavors, and health benefits (10, 11).
Able to resist to the digestive system, beneficial microorganisms occurring in fermented foods are able to act in the intestine. Fermented foods are able to modify the composition of the intestinal microbiota, improve the control of intestinal permeability, increase its barrier function (10–12), activate digestive enzymes, and assist in the production of short-chain fatty acids and vitamins. In addition, fermented foods have bioactive compounds and peptides with prebiotic, antimicrobial, anti-inflammatory, and anti-oxidant activities. Thus, the consumption of fermented foods has been reported to reduce the risk of incidence of certain diseases, such as metabolic syndrome, cardiovascular diseases, diabetes, and cancer; relieves lactose intolerance symptoms; and increases immunity and health in general (10, 11).
Probiotics stand out among fermented foods (10). According to the definition, probiotics are living microorganisms that provide health benefits to those who consume them (13, 14). From the beginning of Elie Metchnikoff's studies (1907) to the present day, Lactobacillus and Bifidobacteria are important species of lactic acid bacteria used as probiotic with evidence of their importance in human health (9, 10). In addition, in the scenario of diseases caused by changes in the intestinal microbiota, the use of probiotics stands out since the probiotics are capable of promoting homeostasis of the intestinal microbiota through mucin production, competition for pathogen adherence, inflammation control, pH change, cytokine production, as well as having immunomodulatory and anti-inflammatory properties, which results in a better healthy condition (7, 15).
Kefir is a fermented product (11, 16) formed by a single culture composed of lactic and acetic acid bacteria and yeast (17). It is a low-cost food, accessible to the general population, easy to handle and with great functional potential (18) due to its bioactive compounds (11, 18) like exopolysaccharides, conjugated fatty acids and peptidases (11, 16). Kefir consumption does not seem to result in negative effects (18) for adult humans (19) or animals (20). In kefir produced with whole milk, high cholesterol content can be observed, and in individuals with intolerance to lacteal proteins, allergic reactions may be observed, that can be avoided by replacing the milk matrices for water and sugar, which reinforces the absence of adverse effects of kefir (21).
Kefir peptides are able to improve parameters related to obesity in Sprague Dawley rats with diet-induced obesity. They act to inhibit lipogenesis by downregulating the fatty acid synthase (FAS) enzyme and increasing the phosphorylated acetyl coenzyme A carboxylase (p-ACC) protein; rising lipid oxidation and the expression of AMP-activated protein kinase (p-AMPK), peroxisome proliferator-activated receptor alpha (PPAR-α) and carnitine palmitoyltransferase 1 (CPT1); and decreasing the inflammatory response and oxidative modulation—with a reduction in tumor necrosis factor alpha (TNF-α), interleukin-1 beta (IL-1β) and transforming growth factor beta (TGF-β) cytokines (22) (Table 1). In addition, the strains found in kefir, such as Lactobacillus harbinensis, Lactobacillus paracasei, and Lactobacillus plantarum, have a role in the tolerance to bile acids and salts, in the adhesion of the intestinal mucosa and in antimicrobial resistance, indicating that bacteria occurring in kefir are capable of standing the gastrointestinal tract, as well as having probiotic and antioxidant activities (43).
Given the above, we will discuss studies with animals and humans that reported the role of kefir and its bioactive compounds on the treatment of diseases characterized by shifts in intestinal microbiota.
Non-communicable diseases are those diseases with a long duration and multifactorial causes which include genetic, psychological, environmental and behavioral factors. Also known as chronic diseases, some examples are cardiovascular disease, cancer and diabetes (44).
The consumption of milk-based fermented drinks is related to the prevention of chronic non-communicable diseases, with an association between the consumption of yogurt and a reduction in weight gain, and lower risk of obesity and cardiovascular diseases (45). Fermented milks also aid in the digestion of lactose and are a source of calcium and protein, therefore presenting interesting nutritional value (18, 46).
The consumption of yogurt and fermented drinks varies widely among countries: Brazil has a consumption similar to the United States (46) being, Argentina and Chile the countries with the highest consumption in Latin America (45).
According to data from the American Study of Nutrition and Health (ELANS), which aims to identify the consumption of food directly related to the occurrence of chronic non-communicable diseases, < 3.5% of the Latin American population has a good consumption of yogurt (45). This consumption is considered low and indicates that there is a consumption gap to be filled, since fermented drinks can contribute to improving the health of those who consume it (46), contributing to the prevention and treatment of chronic non-communicable diseases.
In Brazil, the consumption of yogurt has been increasing among populations with higher income, being typically consumed outside home (47) and, in Latin America, a similar movement is observed, with consumption mainly among the youngest and with highest socioeconomic level (45). Therefore, as an alternative to the consumption of yogurt, we highlight the use of milk kefir, which is also a fermented product, with health benefits due to its probiotic content and the presence of bioactive compounds (18).
Medicines and supplements for the treatment of chronic non-communicable diseases, such as probiotics, can have significant costs for the Latin American population, which may turn their consumption impossible (48). Kefir, on the other hand, can be produced by the consumer himself, using baits acquired by donation, which significantly reduces production and consumption costs (18, 49–51). This allows the use of kefir by a larger portion of the Latin American population. In addition, among the high-income population, kefir consumption also stands out, as there is a tendency, in this audience, to seek healthier and more nutritious foods, such as kefir (52).
The benefits of kefir have not yet been widely publicized among the Latin American population. However, scientific interest in this fermented product is gaining more researchers in Latin America. The antifungal property of kefir, for example, has been studied both in the production of cereals and in the preservation of food. In a study by Gamba et al. (53) there is significant resistance to natural and artificial fungal contamination in arepas (typical dish from Venezuela, Colombia, Bolivia, and Panama) cooked in kefir. In addition, the arepas maintained the organoleptic characteristics of the traditional product after adding kefir, with an improvement in the product's useful life.
The use of kefir to treat diseases has also been studied by Latin American researchers, observing reductions in pre-neoplastic lesions in the intestinal colon (54) and improvement of parameters related to obesity after consumption of this fermented drink (37) in animal models. Better quality of life for individuals with lactose intolerance and osteoporosis (55) has been also observed.
Studies analyzing the effects of kefir on the modulation of the intestinal microbiota (56) and the immune system (57) have also been carried out. In addition, the microbial non-kefir fraction, known as post-biotics (metabolites produced in the fermentation process of dietary components as well as the endogenous components generated by bacteria-host interactions that influence human health) have been widely studied due to their antagonism against pathogenic bacteria such as Escherichia coli, Salmonella spp. and Bacillus cereus (58, 59). This indicates that kefir produced in Latin America is indeed a food with health potential, being interesting its use by the Latin American population.
The components of intestinal microbiota, that is, archaea, fungi, helminths, bacteria and other microorganisms (5), can take two forms: in balance or not. In the first case, known as eubiosis, microbiota tolerates small changes, which may come from the environment, the diet or the water consumed, presenting flexibility to maintain its balance. Cases of major changes, however, such as translocation or growth of a specific bacterial groups, colonization by pathogenic bacteria, use of antibiotics, and changes in lifestyle, lead to imbalance, that is, dysbiosis (60, 61).
Regardless of balance, intestinal microbiota affects the functionality of various organs such as brain, liver, pancreas, intestine, and heart (60, 61). Furthermore, the intestinal microbiota participates in the development and maturation of organs and physiological processes (60), which suggests that modulation of gut microbiota may be a key event for the treatment of diseases and maintenance of health.
Fortunately, modulation of the intestinal microbiota is a reality. Through technological advances, such as interventions with bariatric surgery (62) or fecal transplant (63), or via food (19, 20, 32), the obtained results are promising, showing, in fact, changes in the composition of the intestinal microbiota.
In a longitudinal study that evaluated obese patients undergoing bariatric surgery, body weight reduction and metabolic improvements occurred via modulation of the intestinal microbiota. Compared to individuals of normal weight, patients who underwent bariatric surgery had variation in the Firmicutes, Fusobacteria, and Verrucomicrobia phyla. In addition, after bariatric surgery, the presence of Akkermansia muciniphila was observed. It is a species positively associated with lipid metabolism and negatively associated with adipose tissue inflammation and with circulating levels of glucose, insulin, leptin, and triglycerides, which suggests that its presence indicates improvement in the expression of healthy metabolism markers (62).
Fecal microbiota transplantation also shows its potential modulating role. In a double-blind study with 22 obese patients [body mass index (BMI) ≥ 35 kg/m2] without associated metabolic changes, such as type 2 diabetes, non-alcoholic hepatitis, and metabolic syndrome, the researches worked by offering the transplantation of fecal microbiota (TMF) of a thin donor using capsules (63).
The consumption of TMF capsules was considered safe. A positive modulation of the intestinal microbiota was observed. At the beginning of the experiment, obese individuals had an intestinal microbiota with a specific profile; however, after TMF intake, it was observed a similarity between the microbiota profile of obese patients and that of the thin donor, as well as changes in beta diversity, that is, changes in the species composition of the microbiota (63).
Reduction of taurocholic acid was also observed in the feces of individuals who received TMF (63), and this change is related to the alterations seen in the composition of the intestinal microbiota. Secondary bile acids, for example, are produced in the colon and are influenced by the microbiota present on it. This production varies according to the colonic microbiota and may contribute to a state of health or disease (64).
In addition, bile acids have antibacterial capacity. They also participate in the progression of diseases associated with changes in intestinal microbiota, such as obesity and gastrointestinal diseases, contributing to the modulation of inflammatory processes and signaling energy metabolism events and through their performance as a biological detergent (64). Hence, there is a two-way path where both gut microbiota influences the production of bile acids, and bile acids contribute to intestinal modulation performance.
In a study that sought to evaluate the role of kefir in the modulation of the intestinal microbiota of mice, it was observed that the consumption of this drink was not able to change the total number of bacteria in intestinal microbiota; however, when evaluating the phyla in the group that consumed kefir, a reduction in Enterobacteriaceae and an increase in Lactobacillus and Lactococcus contents were observed during the 3-week intervention period; also, a decrease in Firmicutes and Proteobacteria and an increase in Bacteroidetes, Lactobacillus and Lactococcus at the end of the experiment was observed. On the other hand, there was a significant increase in fecal yeasts after the consumption of kefir (32) (Table 1).
Such results indicate that kefir was able to improve the intestinal microbiota of mice that consumed it, with an emphasis on Enterobacteriaceae reduction. This is considered a pathogenic family, usually dysregulated in situations of behavioral and metabolic changes—such as the consumption of diets which are high in fat and low in fiber, during aging, and in cases of inflammation—(32) emphasizing that such eating habits and inflammation are characteristic of obesity (62, 65–67).
Similarly, a study with dogs confirmed that kefir was able to modulate intestinal microbiota. It decreased the Firmicutes:Bacteroidetes ratio and increased the lactic acid bacteria:Enterobacteriaceae ratio, which suggests an improvement in the animal's health. There were also changes in phylum, family and species level, which indicates that kefir was able to modulate the canine intestinal microbiota (20) (Table 1).
In humans, modulation of intestinal microbiota also occurs after the consumption of kefir, as discussed by Bellikci-Koyu et al. (19) who investigated individuals with metabolic syndrome that were supplemented with kefir for 12 weeks (Table 1). After the intervention, there was a significant increase in Actinobacteria, as well as changes in the genera of the phyla Bacteriodetes and Firmicutes, in the group that consumed kefir (19).
This intestinal modulation interferes with metabolic parameters, characteristic of metabolic syndrome, showing improvement in fasting insulin and insulin resistance index (HOMA-IR), and a decrease in pro-inflammatory cytokines, such as TNF-α and interferon-gama (IFN-γ), and in systolic and diastolic pressure. Moreover, a correlation was observed between these parameters and the intestinal microbiota: body weight gain and BMI were positively correlated with the relative abundance of Firmicutes and Proteobacteria and negatively correlated with the relative abundance of Clostridia. Correlations between the composition of the intestinal microbiota and the amount of fat mass, waist circumference, LDL-cholesterol, homocysteine, insulin, and blood pressure were also found (19).
There are many factors that can alter the composition of the intestinal microbiota in humans, such as age, sex, initial formation of intestinal microbiota, food consumption—with emphasis on fiber consumption–, lifestyle, and use of medications—especially antibiotics (1–4). Therefore, the results observed by Bellikci-Koyu et al. (19) are relevant, paving the way for further studies that prove the modulation of the human intestinal microbiota through the use of kefir in individuals with metabolic syndrome.
Dysbiosis and other determinants of intestinal microbiota formation in childhood, such as birth via cesarean section or formula feeding, are associated with a higher risk of developing obesity, both in child and adulthood (3, 68). Children treated with antibiotics, specially macrolides, amoxicillin, cefdinir, vancomycin, and tetracyclines (69) had a higher incidence of obesity (3), since antibiotics can alter the composition of the intestinal microbiota, leading to a dysbiotic state (3, 70). In addition, the intestinal microbiota is demonstrably different in obese and eutrophic conditions (62, 71), which reinforces the relationship between intestinal microbiota and obesity. Thus, obesity and overweight, characterized by the excess or accumulation of body fat with a consequent increase in health risk (72), can also be influenced by the intestinal microbiota.
Kefir could act against obesity by inhibiting enzymes related to the digestion of carbohydrates and lipids, which will result in less energy release. For example, Tiss et al. (39) using a fermented drink with kefir produced from soymilk, evaluated the activity of lipase and α-amylase in vitro and in the intestine and pancreas of rats under high-calorie diet-induced obesity (Table 1).
In the in vitro analysis, the study showed the ability of kefir to inhibit α-amylase and pancreatic lipase. Authors assumed that this ability is related to the presence of isoflavone aglycones, such as genistein, daidzein and glycitein, present in the drink after the fermentation process. In the in vivo section, the obese animals treated with the fermented drink were more stimulated to perform physical activity. Intestinal and pancreatic lipase activity decreased in the groups receiving kefir, leading to a reduction in total cholesterol and LDL-cholesterol, and an increase in HDL-cholesterol rates, as well as body weight loss. Inhibition of intestinal and pancreatic α-amylase activity and, consequently, decreased blood glucose and protection of liver and kidney tissues from toxicity were also observed after kefir consumption; that is, this fermented drink was able to reverse parameters related to obesity (39).
Bourrie et al. (28) also evaluated kefir regarding the reduction of weight gain and plasma cholesterol in C57BL/6 female mice with obesity induced with a diet of 40% calories from fat and 1.25% cholesterol. The animals received 100 μL of kefir or milk over a period of 12 weeks, with 4 different drinks of traditional kefir and 1 of commercial kefir (28) (Table 1).
Among traditional kefir, two types decreased weight gain and plasma cholesterol and one type reduced hepatic triglyceride deposit, which indicates its potential in controlling obesity with improved metabolic function. The difference between the results is due to the different microbiological compositions, viscosity, and pH of fermented drinks (28).
Kim et al. (33) evaluated the anti-obesity effects of kefir in C57BL/6 mice with high-fat diet-induced obesity and non-alcoholic fatty liver disease (Table 1). The Lactobacillus kefiri DH5 strain was able to decrease body weight, adipose tissue and plasma lipid parameters, acting through the reduction of cholesterol in the intestinal lumen and the upregulation of PPARα in adipose tissue. PPARα is a transcription factor involved in the process of lipid oxidation and consequent metabolism of carbohydrates and lipids, noting that its activation is related to an increase in hepatic steatosis and inflammation (73).
Moreover, the animals that consumed this strain presented a variation in the composition of their intestinal microbiota, with a lower number of Proteobacteria and Enterobacteriaceae, when compared to the non-supplemented animals. These results indicate that Lactobacillus kefiri DH5 is a potential probiotic strain for the treatment of obesity (33).
Lim et al. (35) evaluated the effect of exopolysaccharides derived from kefir grains, showing that the beneficial effects found could be related to the viscosity of exopolysaccharides produced by the bacteria present in kefir (Table 1). Authors observed that exopolysaccharides were able to suppress obesity in vitro, through the supply of adipogenesis. Also, reductions in body weight gain, adipose tissue weight and plasma very low-density lipoprotein cholesterol concentration (VLDL) occurred in vivo (35).
Such in vivo results were explained by both the presence of bacterial metabolites and by the product's viscosity, which generates appetite suppression and reduces energy consumption as well as glucose and lipid absorption. Furthermore, the supply of kefir exopolysaccharides was able to increase the abundance of Akkermansia (35). Akkermansia muciniphila undergoes changes according to the diet consumed, modulates the intestinal microbiota, changes inflammatory conditions in adipose tissue, and improves metabolic parameters, like body weight, adiposity, inflammation markers and biochemical parameters, which suggests a great potential for the treatment of obesity (74).
The development of diabetes mellitus is associated with low-grade chronic inflammation. Changes in intestinal permeability, which are favored by an imbalance in the intestinal microbiota, encourage the occurrence of this inflammation, which leads to resistance to systemic insulin, with consequent development of diabetes (3, 75). In addition, factors that affect the formation of the intestinal microbiota—such as maternal health during pregnancy, birth via cesarean section, the use of antibiotics during childhood, and the presence of intestinal dysbiosis in childhood—are also related to the development of diabetes (3).
In a study with Wistar rats with monosodium glutamate-induced metabolic syndrome, it was observed that whole milk kefir (via gavage, for 10 weeks) was able to reduce insulin resistance. Such results were attributed to the calcium content consumed by the animals, as well as the bioactive compounds produced during the fermentation of kefir. Moreover, kefir stimulated uptake of glucose by muscle cells, which contributed to the reduction of insulin resistance (37) (Table 1).
Lactobacillus mali APS1 is a strain isolated from kefir grain that may be useful in the treatment of diabetes. In a study (Table 1) with mice consuming a high-fat diet, the administration of this strain was able to reduce serum glucose and HOMA index, increasing the levels of glucagon-like peptide (GLP-1) and butyrate (29). The reduction in the HOMA index indicates glycemic control (76) and the increase in GLP-1 indicates control of hunger and possible protection of pancreatic beta cells, which are insulin-producing cells, essential for maintaining glycidic homeostasis (77). In addition, a recent review discusses the decrease in butyrate content as a characteristic of intestinal dysbiosis in diabetes (78). Therefore, these results are positive.
In humans, a beneficial role of kefir in the treatment of diabetes mellitus has been also observed, as discussed in a work with 60 diabetic patients, aged 35–65 years. The patients were divided into 2 groups: the kefir probiotic group and the conventional fermented milk group, and both received 600 mL/day of the treatment drink for 8 weeks. After the intervention, patients supplemented with kefir presented lower values of fasting glucose and glycated hemoglobin than those that received the other fermented drink (24) (Table 1).
The healthy outcomes generated by kefir were attributed to its probiotic composition, mainly Lactobacillus and Bifidobacterium. These bacteria present a hypoglycemic effect since they stimulate the production of insulinotropic peptides and glucagon-like peptides, leading to an increase in the uptake of glucose by muscle cells, as well as stimulating the production of hepatic glycogen, which uses the glucose available in the bloodstream (24).
Toxins produced by intestinal microbiota and a picture of metabolic endotoxemia—that is, altered intestinal permeability—allow the development of a low-grade chronic inflammation. This inflammatory condition stimulates the activation of toll-like receptors and macrophages, which generates hepatic and systemic inflammation, explaining the relationship between the intestinal microbiota and the occurrence of liver diseases (75).
In addition to its effect on obesity, the consumption of Lactobacillus kefiri DH5 presented a hepatoprotective effect. The visual aspect of the liver of the animals that consumed this strain was similar to the animals that did not consume a high-fat diet, as well as presenting, microscopically, less lipid accumulation and smaller fat cells (33).
The hepatoprotective capacity of kefir was also assessed by Golli-Bennour et al. (31) who studied the effect of kefir on hepatotoxicity caused by a pesticide: deltamethrine (Table 1). In Wistar rats, the authors observed that deltamethrine altered liver parameters, such as aspartate aminotransferase (AST), alanine aminotransferase (ALT), bilirubin, and cholesterol, when compared to control groups without the pesticide; however, with kefir intake, these parameters were lower. Furthermore, the supply of pesticide and kefir was able to decrease the levels of carbonylated protein and malondialdehyde, as well as increasing the levels of catalase and superoxide dismutase when compared to the group that received only deltamethrine. These protein and lipid peroxidation disorders indicate oxidative stress and toxicity development by the pesticide. On the other hand, kefir was not able to induce oxidative stress by itself, and was able to revert the inflammatory condition (31).
Less histological and DNA damage was also observed in groups treated with pesticide and kefir when compared to the groups that received only deltamethrine, with this histological and genetic disarchitecture resulting from the inflammatory process previously described. It was concluded that kefir was not only able to revert the inflammatory condition but did not generate any damage, since the group treated with kefir alone obtained similar results to the control group. This finding indicates that kefir has a good antioxidant capacity (31), being a potential tool in the prevention and treatment of liver damage caused or mediated by oxidative stress.
The effect of Lactobacillus mali APS1, isolated from sugary kefir grains, was also tested in the fatty liver of rat fed with a high-fat diet, showing a significant reduction in weight, weight gain, hepatic lipid accumulation, and serum levels of AST and ALT. This strain acted through changes in intestinal microbiota composition, reducing the proportion of bacteria associated with non-alcoholic liver diseases and regulating lipid metabolism and oxidative stress response, which leads to suppression of hepatic steatosis progression (29).
Cardiovascular diseases are also related to obesity's intestinal dysbiosis (4). In addition, changes in the intestinal microbiota can lead to the production of compounds such as trimethylamine N-oxide (TMAO), which increase the risk of developing cardiovascular diseases (79, 80).
Tung et al. (40) evaluated the effect of kefir peptides in Apo E –/– mice with high-fat diet-induced atherosclerosis (Table 1). After a 12-week intervention, the consumption of kefir led to a decrease in the evolution of atherosclerotic lesions, with less lipid deposition at the root of the aorta and suppression of the inflammatory immune response, through a reduction in oxidative stress, the accumulation of macrophages and the release of IL-1β and TNF-α cytokines. Moreover, kefir prevented the endothelial adhesion of monocytes, decreasing the evolution of the atherosclerotic lesion. These results indicate that the consumption of kefir could be useful in the prevention and treatment of atherosclerosis (40).
One of the risk factors for the occurrence of cardiovascular diseases is dyslipidemia. In this sense, in the host, there is less diversity of intestinal microbiota and a greater chance of dysbiosis. That is, a greater chance of inflammation and changes in intestinal permeability is observed, with negative consequences for the health of the host (81).
In dyslipidemia, changes in the production of short-chain fatty acids and bile acids have been observed. Short-chain fatty acids are metabolite substrates that participate in energy production, lipogenesis, gluconeogenesis, and cholesterol synthesis. On the other hand, primary bile acids can bind to the farnesoid X receptor, and this molecule is also related to the development of obesity. Both factors participate in lipid metabolism and intestinal microbiota change (81), indicating that modulation of intestinal microbiota may be a therapeutic alternative to prevent dyslipidemia.
Kefir may be an option in the treatment of dyslipidemia. Choi et al. (30) observed that kefir prevented the increase of lipid parameters in mice fed with an obesogenic diet, proposing that kefir acted by preventing lipid intestinal absorption (Table 1). In humans, the consumption of kefir drink (250 ml) for 8 weeks also improved the lipid profile, which was similar to the control group that consumed low-fat milk. This improvement in the lipid profile was related to the loss of body weight, achieved with the consumption of kefir, as well as to the changes generated in the intestinal microbiota, which led to an increase in the production of short-chain fatty acids and bile acids (23) (Table 1); however, it is noteworthy that the amount of kefir offered and the intervention time are fundamental to achieve the desired result for improving dyslipidemia (27) (Table 1).
The correct formation of intestinal microbiota during childhood is fundamental for the complete development of the baby, since the intestinal microbiota is part of the immune system, being important especially in the first months of life, when the rest of the immune system is still forming. In this context, premature babies may have immaturity in the immune, respiratory and neurological systems, suggesting a possible relationship between them (3). In addition, the immune system and the intestinal microbiota play a symbiotic function, maintaining a picture of non-inflammatory homeostasis: in cases of intestinal dysbiosis, there is an activation of the immune system, which leads to changes in the host's immunity. These changes can impair the maturation of the innate immune system or lead to autoimmune diseases, such as type 1 diabetes (61).
The immunomodulatory capacity of kefir has been tested in BALB/c mice by using different concentrations of commercial kefir (diluted at 1/10, 1/50, 1/100, or 1/200 proportions) and pasteurized kefir (diluted at 1/6, 1/10, 1/50, 1/100 proportions). The results show that kefir modulates the immune response in a dose-dependent manner by stimulating intestinal IgA production and inhibiting the Th1-type immune response (41) (Table 1).
Le Barz et al. (34) also evaluated kefir for probiotic strains with immunometabolic properties (Table 1). Lactobacillus rhamnosus Lb102 and Bifidobacterium Bf141 showed good results in the treatment of obesity and in metabolic syndrome, with a reduction in visceral fat and inflammation, and an improvement in glucose tolerance and insulin sensitivity. These results were explained by a modulation of the intestinal microbiota and the maintenance of intestinal integrity by the probiotics (34).
In humans, the action of kefir on intestinal integrity has also been observed. A study encompassing 28 healthy, overweight and asymptomatic adults found that the use of kefir modified serum zonulin concentration (26) (Table 1). Zonulin is a protein that participates in the integrity of tight junctions, that is, the maintenance of intestinal integrity. In the presence of tight junctions, there is control of the passage of molecules through the paracellular content, which prevents the development of inflammatory processes and the occurrence of diseases. However, the increase in zonulin production leads to loss of function of the intestinal barrier with consequent passage of antigens through the paracellular content and activation of the innate immune response (82).
The probiotic capacity of kefir was able to modulate intestinal microbiota composition, which prevented excessive intestinal permeability by increasing serum zonulin concentrations. Consequently, there was a control of low grade chronic inflammation generated in cases of altered intestinal permeability, as has been proposed in obesity (26).
Neurological changes have multifactorial causes and intestinal microbiota also participates in this process. The composition of intestinal microbiota impacts the health of the microglia and the development of neuronal circuits, which contribute to neurological health (4). In addition, individuals with autism have a characteristic intestinal microbiota, which strengthens the relationship between intestinal microbiota and neurological disorders (1–4).
Noori et al. (36) assessed the role of kefir, fermented in both soy and cow's milk, in the treatment of depression, anxiety and cognitive impairment in an animal model subjected to stress due to the use of nicotine (Table 1). For this purpose, the animals were submitted to elevated plus maze (EPM) to assess anxiety, open field test (OFT) to assess locomotor activity and anxiety and forced swim test (FST) to assess depression. Both types of kefir were able to improve anxiety, decrease the severity of depression, and improve cognitive function throughout the treatment. Since kefir is a food rich in tryptophan, which is the precursor of serotonin, it is believed that kefir may be able to act on serotonin metabolism (36). Depression is related to changes in neuroplasticity being serotonin a neuromodulator capable of stimulating the development of neuronal plasticity (83). So, modulation in serotonin is a classic way of treating depression (36, 83).
Kefir has been reported to protect neurons from degradation through its anti-inflammatory capacity. Additionally, kefir may be able to activate receptors in the brain that stimulate learning and memory. So, in view of the cognitive improvement found, kefir can be potentially used for both prevention and treatment of depression and anxiety, especially in cases related to nicotine consumption. The authors believed that the results can be extrapolated to humans; however, further studies are needed to confirm this hypothesis (36).
The ability to modulate the intestinal microbiota and, consequently, anxiety and depression also suggests a positive role of kefir in these diseases (38, 42) (Table 1). Animals fed with kefir presented a specific intestinal microbiota composition, which presumably acts positively on the gut-brain axis. In addition, through analysis of the microbiome, authors suggest that kefir was able to stimulate the production of the gamma aminobutyric acid (GABA). It is hypothesized that Lactobacillus reuteri possibly converted 2-oxoglutarate to glutamate, which was subsequently converted into GABA, because of modulation of the intestinal microbiota by kefir, that leads to an increase in the production of Lactobacillus reuteri, a bacterial strain with benefits to the host's immune and metabolic system (42).
Kefir was also evaluated in an animal model simulating human depression, with stress induced through 7 stressors during 6 weeks. Mice supplemented with Lactobacillus kefiranofaciens ZW3 strain, isolated from kefir, had more movement (which indicates an increase in the ability to explore the environment and socialize), a greater preference for sucrose (which indicates that the animals returned to have pleasure) and a higher amount of water in their stools (which indicates a lower probability of having constipation, which is a condition associated with the occurrence of depression). In addition, there was an improvement in tryptophan metabolism, an increase in anti-inflammatory cytokines, a reduction in pro-inflammatory cytokines, and changes in the composition of the intestinal microbiota, with an increase in Actinobacteria, Bacteroides, Lachnospiraceae, Coriobacteriaceae, Bifidobacteriaceae and Akkermansia, and reduction in Proteobacteria (38).
All of these benefits indicate that the consumption of kefir was able to alter the metabolic pathways that led to the development of depression; however, further studies are needed to identify the optimal dosage to be consumed, so that the benefits of kefir are acquired by the host that consumes it (38).
The role of kefir on neurological diseases has also been studied in humans. For example, Ozcan et al. (25) assessed the consumption of kefir on sleep quality, quality of life and depression in post-menopausal women (Table 1). The consumption of kefir was positively correlated with the quality of life and quality of sleep, which might be a simple strategy, with good cost benefit and an alternative for the treatment of menopause; however, there were no promising results regarding to depression (25).
Studies that evaluate the psychobiotic activities of kefir on depression may be of great relevance, since the consumption of most antidepressants can generate side effects such as weight gain. In a study performed in Canada, for example, there was an association between obesity and high prevalence of antidepressants' prescription. Moreover, a greater prescription of antidepressants in the most severe grades of obesity (classes II and III) is commonly observed. The use of kefir, in this case, is particularly interesting since the use of medications can worsen obesity and decrease the chance of a positive response to treatment (84). Kefir could also be useful for the care of obese and depressed patients, although more intervention studies should be undertaken to properly evaluate its potential.
The inclusion of kefir in the Latin American market presents a good alternative as an adjuvant therapy in non-communicable diseases and may display an economic prognosis, since, in 2016, the value of kefir market in Latin America was amounted to 150.8 million U.S. dollars and it is expected to rise to about 204.7 million U.S. dollars by 2021 (85).
Alterations in gut microbiota, in the form of dysbiosis or metabolic endotoxemia shows systemic activity (Figure 1), since they allow the occurrence of low-grade chronic inflammations that affect the organism as a whole.
Figure 1. The dynamics of changes in the intestinal microbiome can lead to undesirable modifications, such as dysbiosis, triggering low-grade inflammation-related chronic diseases. On the other hand, the use of probiotics and fermented drinks, such as kefir, can have an impact on the regulation of organism homeostasis, with a direct effect on the gut-brain axis, being a possible strategy for the prevention of metabolic diseases.
The modulation of the intestinal microbiota, thus, stands out as a good strategy for the prevention and treatment of diseases. The use of fermented foods with probiotic activity is a nutritional alternative to drug treatments, and kefir, due to the absence of harmful effects regarding its consumption—in animals and humans–, low cost, ease of preparation, and microbiological composition—rich in bioactive compounds, metabolites, and peptides—stands out as a potential food with functional benefits. Besides that, promising effects as immunomodulatory, hypocholesterolemic, antihypertensive and glycemic control are expected. However, it is mandatory to deepen into the molecular mechanisms and the microorganisms involved, and more well-controlled human intervention studies are required.
Kefir is a low-cost fermented product that is gaining interest due to its potential impact in the prevention and treatment of non-communicable diseases. Yet, the microbiological composition of kefir varies according to the geographical location, fermentation matrix (water solution with sugar, whole cow's milk, skimmed cow's milk, goat's milk, donkey milk, among others), environmental conditions (temperature and fermentation time), and grain (g)/drink (mL) ratio used in the fermentation of the product. Also, the presence of yeasts and their proportion in the drink, as well as the production conditions, among others, can generate drinks with different compositions and characteristics (Table 2). Consequently, as a future perspective, it is believed that the next studies will focus on the development of a unified production protocol, as well as on the determination of which microorganisms should be present in the starter culture and the drink, in order to consider that the final product, in fact, can be classified as a kefir.
Table 2. Microbiological and nutritional characterization of the kefir used in the different studies and production protocols (when available).
As previously discussed, there are many factors involved in the production and dosage of kefir. This variability hinders the reproducibility of the results discussed in this narrative review and impairs future meta-analyses, considering this factor as a limitation.
MCGP has been responsible for conception of this article. MCGP and MMD have been involved in aquisition, analysis and interpretation of data, as well as in drafting the manuscript, and revising it critically. JAM and FIM have revised the manuscript critically, giving important intelectual content. All authors have read and approved the final manuscript.
The authors would like to thank Coordenação de Aperfeiçoamento de Pessoal de Nível Superior (CAPES) for granting scholarships in Spain to MCGP and MMD through Programa Institucional de Internacionalização (PRINT). Government of Navarra (Biofood Project, ref 0011-1411-2019) and CIBERobn are also acknowledged.
The authors declare that the research was conducted in the absence of any commercial or financial relationships that could be construed as a potential conflict of interest.
AST, aspartate aminotransferase; ALT, alanine aminotransferase; CPT1, carnitine palmitoyltransferase 1; ELANS, American study of nutrition and health; EPM, elevated plus maze; FAS, fatty acid sintase enzyme; FST, forced swim test; GABA, gamma aminobutyric acid; GLP-1, glucagon-like peptide; HOMA-IR, insulin resistance index; IFN-γ, interferon-gama; IL-1β, interleukin-1 beta; mL, mililiter; OFT, open field test; p-AMPK, AMP-activated protein kinase; PPAR α, peroxisome proliferator-activated receptor alpha; TGF-β, transforming growth factor beta; TMAO, trimethylamine N-oxide; TMF, transplantation of fecal microbiota; TNF-α, tumor necrosis factor alpha.
2. Butel MJ, Waligora-Dupriet AJ, Wydau-Dematteis S. The developing gut microbiota and its consequences for health. J Dev Orig Health Dis. (2018) 9:590–7. doi: 10.1017/S2040174418000119
3. Milani C, Duranti S, Bottacini F, Casey E, Turroni F, Mahony J, et al. The first microbial colonizers of the human gut: composition, activities, and health implications of the infant gut microbiota. Microbiol Mol Biol Rev. (2017) 81:e00036–17. doi: 10.1128/MMBR.00036-17
4. Zhuang L, Chen H, Zhang S, Zhuang J, Li Q, Feng Z. Intestinal microbiota in early life and its implications on childhood health. Genomics Proteomics Bioinformatics. (2019) 17:13–25. doi: 10.1016/j.gpb.2018.10.002
5. Dominguez-Bello MG, Godoy-Vitorino F, Knight R, Blaser MJ. Role of the microbiome in human development. Gut. (2019) 68:1108–14. doi: 10.1136/gutjnl-2018-317503
6. Thursby E, Juge N. Introduction to the human gut microbiota. Biochem J. (2017) 474:1823–36. doi: 10.1042/BCJ20160510
7. World Gastroenterology Organisation. World Gastroenterology Organisation Global Guidelines. (2017). Available online at: https://www.worldgastroenterology.org/guidelines/global-guidelines/probiotics-and-prebiotics/probiotics-and-prebiotics-english (accessed January 12, 2021).
8. Cani PD, Hul MV, Lefort C, Depommier C, Rastelli M, Everard A. Microbial regulation of organismal energy. Nat Metab. (2019) 1:34–46. doi: 10.1038/s42255-018-0017-4
9. Smolyansky J. Probiotics: a historical perspective. In: Watson RR, Victor R, editors. Bioactive Foods in Promoting Health. Probiotics and Prebiotics. Preedy. Medical (2010). p. 43–6. doi: 10.1016/B978-0-12-374938-3.00003-7
10. Kok CR, Hutkins R. Yogurt and other fermented foods as sources of health-promoting bactéria. Nutr Rev. (2018) 76:4–15. doi: 10.1093/nutrit/nuy056
11. Sanlier N, Gökcen BB, Sezgin AC. Health benefits of fermented foods. Crit Rev Food Sci Nutr. (2019) 59:506–27. doi: 10.1080/10408398.2017.1383355
12. Bell V, Ferrão J, Pimentel L, Pintado M, Fernandes T. One health, fermented foods and gut microbiota. Foods. (2018) 7:195. doi: 10.3390/foods7120195
13. Food and Agriculture Organization of the United Nations. Expert Consultation on Evaluation of Health and Nutritional Properties of Probiotics in Food including Powder Milk with Live Lactic Acid Bacteria. Córdoba (2001).
14. Hill C, Guarner F, Reid G, Gibson GR, Merenstein DJ, Pot B, et al. The international scientific sssociation for probiotics and prebiotics consensus statement on the scope and appopriate use of the term probiotic. Nat Rev Gastroenterol Hepatol. (2014) 11:506–14. doi: 10.1038/nrgastro.2014.66
15. Kim SK, Guevarra RB, Kim YT, Kwon J, Kim H, Cho JH, et al. Role of probiotics in human gut microbiome-associated diseases. J Microbiol Biotechnol. (2019) 29:1335–40. doi: 10.4014/jmb.1906.06064
16. Bengoa AA, Iraporda C, Garrote GL, Abraham AG. Kefir micro-organisms: their role in grain assembly and health properties of fermented milk. J Appl Microbiol. (2019) 126:686–700. doi: 10.1111/jam.14107
17. Codex Alimentarius. Codex Standard for Fermented Milks. (2003). Available online at: http://www.fao.org/fao-who-codexalimentarius. (accessed Octber 3, 2020).
18. Rosa DD, Dias MMS, Grzes'kowiak LM, Reis SA, Conceição LL, Peluzio MCG. Milk kefir: nutritional, microbiological and health benefits. Nutr Res Rev. (2017) 30:82–96. doi: 10.1017/S0954422416000275
19. Bellikci-Koyu E, Sarer-Yurekli BP, Akyon Y, Aydin-Kose F, Karagozlu C, Ozgen AG, et al. Effects of regular kefir consumption on gut microbiota in patients with metabolic syndrome: a parallel-group, randomized, controlled study. Nutrients. (2019) 11. doi: 10.3390/nu11092089
20. Kim DH, Jeong D, Kang II-B, Lim HW, Cho Y, Seo KH. Modulation of the intestinal microbiota of dogs by kefir as a functional dairy product. J Dairy Sci. (2019) 102:3903–11. doi: 10.3168/jds.2018-15639
21. Farag MA, Jomaa AS, El-Wahed AA, El-Seedi HR. The many faces of kefir fermented dairy products: quality characteristics, flavour chemistry, nutritional value, health benefits, and safety. Nutrients. (2020) 12:346. doi: 10.3390/nu12020346
22. Tung YT, Chen HS, Wu HS, Ho MS, Chong KY, Chen CM. Kefir peptides prevent hyperlipidemia and obesity in high-fat-diet-induced obese rats via lipid metabolism modulation. Mol Nutr Food Res. (2018) 62. doi: 10.1002/mnfr.201700505
23. Fathi Y, Faghih S, Zibaeenezhad MJ, Tabatabaei SHR. Kefir drink leads to a similar weight loss, compared with milk, in a dairy-rich non-energy-restricted diet in overweight or obese premenopausal women: a randomized controlled trial. Eur J Nutr. (2016) 55:295–304. doi: 10.1007/s00394-015-0846-9
24. Ostadrahimi A, Taghizadeh A, Mobasseri M, Farrin N, Payahoo L, Gheshlaghi ZB, et al. Effect of probiotic fermented milk (kefir) on glycemic control and lipid profile in type 2 diabetic patients: a randomized double-blind placebo-controlled clinical trial. Iran J Public Health. (2015) 44:228–37.
25. Ozcan H, Oskay U, Bodur AF. Effects of kefir on quality of life and sleep disturbances in postmenopausal women. Holist Nurs Pract. (2019) 33:207–13. doi: 10.1097/HNP.0000000000000310
26. Praznikar ZJ, Kenig S, Vardjan T, Cernelič-Bizjak MC, Petelin A. Effects of kefir or milk supplementation on zonulin in overweight subjects. J Dairy Sci. (2020) 103:3961–70. doi: 10.3168/jds.2019-17696
27. St-Onge MP, Farnworth ER, Savard T, Chabot D, Mafu A, Jones PJH. Kefir consumption does not alter plasma lipid levels or cholesterol fractional synthesis rates relative to milk in hyperlipidemic men: a randomized controlled trial. BMC Complement Altern Med. (2002) 2:1. doi: 10.1186/1472-6882-2-1
28. Bourrie BCT, Cotter PD, Willing BP. Traditional kefir reduces weight gain and improves plasma and liver lipid profiles more successfully than a commercial equivalent in a mouse model of obesity. J Funct Foods. (2018) 46:29–37. doi: 10.1016/j.jff.2018.04.039
29. Chen YT, Lin YC, Lin JS, Yang NS, Chen MJ. Sugary efir train Lactobacillus mali APS1 ameliorated hepatic steatosis by regulation of SIRT-1/Nrf-2 and gut microbiota in rats. Mol Nutr Food Res. (2018) 62:e1700903. doi: 10.1002/mnfr.201700903
30. Choi JW, Kang HW, Lim WC, Kim MK, Lee IY, Cho HY. Kefir prevented excess fat accumulation in diet-induced obese mice. Biosci Biotechnol Biochem. (2017) 81:958–65. doi: 10.1080/09168451.2016.1258984
31. Golli-Bennour EE, Timoumi R, Annaibi E, Mokni M, Omezzine A, Bacha H, et al. Protective effects of kefir against deltamethrin-induced hepatotoxicity in rats. Environ Sci Pollut Res Int. (2019) 26:18856–65. doi: 10.1007/s11356-019-05253-4
32. Kim DH, Chon JW, Kim H, Seo KH. Modulation of intestinal microbiota in mice by kefir administration. Food Sci Biotechnol. (2015) 24:1397–403. doi: 10.1007/s10068-015-0179-8
33. Kim DH, Jeong D, Kang IB, Kim H, Song KY, Seo KH. Dual function of Lactobacillus kefiri DH5 in preventing high-fat-diet-induced obesity: direct reduction of cholesterol and upregulation of PPAR- in adipose tissue. Mol Nutr Food Res. (2017) 61:1700252. doi: 10.1002/mnfr.201700252
34. Le Barz M, Daniel N, Varin TV, Naimi S, Demers-Mathieu V, Pilon G, et al. In vivo screening of multiple bacterial strains identifies Lactobacillus rhamnosus Lb102 and Bifidobacterium animalis ssp. Lactis Bf141 as probiotics that improve metabolic disorders in a mouse model of obesity. FASEB J. (2019) 33:4921–35. doi: 10.1096/fj.201801672R
35. Lim J, Kale M, Kim DH, Kim HS, Chon JW, Seo KH, et al. Anti-obesity effect of exopolysaccharides isolated from kefir grains. J Agric Food Chem. (2017) 65:10011–9. doi: 10.1021/acs.jafc.7b03764
36. Noori N, Bangash MY, Motaghinejad M, Hosseini P, Noudoost B. Kefir protective effects against nicotine cessation-induced anxiety and cognition impairments in rats. Adv Biomed Res. (2014) 3:251. doi: 10.4103/2277-9175.146377
37. Rosa DD, Grześkowiak LM, Ferreira CLLF, Fonseca ACM, Reis BEM, Dias MM, et al. Kefir reduces insulin resistance and inflammatory cytokine expression in an animal model of metabolic syndrome. Food Funct. (2016) 7:3390–401. doi: 10.1039/C6FO00339G
38. Sun Y, Geng W, Pan Y, Wang J, Xiao P, Wang Y. Supplementation with Lactobacillus kefiranofaciens ZW3 from Tibetan Kefir improves depression-like behavior in stressed mice by modulating the gut microbiota. Food Funct. (2019) 10:925–37. doi: 10.1039/C8FO02096E
39. Tiss M, Souiy Z, Bem Abdeljelil N, Njima M, Achou L, Hamden K. Fermented soy milk prepared using kefir grains prevents and ameliorates obesity, type 2 diabetes, hyperlipidemia and liver-kidney toxicities in HFFD-rats. J Funct Foods. (2020) 67:1–8. doi: 10.1016/j.jff.2020.103869
40. Tung MC, Lan YW, Li HH, Chen HL, Chen SY, Chen YH, et al. Kefir peptides alleviate high-fat diet-induced atherosclerosis by attenuating macrophage accumulation and oxidative stress in ApoE knockout mice. Sci Rep. (2020) 10:8802. doi: 10.1038/s41598-020-65782-8
41. Vinderola CG, Duarte J, Thangavel D, Perdigon G, Farnworth E, Matar C. Immunomodulating capacity of kefir. J Dairy Res. (2005) 72:195–202. doi: 10.1017/S0022029905000828
42. Wouw Mvd, Walsh AM, Crispie F, Leuven Lv, Lyte JM, Boehme M, et al. Distinct actions of the fermented beverage kefir on host behaviour, immunity and microbiome gut-brain modules in the mouse. Microbiome. (2020) 8:67. doi: 10.21203/rs.2.19926/v1
43. Talib N, Mohamad NE, Yeap SK, Hussin Y, Aziz MNM, Masarudin MJ, et al. Isolation and characterization of Lactobacillus spp. from kefir samples in Malaysia. Molecules. (2019) 24:2606. doi: 10.3390/molecules24142606
44. Forouzanfar MH, Afshin A, Alexander LT, Anderson HR, Bhutta ZA, Biryukov S, et al. Global, regional, and national comparative risk assessment of 79 behavioural, environmental and occupational, and metabolic risks or clusters of risks, 1990–2015: a systematic analysis for the Global Burden of Disease Study 2015. Lancet. (2016) 388:1659–724. doi: 10.1016/S0140-6736(16)31679-8
45. Kovalskys I, Rigotti A, Koletzko B, Fisberg M, Gómez G, Herrera-Cuenca M, et al. Latin America consumption of major food groups: results from the ELANS study. PLoS ONE. (2019) 14:e0225101. doi: 10.1371/journal.pone.0225101
46. Fisberg M, Machado R. History of yogurt and current patterns of consumption. Nutr Rev. (2015) 73:4–7. doi: 10.1093/nutrit/nuv020
47. Ministério da Economia. Instituto Brasileiro de Geografia e Estatística. Pesquisa de Orçamentos Familiares 2017-2018: Análise do Consumo Alimentar Pessoal no Brasil/ IBGE, Coordenação de Trabalho e Rendimento. Rio de Janeiro: IBGE (2020).
48. Kerry RG, Patra JK, Gouda S, Park Y, Shin H-S, das G. Benefaction of probiotics for human health: a review. J Food Drug Anal. (2018) 26:927–39. doi: 10.1016/j.jfda.2018.01.002
49. Santos F, Silva EO, Barbosa AO, Silva JO. Kefir: uma nova fonte alimentar funcional? Diálogos Ciência. (2012) 10:1–14. doi: 10.7447/dc.2012.001
50. Santos JS, Barbosa AGF, Oliveira MPP, Borges KR, Nascimento AS, Amorim GM, et al. Desenvolvimento biotecnológico de bebida funcional à base de kefir de cacau. In: editor Silva FF. Qualidade de Produtos de Origem Animal. Ponta Grossa: Editora Atena; Ponta Grossa (2019). p. 9–15. doi: 10.22533/at.ed.6591912112
51. Sottoriva HM, Melo DR, Matias TCF, Furioso AA, Santos LP, Alves G. Characteristics and properties of kefir. Arquivo Ciências Vet Zool UNIPAR. (2018) 21:141–2. doi: 10.25110/arqvet.v21i4.2018.7340
52. Siqueira KB. O Mercado Consumidor de Leite e Derivados. Circular Técnica 120; Embrapa; Juiz de Fora (2019).
53. Gamba RR, Caro CA, Martínez OL, Moretti AF, Giannuzzi L, De Antoni GL, et al. Antifungal effect of kefir fermented milk and shelf life improvement of corn arepas. Int J Food Microbiol. (2016) 235:85–92. doi: 10.1016/j.ijfoodmicro.2016.06.038
54. Reis AS, Conceição LL, Dias MM, Siqueira NP, Rosa DD, Oliveira LL, et al. Kefir reduces the incidence of pre-neoplastic lesions in an animal model for colorectal cancer. J Funct Foods. (2019) 53:1–6. doi: 10.1016/j.jff.2018.11.050
55. Dinamarca MA, Ibacache-Quiroga C, Ascencio E, Riquelme V, Doberti T, Leiva G. “Yogurt De Pajaritos”: a chilean kefir with properties of interest for lactose intolerance, osteoporosis and its potential applications on human health. J Int Soc Microb. (2015) 72.
56. Bengoa AA, Dardis C, Gagliarini N, Garrote GL, Abraham AG. Exopolysaccharides from lactobacillus paracasei isolated from kefir as potential bioactive compounds for microbiota modulation. Front Microbiol. (2020) 11:583254. doi: 10.3389/fmicb.2020.583254
57. Bengoa AA, Iraporda C, Acurcio LB, Sandes SHC, Costa K, Guimarães GM, et al. Physicochemical, immunomodulatory and safety aspects of milks fermented with Lactobacillus paracasei isolated from kefir. Food Res Int. (2019) 123:48–55. doi: 10.1016/j.foodres.2019.04.041
58. Zanirati DF, Abatemarco M Jr, Sandes SHC, Nicoli JR, Nunes AC, Neumann E. Selection of lactic acid bacteria from Brazilian kefir grains for potential use as starter or probiotic cultures. Anaerobe. (2015) 32:70–6. doi: 10.1016/j.anaerobe.2014.12.007
59. Iraporda C, Abatemarco M Jr, Neumann E, Nunes AC, Nicoli JR, Abraham AG, et al. Biological activity of the non-microbial fraction of kefir: antagonism against intestinal pathogens. J Dairy Res. (2017) 84:339–45. doi: 10.1017/S0022029917000358
60. Iebba V, Totino V, Gagliardi A, Santangelo F, Cacciotti F, Trancassini M, et al. Eubiosis and dysbiosis: the two sides of the microbiota. New Microbiol. (2016) 39:1–12.
61. Weiss GA, Hennet T. Mechanisms and consequences of intestinal dysbiosis. Cell Mol Life Sci. (2017) 74:2959–77. doi: 10.1007/s00018-017-2509-x
62. Palmisano S, Campisciano G, Silvestri M, Guerra M, Giuricin M, Casagranda B, et al. Changes in gut microbiota composition after bariatric surgery: a new balance to decode. J Gastrointest Surg. (2020) 24:1736–46. doi: 10.1007/s11605-019-04321-x
63. Allegretti JR, Zain K, Mullish BH, Chiang A, Carrellas M, Hurtado J, et al. Effects of fecal microbiota transplantation with oral capsules in obese patients. Clin Gastroenterol Hepatol. (2020) 18:855–63. doi: 10.1016/j.cgh.2019.07.006
64. Joyce SA, Gahan CGM. Bile acid modifications at the microbe-host interface: potential for nutraceutical and pharmaceutical interventions in host health. Annu Rev Food Sci Technol. (2016) 7:313–33. doi: 10.1146/annurev-food-041715-033159
65. Bortolin RC, Vargas AR, Gasparotto J, Chaves PR, Schnorr CE, da Boit Martinello K, et al. A new animal diet based on human Western diet in a robust diet-induced obesity model: comparison to high-fat and cafeteria diets in metabolic and gut microbiota disruption. Int J Obes. (2018) 42:525–34. doi: 10.1038/ijo.2017.225
66. Cani PD, Amar J, Iglesias MA, Poggi M, Knauf C, Bastelica D, et al. Metabolic endotoxemia initiates obesity and insulin resistance. Diabetes. (2007) 56:1761–72. doi: 10.2337/db06-1491
67. Fak F, Jakobsdottir G, Kulcinskaja E, Marungruang N, Matziouridou C, Nilsson U, et al. The physico-chemical properties of dietary fibre determine metabolic responses, short-chain fatty acid profiles and gut microbiota composition in rats fed low- and high-fat diets. PLoS ONE. (2015) 10:e0127252. doi: 10.1371/journal.pone.0127252
68. Ficara M, Pietrella E, Spada C, Della Casa Muttini E, Lucaccioni L, Iughetti L, et al. Changes of intestinal microbiota in early life. J Matern Fetal Neonatal Med. (2020) 33:1036–43. doi: 10.1080/14767058.2018.1506760
69. Principi N, Esposito S. Antibiotic administration and the development of obesity in children. Int J Antimicrob Agents. (2016) 47:171–7. doi: 10.1016/j.ijantimicag.2015.12.017
70. Kim H, Sitarik AR, Woodcroft K, Zoratti E, Johnson CC. Birth mode, breastfeeding, pet exposure, and antibiotic use: associations with the gut microbiome and sensitization in children. Curr Allergy Asthma Rep. (2019) 19:22. doi: 10.1007/s11882-019-0851-9
71. Hou YP, He QQ, Ouyang HM, Peng HS, Wang Q, Li J, et al. Human gut microbiota associated with obesity in chinese children and adolescents. Biomed Res Int. (2017) 2017:7585989. doi: 10.1155/2017/7585989
72. Schetz M, De Jong A, Deane AM, Druml W, Hemelaar P, Paolo P, et al. Obesity in the critically ill: a narrative review. Intensive Care Med. (2019) 45:757–69. doi: 10.1007/s00134-019-05594-1
73. Pawlak M, Lefebvre P, Staels B. Molecular mechanism of PPARa action and its impact on lipid metabolism, inflammation and fibrosis in non-alcoholic fatty liver disease. J Hepatol. (2015) 62:720–33. doi: 10.1016/j.jhep.2014.10.039
74. Schneeberger M, Everard A, Gómez-Valadés AG, Matamoros S, Ramírez S, Delzenne NM, et al. Akkermansia muciniphila inversely correlates with the onset of inflammation, altered adipose tissue metabolism and metabolic disorders during obesity in mice. Sci Rep. (2015) 5:16643. doi: 10.1038/srep16643
75. Cani PD, Osto M, Geurts L, Everard A. Involvement of gut microbiota in the development of low-grade inflammation and type 2 diabetes associated with obesity. Gut Microbes. (2012) 3:279–88. doi: 10.4161/gmic.19625
76. Antunes LC, Elkfury JL, Jornada MN, Foletto KC, Bertoluci MC. Validation of HOMA-IR in a model of insulin-resistance induced by a high-fat diet in Wistar rats. Arch Endocrinol Metab. (2016) 60:138–42. doi: 10.1590/2359-3997000000169
77. Meier JJ. GLP-1 receptor agonists for individualized treatment of type 2 diabetes mellitus. Nat Rev Endocrinol. (2012) 8:728–42. doi: 10.1038/nrendo.2012.140
78. Noureldein MH, Bitar S, Youssef N, Azar S, Eid AA. Butyrate modulates diabetes-linked gut dysbiosis: epigenetic and mechanistic modifications. J Mol Endocrinol. (2020) 64:29–42. doi: 10.1530/JME-19-0132
79. Tang WHW, Kitai T, Hazen SL. Gut microbiota in cardiovascular health and disease. Circ Res. (2017) 120:1183–96. doi: 10.1161/CIRCRESAHA.117.309715
80. Peluzio MCG, Martinez JA, Milagro FI. Postbiotics: metabolites and mechanisms involved in microbiota-host interactions. Trends Food Sci Technol. (2021) 108:11–26. doi: 10.1016/j.tifs.2020.12.004
81. Schoeler M, Caesar R. Dietary lipids, gut microbiota and lipid metabolism. Rev Endocr Metab Disord. (2019) 20:461–72. doi: 10.1007/s11154-019-09512-0
82. Sturgeon C, Fasano A. Zonulin, a regulator of epithelial and endothelial barrier functions, and its involvement in chronic inflammatory diseases. Tissue Barriers. (2016) 4:e1251384. doi: 10.1080/21688370.2016.1251384
83. Kraus C, Castrén E, Kasper S, Lanzenberger R. Serotonin and neuroplasticity – Links between molecular, functional and structural pathophysiology in depression. Neurosci Biobehav Rev. (2017) 77:317–26. doi: 10.1016/j.neubiorev.2017.03.007
84. Puzhko S, Schuster T, Barnett TA, Renoux C, Rosenberg E, Barber D, et al. Evaluating prevalence and patterns of prescribing medications for depression for patients with obesity using large primary care data (Canadian Primary Care Sentinel Surveillance Network). Front Nutr. (2020) 7:24. doi: 10.3389/fnut.2020.00024
85. Available online at: https://www.statista.com/aboutus/ (acessed December 3, 2020).
Keywords: kefir, probiotics, health, fermented foods, gut microbiota
Citation: Peluzio MCG, Dias MM, Martinez JA and Milagro FI (2021) Kefir and Intestinal Microbiota Modulation: Implications in Human Health. Front. Nutr. 8:638740. doi: 10.3389/fnut.2021.638740
Received: 07 December 2020; Accepted: 26 January 2021;
Published: 22 February 2021.
Edited by:
Marcia Cristina Teixeira Martins, University of São Paulo, BrazilReviewed by:
Luigi Santacroce, University of Bari Aldo Moro, ItalyCopyright © 2021 Peluzio, Dias, Martinez and Milagro. This is an open-access article distributed under the terms of the Creative Commons Attribution License (CC BY). The use, distribution or reproduction in other forums is permitted, provided the original author(s) and the copyright owner(s) are credited and that the original publication in this journal is cited, in accordance with accepted academic practice. No use, distribution or reproduction is permitted which does not comply with these terms.
*Correspondence: Maria do Carmo Gouveia Peluzio, bXBlbHV6aW9AdWZ2LmJy
Disclaimer: All claims expressed in this article are solely those of the authors and do not necessarily represent those of their affiliated organizations, or those of the publisher, the editors and the reviewers. Any product that may be evaluated in this article or claim that may be made by its manufacturer is not guaranteed or endorsed by the publisher.
Research integrity at Frontiers
Learn more about the work of our research integrity team to safeguard the quality of each article we publish.