- 1Graduate Program in Clinical Biochemistry and Molecular Medicine, Department of Clinical Chemistry, Faculty of Allied Health Sciences, Chulalongkorn University, Bangkok, Thailand
- 2Department of Clinical Chemistry, Faculty of Allied Health Sciences, Chulalongkorn University, Bangkok, Thailand
- 3Leonard Davis School of Gerontology, University of Southern California, Los Angeles, CA, United States
- 4College of Horticulture, Fujian Agriculture and Forestry University, Fuzhou, China
- 5Institute of Pharmacy and Molecular Biotechnology, Heidelberg University, Heidelberg, Germany
- 6Department of Biotechnology, School of Environmental and Chemical Engineering, Dalian Jiaotong University, Dalian, China
- 7Natural Products for Neuroprotection and Anti-Ageing Research Unit, Department of Clinical Chemistry, Faculty of Allied Health Sciences, Chulalongkorn University, Bangkok, Thailand
Vitis vinifea has been used for traditional medicines, food, beverages, and dietary antioxidant supplements. The chemical compositions and biological activities of the fruits and seeds have been extensively investigated. However, the biological effects of the leaves are limited, and its anti-neurodegeneration or antiaging activities are little known. The current work aims to study the beneficial effects of V. vinifera leaf extract on neuroprotective effects in HT22 cells, antiaging, and oxidative stress resistance properties in the Caenorhabditis elegans model. The ethanol extract was characterized by phytochemical composition using gas/liquid chromatography–mass spectrometry and reversed-phase high-performance liquid chromatography. The beneficial effects of V. vinifera ethanol (VVE) extract on antioxidant properties, neuroprotective effects, and the underlying mechanisms were studied by in vitro and in vivo studies. In HT22 cells, we found that VVE has a protective effect against glutamate-mediated oxidative stress-induced cell death. The gene expression of cellular antioxidant enzymes such as CAT, SODs, GSTs, and GPx was upregulated by VVE treatment. Moreover, VVE was also shown to alleviate oxidative stress and attenuate reactive oxygen species accumulation in C. elegans. We demonstrated that VVE could upregulate the expression of stress-response genes gst-4 and sod-3 and downregulate the expression of hsp-16.2. Our results suggest that the oxidative stress resistance properties of VVE are possibly involved in DAF-16/FoxO transcription factors. VVE reduced age-related markers (lipofuscin) while did not extend the life span of C. elegans under normal conditions. This study reports the neuroprotective effect and antioxidant activity of V. vinifera leaf extract and suggests its potential as a dietary or alternative supplement to defend against oxidative stress and age-related diseases.
Introduction
Reactive oxygen species (ROS) imbalance is associated with various neurodegenerative diseases, in particular, Alzheimer's disease (AD) (1, 2). Glutamate is the main excitatory neurotransmitter in the brain, which is considered as one of the initiating factors for neuronal damage (2, 3). A high accumulation of glutamate can cause neuron death via accumulated ROS and impaired mitochondrial function (1). The new AD treatment has been focusing on neuroprotection by means of reducing glutamate-induced oxidative toxicity (4). Natural products from herbs or plant extracts that have antioxidant activity and neuroprotective effects could be a potential alternative treatment in neurodegenerative diseases. Herbal compounds have been considered as potential agents for the prevention of AD.
DMSO, dimethyl sulfoxide; LC-MS, gas/liquid chromatography–mass spectrometry; HPLC, high-performance liquid chromatography; ROS, reactive oxygen species; ABTS, 2,2-azino-bis(3-ethylbenzothiazoline-6-sulfonic acid) diammonium salt; DPPH, 2,2-diphenyl-1-picrylhydrazyl; Juglone, 5-hydroxy-1,4-naphthoquinone; SODs, superoxide dismutase; CAT, catalase; GPx, glutathione peroxidase; GSTs, glutathione-S-transferase; SOD-3, superoxide dismutase-3; GST-4, glutathione S-transferase 4; DAF-16/FoxO, Forkhead box protein O.
Vitis vinifera L. (grape) has been used for food, beverages, and traditional medicine. The leaves have been used in hemorrhoid and diabetic treatments (5). The therapeutic effects are mainly attributed to the phenolic compounds in the fruits, including flavonoids, anthocyanins, and proanthocyanidins (6). These compounds have an antioxidant capacity and antibiotic, antiallergic, antidiarrhea, antiulcer, and anti-inflammatory effects (7, 8). Evidence suggests that the grape seed and skin extracts have a lifespan-extending effect in C. elegans (9). The leaf extract of V. vinifera has antioxidant and anti-inflammatory activities (10). However, the neuroprotective effects and oxidative stress resistance properties of V. vinifera leaf extract in C. elegans have not been reported.
In the current study, the neuroprotective effects of V. vinifera leaf extract against glutamate-induced cytotoxicity in HT22 cells, oxidative stress resistance properties, and antiaging in C. elegans were investigated. This study reports novel neuroprotective effects and antioxidant activity of the V. vinifera leaf extract and suggests novel dietary supplements to defend against oxidative stress and age-associated neurodegenerative diseases.
Materials and Methods
Chemicals and Reagents
5-Hydroxy-1, 4-naphthoquinone (Juglone) and 2, 7-dichlorofluorescein diacetate were purchased from Sigma-Aldrich GmbH (Steinheim, Germany) and sodium azide from AppliChem GmbH (Darmstadt, Germany). Two, 2-Azino-bis (3-ethylbenzothiazoline-6-sulfonic acid) diammonium salt (ABTS), 2, 2-diphenyl-1-picrylhydrazyl (DPPH), dimethyl sulfoxide (DMSO), Folin–Ciocalteu reagent, L-glutamic acid, quercetin, fetal bovine serum, and Dulbecco's modified Eagle's medium (DMEM) were obtained from Sigma-Aldrich (MO, USA). Gallic acid was purchased from TCI America (OR, USA), 3-(4, 5-Dimethylthiazol-2-yl)-2, 5-diphenyltetrazolium bromide (MTT) from Bio Basic (Ontario, Canada), and Trizol from Invitrogen (CA, USA). Penicillin/Streptomycin solution was purchased from Gibco (MA, USA), CytoTox 96® kit for lactate dehydrogenase (LDH) assay from Promega (WI, USA), reverse transcription (RT) PreMix, and quantitative polymerase chain reaction (qPCR) Master Mix solution from Bioneer (Daejeon, South Korea). The reagents for plant extraction were purchased from RCI Labscan (Bangkok, Thailand).
Plant Material and Extraction
The leaves of V. vinifera were collected from the Pak Chong district, Nakhon Ratchasima Province, Thailand (14.7125°N, 101.421944°E) in July 2016. A voucher specimen of V. vinifera (BCU-016295) has been deposited at the herbarium of Kasin Suvatabhandhu, Department of Botany, Faculty of Science, Chulalongkorn University, Thailand.
The leaves of V. vinifera were dried at shadow for 1–2 weeks and were grounded into a powder. The powder sample (40 g) was subjected to sequential extraction with solvents of different polarities (hexane, dichloromethane, and ethanol at boiling temperature 70–80°C) by Soxhlet for 36 h (11, 12). The supernatants were combined, subsequently filtered (Whatman No. 1 filter paper), and evaporated at 35–45°C by using a vacuum evaporator. The crude extracts were stored at −20°C as a stock. The residue was dissolved in DMSO to a final concentration of 100 mg/ml as a stock solution before the experiments.
The extraction yields of hexane, dichloromethane, and ethanol fractions were 1.32, 0.35, and 18.93%, respectively. The therapeutic effects of V.vinifera products are mainly attributed to the phenolic compounds (6). Ethanol has been frequently used as a solvent for polyphenol extraction and is safe for human consumption (13). Moreover, the ethanol fraction showed the highest yield compared with hexane and dichloromethane fractions. Thus, the V. vinifera ethanol extract was used in this study.
Qualitative Phytochemical Screening
The phytochemical composition of the ethanol extract was analyzed using gas/liquid chromatography–mass spectrometry (LC-MS) (Institute of Systems Biology, University Kebangsaan Malaysia, Malaysia) and reversed-phase high-performance liquid chromatography (HPLC) (RSU Science and Technology Research Equipment Center, Rangsit University, Pathumtani, Thailand) (14) (Supplementary Materials).
In vitro Evaluation of Antioxidant Properties
Radical Scavenging Activity
The antioxidant activity of the V. vinifera ethanol (VVE) extract was determined by measuring the decrease in the absorbance of the stable free radical ABTS and DPPH, following our methods as described previously (15). Briefly, the DPPH and ABTS were prepared in ethanol at 0.2 mg/ml. The reaction consisted of ABTS or DPPH solution and different concentrations of the VVE extract at a 9:1 ratio. The mixture was incubated in the dark for 30 min at RT. The absorbance values of DPPH and ABTS were measured at 734 nm or 517 nm, respectively, using an EnSpire® Multimode Plate Reader (Perkin-Elmer). The percent inhibition values of the radical and IC50 were calculated as described previously (15). The antioxidant capacity was expressed as vitamin C equivalent antioxidant capacity in milligrams per gram of dry weight plant extract (15).
Total Phenolic Content
The assay was carried out according to the Folin–Ciocalteu method and described in our previous work (15). In brief, a Folin–Ciocalteu's phenol reagent (10-fold diluted) and the extract (1 mg/ml) were mixed in a 1:1 ratio and incubated for 20 min. Next, a 7.5% (w/v) Sodium carbonate solution was added to the mixture and kept in the dark at RT for 20 min. The absorbance was read at 760 nm using an EnSpire® Multimode Plate Reader (Perkin-Elmer) as described previously (15). The calibration curve of standard (gallic acid) was used to calculate the total phenolic content, expressed as gallic acid equivalents (GAE.g of plant extracts).
Total Flavonoid Content
The assay was carried out according to the aluminum chloride colorimetric method and described in our previous work (15). Briefly, the extract was mixed with 10% (v/v) aluminum chloride solution and 1-M sodium acetate solution, followed by incubating for 40 min in the dark. After that, the absorbance was measured at 415 nm. The calibration curve of standard (quercetin) was used to calculate the total flavonoid content, expressed as quercetin equivalents (QE.g of plant extracts).
Cell Culture
Mouse hippocampal HT22 cells were obtained from Professor David Schubert (Salk Institute, San Diego, CA, USA) and cultured in DMEM supplemented with 10% fetal bovine serum and 1% penicillin/streptomycin under 5% carbon dioxide at 37°C.
Cell Treatment
HT22 cells were seeded in tissue culture plates (5,000 cells/well in 96 well-plates, 8,000–10,000 cells/well in 12 well-plates) for 12–18 h. After that, cells were treated with different concentrations of VVE extract (10–100 μg/ml) for 48 h. To induce 40–50% cell toxicity, the culture medium was added with 5-mM glutamate and incubated for 18 h. Stock solutions of glutamate and VVE extract were prepared in DMEM and DMSO, respectively. For the control group, cells were treated with 0.1% (v/v) DMSO.
Determination of Cell Viability
Cell viability was evaluated by using MTT and LDH assay (Supplementary Materials).
Measurement of Intracellular Reactive Oxygen Species in HT22 cells
ROS production was quantified by the dichlorofluorescein-diacetate (DCFH-DA) method (Supplementary Materials).
RNA Isolation and Quantitative Reverse Transcription Polymerase Chain Reaction
Total RNA was extracted using the Trizol reagent (Invitrogen) following the manufacturer's instructions. Reverse transcription was done according to the recommended manufacturers' protocols of AccuPower RT PreMix (Bioneer). The q-PCR was performed in an Exicycler™ 96 (Bioneer). The PCR results were measured using fluorescent signals. The PCR conditions were: 95°C for 15 min, denaturation at 95°C for 15 s for 45–55 cycles, and primer annealing/extension at 55°C for 30 s. The primer specificity test was performed by melting curve. β-actin (internal control gene) was used to normalize the relative expression levels by using the 2−ΔΔCT method. The gene-specific sequences of primers were CAT, SOD1, GPx, GSTo1, GSTa2, and β-actin (3) (Supplementary Materials).
C. elegans Strains and Culture Conditions
The strains N2 (wild type), TJ375 [gpIs1(hsp-16-2::GFP)], TJ356 [zIs356 (daf-16p::daf-16a/b::GFP+rol-6)], CF1553 {muls84[pAD76(sod-3::GFP)]}, CF1038 [daf-16(mu86)I], BA17 [fem-1(hc17)IV], CL2166 [(pAF15)gst-4p::GFP::NLS], and Escherichia coli OP50 were obtained from the Caenorhabditis Genetics Center at the University of Minnesota, USA. All strains were maintained at 20°C and cultured on nematode growth media (NGM) plates with living E. coli OP50. For all assays, the larvae (L1 stage) were seeded in liquid medium (S-medium), inoculated with E. coli OP50. Synchronous populations were obtained by using hypochlorite treatment (5-M sodium hydroxide and 5% sodium hypochlorite). The eggs were allowed to hatch in M9 buffer as described previously (15, 16). For the treatment groups, worms were treated with different concentrations of VVE extract: 25, 50, and 100 μg/ml. For the control group, worms were treated with 0.1% (v/v) DMSO.
Survival Assay Under Juglone-Induced Oxidative Stress
L1 larvae of wild-type (N2) and CF1038 transgenic strains were treated with different concentrations of VVE extract in S-medium for 48 h. After treatment, worms were exposed to the pro-oxidant juglone at 80 μM for 24 h. The dead and live worms were counted by gentle touch with a platinum wire.
Measurement of Intracellular Reactive Oxygen Species in C. elegans
L1 larvae of wild-type (N2) and CF1038 transgenic strains were treated with different concentrations of VVE extract in S-medium for 48 h. After treatment, ROS production was quantified by the DCFH-DA method according to our previous work (15, 17). The 50-μM DCFH-DA was added into S-medium and incubated in the dark at 20°C for 1 h.
Worm images were examined under a fluorescent microscope (Keyence Deutschland GmbH, Neu-Isenburg, Germany) at least 30 worms per group. The relative fluorescence of the whole body was examined using ImageJ software (National Institutes of Health, Bethesda, MD). The results are presented as mean fluorescence ± SEM.
Quantification of hsp-16.2 Expression
L1 larvae of TJ375 transgenic worms, which carry hsp-16.2 promoter regions fused with a green fluorescent protein (GFP) reporter, were treated with different concentrations of VVE extract in S-medium at 20°C for 72 h. Then, the worms were induced by exposing a nonlethal dose of 20-μM juglone for 24 h. After incubation, worms were anesthetized by the addition of 10-mM sodium azide. Then, worms were mounted on a microscopic glass slide. The expression of hsp-16.2 was examined by observing the fluorescence at the anterior part from the back of the pharynx as described previously (15, 18).
Quantification of sod-3 Expression
L1 larvae of CF1553 transgenic worms, which carry sod-3 promoter regions fused with a GFP reporter, were treated with different concentrations of VVE extract in S-medium at 20°C for 72 h. Then, worms were submitted to fluorescence microscopy as described previously (15, 18).
Quantification of gst-4 Expression
L1 larvae of CL2166 transgenic worms, which carry gst-4 promoter regions fused with a GFP reporter, were treated with different concentrations of VVE extract in S-medium at 20°C for 48 h. Next, the worms were induced by exposing a nonlethal dose of 20-μM juglone for 24 h. Fluorescence images were taken by fluorescence microscopy as described previously (15, 18).
Determination of Subcellular Localization of DAF-16
L1 larvae of TJ356 transgenic worms were treated with different concentrations of VVE extract in S-medium for 48 h and submitted to fluorescence microscopy as described previously (15, 18).
Assessment of Autofluorescent Pigment
BA17 transgenic worms were used for measuring the accumulation level of the autofluorescent pigment lipofuscin. L1 larvae of BA17 transgenic worms were treated with different concentrations of VVE extract in S-medium and maintained at 25°C to prevent egg-laying. The media was changed every second day. On day 16, the worms were anesthetized by the addition of 10-mM sodium azide, mounted on a glass slide, and photographed. Worms were photographed using a BIOREVO BZ-9000 fluorescence microscope (λex 360/20 nm, λem 460/38 nm) as described previously (15).
Longevity Assay
The wild-type (N2) worms were used for the lifespan assay under normal conditions. Synchronization and treatment were conducted as described previously (15). In brief, N2 worms were synchronized at the L4 larval stage on NGM agar plates supplemented with VVE extracts and E. coli OP50 at 20°C. The treatment plate was prepared by mixing VVE extracts (final concentration 50 μg/ml) with E. coli OP50 and adding on NGM agar plate overnight before use. The worms were counted during the transfer to fresh medium every day. After that, the percentages of surviving worms were documented. Worms that failed to respond to a gentle touch with a platinum wire were scored as dead and excluded from the plates. Internal hatched progeny worms were scored as censors and discarded from the assay.
Brood Size and Body Length Assay
To analyze the potential toxic effect of the extract on the reproductive system, brood size was measured as described in our previous work (15, 18) (Supplementary Materials).
Statistical Analysis
In these studies, the results are presented as the mean ± SEM and were analyzed with GraphPad Prism 8. The experiments were performed in at least triplicate. One-way analysis of variance (ANOVA) following Bonferroni's method (post hoc) analyzed a comparison between the control and treatments. Differences were considered significant at the P ≤ 0.05 level.
Results and Discussion
Phytochemical Constituents of V. vinifera Ethanol Extract
In this study, LC-MS and HPLC were carried out for the tentative identification of the phytoconstituents in the VVE extract. A phytochemical profile is shown in Figure 1. The detected and identified compounds are listed in Supplementary Table 1 with the corresponding retention and MS/MS fragmentation data.
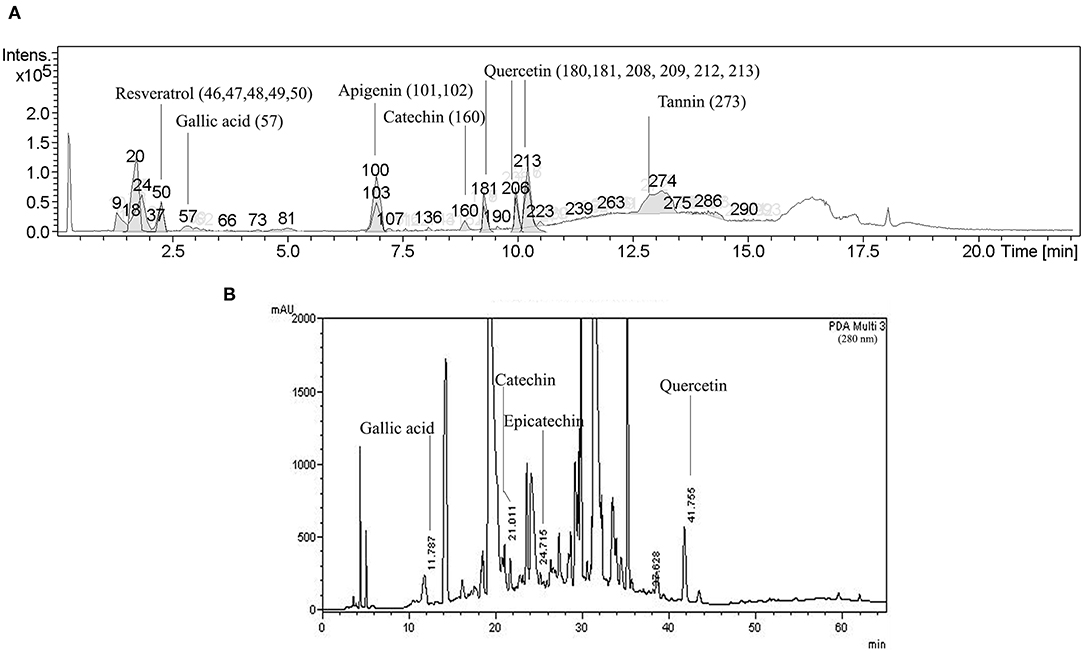
Figure 1. Representative bioactive compounds in VVE extract. LC-MS-MS (A) and HPLC (B) profiles of major compounds in VVE extract.
We tentatively identified the main compounds in the VVE extract, including resveratrol, gallic acid, apigenin, catechin, quercetin, and tannin. Fingerprinting analysis of VVE extracts using HPLC showed the presence of the bioactive compound gallic acid (18.26 mg/100 g of crude extract), catechin (55.10 mg/100 g of crude extract), epicatechin (14.22 mg/100 g of crude extract), and quercetin (197.73 mg/100 g of crude extract) (Supplementary Figure 1B, Table 2). Our results thus agree with the published chemical composition of V. vinifera leaf extracts (6, 10).
In vitro Studies
Effect of V. vinifera Ethanol Extract on Glutamate-Induced Cytotoxicity in HT22 Cells
Excessive glutamate induced oxidative stress leading to neurotoxicity and neuronal cell death (2). The immortalized mouse hippocampal HT22 cells are common cell models to evaluate glutamate toxicity caused by oxidative stress. These cells lack ionotropic glutamate receptors, which exclude excitotoxicity as a cause of glutamate-triggered cell death (2).
To investigate whether the VVE extract could prevent cell death induced by glutamate, the protective effects against glutamate-induced oxidative toxicity were explored in HT22 cells using MTT, LDH assay, and cell morphological examination. First, we determined the non-cytotoxic concentration of the extract and the optimum condition of glutamate in HT22 cells. We found that the VVE extract was relatively non-cytotoxic at the tested doses (10–100 μg/ml VVE, 48 h) (Figure 2a), and the cell viability was reduced by approximately 50% at the tested doses (5-mM glutamate, 18 h) (53.9 ± 0.6% (p < 0.001) (Figure 2b).
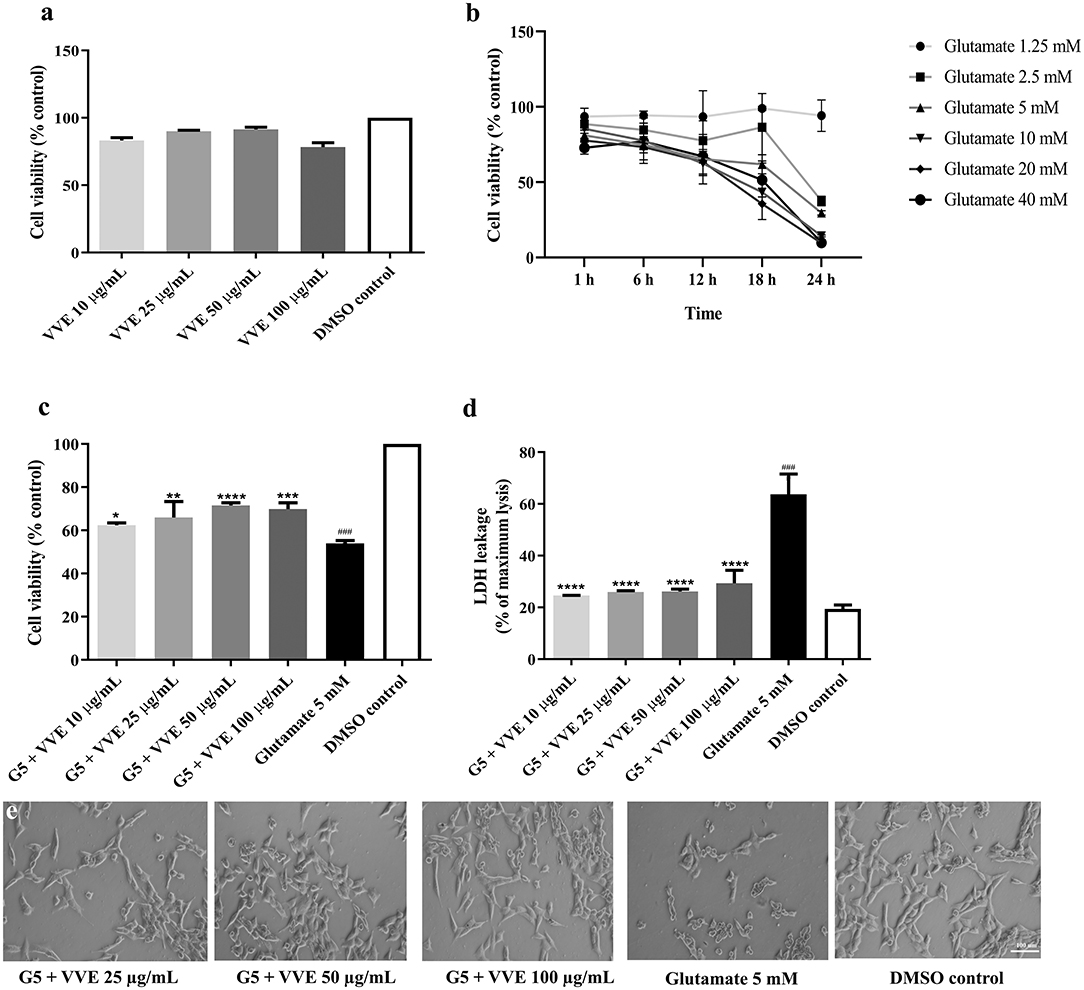
Figure 2. Protective effects of VVE extracts against glutamate-induced toxicity in HT22 cells. Cell viability by treatment with different concentrations of VVE extracts for 48 h (a) and cell viability by treatment with different concentrations of glutamate for different times (b). Cells were treated with different concentrations of VVE extracts for 48 h and exposed to 5-mM glutamate for 18 h. Then, cell viability was measured by MTT (c) and LDH (d) assay. Cell morphology was observed under a microscope at 5 × magnification (e). Samples were exposed to 5-mM glutamate (g) to induce toxicity. All data are shown as mean ± SEM of at least three independent experiments. ####p < 0.0001 vs. DMSO control; *p < 0.05, **p < 0.01, ***p < 0.001, and ****p < 0.0001, compared with glutamate-treated cells by one-way ANOVA following Bonferroni's method (post hoc).
Surprisingly, the viability of the HT22 cells pretreated with VVE extract had significantly lower glutamate-induced cell death compared with that of the cells exposed to glutamate alone [(Figures 2c–e) 10, 25, 50, and 100 μg/ml VVE-induced survival rate against glutamate-induced cell death by 8.4 ± 0.6% (p < 0.05), 11.9 ± 4.3% (p < 0.01), 17.6 ± 0.7% (p < 0.0001), and 14.9 ± 1.7% (p < 0.001), respectively]. The results suggest that VVE extract exerts a potent neuroprotective effect against glutamate-induced cytotoxicity in HT22 cells.
Effect of V. vinifera Ethanol Extract on Glutamate-Induced Oxidative Stress in HT22 Cells
Oxidative stress mediates glutamate-induced neuronal cell death, which plays an essential role in neurodegenerative diseases (2). To investigate whether VVE extract could suppress glutamate-induced oxidative stress, we illustrated the antioxidant properties of VVE extract in vitro and in cells. The VVE extract showed powerful antioxidant activity in vitro with high phenolic and flavonoid contents (Supplementary Figure 1, Table 3). Moreover, the elevated levels of intracellular ROS induced by glutamate were attenuated in the cells pretreated with VVE extract (Figures 3a,c) [10, 25, 50, and 100 μg/ml VVE reduced intracellular ROS levels by 87.1 ± 5.1% (p < 0.0001), 81.1 ± 3.9% (p < 0.0001), 86.9 ± 2.7% (p < 0.0001), and 74.4 ± 1.4% (p < 0.0001), respectively].
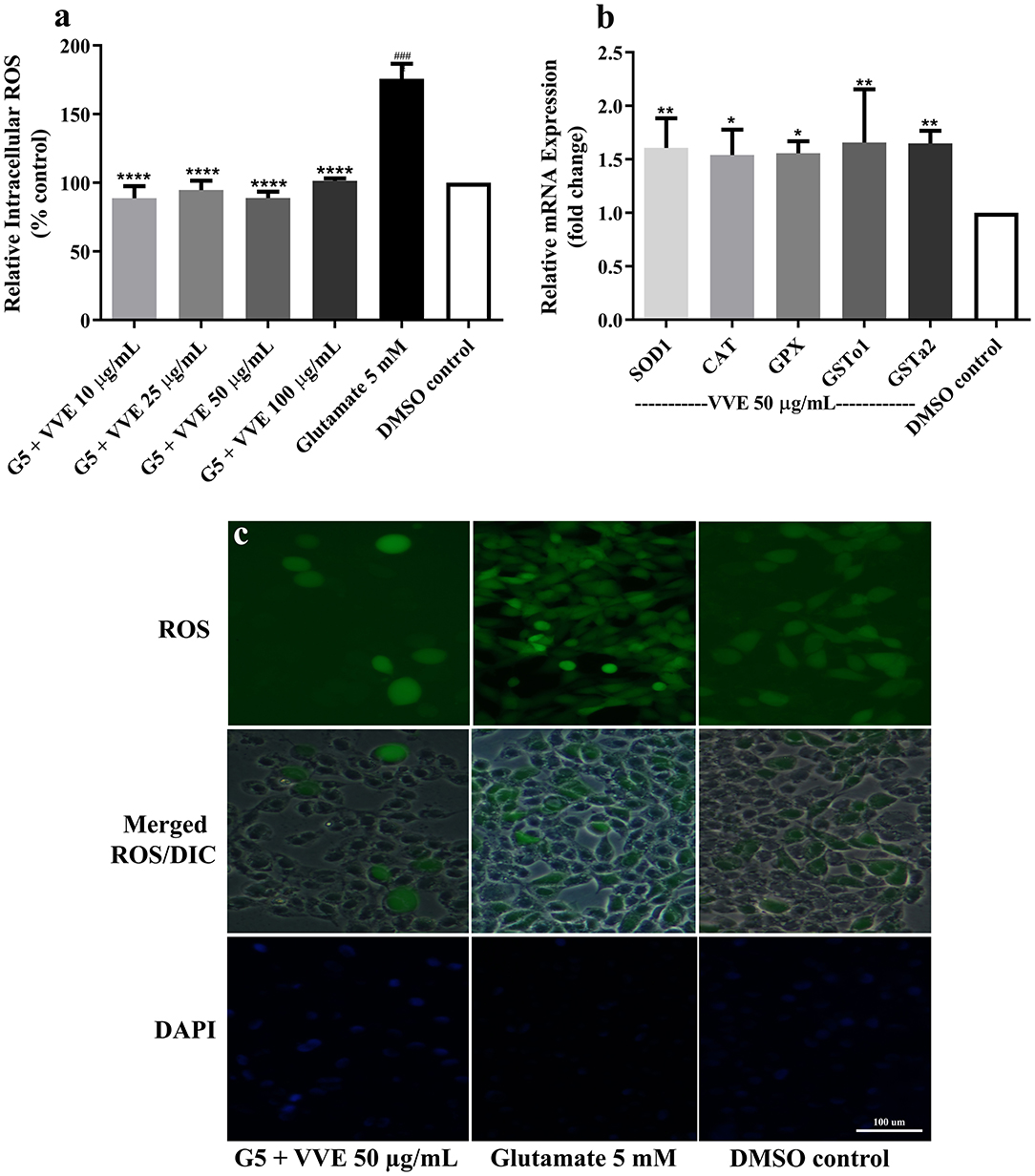
Figure 3. Protective effect of VVE extracts against glutamate-induced oxidative stress in HT22 cells. VVE extracts treatment reduced ROS levels in HT22 cells when compared with glutamate-treated cells (a). VVE extract treatment increased endogenous antioxidant gene expression in HT22 cells when compared with DMSO control (b). Samples were pretreatment with VVE extracts for 48 h and exposed to 5-mM glutamate (G5) for 12 h to induce oxidative stress. Representative fluorescence micrographs of cells stained with H2DCFDA were observed under a fluorescence microscope (c). All data are shown as mean ± SEM of at least three independent experiments. *p < 0.05, **p < 0.01, ***p < 0.001, and ****p < 0.0001, compared with glutamate-treated cells; ###p < 0.001, compared with DMSO control by one-way ANOVA following Bonferroni's method (post hoc).
Both antioxidant properties of VVE extract in vitro and in cells suggest that VVE extract protects against glutamate-induced cytotoxicity by inhibiting the accumulation of intracellular ROS. Previous research has indicated that an antioxidant, such as phenolic and flavonoids, strongly prevented ROS-induced neuronal cell death (19). Neuroprotective properties of resveratrol (20, 21), gallic acid (22), apigenin (21), catechin (23), and quercetin (24) were also highlighted in several recent studies. Our results agreed with literature data indicating that the phenolic compounds (resveratrol, gallic acid, apigenin, catechin, quercetin, and tannin) in VVE extract may mediate antioxidant activity and neuroprotective effects in HT22 cells.
Effect of V. vinifera Ethanol Extract on Gene Expression of Antioxidant Enzymes in HT22 Cells
The antioxidant and phase II enzymes, including superoxide dismutase (SOD), catalase (CAT), glutathione peroxidase (GPx), and glutathione-S-transferase (GST), have been known as a central role of ROS-mediated cellular damage prevention (3). To further examine the mechanism of antioxidant-mediated neuroprotective effects of the VVE extract, we examined the effects of the VVE extract on antioxidant enzyme (SOD, CAT, GPx, and GST) gene expression. We found that VVE extract (50 μg/ml) significantly upregulated the expression of endogenous antioxidant enzymes, including SOD1, CAT, GPx, GSTo1, and GSTa2 (Figure 3b).
Our results are in agreement with other studies where grape leaf extracts (V. vinifera) were found to protect against oxidative damage by promoting antioxidant gene response in several models, including neuronal cells (25), C. elegans (26), and rodents (25, 27, 28). In accordance with previous studies, the bioactive compounds in grape leaf extracts such as resveratrol (29), catechin (29), gallic acid (22), and quercetin (30) also increased antioxidant gene expression.
In the brain, an imbalance of ROS homeostasis is involved in the pathogenesis of several neurodegenerative events (31). Antioxidant balance inside the cells requires intrinsic (endogenous enzymes) and extrinsic (dietary supplements) antioxidants for neutralizing ROS. Natural plants with antioxidant properties have been recognized as precious sources for drug discovery in age-related diseases (24, 32–35). The current results demonstrated that the protective effect of VVE extract against glutamate-induced cytotoxicity is not only through suppressing intracellular ROS production but also through enhancing endogenous antioxidant and phase II enzymes in neuronal HT22 cells.
In vivo Studies
Effect of V. vinifera Ethanol Extract on Juglone-Induced Oxidative Stress in C. elegans
C. elegans is a valuable model for aging research in studying genetic and pharmacological influences of ROS (36, 37). To further elucidate the antioxidant activities of the VVE extract in vivo, the oxidative resistance properties of the VVE extract were conducted in a C. elegans model. We first determined the survival of nematodes under oxidative stress conditions.
Treatment with different concentrations of VVE extract (25–100 μg/ml) caused no significant changes in the survival rate of wild-type worms compared with the DMSO control (Supplementary Figure 2). However, under oxidative stress conditions (80-μM juglone for 24 h), the survival rate of the wild-type worms pretreated with the VVE extract was significantly increased when compared with the DMSO control (21.1 ± 1.9%) (Figure 4a) [25, 50, and 100 μg/ml VVE reduced mortality by 32.8 ± 1.5% (p < 0.01), 31.5 ± 1.8% (p < 0.01) and 33.6 ± 1.2% (p < 0.001), respectively]. Stress resistance properties are closely related to antioxidant activity (31). Although VVE extracts improved the survival rate of the wild-type worms, compared with control, the survival rate did not improve in a similar range as the epigallocatechin gallate, which is a powerful antioxidant in green tea (38). Similarly, VVE extract exhibited lower scavenging activity than epigallocatechin gallate (Supplementary Figure 2). These results suggest that the antioxidant activity of VVE extract might be partially attributed to improving the survival rate.
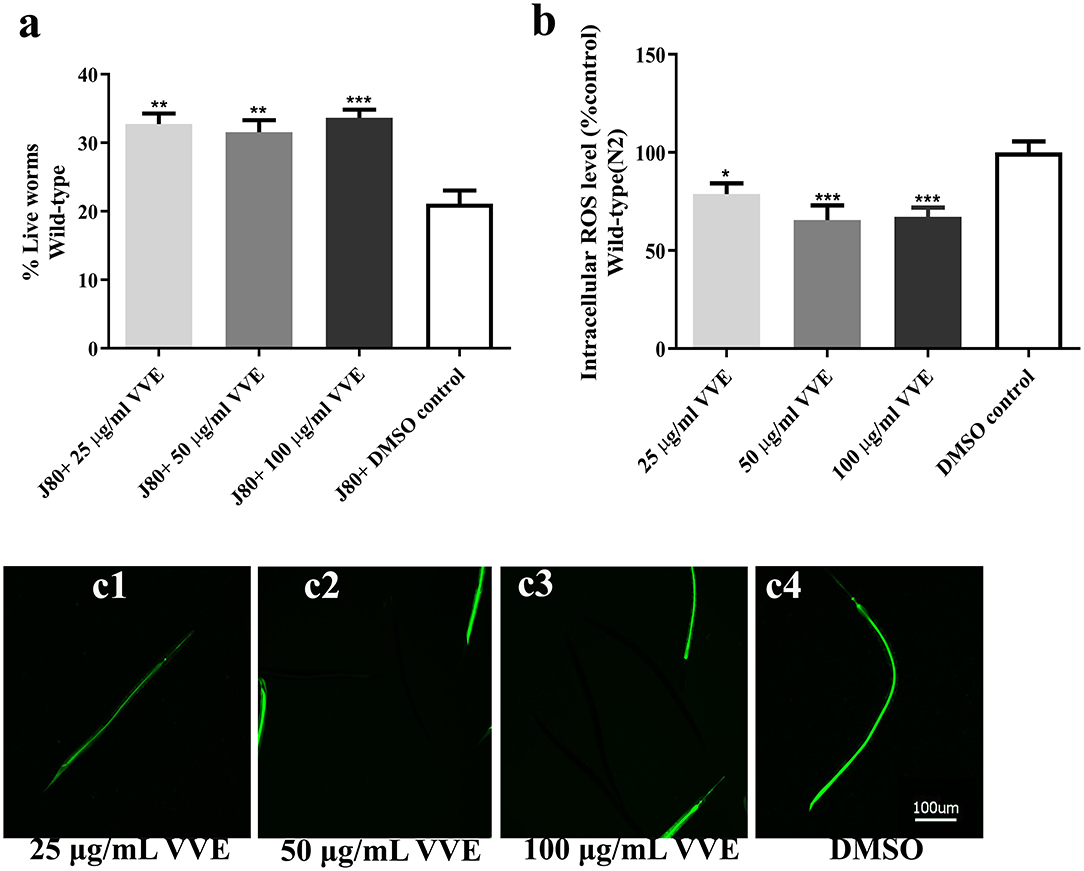
Figure 4. Protective effect of VVE extracts against juglone-induced oxidative stress in C. elegans. VVE extracts protect against oxidative stress in wild-type C. elegans. Survival rate of wild-type (N2) worms was significantly enhanced after pretreatment with extracts (a). VVE extracts treatment reduced ROS levels in N2 worms when compared with DMSO control (b). Representative pictures of DCFDA fluorescence in wild-type (N2) worms treated with 25 μg/ml VVE (c1); 50 μg/ml VVE (c2); 100 μg/ml VVE (c3); and DMSO control (c4). In survival assay, samples were exposed to 80-μM juglone (J) to induce oxidative stress. All data are shown as mean ± SEM of at least three independent experiments.*p < 0.05, **p < 0.01, ***p < 0.001, and ****p < 0.0001, compared with DMSO control by one-way ANOVA following Bonferroni's method (post hoc).
Subsequently, the intracellular ROS accumulation was measured to confirm the antioxidant properties of the VVE extract. The ROS indicator DCFH-DA was used to determine the accumulation of ROS levels in wild-type worms. The fluorescence intensity is correlated with the intracellular ROS level. The intracellular ROS accumulations were significantly reduced in the wild- type worms treated with VVE extract groups [25, 50, and 100 μg/ml VVE reduced intracellular ROS accumulation by 21.2 ± 5.5% (p < 0.05), 34.4 ± 7.5% (p < 0.001), and 32.8 ± 4.8% (p < 0.001), respectively] (Figure 4b) (Representative microscopy images from individual worms can be found in Figure 4c). Interestingly, under oxidative stress conditions (a nonlethal dose of 20-μM juglone), VVE extracts also reduced intracellular ROS level in wild-type worms when compared with the worms exposed to juglone alone [25, 50, and 100 μg/ml VVE reduced intracellular ROS accumulation by 74.1 ± 4.6% (p < 0.0001), 75.2 ± 5.2% (p < 0.0001), and 80.3 ± 4.8% (p < 0.0001), respectively] (Supplementary Figure 3A).
However, we found that the lower concentrations of VVE extract (<10 μg/ml in HT22 cells and 25 μg/ml in worms) neither decreased intracellular ROS accumulation level nor increased survival rate under oxidative stress condition compared with the DMSO control (Supplementary Figure 4). These results suggest that the VVE extracts at moderate concentrations have antioxidant activities.
Previous works have reported that grape seed and skin extracts have antioxidants (26) and lifespan-extending effects in C. elegans (9). The data support our assumption that the VVE leaf extract contains polyphenols (resveratrol, gallic acid, apigenin, catechin, quercetin, and tannin), which have protective effects against oxidative stress to reduce endogenous ROS levels in C. elegans.
Effect of V. vinifera Ethanol Extract on Stress Resistance Properties Mediated by the DAF-16/FoxO Pathway in C. elegans
DAF-16, the C. elegans homolog of the mammalian FOXO transcription factor, is the main transcription factor involved in stress response, metabolism, and longevity (39). DAF-16/FoxO remains inactive in the cytosol under normal conditions. In contrast, stress or specific ligands can stimulate its translocation to the nucleus, influencing stress response genes expression such as hsp-16.2, sod-3, and gst-4 (39).
To examine the influence of VVE extract on DAF-16 nuclear translocation, DAF-16 transgenic (TJ356) (DAF-16::GFP) worms were used. The majority of the worms treated with DMSO control showed a cytosolic DAF-16::GFP localization (63.3 ± 7.3% cytosolic, 23.5 ± 7.7% intermediate, and 13.6 ± 4.9% nuclear). However, VVE extract significantly increased the level of nuclear location of DAF-16::GFP when compared with the DMSO control [25, 50, and 100 μg/ml VVE induced DAF16::GFP nuclear location by 65.6 ± 6.4% (p < 0.001), 63.2 ± 7.4% (p < 0.001), and 52.5 ± 8.5% (p < 0.001), respectively]. (Figure 5c) (Representative microscopy images from individual worms can be found in Figure 5d).
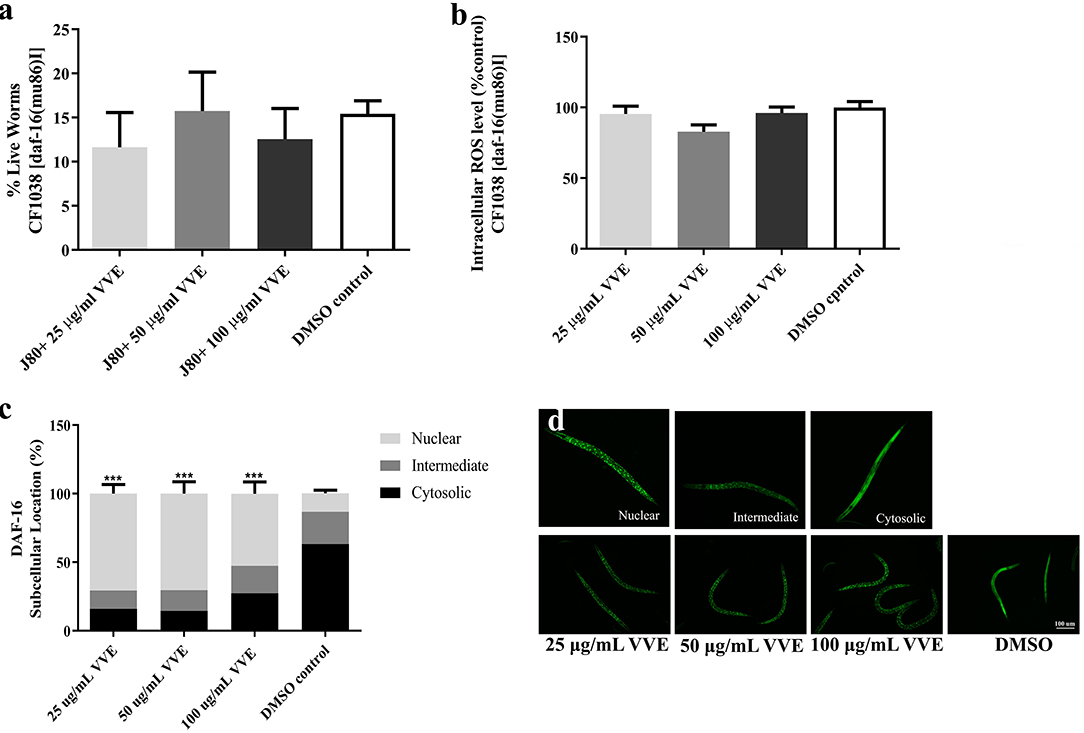
Figure 5. Stress resistance properties of VVE extracts mediated DAF-16/FoxO pathway in C. elegans. VVE extracts failed to increase survival rate (a) and decrease ROS levels (b) in CF1038 worms. Moreover, VVE extracts induced a significant translocation of DAF-16::GFP in mutant TJ356 worms (daf-16p::daf-16a/b::GFP + rol-6) (c). Representative fluorescent images of subcellular location of DAF-16 in nucleus, intermediate, cytosolic regions, and TJ356 worms after treated with VVE extracts (d). All data are shown as mean ± SEM of at least three independent experiments.*p < 0.05, **p < 0.01, ***p < 0.001, and ****p < 0.0001, compared with DMSO control by one-way ANOVA following Bonferroni's method (post hoc).
In oxidative stress conditions, such as juglone treatment, DAF16 has induced translocation into the nucleus (40). We found that the juglone treatment group significantly increased the level of nuclear location of DAF-16::GFP when compared with the untreated control group [by 43.6 ± 7.4% (p < 0.0001)] (Supplementary Figure 3B). However, VVE extract blocked the juglone-induced nuclear translocation of DAF-16, suggesting that VVE extract indeed prevented the juglone-induced oxidative stress in C elegans.
To further investigate the effects of VVE extract that mediates antioxidant activity through the DAF-16/FoxO pathway, the transgenic CF1038 worms, which are the DAF-16 loss-of-function mutant, were used in survival (Figure 5a) and intracellular ROS accumulation assay (Figure 5b). Interestingly, VVE extract failed to increase the survival rate under oxidative stress (Figure 5a) and attenuate intracellular ROS levels (Figure 5b) in CF1038 worms. The data indicate that VVE extract has antioxidant activity and stress resistance in C. elegans through the DAF-16/FoxO pathway.
Effect of V. vinifera Ethanol Extract on Gene Expression of Stress Response (hsp-16.2::GFP, sod-3::GFP, and gst-4::GFP) in C. elegans
More evidence that VVE extract can attenuate oxidative stress was obtained by measuring the expression of DAF-16/FoxO downstream genes (hsp-16.2, sod-3, and gst-4). Heat-shock proteins have been known as a sensor of oxidative stress function induced by oxidative stress and heat shock conditions (39). Under mild oxidative stress conditions (20-μM juglone), the head of the transgenic worms (TJ375) exhibited high-intensity GFP fluorescence representative hsp-16.2 gene induction. However, VVE extract significantly reduced the level of the fluorescence intensity of hsp-16.2::GFP when compared with the DMSO control [25, 50, and 100 μg/ml VVE reduced expression level of hsp-16.2 by 25.9 ± 4.5% (p < 0.0001), 32.4 ± 3.8% (p < 0.0001), and 64.3 ± 1.4% (p < 0.0001), respectively] (Figure 6a).
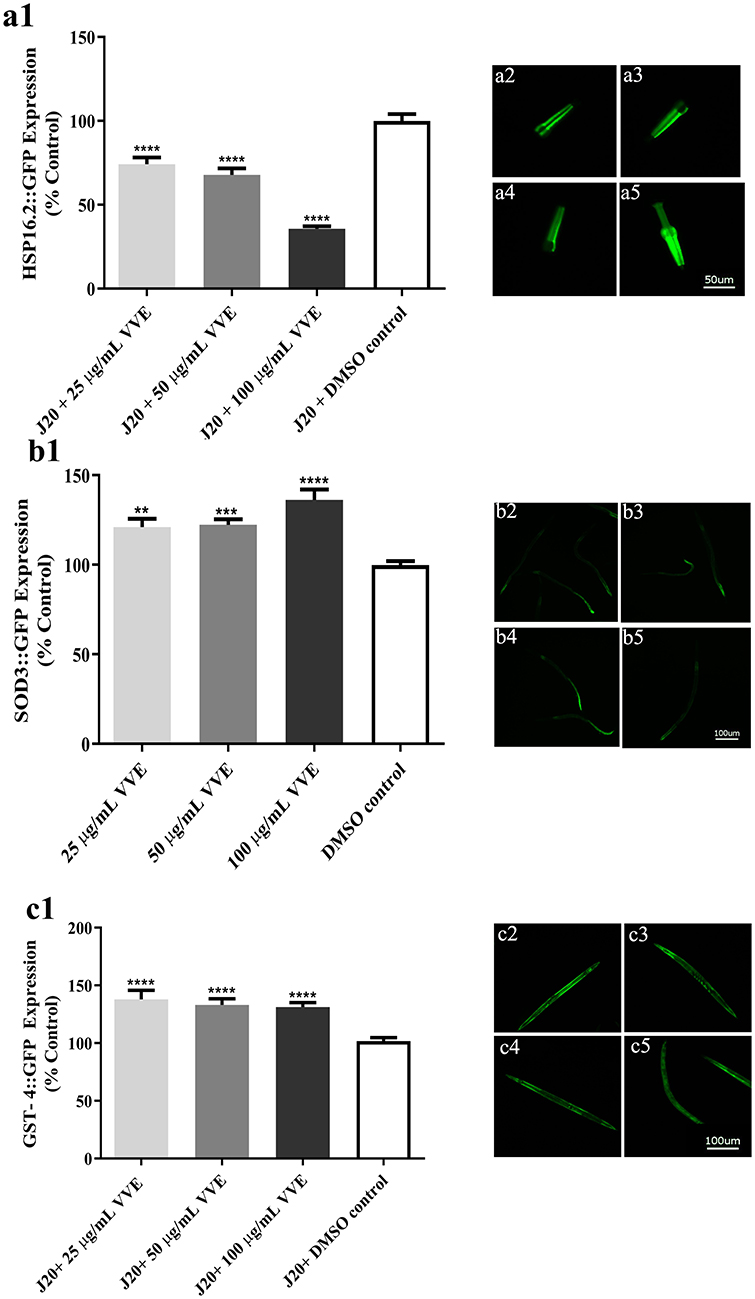
Figure 6. Effect of VVE extracts on expression of stress resistance-related genes in C. elegans. VVE extracts decreased hsp-16.2 expression in mutant TJ375 worms (a), increased sod-3 expression in mutants CF1553 worms (b), and gst-4 expression in mutants CL2166 worms (c). a2–a5, b2–b5, c2–c5: Representative pictures of GFP fluorescence in worms treated with 25 μg/ml VVE (a2/b2/c2); 50 μg/ml VVE (a3/b3/c3); 100 μg/ml VVE (a4/b4/c4); and DMSO control (a5/b5/c5). TJ375 and CL2166 worms were exposed to 20-μM juglone to induce mild oxidative stress. *p < 0.05, **p < 0.01, ***p < 0.001, and ****p < 0.0001, compared with DMSO control by one-way ANOVA following Bonferroni's method (post hoc).
We further investigate the antioxidant properties of the extract by determining the expression of antioxidant enzymes including SOD-3 (superoxide dismutase 3) and GST-4 (glutathione S-transferase 4). We found that the VVE extract significantly increased the level of the fluorescence intensity of sod3::GFP (Figure 6b) and gst-4::GFP (Figure 6c), in transgenic worms CF1553 and CL2166 worms, respectively [sod3::GFP; 25, 50, and 100 μg/ml VVE-induced expression level of Sod-3 by 21.1 ± 4.6% (p < 0.01), 22.3 ± 3.2% (p < 0.001), and 36.1 ± 5.9% (p < 0.0001), respectively] [gst-4::GFP; 25, 50, and 100 μg/ml VVE-induced expression level of gst-4 by 38.0 ± 7.7% (p < 0.0001), 33.1 ± 5.3% (p < 0.0001), and 31.3 ± 3.8% (p < 0.0001), respectively].
The data indicate that VVE extract exhibited antioxidant properties, not only by suppressing intracellular ROS but, additionally, by modulation of the expression of stress-response genes in C. elegans, such as hsp-16.2, sod-3, and gst-4. These abilities were similar to the effects of resveratrol (41), gallic acid (15, 17), catechin (32, 35), and quercetin (15, 24, 42) on oxidative stress resistance in C. elegans via the transcription factor DAF-16/FoxO. Taken together, the results of this study strongly suggest that the VVE extract mediated antioxidant activity and stress resistance in C. elegans via the DAF-16/FoxO pathway. However, further studies are required to clarify the underlying mechanisms of the VVE extract on the neuroprotective effect in C. elegans.
Effect of V. vinifera Ethanol Extract in Aging
C. elegans is a popular model of aging and longevity (36). Several polyphenols have been reported as antiaging agents in C. elegans, such as resveratrol (43), anthocyanin (33), and quercetin (24). To examine the possible influence of VVE extract on aging, the autofluorescent pigment (lipofuscin) accumulation and lifespan were measured. The accumulation of intestinal autofluorescence (lipofuscin) in C. elegans during aging is often used as a marker of health or aging (44). We found that the VVE extract significantly decreased the level of lipofuscin accumulation in late adult worms (16 days) (Figure 7a).
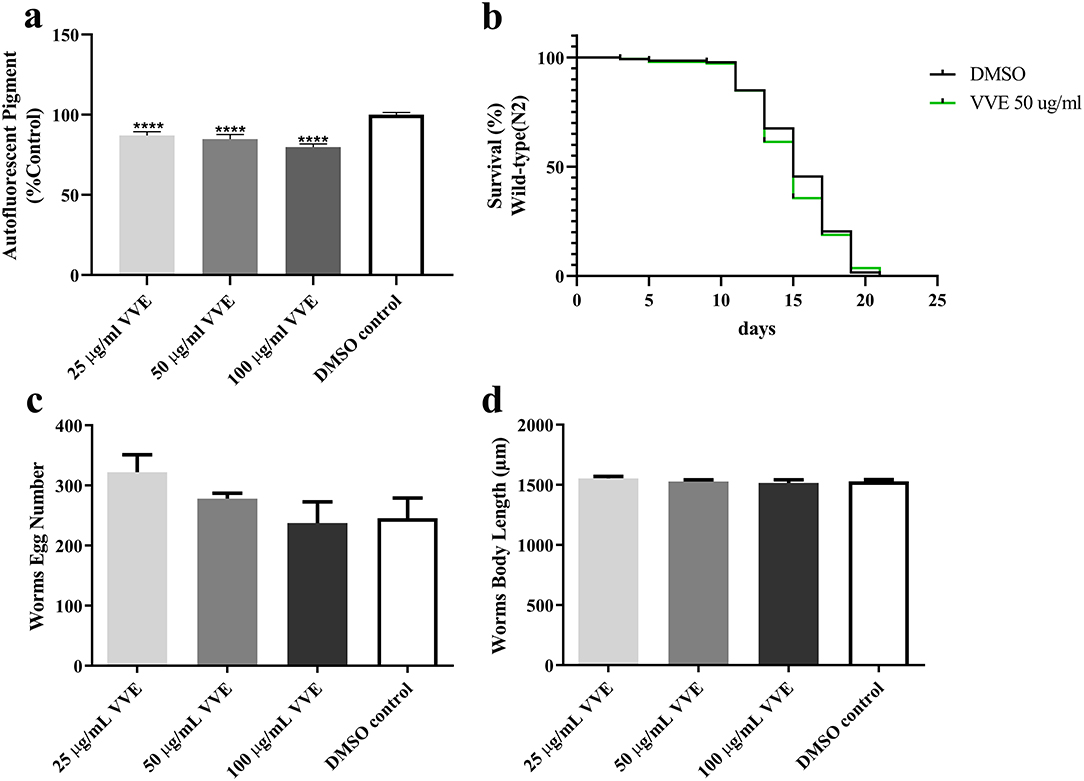
Figure 7. Effect of VVE extracts on aging in C. elegans. VVE extracts attenuated autofluorescent pigment in BA17 worms (a). Autofluorescent granules were measured under blue wavelength band. VVE extracts had no effect on life span of wild-type (N2) worms in normal conditions (b). Brood size (c) and body length (d) of wild-type (N2) worms after VVE extracts treatment. Treatment with VVE extracts had no effect on egg-laying activity and body length. Results are expressed as mean ± SEM from three independent experiments (n = 30 worms in each experiment). Treatment groups are compared with DMSO control by one-way ANOVA following Bonferroni's method (post hoc).
Despite the antioxidant capacity in vitro and in vivo and aging marker reduction, VVE extract did not show any lifespan-prolonging effects in wild-type worms in normal conditions (Figure 7b). These abilities were similar to the effects of resveratrol in the life span of C. elegans under normal conditions (43). However, the resveratrol show strongly increased life span effects in C. elegans under conditions of oxidative stress (43). Possibly, the antiaging effects of VVE extracts are linked to antioxidant effects. The effects of VVE extract on the life span of C. elegans under oxidative stress conditions will be an interesting topic for future study.
To exclude the toxic effect on the reproductive system and dietary restriction system induced by VVE extract, we further measured brood size and body length. Brood size (Figure 7c) and body length (Figure 7d) in wild-type worms were not affected by different concentrations of VVE extract. These data indicated that the effects of VVE extract did not interfere with the fertility rate nor with body development (e.g., via dietary restriction) as mentioned in the literature as toxicity markers (33).
Conclusion
Oxidative stress has been connected to neurodegenerative diseases (1, 2). In this study, HT22 hippocampal neuronal cells and C. elegans models were used to study the protective effects of VVE extract against oxidative stress as in vitro and in vivo studies. We found that the VVE extract protects against glutamate-induced oxidative toxicity in HT22 hippocampal neuronal cells and against juglone-induced oxidative stress in C. elegans. The neuroprotective action of the VVE extract in hippocampal neuronal (HT22) cells is mediated via inhibition of ROS accumulation and enhancing endogenous antioxidant enzymes. In addition, the VVE extract exhibits oxidative resistance properties in C. elegans involved in the DAF-16/FoxO signaling pathway. VVE reduced age-related markers (lipofuscin), although it did not extend the life span of C. elegans under normal conditions. These studies first report the phytochemical constituents and antioxidant properties of V.vinifera leaf extract. The leaf extract might be considered as an alternative supplement or medicine to defend against oxidative stress and neurodegenerative diseases. In vivo intervention studies with more complex model organisms are required to support the therapeutic potential of the VVE extract for age-related neurodegenerative disorders.
Data Availability Statement
The original contributions presented in the study are included in the article/Supplementary Material, further inquiries can be directed to the corresponding authors.
Author Contributions
CD performed the experiments, analyzed data, and was a major contributor in writing the manuscript. PR performed the gene expression assay by RT-PCR. CD, PR, SZ, and XG designed the study and prepared media and reagents. MW review and editing the manuscript. MW and TT provided materials for the study and conceived and supervised research. MW, TT, PR, SZ, and XG corrected the manuscript. All authors contributed to the article and approved the submitted version.
Funding
This work was supported by a scholarship from the 72nd Birthday Anniversary of His Majesty the King's for Doctoral Scholarship, The 90th Anniversary Chulalongkorn University Fund (Ratchadaphiseksomphot Endowment Fund) funding code GCUGR1125603032D No. 29 for research expenses, and also. The Overseas Research Experience Scholarship for Graduate Students from Graduate School, Chulalongkorn University.
Conflict of Interest
The authors declare that the research was conducted in the absence of any commercial or financial relationships that could be construed as a potential conflict of interest.
Acknowledgments
Special thanks are due to Mariana Roxo, Pille Link for providing instruction on C. elegans experiments and discussing the experiments. Our appreciation goes to Dr. Siriporn Chuchawankul (Immunomodulation of Natural Products Research Group, Chulalongkorn University) for her technical support.
Supplementary Material
The Supplementary Material for this article can be found online at: https://www.frontiersin.org/articles/10.3389/fnut.2021.634100/full#supplementary-material
Abbreviations
VVE, Vitis vinifera ethanol; AD, Alzheimer's disease; DMEM, Dulbecco's modified Eagle's medium; LDH, Lactate dehydrogenase; MTT, 3-(4,5-dimethylthiazol-2-yl)-2,5-diphenyltetrazolium bromide; PCR, polymerase chain reaction; DNA, deoxyribonucleic acid; RNA, ribonucleic acid.
References
1. Xu W, Chi L, Xu R, Ke Y, Luo C, Cai J, et al. Increased production of reactive oxygen species contributes to motor neuron death in a compression mouse model of spinal cord injury. Spinal Cord. (2005) 43:204–13. doi: 10.1038/sj.sc.3101674
2. Fukui M, Song JH, Choi J, Choi HJ, Zhu BT. Mechanism of glutamate-induced neurotoxicity in HT22 mouse hippocampal cells. Eur J Pharmacol. (2009) 617:1–11. doi: 10.1016/j.ejphar.2009.06.059
3. Sukprasansap M, Chanvorachote P, Tencomnao T. Cleistocalyx nervosum var. paniala berry fruit protects neurotoxicity against endoplasmic reticulum stress-induced apoptosis Food Chem Toxicol. (2017) 103:279–88. doi: 10.1016/j.fct.2017.03.025
4. Wang R, Reddy PH. Role of glutamate and NMDA receptors in Alzheimer's Disease. J Alzheimers Dis. (2017) 57:1041–8. doi: 10.3233/JAD-160763
5. Orhan N, Aslan M, Orhan DD, Ergun F, Yesilada E. In-vivo assessment of antidiabetic and antioxidant activities of grapevine leaves (Vitis vinifera) in diabetic rats. J Ethnopharmacol. (2006) 108:280–6. doi: 10.1016/j.jep.2006.05.010
6. Kedrina-Okutan O, Novello V, Hoffmann T, Hadersdorfer J, Occhipinti A, Schwab W, et al. Constitutive polyphenols in blades and veins of grapevine (Vitis vinifera L) healthy leaves. J Agric Food Chem. (2018) 66:10977–90. doi: 10.1021/acs.jafc.8b03418
7. Prior RL. Fruits and vegetables in the prevention of cellular oxidative damage. Am J Clin Nutr. (2003) 78:570s−8s. doi: 10.1093/ajcn/78.3.570S
8. Varoni EM, Lo Faro AF, Sharifi-Rad J, Iriti M. Anticancer molecular mechanisms of resveratrol. Front Nutr. (2016) 3:8. doi: 10.3389/fnut.2016.00008
9. Ozpinar H, Ozpinar N, Karakus S. The effects of erzincan grape (Vitis vinifera spp., Cimin) and benzothiazol on a caenorhabditis elegans organism model. Pharmacogn Mag. (2017) 13:S380–4. doi: 10.4103/0973-1296.210164
10. Aouey B, Samet AM, Fetoui H, Simmonds MSJ, Bouaziz M. Anti-oxidant, anti-inflammatory, analgesic and antipyretic activities of grapevine leaf extract (Vitis vinifera) in mice and identification of its active constituents by LC-MS/MS analyses. Biomed Pharmacother. (2016) 84:1088–98. doi: 10.1016/j.biopha.2016.10.033
11. Redfern J, Kinninmonth M, Burdass D, Verran J. Using soxhlet ethanol extraction to produce and test plant material (essential oils) for their antimicrobial properties. J Microbiol Biol Educ. (2014) 15:45–6. doi: 10.1128/jmbe.v15i1.656
12. Nn A. A review on the extraction methods use in medicinal plants, principle, strength and limitation. Med Arom Plants. (2015) 4:1–6. doi: 10.4172/2167-0412.1000196
13. Do QD, Angkawijaya AE, Tran-Nguyen PL, Huynh LH, Soetaredjo FE, Ismadji S, et al. Effect of extraction solvent on total phenol content, total flavonoid content, and antioxidant activity of Limnophila aromatica. J Food Drug Anal. (2014) 22:296–302. doi: 10.1016/j.jfda.2013.11.001
14. Baharum SN, Bunawan H, Ghani MA, Mustapha WA, Noor NM. Analysis of the chemical composition of the essential oil of Polygonum minus Huds. Using two-dimensional gas chromatography-time-of-flight mass spectrometry (GC-TOF MS). Molecules. (2010) 15:7006–15. doi: 10.3390/molecules15107006
15. Duangjan C, Rangsinth P, Gu X, Wink M, Tencomnao T. Lifespan extending and oxidative stress resistance properties of a leaf extracts from anacardium occidentale l. In Caenorhabditis elegans. Oxid Med Cell Longev. (2019) 2019:9012396. doi: 10.1155/2019/9012396
16. Duangjan C, Rangsinth P, Gu X, Zhang S, Wink M, Tencomnao T. Data on the effects of glochidion zeylanicum leaf extracts in caenorhabditis elegans. Data Brief. (2019) 26:104461. doi: 10.1016/j.dib.2019.104461
17. Duangjan C, Rangsinth P, Gu X, Zhang S, Wink M, Tencomnao T. Glochidion zeylanicum leaf extracts exhibit lifespan extending and oxidative stress resistance properties in caenorhabditis elegans via DAF-16/FoxO and SKN-1/Nrf-2 signaling pathways. Phytomedicine. (2019) 64:153061. doi: 10.1016/j.phymed.2019.153061
18. Rangsinth P, Prasansuklab A, Duangjan C, Gu X, Meemon K, Wink M, et al. Leaf extract of caesalpinia mimosoides enhances oxidative stress resistance and prolongs lifespan in caenorhabditis elegans. BMC Complement Altern Med. (2019) 19:164. doi: 10.1186/s12906-019-2578-5
19. Uttara B, Singh AV, Zamboni P, Mahajan RT. Oxidative stress and neurodegenerative diseases: a review of upstream and downstream antioxidant therapeutic options. Curr Neuropharmacol. (2009) 7:65–74. doi: 10.2174/157015909787602823
20. Caruana M, Cauchi R, Vassallo N. Putative role of red wine polyphenols against brain pathology in Alzheimer's and Parkinson's disease. Front Nutr. (2016) 3:31. doi: 10.3389/fnut.2016.00031
21. Whitehouse S, Chen P-L, Greenshields AL, Nightingale M, Hoskin DW, Bedard K. Resveratrol, piperine and apigenin differ in their NADPH-oxidase inhibitory and reactive oxygen species-scavenging properties. Phytomedicine. (2016) 23:1494–503. doi: 10.1016/j.phymed.2016.08.011
22. Ogunlade B, Adelakun SA, Agie JA. Nutritional supplementation of gallic acid ameliorates Alzheimer-type hippocampal neurodegeneration and cognitive impairment induced by aluminum chloride exposure in adult Wistar rats. Drug Chem Toxicol. 1–12. doi: 10.1080/01480545.2020.1754849
23. Ng KW, Cao ZJ, Chen HB, Zhao ZZ, Zhu L, Yi T. Oolong tea: a critical review of processing methods, chemical composition, health effects, and risk. Crit Rev Food Sci Nutr. (2018) 58:2957–80. doi: 10.1080/10408398.2017.1347556
24. Koch K, Weldle N, Baier S, Büchter C, Wätjen W. Hibiscus sabdariffa L. Extract prolongs lifespan and protects against amyloid-β toxicity in caenorhabditis elegans: involvement of the FoxO and Nrf2 orthologues DAF-16 and SKN-1. Eur J Nutr. (2020) 59:137–50. doi: 10.1007/s00394-019-01894-w
25. Solanki N, Salvi A, Patki G, Salim S. Modulating oxidative stress relieves stress-induced behavioral and cognitive impairments in rats. Intern J Neuropsychopharmacol. (2017) 20:550–61. doi: 10.1093/ijnp/pyx017
26. Ayuda-Durán B, González-Manzano S, Gil-Sánchez I, Moreno-Arribas MV, Bartolomé B, Sanz-Buenhombre M, et al. Antioxidant characterization and biological effects of grape pomace extracts supplementation in caenorhabditis elegans. Foods. (2019) 8:75. doi: 10.3390/foods8020075
27. Pari L, Suresh A. Effect of grape (Vitis vinifera L.) leaf extract on alcohol induced oxidative stress in rats. Food Chem Toxicol. (2008) 46:1627–34. doi: 10.1016/j.fct.2008.01.003
28. Amen Y, Sherif AE, Shawky NM, Abdelrahman RS, Wink M, Sobeh M. Grape-leaf extract attenuates alcohol-induced liver injury via interference with NF-κB signaling pathway. Biomolecules. (2020) 10:558. doi: 10.3390/biom10040558
29. Mandil R, Prakash A, Rahal A, Singh SP, Sharma D, Kumar R, et al. In vitro and in vivo effects of flubendiamide and copper on cyto-genotoxicity, oxidative stress and spleen histology of rats and its modulation by resveratrol, catechin, curcumin and alpha-tocopherol. BMC Pharmacol Toxicol. (2020) 21:29. doi: 10.1186/s40360-020-00405-6
30. Li Y, Tian Q, Li Z, Dang M, Lin Y, Hou X. Activation of Nrf2 signaling by sitagliptin and quercetin combination against beta-amyloid induced Alzheimer's disease in rats. Drug Dev Res. (2019) 80:837–45. doi: 10.1002/ddr.21567
31. Dumont M, Beal MF. Neuroprotective strategies involving ROS in Alzheimer disease. Free Radic Biol Med. (2011) 51:1014–26. doi: 10.1016/j.freeradbiomed.2010.11.026
32. Abbas S, Wink M. Epigallocatechin gallate inhibits beta amyloid oligomerization in Caenorhabditis elegans and affects the daf-2/insulin-like signaling pathway. Phytomedicine. (2010) 17:902–9. doi: 10.1016/j.phymed.2010.03.008
33. Peixoto H, Roxo M, Krstin S, Rohrig T, Richling E, Wink M. An anthocyanin-rich extract of acai (Euterpe precatoria Mart) increases stress resistance and retards aging-related markers in caenorhabditis elegans. J Agric Food Chem. (2016) 64:1283–90. doi: 10.1021/acs.jafc.5b05812
34. Prasansuklab A, Meemon K, Sobhon P, Tencomnao T. Ethanolic extract of streblus asper leaves protects against glutamate-induced toxicity in HT22 hippocampal neuronal cells and extends lifespan of caenorhabditis elegans. BMC Complement Altern Med. (2017) 17:551. doi: 10.1186/s12906-017-2050-3
35. Zhang S, Duangjan C, Tencomnao T, Liu J, Lin J, Wink M. Neuroprotective effects of oolong tea extracts against glutamate-induced toxicity in cultured neuronal cells and β-amyloid-induced toxicity in caenorhabditis elegans. Food Funct. (2020) 11:8179–92. doi: 10.1039/D0FO01072C
36. Galas S, Château MT, Pomiès P, Wang J, Menardo J, Puel JL, et al. [The diversity of aging models]. Med Sci. (2012) 28:297–304. doi: 10.1051/medsci/2012283018
37. Matsunami K. Frailty and caenorhabditis elegans as a benchtop animal model for screening drugs including natural herbs. Front Nutr. (2018) 5:111. doi: 10.3389/fnut.2018.00111
38. Abbas S, Wink M. Green tea extract induces the resistance of caenorhabditis elegans against oxidative stress. Antioxidants. (2014) 3:129–43. doi: 10.3390/antiox3010129
39. Murphy CT, Mccarroll SA, Bargmann CI, Fraser A, Kamath RS, Ahringer J, et al. Genes that act downstream of DAF-16 to influence the lifespan of caenorhabditis elegans. Nature. (2003) 424:277–83. doi: 10.1038/nature01789
40. Przybysz AJ, Choe KP, Roberts LJ, Strange K. Increased age reduces DAF-16 and SKN-1 signaling and the hormetic response of caenorhabditis elegans to the xenobiotic juglone. Mech Ageing Dev. (2009) 130:357–69. doi: 10.1016/j.mad.2009.02.004
41. Fischer N, Buchter C, Koch K, Albert S, Csuk R, Watjen W. The resveratrol derivatives trans-3,5-dimethoxy-4-fluoro-4'-hydroxystilbene and trans-2,4',5-trihydroxystilbene decrease oxidative stress and prolong lifespan in caenorhabditis elegans. J Pharm Pharmacol. (2017) 69:73–81. doi: 10.1111/jphp.12657
42. Wang H, Liu J, Li T, Liu RH. Blueberry extract promotes longevity and stress tolerance via DAF-16 in caenorhabditis elegans. Food Funct. (2018) 9:5273–82. doi: 10.1039/C8FO01680A
43. Chen W, Rezaizadehnajafi L, Wink M. Influence of resveratrol on oxidative stress resistance and life span in caenorhabditis elegans. J Pharm Pharmacol. (2013) 65:682–8. doi: 10.1111/jphp.12023
Keywords: vitis vinifera, glutamate toxicity, neuroprotection, HT22, oxidative stress resistanc, daf-16, aging, caenorhabditis elegans
Citation: Duangjan C, Rangsinth P, Zhang S, Gu X, Wink M and Tencomnao T (2021) Vitis Vinifera Leaf Extract Protects Against Glutamate-Induced Oxidative Toxicity in HT22 Hippocampal Neuronal Cells and Increases Stress Resistance Properties in Caenorhabditis Elegans. Front. Nutr. 8:634100. doi: 10.3389/fnut.2021.634100
Received: 27 November 2020; Accepted: 27 April 2021;
Published: 11 June 2021.
Edited by:
Marcello Iriti, University of Milan, ItalyReviewed by:
Neville Vassallo, University of Malta, MaltaAzlina Abdul Aziz, University of Malaya, Malaysia
Copyright © 2021 Duangjan, Rangsinth, Zhang, Gu, Wink and Tencomnao. This is an open-access article distributed under the terms of the Creative Commons Attribution License (CC BY). The use, distribution or reproduction in other forums is permitted, provided the original author(s) and the copyright owner(s) are credited and that the original publication in this journal is cited, in accordance with accepted academic practice. No use, distribution or reproduction is permitted which does not comply with these terms.
*Correspondence: Tewin Tencomnao, dGV3aW4udEBjaHVsYS5hYy50aA==; Michael Wink, V2lua0B1bmktaGVpZGVsYmVyZy5kZQ==