- 1Department of Sports and Sport Science, University of Freiburg, Freiburg, Germany
- 2Department of Sports Science, Institute for Nutrition and Sports, University of Vienna, Vienna, Austria
The purpose was to examine the effects of concurrent training (CT) combined with specific collagen peptides (SCP) intake on cardiometabolic parameters and performance indices in women. In a double-blind, placebo-controlled, randomized trial recreationally active women (n = 59) completed a 12-week CT training (3 day/week) and ingested 15 g of SCP (treatment group [TG]) or placebo (control group [CG]) on a daily basis. Running distance as a marker of endurance performance (time trial), velocity and heart rate at the lactate and anaerobic threshold (incremental running test) and body composition (bioelectrical impedance analysis [BIA]) were measured. BIA measurements included determination of fat mass (FM) and fat free mass (FFM). Additionally, muscular strength (one-repetition-maximum [1RM]) and muscular endurance (60% of 1RM) were assessed. After 12-weeks, TG had a higher increase in running distance (1,034 ± 643 m) compared to the CG (703 ± 356 m) indicated by a significant interaction effect (p < 0.05). Velocity at lactate and anaerobic threshold improved in both groups over time (p < 0.001), with no significant differences between groups. Similarly, heart rate at lactate threshold decreased over time (p < 0.001), with no time × group interaction. TG declined more in heart rate at anaerobic threshold (−8 ± 14 bpm) than the CG (−1 ± 7 bpm), which resulted in a significant interaction effect (p < 0.01). FM decreased over time in TG and CG (p < 0.001), with no group differences. On contrary, TG had a higher increase in FFM (0.8 ± 0.9 kg) compared to the CG (0.3 ± 1.0 kg) (time × group interaction: p < 0.05). Both, 1RM and muscular endurance improved over time (p < 0.001), with no significant group differences. In conclusion, supplementation of SCP in combination with CT resulted in a significant increase in endurance performance compared to the control group. This might potentially be a consequence of improved structural and cardiometabolic adaptations.
Introduction
Since 1975, obesity has nearly tripled worldwide according to World Health Organization (WHO). Physical inactivity and poor nutritional practices are considered to be major contributing factors (1). Besides lifestyle-related factors, the physiological aging process leads to an unfavorable change in body composition. Scientific evidence indicates that the age-related loss in muscle mass and function (sarcopenia) (2) and the increase in fat mass cause a majority of chronic degenerative diseases. Obesity exacerbates sarcopenia and enhances the infiltration of fat into the muscle (3).
According to the WHO global recommendations on physical activity for health in adults, resistance and endurance exercises seem to be an effective strategy to improve metabolic health (4). Typically, resistance training induces hypertrophic adaptations (5) that augment total energy expenditure, as fat free mass is a main determinant of resting metabolic rate (6). In contrast, endurance training increases muscle aerobic capacity and mitochondrial content (7) that shift fuel utilization toward fat metabolism at rest and during submaximal exercise (8–11). Comparisons between running and cycling have shown a higher rate of fat oxidation during running at the same relative intensity, which is associated with higher total energy expenditure (10). Especially in premenopausal women, these effects seem to occur through direct adaptions in the lipid metabolic pathway or indirectly through a reduction in fat mass. Investigating the underlying mechanisms of these metabolic adaptations, it is generally accepted that the use of bigger muscle groups during the eccentric muscle loading phase in running causes larger increases in the fat oxidation, whereas in cycling concentric muscle loading phase are in the foreground (12, 13). In addition, this divergence in contraction types in running compared to cycling may also provide a sufficient mechanical stimulus for collagen synthesis in the connective tissue (14).
Recent research suggests that protein intake may have synergistic effects on the maintenance and promotion of muscle growth, reducing a catabolic metabolic state and optimize the regeneration in the post-exercise phase following resistance and endurance training (15–17). Given the small body of research currently available, further long-term investigations are needed which strengthen the evidence of protein supplementation on physiological outcomes and performance parameters. Regarding the type of protein, whey, soy and casein have been investigated (18–20). Recently, collagen peptides have also received attention due to their beneficial influence on body composition during resistance training regimes (21, 22) and recovery of muscle function following strenuous physical exercise (23). However, the exact physiological mechanisms how collagen peptides affect body composition and muscular performance in humans have not been fully elucidated. In particular, the fact that collagen peptides are not a complete protein and the small concentration of some amino acids, especially leucine, has been cited as a point of criticism.
Collagen peptides are produced for nutritional administration by a specific hydrolysis of collagen. Therefore, due to the small molecule size and lack of cross-links, collagen peptides have a high biological availability, which has been demonstrated in preclinical (24, 25) and clinical studies (26, 27). In addition, collagen di- and tripeptides enter the bloodstream via the peptide transporter 1 or in the form of free amino acids (28–30). From a molecular point of view, collagen peptides are high in glycine, proline and hydroxyproline (31). Both, hydroxyproline and glycine have been measured in human blood in considerable amounts following ingestion of collagen peptides (32). Collagen-derived peptides have shown to accumulate in protein and collagen-containing tissues such as skin, cartilage or muscle (30, 33). Moreover, first clinical interventions have demonstrated, that the ingestion of specific collagen peptides has induced an upregulation in the synthesis of proteins, which are predominantly associated with the metabolism of contractile elements (22). Previously, an in vitro investigation suggested that the collagen derived peptide hydroxyprolyl-glycine seems to stimulate mTOR phosphorylation in myoblasts (34).
Most recently, an in vivo study demonstrated a significant increase in fat free mass and strength as well as an above average decrease in fat mass when supplementing specific collagen peptides (SCP) during a 12-week resistance training period (21). With respect to a significant decrease in fat mass, a direct influence on fat metabolism by SCP could be presumed as a possible cause. It has been shown that glycine, an amino acid particularly high in collagen peptides, stimulates the secretion of glucagon by increasing glucagon-like peptide-1 (GLP-1) (35). It is well established that signals such as GLP-1 activates transcription factors and increase peroxisome proliferator co-activator-1α (PGC-1α) expressions (7, 36). In addition, the interaction of PGC-1α with peroxisome proliferator-activated receptor (PPAR) stimulates fatty acid utilization which is accompanied by an increased fat metabolism (37), especially after ingestion of SCP (38, 39).
Therefore, despite the fact of e.g. low leucine concentration, this poses the question whether the supplementation of SCP might also influence cardiometabolic parameters and performance indices in endurance runners. As a previous meta-analysis has demonstrated that plain endurance training is often associated with smaller gains in lower body strength and hypertrophy than strength and concurrent training alone (40), we included resistance training features in the exercise program in order to simultaneously maintain or even improve both parameters. Long-term trials from our and other laboratories have revealed that SCP enhances strength outcomes when combined with resistance exercise (21, 22). Thus, we hypothesize that a concurrent training (CT) with endurance and resistance exercise features enhances cardiometabolic parameters, body composition and muscle strength in women when combined with a SCP supplementation. Based on these findings, the main purpose of this study was to examine the influence of CT with SCP supplementation on cardiometabolic parameters in women compared to a CT group without SCP. Changes in cardiometabolic parameters were analyzed indirectly by running distance (1), heart rate and velocity at lactate thresholds (2). Another aim was to test alterations in fat mass (3), fat free mass (4), strength (5) and muscular endurance for lower limbs (6).
Materials and Methods
Participants
Upon approval from the ethical committee of the University of Freiburg (ETK: 43/18-190309) 90 healthy women aged between 18 and 40 years with a BMI between 18.5 and 26 kg m−2 were recruited. Prior to study participation, participants read and signed informed consent. This trial was registered at the German Clinical Trials Registry (DRKS-ID: DRKS00020534).
In this investigation, exclusively recreational active female runners with a body fat percentage of > 20% were included, who regularly completed a running training twice a week for 60 min each with a minimum of running experience of 6 months. This was based on the fact that we wanted to avoid overload damage and high dropout rates. To ensure that the female runners would be amenable to training related adaptations, running activity was restricted to <120 min per week. In general, physical activity were assessed with a validated questionnaire (41). All the participants completed a comprehensive medical examination and routine blood testing for analysis of safety variables such as hemogram, creatinine, urea, aspartate transaminase, alanine aminotransferase and D-dimer.
Female runners were not eligible if they had any contraindications with regard to physical activity according to the American College of Sports Medicine (ACSM) guidelines such as cardiovascular, metabolic or renal diseases (42). Furthermore, participants were excluded from the investigation, if they had supplemented collagen peptides or other protein supplements in the past 6 months, and had intolerance or allergy against collagen peptides and silicea. Participants were also excluded from the trial if any health problems during or after physical activity or unstable weight and eating behaviors were present.
In total, 226 women wanted to take part in the study (Figure 1). After a telephone interview (prescreening), 136 subjects were not eligible. Thus, 90 participants were randomly assigned to the study groups. Due to non-compliance with the study protocol or non-appearance at the final examination date, 31 participants were excluded from the per protocol analysis.
Study Design
The present investigation is a monocentric, prospective, placebo-controlled, double-blind, randomized trial with pre- and post-test measurements conducted at the University of Freiburg in Germany. For this purpose, a block randomization was performed using a random number generator (43). Investigators and participants were blinded until all the data had been entered and the statistical analysis had been performed.
After a preliminary screening to check the inclusion criteria, subjects were randomly assigned either into the treatment group (TG) receiving specific collagen peptides or the control group (CG) receiving silicea as placebo. Subsequently, all subjects were familiarized with testing procedures and exercise techniques by a certified sport scientist. All familiarizations were conducted in individual sessions for each test by three trials on non-consecutive days before the measurement for the study was started.
At baseline and after 12 weeks, time trial, incremental running test on a treadmill, body composition and strength testing were performed. The main outcome of this trial was the difference in time trial performance by measuring the running distance before and after the CT between the TG and the CG. As a secondary outcome, changes in heart rate and velocity at the aerobic and anaerobic threshold were measured by incremental treadmill test. In addition, the alteration in fat free mass (FFM) and fat mass was compared between the two groups. Body composition was quantified by using a bioelectric impedance analysis (BIA). Finally, one-repetition maximum testing (1RM for back squat) and muscular endurance testing (60% of 1RM for back squat) of the legs were analyzed before and after 12 weeks.
Experimental Protocol
On three non-consecutive days (e.g., Monday, Wednesday, and Friday), time trial, incremental running test and strengths measurements were conducted in a standardized order. Each test was done in the morning after a standardized 12 h overnight fast. Furthermore, 2 days before the measurements, subjects documented their diet and exercise patterns to standardize macronutrients intake and physical activity. A standardized test meal was provided 2 h before the test. Within 10 min the test meal and water had to been consumed (44, 45).
Time Trial
After the standardized test meal, subjects completed a 1-h time trial on a 400 m center track. Female runners were not provided visual or verbal feedback on performance times, heart rate and covered distance (46–48). Every 10 min they were asked for their rating of perceived exertion (RPE) on a 6–20 scoring Borg scale (49). Besides the evaluator, the covered distance and HR were continuously tracked by GPS (Polar M200, Kempele, Finland). All measurements were conducted in the morning with the aim of maintaining similar experimental conditions throughout. Temperatures ranging from 17–25°C and humidity were between 50 and 60%.
Incremental Running Test
At the beginning, subjects were instructed to determine their RPE during the performance tests. Additionally, heart rate was monitored throughout all tests using a heart rate monitor (Polar M 200®, Kempele, Finland). The incremental test on the treadmill (hp cosmos quasar®, Nussdorf-Traunstein, Germany) was used to determine the velocity at lactate threshold (VLT), velocity at individual anaerobic threshold (VIAT), heart rate at lactate threshold (HRLT), heart rate at individual anaerobic threshold (HRIAT), lactate threshold (LT) and individual anaerobic threshold (IAT). Starting with 6 km·h−1 the velocity was increased by 2 km·h−1 every 3 min until exhaustion. Blood lactate concentrations were analyzed in between stages from capillary blood and were taken from the hyperemized earlobe (50). In addition, HR and RPE were continuous recorded. Incremental running tests were conducted in an air-conditioned laboratory with temperature set at 20°C and relative humidity of 50%.
Blood Lactate Analysis
Slope of the blood lactate rise in response to exercise was used to indirectly analyze aerobic and anaerobic metabolic adaptations (51, 52). With an end-to-end plastic capillary 25 μL of capillary blood was drawn from the earlobe of each subject. Subsequently, the samples were collected in microtubes (Eppendorf Tubes®, Eppendorf, Germany) containing 50 μL of 1% sodium fluoride (NaF) for analysis of blood lactate concentration, using the enzymatic-amperometric methods via blood lactate analyzer (Biosen S-line, EKF Diagnostics, Barleben, Germany). Blood lactate concentrations were analyzed in between stages, on lactate threshold and individual anaerobic threshold. In addition, velocity and heart rate on each stage were detected. All parameters were automatically evaluated by computer software (Ergonizer 4.7.4, Freiburg, Germany). Thereby, LT reflects the first measurable increase in blood lactate concentration during physical activity (53, 54). The IAT detects the highest aerobic exercise intensity that can be maintained over time without metabolic energy from continuing net lactate production. In the present study, the IAT is determined as the velocity at a net increase in lactate concentration 1.5 mmol·l−1 above the lactate concentration at LT (55).
Body Composition
Body composition measurements were performed using a procedure, which has previously been described in detail (21). Briefly, according to the guidelines of the European Society for Clinical Nutrition and Metabolism (ESPEN) (56), fat mass (FM) and fat free mass (FFM), skeletal muscle mass (SMM), total body water (TBW), extracellular water (ECW) and intracellular water (ICW) were pre- and post-tested on preliminary screening day using a seca Medical Body Composition Analyzer 515 (seca© mBCA 515, Hamburg, Germany). Standardized BIA assessments were performed after a 12-h overnight fast and each measurements were performed in the morning for the same time of day. All requirements for an accurate BIA utilization in clinical practice were stringently followed in order to reduce biological and technical error in this study (56, 57). Therefore, participants were instructed to abstain from exercising (48 h), consuming alcohol (48 h) and caffeine (12 h). Metal and accessories were removed and subjects wore tight fitting clothing. In addition, each individual was asked to void its bladder prior to testing. Before body composition analyses were conducted in a standing position with bare feet placed properly on the contact electrodes, body height was obtained with the Stadiometer (seca© 274, Hamburg, Germany) to the nearest 1 mm.
Strength Testing
On the basis of the strength test protocol by Rana et al. (58) each subject was pre- and post-tested in back squats for 1RM and muscular endurance. The procedures utilized for each subject and test, were identical.
In preparation for the measurements, subjects completed a standardized 5-min submaximal warm-up on a cycle ergometer. Subsequently, a specific warmup for back squats was performed using a set of 8–10 repetitions (40–60% of 1RM), a set of 2–3 repetitions (75% of 1RM), and a set of 1 repetition (90% of 1RM). Final 1RM was determined as the maximum load lifted through full range of motion and was detected in less than five 1RM attempts. Following each successful lift, the load was increased by 5–10% until the subject failed to lift the load with a proper technique through the entire range of motion. Single sets separated by a 3–5 min resting period to insure adequate recovery of these sets. Moreover, the execution speed was fixed at 1.5 s for the eccentric phase and 1.5 s for the concentric phase via metronome and verbal counting by the investigator. No isometric phase was allowed during the testing.
Following assessment of 1RM, the subjects given 10 min of rest. Afterwards, the resistance was set at 60% of the subject's 1RM and they performed as many repetitions as possible until fatigue, which was quantified by the rating of perceived exertion. The muscular endurance test was conducted in an identical manner as the 1RM test. As a result, the number of repetitions performed served as the measure for muscular endurance.
Test Meal
Endurance performance may be influenced by the previous meal. Therefore, subjects refrained from strenuous exercise, caffeine and alcohol consumption in the 24-h period before each experimental trial. In addition, subjects were restricted from food or fluid intake 12 h prior testing (45).
Two hours before the onset of each measurement subjects consumed a standardized test meal in the laboratory. The test meal contained 1 g carbohydrate per kg body weight and was similar in macronutrient content. It consists of wholegrain oats and semi skimmed milk. To ensure that each subject consumed a standardized volume of 650 ml fluid within 10 min, a specific amount of water and milk with each test meal was prepared (44).
Test Product Supplementation
The test product used in this study (SCP; Gelita AG, Eberbach, Germany) is composed of specific collagen peptides with a high safety (GRAS status). During the 12-weeks intervention subjects daily supplemented either 15 g of SCP or 15 g of silicea as a placebo (silicon dioxide). Two hours before each training session, both groups ingested 7.5 g dissolved in 250 ml water. Immediately after each exercise session another 7.5 g were consumed. Similarly, on days without training subjects were also instructed to supplement doses in a split fashion at the same time as on training days. The timing of the pre and post exercise beverages were corresponded to the recommendation of Iwai et al. (26), with the aim of ensuring a high amount of collagen peptides in human blood before and after each training session. On days without training, subjects were instructed to consume the drinks at the same time as on the day before. All of the subjects were blinded to which supplementation was ingested during the investigation. Supplementations had similar color and taste.
Dietary Intake and Energy Expenditure
Before and after the intervention subjects recorded their dietary intake for two weekdays and one weekend day on three consecutive days to gain perspective on eating habits. In doing so, all subjects were given detailed instructions from a nutritionist on how to quantify food proportions and thoroughness of meal description needed for precise computation. Subjects were instructed not to add the test product supplementation to their food logs. Energy and macronutrient intakes were examined using Nutriguide 4.6 (Nutri Science GmbH, Hausach, Germany).On the basis of this information, total energy intake, protein, amino acids, carbohydrate, fat and fluid intake were calculated.
Besides dietary intake, subjects documented their physical activity before and after the investigation to gain perspective on physical activity habits. Physical activity was measured by the self-reported Freiburg Questionnaire of Physical Activity (FFKA) (41). The FFKA contained 12 standardized questions, including basic, leisure, and sports-type activities. Additionally, subjects were also instructed not to add the strength and endurance training to the FFKA, which were part of the intervention. According to the Compendium of Physical Activities, we determined the energy expenditure. This Compendium provides a five-digit coding scheme linking categories and types of physical activity with their respective metabolic equivalent of task (MET) intensity values. By multiplying the bodyweight in kg by the MET intensities values and duration of physical activity, we estimated the kcal of energy expenditure to the subject's individual bodyweight per day (59, 60).
Based on the data of the pre-examination, subjects were encouraged to maintain their dietary and physical activity habits during the study.
Training Protocol
Over a period of 12 weeks, subjects performed a supervised concurrent resistance and endurance training, three times a week with at least 1 day of rest between two sessions.
Each training session started with the resistance exercises based on Klika and Jordan (61), performing 3 sets squats, lunges and one legged heel rises using the subject's bodyweight as resistance. During the training period, number of repetitions gradually increased: week 1–4: 20 repetitions, week 5–8: 25 repetitions, and week 9–12: 30 repetitions. Loading strategies, with respect to sets and repetition range, were in accordance with previous recommendations for increasing muscular endurance (5, 62). Subjects were encouraged to maintain an execution speed of 2 s, which was equally split between concentric and eccentric phase. Between the sets a 30 s rest interval was provided.
After the resistance training 1 h endurance training was performed on a 400 m running track. Individual anaerobic threshold was determined during the training period, intensities progressively increased from week 1–4: 80% of VIAT, week 5–8: 85% of VIAT and week 9–12: 90% of VIAT (63, 64).
All training sessions were conducted at the University of Freiburg and were supervised by highly experienced exercise instructors.
Statistical Analysis
Per protocol analyses were utilized using IBM SPSS Statistics 25 (IBM, Armonk, NY, USA). Data are presented as mean ± standard deviation (SD) in tables and figures. The level of significance was set to α < 0.05 for all performed two-sided tests. Since the variable data of all groups showed normal distribution according to the results of Kolmogorov-Smirnoff test, the homogeneity of the baseline values between study groups was checked via independent t-tests. To detect changes over time and respective differences between the groups, a repeated-measures ANOVA (rmANOVA) with factors time (pre, post) × group (TG, CG) was performed to test for interaction effects. In the case of significant interaction effects from the rmANOVA, Bonferroni corrected Student's t-tests were calculated for any pre to post differences. In order to describe, if effects have a relevant magnitude, Cohen's d was conducted between the groups. If both groups improved significantly, Cohen's d was performed within the groups.
Results
Subject Characteristics
In total, 59 subjects completed the investigation and were included in the per protocol analysis. Neither the TG (n = 28) nor the CG (n = 31) differed statistically significantly in age, height, weight, body mass index (BMI), FM, FFM or hydration status (Table 1). Furthermore, baseline data of the outcomes did not significantly differ between the groups except for the HR at IAT (Table 3). All 31 dropouts failed to comply with the study design, mainly missing too many (<80%) training days or could not perform their training protocol sufficiently due to illness, injury or other reasons. No dropout was related to side effects of the supplemented SCP or placebo and no pathological findings were observed in the routine blood test.
Dietary Intake and Energy Expenditure
As illustrated in Table 2, the groups did not show significant differences in energy intake, macronutrients, amino acids, fluid intake and energy expenditure at baseline (p > 0.05). After the intervention, no significant main effect of time (p > 0.05) or time × group interaction (p > 0.05) was determined for these lifestyle-related parameters.
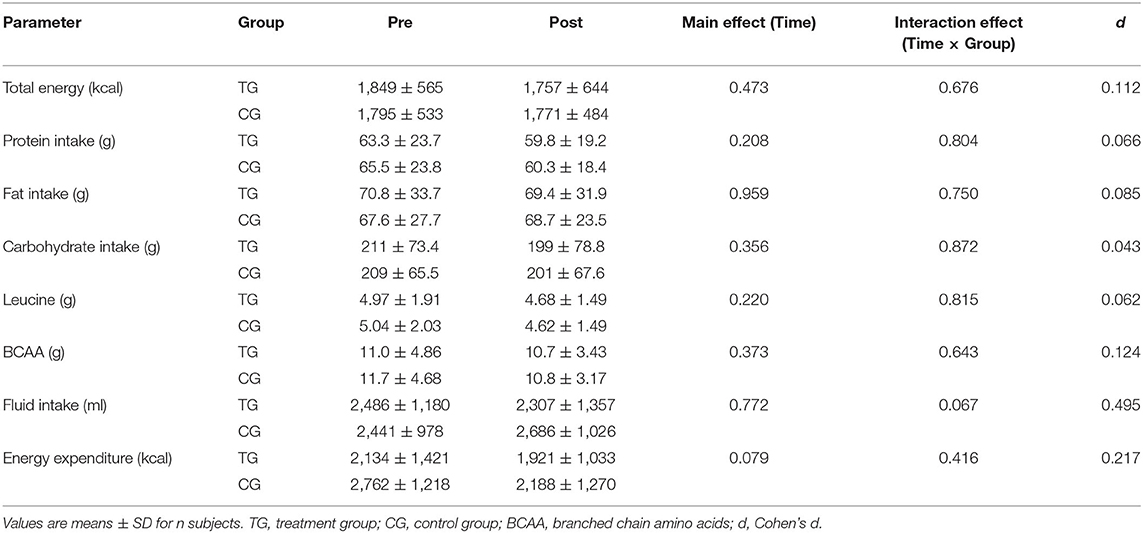
Table 2. Changes in energy intake, macronutrients, amino acids, fluid intake, and energy expenditure before (Pre) and after (Post) supplementation with TG (n = 28) or CG (n = 31).
Time Trial
As shown in Figure 2, the TG had a greater increase in running distance (1,034 ± 643 m; p < 0.001; d = 0.860) compared to the CG (703 ± 356 m; p < 0.001; d = 0.507), which resulted in a statistically significant main effect of time (p < 0.001) and time × group interaction (p < 0.05; d = 0.658).
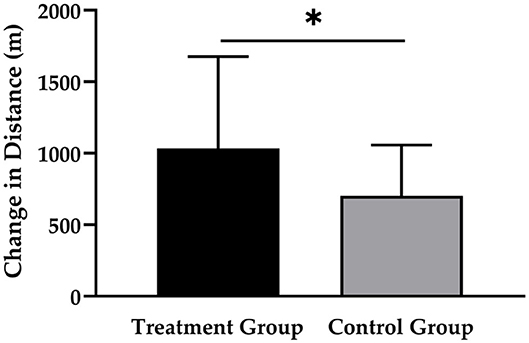
Figure 2. Changes in running distance after 12 weeks of intervention in treatment group (n = 28) and control group (n = 31). Values are means ± SD for n subjects. *Significantly different (p < 0.05) by repeated-measures ANOVA (time × group interaction).
Incremental Running Test
An elevation in the velocity at LT was observed in both TG (0.71 ± 0.70 km·h−1) and CG (0.54 ± 0.60 km·h−1), with a significant main effect of time (p < 0.001), but with no significant time × group effect (p > 0.05; d = 0.198). Similarly, velocity at IAT increased by 0.86 ± 0.61 km·h−1 in the TG and by 0.77 ± 0.54 km·h−1 in the CG with a significant main effect of time (<0.001), but with no significant time × group effect (p > 0.05; d = 0.155) (Table 3).
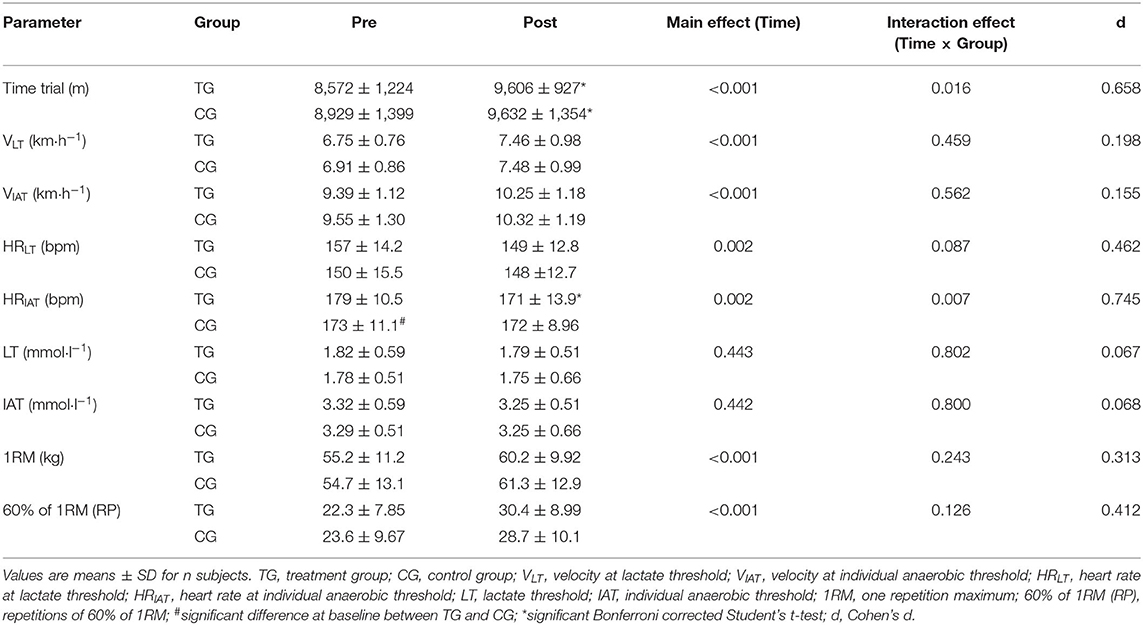
Table 3. Changes in time trial, lactate thresholds and muscle strength before (Pre) and after (Post) supplementation with TG (n = 28) or CG (n = 31).
After 12-weeks of CT, the administration of SCP was accompanied by a reduction in HR at LT by −8 ± 11 bpm and a decrease by −2 ± 12 bpm of HR in the CG (Figure 3). These results revealed a statically relevant time effect (p < 0.01) with no time × group interaction (p > 0.05; d = 0.462). Furthermore, the TG had a higher decrease in HR at IAT (−8 ± 14 bpm; p < 0.01) in contrast to the CG (−1 ± 7 bpm; p > 0.05), which resulted in a significant time (p < 0.01) and time × group (p < 0.01; d = 0.745) effect.
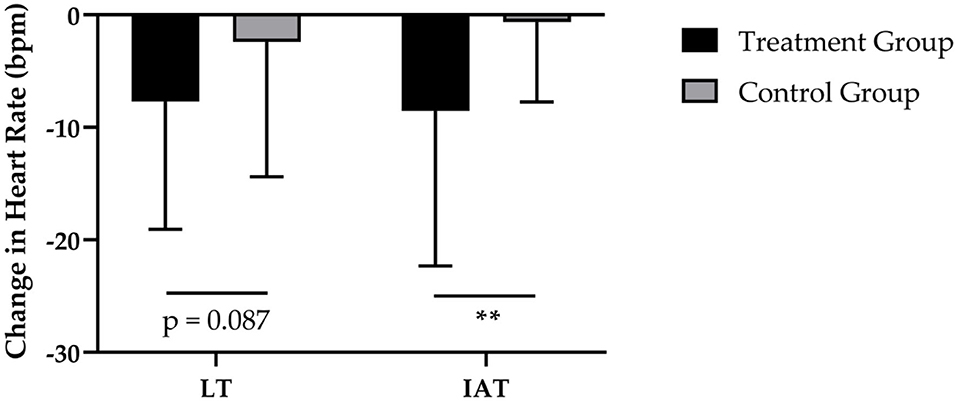
Figure 3. Changes in HR at LT and HR at IAT after 12 weeks of intervention in treatment group (n = 28) and control group (n = 31). Values are means ± SD for n subjects. **Significantly different (p < 0.01) by repeated-measures ANOVA (time × group interaction).
A marginal decrease in LT was shown in both TG (0.03 ± 0.54 mmol·l−1) and CG (0.03 ± 0.59 mmol·l−1), with no significant time (p > 0.05) or time × group (p > 0.05; d = 0.067) effect. To the same extant, IAT reduced by 0.07 ± 0.54 mmol·l−1) in TG and by (0.04 ± 0.59 mmol·l−1) in the CG with neither a significant time (p > 0.05) nor time × group (p > 0.05; d = 0.068) effect (Table 3).
Body Composition
After 12 weeks of CT, the supplementation of SCP was accompanied by a reduction in FM by −1.0 ± 1.5 kg and a decrease by −0.6 ± 1.7 kg in the CG (Figure 4). Evaluation of changes in FM revealed a significant main effect of time (p < 0.001), with no significant time × group effect (p > 0.05; d = 0.233).
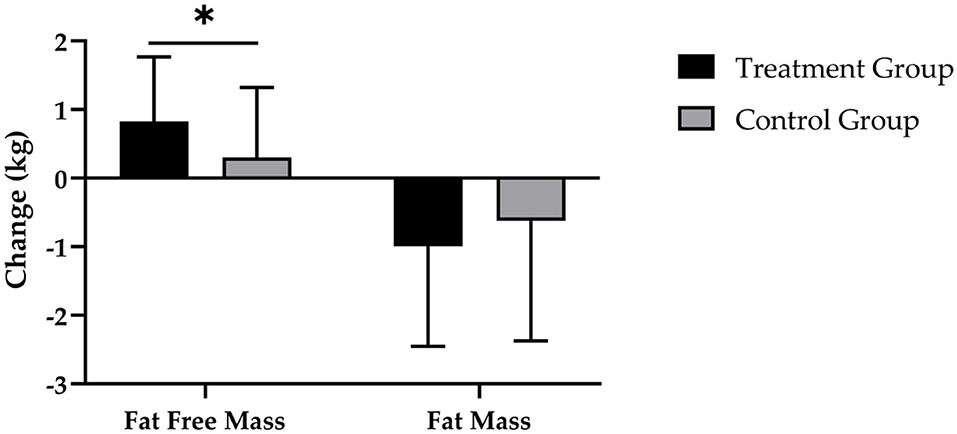
Figure 4. Changes in fat free mass and fat mass after 12 weeks of intervention in treatment group (n = 28) and control group (n = 31). Values are means ± SD for n subjects. *Significantly different (p < 0.05) by repeated-measures ANOVA (time × group interaction).
Figure 4 demonstrates that the TG had a statistically significantly higher increase in FFM (0.8 ± 0.9 kg; p < 0.001) compared with the CG (0.3 ± 1.0 kg; p > 0.05), which resulted in a statistically significant main effect of time (p < 0.001) and time × group interaction (p < 0.05; d = 0.543).
In addition, subjects in the TG exhibited a higher increase in SMM (0.6 ± 0.7 kg) in contrast to the CG (0.3 ± 0.7 kg). These results revealed a statistically relevant time effect (p < 0.001) and a strong trend toward a time × group interaction effect (p = 0.052; d = 0.526) (Table 4).
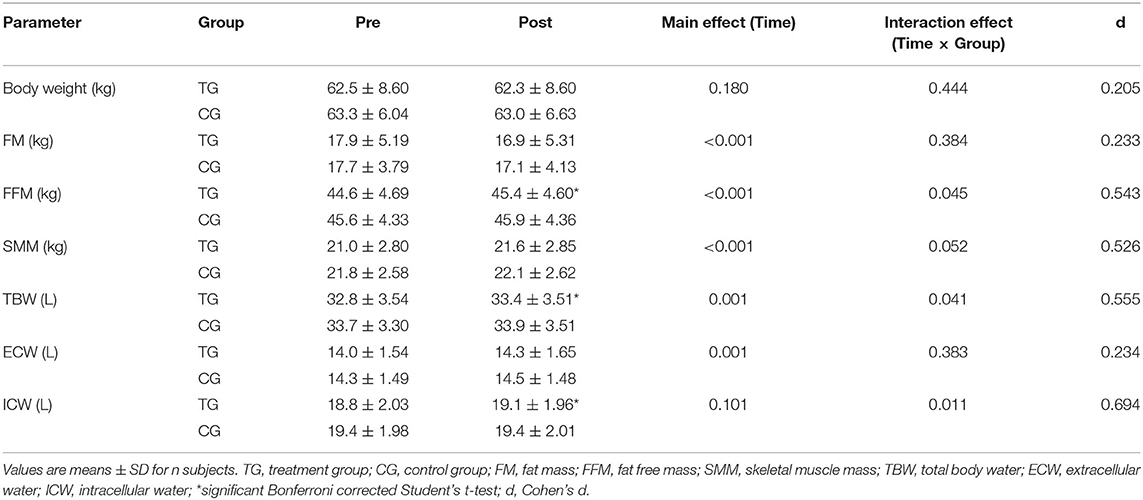
Table 4. Changes in body composition before (Pre) and after (Post) supplementation with TG (n = 28) or CG (n = 31).
Moreover, TBW increased by 0.6 ± 0.8 L in the TG (Table 4). In the CG a rise by 0.2 ± 0.8 L was detected. There was a significant main effect of time (p < 0.001) and time × group effect (p < 0.05; d = 0.555).
An increase in the ECW was observed in both the TG (0.3 ± 0.6 L) and CG (0.2 ± 0.5 L), with a significant main effect of time (p = 0.001), but with no significant time × group effect (p > 0.05; d = 0.234) (Table 4).
In contrast, the 3-month administration of SCP was accompanied by an increase of ICW by 0.3 ± 0.5 L (p < 0.05), while no changes were revealed in the CG (Table 4). Calculation of a repeated measures ANOVA revealed no statistically significant main effect of time (p > 0.05), but a significant time × group interaction (p < 0.05; d = 0.694).
Strength Testing
As shown in Table 3, 1RM in leg strength was improved in both TG (5.0 ± 5.2) and CG (6.6 ± 6.3 kg). The results of the rmANOVA showed a significant time effect (p < 0.01), whereas no significant time × group effect (p > 0.05; d = 0.313) was identified.
Finally, muscle endurance in the lower limbs enhanced by 8.1 ± 7.4 rpm in the TG (Table 3). In the training group without SCP administration an increase by 5.1 ± 7.5 rpm was observed. There was a significant main effect of time (p < 0.01), with no significant time × group effect (p > 0.05; d = 0.412).
Discussion
To the best of our knowledge, this study was the first to examine the influence of collagen peptide supplementation following a 12 week concurrent training on endurance performance, cardiometabolic parameters, body composition and muscle endurance in recreationally active women. The major findings were that supplementation with SCP further improved the positive effects of CT regarding time trial performance, HR at the IAT and the increase in FFM in the subjects investigated.
In the present study, the SCP group showed an improved endurance performance in the time trial which could be explained by an improved aerobic metabolism. Moreover, although not statistically different, a more pronounced improvement in velocity at both the LT and the IAT in favor of the SCP group could be observed. The IAT represents a reliable parameter to assess endurance performance capacity (64). Since, Achten et al. (52) have demonstrated that the onset of plasma lactate accumulation occurs at the same exercise intensity as for maximal fat oxidation both thresholds indicate a superior rightward shift fuel utilization toward fats. In addition, the SCP group showed a considerably lower heart rate at the aerobic and anaerobic threshold indicating an improved cardio-circulatory adaptation.
The influence of protein intake following CT on cardiometabolic parameters is largely unknown. Although it has been previously shown by one study that protein supplementation further stimulates fat metabolism during exercise thereby improving endurance performance, the evidence is rather limited (65). Early research data indicated that a 2-week co-ingestion of proteins and carbohydrates significantly elevated PGC-1α expression following an exercise bout compared to carbohydrate feeding alone (66). PGC-1α signaling is involved in mitochondrial biogenesis and improved fat metabolism (67). Evidence from an acute study showed that PGC1α expression was twofold higher with CT compared to a single-mode of endurance training (68). Moreover, a review indicates an up-regulation in AMPK-PGC1α in response to RT and ET, especially when protein is supplemented (69).
Another downstream signaling of PGC-1α involved in fatty acid metabolism is peroxisome proliferator-activated receptor (PPAR). PPARs are a nuclear receptors family consisting of the transcription factors PPARα, PPARδ, and PPARγ and regulate fat metabolism in the body. Acute and chronic exercise leads to an increase of PPARα in muscle cells and PPARγ in liver cells which has been associated with an improved lipid metabolism (70). It has been shown that feeding collagen peptides to mice over a period of 8 weeks resulted in a significant increase in PPARα expression and fatty acid metabolism compared to a control group (39). Similar findings were observed in a 10-week intervention in which PPAR signaling pathway and fatty acid metabolism significantly increased. The authors concluded that collagen peptide ingestion upregulates PPARα expression which leads to an increased lipogenesis, fatty acid beta-oxidation and fatty acid transport (38).Therefore, it might be speculated that the AMP-activated protein kinase (AMPK)-PGC-1α signaling cascades including PPARs, may explain a potential mechanism of increased fat metabolism by supplementing SCP in the present investigation.
In general, the key metabolic adaptations to prolonged endurance training shifts fuel utilization toward fat metabolism (8–11) which is accompanied by an increased mitochondrial content and thus an enhanced aerobic capacity (7). For the SCP used in the current trial the positive impact on mitochondrial content was investigated in a preclinical trial with a rat model. A statistically significantly increased mitochondrial density (in fine-needle biopsies of the Quadriceps femoris) of ca. 57% were determined by transmission electron microscopy after 4 weeks of an oral SCP dosage which was equivalent to a daily intake of 15 g in humans. In contrast, in rats fed with the tap water control no changes in muscle mitochondria content was determined (71). However, the exact mechanisms on how SCP might directly stimulate human fat metabolism remain unclear and need to be further investigated.
It has to be noted that the augmentation in endurance running performance is not solely attributed to aerobic and anaerobic capacity but also to neuromuscular motor competence and running economy (72–74). A systematic review from controlled clinical trials in men and women has shown that also resistance training improved time trial performance, running economy and running velocities in endurance athletes (74). Interestingly, although long-term investigations are scarce, it is generally believed that protein supplementation may further improve endurance performance following a CT (15, 75).
In addition, a previous study showed that CT training led to improved muscle strength, running economy and running velocity with no significant effects on VO2 kinetics pattern in male runners (76). Most of the CT studies (77) use a hypertrophy-oriented full body training with 70–85% of 1RM and 8–12 repetition according to the ACSM guidelines (5). Although body weight resistance training can be an efficient strategy to improve metabolic health, it may be inferior to creating absolute strength compared to commonly used strength trainings regimes (78). However, this trainings method was used to increase maximal aerobic capacity and decreasing body fat (61). Interestingly, a current meta-analysis revealed that hypertrophy adaptations between low- vs. high-load resistance training can be equally achieved across a spectrum of loading ranges (79), which might also be responsible for improvements in running economy and velocity due to an improved muscle strength and endurance in the current investigation. Moreover, body weight resistance training was also used due to its practical application, since the use of equipment or a facility is not required (61).
The current trial is in line with a previous investigation, showing that running CT enhances fat free mass and muscle strength (80). In a recent meta-analysis, Wilson et al. (40) demonstrated superior increases in muscle hypertrophy as well as higher reductions in fat mass following CT compared to endurance training alone. The increase in FFM is attributable to both, a rise in skeletal muscle mass and total body water on a clinically relevant level, as indicated by the calculated effect sizes. Bosy-Westphal et al. (81) have confirmed that the phase-sensitive 8-electrode medical bioelectrical impedance device, which was used in the present investigation, is a highly accurate device for quantifying whole-body skeletal muscle mass (81). Although the influence of different training regimes on FM and FFM has been extensively explored, to the best of our knowledge, no investigation has studied the effects of long-term CT on body water distribution. Previous investigations indicate that maintaining or increasing ICW with a simultaneously elevated FFM and TBW are related to performance, irrespective of sex and type of sports (82–84). However, due to the different populations, investigation or intervention periods and measurement methodologies these findings should be taken with caution when extrapolating it to our results.
It was not the intention of the present study to generate evidence for an increase in muscle mass as this was not within the scope and the primary outcome variables of the investigation. All necessary precautions were taken to assure the outmost pre-analytical and analytical precision before and during the BIA analysis. However, further experiments are needed to investigate the interrelation between changes in FFM, SMM and TBW following SCP supplementation and exercise interventions.
In addition, as a trend, superior repetitions in the muscular endurance test were observed in the TG (+36.3%) compared to the CG (+21.6%). With regard to the interference effect, these results are in line with an earlier meta-analysis regarding simultaneous strength and running endurance training (40). There is an ongoing debate whether the anabolic process can be further enhanced by protein supplementation following a CT (15, 75, 85). Although the data situation is not clear, the interference effect is generally explained by a changed balance between muscle protein synthesis and muscle protein degradation, which are induced by antagonistic signaling pathways of anabolic and endurance stimuli on a molecular level (86).
Recently, experimental results reported that protein administration after a single bout of CT increases myofibrillar protein synthesis and attenuate markers of muscle catabolism in men (87). On the other hand, Ormsbee et al. (88) showed no additional increases in muscle mass and strength in the group with protein supplementation after 6-month of CT in sedentary women. Although there is currently limited knowledge on the signaling effects of collagen peptides on muscle anabolism, the activation of mTOR signaling pathways through collagen peptide intake has been shown in vitro and in a clinical trial (22, 34). A previous investigation in women confirmed the present data by showing a significantly higher increase in FFM following SCP supplementation in combination with resistance training compared to placebo (21). It is important to note, however, that investigations by Ormsbee et al. (88) and Shamim et al. (85) differ in amount, quality and timing of protein. In addition, the initially loading protocols, the recorded parameters and the protocols used to verify endurance and strength performances differ from each other. Therefore, a direct comparison of the effects on these parameters is not possible.
Interestingly, a recent review and meta-analysis reports that an enhanced biochemical properties of the muscle-tendon system lead to improved running economy (72). This might be explained by an improved energy storage capacity and force potential of the muscle with increased tendon stiffness (89, 90). Since human tendons comprise 60–85% of collagen (91), the supplementation of collagen peptides might represent a promising strategy for improving tendo-muscular properties. First evidence from a controlled double-blind trial in men and women with chronic tendinopathy has shown that the administration of collagen peptides in combination with calf muscle resistance training was associated with significantly improved function of the Achilles tendon (92). An improved running economy might also contribute to the marked reduction in heart rate at both, the aerobic as well as the anaerobic threshold. Therefore, although it is still speculative, the differences in running velocity between the groups could also be explained by an improved structural and mechanical property of the muscle-tendon system in the TG group.
With respect to the interpretation of our results, there are some limitations in the present investigation that should be mentioned. Firstly, the study examined cardiometabolic parameter by using heart rate and blood lactate at the LT and IAT as surrogate markers and not muscle biopsies (93) and tracer approaches (94) as gold standards. However, it has been shown that measuring these surrogate markers is an effective way to indirectly assess changes in aerobic and anaerobic metabolic processes (51, 52).
Secondly, there is an ongoing discussion about gender-specific differences in the sensitivity of BIA measurements regarding exercise-training induced changes in body composition of study participants (95, 96). Nevertheless, it has been demonstrated in various experimental settings, that the multifrequency bioelectrical impedance assessment used in this study is a valid and reproducible method to estimate body composition in healthy adults (57, 81, 97).
It must be also emphasized that these results are valid for the investigated specific collagen peptides only and cannot be generalized to other collagen peptides.
Conclusion
In conclusion, our results indicated that supplementation of SCP in combination with CT resulted in a statistically significant increase in the time-trial performance compared to the control group. This might be a potential consequence of improved cardiometabolic parameters, mainly aerobic metabolism, as measured in the incremental treadmill test. Moreover, HR at the IAT was improved after SCP intake, indicating an improved biomechanical motor competence. Both, the metabolic and structural adaptations could have led to an enhanced running velocity and improved running economy. In this context, the additional intake of SCP after CT appears to be beneficial to further improve the effect of endurance training on fat free mass and muscular endurance.
Data Availability Statement
The datasets generated for this study are available on request to the corresponding author.
Ethics Statement
The studies involving human participants were reviewed and approved by the ethics committee of the University of Freiburg (43/18) and was in accordance with the latest revision of the Declaration of Helsinki. The participants provided their written informed consent to participate in this study.
Author Contributions
PJ, JK, CC, AG, and DK designed the study. PJ and DK were responsible for data acquisition. Analysis was performed by PJ, JK, and DK. All authors read and approved the final version of the manuscript. All authors contributed to the article and approved the submitted version.
Funding
This research was in part funded by GELITA GmbH, Eberbach Germany. The specific collagen peptides will be named PeptENDURE®.
Conflict of Interest
The authors declare that the research was conducted in the absence of any commercial or financial relationships that could be constructed as a potential conflict of interest.
Acknowledgments
The authors thank all of the participants and the staff of Nutrition and Training Laboratory (NuTraLab) of Freiburg who supported us by the examination and the implementation of the resistance and endurance training.
References
1. World Health Organization. Obesity and Overweight. (2020). Available online at: https://www.who.int/en/news-room/fact-sheets/detail/obesity-and-overweight (accessed January 16, 2020)
2. Cruz-Jentoft AJ, Bahat G, Bauer J, Boirie Y, Bruyere O, Cederholm T, et al. Sarcopenia: revised European consensus on definition and diagnosis. Age Ageing. (2019) 48:16–31. doi: 10.1093/ageing/afz046
3. Zhang X, Xie X, Dou Q, Liu C, Zhang W, Yang Y, et al. Association of sarcopenic obesity with the risk of all-cause mortality among adults over a broad range of different settings: a updated meta-analysis. BMC Geriatr. (2019) 19:183. doi: 10.1186/s12877-019-1195-y
4. WHO. Global Recommendations on Physical Activity for Health. WHO Guidelines Approved by the Guidelines Review Committee. Geneva: WHO Press (2010).
5. ACSM. American College of Sports Medicine position stand. Progression models in resistance training for healthy adults. Med Sci Sports Exerc. (2009) 41:687–708. doi: 10.1249/MSS.0b013e3181915670
6. Sparti A, DeLany JP, delaBretonne JA, Sander GE, Bray GA. Relationship between resting metabolic rate and the composition of the fat-free mass. Metabolism. (1997) 46:1225–30. doi: 10.1016/S0026-0495(97)90222-5
7. Hoppeler H. Molecular networks in skeletal muscle plasticity. J Exp Biol. (2016) 219:205–13. doi: 10.1242/jeb.128207
8. CallesEscandon J, Goran MI, OConnell M, Nair KS, Danforth E. Exercise increases fat oxidation at rest unrelated to changes in energy balance or lipolysis. Am J Physiol. (1996) 270:E1009–14. doi: 10.1152/ajpendo.1996.270.6.E1009
9. Friedlander AL, Casazza GA, Horning MA, Buddinger TF, Brooks GA. Effects of exercise intensity and training on lipid metabolism in young women. Am J Physiol. (1998) 275:E853–63. doi: 10.1152/ajpendo.1998.275.5.E853
10. Achten J, Jeukendrup AE. Optimizing fat oxidation through exercise and diet. Nutrition. (2004) 20:716–27. doi: 10.1016/j.nut.2004.04.005
11. Gonzalez JT, Stevenson EJ. New perspectives on nutritional interventions to augment lipid utilisation during exercise. Br J Nutr. (2012) 107:339–49. doi: 10.1017/S0007114511006684
12. Knechtle B, Muller G, Willmann F, Kotteck K, Eser P, Knecht H. Fat oxidation in men and women endurance athletes in running and cycling. Int J Sports Med. (2004) 25:38–44. doi: 10.1055/s-2003-45232
13. Achten J, Venables MC, Jeukendrup AE. Fat oxidation rates are higher during running compared with cycling over a wide range of intensities. Metabolism. (2003) 52:747–52. doi: 10.1016/S0026-0495(03)00068-4
14. Kjaer M, Langberg H, Heinemeier K, Bayer ML, Hansen M, Holm L, et al. From mechanical loading to collagen synthesis, structural changes and function in human tendon. Scand J Med Sci Sports. (2009) 19:500–10. doi: 10.1111/j.1600-0838.2009.00986.x
15. Perez-Schindler J, Hamilton DL, Moore DR, Baar K, Philp A. Nutritional strategies to support concurrent training. Eur J Sport Sci. (2015) 15:41–52. doi: 10.1080/17461391.2014.950345
16. Knuiman P, Hopman MTE, Verbruggen C, Mensink M. Protein and the adaptive response with endurance training: wishful thinking or a competitive edge? Front Physiol. (2018) 9:598. doi: 10.3389/fphys.2018.00598
17. Moore DR, Camera DM, Areta JL, Hawley JA. Beyond muscle hypertrophy: why dietary protein is important for endurance athletes. Appl Physiol Nutr Metab. (2014) 39:987–97. doi: 10.1139/apnm-2013-0591
18. van Vliet S, Burd NA, van Loon LJ. The skeletal muscle anabolic response to plant- versus animal-based protein consumption. J Nutr. (2015) 145:1981–91. doi: 10.3945/jn.114.204305
19. Castellanos VH, Litchford MD, Campbell WW. Modular protein supplements and their application to long-term care. Nutr Clin Pract. (2006) 21:485–504. doi: 10.1177/0115426506021005485
20. Morton RW, Murphy KT, McKellar SR, Schoenfeld BJ, Henselmans M, Helms E, et al. A systematic review, meta-analysis and meta-regression of the effect of protein supplementation on resistance training-induced gains in muscle mass and strength in healthy adults. Br J Sports Med. (2018) 52:376–84. doi: 10.1136/bjsports-2017-097608
21. Jendricke P, Centner C, Zdzieblik D, Gollhofer A, König D. Specific collagen peptides in combination with resistance training improve body composition and regional muscle strength in premenopausal women: a randomized controlled trial. Nutrients. (2019) 11:892. doi: 10.3390/nu11040892
22. Oertzen-Hagemann V, Kirmse M, Eggers B, Pfeiffer K, Marcus K, de Marées M, et al. Effects of 12 weeks of hypertrophy resistance exercise training combined with collagen peptide supplementation on the skeletal muscle proteome in recreationally active men. Nutrients. (2019) 11:1072. doi: 10.3390/nu11051072
23. Clifford T, Ventress M, Allerton DM, Stansfield S, Tang JCY, Fraser WD, et al. The effects of collagen peptides on muscle damage, inflammation and bone turnover following exercise: a randomized, controlled trial. Amino Acids. (2019) 51:691–704. doi: 10.1007/s00726-019-02706-5
24. Oesser S, Seifert J. Stimulation of type II collagen biosynthesis and secretion in bovine chondrocytes cultured with degraded collagen. Cell Tissue Res. (2003) 311:393–9. doi: 10.1007/s00441-003-0702-8
25. Walrand S, Chiotelli E, Noirt F, Mwewa S, Lassel T. Consumption of a functional fermented milk containing collagen hydrolysate improves the concentration of collagen-specific amino acids in plasma. J Agric Food Chem. (2008) 56:7790–5. doi: 10.1021/jf800691f
26. Iwai K, Hasegawa T, Taguchi Y, Morimatsu F, Sato K, Nakamura Y, et al. Identification of food-derived collagen peptides in human blood after oral ingestion of gelatin hydrolysates. J Agric Food Chem. (2005) 53:6531–6. doi: 10.1021/jf050206p
27. McAlindon TE, Nuite M, Krishnan N, Ruthazer R, Price LL, Burstein D, et al. Change in knee osteoarthritis cartilage detected by delayed gadolinium enhanced magnetic resonance imaging following treatment with collagen hydrolysate: a pilot randomized controlled trial. Osteoarthritis Cartilage. (2011) 19:399–405. doi: 10.1016/j.joca.2011.01.001
28. Aito-Inoue M, Lackeyram D, Fan MZ, Sato K, Mine Y. Transport of a tripeptide, Gly-Pro-Hyp, across the porcine intestinal brush-border membrane. J Pept Sci. (2007) 13:468–74. doi: 10.1002/psc.870
29. Ohara H, Matsumoto H, Ito K, Iwai K, Sato K. Comparison of quantity and structures of hydroxyproline-containing peptides in human blood after oral ingestion of gelatin hydrolysates from different sources. J Agric Food Chem. (2007) 55:1532–5. doi: 10.1021/jf062834s
30. Watanabe-Kamiyama M, Shimizu M, Kamiyama S, Taguchi Y, Sone H, Morimatsu F, et al. Absorption and effectiveness of orally administered low molecular weight collagen hydrolysate in rats. J Agric Food Chem. (2010) 58:835–41. doi: 10.1021/jf9031487
31. Liu D, Nikoo M, Boran G, Zhou P, Regenstein JM. Collagen and gelatin. Annu Rev Food Sci Technol. (2015) 6:527–57. doi: 10.1146/annurev-food-031414-111800
32. Shigemura Y, Akaba S, Kawashima E, Park EY, Nakamura Y, Sato K. Identification of a novel food-derived collagen peptide, hydroxyprolyl-glycine, in human peripheral blood by pre-column derivatisation with phenyl isothiocyanate. Food Chem. (2011) 129:1019–24. doi: 10.1016/j.foodchem.2011.05.066
33. Oesser S, Adam M, Babel W, Seifert J. Oral administration of (14)C labeled gelatin hydrolysate leads to an accumulation of radioactivity in cartilage of mice (C57/BL). J Nutr. (1999) 129:1891–5. doi: 10.1093/jn/129.10.1891
34. Kitakaze T, Sakamoto T, Kitano T, Inoue N, Sugihara F, Harada N, et al. The collagen derived dipeptide hydroxyprolyl-glycine promotes C2C12 myoblast differentiation and myotube hypertrophy. Biochem Biophys Res Commun. (2016) 478:1292–7. doi: 10.1016/j.bbrc.2016.08.114
35. Gameiro A, Reimann F, Habib AM, O'Malley D, Williams L, Simpson AK, et al. The neurotransmitters glycine and GABA stimulate glucagon-like peptide-1 release from the GLUTag cell line. J Physiol-London. (2005) 569:761–72. doi: 10.1113/jphysiol.2005.098962
36. Puigserver P. Tissue-specific regulation of metabolic pathways through the transcriptional coactivator PGC1-alpha. Int J Obes. (2005) 29:S5–9. doi: 10.1038/sj.ijo.0802905
37. Gerhart-Hines Z, Rodgers JT, Bare O, Kim CLSH, Kim SH, Mostoslavsky R, et al. Metabolic control of muscle mitochondrial function and fatty acid oxidation through SIRT1/PGC-1 alpha. EMBO J. (2007) 26:1913–23. doi: 10.1038/sj.emboj.7601633
38. Tometsuka C, Koyama Y, Ishijima T, Toyoda T, Teranishi M, Takehana K, et al. Collagen peptide ingestion alters lipid metabolism-related gene expression and the unfolded protein response in mouse liver. Br J Nutr. (2017) 26:1913–23. doi: 10.1017/S0007114516004384
39. Woo M, Song YO, Kang K-H, Noh JS. Anti-obesity effects of collagen peptide derived from skate (raja kenojei) skin through regulation of lipid metabolism. Mar Drugs. (2018) 16:1–12. doi: 10.3390/md16090306
40. Wilson JM, Marin PJ, Rhea MR, Wilson SMC, Loenneke JP, Anderson JC. Concurrent training: a meta-analysis examining interference of aerobic and resistance exercises. J Strength Cond Res. (2012) 26:2293–307. doi: 10.1519/JSC.0b013e31823a3e2d
41. Frey I, Berg A, Grathwohl D, Keul J. Freiburg questionnaire of physical activity-development, evaluation and application. Soz Praventivmed. (1999) 44:55–64. doi: 10.1007/BF01667127
42. Riebe D, Franklin BA, Thompson PD, Garber CE, Whitfield GP, Magal M, et al. Updating ACSM's recommendations for exercise preparticipation health screening. Med Sci Sports Exerc. (2015) 47:2473–9. doi: 10.1249/MSS.0000000000000664
43. Urbaniak GC, Plous S. Research Randomizer (Version 4.0). (2011). Available online at: http://www.randomizer.org/ (accessed April 16, 2020).
44. Moore LJS, Midgley AW, Thomas G, Thurlow S, McNaughton LR. The effects of low- and high-glycemic index meals on time trial performance. Int J Sports Physiol Perform. (2009) 4:331–44. doi: 10.1123/ijspp.4.3.331
45. Thomas DT, Erdman KA, Burke LM. Position of the academy of nutrition and dietetics, dietitians of Canada, and the American college of sports medicine: nutrition and athletic perform. J Acad Nutr Diet. (2016) 116:501–28. doi: 10.1016/j.jand.2015.12.006
46. Currell K, Jeukendrup AE. Validity, reliability and sensitivity of measures of sporting performance. Sports Med. (2008) 38:297–316. doi: 10.2165/00007256-200838040-00003
47. Laursen PB, Francis GT, Abbiss CR, Newton MJ, Nosaka K. Reliability of time-to-exhaustion versus time-trial running tests in runners. Med Sci Sports Exerc. (2007) 39:1374–9. doi: 10.1249/mss.0b013e31806010f5
48. Rollo I, Williams C, Nevill A. Repeatability of scores on a novel test of endurance running performance. J Sports Sci. (2008) 26:1379–86. doi: 10.1080/02640410802277452
49. Borg GA. Psychophysical bases of perceived exertion. Med Sci Sports Exerc. (1982) 14:377–81. doi: 10.1249/00005768-198205000-00012
50. Silveira RD, Carlsohn A, Langen G, Mayer F, Scharhag-Rosenberger F. Reliability and day-to-day variability of peak fat oxidation during treadmill ergometry. J Int Soc Sports Nutr. (2016) 13:4. doi: 10.1186/s12970-016-0115-1
51. San-Millan I, Brooks GA. Assessment of metabolic flexibility by means of measuring blood lactate, fat, and carbohydrate oxidation responses to exercise in professional endurance athletes and less-fit individuals. Sports Med. (2018) 48:467–79. doi: 10.1007/s40279-017-0751-x
52. Achten J, Jeukendrup AE. Relation between plasma lactate concentration and fat oxidation rates over a wide range of exercise intensities. Int J Sports Med. (2004) 25:32–7. doi: 10.1055/s-2003-45231
53. Wassermann K, McIlroy MB. Detecting the threshold of anaerobic metabolism in cardiac patients during exercise. Am J Cardiol. (1964) 14:844–52. doi: 10.1016/0002-9149(64)90012-8
54. Wassermann K, Whipp BJ, Koyal SN, Beaver WL. Anaerobic threshold and respiratory gas exchange during exercise. J Appl Physiol. (1973) 35:236–43. doi: 10.1152/jappl.1973.35.2.236
55. Roecker K, Schotte O, Niess AM, Horstmann T, Dickhuth HH. Predicting competition performance in long-distance running by means of a treadmill test. Med Sci Sports Exerc. (1998) 30:1552–7. doi: 10.1097/00005768-199810000-00014
56. Kyle UG, Bosaeus I, De Lorenzo AD, Deurenberg P, Elia M, Gomez JM, et al. Bioelectrical impedance analysis - part II: utilization in clinical practice. Clin Nutr. (2004) 23:1430–53. doi: 10.1016/j.clnu.2004.09.012
57. Bosy-Westphal A, Schautz B, Later W, Kehayias JJ, Gallagher D, Müller MJ. What makes a BIA equation unique? Validity of eight-electrode multifrequency BIA to estimate body composition in a healthy adult population. Eur J Clin Nutr. (2013) 67(Suppl 1):S14–21. doi: 10.1038/ejcn.2012.160
58. Rana SR, Chleboun GS, Gilders RM, Hagerman FC, Herman JR, Hikida RS, et al. Comparison of early phase adaptations for traditional strength and endurance, and low velocity resistance training programs in college-aged women. J Strength Cond Res. (2008) 22:119–27. doi: 10.1519/JSC.0b013e31815f30e7
59. Ainsworth BE, Haskell WL, Herrmann SD, Meckes N, Bassett DR Jr, Tudor-Locke C, et al. 2011 Compendium of physical activities: a second update of codes and MET values. Med Sci Sports Exerc. (2011) 43:1575–81. doi: 10.1249/MSS.0b013e31821ece12
60. Ainsworth BE, Haskell WL, Leon AS, Jacobs DR Jr, Montoye HJ, et al. Compendium of physical activities: classification of energy costs of human physical activities. Med Sci Sports Exerc. (1993) 25:71–80. doi: 10.1249/00005768-199301000-00011
61. Klika B, Jordan C. High-intensity circuit training using body weight: maximum results with minimal investment. Acsms Health Fit J. (2013) 17:8–13. doi: 10.1249/FIT.0b013e31828cb1e8
62. Bird SP, Tarpenning KM, Marino FE. Designing resistance training programmes to enhance muscular fitness: a review of the acute programme variables. Sports Med. (2005) 35:841–51. doi: 10.2165/00007256-200535100-00002
63. Coen B, Schwarz L, Urhausen A, Kindermann W. Control of training in middle- and long-distance running by means of the individual anaerobic threshold. Int J Sports Med. (1991) 12:519–24. doi: 10.1055/s-2007-1024727
64. Faude O, Kindermann W, Meyer T. Lactate threshold concepts: how valid are they? Sports Med. (2009) 39:469–90. doi: 10.2165/00007256-200939060-00003
65. Soenen S, Plasqui G, Smeets AJ, Westerterp-Plantenga MS. Protein intake induced an increase in exercise stimulated fat oxidation during stable body weight. Physiol Behav. (2010) 101:770–4. doi: 10.1016/j.physbeh.2010.08.019
66. Hill KM, Stathis CG, Grinfeld E, Hayes A, McAinch AJ. Co-ingestion of carbohydrate and whey protein isolates enhance PGC-1 alpha mRNA expression: a randomised, single blind, cross over study. J Int Soc Sports Nutr. (2013) 10:8. doi: 10.1186/1550-2783-10-8
67. Margolism LM, Donato AR. Implications of exercise training and distribution of protein intake on molecular processes regulating skeletal muscle plasticity. Calcif Tissue Int. (2015) 96:211–21. doi: 10.1007/s00223-014-9921-0
68. Wang L, Mascher H, Psilander N, Blomstrand E, Sahlin K. Resistance exercise enhances the molecular signaling of mitochondrial biogenesis induced by endurance exercise in human skeletal muscle. J Appl Physiol. (2011) 111:1335–44. doi: 10.1152/japplphysiol.00086.2011
69. Margolis LM, Pasiakos SM. Optimizing intramuscular adaptations to aerobic exercise: effects of carbohydrate restriction and protein supplementation on mitochondrial biogenesis. Adv Nutr. (2013) 4:657–64. doi: 10.3945/an.113.004572
70. Nakamura MT, Yudell BE, Loor JJ. Regulation of energy metabolism by long-chain fatty acids. Prog Lipid Res. (2014) 53:124–44. doi: 10.1016/j.plipres.2013.12.001
71. Sax F. Einfluss unterschiedlich wertiger Proteine auf die Proteinsynthese und Muskelzelldifferenzierung (dissertation) Universität zu Lübeck, Lübeck, Germany (2019).
72. Trowell D, Vicenzino B, Saunders N, Fox A, Bonacci J. Effect of strength training on biomechanical and neuromuscular variables in distance runners: a systematic review and meta-analysis. Sports Med. (2019) 50:133–50. doi: 10.1007/s40279-019-01184-9
73. Paavolainen L, Hakkinen K, Hamalainen I, Nummela A, Rusko H. Explosive-strength training improves 5-km running time by improving running economy and muscle power. J Appl Physiol. (1999) 86:1527–33. doi: 10.1152/jappl.1999.86.5.1527
74. Beattie K, Kenny IC, Lyons M, Carson BP. The effect of strength training on performance in endurance athletes. Sports Med. (2014) 44:845–65. doi: 10.1007/s40279-014-0157-y
75. Pasiakos SM, McLellan TM, Lieberman HR. The effects of protein supplements on muscle mass, strength, and aerobic and anaerobic power in healthy adults: a systematic review. Sports Med. (2015) 45:111–31. doi: 10.1007/s40279-014-0242-2
76. Sedano S, Marin PJ, Cuadrado G, Redondo JC. Concurrent training in elite male runners: the influence of strength versus muscular endurance training on performance outcomes. J Strength Cond Res. (2013) 27:2433–43. doi: 10.1519/JSC.0b013e318280cc26
77. Murlasits Z, Kneffel Z, Thalib L. The physiological effects of concurrent strength and endurance training sequence: a systematic review and meta-analysis. J Sports Sci. (2018) 36:1212–9. doi: 10.1080/02640414.2017.1364405
78. Garber CE, Blissmer B, Deschenes MR, Franklin BA, Lamonte MJ, Lee IM, et al. Quantity and quality of exercise for developing and maintaining cardiorespiratory, musculoskeletal, and neuromotor fitness in apparently healthy adults: guidance for prescribing exercise. Med Sci Sport Exerc. (2011) 43:1334–59. doi: 10.1249/MSS.0b013e318213fefb
79. Schoenfeld BJ, Grgic J, Ogborn D, Krieger JW. Strength and hypertrophy adaptations between low- vs. high-load resistance training: a systematic review and meta-analysis. J Strength Cond Res. (2017) 31:3508–23. doi: 10.1519/JSC.0000000000002200
80. Glowacki SP, Martin SE, Maurer A, Baek W, Green JS, Crouse SF. Effects of resistance, endurance, and concurrent exercise on training outcomes in men. Med Sci Sport Exerc. (2004) 36:2119–27. doi: 10.1249/01.MSS.0000147629.74832.52
81. Bosy-Westphal A, Jensen B, Braun W, Pourhassan M, Gallagher D, Muller MJ. Quantification of whole-body and segmental skeletal muscle mass using phase-sensitive 8-electrode medical bioelectrical impedance devices. Eur J Clin Nutr. (2017) 71:1061–7. doi: 10.1038/ejcn.2017.27
82. Silva AM, Fields DA, Heymsfield SB, Sardinha LB. Body composition and power changes in elite judo athletes. Int J Sports Med. (2010) 31:737–41. doi: 10.1055/s-0030-1255115
83. Silva AM, Matias CN, Santos DA, Rocha PM, Minderico CS, Sardinha LB. Increases in intracellular water explain strength and power improvements over a season. Int J Sports Med. (2014) 35:1101–5. doi: 10.1055/s-0034-1371839
84. Silva AM, Fields DA, Heymsfield SB, Sardinha LB. Relationship between changes in total-body water and fluid distribution with maximal forearm strength in elite judo athletes. J Strength Cond Res. (2011) 25:2488–95. doi: 10.1519/JSC.0b013e3181fb3dfb
85. Shamim B, Devlin BL, Timmins RG, Tofari P, Lee Dow C, Coffey VG, et al. Adaptations to concurrent training in combination with high protein availability: a comparative trial in healthy, recreationally active men. Sports Med. (2018) 48:2869–83. doi: 10.1007/s40279-018-0999-9
86. Fyfe JJ, Bishop DJ, Stepto NK. Interference between concurrent resistance and endurance exercise: molecular bases and the role of individual training variables. Sports Med. (2014) 44:743–62. doi: 10.1007/s40279-014-0162-1
87. Camera DM, West DW, Phillips SM, Rerecich T, Stellingwerff T, Hawley JA, et al. Protein ingestion increases myofibrillar protein synthesis after concurrent exercise. Med Sci Sports Exerc. (2015) 47:82–91. doi: 10.1249/MSS.0000000000000390
88. Ormsbee MJ, Willingham BD, Marchant T, Binkley TL, Specker BL, Vukovich MD. Protein supplementation during a 6-month concurrent training program: effect on body composition and muscular strength in sedentary individuals. Int J Sport Nutr Exerc Metab. (2018) 28:619–28. doi: 10.1123/ijsnem.2018-0036
89. Arampatzis A, De Monte G, Karamanidis K, Morey-Klapsing G, Stafilidis S, Bruggemann GP. Influence of the muscle-tendon unit's mechanical and morphological properties on running economy. J Exp Biol. (2006) 209:3345–57. doi: 10.1242/jeb.02340
90. Albracht K, Arampatzis A. Exercise-induced changes in triceps surae tendon stiffness and muscle strength affect running economy in humans. Eur J Appl Physiol. (2013) 113:1605–15. doi: 10.1007/s00421-012-2585-4
91. Kjaer M. Role of extracellular matrix in adaptation of tendon and skeletal muscle to mechanical loading. Physiol Rev. (2004) 84:649–98. doi: 10.1152/physrev.00031.2003
92. Praet SFE, Purdam CR, Welvaert M, Vlahovich N, Lovell G, Burke LM, et al. Oral supplementation of specific collagen peptides combined with calf-strengthening exercises enhances function and reduces pain in achilles tendinopathy patients. Nutrients. (2019) 11:76. doi: 10.3390/nu11010076
93. Watt MJ, Cheng YS. Triglyceride metabolism in exercising muscle. Bba-Mol Cell Biol L. (2017) 1862:1250–9. doi: 10.1016/j.bbalip.2017.06.015
94. Brook MS, Wilkinson DJ, Atherton PJ, Smith K. Recent developments in deuterium oxide tracer approaches to measure rates of substrate turnover: implications for protein, lipid, and nucleic acid research. Curr Opin Clin Nutr Metab Care. (2017) 20:375–81. doi: 10.1097/MCO.0000000000000392
95. Sillanpaa E, Hakkinen A, Hakkinen K. Body composition changes by DXA, BIA and skinfolds during exercise training in women. Eur J Appl Physiol. (2013) 113:2331–41. doi: 10.1007/s00421-013-2669-9
96. Schoenfeld BJ, Nickerson BS, Wilborn CD, Urbina SL, Hayward SB, Krieger J, et al. Comparison of multifrequency bioelectrical impedance vs. Dual-energy x-ray absorptiometry for assessing body composition changes after participation in a 10-week resistance training program. J Strength Cond Res. (2020) 34:678–88. doi: 10.1519/JSC.0000000000002708
Keywords: running distance, endurance performance, lactate threshold, body composition, concurrent training, collagen peptide, protein supplementation
Citation: Jendricke P, Kohl J, Centner C, Gollhofer A and König D (2020) Influence of Specific Collagen Peptides and Concurrent Training on Cardiometabolic Parameters and Performance Indices in Women: A Randomized Controlled Trial. Front. Nutr. 7:580918. doi: 10.3389/fnut.2020.580918
Received: 07 July 2020; Accepted: 26 October 2020;
Published: 19 November 2020.
Edited by:
Bryan Saunders, University of São Paulo, BrazilReviewed by:
Eimear Dolan, University of São Paulo, BrazilGregory Shaw, Swimming Australia, Australia
Copyright © 2020 Jendricke, Kohl, Centner, Gollhofer and König. This is an open-access article distributed under the terms of the Creative Commons Attribution License (CC BY). The use, distribution or reproduction in other forums is permitted, provided the original author(s) and the copyright owner(s) are credited and that the original publication in this journal is cited, in accordance with accepted academic practice. No use, distribution or reproduction is permitted which does not comply with these terms.
*Correspondence: Patrick Jendricke, cGF0cmljay5qZW5kcmlja2VAc3BvcnQudW5pLWZyZWlidXJnLmRl