- Human Nutrition Unit, Department of Medicine, School of Biological Sciences, University of Auckland, Auckland, New Zealand
Cow's milk and dairy products derived from this complex food source have long been proposed as beneficial to human health, yet underlying clinical evidence of direct benefit continues to raise controversy. Limited evidence supports positive cardiometabolic effects of a number of dairy macro- and micronutrient components including whey protein and casein, unsaturated fats, milk fat globule membrane (MFGM) and polar phospholipids, vitamin D and calcium, in addition to non-bovine components including bacterial and yeast probiotics. More controversial remain lipid components trans fats, including trans vaccenic acid, trans palmitoleic acid, and conjugated cis trans linoleic acid (CLA), plus medium-chain and odd-chain dairy fats. New evidence is rapidly identifying multiple pathways by which these dairy nutrients may effect health. Processing, including fermentation and homogenization, may also have positive effects. Conversely, the high saturated fat content of dairy has long raised concern, aligned with international guidelines to minimize dietary intake of animal-origin saturated fatty acids (SFA) to achieve better cardiometabolic health. However, led in part by observational studies and meta-analyses showing dairy to have no or even an inverse association with cardiometabolic health, evidence from randomized controlled trials (RCTs) has been scrutinized over the last 5 years, and focus on low-fat dairy has been challenged. Recent evidence supports the hypothesis that adverse effects of SFAs on metabolic health may be ameliorated when these fats are consumed within a complex matrix such as milk, cheese or yogurt, and that dairy food categories may influence outcomes as much as total fat content. For example, yogurt and high-fat, high-SFA cheese have a negative association with risk of type 2 diabetes (T2D) in many, not all, published trials. However, large sample dairy RCTs of long duration with CVD or T2D incidence as primary endpoints are lacking. This is a clear research gap, with these clinical studies required if a causative link between dairy and improved cardiometabolic health is to be confirmed and in turn promoted through dietary guidelines. Current advisories from national guidance groups such as American Heart Association (AHA) and European Society of Cardiology (ESC) continue to promote consumption of low-fat dairy products, whilst liquid milk and yogurt remain part of nutrition guidelines from joint American Diabetes Association (ADA)/European Association for Study of Diabetes (EASD) reports, and as part of a “no-one-size-fits-all” answer to diet and T2D by the ADA in their most recent 2019 Consensus Report.
Introduction
Cow's milk and dairy products derived from this complex food source have long been proposed as beneficial to human health, yet underlying clinical evidence supporting direct benefit to cardiometabolic health continues to raise controversy, based primarily on the high saturated fatty acid (SFA) content of whole-fat dairy. Whether high levels of dietary SFAs per se do indeed initiate a cascade of worsening intermediary blood markers including an adverse lipoprotein profile, and in turn lead to higher prevalence of cardiometabolic disease is now under considerable scrutiny. This narrative review aims to present the most recent evidence from both observational cohorts and randomized controlled trials (RCTs) that investigate the relationships between dairy and risk of cardiovascular disease (CVD) and type 2 diabetes (T2D), and to evaluate the evidence provided by these different study types. Also to identify, where possible, potential mechanisms by which dairy nutrients may promote health benefits.
Concern around dairy and potential adverse health outcomes arose from early epidemiologic data which supported a strong association between animal-origin food groups that provide a major source of dietary SFAs and an increased risk of CVD (1). In countries such as the UK, milk and dairy foods contribute almost 30% of SFA intake, and so quite reasonably have been considered a food component of concern (2). However, current literature shows growing support for the proposal that dairy products may have a neutral or even positive effect on CVD outcomes (3), with a number of meta-analyses supporting this relationship (4–9). There is also a new but growing consensus that the matrix of a whole-fat food such as dairy may be more important than the content and composition of component isolated fatty acids. Such that food-based rather than nutrient-based recommendations should be developed for CV health (3, 10, 11). It is notable however that the predominance of this evidence is obtained from observational studies. There is less evidence from RCTs, particularly with reference to incident CVD where long-term dairy interventions evaluating hard CV event points are lacking. This may be critical when aiming to interpret findings suggestive of a positive relationship with regular- and high-fat diary, and in order to develop robust public health recommendations.
A number of meta-analyses and systematic reviews have also focused on dairy and T2D (4, 12–17), again showing an inverse association between dairy intake and risk of T2D in observational studies. A recent expert panel position paper (18) reported a number of key findings including the evidence from large prospective cohort studies that total dairy consumption has a neutral or moderately beneficial effect on T2D risk. Again notably this is an outcome supported only by limited evidence from randomized controlled trials (RCTs) (19), with no long-term interventions investigating the effect of high-fat dairy on incident T2D. Dairy category is clearly important, again with evidence from prospective cohort studies showing fermented yogurt to be most strongly associated with lower T2D risk. There is with sparse evidence from RCTs. Even in 2020, the balance of evidence is predominantly from observational studies. Larger and longer duration clinical intervention trials are needed, with incident T2D as the primary outcome. RCTS are also required for better understanding of the underpinning mechanisms by which dairy may potentially be protective.
Dairy composes about 10% of the energy consumed in, for example, a typical North American diet, of which approximately half is from fluid milk, half from fermented (or “cultured”) cheese, and a small percentage from fermented yogurt (20). Important nutrients found in the myriad of dairy formats include milk proteins, calcium, magnesium, potassium, medium- and odd-chain saturated fats, specific fatty acids, and low-glycemic index (GI) sugars; shown to have beneficial effects on aspects of glucose control, insulin secretion, insulin sensitivity and/or T2D risk (21) as well as a range of CV risk factors (22). Notably a number of authors (23–26) have recently highlighted the importance of focusing on foods and dietary patterns rather than simply individual dietary nutrients when assessing CVD and T2D risk. In turn it is clear that expanding response to these dietary patterns beyond simple body fatness and circulating blood lipids into the multiple risk outcomes now identified as important to CV health is important.
Methods
A literature search was conducted using MEDLINE (via PubMed) to identify observation cohorts and intervention trials that investigated the association between dairy consumption and cardiometabolic health. Medical Subjects Headings (MeSH) included dairy OR milk OR butter OR cheese OR yogurt AND CVD OR diabetes; reviews, including systematic reviews, meta-analyses, umbrella reviews, narrative reviews.
Cardiometabolic Effects of Dairy Macro- and Micro-Nutrients: Evidence From Clinical Studies
Dairy Proteins
Whey and Casein
Among the various types of animal-based protein foods, a higher intake of protein-rich dairy products has been reported to be associated with a beneficial relationship with a range of CV endpoints in addition to glucose regulation and T2D risk reduction (16, 19, 27, 28). Milk proteins can be categorized in the simplest terms into two groups, based on solubility, as serum or whey proteins and caseins. The whey proteins remain soluble at pH 4.6 and 20°C, whilst the caseins (or “curds”) precipitate out. Molecular and physicochemical properties of whey protein and caseins are highly varied, with the ratio of total whey:total casein in cow's milk of ~20:80% (29).
Although a number of large observational cohort studies do support the association between higher intake of dairy foods and neutral or lower risk of adverse CV health, data is sparse from observational studies for whey or casein per se. There however are several systematic reviews of RCTs that identify positive effects of whey protein. Wirunsawanya et al., recently reported on whey supplementation and CV endpoints in 9 RCTs of overweight and obese cohorts, and identified an improvement in serum lipoproteins for total and HDL-cholesterol in addition to improvement in blood pressure (30), although notably these effects were likely driven by body weight loss. There has been a growing literature on positive effects of whey protein on hypertension, where mechanisms are purported to include angiotensin converting enzyme (ACE) inhibition, normalization of endothelial function and opioid receptor-dependent effects, although not all studies show positive long-term outcomes (31). Recently a comprehensive systematic review by Badely et al. >2,000 individuals from 37 published RCTS, again in overweight and obese adults, showed whey protein administered in multiple forms including protein isolate, concentrate, extract, supplement and hydrolysate had a positive outcome on several CV markers. They reported a decrease in fasting triglycerides and blood pressure, but also an adverse decrease in HDL-cholesterol (32). Whilst not reporting change in body weight these outcomes were accompanied by a decrease in waist circumference, which is an indirect measure of central adiposity expected to also be accompanied by weight loss. Whey protein has long been shown to have modest positive effects on body composition during weight loss (33), but whether there are effects of whey protein on CV endpoints independent of change of body weight remains to be convincingly demonstrated. Previous observational studies have reported weight independent effects of total protein intake on cardiometabolic health, with authors in turn noting that the role of protein in CV health likely depends on the specific protein source (34).
Evidence that protein-rich dairy products may be beneficial for T2D has largely come from observational studies (27) where again attribution of positive effects of metabolic health to dairy proteins per se is equivocal. Notably the relationship between increased intake of total protein from the diet and cardiometabolic endpoints is not without controversy (35, 36), in particular for T2D where some cohort studies show high total protein or animal protein intake to be associated with an increased risk of disease (37–39), including some proteins of dairy origin (40). Clearly protein source and quality is of considerable importance when determining dietary guidelines for prevention of T2D. Clinical evidence from RCTs regarding both dairy foods and dairy proteins point to wide ranging interventions reporting enhanced insulin secretion and associated endpoints, which in turn may result in better glycemic control. Wirunsawanya and colleagues, in their meta-analysis of whey protein from nine RCTs in overweight and obese referred to previously, showed significant improvement in fasting glucose albeit alongside a parallel decrease in body weight (30). Badely et al., also reported improvements in blood glucose in their systematic review of 37 published whey protein intervention trials in overweight and obese (32), but again whether this is independent of weight loss is not determined. This body of evidence is predominantly based on short duration studies of intermediary metabolic endpoints rather than long-term trials of incident T2D prevention, with long-term interventions of dairy protein on T2D endpoints or incidence lacking. Two trials planning to investigate moderate-term whey protein intervention for T2D management over a 3 months duration can be identified through review of international clinical trial registries. Almario et al. who have published acute effects (41) were unable to undertake their trial due to personnel changes [information kindly provided by the investigators; (42)]. Jakubowicz et al. (43) showed decrease in both postprandial glucose and HbA1c on the high whey protein arm when compared with a low protein soy control. Notably, long-term dietary interventions for T2D prevention in overweight and high risk individuals to date are focused almost entirely on manipulation of the fat and carbohydrate components of the diet (44). The large European-led 2,500 participant, 3 years RCT, PREVIEW (PREVention of diabetes through lifestyle intervention In Europe and around the World) is the first long-term study to compare a novel higher total protein diet with a higher carbohydrate diet for incident T2D prevention (45). No significant improvement over the current best practice higher carbohydrate diet was found however. No long-term dairy protein RCTs investigating prevention of incident T2D have as yet been reported.
Acute intervention studies have provided some insights into potential mechanisms (46), with evidence that dairy proteins have more potent effects on insulin and incretin secretion compared to other commonly consumed animal proteins (21). Insulinotropic effects are associated with both the amino acid composition and the bioactive peptide profile, where for example inhibition of dipeptidyl peptidase-4 (DPP-4) increases incretin levels, which in turn inhibits glucagon release, increases insulin secretion and decreases gastric emptying (Figure 1). In concert, these in turn decrease circulating blood glucose concentrations. The amino acid content is driven in large part by the major contribution of whey protein to dairy, and consequent high levels of branched-chain amino acids (BCAAs) leucine, isoleucine and valine, as well as lysine (47). Clinical studies have reported dairy AAs, including leucine, isoleucine, glutamine, phenylalanine, proline and lysine, to have beneficial effects on glucose homeostasis. BCAAs stimulate the secretion of insulin and glucagon in addition to incretins glucagon-like peptide-1 (GLP-1) and glucose-dependent insulinotropic polypeptide (GIP) (47). In practical terms however, whether the dose of amino acids required to attain these protective responses can readily be achieved through recommended daily dairy intake is less well established (48). Clearly, more longer-term studies that can unravel the relationship between dairy protein and insulin and glucose control are needed.
In summary, there are no large RCTs evaluating effects of long-term dairy protein intake on either CVD or T2D incidence. There are however some preliminary conclusions to be drawn. Whilst cohort studies relating whey and casein per se to CV and T2D outcomes are lacking, RCTs have identified beneficial moderate-term effects of whey protein on intermediary risk factors including lipids and lipoproteins, blood pressure, and glucose-related parameters including insulin secretion. Acute postprandial studies provide evidence for whey protein and constituent AAs as promoting insulin secretion as a mechanism for improved glycemic control. Since much of the RCT evidence, however, has been obtained in overweight cohorts, the possibility that body weight and adipose mass loss may contribute to improvements in metabolic health seen in these dairy intervention trials cannot be excluded.
Dairy Fats
The recent expert panel position paper referred to previously also reported on evidence underpinning relationships between dairy fatty acids and metabolic health. The panel concluded that medium-chain fatty acids/triglycerides (MCFA/MCT), odd chain fats, very long-chain SFAs and trans-palmitoleic acid were associated with lower T2D risk and improved metabolic health (18). They also noted, however, the possibility that findings were confounded by dairy fatty acids acting as markers of overall dairy consumption. Other evidence has been presented for milk fat globule membrane (MFGM) and polar phospholipids, trans vaccenic acid, and conjugated linoleic acid (CLA) in relation to both T2D and CVD risk, as summarized below.
Medium Chain Triglycerides (MCT)
MCTs are classified as lipids containing medium chain fatty acids of 6–12 carbon chain length. They are minor components of global diets but have long been investigated as a potential dietary lipid substitute for the more abundant long chain triglycerides (LCT). Milk is a reasonable source of saturated MCTs, comprising up to ~15% of total lipid (22). MCTs undergo rapid hydrolysis and absorption, and suppress lipedema, hypothesized to predispose MCTs as cardioprotective (49). Evidence remains preliminary however. MCTs undergo almost complete hydrolysis to free fatty acids (FFA) and are absorbed directly into the portal vein, and hence transported rapidly to the liver for oxidation. This is in contrast to LCTs which are more slowly absorbed and transported by chylomicrons into the systemic circulation prior to oxidation or storage. The concept of rapid oxidation enhancing peripheral satiety signals and in turn promoting body weight control has been investigated in several studies, but with little underpinning evidence from human studies (50).
Evidence of enhanced metabolic health comes from several sources. In vitro studies of skeletal muscle cells show MCTs to enhance oxidative mitochondrial capacity and decrease lipid accumulation relative to LCTs, but not activate NF-κB or decrease insulin sensitivity (IS) (51, 52). Preliminary evidence also exists from in vivo studies, reporting ameliorated body fat accumulation (53) and insulin resistance in animals fed MCTs vs. saturated LCTs, which aligns with these prior studies. In the DairyHealth study, a 12 weeks cow's milk intervention conducted in Europe, dairy MCTs have been shown to induce gene expression of energy metabolism-related pathways in adipose tissue samples collected by biopsy from adults with abdominal obesity (54). These authors also showed a cardiometabolic-protective decrease in inflammation-related gene expression. Conversely, in animal models MCTs have also been shown to increase hepatic de novo lipogenesis and triglyceride accumulation, and adversely decrease hepatic IS, when compared with a lower fat diet (51, 53). Ameliorated lipid storage has also been reported in clinical studies of overweight adults, including following consumption of high-MCT dairy butter in combination with dairy protein (55). The DairyHealth study of abdominally obese adults also reported a higher intake of milk MCT to increase lean body mass and decrease total body fat % over 12 weeks, in an isoenergetic fat quality manipulation (56).
Odd Chain
The odd-chain fatty acids (OCFAs), pentadecanoic (C15:0) and heptadecanoic acid (C17:0), are SFAs that are found in dairy. Minor components that comprise ~1.5% of milk fat, with C15:0 reported as approximately twice as abundant as C17:0. Long investigated as potential independent serum biomarkers of dairy intake (57, 58), these SFAs with odd number of carbons were proposed to be synthesized only by bacterial flora of ruminants (59, 60) with no metabolic precursors in humans. However, this has been challenged by emerging data of endogenous synthesis and metabolism of these OCFAs (61, 62). Adipose tissue depots rather than circulating fatty acids have more recently shown promise, with evidence that adipose C15:0 may reflect both habitual intake as well as changes in intake of dairy foods (63). With respect to health, recent reviews have reported that dairy OCFAs may be inversely associated both with cardiometabolic risk (20) and T2D (63) in cohort studies. C15:0 has also been proposed as an essential fatty acid (64) with some evidence in animal models of amelioration of inflammation and dyslipidemia.
Milk Fat Globule Membrane (MFGM) and Polar Phospholipids
The MFGM is the biological membrane that surrounds the lipid droplets in liquid milk. This and associated phospholipid (PL) components of milk continue to be associated with health benefits including those of metabolic health, as recently reviewed by Anto et al. (65) in a narrative review which presented 11 RCTs of milk PL effects on circulating serum lipids. A growing evidence base is proposed for conditions as varied as adverse lipid metabolism (66–68), insulin resistance (69), inflammation (70), CVD through attenuated development of atherosclerosis (65), gut health (71) and neurodevelopment. Structurally the MFGM encases the fat globules within milk. It is comprised of proteins, cholesterol and polar rather than neutral lipids, including PLs such as phosphatidylcholine (PC) and sphingolipids such as sphingomyelin (SM). Recent mass spectral analysis of bovine milk has confirmed PC, phosphatidylethanolamine (PE) and SM as the most abundant polar lipid classes (72). The total polar lipid content of a product may vary greatly as a result of dairy processing, but typically comprise only ~1% of the total lipid content of milk. Yet they contribute to several classical dairy food performance characteristics. The ability to stabilize oil-in-water emulsions is one of these, such that the polar lipids enable the emulsification of neutral triglyceride in the aqueous phase of liquid milk. When consumed in significant amounts within the diet, PLs can inhibit lipid absorption from the GI tract, acting mainly by altering mobilization of lipid from spherical micelles that form in aqueous solution (73). Disruption of the MFGM, such as during homogenization, is discussed in more detail in sections below.
Trans-vaccenic Acid and Trans-palmitoleic Acid
Trans-11 vaccenic acid (C18:1, t11) is a monounsaturated “natural” trans fat, specific to ruminant-origin products of dairy and meat. There is some evidence (74), although not universal (75, 76), that it lacks the adverse cardiometabolic health outcomes associated with other forms of dietary trans fats, long developed by the food industry through industrial lipid hydrogenation. There are a number of in vivo and in vitro reports of positive effects of dairy trans fats on metabolic endpoints including increased insulin secretion and pancreatic islet β-cell growth (77), decreased hyperlipidemia (78), and ectopic liver fat accumulation (79). These reports however must be balanced against others that do not support a health benefit. A 2010 systematic review of 39 clinical intervention trials by Brouwer et al. (75) included 29 industrial trans fats, 17 CLA and six ruminant trans fats studies. They concluded that all fatty acids with a trans double bond raised the LDL- to HDL-cholesterol ratio in an adverse manner. In an update of this review they noted the contradictory evidence that observational studies fail to demonstrate a higher risk of CVD associated with high intake of ruminant trans fats, despite confirming adverse effects on circulating blood lipid profile (80). Conversely, evidence of positive outcomes for T2D has been building, where monounsaturated trans-palmitoleic acid (16:1, t9) is of particular interest. Associated in recent publications with a lower incidence of T2D (18, 81) and coronary artery disease (CAD) (81), it is both consumed within dairy as well as delivered through endogenous conversion from its metabolic precursor trans-vaccenic acid (82). Evidence for a protective effect of dairy trans-palmitoleic comes from a recent large meta-analysis of 16 prospective cohort studies comprising more than 60,000 participants. Conducted by the fatty acids and outcomes research consortium (FORCE) this analysis showed a significant association of trans-palmitoleic with a lower risk of T2D (58). A note of caution must be added however, with several authors questioning the robust nature of methods used for analysis of fatty acid methyl esters (FAMEs) from bovine milk, citing difficulties in full separation and unequivocal identification of FAMEs within the very complex lipid composition of ruminant milk (83, 84). The various pathways linking these trans fats to CLA are now reasonably well understood, including desaturation of trans-11 vaccenic acid (18:1) by the Δ9-desaturase enzyme within the mammary gland to form cis-9, trans-11 CLA (18:2) (Figure 2). This is in addition to formation of CLA via biohydrogenation of linoleic acid (18:2) within the bovine rumen, and in turn synthesis of trans-vaccenic. Synthesis via Δ9-desaturase has been proposed to be the primary source of cis-9, trans-11 CLA in bovine milk fat (85).
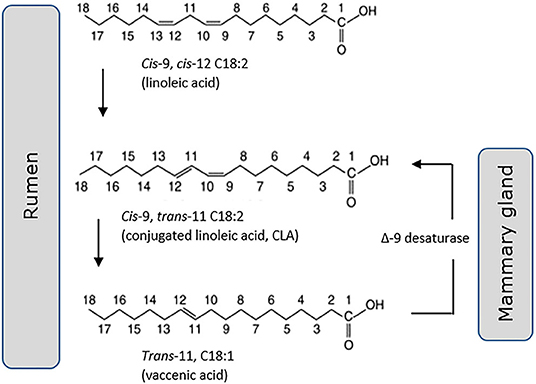
Figure 2. Cis-9, trans-11 CLA (18:2) is generated both through hydrogenation of linoleic acid (18:2) in the rumen and desaturation of trans-11 vaccenic acid (18:1) in the mammary gland.
Conjugated Linoleic Acid (CLA)
CLA is a polyunsaturated fatty acid (PUFA) with conjugated double bonds found in both cis and trans forms, the most common isomer of which is cis-9 trans-11 linoleic acid. Despite being found in ruminant dairy and meat, and in lesser amounts in plant oils and seafood, CLA is also widely produced industrially for sale as a supplement. Much like vaccenic acid, there are reports of cardiometabolic health benefits (86, 87). A systematic review and meta-analysis of 33 RCTs that investigated the effect of ruminant CLA, in either supplement form or from enriched foods, on lipid profile in healthy adult populations reported that both formats significantly decreased LDL-C with minor non-significant effects on other lipid outcomes (87). The review also however noted concerns on potential safety of CLA with reports of increased insulin resistance and lipodystrophy in animal models, in addition to some adverse lipid and C-RP responses in clinical studies. In line with this note of caution, several recent reviews have concluded that many positive outcomes reported in animal models or in vitro have not been adequately replicated in clinical trials (88, 89). The 2018 review by Li et al. reported the lack of clinical data for hard CV endpoints. They identified only 1 observational case-control study showing an inverse relationship between levels of cis-9, trans-11 CLA in adipose tissue and the risk of heart attack (90), and 3 RCTs all showing no significant effect of CLA on blood lipids, glucose, CRP, blood pressure, insulin resistance, body composition or 10-year absolute risk of fatal CVD in obese patients (91). There was a similar lack of efficacy reported in healthy cohorts (92) and patients with atherosclerosis (93). There is evidence of improved C-RP levels from one intervention trial in patients with active CVD (94). Conversely there is evidence, as for vaccinic acid, that CLA adversely affects lipoprotein profile, with increased circulating LDL-cholesterol and total:HDL-cholesterol ratio in an exhaustive review of 48 CLA and 11 ruminant trans fat RCTs (80). In addition, worsening of associated CVD risk factors including the acute phase protein C-RP [Haghighatdoost and Nobakht-M-Gh 2018, (95)] and cytokine TNF-α have been identified in a recently published systematic review (96). In addition, a 2017 meta-analysis of 32 RCTs of food-enriched and supplement CLA has also shown no significant effect on fasting glucose (88). A recent narrative review has noted lack of consensus interpreting effects of CLA on body weight loss and obesity due to small sample size, variable dose, variable CLA isomers, variable intervention durations and population characteristics (97); but that beneficial effects of supplementation on parameters related to body weight and/or adiposity have been reported in a number of trials, with no evidence of adverse metabolic consequences. This review also reports similar absence of consensus for glycemic endpoints, with both beneficial glucose metabolic effects and no clinical benefit reported in RCTs (97).
In summary, there are no RCTs evaluating long-term effects of these specific dairy fat components on either CVD or T2D incidence. Very little evidence supports MCTs as cardioprotective, with preliminary clinical findings related only to modest improvements in body composition. In turn, OCFAs have been associated with decreased CV and T2D risk in cohort studies, but no intervention data is available. Conversely, there is limited evidence that MFGM and the associated PL components of dairy may improve CV and T2D intermediary endpoints, in RCTs of 1 day to 12 weeks duration reporting outcomes including lipid metabolism, inflammatory response and insulin resistance. The evidence that underpins trans-fats is more complex and controversial. Ruminent-origin trans vaccenic acid (trans-VA) may not generate the well-demonstrated adverse response in CV risk endpoints of industrial trans-fats. There is lack of concordance in the evidence base however, with proposed neutral effects of trans-VA observed in cohort studies not being supported by RCT evidence, where systematic review has concluded that all fatty acids containing a trans double bond adversely increase lipoprotein profiles, irrespective of their origin. Conversely there are reported positive T2D risk outcomes for the downstream product of trans-VA, trans-palmitoleic acid (trans-PA) which is also present in minor quantities in dairy, and which cohort studies have correlated with decreased T2D risk. CLA has also been proposed as cardioprotective, with both ruminant-origin and industrial-origin supplements found within the western diet, and highly variable levels of evidence published. From meta-analysis of RCTs showing both significant improvement and significant worsening in lipoprotein risk factors, alongside no significant effects on FPG, to very limited data supporting an association with improved CV incidence in observational studies. There remains no current consensus on outcomes likely due to factors including the highly variable isomers and dose of CLA consumed, size and type of experimental cohorts recruited, and duration of the RCTs.
Micronutrients: Vitamin D, Calcium
Relationships between vitamin D, calcium, and various aspects of cardiometabolic health have long been controversial. In this review, focus has been given to the evidence underpinning relationships between vitamin D and T2D, and calcium and CVD. Dairy naturally contains little vitamin D but is a food category much debated with respect to fortification. Fluid milk and its myriad products have a history of both mandatory or voluntary fortification with vitamin D by the food industry. A recent review reported milk products currently to be systematically, either mandatory or voluntary, fortified with vitamin D only in European Nordic countries of Finland, Norway, Sweden as well as in North American countries of Canada and the United States (98).
There has been conflicting observational evidence for a number of years which has linked low levels of 25-hydroxyvitamin D (25(OH)D) to T2D, and has led to vitamin D replacement interventions conducted with intent to both improve glycemic control and decrease T2D incidence (99). Both synthesized in the skin in response to sunlight exposure and/or consumed within the diet and as a supplement, 25(OH)D is the main circulating form of vitamin D. It has been linked to dysglycaemia and T2D through mechanisms that affect both insulin resistance and pancreatic β-cell insulin secretion, as well as inflammation and accumulation of advanced glycation end (AGE) products (Figure 3). A number of early systematic reviews and meta-analyses supported the protective inverse relationship between vitamin D and T2D. An analysis of 8 observational cohort studies reported that high vitamin D status (>25 ng/ml) was associated with an almost halving of risk of T2D compared with low status (100). Another more recent review of 21 cohort studies showed a similar relative risk of 0.62 and using linear trend analysis also reported that for each 10 nmol/L increment in circulating 25(OH)D levels there was an associated 4% lower risk of T2DM (101). A further meta-analysis of 16 cohort studies also showed a positive outcome (102). Adiposity and adipose mass is a confounding factor in the relationship between vitamin D and T2D. Vitamin D is sequestered in adipose tissue, with the greater adipose mass reported to act as a “reservoir” for vitamin D, and the increased amount of vitamin D required to saturate the large depots of obese individuals predisposing to low levels of circulating 25(OH)D (103). In turn obesity has long been identified as a very strong predisposing factor for T2D (104).
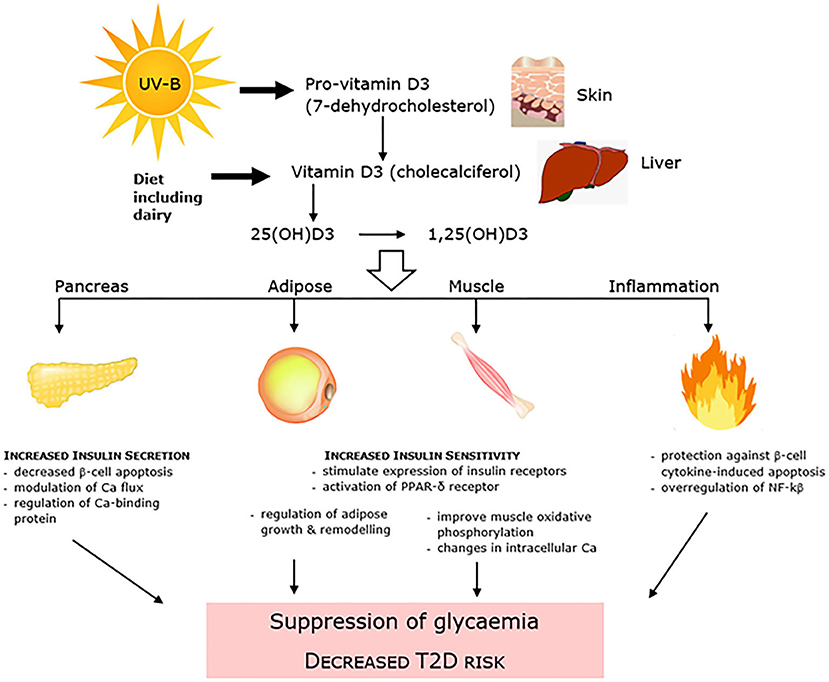
Figure 3. Vitamin D is linked to dysglycaemia and T2D through mechanisms that affect both pancreatic β-cell insulin secretion and insulin resistance, as well as systemic inflammation.
Far fewer clinical intervention trials have been conducted, with confounding issues including failure to reach sufficient circulating levels of vitamin D (105). Required circulating levels of 25(OH)D necessary to effect glycemic control and decrease risk of developing T2D, have not yet been determined. It is speculated that these may be higher than that required for optimal bone health (99). Conclusions have varied widely from meta-analyses that report positive cardiometabolic outcomes, including findings from an analysis of 9 RCTs which showed that vitamin D-fortified yogurt decreased HOMA-IR, fasting serum glucose and total cholesterol in normoglycaemic and T2D patients (106), and from analysis of 19 RCTs which showed improvements in both IR and HbA1c in T2D patients (107). Conversely prior systematic reviews reported no effect of vitamin D supplementation on glycemic outcomes in a meta-analysis of 11 (100), and 15 RCTs of normoglycaemic and T2D patients. The latter however showing that sub-cohort analysis of T2D or IGT patients resulted in a small effect on fasting glucose and IR, but not HbA1c (108).
Calcium and metabolic health has been under considerable scrutiny in recent years. Overall, calcium is not a convincing contributor to cardiometabolic benefits that may be seen from dairy. Commonly used as a supplement to promote bone health with contributions to prevention of fracture risk in the elderly, meta-analysis of long-term RCTs unexpectedly identified calcium supplementation to result in moderately increased risk of heart attack (109, 110). The mechanism that may underpin this risk has been proposed to be postprandial hypercalcemia, in turn contributing to vascular calcification. Interestingly, single-nucleotide polymorphisms (SNPs) related to higher serum calcium levels have more recently been associated with increased risk of coronary artery disease and myocardial infarction in genome wide studies (111). It has become clear, however, that it is important to differentiate the origin of the calcium consumed, with different effects between calcium consumed as a dietary supplement vs. calcium consumed as a food from dairy sources. Calcium supplements, but not calcium consumed within food products, have been proposed to result in a negative risk-benefit, with routine use for prevention or treatment of osteoporosis no longer universally recommended (112). Indeed, current evidence no longer supports routine calcium or vitamin D supplementation for bone health in healthy adults resident within the community, based in major part on the adverse CVD outcomes (113).
The evidence in support of dairy-origin calcium for T2D prevention is also mixed, although as recently reviewed (22), there are multiple mechanisms by which calcium may influence T2D risk factors. This includes regulation of insulin-mediated intracellular processes in specific tissues that respond to this peptide, contribution to secretory function of β-cells within the pancreas, and phosphorylation of insulin receptors. Calcium has also been shown to down-regulate genes encoding pro-in?ammatory cytokines that are involved in IR. In their review Mozaffarian et al. also reported >20 observational studies on associations between calcium intake and T2D prevention (22), of which 3 large cohort studies in US and China showed an inverse association between high calcium intake and low T2D risk in subcohorts of women but not men. A 4th large cohort study in Japan also observed this inverse relationship but only in subcohorts with higher serum vitamin D. The other smaller studies reported mixed outcomes, with no clear consensus. Additional to this review, the Korean Genome and Epidemiology study [KoGES (114)], a prospective cohort trial followed for 10 years, reported the positive outcome that higher dietary calcium, but not serum calcium, was associated with a lower risk of developing T2D in a study of >10,000 adults from an Asian cohort.
Processing: Fermentation, Probiotics, and Homogenization
There is considerable evidence that processing methods including fermentation, addition of probiotic bacteria and yeasts, and homogenization can all influence cardiometabolic health, as presented in the sections below.
Fermentation
Both cheese (“cultured”) and yogurt are commonly consumed fermented dairy products. The fermentation process requires a starter culture, which may vary greatly in order to achieve required texture, flavor and safety profile. Lactic acid bacteria are the major bacteria used in the fermentation process of modern food products, and can include the genera Lactobacillus, Lactococcus, Streptococcus, Leuconostoc, Pediococcus, and Enterococcus, whilst yeasts and molds are also used in cheese production (115). Acidification rate through production of lactic acid in addition to the secretion of secondary metabolites, including bacteriocins, biogenic amines, exopolysaccharides, and proteolytically released peptides, are key aspects of the fermentation process. Notably, neither cheese nor yogurt exert the adverse effects on blood lipids that would be predicted solely by the content and composition of their component SFAs (116, 117). Consumption of yogurt is clearly associated with decreased risk of both T2D and cardiometabolic disease, as reviewed by the recent expert panel of Guo et al. (18). Whilst a number of factors may contribute, the fermentation processes and the probiotic content and composition have both been proposed as significant contributors.
Probiotics
Probiotic bacteria are common components of fermented foods, including dairy set and drinking yogurts and the fermented dairy drink kefir. Kefir, similar to a liquid drinking yogurt, is a more recent introduction to the wider Westernized diet, prepared through inoculation of ruminant milk with kefir “grains,” a product which contains both bacteria and yeasts. Multiple probiotic genera have been evaluated in cohort studies and RCTs, with the two most common being Lactobacillus and Bifidobacterium. Probiotics are long proposed to have positive health effects achieved through alterations in both composition and function of the host large bowel microbiome, and there is a large literature investigating obesity-related metabolic health outcomes (118). Mechanisms proposed include improved intestinal epithelial integrity decreased low grade endotoxemia-induced inflammatory response, and promotion of colonic short chain fatty acids (SCFA) including butyrate as a nutrient source for the large bowel colonocytes (119, 120). It has been reported both that probiotic effects of yogurt may modulate glycaemia and related endpoints, and exert beneficial effects (117), or conversely that the evidence is lacking. Astrup evaluated both cohort studies and RCTs, concluding that observational data supported the significant association between yogurt, decreased risk of body weight gain, and CVD, but that notably this evidence was supported only in part by RCTs. A later 2017 review that reported on 7 RCTs, in addition to animal model evidence, in turn also concluded that the effects of probiotics on glycemic control were conflicting (121). A 2016 narrative review (122) of probiotics, prebiotics and combination symbiotic RCTs concluded that probiotics have only a small (~3%) effect on metabolic endpoints, with outcomes maximized when consumed within fermented milks or yogurts over an extended period of at least 8 weeks.
An important question is whether there is an independent causative relationship between probiotics per se and improvements in CVD, T2D or other metabolic health outcomes, independent of the food matrix such as yogurt. Notably, a recent systematic review and meta-analysis of RCTs evaluated the effects of probiotic yogurt vs. control yogurt on glycemic outcomes in obese individuals and those with T2D and reported no additional benefits of the probiotic yogurt (123). A comprehensive recent systematic review and meta-analysis of 20 prospective cohort studies and 52 RCTs (124) has highlighted the positive effects of fermented milks, yogurts and probiotics. The review reported fermented milk consumption to be associated with decreased CV risk, with probiotics added into dairy matrices lowering serum lipid profile, while yogurt consumption was associated with decreased T2D and metabolic syndrome risk. The authors did note however that heterogeneity of these multiple studies including variability in probiotic strains requires the outcomes to be interpreted with caution.
Homogenisation
Cow's milk is commonly homogenized in order to increase the physical stability of the food product. It aids in the prevention of separation of water and lipid components into bilayers through a decrease in lipid droplet size and incorporation of dairy protein into the droplet interface. The decrease in milk fat globule size, essentially a disruption in the MFGM, which allows them to be dispersed uniformly through the milk product, has been proposed to result in improved digestibility and potentially health outcomes (125). The recent review by Mozaffarian et al. (22) noted that homogenization may destroy MFGM, and proposed that this may have implications for the cardiometabolic effects of dairy lipids. They cited an 8 weeks RCT which compared whipping cream (intact MFGM) with energy-, total dairy- and SFA-matched butter fat (homogenized, decreased MFGM) (67). Butter fat worsened both LDL-C and apolipoprotein B:A-I ratio, with no effects during whipping cream intervention.
Dairy and the Saturated Fat Controversy
The high saturated fatty acid (SFA) content of dairy and its myriad of food products has long raised concern (126), aligned with international guidelines (126, 127) to minimize dietary intake of animal-origin SFAs to achieve better cardiometabolic health. Early systematic reviews supported a relationship between dietary fatty acids and serum lipids, and also serum lipids and CV morbidity and mortality, as recently summarized by Hooper et al. (128). Mechanisms by which animal-origin SFAs may adversely affect circulating lipids include inhibiting LDL-receptor removal of lipoproteins from circulation, enhancing secretion of ApoB100-containing lipoproteins VLDL and LDL into circulating, and packing excess cholesterol in LDL particles; hence in combination increasing circulating LDL-C, TC and LDL-C:HDL-C ratio concentrations. In their Cochrane systematic review these authors in turn have shown a prolonged (2 years+) decrease in dietary SFA to be associated with a significant decrease in combined CV events, with some evidence of a dose-relationship (128). Replacing SFA with PUFA, but possibly not MUFA, and/or carbohydrate has been proposed as the most successful approach. However, over the past 10 years a consensus of studies has built which has questioned the assumption of adverse effects for dairy-origin SFAs for both CV and T2D outcomes. Notably, it is no longer adequate to consider nutrients in isolation, with evidence that the complex matrix of a food may be equally or more important than the fatty acid content and composition alone when predicting cardiometabolic risk (3, 10, 11). It has been proposed that in a complex dairy food such as cheese, for example, the effect of SFAs on blood lipids and disease risk may be counterbalanced by the content of protein, calcium, or other dietary components (23, 129). Aside growing evidence that dietary SFA-induced increases in LDL-cholesterol may not per se adversely affect cardiometabolic health (3).
Evidence that underpins the relationship between dairy fat and cardiometabolic outcomes to date exists predominantly from observational studies. This must be noted since association studies cannot determine cause and effect relationships, and are subject to confounding that may bias relationships between diet and disease outcomes. Human clinical trials investigating dairy interventions characteristically have been of short- or moderate- duration, with CV or T2D intermediary risk endpoints, and not disease incidence, evaluated. A summary of both is presented below.
Observational Studies
CVD: Contrary to prior concerns for high SFA animal-origin food groups (1), there is no consistent evidence from epidemiologic studies that a higher intake of dairy products is associated with increased CVD risk or incident CVD (3, 4, 6, 7, 20, 130). Key systematic reviews, meta-analyses, umbrella and narrative reviews are presented in Table 1a. In 2014 Astrup summarized observational studies which reported that the consumption of milk or dairy products was in fact inversely related to incidence of CVD (117), an outcome supported in a recent state of the art review (3). Inclusion of dairy products in the diet may ameliorate characteristics of adverse metabolic health including dyslipidemia, insulin resistance, hypertension, abdominal obesity, and the metabolic syndrome cluster (137), which together markedly increase the risk of both T2D and CVD. This had been proposed previously in meta-analyses of prospective cohort studies that identified an inverse association between milk and other dairy components and CV endpoints (4, 5, 116), with Elwood et al. originally noting a mismatch between this evidence and perceptions of harm from consumption of dairy (4). In 2015 a meta-analysis of prospective cohort studies provided further evidence supporting the beneficial effect of dairy consumption on CVD. Low-fat dairy products and cheese may protect against stroke or CHD incidence (6), in particular yogurt (134). A 2016 meta-analysis of prospective cohort studies again showed that dairy consumption may be associated with reduced risks of CVD, albeit with the authors noting that additional data are needed to more comprehensively examine potential dose-response patterns (7). It is now apparent that most meta-analyses report no association or even a weak inverse association between intake of dairy products and CVD endpoints (8, 20, 131, 133, 135, 136), or risk biomarkers including serum lipoprotein LDL-cholesterol. Characterized as convincing probable evidence, a recent 2020 umbrella review by Godos et al., reported a decreased association between total dairy and CVD (9). Arguably there is more controversy with respect to butter fat studies (2, 132). The recent large multinational Prospective Urban Rural Epidemiology (PURE) study from 21 countries in five continents has since also reported similar findings, with dairy intake associated with lower risk of mortality and major CVD events (130). However, even in light of the absence of a positive adverse association between dairy and CVD, there is some evidence that replacing dairy fat with PUFAs, especially from plant-origin foods, may be advantageous and confer positive health benefits (20).
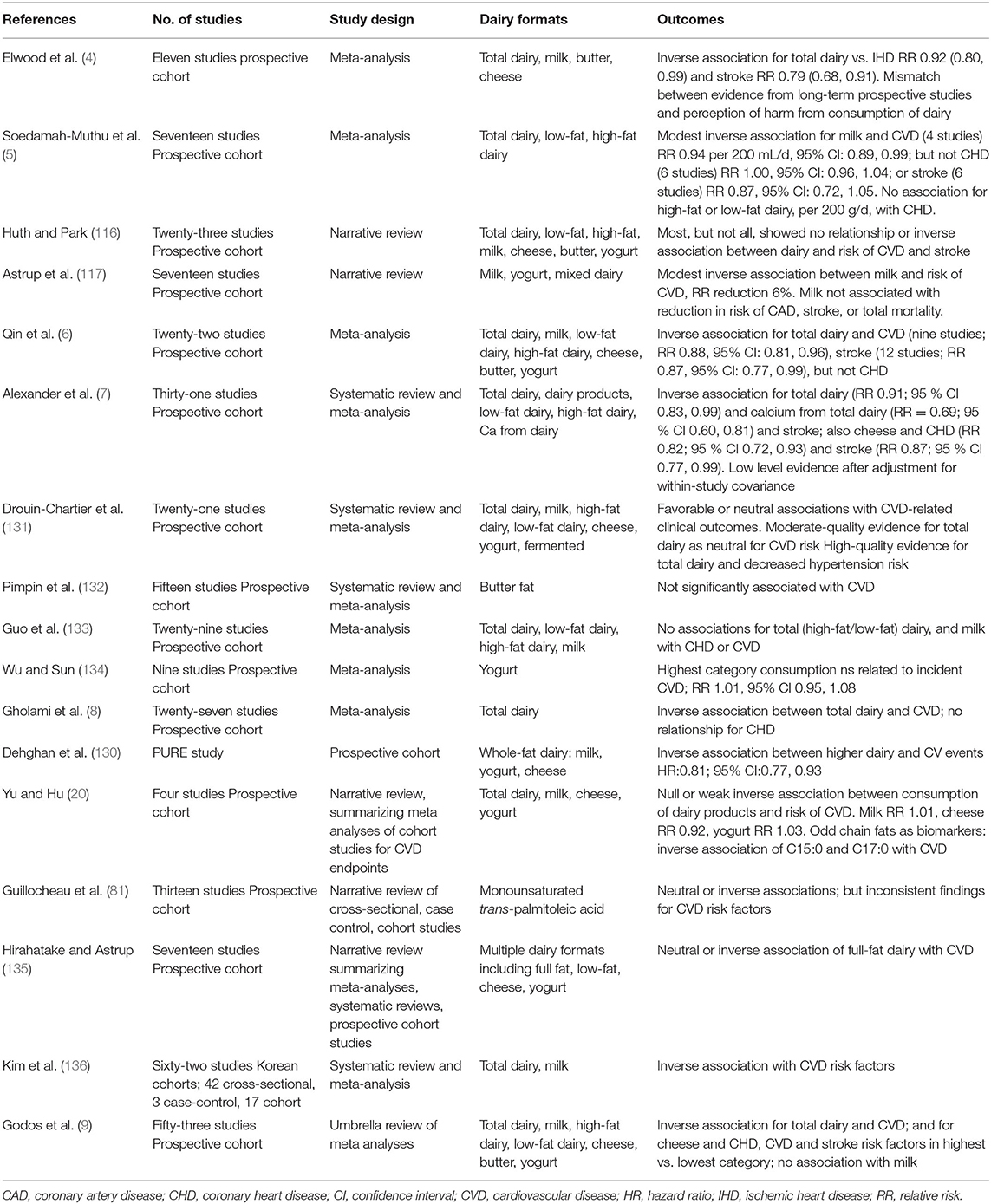
Table 1a. Summary of meta analyses, systematic reviews and narrative reviews reporting CVD outcomes: observational studies.
T2D
Whilst prospective cohort studies have commonly shown a range of animal products, for example red and processed meats, to be associated with increased risk of T2D (138), conversely numerous meta-analyses and systematic reviews report dairy food consumption to be linked to neutral or even decreased T2D risk (see Table 1b). These reviews utilize data from adult populations globally, reporting greatest effects with yogurt and cheese (19), in turn representative of both low-fat and high-fat dairy, respectively. The early meta-analysis of observational cohort studies showed higher total dairy also to be associated with a reduction in T2D risk (4), as did another small meta-analysis of 7 cohort studies (12). In 2013 Aune et al. published a meta-analysis of 17 prospective cohort studies, reporting that a higher consumption of total dairy products and cheese, as well as low-fat dairy, was associated with a lower risk for T2D (13). Further, investigation of dose-response showed a 7% decrease in T2D risk for every 400 g total dairy food consumed per day, which was estimated to be equivalent to ~1.7 servings of liquid milk per day (13). A second meta-analysis of 14 prospective cohort studies confirmed a similar 6% decrease in T2D risk for every 200 g of total dairy per day, plus in turn a 12% decrease for every 200 g low-fat dairy per day (14). This increased further to a 20% lower risk in T2D for every 30 g of cheese, and a 9% lower risk associated with consuming 50 g per day of fermented yogurt showing an inverse association between dairy intake and risk of T2D. Gijsbers et al., meta-analysis also reported an inverse association between dairy intake and risk of T2D (16), as did Schwingshackl et al. (139) and Soedamah-Muthu et al. (140). An updated recent 2019 meta-analysis of 12 studies has also reported total dairy consumption to be associated with lower risk of T2D, with moderate effects of cheese and strong effects of yogurt and low-fat dairy (17), in supported by a 2020 review of 12 prospective cohort studies (9). Notably however, in a combined analysis of men and women from the US Health Professionals Follow-Up Study and the US Nurses' Health Study I and II, no relationships were observed with total dairy, low-fat dairy or high-fat dairy, with only yogurt intake associated with a decreased risk of T2D (15). Also contrary to earlier findings of a significant association with total dairy in these large US cohorts. The authors proposed the longer follow up (+10 years) may account for this change in outcome, in turn observed in a meta-analysis of prior cohort studies published by their research team where benefits of dairy intake were diminished in long follow up studies (15). Pimpin et al. reported an inverse association with incidence of T2D in their more recent meta-analysis of butter fat (132).
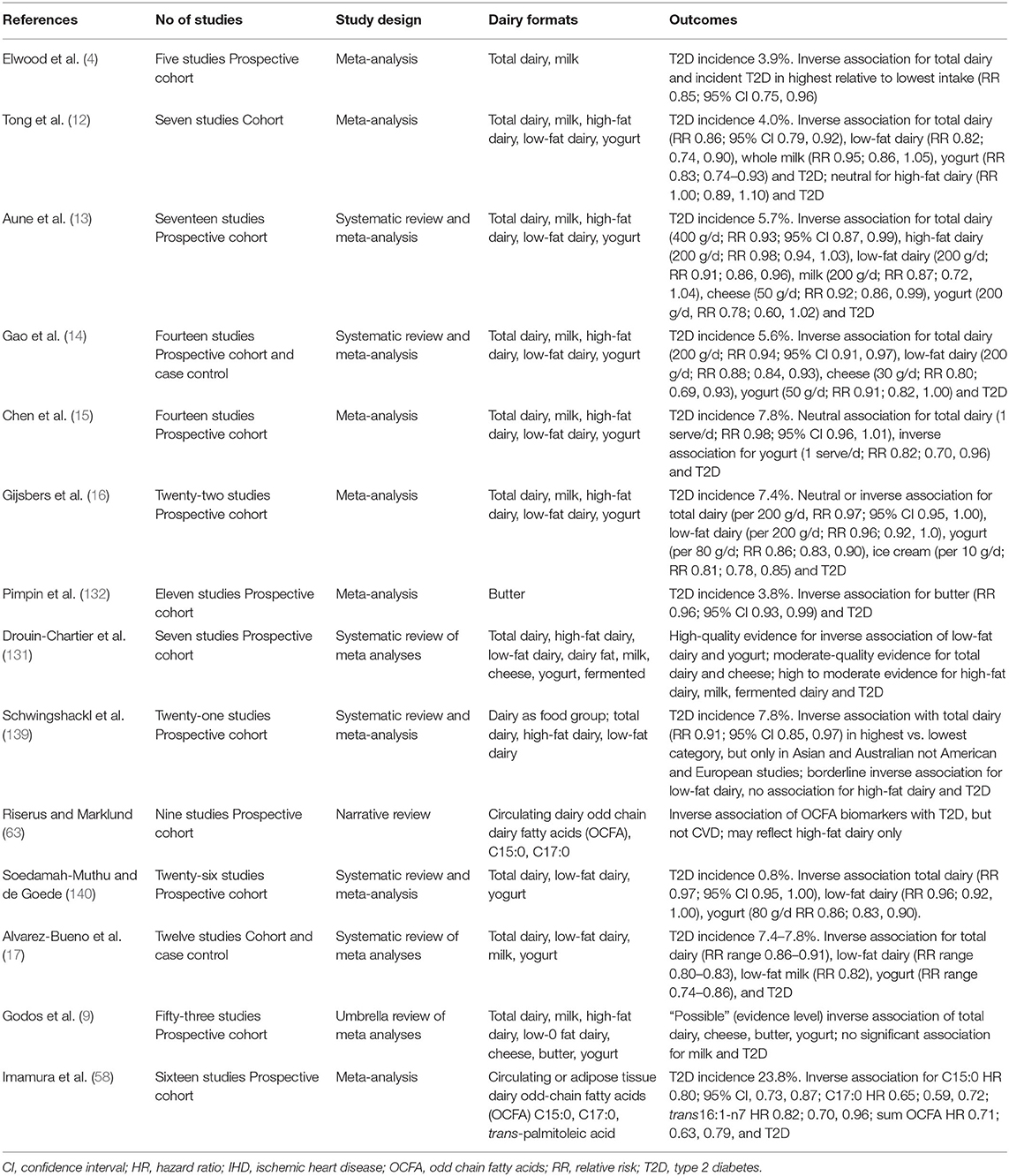
Table 1b. Summary of meta analyses, systematic reviews and narrative reviews reporting T2D outcomes: observational studies.
Other individual large prospective cohort studies in the US support a neutral, not adverse, relationship between total dairy and risk of T2D including postmenopausal women from the Women's Health Initiative Observational Study. Whilst higher dairy food consumption was associated with 40–50% reduced risk, it was notable that these large effects were related to low-fat dairy and yogurt intake (141). Prospective cohort studies conducted outside the US have also found beneficial or neutral effects of total dairy food consumption, as well as for specific dairy food groups which include high-fat dairy and cheese in addition to low-fat dairy or yogurt, on T2D risk in Britain, Europe, Japan and Australia (142–149). This was confirmed in a recent expert panel position paper (18), however, it remains important to note that the possibility of confounding is an aspect of observational studies which requires their review alongside well-designed and conducted RCTs in order to draw balanced consensus.
Overall, there is an extensive body of data from large observational trials of long duration, gathered over the last 20+ years, that support the association between total dairy intake and decreased incidence of CVD and/or T2D. Meta-analysis of prospective cohort studies of CVD-related incidence supports the recent characterization of this evidence as “convincing probable.” Whilst it is not as yet clear as to dose-response patterns for dairy and CVD, there are strong associations that suggest different relationships with different dairy formats, specifically liquid milk, butter, cheese and yogurt. Similar observations are reported for T2D incidence. As noted above, confounding remains of concern if evidence is obtained solely from observational data. There is far less data available from human interventions trials from which consensus might be built on the relationships of CVD or T2D with total dairy or the dairy food groups milk, cheese, butter and yogurt. In particular there is an absence of dairy RCTs designed to evaluate endpoint CVD and T2D events, required in order to conclude that totality of evidence is convincing.
Randomized Controlled Trials (RCTs)
CVD
There is far less data available from RCTs from which consensus might be built on the relationships of CVD with total dairy or the dairy food groups milk, cheese, butter and yogurt (see Table 1c). Huth and Park (116) originally reported on short-term intervention studies and lipid biomarkers, where diets higher in SFA from whole milk and butter adversely increased LDL-C when substituted for carbohydrate or USFA. Adverse effects of high SFA vs high USFA (PUFA+MUFA) butter fat on lipid parameters of lean healthy men have previously been reported from our laboratory (150, 151). Meta-analysis (116) however also showed increased cardio-protective HDL-C, resulting in little or no worsening of the key CVD marker total cholesterol:HDL-C ratio. In addition cheese, when matched to the total and SFA content of butter, was shown to result in significant LDL-C lowering (116), confirming the outcomes from prior observational data. Other authors have since also questioned the role of dairy SFAs in CVD, with evidence that substitution of dairy SFA with (i) plant-derived n-6 PUFA vegetable oils (unless balanced with n-3 PUFA), and (ii) carbohydrate with high glycemic index (GI), may also worsen adverse cardiometabolic endpoints (117). Drouin-Chartier et al. more recently conducted an extensive umbrella review of data obtained from meta-analyses of RCTs, in addition to a large number individual RCTs. Whilst noting that these trials were conducted predominantly in healthy individuals, contrary to Huth and Park (116) they reported no adverse impact of high-SFA dairy consumption on multiple cardiometabolic variables, including various blood lipid fractions, blood pressure, inflammation and vascular function (126). The authors noted that their review narrative failed to address several potential confounders, including change in body weight, the multiple and varied control arms (both dairy and non-dairy dietary components) used in these dairy interventions, and the possibility of inadequate power in multiple trials where the CVD outcomes assessed were not primary end points. Nor did they evaluate potential publication bias. However, the conclusions drawn from this review, that there was no evidence from RCTs to support a detrimental effect of regular- or high-fat dairy on these many CVD risk factors, was in agreement with the observational trials discussed above.
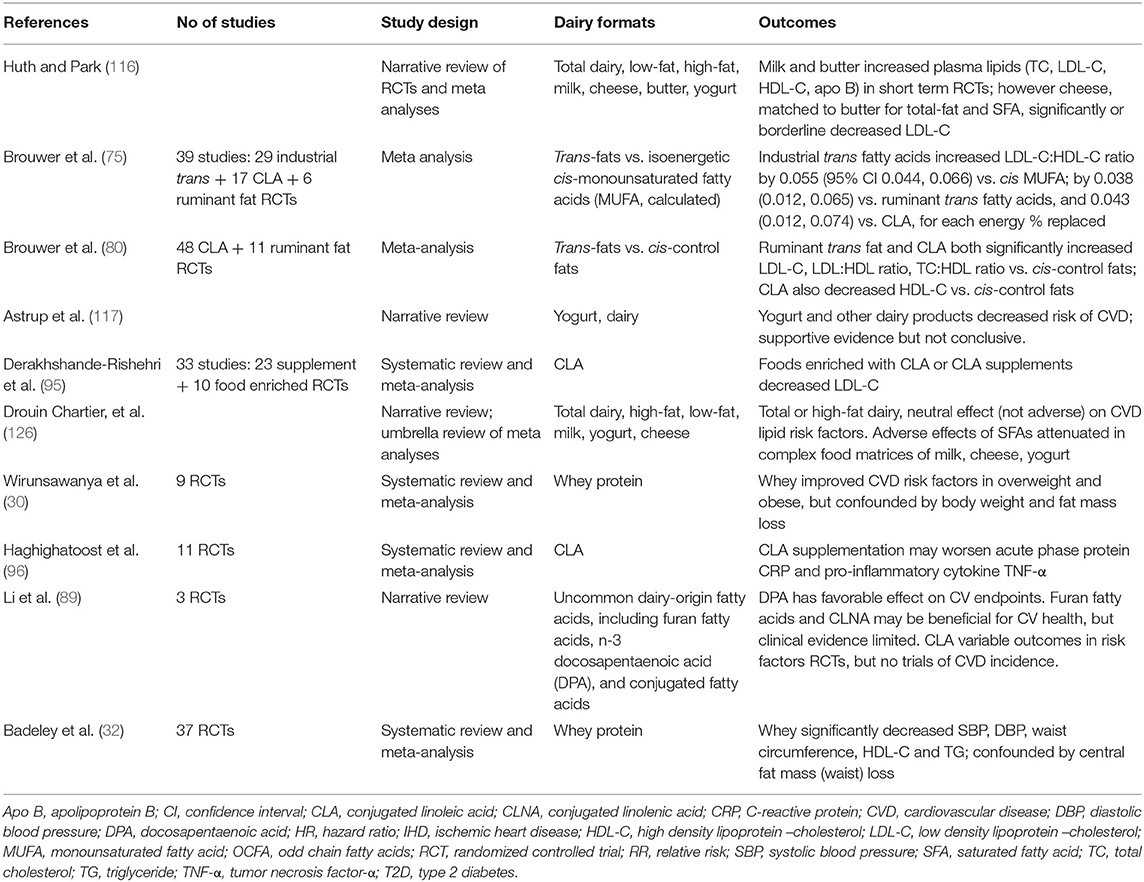
Table 1c. Summary of meta analyses, systematic reviews and narrative reviews reporting CVD outcomes: RCTs.
Table 1c also summarizes meta-analyses of RCTs investigating effects of various specific dairy components on CVD risk factors. Brouwer et al. (75, 80) have reported adverse effects of trans-fats in large meta-analyses of up to 48 industrial CLA and 11 ruminant trans-fat RCTs. Various authors have reported adverse (96), positive (87), and mixed (89) effects of CLA on CV endpoints. Whilst positive effects have been reported in several meta-analyses of whey protein RCTs (30, 32).
A major gap in the interpretation of this evidence is the absence of large, long-term dairy RCTs where CVD incidence is the primary outcome. To date, the majority of data reported has been from short-term interventions in healthy individuals rather than those as high risk, and has assessed accessible endpoints of CV risk. Only very few long-term interventions, such as the Finnish Dietary Prevention of Coronary Heart Disease studies for men and for women (152, 153), cluster-randomized 12 years intervention trials targeting decreased SFA through dairy replacement, have investigated CVD endpoints. These was conducted in two long-stay hospital wards where the majority of dairy fats were replaced by plant-origin n-6 PUFA fats for a 6 years duration, prior to crossover. Notably there was a significant decrease in serum cholesterol and incident CHD in men, smaller in women, when dairy was largely replaced by PUFA. The conundrum as to whether this decrease in clinical events was driven by decreasing SFA (dairy products replaced included whole milk and butter) or increasing PUFA (dairy substitutes of soybean oil in skimmed milk and high PUFA “soft” margarine) cannot be untangled. The critical nature of the comparator (or “control”) arm in the interpretation of such trials has recently been highlighted in the wider discussion concerning the role of dietary SFA in prevention of CVD (154). In a systematic review of large dietary interventions, analyzing the data sets on the basis of SFA being replaced by PUFA, or CHO or by ignoring the composition of the substitute, led to quite different positive (PUFA) or null (CHO, ignore) relationships between SFA and CVD. This clearly highlights the need to conduct long duration RCTs trials where the non-dairy control arm is carefully planned and evaluated.
T2D
Again, as noted previously there is little data available from long-term RCTs that directly attribute amelioration of T2D risk factors, or prevention of T2D, through dairy intake. Rideout et al. conducted a small sample size, cross-over study of 2 x 6 months duration which reported improved insulin sensitivity (155), although notably this was low-fat dairy. A second cross-over trial also of 2 × 6 months duration conversely reported no effect of a high vs. low dairy intake on glycemic endpoints (156). A more recent study in hyperinsulinaemic adults that compared 6 weeks supplementation of adequate (three servings/week) vs. high (three servings/week) dairy intake however did not observe any difference in glucose or insulin outcomes including insulin sensitivity, insulin secretion, and β-cell function (157). Table 1d presents a summary of systematic reviews, meta-analyses and narrative reviews. A systematic review of 10 RCTs of 1 week to 6 months duration, notably in weight stable individuals, failed to find conclusive evidence of improvement or detriment of dairy consumption on glycemic endpoints (158). Only 4 of 10 RCTs had a positive outcome on insulin sensitivity assessed as HOMA-IR. Pasin and Commerford reported on a meta-analysis of 28 dairy RCTs, with limited evidence of fermented dairy, probiotics and dairy proteins improving glycemic control (27). A more recent narrative review of 15 RCTs, of 2 weeks to 6 months duration, in individuals at risk of T2D concluded that whilst dairy does not have detrimental effects on glucose-related outcomes, positive outcomes are not universal (19). High dairy consumption was compared to a variety of no dairy and low dairy treatments. 10 of the studies showed no improvements with high dairy intake, whilst 5 studies showed improved HbA1c, plasma glucose, and insulin resistance (HOMA-IR). O'Connor et al. have also recently published a review of 38 RCTs, in a heterogeneous meta-analysis of children (>5 years) and adults without T2D, where glycemic endpoints were measured in trials comprising a higher vs. lower dairy arm comparison (159). Notably elevated dairy intake was associated with worsening FPG, but not fasting insulin or HbA1c, with the authors recording that the quality of evidence was low with high uncertainty. Sohol et al., have also recently conducted a meta-analysis of 30 RCTs (160) which reported that dairy, in particular low-fat dairy, decreased HOMA-IR assessed insulin resistance. The authors noted a series of limitations of their analysis including absence of discrimination between dairy food groups of milk, cheese, yogurt and components including dairy proteins, Ca and vitamin D, as well as missing data for HOMA-IR analyses in the original papers and absence of baseline glycemic status. They also noted the significant heterogeneity in the RCTs included in the meta-analysis. Table 1d also summarizes a number of other reviews of RCTs where effects of the individual dairy components CLA and whey protein have been assessed. RCTs investigating effects of CLA (88, 97) have reported no significant positive effects on glycemic endpoints, whilst whey protein interventions (30, 32) have caused a significant decreased in FPG.
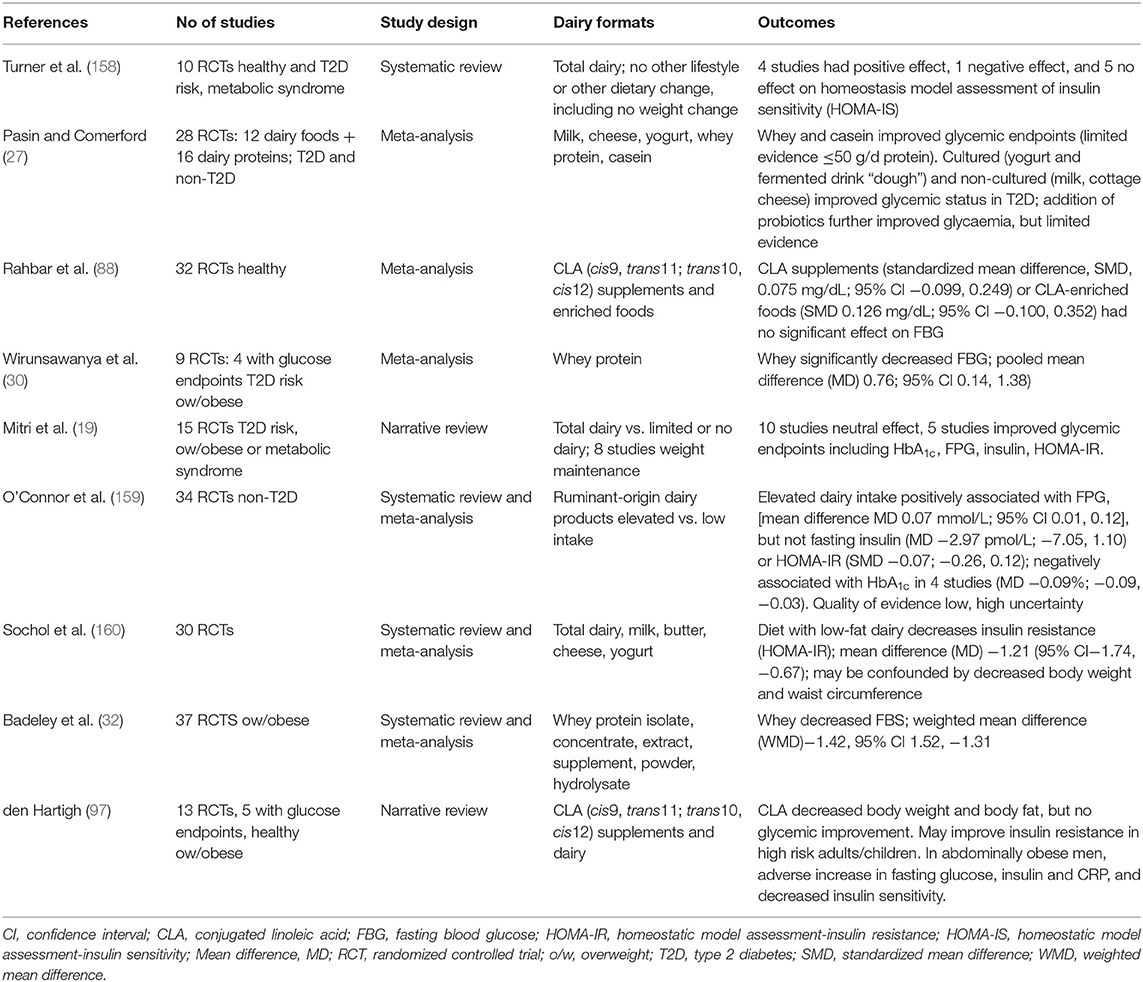
Table 1d. Summary of meta analyses, systematic reviews and narrative reviews reporting T2D outcomes: RCTs.
Again, a major omission in the interpretation of these RCTs is the fact that large, long-term interventions investigating effect of dairy on T2D incidence have not been conducted. To date, the majority of data reported has been from short-term interventions, of both healthy and impaired glucose tolerant individuals assessing commonly measured glycemic endpoints.
Protective Effects of Dairy Food Matrices
Yogurt, Cheese
As referred to in the sections above, the complex matrix of dairy has in recent years been proposed to significantly modulate the nutritional properties of these food products (10, 25, 26, 161), acting both on acute and chronic endpoints. In 2017, a primarily Europe-based, expert panel concluded that evidence from RCTs supported the proposal that intake of whole dairy foods vs. single dairy constituents resulted in different cardiometabolic effects (25), and that this may underpin the protective inverse relationships commonly reported for fermented dairy products, yogurt and cheese. They proposed that food structures and processing methods may enhance interactions between nutrients in the dairy matrix, such that the nutritional value of the product could not be directly related to the simple nutrient content, and introducing the concept of “biofunctionality” of nutrients within dairy food structures. An example of a recent intervention study in healthy adults, where high-fat meals with matched total fat content were delivered across a range of dairy product formats, and where quite different postprandial response of serum lipid and lipoprotein fractions was observed (161).
Cheese provides a clear example, where the predicted adverse effects of SFAs on cardiometabolic health are ameliorated when these FAs are consumed as part of this complex high-fat matrix (10, 126, 129). These outcomes were unexpected in early studies where high-SFA dairy fat when given as cheese failed to raise total- and LDL-cholesterol compared with low-SFA control diets (162) or compared with butter fat (163). Evidence has grown in support of cheese being a neutral food group that fits into a healthy diet (164). This has recently been supported in an expert panel position paper where food matrix was reported to be a stronger determinant of health effects than SFA content per se (18). Cheese is manufactured in a wide range of formats which in turn themselves vary considerably in terms of food matrix, with corresponding variability in physiological response. For example, a recent acute study of commercial cheeses showed a soft format “cream” cheese to induce a greater increase in postprandial triglyceridaemia than butter, whilst hard format cheddar cheese did not. Purported to be linked to variance in GI disintegration processes (26). A longer-term RCT showed that dairy fat consumed within a cheese matrix lowered lipid markers vs. dairy fat delivered within alternative matrices (10).
In addition to cheese, there is evidence also that yogurt does not exert the adverse effects on blood lipids predicted solely by content of SFA (116, 117). In turn, the expert panel noted that consumption of yogurt is associated with decreased risk of T2D and cardiometabolic disease (18), as discussed previously in this review. Whilst a number of factors may contribute including low-total fat content, fermentation process and probiotic content, food matrix has been proposed as an additional significant contributor.
The mechanisms proposed to underpin these effects of food matrix are varied. Dairy products are highly varied themselves, with a vast array of both structure and processing methods used in production. Cheese is a good example. Based on macronutrient content cheese is a high-fat dairy product, as is butter, yet based on structural composition it is more similar to yogurt or liquid milk with a high protein, high calcium and high MFGM content (25). Decreased gastrointestinal (GI) absorption of lipid from dairy products rich in calcium, phosphorus and MFGM has been shown to directly increase lipid losses from the GI tract and so in turn decrease circulating lipid levels including chylomicron (diet-origin) TGs (165). Calcium within the dairy matrix has also been implicated in the suppression of LDL-cholesterol observed during chronic consumption of high fat cheese (25). Calcium phosphate has long been known to increase excretion of fecal bile acids, a mechanism by which LDL-cholesterol is suppressed since bile acids are synthesized from hepatic cholesterol and represent the primary pathway for cholesterol catabolism. It is less clear whether MFGM alone also affects fecal fat excretion, with mechanisms underpinning improvement in lipoprotein profile observed when, for example high-MFGM butter fat is consumed, being decreased GI cholesterol absorption and/or the regulation of expression of genes (25). Fermented dairy also has beneficial effects on blood lipids, hypothesized to be due to favorable effects on gut microbiota resulting in favorable SCFA profile. It has been confirmed that both yogurt and cheese increase levels of fecal SCFAs, with cheese intake increasing fecal butyrate, propionate, and malonate and decreasing fecal acetate and glycerol concentrations (25). Furthermore, there were significant correlations between fecal propionate and butyrate levels and LDL-cholesterol concentrations.
Discussion
The contribution of dairy foods to cardiometabolic health has long been controversial. In particular, adverse effects that animal-origin dairy trans and SFAs may have on T2D and CVD outcomes. Consensus on the components of dairy in the diet has gradually been building, with some evidence of positive associations of whey protein and casein, milk fat globule membrane (MFGM) and polar phospholipids, vitamin D, and calcium with intermediary cardiometabolic endpoints. Conversely there is no consensus in support of the proposal that both trans and saturated dairy lipids have a consistent adverse effect on cardiometabolic health. Cohort studies report a neutral effect of trans-VA, but are not supported by RCT evidence that shows fatty acids containing trans double bonds to adversely alter serum cholesterol, irrespective of ruminant or industrial origin. In RCTs CLA both significantly improves and worsens CV risk factors but with little effect on glycaemia, and limited association with improved CV incidence reported in observational studies. For dairy SFA, evidence has been growing in support of no association or even a protective inverse relationship with cardiometabolic health, but largely driven by observational cohort studies. A clear research gap is the limited causal evidence of improvements in disease incidence obtained from intervention trials, with RCT evidence obtained predominantly from health individuals in trials of only short- or moderate-duration. In addition, it is proposed that the predicted adverse effects of these dairy lipids may be ameliorated when consumed within the complex food matrices of liquid milk, fermented cheese or yogurt.
In conducting this wide ranging review of the relationships between dairy and its myriad associated foods and components and cardiometabolic health, there are necessarily a number of limitations. Firstly this is a narrative and not systematic review, hence the ability to formally evaluate bias in its many forms in the large number of publications included both as individual studies or contributing to other narrative or systematic reviews is limited. The quality of the observational studies reviewed was highly variable, as were the design parameters of the RCTs. Study participants age, gender, anthropometric indices, health status and cardiometabolic risk factors also varied greatly between trials, both within and between within study type. It is not clear whether benefits are greatest in individuals identified as at risk of later disease. In RCTs sample size, composition of the comparator (“control”) arm, baseline dairy intake, dairy product and comparator dose, and duration of intervention are examples of important parameters that are not adjusted for in a narrative review.
Currently, there is little consensus by International dietary guidelines on the recommended fat content of dairy, and whether continued focus on consumption of low-fat dairy is warranted. The issue is complex, with both the quality and quantity of lipids contributed to the diet through dairy needing to be considered, with potential for higher fat products to promote a high energy density diet (166, 167) and body weight gain (168). Dietary diversity, i.e., “eat a variety of foods” has become a gradually accepted recommendation to promote a healthy diet and reduce risk of cardiometabolic disease, however recent evidence from cohort studies has shown this greater diversity to be associated with poor eating patterns and in turn weight gain and obesity. For example, a higher intake of processed foods, refined grains and also sugar sweetened beverages (SSBs), and a lower intake of unprocessed foods such as fish, fruits, and vegetables (169).
Table 2 presents the current dietary advice from both US and European medical associations for the prevention of CVD and T2D, where low-fat dairy is most commonly included. The American Heart Association (AHA) diet and lifestyle recommendations (170, 176), amongst others, continue to promote a healthy eating pattern that emphasizes low-fat dairy products alongside an adequate intake of plant foods including vegetable oils, nuts, whole grains, legumes, fish and poultry, and limited intake of sweets, SSBs and red meat. Conversely, the American Diabetes Association (ADA) annual guidelines continue to recommend a breadth of dairy products as part of a healthy diet, including milk and yogurt, for those with T2D (171), with no specific promotion of low-fat dairy. The European Society for Cardiology (ESC) guidelines (172) and the European Association for the Study of Diabetes (EASD) diabetes and nutrition study group (DNSG) (173) guidelines, expected to be updated in 2020 (177), both provide umbrella recommendations for SFAs to provide <10 en% and for low trans-fats. Alongside other healthy eating recommendations of consuming a diet high in vegetables, legumes, fruits and wholegrain cereals, EASD makes with no specific reference to dairy, whilst ESC recommends components of the Mediterranean Diet with minimal dairy for CVD prevention, and low-fat dairy for treatment of hypertension. A joint consensus report on management of hyperglycemia from ADA (174) and EASD (175) published concurrently in 2018 included diet and physical activity recommendations, with focus on medical nutrition therapy (MNT) of both energy restriction and optimal dietary quality including vegetables, legumes, fruits and whole grains, in addition to inclusion of dairy. This is part of a “no-one-size-fits-all” answer to diet and T2D by the ADA in their most recent 2019 Consensus Report (171).
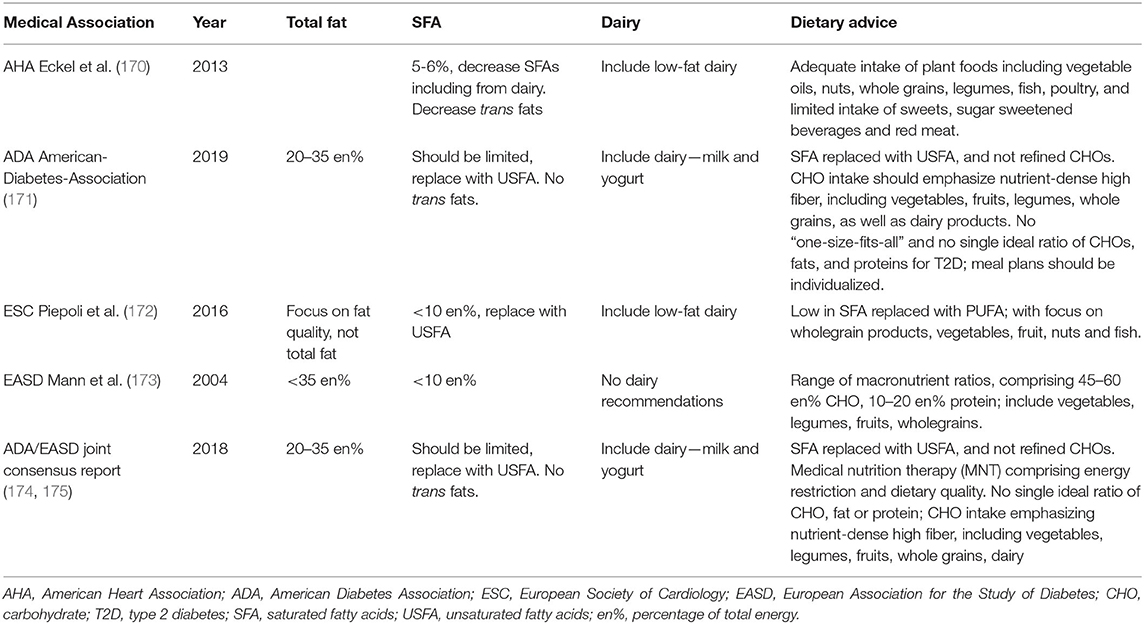
Table 2. Current dietary advice from both US and European medical associations for the prevention of CVD and T2D.
The contribution of bovine milk and its myriad of products to cardiometabolic health is an area of active and still developing research. Large cohort studies, systematic reviews and meta-analyses now point to a neutral or even a protective inverse relationship of dairy with CVD and T2D, but long-term RCTs are as yet lacking. Such long-term well-controlled studies are required in order to build the cause and effect evidence base that is necessary in order for consensus in this long controversial area of nutritional science to finally be reached.
Author Contributions
The author confirms being the sole contributor of this work and has approved it for publication.
Funding
SP received funding from the New Zealand Ministry for Business, Innovation and Employment (MBIE, grant no. 3710040) through the High Value Nutrition National Science Challenge programme, and the Riddet Center of Research Excellence (CoRE).
Conflict of Interest
SP declares that this manuscript was drafted in the absence of any commercial or financial relationships that could be construed as a potential conflict of interest. SP previously held the Fonterra Chair of Human Nutrition at the University of Auckland, New Zealand (2012-2018).
References
1. Hu F, Stampfer M, Manson J. Dietary saturated fats and their food sources in relation to the risk of coronary heart disease in women. Am J Clin Nutr. (1999) 70:1001–8. doi: 10.1093/ajcn/70.6.1001
2. Lovegrove J, Givens D. Dairy food products: good or bad for cardiometabolic disease? Nutr Res Rev. (2016) 29:249–67. doi: 10.1017/S0954422416000160
3. Astrup A, Magkos F, Bier DM, Brenna J, de Oliveira Otto TMC, Hill JO, et al. Saturated fats and health: a reassessment and proposal for food-based recommendations: JACC state-of-the-art review. J Am Coll Cardiol. (2020) 76:844–57. doi: 10.1016/j.jacc.2020.05.077
4. Elwood P, Pickering J, Givens D, Gallacher J. The consumption of milk and dairy foods and the incidence of vascular disease and diabetes: an overview of the evidence. Lipids. (2010) 45:925–39. doi: 10.1007/s11745-010-3412-5
5. Soedamah-Muthu SS, Ding EL, Al-Delaimy WK, Hu FB, Engberink MF, Willett WC, et al. Milk and dairy consumption and incidence of cardiovascular diseases and all-cause mortality: dose-response meta-analysis of prospective cohort studies. Am J Clin Nutr. (2011) 93:158–71. doi: 10.3945/ajcn.2010.29866
6. Qin L, Xu J, Han S, Zhang Z, Zhao Y, Szeto I. Dairy consumption and risk of cardiovascular disease: an updated meta-analysis of prospective cohort studies. Asia Pac J Clin Nutr. (2015) 24:90–100. doi: 10.6133/apjcn.2015.24.1.09
7. Alexander D, Bylsma L, Vargas A, Cohen S, Doucette A, Mohamed M, et al. Dairy consumption and CVD: a systematic review and meta-analysis. Br J Nutr. (2016) 115:737–50. doi: 10.1017/S0007114515005000
8. Gholami F, Khoramdad M, Esmailnasab N, Moradi G, Nouri B, Safiri S, et al. The effect of dairy consumption on the prevention of cardiovascular diseases: A meta-analysis of prospective studies. J Cardiovasc Thorac Res. (2017) 9:1–11. doi: 10.15171/jcvtr.2017.01
9. Godos J, Tieri M, Ghelfi F, Titta L, Marventano S, Lafranconi A, et al. Dairy foods and health: an umbrella review of observational studies. Int J Food Sci Nutr. (2020) 71:138–51. doi: 10.1080/09637486.2019.1625035
10. Feeney EL, Barron R, Dible V, Hamilton Z, Power Y, Tanner L, et al. Dairy matrix effects: response to consumption of dairy fat differs when eaten within the cheese matrix-a randomized controlled trial. Am J Clin Nutr. (2018) 108:667–74. doi: 10.1093/ajcn/nqy146
11. Hansson P, Holven K, Øyri L, Brekke H, Gjevestad G, Thoresen M, et al. Sex differences in postprandal responses to different dairy products on lipoprotein subclasses: a randomized controlled cross-over trial. Br J Nutr. (2019) 18:1–25. doi: 10.1017/S0007114519001429
12. Tong X, Dong J, Wu Z, Li W, Qin L. Dairy consumption and risk of type 2 diabetes mellitus: a meta-analysis of cohort studies. Eur J Clin Nutr. (2011) 65:1027–31. doi: 10.1038/ejcn.2011.62
13. Aune D, Norat T, Romundstad P, Vatten L. Dairy products and the risk of type 2 diabetes: a systematic review and dose-response meta-analysis of cohort studies. Am J Clin Nutr. (2013) 98:1066–8. doi: 10.3945/ajcn.113.059030
14. Gao D, Ning N, Wang C, Wang Y, Li Q, Meng Z, et al. Dairy products consumption and risk of type 2 diabetes: systematic review and dose-response meta-analysis. PLoS ONE. (2013) 8:e73965. doi: 10.1371/journal.pone.0073965
15. Chen M, Sun Q, Giovannucci E, Mozaffarian D, Manson J, Willett W, et al. Dairy consumption and risk of type 2 diabetes: 3 cohorts of US adults and an updated meta-analysis. BMC Med. (2014) 12: 215. doi: 10.1186/s12916-014-0215-1
16. Gijsbers L, Ding EL, Malik VS, de Goede J, Geleijnse JM, Soedamah-Muthu SS. Consumption of dairy foods and diabetes incidence: a dose-response meta-analysis of observational studies. Am J Clin Nutr. (2016) 103:1111–24. doi: 10.3945/ajcn.115.123216
17. Alvarez-Bueno C, Cavero-Redondo I, Martinez-Vizcaino V, Sotos-Prieto M, Ruiz J, Gil A. Effects of milk and dairy product consumption on type 2 diabetes: overview of systematic reviews and meta-analyses. Adv Nutr. (2019) 10:S154–63. doi: 10.1093/advances/nmy107
18. Guo J, Givens DI, Astrup A, Bakker SJ L, Goossens GH, Kratz M, et al. The impact of dairy products in the development of type 2 diabetes: where does the evidence stand in 2019? Adv Nutr. (2019) 10:1066–75. doi: 10.1093/advances/nmz050
19. Mitri J, Mohd Yusof BN, Maryniuk M, Schrager C, Hamdy O, Salsberg V. Dairy intake and type 2 diabetes risk factors: a narrative review. Diabetes Metab Syndr. (2019) 13:2879–87. doi: 10.1016/j.dsx.2019.07.064
20. Yu E, Hu F. Dairy products, dairy fatty acids, and the prevention of cardiometabolic disease: a review of recent evidence. Curr Atheroscler Rep. (2018) 20:24. doi: 10.1007/s11883-018-0724-z
21. Comerford K, Pasin G. Emerging evidence for the importance of dietary protein source on glucoregulatory markers and type 2 diabetes: different effects of dairy, meat, fish, egg, and plant protein foods. Nutrients. (2016) 8:446. doi: 10.3390/nu8080446
22. Mozaffarian D, Wu J. Flavonoids, dairy foods, and cardiovascular and metabolic health: a review of emerging biologic pathways. Circ Res. (2019) 122:369–84. doi: 10.1161/CIRCRESAHA.117.309008
23. Astrup A, Dyerberg J, Elwood P, Hermansen K, Hu F, Jakobsen M, et al. The role of reducing intakes of saturated fat in the prevention of cardiovascular disease: where does the evidence stand in 2010? Am J Clin Nutr. (2011) 93:684–8. doi: 10.3945/ajcn.110.004622
24. Mozaffarian D. Dietary and policy priorities for cardiovascular disease, diabetes, and obesity: a comprehensive review. Circulation. (2016) 133:187–225. doi: 10.1161/CIRCULATIONAHA.115.018585
25. Thorning TK, Bertram HC, Bonjour JP, de Groot L, Dupont D, Feeney E, et al. Whole dairy matrix or single nutrients in assessment of health effects: current evidence and knowledge gaps. Am J Clin Nutr. (2017) 105:1033–45. doi: 10.3945/ajcn.116.151548
26. Turgeon S, Brisson G. Symposium review: The dairy matrix: Bioaccessibility and bioavailability of nutrients and physiological effects. J Dairy Sci. (2019) 19:6727–36. doi: 10.3168/jds.2019-17308
27. Pasin G, Comerford K. Dairy foods and dairy proteins in the management of type 2 diabetes: a systematic review of the clinical evidence. Adv Nutr. (2015) 6:245–59. doi: 10.3945/an.114.007690
28. Poppitt SD. Milk proteins human health. In: Singh BM. Chapter18. Milk Proteins: from Expression to Food. London: Academic Press (2019). doi: 10.1016/B978-0-12-815251-5.00018-9
29. Berry S, Sheehy P, Williamson P, Sharp J, Menzies K, Lefevre C, et al. Defining the origin function of bovine milk proteins through genomics: The biological implications of manipulation modification. In: BM Singh, editor. Milk Proteins: From Expression to Food. London: Academic Press (2020). doi: 10.1016/B978-0-12-815251-5.00004-9
30. Wirunsawanya K, Upala S, Jaruvongvanich V, Sanguankeo A. Whey protein supplementation improves body composition and cardiovascular risk factors in overweight and obese patients: A systematic review and meta-analysis. J Am Coll Nutr. (2018) 37:60–70. doi: 10.1080/07315724.2017.1344591
31. Hodgson JM, Zhu K, Lewis J. R., Kerr D, Meng X, Solah V, et al. Long-term effects of a protein-enriched diet on blood pressure in older women. Br J Nutr. (2012) 107:1664–72. doi: 10.1017/S0007114511004740
32. Badely M, Sepandi M, Samadi M, Parastouei K, Taghdir M. The effect of whey protein on the components of metabolic syndrome in overweight and obese individuals; a systematic review and meta-analysis. Diabetes Metab Syndr. (2019) 13:3121–31. doi: 10.1016/j.dsx.2019.11.001
33. Bergia RE, Hudson JL, Campbell WW. Effect of whey protein supplementation on body composition changes in women: A systematic review and meta-analysis. Nutr Rev. (2018) 76:539–51. doi: 10.1093/nutrit/nuy017
34. Hruby A, Jacques PF. Dietary protein and changes in markers of cardiometabolic health across 20 years of follow-up in middle-aged Americans. Public Health Nutr. (2018) 21:2998–3010. doi: 10.1017/S1368980018001854
35. Rietman A, Schwarz J, Tom,é D, Kok FJ, Mensink M. High dietary protein intake, reducing or eliciting insulin resistance? Eur J Clin Nutr. (2014) 68:973–9. doi: 10.1038/ejcn.2014.123
36. Sluik D, Brouwer-Brolsma EM, Berendsen AA M, Mikkil,ä V, Poppitt SD, et al. Protein intake and the incidence of pre-diabetes and diabetes in 4 population-based studies: the PREVIEW project. Am J Clin Nutr. (2019) 109:1310–8. doi: 10.1093/ajcn/nqy388
37. Song Y, Manson JE, Buring JE, Liu S. A prospective study of red meat consumption and type 2 diabetes in middle-aged and elderly women: the women's health study. Diabetes Care. (2004) 27:2108–15. doi: 10.2337/diacare.27.9.2108
38. Adeva-Andany MM, Rañal-Muíño E, Vila-Altesor M, Fernández-Fernández C, Funcasta-Calderón R, Castro-Quintela E. Dietary habits contribute to define the risk of type 2 diabetes in humans. Clin Nutr ESPEN. (2019) 34:8–17. doi: 10.1016/j.clnesp.2019.08.002
39. Sluijs I, Beulens JWJDL, Van Der A, Spijkerman AMW, Grobbee DE, van der Schouw YT. Dietary intake of total, animal, and vegetable protein and risk of type 2 diabetes in the European Prospective Investigation into Cancer and Nutrition (EPIC)-NL study. Diabetes Care. (2010) 33:43–8. doi: 10.2337/dc09-1321
40. Fan M, Li Y, Wang C, Mao Z, Zhou W, Zhang L, et al. Dietary protein consumption and the risk of type 2 diabetes: A dose-response meta-analysis of prospective studies. Nutrient. (2011) 15: 2783. doi: 10.3390/nu11112783
41. Almario RU, Buchan WM, Rocke DM, Karakas SE. Glucose-lowering effect of whey protein depends upon clinical characteristics of patients with type 2 diabetes. BMJ Open Diabetes Res Care. (2017) 5:e000420. doi: 10.1136/bmjdrc-2017-000420
42. Karakas SE. www.clinicaltrials.gov. Bethesda (MD): National Library of Medicine (US); 2013. NCT01925248: Can whey protein improve glycemic control in type 2 diabetes? Available online at:http://clinicaltrials.gov/ct2/show/NCT01925248 (Accessed 4 Sept 2020).
43. Jakubowicz D, Wainstein J, Landau Z, Ahren B, Barnea M, Bar-Dayan Y, et al. High-energy breakfast based on whey protein reduces body weight, postprandial glycemia and HbA(1C) in Type 2 diabetes. J Nutr Biochem. (2017) 49:1–7. doi: 10.1016/j.jnutbio.2017.07.005
44. Liu AY, Silvestre MP, Poppitt SD. Prevention of type 2 diabetes through lifestyle modification: is there a role for higher-protein diets? Adv Nutr. (2015) 13:665–73. doi: 10.3945/an.115.008821
45. Fogelholm M, Larsen T, Westerterp-Plantenga M, Macdonald I, Martinez A, Boyadjieva N, et al. PREVIEW: PREVention of diabetes through lifestyle Intervention and population studies in Europe and around the World. Design, methods and baseline participant description of an adult cohort enrolled into a 3-year randomized clinical trial. Nutrients. (2017) 9:632. doi: 10.3390/nu9060632
46. Mignone LE, Wu T, Horowitz M, Rayner CK. Whey protein: The whey forward for treatment of type 2 diabetes?. World J Diabetes. (2015) 6:1274–84. doi: 10.4239/wjd.v6.i14.1274
47. Bloomgarden Z. Diabetes and branched-chain amino acids: What is the link? J Diabetes. (2018) 10:350–2. doi: 10.1111/1753-0407.12645
48. Chartrand D, Da Silva M, Julien P, Rudkowska I. Influence of amino acids in dairy products on glucose homeostasis: the clinical evidence. Can J Diabetes. (2017) 41:329–37. doi: 10.1016/j.jcjd.2016.10.009
49. Teng M, Zhao YJ, Khoo AL, Yeo TC, Yong QW, Lim BP. Impact of coconut oil consumption on cardiovascular health: a systematic review and meta-analysis. Nutr Rev. (2020) 78:249–59. doi: 10.1093/nutrit/nuz074
50. Poppitt SD, Strik CS, MacGibbon AK, McArdle BH, Budgett S, McGill AT. Fatty acid chain length, postprandial satiety and food intake in lean men. Physiol Behav. (2010) 101:161–7. doi: 10.1016/j.physbeh.2010.04.036
51. Turner N, Hariharan K, TidAng J, Frangioudakis G, Beale SM, Wright LE, et al. Enhancement of muscle mitochondrial oxidative capacity and alterations in insulin action are lipid species dependent: potent tissue-specific effects of medium-chain fatty acids. Diabetes. (2009) 58:2547–54. doi: 10.2337/db09-0784
52. Montgomery MK, Osborne B, Brown SH, Small L, Mitchell TW, Cooney GJ, et al. Contrasting metabolic effects of medium- versus long-chain fatty acids in skeletal muscle. J Lipid Res. (2013) 54:3322–33. doi: 10.1194/jlr.M040451
53. Chamma C. M., Bargut, T. CCA, Mandarim-de-Lacerda, and Aguila, M. B. A rich medium-chain triacylglycerol diet benefits adiposity but has adverse effects on the markers of hepatic lipogenesis and beta-oxidation. Food & Function. (2017) 8:778–87. doi: 10.1039/C6FO01663D
54. Matualatupauw JC, Bohl M, Gregersen S, Hermansen K, Afman LA. Dietary medium-chain saturated fatty acids induce gene expression of energy metabolism-related pathways in adipose tissue of abdominally obese subjects. Int J Obes. (2017) 41:1348–54. doi: 10.1038/ijo.2017.120
55. Amer B, Clausen MR, Bertram HC, Bohl M, Nebel C, Zheng H, et al. Consumption of whey in combination with dairy medium-chain fatty acids (MCFAs) may reduce lipid storage due to urinary loss of tricarboxylic acid cycle intermediates and increased rates of MCFAs oxidation. Mol Nutr Food Res. (2017) 61. doi: 10.1002/mnfr.201601048
56. Bohl M, Bjørnshave A, Larsen MK, Gregersen S, Hermansen K. The effects of proteins and medium-chain fatty acids from milk on body composition, insulin sensitivity and blood pressure in abdominally obese adults. Eur J Clin Nutr. (2017) 71:76–82. doi: 10.1038/ejcn.2016.207
57. Poppitt SD, Kilmartin P, Butler P, Keogh GF. Assessment of erythrocyte phospholipid fatty acid composition as a biomarker for dietary MUFA, PUFA or saturated fatty acid intake in a controlled cross-over intervention trial. Lipids Health Dis. (2005) 4:30. doi: 10.1186/1476-511X-4-30
58. Imamura F, Fretts A, Marklund M, Ardisson-Korat AV, Yang WS, Lankinen M, et al. Fatty acid biomarkers of dairy fat consumption and incidence of type 2 diabetes: A pooled analysis of prospective cohort studies. PLoS Med. (2011) 15:e1002670. doi: 10.1371/journal.pmed.1002670
59. Smedman AE, Gustafsson IB, Berglund LG, Vessby BO. Pentadecanoic acid in serum as a marker for intake of milk fat: relations between intake of milk fat and metabolic risk factors. Am J Clin Nutr. (1999) 69:22–9. doi: 10.1093/ajcn/69.1.22
60. Wolk A, Furuheim M, Vessby BO. Fatty acid composition of adipose tissue and serum lipids are valid biological markers of dairy fat intake in men. J Nutr. (2001) 131:828–33. doi: 10.1093/jn/131.3.828
61. Jenkins B, West J, Koulman A. A review of odd-chain fatty acid metabolism and the role of pentadecanoic acid (c15:0) and heptadecanoic acid (c17:0) in health and disease. Molecules. (2015) 20:2425–44. doi: 10.3390/molecules20022425
62. Pfeuffer M, Jaudszus A. Pentadecanoic and heptadecanoic acids: Multifaceted odd-chain fatty acids. Adv Nutr. (2016) 7:730–4. doi: 10.3945/an.115.011387
63. Risérus U, Marklund M. Milk fat biomarkers and cardiometabolic disease. Curr Opin Lipidol. (2017) 28:46–51. doi: 10.1097/MOL.0000000000000381
64. Venn-Watson S, Lumpkin R, Dennis EA. Efficacy of dietary odd-chain saturated fatty acid pentadecanoic acid parallels broad associated health benefits in humans: Could it be essential? Sci Rep. (2017) 10: 8161. doi: 10.1038/s41598-020-64960-y
65. Anto L, Warykas SW, Torres-Gonzalez M, Blesso CN. Milk polar lipids: underappreciated lipids with emerging health benefits. Nutrients. (2020) 12:E1001. doi: 10.3390/nu12041001
66. Reis MG, Roy NC, Bermingham EN, Ryan L, Bibiloni R, Young W, et al. Impact of dietary dairy polar lipids on lipid metabolism of mice fed a high-fat diet. JAgric Food Chem. (2013) 61:2729–38. doi: 10.1021/jf303795b
67. Rosqvist F, Smedman A, Lindmark-Månsson H, Paulsson M, Petrus P, Straniero S, et al. Potential role of milk fat globule membrane in modulating plasma lipoproteins, gene expression, and cholesterol metabolism in humans: a randomized study. Am J Clin Nutr. (2015) 102:20–30. doi: 10.3945/ajcn.115.107045
68. Vors C, Joumard-Cubizolles L, Lecomte M, Combe E, Ouchchane L, Drai J, et al. Milk polar lipids reduce lipid cardiovascular risk factors in overweight postmenopausal women: towards a gut sphingomyelin-cholesterol interplay. Gut. (2020) 69:487–501. doi: 10.1136/gutjnl-2018-318155
69. Nestel P, Straznicky N, Mellett N, Wong G, De Souza D, Tull D, et al. Specific plasma lipid classes and phospholipid fatty acids indicative of dairy food consumption associate with insulin sensitivity. Am J Clin Nutr. (2014) 99:46–53. doi: 10.3945/ajcn.113.071712
70. Zhou AL, Ward RE. Milk polar lipids modulate lipid metabolism, gut permeability, and systemic inflammation in high-fat-fed C57BL/6J ob/ob mice, a model of severe obesity. J Dairy Sci. (2019) 102:4816–31. doi: 10.3168/jds.2018-15949
71. Norris GH, Milard M, Michalski MC, Blesso CN. Protective properties of milk sphingomyelin against dysfunctional lipid metabolism, gut dysbiosis, and inflammation. J Nutr Biochem. (2019) 73:108224. doi: 10.1016/j.jnutbio.2019.108224
72. Liu Z, Li C, Pryce J, Rochfort S. Comprehensive characterization of bovine milk lipids: Phospholipids, sphingolipids, glycolipids and ceramides. J Agric Food Chem. (2020) 68:6726–38. doi: 10.1021/acs.jafc.0c01604
73. Cohn JS, Kamili A, Wat E, Chung R, Tandy, S. Dietary phospholipids and intestinal cholesterol absorption. Nutrients. (2010) 2:116–27. doi: 10.3390/nu2020116
74. Field CJ, Blewett HH, Proctor SD, Vine D. Human health benefits of vaccenic acid. Appl Physiol Nutr Metab. (2009) 34:979–91. doi: 10.1139/H09-079
75. Brouwer IA, Wanders AJ, Katan MB. Effect of animal and industrial trans fatty acids on HDL and LDL cholesterol levels in humans-a quantitative review. PLoS ONE. (2010) 5:e9434. doi: 10.1371/journal.pone.0009434
76. Gebauer SK, Destaillats F, Dionisi F, Krauss RM, Baer DJ. Vaccenic acid and trans fatty acid isomers from partially hydrogenated oil both adversely affect LDL cholesterol: a double-blind, randomized controlled trial. Am J Clin Nutr. (2015) 102:1339–46. doi: 10.3945/ajcn.115.116129
77. Wang X, Gupta J, Kerslake M, Rayat G, Proctor SD, Chan CB. Trans-11 vaccenic acid improves insulin secretion in models of type 2 diabetes in vivo and in vitro. Mol Nutr Food Res. (2016) 60:846–57. doi: 10.1002/mnfr.201500783
78. Wang Y, Lu J, Ruth MR, Goruk SD, Reaney MJ, Glimm DR, et al. Trans-11 vaccenic acid dietary supplementation induces hypolipidemic effects in JCR:LA-cp rats. J Nutr. (2008) 138:2117–22. doi: 10.3945/jn.108.091009
79. Jacome-Sosa MM, Borthwick F, Mangat R, Uwiera R, Reaney MJ, Shen J, et al. Diets enriched in trans-11 vaccenic acid alleviate ectopic lipid accumulation in a rat model of NAFLD and metabolic syndrome. J Nutr Biochem. (2014) 25:692–701. doi: 10.1016/j.jnutbio.2014.02.011
80. Brouwer IA, Wanders AJ, Katan MB. Trans fatty acids and cardiovascular health: research completed? Eur J Clin Nutr. (2013) 67:541–7. doi: 10.1038/ejcn.2013.43
81. Guillocheau E, Legrand P, Rioux V. Trans-palmitoleic acid (trans-9-C16:1, or trans-C16:1 n-7): Nutritional impacts, metabolism, origin, compositional data, analytical methods and chemical synthesis. A review Biochimie. (2020) 169:144–60. doi: 10.1016/j.biochi.2019.12.004
82. Jaudszus A, Kramer R, Pfeuffer M, Roth A, Jahreis G, Kuhnt K. Trans palmitoleic acid arises endogenously from dietary vaccenic acid. Am J Clin Nutr. (2014) 99:431–5. doi: 10.3945/ajcn.113.076117
83. Ratnayake WM. Concerns about the use of 15:0, 17:0, and trans-16:1n-7 as biomarkers of dairy fat intake in recent observational studies that suggest beneficial effects of dairy food on incidence of diabetes and stroke. Am J Clin Nutr. (2015) 101:1102–3. doi: 10.3945/ajcn.114.105379
84. Gama MAS, Filho H. G. B, Bizzo HR, Antoniassi R. Analytical shortcomings and other considerations related to the identification of biomarkers of dairy fat intake. Eur J Clin Nutr. (2017) 71:1022–3. doi: 10.1038/ejcn.2017.107
85. Mosley EE, Shafii B, Moate PJ, McGuire MA. cis-9, trans-11 conjugated linoleic acid is synthesized directly from vaccenic acid in lactating dairy cattle. J Nutr. (2006) 136:570–5. doi: 10.1093/jn/136.3.570
86. Koba K, Yanagita T. Health benefits of conjugated linoleic acid (CLA). Obes Res Clin Pract. (2014) 8:e525–32. doi: 10.1016/j.orcp.2013.10.001
87. Derakhshande-Rishehri SM, Mansourian M, Kelishadi R, Heidari-Beni M. Association of foods enriched in conjugated linoleic acid (CLA) and CLA supplements with lipid profile in human studies: a systematic review and meta-analysis. Public Health Nutr. (2015) 18:2041–54. doi: 10.1017/S1368980014002262
88. Rahbar AR, Ostovar A, Derakhshandeh-Rishehri SM, Janani L, Rahbar A. Effect of conjugated linoleic acid as a supplement or enrichment in foods on blood glucose and waist circumference in humans: A meta-analysis. Endocr Metab Immune Disord Drug Targets. (2017) 17:5–18. doi: 10.2174/1570161115999170207113803
89. Li K, Sinclair AJ, Zhao F, Li D. Uncommon fatty acids and cardiometabolic health. Nutrients. (2018) 10. doi: 10.3390/nu10101559
90. Smit LA, Baylin A, Campos H. Conjugated linoleic acid in adipose tissue and risk of myocardial infarction. Am J Clin Nutr. (2010) 92:30–4. doi: 10.3945/ajcn.2010.29524
91. Sluijs I, Plantinga Y, De Roos B, Mennen LI, Bots ML. Dietary supplementation with cis-9,trans-11 conjugated linoleic acid and aortic stiffness in overweight and obese adults. Am J Clin Nutr. (2010) 91:175–83. doi: 10.3945/ajcn.2009.28192
92. Raff M, Tholstrup T, Basu S, Nonboe P, Sorensen MT, Straarup EM. A diet rich in conjugated linoleic acid and butter increases lipid peroxidation but does not affect atherosclerotic, inflammatory, or diabetic risk markers in healthy young men. J Nutr. (2008) 138:509–51. doi: 10.1093/jn/138.3.509
93. Eftekhari M, Aliasghari F, Beigi MA, Hasanzadeh J. The effect of conjugated linoleic acids and omega-3 fatty acids supplementation on lipid profile in atherosclerosis. Adv Biomed Res. (2014) 3:15. doi: 10.4103/2277-9175.124644
94. Hassan Eftekhari M, Aliasghari F, Babaei-Beigi MA, Hasanzadeh J. Effect of conjugated linoleic acid and omega-3 fatty acid supplementation on inflammatory and oxidative stress markers in atherosclerotic patients. ARYA Atheroscler. (2013) 9:311–31.
95. Derakhshandeh-Rishehri SM, Rahbar AR, Ostovar A. Effects of conjugated linoleic acid intake in the form of dietary supplement or enriched food on C-reactive protein and lipoprotein (a) levels in humans: A literature review and meta-analysis. Iran J Med Sci. (2019) 44:359–73.
96. Haghighatdoost FB, Nobakht-M-Gh F. Effect of conjugated linoleic acid on blood inflammatory markers: a systematic review and meta-analysis on randomized controlled trials. Eur J Clin Nutr. (2018) 72:1071–82. doi: 10.1038/s41430-017-0048-z
97. den Hartigh LJ. Conjugated linoleic acid effects on cancer, obesity, and atherosclerosis: A review of pre-clinical and human trials with current perspectives. Nutrients. (2019) 11:370. doi: 10.3390/nu11020370
98. Itkonen ST, Erkkola M, Lamberg-Allardt CJ. Vitamin D fortification of fluid milk products and their contribution to vitamin D intake and vitamin D status in observational studies - A review. Nutrients. (2018) 10:1054. doi: 10.3390/nu10081054
99. Muñoz-Garach A, García-Fontana B, Muñoz-Torres M. Vitamin D status, calcium intake and risk of developing type 2 diabetes: An unresolved issue. Nutrients. (2019) 11:642. doi: 10.3390/nu11030642
100. Mitri J, Muraru MD, Pittas AG. Vitamin D and type 2 diabetes: A systematic review. Eur J Clin Nutr. (2011) 65:1005–15. doi: 10.1038/ejcn.2011.118
101. Song Y, Wang L, Pittas AG, Liana C. Blood 25-hydroxy vitamin D levels and incident type 2 diabetes. A meta-analysis of prospective studies. Diabetes Care. (2013) 36:1422–8. doi: 10.2337/dc12-0962
102. Afzal S, Bojesen SE, Nordestgaard BG. Low 25-hydroxyvitamin D and risk of type 2 diabetes: a prospective cohort study and metaanalysis. Clin Chem. (2013) 59:381–91. doi: 10.1373/clinchem.2012.193003
103. Carrelli ABM, Horst R, Cremers, S, Zhang, C, Bessler, M, Schrope, B, et al. Vitamin D storage in adipose tissue of obese and normal weight women. J Bone Miner Res. (2017) 32:237–42. doi: 10.1002/jbmr.2979
104. Bellou V, Belbasis L, Tzoulaki I, Evangelou E. Risk factors for type 2 diabetes mellitus: an exposure-wide umbrella review of meta-analyses. PLoS ONE. (2019) 13:e0194127. doi: 10.1371/journal.pone.0194127
105. Issa CM. Vitamin D and type 2 diabetes mellitus. Ultraviolet light in human health, diseases and environment. Ahmad, S, Springer. (2017) 996:193–205. doi: 10.1007/978-3-319-56017-5_16
106. Gasparri C, Perna S, Spadaccini D, Alalwan T, Girometta C, Infantino V, et al. Is vitamin D-fortified yogurt a value-added strategy for improving human health? A systematic review and meta-analysis of randomized trials. J Dairy Sci. (2019) 102:8587–603. doi: 10.3168/jds.2018-16046
107. Hu Z, Chen J, Sun X, Wang L, Wang A. Efficacy of vitamin D supplementation on glycemic control in type 2 diabetes patients: A meta-analysis of interventional studies. Medicine (Baltimore). (2019) 98: e14970. doi: 10.1097/MD.0000000000014970
108. George PS, Pearson ER, Witham MD. Effect of Vitamin D supplementation on glycaemic control and insulin resistance: a systematic review and meta-analysis. Diabet Med. (2012) 29:e142–150. doi: 10.1111/j.1464-5491.2012.03672.x
109. Bolland MJ, Grey A, Avenell A, Gamble GD, Reid IR. Calcium supplements with or without vitamin D and risk of cardiovascular events: reanalysis of the Women's Health Initiative limited access dataset and meta-analysis. BMJ. (2011) 342:d2040. doi: 10.1136/bmj.d2040
110. Mao PJ, Zhang C, Tang L, Xian YQ, Li YS, Wang WD, et al. Effect of calcium or vitamin D supplementation on vascular outcomes: a meta-analysis of randomized controlled trials. Int J Cardiol. (2013) 169:106–11. doi: 10.1016/j.ijcard.2013.08.055
111. Larsson SC, Burgess S, Michaelsson K. Association of genetic variants related to serum calcium levels with coronary artery disease and myocardial infarction. JAMA. (2017) 318:371–80. doi: 10.1001/jama.2017.8981
112. Reid IR, Bristow SM, Bolland MJ. Calcium supplements: benefits and risks. Intern Med. (2015) 278:354–68. doi: 10.1111/joim.12394
113. Reid IR, Bolland MJ. Calcium and/or Vitamin D supplementation for the prevention of fragility fractures: Who needs it?. Nutrients. (2020) 12:1011. doi: 10.3390/nu12041011
114. Kim KN, Oh SY, Hong YC. Associations of serum calcium levels and dietary calcium intake with incident type 2 diabetes over 10 Years: The Korean Genome and Epidemiology Study (KoGES). Diabetol Metab Syndr. (2018) 10:50. doi: 10.1186/s13098-018-0349-y
115. Hill D, Sugrue I, Arendt E, Hill C, Stanton C, Rossa RP. Recent advances in microbial fermentation for dairy and health. F1000Res. (2017) 6:751. doi: 10.12688/f1000research.10896.1
116. Huth P, Park K. Influence of dairy product and milk fat consumption on cardiovascular disease risk: a review of the evidence. Adv Nutr. (2012) 3:266–85. doi: 10.3945/an.112.002030
117. Astrup A. Yogurt and dairy product consumption to prevent cardiometabolic diseases: epidemiologic and experimental studies. Am J Clin Nutr. (2014) 99:1235S−42S. doi: 10.3945/ajcn.113.073015
118. Hansen TH, Gøbel RJ, Hansen T. The gut microbiome in cardio-metabolic health. Genome Med. (2015) 7: 33. doi: 10.1186/s13073-015-0157-z
119. Yadav H, Lee JH, Lloyd J, Walter P, Rane SG. Beneficial metabolic effects of a probiotic via butyrate-induced GLP-1 hormone secretion. J Biol Chem. (2013) 288:25088–97. doi: 10.1074/jbc.M113.452516
120. Bron PA, Kleerebezem M, Brummer RJ, Cani PD, Mercenier A, MacDonald TT, et al. Can probiotics modulate human disease by impacting intestinal barrier function? Br J Nutr. (2017) 117:93–107. doi: 10.1017/S0007114516004037
121. Bordalo Tonucci LKM, Olbrich Dos Santos CL, De Luces Fortes, Ferreira, Rocha Ribeiro SM, et al. Gut microbiota and probiotics: focus on diabetes mellitus. Crit Rev Food Sci Nutr. (2017) 57:2296–309. doi: 10.1080/10408398.2014.934438
122. Barengolts E. Gut microbiota, prebiotics, probiotics, and synbiotics in management of obesity and prediabetes: review of randomised controlled trials. Endocr Pract. (2016) 22:1224–34. doi: 10.4158/EP151157.RA
123. Barengolts E, Daviau Smith E, Reutrakul S, Tonucci L, Anothaisintawee T. The effect of probiotic yogurt on glycemic control in type 2 diabetes or obesity: A meta-analysis of nine randomized controlled trials. Nutrients. (2019) 11:671. doi: 10.3390/nu11030671
124. Companys J, Pla-Pag,à L, Calderón-Pérez L, Llaurad,ó E, Sol,à R, Pedret A, et al. Fermented dairy products, probiotic supplementation, and cardiometabolic diseases: A systematic review and meta-analysis. Adv Nutr. (2020) 11:834–63. doi: 10.1093/advances/nmaa030
125. Michalski MC. On the supposed influence of milk homogenization on the risk of CVD, diabetes and allergy. Br J Nutr. (2007) 97:598–610. doi: 10.1017/S0007114507657900
126. Drouin-Chartier J, Côté J, Labonté M, Brassard D, Tessier-Grenier M, Desroches S, et al. Comprehensive review of the impact of dairy foods and dairy fat on cardiometabolic risk. Adv Nutr. (2016) 7:1041–51. doi: 10.3945/an.115.011619
127. Dietary-Guidelines-Advisory-Committee. Scientific report of the 2015 Dietary Guidelines Advisory Committee. Washington: DC: 1689–1699: USDA and US Department of Health and Human Services. (2015).
128. Hooper L, Martin N, Jimoh OF, Kirk C, Foster E, Abdelhamid AS. Reduction in saturated fat intake for cardiovascular disease. Cochrane Database Syst Rev. (2020) 8. doi: 10.1002/14651858.CD011737.pub3
129. de Goede J, Geleijnse JM, Ding EL, Soedamah-Muthu SS. Effect of cheese consumption on blood lipids: A systematic review and meta-analysis of randomized controlled trials. Nutr Rev. (2015) 73:259–75. doi: 10.1093/nutrit/nuu060
130. Dehghan M, Mente A, Rangarajan S, Sheridan P, Mohan V, Iqbal R, et al. Association of dairy intake with cardiovascular disease and mortality in 21 countries from five continents (PURE): A prospective cohort study. Lancet. (2018) 392:2288–97. doi: 10.1016/S0140-6736(18)31812-9
131. Drouin-Chartier JP, Brassard D, Tessier-Grenier M, Côté JA, Labonté ME, Desroches S, et al. Systematic review of the association between dairy product consumption and risk of cardiovascular-related clinical outcomes. Adv Nutr. (2016) 7:1026–40. doi: 10.3945/an.115.011403
132. Pimpin L, Wu JH, Haskelberg H, DelGobbo L, Mozaffarian D. Is butter back? A systematic review and meta-analysis of butter consumption and risk of cardiovascular disease, diabetes, and total mortality. PLoS ONE. (2016) 11:e0158118. doi: 10.1371/journal.pone.0158118
133. Guo J, Astrup A, Lovegrove JA, Gijsbers L, Givens DI, Soedamah-Muthu SS. Milk and dairy consumption and risk of cardiovascular diseases and all-cause mortality: dose-response metaanalysis of prospective cohort studies. Eur J Epidemiol. (2017) 32:269–87. doi: 10.1007/s10654-017-0243-1
134. Wu L, Sun D. Consumption of yogurt and the incident risk of cardiovascular disease: A meta-analysis of nine cohort studies. Nutrients. (2017) 9: 315. doi: 10.3390/nu9030315
135. Hirahatake KM, Astrup A, Hill JO, Slavin JL, Allison DB, Maki KC. Potential cardiometabolic health benefits of full-fat dairy: The evidence base. Adv Nutr. (2020) 11:533–47. doi: 10.1093/advances/nmz132
136. Kim J, Hoang T, Bu SY, Kim JM, Choi JH, Park E, et al. Associations of dietary intake with cardiovascular disease, blood pressure, and lipid profile in the Korean population: a systematic review and meta-analysis. J Lipid Atheroscler. (2020) 9:205–29. doi: 10.12997/jla.2020.9.1.205
137. Clifton P. Metabolic syndrome-role of dietary fat type and quantity. Nutrients. (2019) 11:1438. doi: 10.3390/nu11071438
138. Neuenschwander M, Ballon A, Weber KS, Norat T, Aune D, Schwingshackl L, et al. Role of diet in type 2 diabetes incidence: umbrella review of meta-analyses of prospective observational studies. BMJ. (2019) 366:l2368. doi: 10.1136/bmj.l2368
139. Schwingshackl L, Hoffmann G, Lampousi AM, Knüppel S, Iqbal K, Schwedhelm C, et al. Food groups and risk of type 2 diabetes mellitus: a systematic review and meta-analysis of prospective studies. Eur J Epidemiol. (2017) 32:363–75. doi: 10.1007/s10654-017-0246-y
140. Soedamah-Muthu SS, de Goede J. Dairy consumption and cardiometabolic diseases: systematic review and updated meta-analyses of prospective cohort studies. Curr Nutr Rep. (2018) 7:171–82. doi: 10.1007/s13668-018-0253-y
141. Margolis K, Wei F, de Boer I, Howard B, Liu S, Manson J, et al. A diet high in low-fat dairy products lowers diabetes risk in postmenopausal women. J Nutr. (2011) 141:1969–74. doi: 10.3945/jn.111.143339
142. Sluijs I, Forouhi N, Beulens JY, van der Schouw Agnoli C, Arriola L, Balkau B. The amount and type of dairy product intake and incident type 2 diabetes: results from the EPIC-InterAct Study. Am J Clin Nutr. (2012) 96:382–90. doi: 10.3945/ajcn.111.021907
143. Kirii K, Mizoue T, Iso H, Takahashi Y, Kato K, Inoue M, et al. Calcium, vitamin D and dairy intake in relation to type 2 diabetes risk in a Japanese cohort. Diabetologia. (2009) 52:2542–50. doi: 10.1007/s00125-009-1554-x
144. Fumeron F, Lamri A, Abi Khalil C, Jaziri R, Porchay-Balderelli I, Lantieri O, et al. Dairy consumption and the incidence of hyperglycemia and the metabolic syndrome: results from a french prospective study, Data from the Epidemiological Study on the Insulin Resistance Syndrome (DESIR). Diabetes Care. (2011) 34:813–7. doi: 10.2337/dc10-1772
145. Grantham N, Magliano D, Hodge A, Jowett J, Meikle P, Shaw J. The association between dairy food intake and the incidence of diabetes in Australia: the Australian Diabetes Obesity and Lifestyle Study (AusDiab). Public Health Nutr. (2013) 16:339–45. doi: 10.1017/S1368980012001310
146. Louie J, Flood V, Rangan A, Burlutsky G, Gill T, Gopinath B, et al. Higher regular fat dairy consumption is associated with lower incidence of metabolic syndrome but not type 2 diabetes. Nutr Metab Cardiovasc Dis. (2013) 23:816–21. doi: 10.1016/j.numecd.2012.08.004
147. Soedamah-Muthu S, Masset G, Verberne L, Geleijnse J, Brunner E. Consumption of dairy products and associations with incident diabetes, CHD and mortality in the Whitehall II study. Br J Nutr. (2013) 109:718–26. doi: 10.1017/S0007114512001845
148. Struijk E, Heraclides A, Witte D, Soedamah-Muthu S, Geleijnse J, Toft U, et al. Dairy product intake in relation to glucose regulation indices and risk of type 2 diabetes. Nutr Metab Cardiovasc Dis. (2013) 23:822–8. doi: 10.1016/j.numecd.2012.05.011
149. Ericson U, Hellstrand S, Brunkwall L. C.-, Schulz A, Sonestedt E, Wallström P, et al. Food sources of fat may clarify the inconsistent role of dietary fat intake for incidence of type 2 diabetes. Am J Clin Nutr. (2015) 101:1065–80. doi: 10.3945/ajcn.114.103010
150. Poppitt S, Keogh G, Mulvey T, McArdle B, MacGibbon A, Cooper G. Lipid-lowering effects of a modified butter-fat: a controlled intervention trial in healthy men. Eur J Clin Nutr. (2002) 56:64–71. doi: 10.1038/sj.ejcn.1601282
151. Poppitt S, Keogh G, Mulvey T, Phillips A, McArdle B, MacGibbon A, et al. Effect of moderate changes in dietary fatty acid profile on postprandial lipaemia, haemostatic and related CVD risk factors in healthy men. Eur J Clin Nutr. (2004) 58:819–27. doi: 10.1038/sj.ejcn.1601882
152. Turpeinen O, Karvonen M. J., Pekkarinen M, Miettinen M, Elosuo R, Paavilainen E. Dietary prevention of coronary heart disease: the Finnish mental hospital study. Int J Epidemiol. (1979) 8:99–118. doi: 10.1093/ije/8.2.99
153. Miettinen M, Turpeinen O, Karvonen MJ, Pekkarinen M, Paavilainen E, Elosuo R. Dietary prevention of coronary heart disease in women: the Finnish mental hospital study. Int J Epidemiol. (1983) 12:17–25. doi: 10.1093/ije/12.1.17
154. Lichtenstein A. Dietary fat and cardiovascular disease: ebb and flow over the last half century. Adv Nutr. (2019) 10:S332–9. doi: 10.1093/advances/nmz024
155. Rideout TC, Marinangeli CP, Martin H, Browne RW, Rempel CB. Consumption of low-fat dairy foods for 6 months improves insulin resistance without adversely affecting lipids or bodyweight in healthy adults: a randomized free-living cross-over study. Nutr J. (2013) 12:56. doi: 10.1186/1475-2891-12-56
156. Crichton GE, Howe PRC, Buckley JD, Coates AM, Murphy KJ. Dairy consumption and cardiometabolic health: outcomes of a 12-month crossover trial. Nutr Metab. (2012) 9:19 doi: 10.1186/1743-7075-9-19
157. O'Connor S, Julien P, Weisnagel SJ, Gagnon C, Rudkowska, I. Impact of a high intake of dairy product on insulin sensitivity in hyperinsulinemic adults: A crossover randomized controlled trial. Curr Dev Nutr. (2019) 24:nzz083. doi: 10.1093/cdn/nzz083
158. Turner KM, Keogh JB, Clifton PM. Dairy consumption and insulin sensitivity: a systematic review of short- and long-term intervention studies. Nutr Metab Cardiovasc Dis. (2015) 25:3–8. doi: 10.1016/j.numecd.2014.07.013
159. O'Connor S, Turcotte A. F., Gagnon C, Rudkowska I. Increased dairy product intake modifies plasma glucose concentrations and glycated hemoglobin: A systematic review and meta-analysis of randomized controlled trials. Adv Nutr. (2019) 10:262–79. doi: 10.1093/advances/nmy074
160. Sochol KM, Johns TS, Buttar RS, Randhawa L, Sanchez E, Gal M, et al. The effects of dairy intake on insulin resistance: a systematic review and meta-analysis of randomized clinical trials. Nutrients. (2019) 17:2237. doi: 10.3390/nu11092237
161. Hansson P, Holven K, Øyri L, Brekke H, Biong A, Gjevestad G, et al. Meals with similar fat content from different dairy products induce different postprandial triglyceride responses in healthy adults: A randomized controlled cross-over trial. J Nutr. (2019) 149:422–31. doi: 10.1093/jn/nxy291
162. Nestel P, Chronopulos A, Cehun M. Dairy fat in cheese raises LDL cholesterol less than that in butter in mildly hypercholesterolaemic subjects. Eur J Clin Nutr. (2005) 59:1059–63. doi: 10.1038/sj.ejcn.1602211
163. Hjerpsted J, Leedo E, Tholstrup T. Cheese intake in large amounts lowers LDL-cholesterol concentrations compared with butter intake of equal fat content. Am J Clin Nutr. (2011) 94:1479–84. doi: 10.3945/ajcn.111.022426
164. Dekker L, Vinke P, Riphagen I, Minović I, Eggersdorfer ME, van den Heuvel, Schurgers L, et al. Cheese and healthy diet: associations with incident cardio-metabolic diseases and all-cause mortality in the general population. Front Nutr. (2019) 6:185. doi: 10.3389/fnut.2019.00185
165. Soerensen KV, Thorning TK, Astrup A, Kristensen M, Lorenzen JK. Effect of dairy calcium from cheese and milk on fecal fat excretion, blood lipids, and appetite in young men. Am J Clin Nutr. (2014) 99:984–91. doi: 10.3945/ajcn.113.077735
166. Poppitt SD, Prentice AM. Energy density and its role in the control of food intake: evidence from metabolic and community studies. Appetite. (1996) 26:153–74. doi: 10.1006/appe.1996.0013
167. Hossein Rouhani M, Haghighatdoost F, Surkan PJ, Azadbakht L. Associations between dietary energy density and obesity: a systematic review and meta-analysis of observational studies. Nutrition. (2016) 32:1037–47. doi: 10.1016/j.nut.2016.03.017
168. Alonso A, Zozaya C, Vázquez Z, Martínez JA, Martínez-González MA. The effect of low-fat versus whole-fat dairy product intake on blood pressure and weight in young normotensive adults. J Hum Nutr Diet. (2009) 22:336–42. doi: 10.1111/j.1365-277X.2009.00967.x
169. de Oliveira Otto M, Anderson C, Dearborn J, Ferranti E, Mozaffarian D, Rao G, et al. Dietary diversity: implications for obesity prevention in adult populations: a science advisory from the American Heart Association. Circulation. (2018) 138:e160–168. doi: 10.1161/CIR.0000000000000595
170. Eckel RH, Jakicic JM, Ard J, deJesus DJM, Miller NH, Hubbard VS, et al. 2013 AHA/ACC guideline on lifestyle management to reduce cardiovascular risk: A report of the American College of Cardiology/American Heart Association task force on practice guidelines. Circulation. (2013) 129:S76–99. doi: 10.1161/01.cir.0000437740.48606.d1
171. American-Diabetes-Association. Lifestyle management: Standards of medical care in diabetes. Diabetes Care. (2019) 42:S46–60. doi: 10.2337/dc19-S005
172. Piepoli MF, Hoes AW, Agewall S, Albus C, Brotons C, Catapano AL, et al. 2016 European Guidelines on cardiovascular disease prevention in clinical practice. Eur Heart J. (2016) 37:2315–81. doi: 10.1093/eurheartj/ehw106
173. Mann JI, De Leeuw I, Hermansen K, Karamanos B, Karlstrom B, Katsilambros N, et al. Evidence-based nutritional approaches to the treatment and prevention of diabetes mellitus. Nutr Metab Cardiovasc Dis. (2004) 14:373–94. doi: 10.1016/S0939-4753(04)80028-0
174. Davies MJ, D'Alessio DA, Fradkin J, Kernan WN, Mathieu C, Mingrone G, et al. Management of hyperglycemia in type 2 diabetes, 2018. A consensus report by the American Diabetes Association (ADA) and the European Association for the Study of Diabetes (EASD). Diabetes Care. (2018) 41:2669–701. doi: 10.2337/dci18-0033
175. Davies MJ, D'Alessio DA, Fradkin J, Kernan WN, Mathieu C, Mingrone G, et al. Management of Hyperglycemia in Type 2 Diabetes, 2018. A Consensus Report by the American Diabetes Association (ADA) and the European Association for the Study of Diabetes (EASD). Diabetologia. (2018) 61:2461–98. doi: 10.1007/s00125-018-4729-5
176. van Horn L, Carson JS, Appel LJ, Burke LE, Economos C, Karmally W, et al. Recommended dietary pattern to achieve adherence to the American Heart Association/American College of Cardiology (AHA/ACC) guidelines: A scientific statement from the American Heart Association. Circulation. (2016) 134:e505–29. doi: 10.1161/CIR.0000000000000462
Keywords: fat, protein, dairy, CVD, diabetes, cardiometabolic
Citation: Poppitt SD (2020) Cow's Milk and Dairy Consumption: Is There Now Consensus for Cardiometabolic Health? Front. Nutr. 7:574725. doi: 10.3389/fnut.2020.574725
Received: 21 June 2020; Accepted: 02 November 2020;
Published: 08 December 2020.
Edited by:
David A. Sela, University of Massachusetts Amherst, United StatesReviewed by:
Claudia Vetrani, University of Naples Federico II, ItalyAnestis Dougkas, Institut Paul Bocuse, France
Copyright © 2020 Poppitt. This is an open-access article distributed under the terms of the Creative Commons Attribution License (CC BY). The use, distribution or reproduction in other forums is permitted, provided the original author(s) and the copyright owner(s) are credited and that the original publication in this journal is cited, in accordance with accepted academic practice. No use, distribution or reproduction is permitted which does not comply with these terms.
*Correspondence: Sally D. Poppitt, cy5wb3BwaXR0QGF1Y2tsYW5kLmFjLm56