- 1Retired, Freising, Germany
- 2Biotask AG, Esslingen am Neckar, Germany
- 3Department of Bioactive and Functional Food Chemistry, Institute of Applied Biosciences, Karlsruhe Institute of Technology (KIT), Karlsruhe, Germany
Wheat-based foods have been staple foods since about 10,000 years and constitute a major source of energy, dietary fiber, and micronutrients for the world population. The role of wheat in our diet, however, has recently been scrutinized by pseudoscientific books and media reports promoting the overall impression that wheat consumption makes people sick, stupid, fat, and addicted. Consequently, numerous consumers in Western countries have started to question their dietary habits related to wheat consumption and voluntarily decided to adopt a wheat-free diet without a medical diagnosis of any wheat-related disorder (WRD), such as celiac disease, wheat allergy, or non-celiac gluten sensitivity. The aim of this review is to achieve an objective judgment of the positive aspects of wheat consumption as well as adverse effects for individuals suffering from WRDs. The first part presents wheat constituents and their positive nutritional value, in particular, the consumption of products from whole-grain flours. The second part is focused on WRDs that affect predisposed individuals and can be treated with a gluten-free or -reduced diet. Based on all available scientific knowledge, wheat consumption is safe and healthy for the vast majority of people. There is no scientific evidence to support that the general population would benefit from a wheat-free diet.
Introduction
Wheat is one of the major crops grown worldwide with a production of 7.34 × 108 tons on an area of 2.14 × 106 km2, which is about the size of Greenland (1). Wheat-based foods have been staple foods since wheat was domesticated about 10,000 years ago, and they constitute a major source of macro- and micronutrients and energy (15–20% of the required intake) for the world population, especially in developing countries (2, 3). Many health benefits such as favorable weight management and reductions in the risks for cardiovascular diseases and type 2 diabetes have been shown to be associated with the consumption of wheat-based foods, especially made of whole grains (4–6). Moreover, many non-food products for daily use contain wheat constituents as valuable ingredients. As a result, the positive aspects of wheat were commonly unquestioned. On the other hand, wheat-based foods are known to cause wheat-related disorders (WRDs), such as celiac disease (CD), wheat allergy (WA), and non-celiac gluten sensitivity (NCGS) in predisposed individuals (7). In the last decade, wheat received an increasingly negative image due to a number of pseudoscientific books and press reports, which recommended the avoidance of wheat consumption for the general population, not only for those suffering from WRDs. As a consequence, increasing numbers of individuals in Western countries decided to adopt a gluten-/wheat-free diet even without clear indications of WRDs or medical advice. The percentages of individuals self-reporting a WRD among the general population were 15% in Australia (8), 13% in the United Kingdom (9), 10% in Brazil (10), 8% in Mexico (11), 6% in the Netherlands (12), and 3% in El Salvador (13). In light of this controversial debate, the aims of this review are to provide an objective judgment of the positive aspects of wheat consumption as well as adverse effects for individuals suffering from WRDs.
Origins and Importance of Wheat
Wheat plants are grasses belonging to the monocot family Poaceae. Cultivated wheat (Triticum spp.) consists of three species: diploid (genome AmAm) einkorn (T. monococcum ssp. monococcum), tetraploid (AABB) emmer (T. turgidum ssp. dicoccum) and durum wheat (T. turgidum ssp. durum), and hexaploid (AABBDD) common wheat (T. aestivum ssp. aestivum) and spelt (T. aestivum ssp. spelta) (14). Using recent advances in sequencing techniques, the International Wheat Genome Sequencing Consortium (IWGSC) recently published a detailed description of the total genome of common wheat (cultivar Chinese Spring) and enabled access to 107,891 gene sequences (15). Einkorn developed from wild einkorn 1 (T. monococcum ssp. boeticum) and the cultivation of einkorn started around 10,000 years ago in the Fertile Crescent. The hybridization of a different wild einkorn (T. monococcum ssp. urartu) with an Aegilops speltoides-related species (BB) resulted in wild emmer (T. turgidum ssp. dicoccoides), the ancestor for domesticated emmer. Some subspecies of wild emmer developed free-threshing (naked) grains, known as durum wheat. When durum wheat crossed with Aegilops tauschii (DD), naked hexaploid common wheat evolved. Spelt most likely emerged from hybridization between T. aestivum and T. dicoccum (16).
Common (bread) wheat makes up about 95% of all wheat cultivated globally, and most of the remaining 5% is durum (pasta) wheat. Despite its ability to grow in variable environmental conditions, the selection of qualified sites and soils for wheat cultivation as well as suitable varieties and optimal crop management are important factors to ensure high yields (17, 18). With an estimated additional two billion people on the planet by 2050, food production needs to be increased despite challenges arising from climate change (19, 20). Improved wheat plants, resistant to frost, heat, drought, and/or salty soils, may increase the grain yield and the area suitable for wheat production and thus help ensure food security.
Wheat grains are dry one-seeded fruits (caryopses), in which fruit and seed coats are tightly linked. The husk is fused to the fruit coat in the hulled species einkorn, emmer, and spelt, which means that the husk cannot be separated from the grain by threshing. The grains consist of five main compartments with different constituents and biological functions: Fruit coat (pericarp) (4–5% of grain weight) and seed coat (testa) (≈1%) are the outer layers and surround the whole grain. The inner tissues (endosperm) comprise the aleurone layer (6–9%) and the starchy endosperm (80–85%). The germ (3%) located at the dorsal side of the caryopsis is the embryo, which includes a storage cotyledon and the embryonic axis. Dry (moisture content <12.5%), cool (<10°C), and pest-free storage of wheat grains protects against crop failure by providing a buffer to ensure nutrition security worldwide.
The Smiling Face: Wheat Constituents and Their Health Benefits
The chemical composition of mature grains (water content ≈13%) varies in a relatively small range, although it is influenced by species, variety, and growing conditions. Carbohydrates, mainly present as starch (≈58%) and non-starch polysaccharides (NSP) (≈13%), are predominant, followed by proteins (≈11%), lipids (≈2%), and minerals (≈2%) (Table 1). Vitamins and phytochemicals occur in very small amounts (<0.1%) but are important due to their contribution to human health.
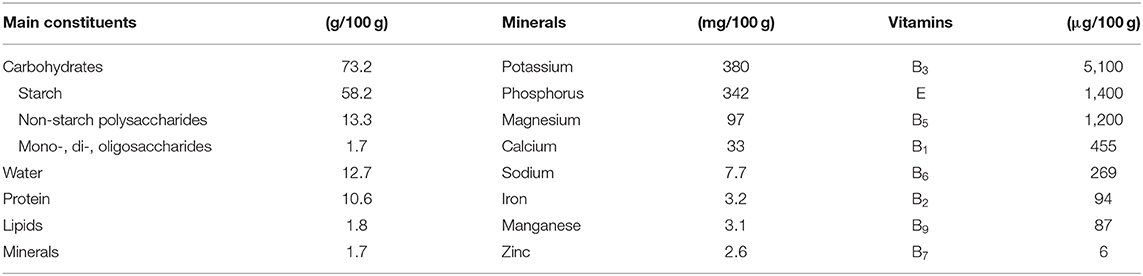
Table 1. Average contents of grain constituents of common wheat (21).
Mono-, Di-, and Oligosaccharides
Wheat grains contain only minor amounts (<0.1%) of the monosaccharides D-glucose and D-fructose, but about 0.5–1.6% of sucrose and 0.1–0.2% of maltose as disaccharides and 0.2–0.7% of the trisaccharide raffinose. The predominant oligosaccharides of wheat are 0.8–1.9% of fructans (22). Wheat fructans are of the graminan-type and comprise three or more fructose monomers linked via β-(2→1) and β-(2→6) glycosidic bonds and may also contain one glucose monomer. The degree of polymerization is 5–7 on average, but may also be below 5 or up to 17–19. Fructans are enriched in the bran and adhering endosperm (3–4%), but also present in the germ (≈2%) and endosperm (1–2%) (23). As part of fermentable oligo-, di-, and monosaccharides and polyols (FODMAPs), fructans and raffinose are metabolized by gut microbiota in the colon and may exert positive health effects similar to NSPs (see the Non-starch Polysaccharides section) by acting as prebiotics (24). In addition to their stimulatory effect on the composition and/or activity of beneficial gut bacteria, inulin-type fructans were shown to have direct immunomodulating and antioxidant protective effects on the gut mucosa (25, 26), but it is still unclear whether graminan-type fructans have the same effects as inulin-type fructans because studies with isolated cereal fructans are still needed (27). However, FODMAPs are also associated with intestinal complaints, as discussed below.
Starch
Starch is restricted to the endosperm, where it is present as large lenticular granules (A-starch) and small spherical granules (B-starch). The major carbohydrates of both granule types are the polysaccharides amylose and amylopectin in a mass ratio of about 25%/75%. Amylose consists of mainly linear α-(1→4)-linked D-glucopyranose units that form a helical structure and has molecular masses between 80,000 and 1 million. Apart from α-(1→4) glycosidic bonds, amylopectin has a branched structure with α-(1→6) bonds occurring every 24–30 glucose units and reaches molecular masses as high as 100 million (28). Starch is important for end-product quality and important for human nutrition. It constitutes the main source of energy (11.4 kJ/g of wholegrain wheat flour and 13.1 kJ/g of white wheat flour) because starch is readily degraded by amylases during human gastrointestinal digestion to its glucose monomers.
Resistant starch is the proportion of starch that escapes digestion in the upper gastrointestinal tract and small intestine (29), resulting in a caloric value of 8 kJ/g compared to 15 kJ/g for rapidly digestible starch. It is inaccessible to amylases depending on the size, shape, and crystallinity of the starch granule, the complexation of amylose with lipids, proteins, and phosphate as well as food processing (30, 31). Similar to NSP, resistant starch forms part of dietary fiber (DF) because it is fermented by microbiota in the colon to short-chain fatty acids. Especially butyrate promotes normal colon function by serving as a source of energy for the epithelial cells and by lowering luminal pH, which, in turn, facilitates the growth, and proliferation of beneficial gut microbiota (32, 33). Further, health benefits of resistant starch include improved insulin sensitivity (34), reduced oxidative stress in the colon (35), and lower body fat levels (36). Despite the different effects discussed before, the EU register on Nutrition and Health Claims contains only one authorized entry asserting the reduction of post-prandial glycemic responses for foods where resistant starch is at least 14% of total starch. Wheat whole grain flour contains about 3% of resistant starch and thus contributes to the estimated intake of 3–6 g/day in Europe (37). However, this is still far from the recommended intake of 20 g of resistant starch/day that is needed to achieve the positive health effects (38).
Non-starch Polysaccharides
NSPs include the cell wall polysaccharides arabinoxylans (AX, 5.5–7.4%), cellulose (1.7–3.0%), and β-glucans (0.5–1.0%) (22), but also minor contents of glucomannan, callose, xyloglucan, and pectins. Compared to amylose and amylopectin, NSPs are non-granular and belong to DF because human gastrointestinal enzymes are not able to cleave the predominant β-glycosidic linkages of the monosaccharide units. Depending on the wheat grain tissue, the composition of NSP varies between the endosperm, the bran, the aleurone, and the outer pericarp (39).
AX consist of a chain of β-(1→4)-linked D-xylopyranose residues carrying substitutions via (2→1)- and/or (3→1)-glycosidic bonds to α-L-arabinofuranose residues. Some arabinofuranose residues may additionally be linked to ferulic acid at C5, so that two adjacent AX chains may become crosslinked via diferulate following oxidation. The extent of diferulate crosslinking affects the solubility and viscosity of AX that can be subdivided into water-extractable (WE)-AX and water-unextractable (WU)-AX. Cellulose consists of linear β-(1→4)-linked D-glucopyranose units, whereas β-glucans are β-D-glucopyranose units linked via (1→3)- and (1→4)-glycosidic bonds. Compared to oats and barley, wheat β-glucans are poorly soluble. Wheat bran composition is significantly influenced by genotype × environment interactions, and the content of neutral detergent fiber ranged from 19 to 31%, that of acid detergent fiber from 5 to 10%, that of cellulose from 3 to 9% and that of hemicellulose from 14 to 21% (40).
All NSPs as well as lignin and fructans are summarized as DF. Wholegrain wheat flour contains 10.3–15.5% of total DF, whereas white flour only has 1.9–6.3% (41). Countless studies support the beneficial effects of wheat NSP on human health, including the comprehensive review by the UK Scientific Advisory Committee on Nutrition (SACN) (42). The most important effects include the regulation of colonic functions, protection against colonic cancer, normalization of serum lipid levels, and attenuation of post-prandial glucose response. A number of studies have reported the protective effects of wheat DF against colon, small intestinal, pancreatic, prostate, and breast cancer, with the effects on colorectal cancer being most evident (43–45). The influence of DF on the incidence of cardiovascular diseases has been the subject of many studies, and the relation between whole-grain intake and improved cardiovascular functions were clearly demonstrated with reduced risks for coronary events, stroke, elevated blood pressure, and hypertension (46). Many studies demonstrated that DF, enriched in whole grain products, has the ability to reduce insulin and glucose response significantly and, thus, reduce the occurrence of type 2 diabetes (47–49). Whether the consumption of DF-rich foods reduces appetite and contributes to weight control needs further clinical investigations (50). Currently, the EU Register on Nutrition and Health Claims contains two authorized entries for wheat bran fiber, one claiming reduced intestinal transit time and the other increased fecal bulk. Wheat AX is listed as suitable for the reduction of post-prandial glycemic responses. About 40 more entries related to DF were submitted, but not authorized, mostly due to the fact that the food constituent was not sufficiently characterized in relation to the claimed effects and a missing cause and effect relationship.
Proteins
Together with yield, grain protein content is of primary importance for wheat breeding because the content and composition of wheat proteins largely determine the bread making quality of wheat (51). Both protein content and composition are influenced by genetic and environmental factors, but the interaction between the two complicates the identification of molecular markers linked to these traits (15). Among environmental factors, nitrogen fertilization is the most prominent determinant linked to protein content and composition, but other factors such as soil fertility, precipitation, temperature, and altitude also play a role (52). The protein content of wheat may range from 7 to 22%, but mostly lies between 10 and 15% (53). The highest percentages of proteins within the grain are found in the germ (34%), followed by the aleurone (23%) and 5–6% in the outer layers. Consequently, the protein content of whole-grain flour is usually about 2% higher compared to white flour.
The protein fraction of wheat consists of over 100 individual proteins, which can be classified according to their functions: storage proteins, metabolic proteins, protective proteins, and miscellaneous proteins with further specific functions. Gluten proteins are storage proteins located in the endosperm (54), representing around 80% of total grain protein and can be grouped into monomeric gliadins soluble in aqueous alcohols and insoluble polymeric glutenins (55, 56). Gliadins have molecular masses between 30,000 and 55,000 and are structurally differentiated into four types: ω5-, ω1,2-, and α- and γ-gliadins. Glutenins are linked by interchain disulfide bonds and have molecular masses between 600,000 and more than 10 million. The respective monomers are classified into low-molecular-weight (LMW) and high-molecular-weight (HMW) glutenin subunits (GS) with molecular masses around 30,000 and 75,000, respectively. Metabolic proteins include enzymes like hydrolases, which cleave starch (amylases), proteins (peptidases), and lipids (lipases), as well as other oxidoreductases, transferases, and further enzymes (57). The majority of protective proteins are enzyme inhibitors that inhibit external amylase and peptidase activities, some of which are bifunctional like α-amylase/trypsin inhibitors (ATIs). The group of miscellaneous proteins includes, e.g., puroindolines, purothionins, and agglutinins (58, 59).
The nutritional value of wheat proteins is determined by their relative contents of the essential amino acids valine, leucine, isoleucine, phenylalanine/tyrosine, tryptophan, threonine, methionine/cysteine, lysine, and the semi-essential arginine and histidine (60). Lysine is the first limiting amino acid in wheat grains, whereas the other essential amino acids are present in adequate amounts (41). The biological value of white wheat flour is estimated to be 52 and that of whole-grain wheat flour is 17–26% higher (61). This difference is due to the fact that white flour contains higher proportions of gluten proteins compared to whole-grain flour, and the amino acid composition of gluten is characterized by exceptionally high contents of non-essential glutamine (26–53%) and proline (10–29%) (62).
Lipids
Wheat lipids constitute about 2–2.5% of the flour and can be classified into non-polar lipids (acylglycerols and free fatty acids) and polar lipids (phospholipids and glycolipids). The major components of non-polar lipids are triacylglycerols (≈40% of lipids) and free fatty acids (≈15%), while the percentages of mono- and diacylglycerols (≈1 and 4%, respectively) and sterol lipids (<1%) are low. Wheat phospholipids are composed of lysophosphatidylcholine (≈2%), phosphatidylcholine (≈1%), and <1% each of phosphatidylethanolamine, N-acylphosphatidylethanolamine, phosphatidylglycerol, and phosphatidyl inositol. Wheat glycolipids are comprised of digalactosyldiacylglycerol (DGDG) (≈15%), monogalactosyldiacylglycerol (MGDG) (≈4%), as well as DGMG and MGMG (about 1% each) (63, 64). Regarding nutritional benefits, wheat has high amounts of oleic acid (≈14%) as well as linoleic acid (≈60%) and linolenic acid (≈4%) as unsaturated fatty acids, and therefore a favorable ratio of unsaturated to saturated fatty acids of about 78%/22% (21). Wheat also contains phenolic lipids, known as alkylresorcinols (1,3-dihydroxybenzene derivatives with an odd-numbered alkyl chain at position 5 of the benzene ring) that may serve as markers of whole-grain cereals in food (65) and as biomarkers of whole-grain wheat intake (66). They have been reported to prevent colon cancer in mouse and in vitro models based on their antimutagenic and apoptotic activity (44, 45).
Vitamins and Minerals
Wheat grains are important sources of vitamin E (mainly α-tocopherol) and B vitamins, especially thiamine (B1), riboflavin (B2), niacin (B3), pantothenic acid (B5), pyridoxine (B6), and folates (B9) (Table 1). Whole-grain flours have considerably higher vitamin contents than white flours because vitamins are predominantly found in the bran and germ. One important point to consider related to nutrition is the bioavailability of vitamins. For example, most of the niacin present in wheat bran is bound, and only about 10–20% was found to be bioavailable (67).
The major minerals are potassium, phosphorus, magnesium, and calcium, followed by zinc, manganese, and iron in lower amounts (Table 1). Copper and selenium are trace minerals. All vitamins and minerals present in wheat have well-known functions in supporting growth and in maintaining the health and well-being of humans.
Phytochemicals
Wheat grains contain small amounts of phytochemicals that are defined as non-nutritive biologically active molecules that function in the human body to achieve health benefits, promote well-being, and prevent certain disease processes. The two major classes of phytochemicals found in wheat are phenolic compounds and terpenoids, derived from the shikimate and mevalonate or methylerythritol phosphate biosynthetic pathways, respectively (22, 68). Phenolic compounds are a structurally diverse group comprising phenolic acids (e.g., cinnamic acid and benzoic acid derivatives), flavonoids (e.g., flavanols and anthocyanins/anthocyanidins), and lignans. Terpenoids include sterols and stanols, with β-sitosterol as the primary compound in wheat, that may occur either as free form, esterified, glycosylated, or acylated and glycosylated. Other terpenoids are tocopherols and tocotrienols (α-tocopherol is commonly known as vitamin E) as well as carotenoids that can be subdivided into oxygen-containing xanthophylls (e.g., lutein and zeaxanthin) and oxygen-free carotenes (e.g., α- and β-carotene). Depending on their molecular structures, some carotenes may be converted to vitamin A in humans. With phytochemicals being mainly located in the aleurone and bran, they occur in the mg/kg range in whole-grain flours, but with a wide range of concentrations determined by natural genetic and environmental variations (69, 70).
Phenolic acids are known as strong antioxidants (71, 72), and there is evidence that phenolic compounds improve vascular functions in humans (73) and may have antitumor activity (44, 74). Considering the low overall concentrations of phytochemicals in whole-grain flours and absorbance rates of 5–10% in the human small intestine, direct antioxidant effects appear to be unlikely. However, the remaining 90–95% of phytochemicals are transferred to the colon where they are metabolized by microbiota and may exert positive effects on colon health through this route.
Wheat Constituents Related to Consumption
In summary, wheat grains contain low amounts of sugars, sodium, fat, saturated fatty acids, and are free of cholesterol, all of them considered to restrict health. As most components related to health are concentrated in the outer layers (bran) and germ of the grain, their contents are reduced in flours with low extraction rates (e.g., white flour), which are used to make the majority of wheat products (e.g., bread, pasta, and noodles) consumed in Western countries (Figure 1). Products made from white flour are usually preferred by consumers because the high bran content of wholegrain flour may give a dark color, bitterness, gritty texture, and short shelf life. Changing consumer preferences is difficult, and thus, whole-grain intake is still below daily recommendations in most countries. To improve the situation, research and development should be focused on combining innovative processing with better product quality to increase the utilization of whole-grain flours. Moreover, physicians, nutritionists, and the media should advocate the consumption of products made from whole grains (75).
Altogether, the consumption of wheat-based foods, especially whole-grain products, is highly recommended owing to excellent nutritional profiles and their importance as sources of energy, proteins, DF, B, and E vitamins, minerals, and different micronutrients, all contributors to a healthy diet. Depending on the average annual per capita consumption, wheat contributes different amounts of nutrients in relation to the overall diet. The average per capita consumption of wheat worldwide was 67.0 kg (2016–2018), but with considerable regional variations ranging from 50.4 kg in Africa to 109 kg in Europe, considering continents, but even ranging from 16.2 kg in Thailand to 209.7 kg in Turkey, considering countries (76). In Germany, wheat consumption contributes 23% of energy, 34% of digestible carbohydrates, 34% of protein, 24% of DF, and 20–30% of vitamins and 10–20% of minerals compared to the recommended average intake values (77). However, considering the wide span of per capita wheat consumption, these values will vary in the same wide range and the contribution of wheat to dietary nutrient intake needs to be considered individually for each country.
The Sad Face: Wheat-Related Disorders
Immune-mediated adverse reactions to wheat may occur in predisposed individuals. These hypersensitivities commonly referred to as wheat-related disorders (WRDs) can be classified into CD, gluten ataxia, and dermatitis herpetiformis characterized by an autoimmunogenic response (IgA and IgG antibodies), into IgE- and non-IgE-mediated WA and into NCGS characterized by an innate immune response (78) (Figure 2). A subgroup of patients with diarrhea-predominant irritable bowel syndrome may also be affected by wheat consumption.
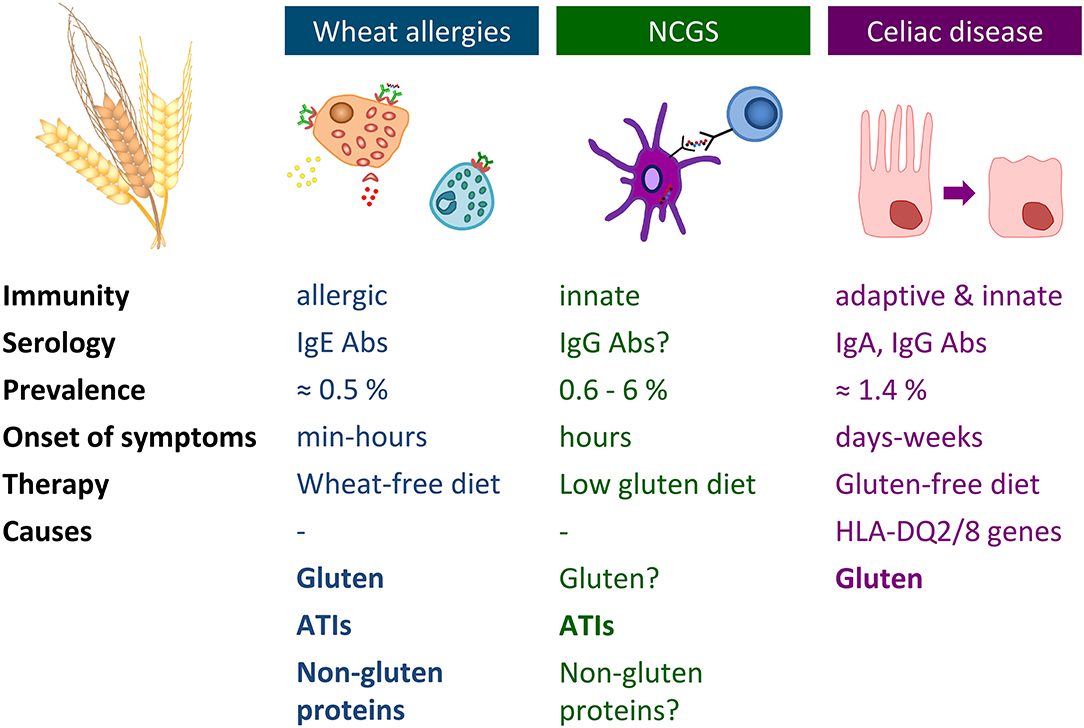
Figure 2. Overview of wheat allergy, non-celiac gluten sensitivity, and celiac disease. Abs, antibodies; ATIs, α-amylase/trypsin-inhibitors; HLA, human leukocyte antigen; Ig, immunoglobulin; NCGS, non-celiac gluten sensitivity.
Celiac Disease
Definition and Prevalence
CD belongs to the most common food-related lifelong disorders worldwide. It is defined as a chronic immune-mediated small intestinal enteropathy precipitated by exposure to dietary gluten in genetically predisposed individuals (7). The term “gluten” comprises the closely related storage proteins of wheat (gliadins and glutenins), rye (secalins), and barley (hordeins). No species or variety of these cereals is currently safe for patients with CD. Recent epidemiological data suggest a mean worldwide prevalence of 1.4% [1.1–1.7%] based on positive serology and of 0.7% [0.5–0.9%] based on biopsy-confirmed diagnosis (79). The serology-confirmed prevalence values were 1.1% in Africa, 1.3% in Europe and South America, 1.4% in North America and Oceania, and 1.8% in Asia. Only people from sub-Saharan Africa appear to be hardly affected. There are considerable regional differences in seroprevalence ranging as high as 2.1–8.5% in Algeria, Czech Republic, India, Israel, Mexico, Malaysia, Saudi Arabia, Sweden, Portugal, and Turkey, or as low as 0.2–0.8% in Estonia, Germany, Iceland, Libya, Poland, Spain, and Switzerland. CD can occur at any age, but the biopsy-confirmed prevalence was significantly greater in children (0.9%) than in adults (0.5%) and also higher in female (0.6%) compared to male (0.4%) individuals. The worldwide prevalence of CD increased from 0.6% [0.5–0.7%] (years 1991–2000) to 0.8% [0.5–1.0%] (years 2001–2016), but the reasons are still unknown, although environmental factors are most likely (80).
Causes
A combination of environmental and genetic factors is necessary to trigger CD in susceptible individuals. Consumption of gluten from wheat, rye, or barley is the decisive environmental factor necessary for CD onset. Although comparative data on the CD activity of single gluten proteins is unavailable, most findings suggest that all gluten proteins are relevant. The unique structural features of gluten proteins are long repetitive amino acid sequences rich in glutamine and proline (62). These sequences are resistant to human gastrointestinal digestive enzymes, so that high amounts of long gluten peptides reach the upper small intestinal mucosa, pass the epithelium, and arrive at the lamina propria, where CD-specific immune reactions are induced (81). Recent evidence suggests that non-gluten proteins, such as ATIs, may also be involved in fueling the CD-specific immune response (82, 83).
The genetic susceptibility to develop CD is associated with the major histocompatibility class II genes on chromosome 6 coding for human leukocyte antigens (HLA)-DQ2 and -DQ8. HLA-DQ proteins are heterodimeric receptors expressed on the surface of antigen-presenting cells (APCs) that are responsible for binding gluten peptides and presenting them to gluten-specific CD4+ T cells. Depending on genetic expression patterns, either the HLA-DQ2.5, -DQ8, or -DQ2.2 heterodimers are present and associated with a very high, high, or low predisposition for CD, respectively (84, 85). These different levels of CD risk are due to HLA-DQ2.5 being capable of binding a large repertoire of gluten peptides that are resistant to gastrointestinal degradation, whereas HLA-DQ8 and -DQ2.2 bind a small to very small selection of gluten peptides that are also less resistant to degradation (86). About 95–97% of CD patients are HLA-DQ2/8 positive, but this genetic predisposition is also present in about 30% of the healthy population. Thus, the absence of HLA-DQ2/8 is a reliable criterion to exclude CD, but its presence is not sufficient to cause CD. A variety of non-HLA genes, mostly encoding for T cells or APCs, has been associated with CD development, but each of these genes most likely only contributes a small percentage to increase the risk of CD (87). It is interesting to note that there is a significant correlation between the level of wheat consumption, the frequency of HLA-DQ2/8 and the prevalence of CD, but with several outlier populations in regions such as northwestern India, northern Africa, Mexico, Finland, and Russia. For example, the prevalence of CD in Algeria is among the highest worldwide (5.6%), whereas that of Tunisia is very low (0.3%), although both countries share similar levels of wheat and barley consumption and frequencies of HLA-DQ2/8 (84). This discrepancy can only be explained by further environmental factors that cause a loss of tolerance to dietary gluten and initiate CD. The most likely factors are infections (rotavirus, adenovirus 12), changes of intestinal microbiota, increased small intestinal permeability, and the so-called hygiene hypothesis that proposed a lower incidence of infections in early childhood as an explanation for the rise in immune-mediated hypersensitivities (88). Although childbirth (natural vs. cesarean section), duration of breastfeeding, and the time of gluten introduction into the child's diet have been discussed, the associations are far from clear, and the cumulative incidence of CD in later childhood was similar independent of breastfeeding or timing of gluten introduction (89, 90). Currently, there is no possibility to prevent CD (91).
Symptoms
The clinical appearance of CD is highly variable and can range from asymptomatic to full-blown symptoms due to the multisystemic nature of CD (92). Classical gastrointestinal symptoms are chronic diarrhea, abdominal pain, vomiting, and steatorrhea. Extraintestinal manifestations include chronic fatigue, night blindness, anemia, osteoporosis, thyroid dysfunction, and reproductive disease (93) and are usually caused by generalized malabsorption of essential nutrients, e.g., vitamins and minerals. Slow growth rates and delayed sexual maturation are known complications of CD in children and adolescents. The characteristic feature of CD is damage to the upper small intestine (duodenal bulb, duodenum, proximal jejunum) characterized by increased infiltration of intraepithelial lymphocytes (IELs), crypt hyperplasia, and partial to total villous atrophy. The degree of mucosal damage is classified according to Marsh–Oberhuber (into types 0, 1, 2, 3a–c, and 4) (94) or Corazza (into grades normal, A, B1, and B2) considering the numbers of IELs per 100 enterocytes, ratio of villous height to crypt depth, and degree of villous atrophy [overview in Ludvigsson et al. (7)]. Asymptomatic CD patients have minimal or no symptoms, but they have increased CD-specific serum antibody levels and villous atrophy. Potential CD patients have no symptoms and a normal mucosa, but increased levels of antibodies indicating an increased risk of developing CD. Most individuals with asymptomatic or potential CD have not yet been diagnosed for CD. They may be diagnosed through case-finding approaches in at-risk populations such as first-degree relatives of CD patients or patients with autoimmune diseases (e.g., diabetes mellitus type I, autoimmune hepatitis, autoimmune thyroid disease) or genetic disorders (e.g., Down syndrome) with known associations to CD (95–97).
Refractory CD, classified into type I (normal IEL phenotype) and II (aberrant IEL phenotype), is a rare but very serious complication, which is characterized by persistence of CD-specific symptoms and mucosal lesions despite a permanent, strict gluten-free diet (GFD) (98). Type I can usually be treated with corticosteroids, but type II imposes a serious risk of progression to enteropathy-associated T-cell lymphoma, small intestinal adenocarcinoma, and ulcerative jejunitis.
Diagnosis
The diagnosis of CD requires a high level of clinical suspicion and a stepwise approach. The diagnostic scheme may consist of five steps: (a) clinical history and symptoms, (b) serology, (c) small intestinal histology, (d) response to a GFD, and (e) HLA status. The most recent diagnostic algorithm developed for children by the European Society for Pediatric Gastroenterology, Hepatology, and Nutrition (ESPGHAN) recommends testing symptomatic patients or risk groups for IgA anti-tissue transglutaminase antibodies (TGA) and total serum IgA to exclude IgA deficiency. If IgA TGA is negative and total IgA is normal, CD is unlikely. If IgA TGA levels are <10 × the upper limit of normal (ULN), the patient has to undergo upper endoscopy with multiple biopsies including at least four from the descending part of the duodenum and at least one from the duodenal bulb. If IgA TGA levels are >10 × ULN, the patient is additionally tested for IgA anti-endomysium antibodies (EMAs). If EMAs are positive, the diagnosis of CD is established, and a GFD is initiated with subsequent follow-up for improvement of symptoms and decline of antibodies to substantiate the correct diagnosis. If EMA is negative, additional testing including biopsies is needed. Gluten challenge and repetitive biopsies are only necessary in ambiguous cases. Especially in children, biopsies are avoided, if possible, but are still recommended, if there are uncertainties related to the performance of the kit used to measure IgA TGA (99). The same algorithm essentially applies to adults, with the only difference that a duodenal biopsy is advisable in almost all cases because adults are more likely to have an alternative diagnosis or to be non-responsive to a GFD (100). There are many clinical situations that require special considerations and additional tests, such as patients under the age of two and patients with selective IgA deficiency, other immunodeficiencies, or on immunosuppressive medication (101). In case of potential CD, regular follow-up on a normal diet is recommended, whereas patients with suspected, but undocumented CD already adhering to a GFD need to undergo gluten challenge (102). The final confirmation of CD is established by a clinical, serological, and histological response to a strict GFD. Genetic tests based on HLA-DQ2 and -DQ8 alleles can be used to rule out CD in ambiguous cases because of their high negative predictive value. The need for mass screening for CD in the general population is discussed controversially (103). At present, screening is recommended for close relatives of CD patients and for persons with diseases known to be associated with CD such as autoimmune diseases.
Pathomechanism
The pathomechanism of CD is complex and involves both adaptive and innate immune responses (104). Due to their high contents of proline and glutamine, gluten proteins are resistant to complete digestion by human gastrointestinal enzymes. As a consequence, peptides with a length of nine amino acid residues and more stay intact and pass the small intestinal epithelium, either by the trans- or the paracellular routes into the lamina propria (105). More than 1,000 CD-active (CD-toxic and/or -immunogenic) gluten peptides derived from gliadins, glutenins, secalins, hordeins, and avenins have been identified, seven of which are classified as CD-toxic (tested in vivo or on organ cultures), five of which are classified as CD-toxic and CD-immunogenic (tested by T-cell proliferation assays), and the vast majority of which are classified as CD-immunogenic (106). Typical features of CD-active peptides are high contents of proline (P) residues and a left-handed polyproline II helical conformation that protects from enzymatic degradation as well as high contents of glutamine (Q) residues that serve as substrates for deamidation or transamidation by human tissue transglutaminase (TG2) (107). Having reached the lamina propria, gluten peptides with QXP or QXXJ motifs (X, any amino acid, J, hydrophobic amino acid) are specifically deamidated (Q → E, introduction of a negatively charged glutamic acid residue) or transamidated by TG2 (either to itself or to other lysine donors) (108, 109), whereas QP and QXXP motifs are left unmodified (110). Then, gluten peptides are bound to the heterodimeric HLA-DQ2 or -DQ8 receptors on the surface of APCs. Deamidation at positions 4, 6, and 7 of the gluten peptides strongly favors binding to HLA-DQ2, while HLA-DQ8 prefers deamidation at positions 1 and 9 (respective locations of positively charged amino acid residues in the binding pockets of HLA-DQ2 or -DQ8) (86). APCs subsequently present gluten peptides to the receptor of naive CD4+ T cells and promote T-cell activation and differentiation into gluten-specific inflammatory effector T cells. On the one hand, these gluten-specific CD4+ T cells secrete proinflammatory cytokines, such as interferon-γ and tumor necrosis factor (TNF)-α, which stimulate the release and activation of matrix metallopeptidases. These break down extracellular matrix proteins and thus lead to the destruction of the small intestinal epithelium (proinflammatory Th1-pathway, adaptive immune response). On the other hand, gluten-specific CD4+ T cells help B cells that carry internalized TG2-gluten peptide complexes. This results in B-cell activation and differentiation into plasma cells that produce IgA and IgG antibodies against gliadin and deamidated gluten peptides as external antigens, and against endomysium and TG2 as autoantigens (anti-inflammatory Th2-pathway, adaptive immune response) (84, 111).
Additionally, gluten peptides stimulate the innate immune response and trigger the secretion of interleukin (IL)-15 by activating enterocytes, macrophages, and dendritic cells. As a result, lymphocytes are stimulated to express the receptor NKG2D and epithelial cells to express MICA (major histocompatibility complex class I chain-related molecule A), the ligand for NKG2D. Once MICA has bound to NKG2D, IELs start to destroy epithelial cells. The key factor explaining why tolerance to gluten is lost involving the switch from a tolerogenic Foxp3+ regulatory T-cell response to a proinflammatory Th1 response still remains elusive (112).
Treatment
A strict GFD with a daily gluten intake below 20 mg is currently the only safe and efficient therapy for CD to fully restore patient health. Dietetic gluten-free products are made from safe cereals (e.g., corn, rice, sorghum, or millet), pseudocereals (e.g., amaranth, buckwheat, or quinoa), other sources of flour or starch (potatoes or chestnut), and “gluten replacers” (xanthan or guar gum) (113). Owing to the restricted availability and high costs of gluten-free alternatives and their poorer quality (114), many CD patients regard the GFD as a substantial burden that decreases quality of life, especially when eating out or traveling. Therefore, their most pronounced desire is the development of a pill or a vaccine that will allow them to eat gluten-containing foods, at least sometimes. Therefore, alternative therapies targeting different steps in the pathomechanism of CD are in various stages of development. Therapies that have already reached clinical trial stage include glucocorticoids (budesonide), oral administration of gluten-degrading enzymes (ALV003, a mixture of cysteine endopeptidase B, isoform 2, and a prolyl endopeptidase from Sphingomonas capsulata), oral intake of gluten-sequestering polymeric resins [poly(2-hydroxyethylmethacrylate-co-styrene-4-sulfonic acid, sodium salt)], zonulin antagonists (larazotide acetate), vaccination (Nexvax2), probiotics, and hookworm infection (115). All alternative therapies still need to demonstrate that they are tolerable and safe and have no adverse long-term side-effects. Especially for gluten-degrading or -removing agents, the amount of gluten that can be safely ingested needs to be determined considering all other ingredients of the meal that may hinder the efficacy of the agent. Finally, the benefits and risks of alternative therapies have to be carefully weighed against the GFD to ensure that CD patients receive the best treatment option available.
Dermatitis Herpetiformis and Gluten Ataxia
With a prevalence of 0.03–0.07%, dermatitis herpetiformis (DH) is often referred to as the skin manifestation of CD. Both diseases are caused by gluten, respond to treatment with a GFD, and share the genetic predisposition caused by HLA-DQ2 or -DQ8. Typical symptoms are intense itching and burning papules, macules, and blisters especially on the elbows, knees, and buttocks. DH is ideally diagnosed by direct immunofluorescence biopsy of unaffected skin close to an active lesion that reveals granular IgA deposits in the papillary dermis. The autoantigen in DH is epidermal transglutaminase (TG3). The most likely pathogenic route starts from potential or asymptomatic CD in the small intestine with secretion of IgA against TG2 and TG3 into the blood circulation and results in the deposition of TG3 and IgA against TG3 in the papillary dermis. Due to the presence of active TG3, IgA–TG3 complexes are formed and crosslinked to fibrinogen in the skin (116).
Gluten ataxia (GA) can be regarded as rare neurological manifestation of CD and is defined as idiopathic sporadic ataxia characterized by the presence of IgA or IgG against gliadin in the blood. GA presents with gait and lower limb ataxia, nystagmus, and other visual disorders. Up to 40% of patients show small intestinal damage as in CD and up to 60% of patients have evidence of cerebellar atrophy. Much like in DH, the autoantigen in GA is the primarily brain-expressed transglutaminase (TG6) and IgA against TG6 are serological markers for GA. TG6 and IgA against TG6 accumulate in the brain stem and cerebellum causing infiltration of white matter with lymphocytes and irreversible loss of Purkinje cells in the cerebellar cortex. Therefore, a fast diagnosis of GA and treatment with a GFD are essential to prevent progression of cerebellar dysfunction (117).
IgE-Mediated Wheat Allergies
WAs are defined as adverse immune responses to wheat proteins that reproducibly occur in affected individuals within minutes to hours after exposure (118, 119). A wide variety of wheat proteins including gluten and non-gluten proteins have been identified as allergens [overviews in Brouns et al. (120), Juhasz et al. (121), and Tatham and Shewry (122)]. Depending on the route of allergen exposure and the underlying pathomechanism, WAs can be classified into food allergy, wheat-dependent exercise-induced anaphylaxis (WDEIA), respiratory allergy, and skin allergy. The prevalence estimates for WAs depend on the assessment method used, but range from 0.1% (positive food challenge) to 3.6% (lifetime self-reported prevalence) (123).
Common diagnostic procedures include patient history reporting reproducible symptoms after allergen exposure, skin prick tests, analysis of specific IgE antibodies, or functional assays such as in vitro basophil activation tests and oral food challenge. While oral food challenge is generally regarded as a gold standard for the diagnosis of wheat allergy and can offer clarity in ambiguous cases, it is difficult to undertake in routine clinical practice and puts patients at risk of experiencing a severe allergic reaction. The treatment for wheat allergy mostly involves avoidance of exposure to allergens, either in the form of flours and flour dust or elimination of wheat products from the diet. Antihistamines or corticosteroids can be used to treat acute cases (124, 125).
The mechanism of IgE-mediated allergies includes two phases. The first step is sensitization to the allergen upon initial contact, followed by an allergic reaction upon reexposure to the allergen (126). When an allergen is encountered for the first time, it is internalized by APCs (e.g., dendritic cells) and presented to naive CD4+ T cells. In the presence of cytokines, the naive CD4+ T cells become activated and differentiate into Th2 cells that subsequently produce different ILs. IL-4 turns on IgE-producing B cells and sustains the development of Th2 cells, IL-5 activates eosinophils, IL-9 enhances IgE production, mast cell growth and expression of the high-affinity IgE receptor (FcεRI), and IL-13 acts on epithelial cells to stimulate mucus secretion. The secreted IgE antibodies bind to FcεRI on the surface of mast cells and basophils. When the mast cell carrying IgE antibodies is reexposed to the allergen, the multivalent allergen crosslinks two adjacent IgE antibodies and the underlying FcεRI. This bridging leads to mast cell degranulation with discharge of primary (preformed) mediators (histamine, neutral peptidases, acid hydrolases, and proteoglycans such as heparin and chondroitin sulfate) and synthesis and release of secondary mediators (leukotrienes, prostaglandin D2, platelet-activating factor, cytokines, and chemokines). The rapid release of histamine and leukotrienes is responsible for the intense early allergic response characterized by wheezing, sneezing, urticaria, and mucus secretion. The survival of mast cells and enhanced expression of the FcεRI receptor is sustained by signals from these receptors, thus providing a mechanism of amplification. In the following phase, eosinophils are activated by IL-5 and attracted to the site of the immediate reaction by chemokines (e.g., eotaxin). By producing cytokines, leukotrienes, and proteins (major basic protein and eosinophil cationic protein) that are toxic to epithelial cells, the inflammatory response is amplified and sustained without additional exposure to the allergen. These events lead to the late allergic response, which involves further wheezing, nasal blockage, and eczema. Basophils play a similar role to that of mast cells, but they circulate in the blood rather than being present in the affected tissues.
Respiratory and Skin Allergy to Wheat
Respiratory WA comprises baker's asthma and allergic rhinitis, which are allergic responses to the inhalation of flours and dust from wheat and other cereals (rye, barley) known since Roman times. Both allergies rank among the most prevalent occupational diseases and affect 1–10% (baker's asthma) and 18–29% (allergic rhinitis) of bakers, millers, and confectioners. Depending on the severity of the reaction, vocational retraining may be necessary. More than 100 IgE-binding proteins were identified, of which chloroform–methanol-soluble (CM-) ATIs, lipid transfer (LTP) and non-specific lipid transfer proteins (nsLTP) are the major allergens (127).
Contact urticaria is an allergic reaction on the skin following contact with an eliciting allergen (128). Typical symptoms of urticaria are localized wheal-and-flare reactions such as hives and a raised, burning, and/or pruritic swelling of the skin, often accompanied by angioedema that appear within 10–30 min after allergen exposure and fade away within hours. In contrast, contact dermatitis is accompanied by the appearance of large, burning, and itchy rashes, blisters, and wheals that take several days to weeks to heal (129). Similar to respiratory WA, millers, bakers, and flour handlers are most frequently affected.
Food Allergy to Wheat
Wheat is the third most common cause of food allergy (only surpassed by milk and egg) and has to be labeled on prepacked foods according to Codex Alimentarius Standard 1–1985 (130) and also non-prepacked foods according to EU regulation 1169/2011. Wheat food allergy occurs within a few hours of wheat ingestion and may present with symptoms on the skin (e.g., atopic dermatitis, urticaria, angioedema), in the respiratory tract (e.g., wheezing, bronchial obstruction), in the gastrointestinal tract (e.g., abdominal pain, bloating, diarrhea), and even anaphylaxis. In comparison to other allergies such as peanut allergy, the dose of wheat proteins needed to trigger allergic reactions is usually quite high (about 1 g), but may also be lower (10 mg) depending on individual sensitivities (131). The causative factors are non-gluten proteins as well as gluten proteins, with ATIs 0.19, CM1, CM2, CM3, and CM16, LTP, and nsLTP as well as α-gliadins, γ-gliadins, and HMW-GS as major allergens (132). The IgE-binding epitopes derived from the repetitive sequences of gluten proteins such as QFPQQQFPQQ (ω5-gliadins), QQSFPLQPQQ (ω1,2-gliadins), VQQQQFPGQQ (α-gliadins), QQLPQPQQP (γ-gliadins), and SQQQPPF (LMW-GS) with the consensus motif QQX1PX2QQ (with X1 being L, F, S, or I and X2 being Q, E, or G) are different to those reported for CD (133).
Wheat-Dependent Exercise-Induced Anaphylaxis
WDEIA is a special form of WA because wheat intake alone does not trigger the allergic reaction, but in combination with augmenting cofactors, such as physical exercise. Further cofactors are alcohol, acetylsalicylic acid (aspirin®), and other non-steroidal anti-inflammatory drugs and stress (134, 135). The estimated prevalence of WDEIA is <0.1%. Clinical features range from urticaria and angioedema to dyspnea, hypotension, collapse, and anaphylactic shock. The major triggers are ω5-gliadins and HMW-GS, but other gluten protein types may also be involved. A special form of WDEIA is an allergic reaction caused by epicutaneous sensitization with hydrolyzed wheat proteins in cosmetics (136). Although WDEIA is regarded as the best-studied model of cofactor-induced anaphylaxis, it is still not clear how exactly the cofactors act as adjuvants causing the reaction. A decreased activation threshold of mast cells and basophils has been discussed, but it appears to be more likely that cofactors increase the bioavailability of allergens by promoting small intestinal permeability (137).
Non-IgE-Mediated Wheat Allergies
Non-IgE-mediated food allergies are well-known in children under the age of three, and they are divided into three main clinical conditions: food protein-induced (FPI) enterocolitis syndrome, FPI proctocolitis, and FPI enteropathies. The pathomechanism is currently not well-known, but these conditions are characterized by high levels of IL-13 and TNF-α as drivers of intestinal epithelial damage and eosinophil infiltration. The most common trigger for all three conditions is cow's milk, but soy, rice, and wheat have also been reported (138). Non-IgE-mediated food allergies are less well-recognized in adults (139), but there is an increasing body of evidence that they may be under-recognized. NCGS might in fact be a non-IgE-mediated food allergy because of patient history (food allergy during childhood or presence of atopic diseases) and serological and histological findings such as positive serum anti-gliadin antibodies, in vitro basophil activation, and presence of eosinophils in the intestinal mucosa (140).
Non-Celiac Gluten Sensitivity
NCGS, frequently termed gluten or (non-celiac) wheat sensitivity, may be defined as a gluten (wheat)-dependent disorder with symptoms similar to CD, but usually normal small intestinal mucosa (141). Moreover, NCGS is characterized by the lack of serum TG2 antibodies and the missing association to HLA-DQ2/8 alleles. The exact prevalence is still unknown and may be similar to that of CD with reports ranging from about 1% in El Salvador (13) and Mexico (11) to 1.7% in Brazil (10), but may also be higher with up to 6% (142). The clinical differentiation of NCGS from other WRDs is difficult due to several common features: similar symptoms, wheat proteins as the triggering factor, and a GFD as recommended treatment (78). Typical gastrointestinal manifestations of NCGS are abdominal pain, bloating, and chronic diarrhea. Frequent extraintestinal complaints include headache, “foggy mind,” fatigue, anxiety, depression, numbness in the legs, arms and fingers, and joint pain (143). In contrast to CD, NCGS is not associated with malabsorption, nutritional deficiencies, or increased risk for autoimmune diseases or malignancy.
Because symptoms disappear on a GFD, gluten proteins have been considered as a precipitating factor (144), but it is still not clear whether gluten is responsible. Other wheat constituents such as non-gluten proteins and FODMAPs might be additionally responsible for NCGS (145). Recent studies proposed that ATIs contribute to the development of NCGS by an innate immune response mediated by the toll-like receptor pathway (146). Due to the absence of NCGS-specific biomarkers, the diagnosis is currently made by exclusion of CD, WA, other food intolerances, and irritable bowel syndrome (147). The diagnosis is definitely proven by oral wheat challenge after at least 3 weeks on a GFD and the subsequent occurrence of typical symptoms (148). A GFD is recommended as treatment, whereby symptoms usually improve rapidly. In contrast to CD, where strict GFD has to be maintained, patients with NCGS could adapt a more liberal diet reducing the gluten intake by ≈90% (146).
Irritable Bowel Syndrome
Irritable bowel syndrome (IBS) is the most common and extensively evaluated functional bowel disorder (149). Its prevalence in adult individuals has been estimated in a range from 5 to 20%. IBS is not a single disease but rather a symptom cluster resulting from diverse pathologies (150). Typical symptoms, including abdominal discomfort and pain, gas, bloating, and diarrhea with and without constipation, are similar to those of CD, NCGS, intestinal bacterial overgrowth, and lactose intolerance (151). The Rome IV criteria categorize IBS by the most predominant presenting symptoms: diarrhea, constipation, mixed, or unspecified (152). The majority of patients perceive their symptoms as being related to specific meals, in particular, foods rich in carbohydrates. Wheat is regarded as one of the most relevant IBS triggers, although which component of this cereal is involved remains unclear (153). Gluten, FODMAPs, other wheat proteins, for example, ATIs, have been suggested as possible factors for symptom generation. The experimental and clinical evidence on the role of gluten/wheat in IBS has been presented by Volta et al. (154). Some types of IBS, especially diarrhea-predominant cases, show symptomatic improvement on a GFD (155). Today, the diagnosis is not only based on the exclusion of other food hypersensitivities, but on positive diagnosis using symptom-related criteria (“Rome III diagnostic criteria”) (156). The pathomechanism of IBS is not completely understood; factors important to the development of IBS include alterations in the gut microbiome, intestinal permeability, gut immune function, motility, visceral sensation, brain–gut interactions, and psychosocial status (157). Strategies for treatment are based on general recommendations such as avoidance of foods rich in fat and carbohydrates, dairy products, caffeine, and alcohol, on the one hand, and increased intake of DF, on the other hand. If patients can associate the ingestion of certain foods with the complaints, an improvement may be achieved after restriction of these foods. Diarrhea-predominant patients are advised to test a GFD for several months (155).
The Sources of Confusion Around the Sad Face of Wheat
Unsubstantiated Statements Blaming Wheat
Over the last decade, wheat has been the center of a vigorous debate related to health and nutrition, and it has gained an increasingly negative reputation among the Western population. The first and main source of confusion arose from several pseudoscientific books such as “Wheat Belly” (158) and “Grain Brain” (159), numerous media reports and statements by celebrities promoting the overall impression that wheat consumption has adverse health effects for the general population. The main statements claimed that wheat consumption was the cause of overweight and obesity, that wheat caused a whole number of other disorders such as diabetes type 2, asthma, reflux disease, sleep disorders, neuronal complaints, etc., that wheat bread had an overly high glycemic index, that wheat contained opioids that caused addiction, and that modern wheat had been genetically modified and contained unique toxic proteins that caused the higher prevalence of WRDs. In conclusion, the general population was advised to avoid the consumption of wheat products.
However, these books intermingle sound scientific evidence with theoretical, controversial, and wrong statements and make it virtually impossible for consumers to separate the truth from myths. The essential summary that “foods made from wheat make people sick, stupid, fat, and addictive” (158, 159) led to great uncertainties among consumers. As a consequence, the popularity of a GFD has increased, with up to 5% of the population in New Zealand reporting gluten avoidance (160) and up to 13% of the UK population self-reporting a WRD (9). The consumption of gluten-free foods has significantly increased over the last years, up from a global retail sales value of 1.95 × 109 USD in 2012 to 3.84 × 109 USD in 2017, with further increases projected in the coming years (161). The reasons why some people voluntarily adopt a GFD include that they think it might help them reduce weight, that they perceive a GFD as healthier and better for their overall well-being and that they self-diagnosed a WRD.
To counteract the increasing uncertainty among consumers, numerous counterstatements of the scientific community [e.g., (120, 162–165)] have emphasized that wheat consumption is safe for the vast majority of the population and that wheat avoidance is only necessary after medical diagnosis of a true WRD. These reviews have compiled convincing evidence to refute the abovementioned statements and assert that the regular consumption of whole-grain products is associated with reduced risks of type 2 diabetes and of colorectal cancer, likely reduced risks of colon cancer and cardiovascular diseases, and more favorable weight management (166).
The Increasing Prevalence of Wheat-Related Disorders
The second source of confusion related to wheat consumption is based on the increasing amount of evidence from well-founded epidemiological studies that show a rise in the prevalence of WRDs over the past 50 years. While this rise can be partially explained by better diagnostics and improved awareness, recent reports, e.g., from Denmark (167), Italy (168), the United States (169), and worldwide (79) show that the prevalence of CD has indeed increased over time. The same is reported for NCGS (170) and also for allergies and autoimmune diseases, in general (171). Despite ongoing research, the underlying causative factors have not been unambiguously identified so far. WRDs are initiated through a loss of immunotolerance to wheat proteins at a certain point in time, but the factors causing this initial loss are the subject of ongoing investigations and may also be different depending on the genetic predisposition, dietary habits, and overall lifestyle of each individual.
The most likely factors include the hygiene hypothesis that was originally based on the observation that the decreased frequency of overall infectious and parasitic diseases was inversely correlated to the increased frequency of allergic and autoimmune diseases seen in industrialized countries since the 1950s (88). The hygiene hypothesis is supported by studies on migrants, who are as likely to develop an autoimmune disease as individuals in the host country with a high incidence of autoimmune diseases, even if they originally came from a country with a low incidence of autoimmune diseases, but moved at a young age (172). Studies in mice support the protective effects of pathogenic bacteria, viruses, and parasites on autoimmunity and the additional positive effects of commensals that stimulate innate and adaptive immune regulatory pathways (88). In contrast, a lack of physical activity, a lot of time spent indoors, and a diet rich in saturated fats and digestible carbohydrates, but poor in DF, are associated with a loss of microbial diversity of the gut, skin, and other tissues and a subsequent loss of symbiotic relationships with parasites and bacteria that used to exist during human evolution (173–175). Additional factors contributing to changes in the microbiome are antibiotics and vaccinations and decreased exposure to airborne bacteria, all of which may contribute to alterations in intestinal permeability. Intestinal barrier dysfunction has been associated with a variety of intestinal and systemic diseases, including CD (176).
Seen from the side of wheat and related cereals, changes in protein composition due to breeding, heat and cold stress, or agricultural practices have been postulated as potential contributors to a higher immunostimulatory potential of modern wheat species compared to landraces and heritage wheats. While protein expression patterns do differ between wheat species, i.e., diploid einkorn, tetraploid emmer, and durum wheat as well as hexaploid spelt and common wheat (177–179), there are currently too few comparative in vitro or in vivo studies available to allow a precise assessment as to whether these differences might be related to the prevalence of WRDs or not. For example, the 33-mer peptide from α2-gliadin that is frequently described as the immunodominant peptide in CD, was only detected in common wheat and spelt, but it was not present in emmer, durum wheat, or einkorn (180). Ancient wheats like einkorn, emmer and spelt were suggested to provide health benefits compared to common wheat, but recent reviews collected evidence demonstrating that they differ little in their composition. Thus, ancient wheats do not appear to be “healthier” than modern wheats (41), with some exceptions, e.g., high lutein and steryl ferulate contents in einkorn (181).
Several comparative studies on old and modern cultivars within the species Triticum aestivum set out to study the influence of breeding during the last century (182). So far, the results are somewhat inconclusive because one study from Canada reported an increase (183), two studies from the United States essentially reported no change (184, 185), and three others from the United Kingdom, Germany, and the United States reported a decrease in protein contents over time (186–188). All studies report a substantial influence of the growing conditions on the content and composition of wheat proteins. Regarding protein composition, an increase in glutenins and a decrease in gliadins and gliadin/glutenin ratios, but essentially no changes for albumins/globulins and gluten, were observed in German winter wheat cultivars from 1891 to 2010, all grown at the same location in three consecutive years (189). Similar results were reported by Ozuna and Barro (190). Principal component analysis of the chromatographic fingerprints of albumins/globulins, gliadins, and glutenins showed a cluster formation of the most modern cultivars (first registered from 1981 to 2010) and of the oldest ones (1891–1920), but with exceptions and samples from 1951 to 1980 in between. The largest variability in protein profiles was observed for the samples from 1921 to 1950 [Figure 3, (191)]. Altogether, the evidence, so far, points to the conclusion that old and modern wheat cultivars do show changes in protein composition due to breeding, but so far, none of these changes seems to be linked to the prevalence of WRDs.
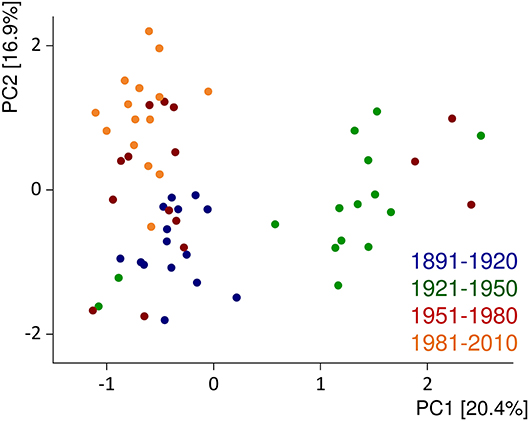
Figure 3. Principal component (PC) analysis biplot of protein fingerprints of albumins/globulins, gliadins, and glutenins relative to the sum of extractable proteins. The data are displayed for 60 German winter wheat cultivars first registered from 1891 to 2010 and show the average of three harvest years (2015–2017). Figure modified from Pronin et al. (191).
As wheat is part of a huge variety of products, the amounts of immunoreactive proteins in the end-product that is eventually consumed is more important than in the original flour. Wheat processing techniques as well as strategies to reduce exposure have been extensively reviewed recently (165). The use of ungerminated grains, of refined white flour instead of wholegrain flour, of fast straight-dough yeast fermentation instead of diverse and long sourdough fermentations, as well as the use of wheat gluten as a technofunctional additive in a number of food products (192) have been discussed as additional factors that may contribute to causing WRDs (165, 193). Consumption of these wheat products may have increased the total amount of gluten in the diet, thus surpassing a certain threshold level necessary to trigger WRDs. However, credible data about adverse effects of modern wheat processing are not available, and no epidemiological studies have evaluated the contribution of modern processing on the increasing prevalence of WRDs. For example, there is no proof that modern bread making, including short and non-acidic fermentation of doughs or addition of vital gluten, resulted in higher gluten immunoreactivity. Epidemiological data demonstrate that countries, in which long-fermented sourdough breads are common (e.g., Finland and Sweden) even have higher CD prevalences than countries, where short-fermented yeast-leavened breads are consumed almost exclusively (e.g., Italy and Spain) (194).
The Unknowns
The third source of confusion mostly arises from the fact that the triggers for NCGS and wheat-sensitive IBS have not been clearly identified, so far (140), partly due to short-comings in the design of the nutritional intervention studies, placebo and nocebo effects (195), and/or insufficient characterization of the wheat product or wheat extract administered. The constituents that are discussed as causes of NCGS are gluten, non-gluten proteins (e.g., ATIs), and FODMAPs. In many cases, products containing wheat flour were used for oral food challenge, and in this case, gluten, non-gluten proteins, and FODMAPs were present (196). In other cases, wheat gluten was used, but these isolates also contain non-gluten proteins, so that the effects of gluten and ATIs cannot be clearly distinguished (195, 197). Gluten, as a cause of NCGS, has mostly been inferred from the fact that NCGS patients' symptoms are alleviated if they follow a GFD, but several studies have reported that gluten may not be the causative factor in NCGS, but rather FODMAPs (195, 198). ATIs were identified as triggers of innate immunity via the toll-like receptor 4, and they have been implicated in causing NCGS (146) and acting as adjuvants of other inflammatory diseases (78).
Furthermore, there is a significant overlap of symptoms of CD, NCGS, and IBS. It is clear that CD patients need to follow a strict GFD, and no positive effects of a GFD or a low FODMAP diet have been proven for healthy individuals (199). In between, both NCGS and IBS patients benefit from a low FODMAP diet, but even more so of a GFD, probably because of a multifactorial etiology of NCGS that combine a function effect caused by FODMAPs with a mild immune reaction combined by a dysbalance of microbiota (200). In most cases, gluten-free raw materials are naturally low in FODMAPs and also low in ATIs, so that a GFD is low in all potential causes for NCGS. In this context, sourdough fermented breads may also be better tolerated by NCGS and IBS patients, because Lactobacillaceae and Bifidobacteriaceae, as well as fungi and yeasts, possess enzymes capable of degrading gluten and FODMAPs (201). Due to the overlap between a low FODMAP diet and a GFD, it is likely that patients with NCGS, especially self-diagnosed ones, are more likely to suffer from IBS. Vice versa, there is a subgroup of NCGS patients among the IBS patients (140). A FODMAP-restricted diet is recommended to treat IBS, but not in the long term, because FODMAPs are also part of DF, and a complete elimination will have a negative effect on gut microbial diversity. Further well-designed dietary intervention studies with appropriate controls and sufficient characterization of the challenge materials are still needed to identify the causes of NCGS and also differentiate which patients will benefit from a GFD or rather a low FODMAP diet.
The Smiling Face of Wheat Prevails
In the last 10 years, wheat has received much negative attention because several pseudoscientific books and numerous media reports fueled the overall assumption that wheat consumption makes people sick. Despite the common consumer perception of a GFD as being healthy, gluten-free foods had higher contents of fat, saturated fat, sugar, and salt compared to gluten-containing foods (202). The most common deficiencies on a GFD are insufficient amounts of DF, vitamins, calcium, iron, magnesium, and zinc. Moreover, a strict GFD decisively reduces the quality of life, as CD patients can confirm, and may lead to low compliance (203).
Wheat is among the oldest and most extensively grown crops and one foundation to ensure food security for the increasing world population. Technological advances in breeding, farming, and processing have paved the way for wheat to become one of the most widespread and cheapest raw materials for food and non-food applications. No other food crop supplies humans with such a huge diversity of products from bread to other baked goods and pasta products that serve as staple foods all over the world. Wheat-based foods provide valuable nutrients such as proteins, DF, vitamins, minerals, and bioactive phytochemicals and supply up to 20% of the energy intake of the global population. Additionally, wheat is important for many non-food applications, and a number of wheat constituents like starch and gluten are present in items of daily use. Considering all available evidence, so far, there is no reason to eliminate wheat from the diet, except for individuals suffering from WRDs.
Author Contributions
HW wrote the original draft. PK and KS wrote, reviewed, and edited the manuscript. All authors contributed to the article and approved the submitted version.
Conflict of Interest
The authors declare that the research was conducted in the absence of any commercial or financial relationships that could be construed as a potential conflict of interest.
Acknowledgments
We acknowledge support by the KIT-Publication Fund of the Karlsruhe Institute of Technology.
References
1. FAOSTAT. Food and Agriculture Organization of the United Nations (2020). Available online at: http://www.fao.org/faostat/en/#data
2. Balfourier F, Bouchet S, Robert S, De Oliveira R, Rimbert H, Kitt J, et al. Worldwide phylogeography and history of wheat genetic diversity. Sci. Adv. (2019) 5:eaav0536. doi: 10.1126/sciadv.aav0536
3. Shiferaw B, Smale M, Braun HJ, Duveiller E, Reynolds M, Muricho G. Crops that feed the world 10. Past successes and future challenges to the role played by wheat in global food security. Food Sec. (2013) 5:291–317. doi: 10.1007/s12571-013-0263-y
4. Chen GC, Tong X, Xu JY, Han SF, Wan ZX, Qin JB, et al. Whole-grain intake and total, cardiovascular, and cancer mortality: a systematic review and meta-analysis of prospective studies. Am J Clin Nutr. (2016) 104:164–72. doi: 10.3945/ajcn.115.122432
5. Jonnalagadda SS, Harnack L, Hai Liu R, McKeown N, Seal C, Liu S, et al. Putting the whole grain puzzle together: health benefits associated with whole grains—summary of american society for nutrition 2010 satellite symposium. J Nutr. (2011) 141:1011S−22S. doi: 10.3945/jn.110.132944
6. Reynolds AN, Akerman AP, Mann J. Dietary fibre and whole grains in diabetes management: systematic review and meta-analyses. PLoS Med. (2020) 17:e1003053. doi: 10.1371/journal.pmed.1003053
7. Ludvigsson JF, Leffler DA, Bai JA, Biagi F, Fasano A, Green PHR, et al. The oslo definitions for coeliac disease and related terms. Gut. (2013) 62:43–52. doi: 10.1136/gutjnl-2011-301346
8. Potter MD, Walker MM, Jones MP, Koloski NA, Keely S, Talley NJ. Wheat intolerance and chronic gastrointestinal symptoms in an Australian population-based study: association between wheat sensitivity, celiac disease and functional gastrointestinal disorders. Am J Gastroenterol. (2018) 113:1036–44. doi: 10.1038/s41395-018-0095-7
9. Aziz I, Lewis NR, Hadjivassiliou M, Winfield SN, Rugg N, Kelsall A, et al. A UK study assessing the population prevalence of self-reported gluten sensitivity and referral characteristics to secondary care. Eur J Gastroenterol Hepatol. (2014) 26:33–9. doi: 10.1097/01.meg.0000435546.87251.f7
10. Arámburo-Gálvez JG, Beltrán-Cárdenas CE, Geralda André T, Carvalho Gomes I, Macêdo-Callou MA, Braga-Rocha ÉM, et al. Prevalence of adverse reactions to gluten and people going on a gluten-free diet: a survey study conducted in Brazil. Medicina. (2020) 56:163. doi: 10.3390/medicina56040163
11. Ontiveros N, López-Gallardo JA, Vergara-Jiménez MJ, Cabrera-Chávez F. Self-reported prevalence of symptomatic adverse reactions to gluten and adherence to gluten-free diet in an adult Mexican population. Nutrients. (2015) 7:6000–15. doi: 10.3390/nu7075267
12. Van Gils T, Nijeboer P, IJssennagger CE, Sanders DS, Mulder CJ, Bouma G. Prevalence and characterization of self-reported gluten sensitivity in The Netherlands. Nutrients. (2016) 8:714. doi: 10.3390/nu8110714
13. Ontiveros N, Rodríguez-Bellegarrigue CI, Galicia-Rodríguez G, Vergara-Jiménez MDJ, Zepeda-Gómez EM, Arámburo-Galvez JG, et al. Prevalence of self-reported gluten-related disorders and adherence to a gluten-free diet in Salvadoran adult population. Int J Environ Res Public Health. (2018) 15:786. doi: 10.3390/ijerph15040786
14. Feuillet C, Langridge P, Waugh R. Cereal breeding takes a walk on the wild side. Trends Genet. (2008) 24:24–32. doi: 10.1016/j.tig.2007.11.001
15. International Wheat Genome Sequencing Consortium (IWGSC). Shifting the limits in wheat research and breeding using a fully annotated reference genome. Science. (2018) 361:eaar7191. doi: 10.1126/science.aar7191
16. Pont C, Leroy T, Seidel M, Tondelli A, Duchemin W, Armisen D, et al. Tracing the ancestry of modern bread wheats. Nat Genet. (2019) 51:905–11. doi: 10.1038/s41588-019-0393-z
17. Mondal S, Rutkoski JE, Velu G, Singh PK, Crespo-Herrera LA, Guzmán C, et al. Harnessing diversity in wheat to enhance grain yield, climate resilience, disease and insect pest resistance and nutrition through conventional and modern breeding approaches. Front Plant Sci. (2016) 7:991. doi: 10.3389/fpls.2016.00991
18. Reganold JP, Wachter JM. Organic agriculture in the twenty-first century. Nat Plants. (2016) 2:15221. doi: 10.1038/nplants.2015.221
19. Asseng S, Ewert F, Martre P, Rötter RP, Lobell DB, Cammarano D, et al. Rising temperatures reduce global wheat production. Nat Clim Change. (2015) 5:143–7. doi: 10.1038/nclimate2470
20. Trnka M, Hlavinka P, Semenov MA. Adaptation options for wheat in Europe will be limited by increased adverse weather events under climate change. J R Soc Interface. (2015) 12:20150721. doi: 10.1098/rsif.2015.0721
21. Souci SW, Fachmann W, Kraut H. Lebensmitteltabelle für die Praxis (5. Aufl). Germany: Wissenschaftliche Verlagsgesellschaft Stuttgart (2011). p. 241.
22. Andersson AA, Andersson R, Piironen V, Lampi AM, Nyström L, Boros D, et al. Contents of dietary fibre components and their relation to associated bioactive components in whole grain wheat samples from the HEALTHGRAIN diversity screen. Food Chem. (2013) 15:1243–8. doi: 10.1016/j.foodchem.2012.09.074
23. Haska L, Nyman M, Andersson R. Distribution and characterisation of fructan in wheat milling fractions. J Cereal Sci. (2008) 48:768–74. doi: 10.1016/j.jcs.2008.05.002
24. Roberfroid M, Gibson GR, Hoyles L, McCartney AL, Rastall R, Rowland I, et al. Prebiotic effects: metabolic and health benefits. Brit J Nutr. (2010) 104:S1–63. doi: 10.1017/S0007114510003363
25. Pasqualetti V, Altomare A, Guarino MPL, Locato V, Cocca S, Cimini S, et al. Antioxidant activity of inulin and its role in the prevention of human colonic muscle cell impairment induced by lipopolysaccharide mucosal exposure. PLoS ONE. (2014) 9:e98031. doi: 10.1371/journal.pone.0098031
26. Vogt LM, Meyer D, Pullens G, Faas MM, Venema K, Ramasamy U, et al. Toll-Like receptor 2 activation by β2→ 1-fructans protects barrier function of T84 human intestinal epithelial cells in a chain length-dependent manner. J Nutr. (2014) 144:1002–8. doi: 10.3945/jn.114.191643
27. Verspreet J, Dornez E, Van den Ende W, Delcour JA, Courtin CM. Cereal grain fructans: structure, variability and potential health effects. Trends Food Sci Technol. (2015) 43:32–42. doi: 10.1016/j.tifs.2015.01.006
28. Delcour JA, Hoseney RC. Starch. In: Principles of Cereal Science and Technology. 3rd Ed. St. Paul: AACCI (2010). p. 23–51. doi: 10.1094/9781891127632
29. Englyst HN, Cummings JH. Digestion of the polysaccharides of some cereal foods in the human small intestine. Am J Clin Nutr. (1985) 42:778–87. doi: 10.1093/ajcn/42.5.778
30. Birt DF, Boylston T, Hendrich S, Jane JL, Hollis J, Li L, et al. Resistant starch: promise for improving human health. Adv Nutr. (2013) 4:587–601. doi: 10.3945/an.113.004325
31. Hasjim J, Lee SO, Hendrich S, Setiawan S, Ai Y, Jane JL. Characterization of novel resistant-starch and its effects on postprandial plasma-glucose and insulin responses. Cereal Chem. (2010) 87:257–62. doi: 10.1094/CCHEM-87-4-0257
32. Ashwar BA, Gani A, Shah A, Wani IA, Masoodi FA. Preparation, health benefits and applications of resistant starch – a review. Starch Stärke. (2015) 68:287–301. doi: 10.1002/star.201500064
33. Topping DL, Bajka BH, Bajka AR, Bird JM, Clarke L, Cobiac MA, et al. Resistant starches as a vehicle for delivering health benefits to the human large bowel. Microb. Ecol. Health Dis. (2008) 20:103–8. doi: 10.1080/08910600802106541
34. Robertson MD, Bickerton AS, Dennis AL, Vidal H, Frayn KN. Insulin-sensitizing effects of dietary resistant starch and effects on skeletal muscle and adipose tissue metabolism. Am J Clin Nutr. (2005) 82:559–67. doi: 10.1093/ajcn/82.3.559
35. Hamer HM, Jonkers DM, Bast A, Vanhoutvin SA, Fischer MA, Kodde A, et al. Butyrate modulates oxidative stress in the colonic mucosa of healthy humans. Clin Nutr. (2009) 28:88–93. doi: 10.1016/j.clnu.2008.11.002
36. Pawlak DB, Kushner JA, Ludwig DS. Effects of dietary glycemic index on adiposity, glucose homeostasis, and plasma lipids in animals. Lancet. (2004) 364:778–85. doi: 10.1016/S0140-6736(04)16937-7
37. Bednar GE, Patil AR, Murray SM, Grieshop CM, Merchen NR, Fahey GC Jr. Starch fiber fractions in selected food feed ingredients affect their small intestinal digestibility fermentability their large bowel fermentability in vitro in a canine mode. J Nutr. (2001) 131:276–86. doi: 10.1093/jn/131.2.276
38. Baghurst K, Baghurst PA, Record SJ. Dietary fiber, non-starch polysaccharide, and resistand starch intakes in Australia. In: Spiller GA, editor. CRC Handbook of Dietary Fiber in Human Health. Boca Raton, FL: CRC Press (2001). p. 583–91.
39. Shewry PR, Piironen V, Lampi AM, Edelmann M, Kariluoto S, Nurmi T, et al. The HEALTHGRAIN wheat diversity screen: effects of genotype and environment on phytochemicals and dietary fiber components. J Agric Food Chem. (2010) 58:9291–8. doi: 10.1021/jf100039b
40. Hossain K, Ulven C, Glover K, Ghavami F, Simsek S, Alamri MS, et al. Interdependence of cultivar and environment on fiber composition in wheat bran. Aust J Crop Sci. (2013) 7:525–31.
41. Shewry PR, Hey S. Do “ancient” wheat species differ from modern bread wheat in their contents of bioactive components? J Cereal Sci. (2015) 65:236–43. doi: 10.1016/j.jcs.2015.07.014
42. Scientific Advisory Committee on Nutrition Draft Carbohydrate and Health Report 2014 (2015). Available online at: https://assets.publishing.service.gov.uk/government/uploads/system/uploads/attachment_data/file/445503/SACN_Carbohydrates_and_Health.pdf
43. Wcislo G, Szarlej-Wcislo K. Colorectal cancer prevention by wheat consumption: a three-valued logic – true, false, or otherwise? In: Watson RR, Preedy VR, Zibadi S, editors. Wheat Rice in Disease Prevention Health. London; Waltham, MA; San Diego: Academic Press, Elsevier (2014). p. 91–111. doi: 10.1016/B978-0-12-401716-0.00008-8
44. Sang S, Zhu Y. Bioactive phytochemicals in wheat bran for colon cancer prevention. In: Wheat Rice In: Watson RR, Preedy VR, Zibadi S, editors. Disease Prevention Health. London; Waltham, MA; San Diego: Academic Press; Elsevier (2014). p. 121–9. doi: 10.1016/B978-0-12-401716-0.00010-6
45. Zhu Y, Conklin DR, Chen H, Wang L, Sang S. 5-alk(en)ylresorcinols as the major active components in wheat bran inhibit human colon cancer cell growth. Bioorg Med Chem. (2011) 19:3973–82. doi: 10.1016/j.bmc.2011.05.025
46. Aune D, Keum N, Giovannucci E, Fadnes LT, Boffetta P, Greenwood DC, et al. Whole grain consumption and risk of cardiovascular disease, cancer, and all cause and cause specific mortality: systematic review and dose-response meta- analysis of prospective studies. BMJ. (2016) 353:i2716. doi: 10.1136/bmj.i2716
47. Nieman KM, Rains TM, Dicklin MR, Maki KC. Wheat fiber in postprandial metabolic profile health. In: Watson RR, Preedy VR, Zibadi S, editors. Wheat Rice in Disease Prevention Health. London; Waltham, MA; San Diego: Academic Press; Elsevier (2014) p. 59–66. doi: 10.1016/B978-0-12-401716-0.00005-2
48. Chanson-Rolle A, Meynier A, Aubin F, Lappi J, Poutanen K, Braesco V. Systematic review and meta-analysis of human studies to support a quantitative recommendation for whole grain intake in relation to type 2 diabetes. PLoS ONE. (2015) 10:e0131377. doi: 10.1371/journal.pone.0131377
49. Aune D, Norat T, Romundstad P, Vatten L. Whole grain and refined grain consumption and the risk of type 2 diabetes: a systematic review and dose-response meta-analysis of cohort studies. Eur J Epidemiol. (2013) 28:845–58. doi: 10.1007/s10654-013-9852-5
50. Svihus B. Nutritive digestive effects of starch fiber in whole wheat. In: Watson RR, Preedy VR, Zibadi S, editors. Wheat Rice in Disease Prevention Health. London; Waltham, MA; San Diego: Academic Press; Elsevier (2014). p. 81–87. doi: 10.1016/B978-0-12-401716-0.00007-6
52. Triboi E, Martre P, Triboi-Blondel AM. Environmentally-induced changes in protein composition in developing grains of wheat are related to changes in total protein content. J Exp Bot. (2003) 54:1731–42. doi: 10.1093/jxb/erg183
53. Groos C, Robert N, Bervas E, Charmet G. Genetic analysis of grain protein-content, grain yield and thousand-kernel weight in bread wheat. Theor Appl Genet. (2003) 106:1032–40. doi: 10.1007/s00122-002-1111-1
54. Shewry P. What is gluten – why is it special? Front Nutr. (2019) 6:101. doi: 10.3389/fnut.2019.00101
55. Wieser H, Koehler P, Konitzer K. Gluten – the precipitating factor. In: Celiac disease and gluten – Multidisciplinary challenges and opportunities. 1st ed. London; Waltham, MA; San Diego: Academic Press; Elsevier (2014). p. 97–148. doi: 10.1016/B978-0-12-420220-7.00002-X
56. Scherf KA. Immunoreactive cereal proteins in wheat allergy, non-celiac gluten/wheat sensitivity (NCGS) and celiac disease. Curr Opin Food Sci. (2019) 25:35–41. doi: 10.1016/j.cofs.2019.02.003
57. Brijs K, Courtin CM, Goesaert H, Gebruers K, Delcour JA, Shewry PR, et al. Enzymes and enzyme inhibitors endogenous to wheat. In: Khan K, Shewry PR, et al. Wheat – Chemistry and Technology. St Paul, MN: AACC (2009). p. 401–35. doi: 10.1094/9781891127557.011
58. Altenbach SB, Vensel WH, Dupont FM. The spectrum of low molecular weight α-amylase/protease inhibitor genes expressed in the US bread wheat cultivar Butte 86. BMC Res Notes. (2011) 4:242–54. doi: 10.1186/1756-0500-4-242
59. Dupont FM, Vensel WH, Tanaka CK, Hurkman WJ, Altenbach SB. Deciphering the complexities of the wheat flour proteome using quantitative two-dimensional electrophoresis, three proteases and tandem mass spectrometry. Proteome Sci. (2011) 9:10. doi: 10.1186/1477-5956-9-10
60. FAO/WHO/UNO. Protein and amino acid requirements in human nutrition. WHO Press (2007). p. 265. Available online at: https://www.who.int/nutrition/publications/nutrientrequirements/WHO_TRS_935/en/
61. Chick H. Biological value of the proteins contained in wheat flours. Lancet. (1942) 242:405–8. doi: 10.1016/S0140-6736(00)79573-0
62. Scherf KA, Koehler P, Wieser H. Gluten and wheat sensitivities – an overview. J Cereal Sci. (2016) 67:2–11. doi: 10.1016/j.jcs.2015.07.008
63. González-Thuillier I, Salt L, Chope G, Penson S, Skeggs P, Tosi P, et al. Distribution of lipids in the grain of wheat (cv. Hereward) determined by lipidomic analysis of milling and pearling fractions. J Agric Food Chem. (2015) 63:10705–16. doi: 10.1021/acs.jafc.5b05289
64. Schaffarczyk M, Østdal H, Koehler P. Lipases in wheat breadmaking: analysis functional effects of lipid reaction products. J Agric Food Chem. (2014) 62:8229–37. doi: 10.1021/jf5022434
65. Chen Y, Ross AB, Aman P, Kamal-Eldin A. Alkylresorcinols as markers of whole grain wheat and rye in cereal products. J Agric Food Chem. (2004) 52:8242–6. doi: 10.1021/jf049726v
66. Koskela A, Samaletdin A, Aubertin-Leheudre M, Adlercreutz H. Quantification of alkylresorcinol metabolites in plasma by high-performance liquid chromatography with coulometric electrode array detection. J Agric Food Chem. (2008) 56:7678–81. doi: 10.1021/jf801252s
67. Shewry PR, Van Schaik F, Ravel C, Charmet G, Rakszegi M, Bedo Z, et al. Genotype and environment effects on the contents of vitamins B1, B2, B3, and B6 in wheat grain. J Agric Food Chem. (2011) 59:10564–71. doi: 10.1021/jf202762b
68. Liu RH. Whole grain phytochemicals and health. J Cereal Sci. (2007) 46:207–19. doi: 10.1016/j.jcs.2007.06.010
69. Chung OK, Ohm JB, Ram MS, Park SH, Howitt CA. Wheat Lipids. In Khan K, Shewry PR, editors. Wheat: chemistry technology, 4th ed. St Paul, MN: AACC (2009). p. 363–99. doi: 10.1094/9781891127557.010
70. Piironen V, Lampi AM, Ekholm P, Salmenkallio-Marttila M, Liukkonen H, et al. Micronutrients phytochemicals in wheat grain. In: Khan K, Shewry PR, editors. Wheat: chemistry and technology, 4th ed. St Paul, MN: AACC (2009). p. 179–222. doi: 10.1094/9781891127557.007
71. Beta T, Nam S, Dexter JE, Sapirstein HD. Phenolic content and antioxidant activity of pearled wheat and roller-milled fractions. Cereal Chem. (2005) 82:390–3. doi: 10.1094/CC-82-0390
72. Zhu Y, Sang S. Phytochemicals in whole grain wheat and their health-promoting effects. Mol Nutr Food Res. (2017) 61:1600852. doi: 10.1002/mnfr.201600852
73. Badawy D, El-Bassossy HM, Fahmy A, Azhar A. Aldolase reductase inhibitors zopolrestat and ferulic acid alleviate hypertension associated with diabetes: effect on vascular reactivity. Can J Physiol Pharmacol. (2013) 91:101–7. doi: 10.1139/cjpp-2012-0232
74. Drankhan K, Carter J, Madl R, Klopfenstein C, Padula F, Lu Y. Antitumor activity of wheat with high orthophenolic content. Nutr Cancer. (2003) 47:188–94. doi: 10.1207/s15327914nc4702_12
75. Hemdane S, Jacobs PJ, Dornez E, Verspreet J, Delcour JA, Courtin CM. Wheat (Triticum aestivum L.) bran in bread making: a critical review. Compr Rev Food Sci Food Safety. (2016) 15:28–42. doi: 10.1111/1541-4337.12176
76. OECD-FAO. Agricultural Outlook OECD Agriculture Statistics (Database) (2019). Available online at: https://stats.oecd.org/
78. Schuppan D, Zevallos V. Wheat amylase trypsin inhibitors as nutritional activators of innate immunity. Dig Dis. (2015) 33:260–3. doi: 10.1159/000371476
79. Singh P, Arora A, Strand TA, Leffler DA, Catassi C, Green PH, et al. Global prevalence of celiac disease: systematic review and meta-analysis. Clin Gastroenterol Hepatol. (2018) 16:823–36. doi: 10.1016/j.cgh.2017.06.037
80. Ludvigsson JF, Murray JA. Epidemiology of celiac disease. Gastroenterol. Clin. North Am. (2019) 11:54–60. doi: 10.1016/j.gtc.2018.09.004
81. Plugis NM, Khosla C. Therapeutic approaches for celiac disease. Best Pract Res Clin Gastroenterol. (2015) 29:503–21. doi: 10.1016/j.bpg.2015.04.005
82. Huebener S, Tanaka CK, Uhde M, Zone JJ, Vensel WH, Kasarda DD, et al. Specific nongluten proteins of wheat are novel target antigens in celiac disease humoral response. J Proteome Res. (2015) 14:503–11. doi: 10.1021/pr500809b
83. Junker Y, Zeissig S, Kim SJ, Barisani D, Wieser H, Leffler DA, et al. Wheat amylase trypsin inhibitors drive intestinal inflammation via activation of toll-like receptor 4. J Exp Med. (2012) 209:2395–408. doi: 10.1084/jem.20102660
84. Abadie V, Sollid LM, Barreiro LB, Jabri B. Integration of genetic and immunological insights into a model of celiac disease pathogenesis. Annu Rev Immunol. (2011) 29:493–525. doi: 10.1146/annurev-immunol-040210-092915
85. Ricano-Ponce I, Wijmenga C, Gutierrez-Achury J. Genetics of celiac disease. Best Pract Res Clin Gastroenterol. (2015) 29:399–412. doi: 10.1016/j.bpg.2015.04.004
86. Koning F. Celiac disease: quantity matters. Semin Immunopathol. (2012) 34:541–9. doi: 10.1007/s00281-012-0321-0
87. Pes GM, Bibbo S, Dore MP. Celiac disease: beyond genetic susceptibility and gluten. Narrative Rev Ann Med. (2019) 9:1–47. doi: 10.1080/07853890.2019.1569254
88. Bach JF. The hygiene hypothesis in autoimmunity: the role of pathogens and commensals. Nat Rev Immunol. (2018) 18:105–20. doi: 10.1038/nri.2017.111
89. Lebwohl B, Murray JA, Verdu EF, Crowe SE, Dennis M, Fasano A, et al. Gluten introduction, breastfeeding, and celiac disease: back to the drawing board. Am J Gastroenterol. (2016) 111:12–4. doi: 10.1038/ajg.2015.219
90. Szajewska H, Shamir R, Mearin L, Ribes-Koninckx C, Catassi C, Domellöf M, et al. Gluten introduction and the risk of coeliac disease: a position paper by the european society for pediatric gastroenterology, hepatology, and nutrition. J Pediatr Gastroenterol Nutr. (2016) 62:507–13. doi: 10.1097/MPG.0000000000001105
91. Meijer C, Shamir R, Szajewska H, Mearin L. Celiac disease prevention. Front Pediatr. (2018) 6:368. doi: 10.3389/fped.2018.00368
92. Green PH, Krishnareddy S, Lebwohl B. Clinical manifestations of celiac disease. Dig Dis. (2015) 33:137–40. doi: 10.1159/000370204
93. Leffler DA, Green PH, Fasano A. Extraintestinal manifestations of coeliac disease. Nat Rev Gastroenterol Hepatol. (2015) 12:561–71. doi: 10.1038/nrgastro.2015.131
94. Oberhuber G, Granditsch G, Vogelsang H. The histopathology of coeliac disease: time for a standardized report scheme for pathologists. Eur J Gastroenterol. Hepatol. (1999) 11:1185–94. doi: 10.1097/00042737-199910000-00019
95. Conti L, Lahner E, Galli G, Esposito G, Carabotti M, Annibale B. Risk factors associated with the occurrence of autoimmune diseases in adult coeliac patients. Gastroenterol Res Pract. (2018) 2018:3049286. doi: 10.1155/2018/3049286
96. Kaukinen K, Lindfors K, Collin P, Koskinen O, Mäki M. Coeliac disease – a diagnostic and therapeutic challenge. Clin Chem Lab Med. (2010) 48:1205–16. doi: 10.1515/cclm.2010.241
97. Kim HS, Unalp-Arida A, Ruhl CE, Choung RS, Murray JA. Autoimmune and allergic disorders are more common in people with celiac disease or on a gluten-free diet in the United States. J Clin Gastroenterol. (2019) 53:e416–23. doi: 10.1097/MCG.0000000000001100
98. Malamut G, Cellier C. Refractory celiac disease. Gastroenterol Clin North Am. (2019) 48:137–44. doi: 10.1016/j.gtc.2018.09.010
99. Husby S, Koletzko S, Korponay-Szabó I, Kurppa K, Mearin ML, Ribes-Koninckx C, et al. European society paediatric gastroenterology, hepatology nutrition guidelines for diagnosing coeliac disease 2020. J Pediatr Gastroenterol Nutr. (2020) 70:141–56. doi: 10.1097/MPG.0000000000002497
100. Reilly N, Husby S, Sanders DS, Green PH. Coeliac disease: to biopsy or not? Nat Rev Gastroenterol Hepatol. (2018) 15:60–6. doi: 10.1038/nrgastro.2017.121
101. Hujoel IA, Reilly NR, Rubio-Tapia A. Celiac disease: clinical features and diagnosis. Gastroenterol Clin North Am. (2019) 48:19–37. doi: 10.1016/j.gtc.2018.09.001
102. Korponay-Szabó IR, Troncone R, Discepolo V. Adaptive diagnosis of coeliac disease. Best Pract Res Clin Gastroenterol. (2015) 29:381–98. doi: 10.1016/j.bpg.2015.05.003
103. Lundin KE, Wijmenga C. Coeliac disease and autoimmune disease – genetic overlap and screening. Nat Rev Gastroenterol Hepatol. (2015) 12:507–15. doi: 10.1038/nrgastro.2015.136
104. Schuppan D, Junker Y, Barisani D. Celiac disease: from pathogenesis to novel therapies. Gastroenterology. (2009) 137:1912–33. doi: 10.1053/j.gastro.2009.09.008
105. Valitutti F, Fasano A. Breaking down barriers: how understanding celiac disease pathogenesis informed the development of novel treatments. Dig Dis Sci. (2019) 64:1748–58. doi: 10.1007/s10620-019-05646-y
106. Goodman RE, Ebisawa M, Ferreira F, Sampson HA, van Ree R, Vieths S, et al. AllergenOnline: A peer-reviewed, curated allergen database to assess novel food proteins for potential cross-reactivity. Mol Nutr Food Res. (2016) 60:1183–98. doi: 10.1002/mnfr.201500769
107. Dieterich W, Ehnis T, Bauer M, Donner P, Volta U, Riecken EO, et al. Identification of tissue transglutaminase as the autoantigen of celiac disease. Nat Med. (1997) 3:797–801. doi: 10.1038/nm0797-797
108. Fleckenstein B, Qiao SW, Larsen MR, Jung G, Roepstorff P, Sollid LM. Molecular characterization of covalent complexes between tissue transglutaminase and gliadin peptides. J Biol Chem. (2004) 279:17607–16. doi: 10.1074/jbc.M310198200
109. Lexhaller B, Ludwig C, Scherf KA. Identification of isopeptides between human tissue transglutaminase and wheat, rye, and barley gluten peptides. Sci Rep. (2020) 10:7426. doi: 10.1038/s41598-020-64143-9
110. Vader LW, de Ru A, van der Wal Y, Kooy YMC, Benckhuijsen W, Mearin ML, et al. Specificity of tissue transglutaminase explains cereal toxicity in celiac disease. J Exp Med. (2002) 195:643–9. doi: 10.1084/jem.20012028
111. Sollid LM, Iversen R, Steinsbo O, Qiao SW, Bergsen E, Dorum S, et al. Small bowel celiac disease and adaptive immunity. Dig Dis. (2015) 33:115–21. doi: 10.1159/000369512
112. Kim SM, Mayassi T, Jabri B. Innate immunity: actuating the gears of celiac disease pathogenesis. Best Pract Res Clin Gastroenterol. (2015) 29:425–35. doi: 10.1016/j.bpg.2015.05.001
113. Rai S, Kaur A, Chopra CS. Gluten-free products for celiac susceptible people. Front Nutr. (2018) 5:116. doi: 10.3389/fnut.2018.00116
114. White LE, Bannerman E, Gillett PM. Coeliac disease and the gluten-free diet: a review of the burdens; factors associated with adherence and impact on health-related quality of life, with specific focus on adolescence. J Hum Nutr Diet. (2016) 29:593–606. doi: 10.1111/jhn.12375
115. Serena G, Kelly CP, Fasano A. Nondietary therapies for celiac disease. Gastroenterol Clin North Am. (2019) 48:145–63. doi: 10.1016/j.gtc.2018.09.011
116. Reunala T, Salmi TT, Hervonen K, Kaukinen K, Collin P. Dermatitis herpetiformis: a common extraintestinal manifestation of coeliac disease. Nutrients. (2018) 10:e602. doi: 10.3390/nu10050602
117. Hadjivassiliou M, Sanders DD, Aeschlimann DP. Gluten-related disorders: gluten ataxia. Dig Dis. (2015) 33:264–8. doi: 10.1159/000369509
118. Sapone A, Bai JC, Ciacci C, Dolinsek J, Green PH, Hadjivassiliou M, et al. Spectrum of gluten-related disorders: consensus on new nomenclature and classification. BMC Med. (2012) 10:13. doi: 10.1186/1741-7015-10-13
119. Pasha I, Saeed F, Sultan MT, Batool R, Aziz M, Ahmed W. Wheat allergy and intolerance: recent updates and perspectives. Crit Rev Food Sci Nutr. (2016) 5:13–24. doi: 10.1080/10408398.2012.659818
120. Brouns F, van Rooy G, Shewry P, Rustgi S, Jonkers D. Adverse reactions to wheat or wheat components. Compr Rev Food Sci Food Saf. (2019) 18:1437–52. doi: 10.1111/1541-4337.12475
121. Juhasz A, Belova T, Florides CG, Maulis C, Fischer I, Gell G, et al. Genome mapping of seed-borne allergens and immunoresponsive proteins in wheat. Sci Adv. (2018) 4:eaar8602. doi: 10.1126/sciadv.aar8602
122. Tatham AS, Shewry PR. Allergens to wheat and related cereals. Clin Exp Allergy. (2008) 38:1712–26. doi: 10.1111/j.1365-2222.2008.03101.x
123. Nwaru BI, Hickstein L, Panesar SS, Roberts G, Muraro A, Sheikh A. Prevalence of common food allergies in Europe: a systematic review and meta- analysis. Allergy. (2014) 69:992–1007. doi: 10.1111/all.12423
124. Muraro A, Werfel T, Hoffmann-Sommergruber K, Roberts G, Beyer K, Bindslev-Jensen C, et al. EAACI food allergy and anaphylaxis guidelines: diagnosis and management of food allergy. Allergy. (2014) 69:1008–25. doi: 10.1111/all.12429
125. Sicherer SH, Sampson HA. Food allergy: epidemiology, pathogenesis, diagnosis, and treatment. J Allergy Clin Immunol. (2014) 133:291–307. doi: 10.1016/j.jaci.2013.11.020
126. Hawrylowicz CM, O'Garra A. Potential role of interleukin−10-secreting regulatory T cells in allergy and asthma. Nat Rev Immunol. (2005) 5:271–83. doi: 10.1038/nri1589
127. Sander I, Flagge A, Merget R, Halder TM, Meyer HE, Baur X. Identification of wheat flour allergens by means of 2-dimensional immunoblotting. J Allergy Clin Immunol. (2001) 107:907–13. doi: 10.1067/mai.2001.113761
128. Bhatia R, Alikhan A, Maibach HI. Contact urticaria: present scenario. Indian J Dermatol. (2009) 54:264–8. doi: 10.4103/0019-5154.55639
129. Matsuo H, Uemura M, Yorozuya M, Adachi A, Morita E. Identification of IgE-reactive proteins in patients with wheat protein contact dermatitis. Contact Dermatitis. (2010) 63:23–30. doi: 10.1111/j.1600-0536.2010.01741.x
130. Codex Standard 1-1985. Codex Alimentarius Commission. Amendment 7 (2010). Available online at: http://www.fao.org/3/Y2770e/y2770e02.htm#bm02
131. Hischenhuber C, Crevel R, Jarry B, Maeki M, Moneret-Vautrin DA, Romano A, et al. Review article: safe amounts of gluten for patients with wheat allergy or coeliac disease. Aliment Pharmacol Therapeut. (2006) 23:559–75. doi: 10.1111/j.1365-2036.2006.02768.x
132. Mäkelä MJ, Eriksson C, Kotaniemi-Syrjaenen A, Palosuo K, Marsh J, Borres M, et al. Wheat allergy in children – new tools for diagnostics. Clin Exp Allergy. (2014) 44:1420–30. doi: 10.1111/cea.12393
133. Battais F, Pineau F, Popineau Y, Aparicio C, Kanny G, Moneret-Vautrin DA, et al. Food allergy to wheat: identification of immunoglobulin E and immunoglobulin G-binding proteins with sequential extracts and purified proteins from wheat flour. Clin Exp Allergy. (2003) 33:962–70. doi: 10.1046/j.1365-2222.2003.01592.x
134. Scherf KA, Brockow K, Biedermann T, Koehler P, Wieser H. Wheat-dependent exercise-induced anaphylaxis. Clin Exp Allergy. (2016) 46:10–20. doi: 10.1111/cea.12640
135. Wölbing F, Fischer J, Köberle M, Kaesler S, Biedermann T. About the role and underlying mechanismens of cofactors in anaphylaxis. Allergy. (2013) 68:1085–92. doi: 10.1111/all.12193
136. Yokooji T, Kurihara S, Murakami T, Chinuki Y, Takahashi H, Morita E, et al. Characterization of causative allergens for wheat-dependent exercise-induced anaphylaxis sensitized with hydrolyzed wheat proteins in facial soap. Allergol Int. (2013) 62:435–45. doi: 10.2332/allergolint.13-OA-0561
137. Suzuki T. Regulation of intestinal epithelial permeability by tight junctions. Cell Mol Life Sci. (2013) 70:631–59. doi: 10.1007/s00018-012-1070-x
138. Turnbull JL, Adams HN, Gorard DA. Review article: the diagnosis and management of food allergy and food intolerances. Aliment Pharmacol Therapeut. (2015) 41:3–25. doi: 10.1111/apt.12984
139. Yu Y, Blokhuis BR, Garssen J, Redegeld FA. Non-IgE mediated mast cell activation. Eur. J. Pharmacol. (2016) 778:33–43. doi: 10.1016/j.ejphar.2015.07.017
140. Catassi C, Alaedini A, Bojarski C, Bonaz B, Bouma G, Carroccio A, et al. The overlapping area of non-celiac gluten sensitivity (NCGS) and wheat-sensitive irritable bowel syndrome (IBS): an update. Nutrients. (2017) 9:1268. doi: 10.3390/nu9111268
141. Volta U, de Giorgio R, Caio G, Uhde M, Manfredini R, Alaedini A. Nonceliac wheat sensitivity: an immune-mediated condition with systemic manifestations. Gastroenterol Clin North Am. (2019) 48:165–82. doi: 10.1016/j.gtc.2018.09.012
142. Mansueto P, Seidita A, D'Alcamo A, Carroccio A. Non-celiac gluten sensitivity: literature review. J Am Coll Nutr. (2014) 33:39–54. doi: 10.1080/07315724.2014.869996
143. Losurdo G, Principi M, Iannone A, Amoruso A, Ierardi E, di Leo A, et al. Extra-intestinal manifestations of non-celiac gluten sensitivity: an expanding paradigm. World J Gastroenterol. (2018) 24:1521–30. doi: 10.3748/wjg.v24.i14.1521
144. Di Sabatino A, Volta U, Salvatore C, Biancheri P, Caio G, de Giorgio R, et al. Small amounts of gluten in subjects with suspected nonceliac gluten sensitivity: a randomized, double-blind, placebo-controlled, cross-over trial. Clin Gastroenterol Hepatol. (2015) 13:1604–12.e3. doi: 10.1016/j.cgh.2015.01.029
145. Gibson PR, Muir JG, Newnham ED., Other dietary confounders: FODMAPS, et al. Dig Dis. (2015) 33:269–76. doi: 10.1159/000371401
146. Zevallos VF, Raker V, Tenzer S, Jimenez-Calvente C, Ashfaq-Khan M, Rüssel N, et al. Nutritional wheat amylase-trypsin inhibitors promote intestinal inflammation via activation on myeloid cells. Gastroenterology. (2017) 152:1100–13.e12. doi: 10.1053/j.gastro.2016.12.006
147. Branchi F, Aziz I, Conte D, Sanders DS. Noncoeliac gluten sensitivity: a diagnostic dilemma. Curr Opin Clin Nutr Metab Care. (2015) 18:508–14. doi: 10.1097/MCO.0000000000000207
148. Catassi C, Elli L, Bonaz B, Bouma G, Carroccio A, Castillejo G, et al. Diagnosis of non-celiac gluten sensitivity (NCGS): the Salerno experts‘ criteria. Nutrients. (2015) 7:4966–77. doi: 10.3390/nu7064966
149. Rej A, Sanders DS. Gluten-free diet and its ‘cousins’ in irritable bowel syndrome. Nutrients. (2018) 10:e1727. doi: 10.3390/nu10111727
150. Chey W, Kurlander J, Eswaran S. Irritable bowel syndrome: a clinical review. JAMA. (2015) 313:949–58. doi: 10.1001/jama.2015.0954
151. Borghini R, Donato G, Alvaro D, Picarelli A. New insights in IBS-like disorders. Pandora's box has been opened; a review. Gastroenterol Hepatol Bed Bench. (2017) 10:79–89.
152. Drossmann DA. Functional gastrointestinal disorders: history, pathophysiology, clinical features and Rome IV criteria. Gastroenterology. (2016) 150:1262–79. doi: 10.1053/j.gastro.2016.02.032
153. De Giorgio R, Volta U, Gibson PR. Sensitivity to wheat, gluten and FODMAPs in IBS: facts or fiction? Gut. (2016) 65:169–78. doi: 10.1136/gutjnl-2015-309757
154. Volta U, Pinto-Sanchez MI, Boschetti E, Caio G, de Giorgio R, Verdu EF. Dietary triggers in irritable bowel syndrome: is there a role for gluten? J Neurogastroenterol Motil. (2016) 22:547–57. doi: 10.5056/jnm16069
155. Zanwar VG, Pawar SV, Gambhire PA, Jain SS, Surude RG, Shah VB, et al. Symptomatic improvement with gluten restriction in irritable bowel syndrome: a prospective, randomized, double-blinded placebo controlled trial. Intest Res. (2016) 14:343–50. doi: 10.5217/ir.2016.14.4.343
156. Longstreth GF, Thompson WG, Chey WD, Houghton LA, Mearin F, Spiller RC. Functional bowel disorders. Gastroenterology. (2006) 130:1480–91. doi: 10.1053/j.gastro.2005.11.061
157. Bellini M, Gambaccini D, Stasi C, Urbano, MT, Marchi S, Usai-Satta P. Irritable bowel syndrome: a disease still searching for pathogenesis, diagnosis and therapy. World J Gastroenterol. (2014) 20:8807–20. doi: 10.3748/wjg.v20.i27.8807
160. Tanpowpong P, Ingham TR, Lampshire PK, Kirchberg FF, Epton MJ, Crane J, et al. Coeliac disease and gluten avoidance in New Zealand children. Arch Dis Child. (2012) 97:12–6. doi: 10.1136/archdischild-2011-300248
161. Statista Database (2018). Available online at: https://www.statista.com/statistics/819377/global-gluten-free-retail-sales/
162. Jones J. Wheat belly – an analysis of selected statements and basic theses from the book. Cereal Foods World. (2012) 57:177–89. doi: 10.1094/CFW-57-4-0177
163. Brouns FJ, van Buul VJ, Shewry PR. Does wheat make us fat and sick? J Cereal Sci. (2013) 58:209–15. doi: 10.1016/j.jcs.2013.06.002
164. Gaesser GA, Angadi SS. Navigating the gluten-free boom. JAAPA. (2015) 28:1–7. doi: 10.1097/01.JAA.0000469434.67572.a4
165. Rustgi S, Shewry P, Brouns F, Deleu LJ, Delcour JA. Wheat seed proteins: factors influencing their content, composition, and technological properties, and strategies to reduce adverse reactions. Compr Rev Food Sci Food Saf. (2019) 18:1751–69. doi: 10.1111/1541-4337.12493
166. Tieri M, Ghelfi F, Vitale M, Vetrani C, Marventano S, Lafranconi A, et al. Whole grain consumption human health: an umbrella review of observational studies. Int J Food Sci Nutr. (2020) 71:668–77. doi: 10.1080/09637486.2020.1715354
167. Grode L, Bech BH, Jensen TM, Humaidan P, Agerholm IE, Plana-Ripoll O, et al. Prevalence, incidence, and autoimmune comorbidities of celiac disease: a nation-wide, population-based study in Denmark from 1977 to 2016. Eur J Gastroenterol Hepatol. (2018) 30:83–91. doi: 10.1097/MEG.0000000000000992
168. Gatti S, Lionetti E, Balanzoni L, Verma AK, Galeazzi T, Gesuita R, et al. Increased prevalence of celiac disease in school-age children in Italy. Clin Gastroenterol Hepatol. (2020) 18:596–603. doi: 10.1016/j.cgh.2019.06.013
169. Almallouhi E, King KS, Patel B, Wi C, Juhn YJ, Murray JA, et al. Increasing incidence and altered presentation in a population-based study of pediatric celiac disease in North America. J Pediatr Gastroenterol Nutr. (2017) 65:432–7. doi: 10.1097/MPG.0000000000001532
170. Fasano A, Sapone A, Zevallos V, Schuppan D. Nonceliac gluten sensitivity. Gastroenterology. (2015) 148:1195–204. doi: 10.1053/j.gastro.2014.12.049
171. Verdu EF, Danska JS. Common ground: shared risk factors for type 1 diabetes and celiac disease. Nat Immunol. (2018) 19:685–95. doi: 10.1038/s41590-018-0130-2
172. Kuehni CE, Strippoli MP, Low N, Silverman M. Asthma in young south Asian women living in the United Kingdom: the importance of early life. Clin Exp Allergy. (2007) 37:47–53. doi: 10.1111/j.1365-2222.2006.02627.x
173. Hanski I, von Hertzen L, Fyhrquist N, Koskinen K, Torppa K, Laatikainen T, et al. Environmental biodiversity, human microbiota, and allergy are interrelated. Proc Nat Acad Sci USA. (2012) 109:8334–9. doi: 10.1073/pnas.1205624109
174. Lambrecht B, Hammad H. The immunology of the allergy epidemic and the hygiene hypothesis. Nat Immunol. (2017) 18:1076–83. doi: 10.1038/ni.3829
175. Rook GA. Hygiene hypothesis and autoimmune diseases. Clin Rev Allergy Immunol. (2012) 42:5–15. doi: 10.1007/s12016-011-8285-8
176. Odenwald MA, Turner JR. The intestinal epithelial barrier: a therapeutic target? Nat Rev Gastroenterol Hepatol. (2017) 14:9–21. doi: 10.1038/nrgastro.2016.169
177. Prandi B, Tedeschi T, Folloni S, Galaverna G, Sforza S. Peptides from gluten digestion: a comparison between old and modern wheat varieties. Food Res Int. (2017) 91:92–102. doi: 10.1016/j.foodres.2016.11.034
178. Geisslitz S, Ludwig C, Scherf KA, Koehler P. Targeted LC-MS/MS reveals similar contents of α-amylase/trypsin-inhibitors as triggers of non-celiac gluten sensitivity in all wheat species except einkorn. J Agric Food Chem. (2018) 66:12395–403. doi: 10.1021/acs.jafc.8b04411
179. Geisslitz S, Longin CFH, Scherf KA, Koehler P. Comparative study on gluten protein composition of ancient (einkorn, emmer and spelt) and modern wheat species (durum and common wheat). Foods. (2019) 8:409. doi: 10.3390/foods8090409
180. Schalk K, Lang C, Wieser H, Koehler P, Scherf KA. Quantitation of the immunodominant 33-mer peptide from α-gliadin in wheat flours by liquid chromatography tandem mass spectrometry. Sci Rep. (2017) 7:45092. doi: 10.1038/srep45092
181. Ziegler JU, Schweiggert RM, Würschum T, Longin CFH, Carle R. Lipophilic antioxidants in wheat (Triticum spp.): a target for breeding new varieties for future functional cereal products. J Func Foods. (2016) 20:594–605. doi: 10.1016/j.jff.2015.11.022
182. Ribeiro M, Rodriguez-Quijano M, Nunes FM, Carrillo JM, Branlard G, Igrejas G. New insights into wheat toxicity: breeding did not seem to contribute to a prevalence of potential celiac disease‘s immunostimulatory epitopes. Food Chem. (2016) 213:8–18. doi: 10.1016/j.foodchem.2016.06.043
183. Hucl P, Briggs C, Graf RJ, Chibbar RN. Genetic gains in agronomic and selected end-use quality traits over a century of plant breeding of canada western red spring wheat. Cereal Chem. (2015) 92:537–43. doi: 10.1094/CCHEM-02-15-0029-R
184. Kasarda DD. Can an increase in celiac disease be attributed to an increase in the gluten content of wheat as a consequence of wheat breeding? J Agric Food Chem. (2013) 61:1155–9. doi: 10.1021/jf305122s
185. Malalgoda M, Meinhardt SW, Simsek S. Detection and quantitation of immunogenic epitopes related to celiac disease in historical and modern hard red spring wheat cultivars. Food Chem. (2018) 264:101–7. doi: 10.1016/j.foodchem.2018.04.131
186. Shewry PR, Pellny TK, Lovegrove A. Is modern wheat bad for health? Nat Plants. (2016) 2:16097. doi: 10.1038/nplants.2016.97
187. Gulati P, Brahma S, Graybosch RA, Chen Y, Rose DJ. In vitro digestibility of proteins from historical and modern wheat cultivars. J Sci Food Agric. (2020) 100:2579–84. doi: 10.1002/jsfa.10283
188. Laidig F, Piepho H-P, Rentel D, Drobek T, Meyer U, Huesken A. Breeding progress, environmental variation and correlation of winter wheat yield and quality traits in German official variety trials and on-farm during 1983-2014. Theor Appl Genet. (2017) 130:223–45. doi: 10.1007/s00122-016-2810-3
189. Pronin D, Börner A, Weber H, Scherf KA. Wheat (Triticum aestivum L.) breeding from 1891 to 2010 contributed to increasing yield glutenin contents but decreasing protein gliadin contents. J Agric Food Chem. (2020). doi: 10.1021/acs.jafc.0c02815. [Epub ahead of print].
190. Ozuna CV, Barro F. Characterization of gluten proteins and celiac disease-related immunogenic epitopes in the Triticeae: cereal domestication and breeding contributed to decrease the content of gliadins and gluten. Mol Breeding. (2018) 38:22. doi: 10.1007/s11032-018-0779-0
191. Pronin D, Geisslitz S, Börner A, Scherf KA. Fingerprinting of wheat protein profiles for improved distinction between wheat cultivars and species. Cereal Chem. (2020). 97:999–1009. doi: 10.1002/cche.10323
192. Ortolan F, Steel CJ. Protein characteristics that affect the quality of vital wheat gluten to be used in baking: a review. Compr Rev Food Sci Food Safety. (2017) 16:369–81. doi: 10.1111/1541-4337.12259
193. Kucek LK, Veenstra LD, Amnuaycheewa P, Sorrells ME. A grounded guide to gluten: how modern genotypes and processing impact wheat sensitivity. Compr Rev Food Sci Food Saf. (2015) 14:285–302. doi: 10.1111/1541-4337.12129
194. Lionetti E, Catassi C. Co-localization of gluten consumption and HLA-DQ2 and-DQ8 genotypes, a clue to the history of celiac disease. Dig Liv Dis. (2014) 46:1057–63. doi: 10.1016/j.dld.2014.08.002
195. Biesiekierski JR, Peters SL, Newnham ED, Rosella O, Muir JG, Gibson PR. No effects of gluten in patients with self-reported non-celiac gluten sensitivity after dietary reduction of fermentable, poorly absorbed, short-chain carbohydrates. Gastroenterology. (2013) 145:320–8. doi: 10.1053/j.gastro.2013.04.051
196. Carroccio A, Mansueto P, Iacono G, Soresi M, D'Alcamo A, Cavataio F, et al. Non-celiac wheat sensitivity diagnosed by double-blind placebo-controlled challenge: exploring a new clinical entity. Am J Gastroenterol. (2012) 107:1898–906. doi: 10.1038/ajg.2012.236
197. Zanini B, Baschè R, Ferraresi A, Ricci C, Lanzarotto F, Marullo M, et al. Randomised clinical study: gluten challenge induces symptom recurrence in only a minority of patients who meet clinical criteria for non-coeliac gluten sensitivity. Aliment Pharmacol Therapeut. (2015) 42:968–76. doi: 10.1111/apt.13372
198. Skodje GI, Sarna VK, Minelle IH, Rolfsen KL, Muir JG, Gibson PR, et al. Fructan, rather than gluten, induces symptoms in patients with self-reported non-celiac gluten sensitivity. Gastroenterology. (2018) 154:529–39. doi: 10.1053/j.gastro.2017.10.040
199. Dieterich W, Zopf Y. Gluten and FODMAPS - Sense of a restriction/When is restriction necessary? Nutrients. (2019) 11:1957. doi: 10.3390/nu11081957
200. Dieterich W, Schuppan D, Schink M, Schwappacher R, Wirtz S, Agaimy A, et al. Influence of low FODMAP and gluten-free diets on disease activity and intestinal microbiota in patients with non-celiac gluten sensitivity. Clin Nutr. (2019) 38:697–707. doi: 10.1016/j.clnu.2018.03.017
201. Muir JG, Varney JE, Ajamian M, Gibson PR. Gluten-free and low-FODMAP sourdoughs for patients with coeliac disease and irritable bowel syndrome: a clinical perspective. Int J Food Microbiol. (2019) 290:237–46. doi: 10.1016/j.ijfoodmicro.2018.10.016
202. Fry L, Madden AM, Fallaize R. An investigation into the nutritional composition and cost of gluten-free versus regular food products in the UK. An investigation into the nutritional composition and cost of gluten-free versus regular food products in the UK. J Hum Nutr Diet. (2018) 31:108–20. doi: 10.1111/jhn.12502
Keywords: allergy, baking, breeding, celiac disease, gluten, non-celiac gluten sensitivity (NCGS), nutritional value, wheat
Citation: Wieser H, Koehler P and Scherf KA (2020) The Two Faces of Wheat. Front. Nutr. 7:517313. doi: 10.3389/fnut.2020.517313
Received: 03 December 2019; Accepted: 16 September 2020;
Published: 21 October 2020.
Edited by:
José M. Alvarez-Suarez, University of the Americas, EcuadorReviewed by:
Peter Shewry, Rothamsted Research, United KingdomFrancisco Cabrera-Chavez, Autonomous University of Sinaloa, Mexico
Copyright © 2020 Wieser, Koehler and Scherf. This is an open-access article distributed under the terms of the Creative Commons Attribution License (CC BY). The use, distribution or reproduction in other forums is permitted, provided the original author(s) and the copyright owner(s) are credited and that the original publication in this journal is cited, in accordance with accepted academic practice. No use, distribution or reproduction is permitted which does not comply with these terms.
*Correspondence: Katharina A. Scherf, a2F0aGFyaW5hLnNjaGVyZkBraXQuZWR1