- 1Plant Bioactive Compound Laboratory (BAC), Department of Plant and Soil Sciences, Faculty of Agriculture, Chiang Mai University, Chiang Mai, Thailand
- 2Cluster of Research and Development of Pharmaceutical and Natural Products Innovation for Human or Animal, Chiang Mai University, Chiang Mai, Thailand
- 3Landscape Design and Environmental Management Studio, Faculty of Agriculture, Chiang Mai, Thailand
- 4Department of Animal and Aquatic Sciences, Faculty of Agriculture, Chiang Mai University, Chiang Mai, Thailand
- 5Department of Pharmaceutical Science, Faculty of Pharmacy, Chiang Mai University, Chiang Mai, Thailand
In this research, a novel source of phytopigment crocins from fully open mature flowers of cape jasmine (Gardenia jasminoides) is introduced. Methanol and deionized water were appropriate solvents for pigment recovery with maximum yields of at least 17% from the floral tissue. Pigment separation by thin layer chromatography also confirmed the presence of the carotenoids, which dissolved well in these high-strength polar solvents, in fruit, flower, and leaf materials. The spectral patterns of the extracts from ultraviolet and nuclear magnetic resonance showed maximum absorption at ~420 nm and the chemical shift values were similar to those of crocetin aglycones (crocins) in the methanol extracts of a commercial source of yellow gardenia (fructus or fruit of Gardenia florida). Chemical compositions were then evaluated using aqueous-phase capillary electrophoresis of the methanol extracts. The methanolic extracts of the flowers and fruit had 11 principal ingredients in common. Among these, crocetin and crocin 2 belong to the crocin group and are known to be the major components of commercial yellow Gardenia. This research not only demonstrates a sustainable means of raw material utilization for natural product recovery, but also encourages a movement toward an edible landscape for the community.
Introduction
Natural dye extracts have been mainly used as colorants, additives, or antioxidants for the food, pharmaceutical, and textile industries for almost two centuries. However, the development of synthetic chemical dyes has brought about almost complete substitution of natural products owing to their durable and functional properties, such as color stability, time efficiency, and convenience (1). Fortunately, the “green” movement has been working against the synthesized dye industry because of the negative impacts on the environment of synthetic additives as well as the adverse effects for humans, e.g., toxic or allergic reactions (2). As a result, ecological and economic restrictions on the toxic chemicals, including bans on certain products containing synthetic dyes, have been established (3). Natural dyes are biodegradable and generally environmentally friendly. They also possess potential properties including antioxidant and antimicrobial activities for commercial applications such as food additives, cosmetics, and pharmaceuticals (4–6). Furthermore, the connection between local resources and humans has been challenged through the widespread use of chemical dyes that encourage the need for conservation of local species (7).
Gardenia is an evergreen shrub used mostly for landscape functions and is known for its sweet, subtly fragrant flowers. A hybrid variety of this plant, i.e., Gardenia jasminoides Ellis or cape jasmine, has been generally used in Thailand for aromatherapy, hair decoration, and religious services (8). There is also a record of the use of the flowers for culinary purposes in Asian countries (9, 10). The fruit of Gardenia (fructus or Zhizhi) (G. florida L.) is well-recognized in Traditional Chinese Medicine because of its bitter flavor and cold properties. As a result, a medicinal formula containing fructus is used to relieve hepatic and abdominal pains with underlying anti-phlogistic, diuretic, laxative, choleretic, and homeostatic properties (11). The fruit is also a major source of yellow coloring as it is a typical plant carotenoid, the major constituents of which are crocin and crocetin (12, 13). The others are blue genipin-derived pigments from reaction with the amine groups (14). The pigments from this well-known Gardenia are utilized as food additives, medicines, and, more recently, natural photosensitizers for dye-sensitized solar cells (15–17) and in the textile industry (3, 17, 18). Besides the extensive use of the fruits as natural pigments, the leaf and floral tissues are also known to have appealing pigments. It has been observed that white, fully open flowers of Gardenia turn an appealing yellow after they reach maturity (19). Moreover, it is generally understood that, because of the complex floral structure of G. jasminoides, natural fruit setting of this common landscape plant is rarely possible (J. F. Maxwell, personal communication).
Despite the above-mentioned studies, there is a lack of information on the chemical characteristics of yellow Gardenia from the floral tissue. This study was, therefore, conducted to characterize the pigments from a substitute raw material source that could replace the use of the propagating materials, i.e., the fruit. The outcomes of this study could also become the platforms for the development of novel environmentally friendly additive products for functional food and textile industries as well as encourage the movement toward a sustainably edible landscape.
Materials and Methods
Plant Materials
Inflorescences of the leaves and flowers of the landscape gardenia (G. jasminoides) were harvested from the botanical collection garden at the Faculty of Agriculture, Chiang Mai University, Thailand. Flowers at their mature stages (yellowish-white, fully open) and leaves (dark green) were selected (Figures 2B1,B2). Gardenia fructus (G. florida) were obtained from a local farm located in Mea Ei District, Chiang Mai, Thailand. They were pest free, clean, ca. 2–3 cm in diameter, 5–6 cm long, and yellow-orange in color (Figure 2A). All materials were dried at 60°C in a hot-air oven for 48 h and stored at room temperature (25°C) in a room without direct sunlight until used. Moisture, fat, and ash contents were analyzed according to AOAC official methods of analysis (20). Plant species were confirmed by a botanical specialist (Dr. J. F. Maxwell, Department of Biology, Faculty of Science, Chiang Mai University, Thailand) using specimens collected from the field. The specimens were also deposited at the herbarium belonging to the Plant Bioactive Compound Laboratory (BACH), Faculty of Agriculture for future reference with voucher record numbers BACH151 and BACH152 for those belonging to G. jasminoides and G. florida, respectively.
Thin-Layer Chromatography
Dried tissues (5.0 g each) were ground to fine particles and soaked in 200 mL of each of the following solvents: deionized water (DIH2O), dichloromethane, ethyl acetate, and methanol for 48 h. The debris from the plant materials was removed and the extract was evaporated to dryness under vacuum. The yield (%) of the extracts was then obtained. To adjust the concentration, dried extract (0.5 mg) was redissolved in 100 μL of DIH2O, chloroform, ethyl acetate, and methanol. Aliquots (5 μL) of the extracts were spotted onto silica gel thin-layer chromatography (TLC) plates (Merk-60 F254, 0.25 mm), dried, and developed in TLC tanks with different types of solvent systems, such polar, medium polar, and non-polar, to separate the polar and non-polar components from the plant materials (21). The mobile phases were (i) benzene–ethyl acetate (18:2) and (ii) chloroform–methanol (19:5).
Ultraviolet and Nuclear Magnetic Resonance Spectra
The absorption spectra of the extracts were evaluated with wavelengths in the range 200–700 nm by means of an ultraviolet (UV)-visible spectrophotometer (SPECTROstar Nano; BMG LABTECH, Offenburg, Germany) (22, 23). For, 1H nuclear magnetic resonance (NMR) spectra, the extracts (1 mg) were dissolved in deuterated dimethyl sulfoxide (DMSO-d6)/deuterium oxide (D2O) and the spectral data were recorded on an Avance 300 MHz NMR spectrometer (Bruker, Rheinstetten, Germany). Structural elucidation of the pigments was accomplished by comparison of the 1H NMR spectra as described by Choi et al. (22) and Sobolev et al. (24).
Capillary Electrophoresis–Mass Spectrometry
For an aqueous system, the background electrolyte consisted of 10 mM ammonium acetate, titrated to pH 4.75 with acetic acid, and a constant positive voltage of +30 kV was applied for separation. The sheath–liquid interface was supplied with a 1:1 mixture of 2-propanol with DIH2O (resistance >18 MΩ) and 1% acetic acid at a rate of 4 μL/min. Capillary electrophoresis–mass spectrometry (CE-MS) was performed using a 7100 Capillary electrophoresis system (Agilent Technologies, Waldbronn, Germany) coupled with quadrupole time-of-flight–mass spectrometry (qToF-MS) and a 6340 Ion Trap (Agilent Technologies, Santa Clara, CA, USA). A bare fused silica capillary (ø 50 μm and length 67.5 cm) was flushed step-wise with the prepared buffers according to the protocol described by Posch et al. (25). Prior to sample injection, the extracts were diluted (1:100) with methanol and injected hydrodynamically for 10 s at 5 kPa. Only the alcoholic extract was chosen for this test as it is has been proven to be effective for the extraction of crocins and other metabolites from the fructus (13, 26–28). The nebulizer was set to 13.8 kPa during injection and vial handling to prevent air bubbles forming; this was subsequently increased to the desired value of 41.4 kPa at the beginning of voltage application. Instrumental settings for the qToF instrument were as described in Posch et al. (25, 29). Tentative identification of the chemical compounds was performed by using their accurate mass from the qToF–MS and the Sequential product-ion spectra (MS3) from the ion trap.
Statistical and Chemometric Analyses
Analyses of the chemical compositions and yields were done in triplicate and reported as the mean ± standard error. Differences between samples were determined by Duncan's multiple range tests in SPSS statistical program v.17 (SPSS Inc., Chicago, IL, USA). Principal component analysis (PCA) was conducted to describe and interpret the interdependence of the data using the 2018 trial version of XLSTAT (New York, NY, USA). A Venn plot was generated with BioVinci software (BioTuring Inc., San Diego, CA, USA).
Results and Discussion
Chemical Compositions and Solvent Extraction of Yellow Dye Additives
Table 1 illustrates the moisture, fat, and ash contents of the leaves, flowers, and fruit of Gardenia spp. The fructus had a fat content as high as 17%, followed by the floral and leaf tissues, respectively. Siwawej and Jarayapun (16) reported that the average fat content of Gardenia fruit without the pericarp was around 20–30%. The ash content was, however, higher in the leaves, followed by fruit and flower tissues (~7%). The value falls within the reference range of ash content in Gardenia fruit (30). Phytopigments are mainly classified into fat-soluble pigments, which are mainly present in the plastid of the plant protoplasm, and water-soluble pigments, which dissolve in the cell sap (6). The fat-soluble pigments are, for instance, chlorophylls and carotenoids and the water-soluble pigments include those belonging to the flavonoids, such as anthoxanthins and anthocyanins (14, 31, 32). It has been generally described that the analyzed fat and ash contents correlate well with the color intensity of both fat- and water-soluble pigments, respectively. Siwawej and Jarayapun (16) described that the lower fat content of Gardenia fruit corresponded to high color absorption intensity. In wheat flour, the yellow pigments were also shown to have a strong relation with ash content (33).
Organic Solvent Extraction and Thin Layer Chromatography
Different solvents were used to extract natural yellow dyes from different Gardenia parts (Table 2). Among the solvent combinations tested, methanol gave the highest yield followed by DIH2O, ethyl acetate, and dichloromethane. Kesavan et al. (30) reported a low yield of Gardenia extracts when low polar organic solvents (namely, ethyl acetate and dichloromethane) were used. The yellow pigment appeared in high polar organic solvents (methanol, DIH2O, and ethyl acetate), mainly from the fruit and floral tissues of Gardenia. Methanol and DIH2O extracts of leaf tissue were green, whereas ethyl acetate extracts of leaf and flower tissues and dichloromethane extracts were black (Table 2).
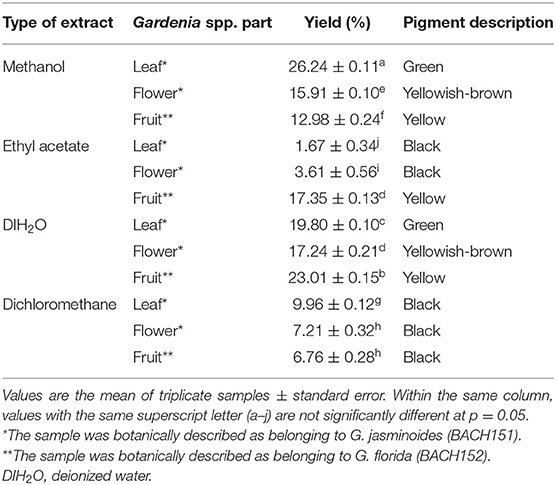
Table 2. Yield (%) and color description of the Gardenia spp. extracts from different parts of the plant.
TLC is the technique used for separation of pigments, including chlorophylls and carotenoids, from plants and other biological substances (34, 35). Different types of extracts were run on the chromatogram plates using hydrophobic mobile-phase systems of different polarity. In our results, it is apparent that chlorophylls from Gardenia leaf extracted with non-polar solvents, namely ethyl acetate (Figure 1A, band 4) and dichloromethane (Figure 1A, band 10), are well-separated in the non-polar mobile phase (benzene–ethyl acetate). The result was in agreement with Katayama et al. (36), who demonstrated that hydrophobic solvents should be used to eliminate water contamination that could prevent clear chromatographic separation of the pigments. Based upon the power of solubility in the mobile phase, a retention factor (Rf) of 1–5 could represent different photosynthesis pigments, including pheophytins a and b, chlorophylls a and b, and xanthophyll (37). In another system, yellow pigments were separated well using methanol and DIH2O extracts of Gardenia fruit (Figure 1B, bands 5–9 and 12–16). Also, bands of flower and leaf samples in the same extracting solvents gave the same Rf values as those from fruit (Rf 8, 12, and 14). In a similar study, six bands representing yellow pigments were isolated from Gardenia fruits using high polar solvents (H2O and methanol), which were later identified as crocin and crocetin of the carotenoid groups (16, 38).
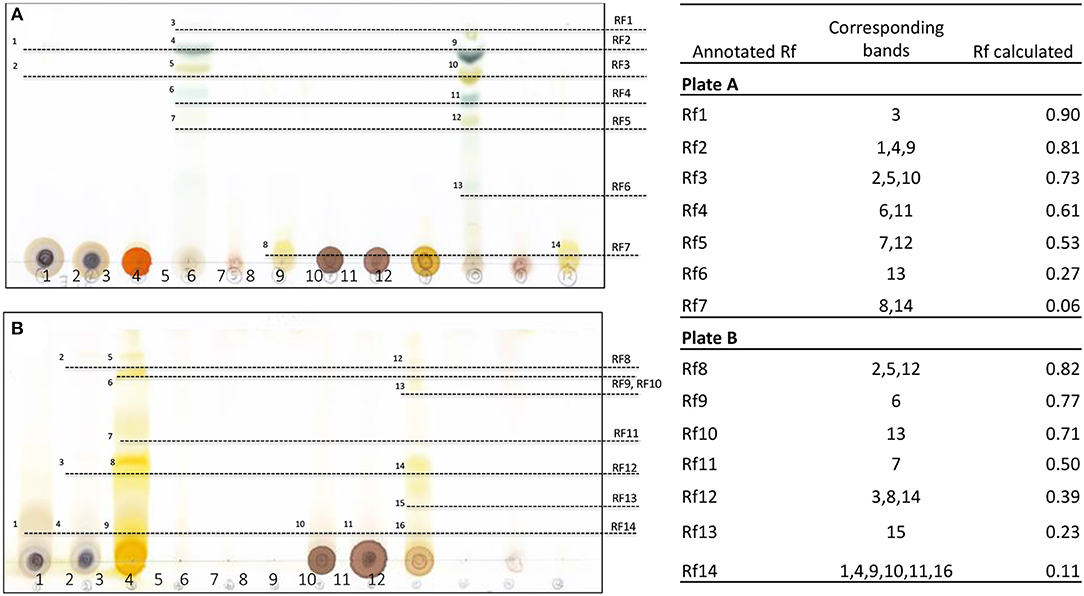
Figure 1. Thin-layer chromatography and calculated retention factor (Rf) values of separated bands of Gardenia extracts run on different mobile phase systems, namely benzene–ethyl acetate (A) and chloroform–methanol (B); 1–3 are methanol extracts, 4–6 are ethyl acetate extracts, 7–9 are DIH2O extracts, and 10–12 are dichloromethane extracts of the leaves and flowers of G. jasminoides (BACH151) and fruit of G. florida (BACH152), respectively.
To further characterize the pigments, methanol and DIH2O extracts of leaf, flower, and fruit and ethyl acetate extracts of fruit were chosen as they showed some trace evidence of yellow pigment by observation and TLC separation.
Ultraviolet and Nuclear Magnetic Resonance Spectra
The UV spectra of dye extracts from leaves, flowers, and fruits of Gardenia obtained by water and methanol extraction are illustrated in Figure 2. There was a single peak at 325 nm for the Gardenia fruit and flower extracts and two obvious peaks at 325 and 440 nm for ethyl acetate extracts of Gardenia fruits. The yellow pigments of the carotenoids usually give the maximum absorbance between 420 and 470 nm. The maximum absorbance of pure crocetin is at 420 nm, while crocin and crocetin with saccharide moieties exhibit maximum wavelengths at about 440 nm (16, 25, 26). Moras et al. (39) showed that the cis form of crocetin could absorb another maximum wavelength at ~300 nm.
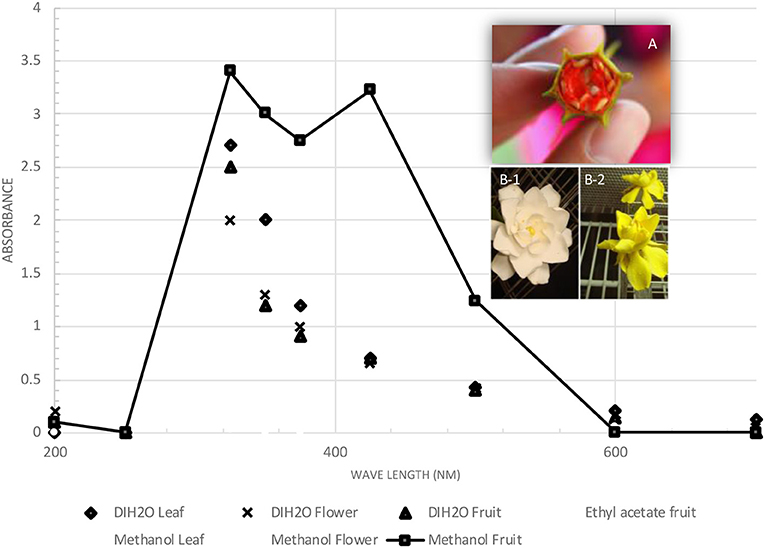
Figure 2. Ultraviolet spectra (200–700 nm) of Gardenia yellow extracts from different parts of Gardenia spp. (A) Gardenia fructus; (B) flowers of Gardenia jasminoides (BACH151).
As petals of mature Gardenia flower turned yellow over time (Figure 2B2), we anticipated that there should be some evidence of the yellow pigments in flower extracts and therefore structural elucidation by NMR was undertaken. Fruit and flower tissues extracted with methanol and DIH2O were scanned for 300 mHz 1H NMR spectrums (Figures 3A–D). By using the 1H NMR chemical shifts and integration signals (peaks) together, with data that have been reported from NMR spectroscopy of Gardenia pigments (16, 22, 24, 40, 41). The results illustrate that fruit and floral (DIH2O) extracts consisted of the major chemical shifts of CH-10 at 7.51 ppm and CH-10,10′ at 7.44 ppm of the trans form (peaks 1–4) as well as signals at ~2.0 ppm (peaks 13 and14) that are similar to those of crocetins (23, 38). Peaks 5–10 could also indicate aglycones (sugar moieties) such as β-D-gentiobiosyl, β- D -glucosyl, and α-glucose with the obvious chemical shifts of CH-1 at ~5.5 ppm and CH2-6′,6′ at 3.7 ppm (24). This confirms that the chemical structures of Gardenia yellow comprise crocetin esters, including, for example, crocetin digentiobioside ester (crocin), crocetin monogentiobioside monoglucoside ester, and crocetin diglucoside ester (22). Peaks 11 and 12 (chemical shifts at 4.2 ppm) together with peak 13 (signal at 2.1 ppm) with CH resonances at 3.21–3.23, 3.26–3.28, 3.23–3.25, and 3.17–3.19 ppm and CH-2 resonances at 3.67 and 3.45–3.47 ppm also indicate the existing xanthophylls in Gardenia fruit extract (41). The chemical structures of crocetin and its ester formed with aglycones (gentiobiosyl and glycosyl) are illustrated in Scheme 1.
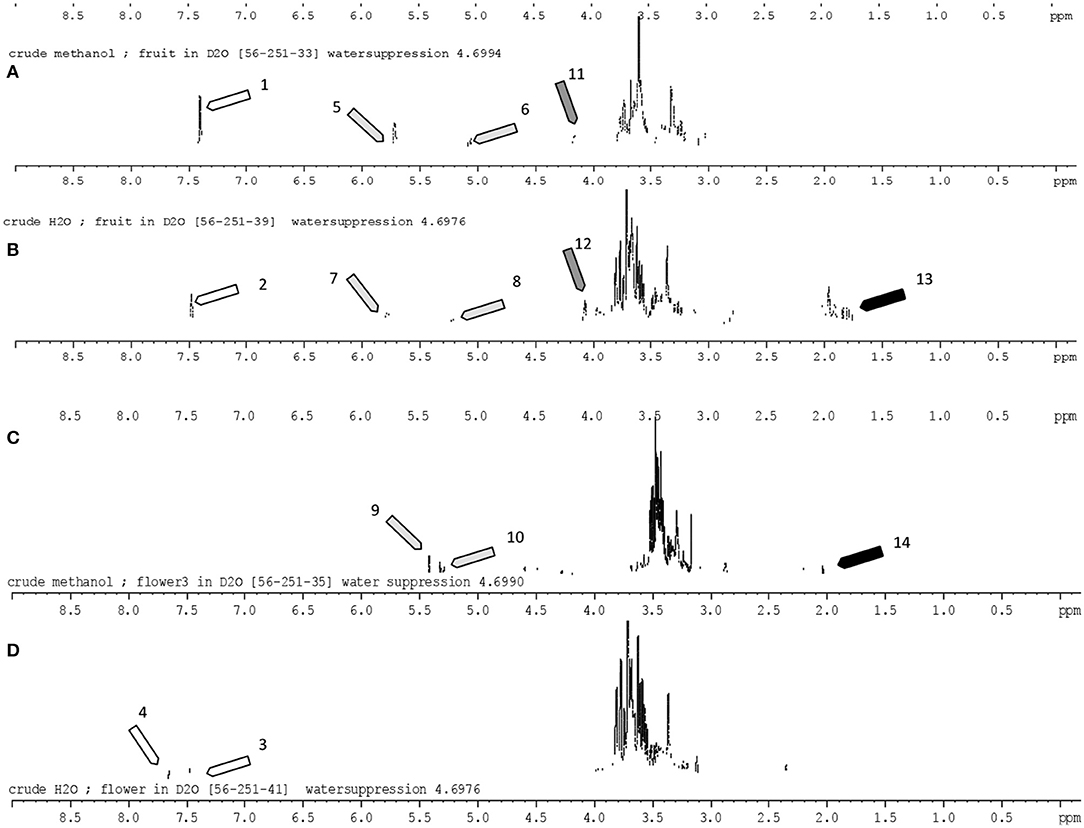
Figure 3. 1H Nuclear magnetic resonance (300 mHz) spectrum of Gardenia fruit and flower extracts (in high polar solvents; DIH2O and methanol). The identified peaks corresponding to the peak number were in accord with the literature [Siwawej and Jarayapun (16); Choi et al. (22); Sobolev et al. (24); Srivastava et al. (40); Singh (41)]. Assignments: 1–3, all-trans-crocin; 4, 13-cis-crocin; 5,7, β-D-gentiobiosyl; 6,8, α-glucose; 9, DB (double bond); 10, CH-1 α-glucose; 11,12, xanthophylls; 13,14, CH3-19,20 crocetin. (A,B) Crude methanol and water extracts of Gardenia fruit; (C,D) crude methanol and water extracts of Gardenia flowers.
Capillary Electrophoresis–Mass Spectrometry
The methanolic extracts of the Gardenia parts were first run with an aqueous buffer system to enable the analyses of cations and anions in a single run. This allowed us to separate or detect all compounds in the positive detection mode. Figures 4A–C illustrates the complex electropherograms of methanolic extracts of different parts of Gardenia. The Venn diagram illustrates that the extracts of floral and fruit tissues share 11 metabolites in common (Figure 4D). As it was impossible to identify such an intricate matrix, the 20 most abundant analytes for each extract were obtained with their corresponding m/z-values and peak area. As nearly all of the analytes are singly charged, the denoted m/z-values correspond to the mass of the pseudomolecular ion (positive ion). The relationship between the compounds analyzed from the methanolic extracts was evaluated in the form of mass trace PCA, as shown in Figure 5. In agreement with the Venn diagram, PCA illustrates the similarity of the compounds' patterns in the fruit and flower extracts leading to 11 compounds with a positive ion charge, as shown in Table 3. The crocins (crocetin and crocin 2) and the iridoids such as genipin gentiobioside and 8-epiapodantheroside were among the major components found in both Gardenia fruit and flower. Generally, the crocins are able to absorb at 430 nm. The other miscellaneous components, including jasminodiol, genistein, and gardenoside, absorb the maximum wavelength at ~240 nm. In other studies, numerous bioactive ingredients were isolated and identified from fruit of Gardenia, including the iridoids (42, 43), which show health benefits such as anti-inflammation, anti-depression, and anti-diabetic properties, anti-thrombotic activity, protection against lipopolysaccharide-induced apoptotic liver damage, and a proven antagonistic effect against Alzheimer's disease (43–45). Crocins are the glycosides (monoglycosyls or diglycosylesters) of C-20 carotenoid aglycone crocetins. They are also known to be the major compounds responsible for the unique yellow color of Gardenia and saffron (17, 22, 24). The other components isolated from fruits with bioactive functionality include the flavonoids, diterpenoids, and triterpenoids (46). A few studies have highlighted the isolation and characterization of phytochemical ingredients in the flower tissues of Gardenia, such as iridoids and triterpenoids (45, 47–49). In other studies the phenylpropanoid glucoside along with phenolic acids and flavones were isolated from the edible floral tissue of G. jasminoides and Zhizhi (9, 50). These compounds possess high antioxidant potential and elucidated neuroprotective, hepatoprotective, anti-inflammatory, antitumor, hypoglycemic, and hypolipidemic activities (51, 52). Thus, both the fruits and flowers of Gardenia spp. have the potential to be developed into medicinal food and pharmaceutical products (9). These studies, however, did not confirm the presence of crocins in the flower, in particular when the flower reached the mature stage. This paper is, therefore, the first evidence that the yellow dye crocins detected in the flower of Gardenia could be useful for finding alternative sources of natural food additives in a sustainable manner.
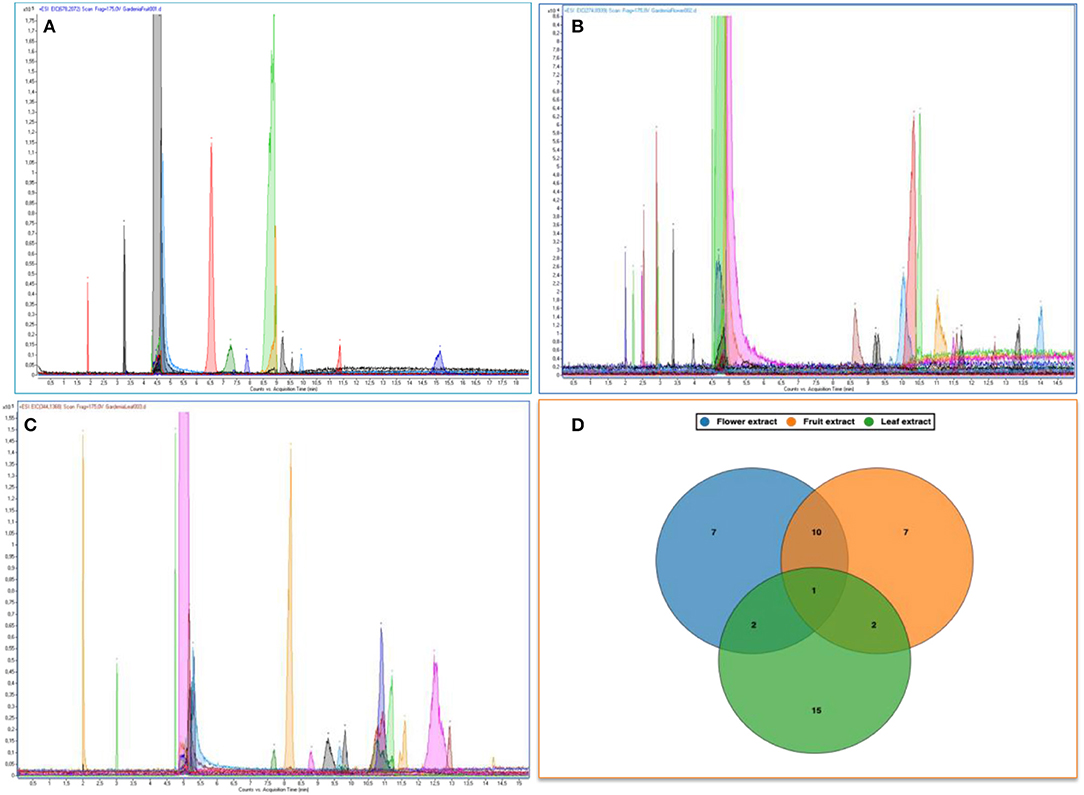
Figure 4. Electropherograms of methanolic extracts of Gardenia spp. fruit (A), flower (B), and leaf (C). Venn diagrams of the common metabolites found in those extracts (D).
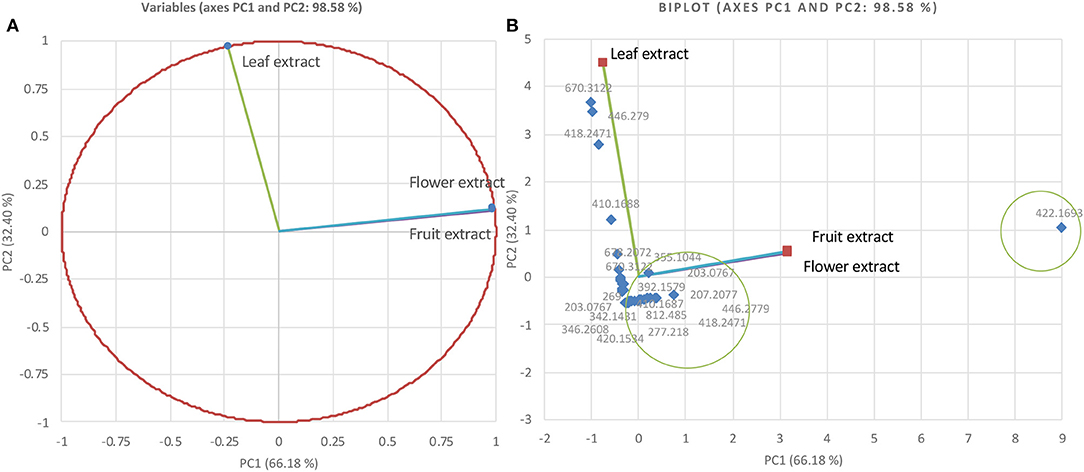
Figure 5. Principal component analysis relationship of mass values (m/z; positive ions) of the 20 most abundant components from Gardenia methanolic extracts (A) and biplot cluster analysis (B).
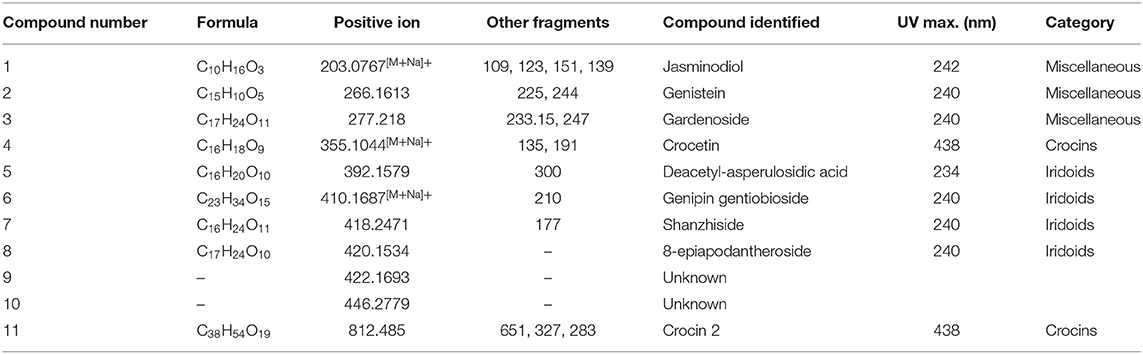
Table 3. Principal trace compounds identified from methanolic extracts of fruit and flower of Gardenia spp.
Conclusion
In this study, crocin 2 and crocetin were characterized for the first time from methanol and DIH2O extracts of fully mature flowers of G. jasminoides. They were structurally confirmed by means of NMR patterns and MS after separation by capillary electrophoresis in comparison with those of Gardenia yellow from Zhizhi. The other compounds were iridoids and triterpenoids, which have beneficial bioactivities that could be potential used as food additives and medicinally functional ingredients. In this sense, cape jasmine, a cultural ornamental plant, provides an opportunity to develop sustainable edible landscapes. The research information from this study could also increase the market value of the over-mature flowers that are often discarded.
Data Availability Statement
The datasets generated for this study are available on request to the corresponding author.
Author Contributions
The project idea and experimental design was developed and data were analyzed by SS. Sample collections were performed and the manuscript was written by SS and PS. The laboratory experiments were run by SS, KS, WR, and TJ. All authors contributed to the article and approved the submitted version.
Funding
This research work was partially supported by Chiang Mai University.
Conflict of Interest
The authors declare that the research was conducted in the absence of any commercial or financial relationships that could be construed as a potential conflict of interest.
Acknowledgments
The authors wish to thank Dr. Tjorben Nils Posch (Forschungszentrum, Jülich GmbH, Germany) for his kind assistance with analytical chemistry. We also thank Dr. James Franklin Maxwell (1945–2015), Botany Department, Faculty of Science, Chiang Mai University, Thailand, for plant identification. Our condolences to his family and friends for his loss.
References
1. Liu J, Zhu P, Zhao C, Sui S, Dong Z, Zhang, et al. Study on the dyeing of wool fabrics with Phytolacca berry natural dyes. Fibers Polym. (2014) 15:1601–8. doi: 10.1007/s12221-014-1601-1
2. Liu L, Tang RC. Adsorption property of gardenia yellow onto chitosan fiber. Dye. (2013) 39:1–4. Availabe online at: http://en.cnki.com.cn/Article_en/CJFDTOTAL-YIRA201307001.htm?fbclid=IwAR2sytDKNW0x8aHnV9fMZdXja677AI79U3yoQr5RXSrfEQ9fsTsnV-0VJN8
3. Shahid I, Shahid M, Mohammad F. Perspectives for natural product based agents derived from industrial plants in textile applications – a review. J Clean Prod. (2013) 57:2–18. doi: 10.1016/j.jclepro.2013.06.004
4. Bechtold T, Mussak R, Mahmud-Ali A, Ganglberger E, Geissler S. Extraction of natural dyes for textile dyeing from coloured plant wastes released from the food and beverage industry. J Sci Food Agric. (2006) 86:233–42. doi: 10.1002/jsfa.2360
5. Boo HO, Hwang SJ, Bae CS, Park SH, Heo BG, Gorinstein, et al. Extraction and characterization of some natural plant pigments. Ind Crops Prod. (2012) 40:129–35. doi: 10.1016/j.indcrop.2012.02.042
6. El-Shishtawy R, Shokry GM, Ahmed NE, Kamel MM. Dyeing of modified acrylic fibers with curcumin and madder natural dyes. Fibers Polym. (2009) 10:617–24. doi: 10.1007/s12221-010-0617-4
7. Pyle RM. Nature matrix: reconnecting people and nature. Oryx. (2003) 37:206–14. doi: 10.1017/S0030605303000383
8. Chaichana J, Niwatananun W, Vejabhikul S, Somna S, Chansakaow S. Volatile constituents biological activities of Gardenia jasminoides. J Health Sci. (2009) 23:141–5. Availabe online at: https://he01.tci-thaijo.org/index.php/jhealthres/article/view/156505/113552?fbclid=IwAR2CHkf7OI54KD1dUyBPRDjiNxJKcUFPX3cvKnPy36civPdCXnO63uLaYoo
9. Zhang H, Feng N, Xu YT, Li TX, Gao XM, Zhu Y, et al. Chemical constituents from the flowers of wild Gardenia jasminoides Ellis. Chem Biodivers. (2017) 14:e1600437. doi: 10.1002/cbdv.201600437
10. Kantadoung K, Rachkeeree A, Puangpradab R, Sommano S, Suksathan R. Nutritive values of some edible flowers found in northern Thailand during the rainy season. Acta Hortic. (2018) 1210:263–72. doi: 10.17660/ActaHortic.2018.1210.37
11. Fan Y, Ge Z, Luo A. In vitro antioxidant activity of polysaccharide from Gardenia jasminoides Ellis. J Med Plants Res. (2011) 5:2963–8. doi: 10.5897/JMPR10.794
12. Chen Y, Cai L, Zhao C, Xu H-C, Cao Y, Liu Y, et al. Spectroscopic, stability and radical-scavenging properties of a novel pigment from gardenia. Food Chem. (2008) 109:269–77. doi: 10.1016/j.foodchem.2007.10.023
13. Chen Y, Zhang H, Li YX, Cai L, Huang J, Zhao C, et al. Crocin and geniposide profiles and radical scavenging activity of gardenia fruits (Gardenia jasminoides Ellis) from different cultivars and at the various stages of maturation. Fitoterapia. (2010) 81:269–73. doi: 10.1016/j.fitote.2009.09.011
14. Neri-Numa IA, Pessoa MG, Paulino BN, Pastore GM. Genipin: a natural blue pigment for food and health purposes. Trends Food Sci Technol. (2017) 67:271–9. doi: 10.1016/j.tifs.2017.06.018
15. Park KH, Kim TY, Park JY, Jin EM, Yim SH, Choi DY, et al. Adsorption characteristics of gardenia yellow as natural photosensitizer for dye-sensitized solar cells. Dyes Pigm. (2013) 96:595–601. doi: 10.1016/j.dyepig.2012.10.005
16. Siwawej S, Jarayapun A. The food color extraction from Gardenia Fruit (Gardenia jasminodes Ellis forma var. gardiflora Makino): the appropriate extraction conditions. Kasetsart J. (1998) 32:520–8.
17. Zhou Y, Zhang J, Tang R-C, Zhang J. Simultaneous dyeing and functionalization of silk with three natural yellow dyes. Ind Crops Prod. (2014) 64:224–32. doi: 10.1016/j.indcrop.2014.09.041
18. Shen J, Gao P, Ma H. The effect of tris(2-carboxyethyl)phosphine on the dyeing of wool fabrics with natural dyes. Dyes Pigm. (2014) 108:70–5. doi: 10.1016/j.dyepig.2014.04.027
19. Sommano S, Suksathan R, Colelli G, Kumpuan W. Potentials of Thai tropical edible flowers as fresh cut salad mix. Acta Hortic. (2018) 1194:1187–94. doi: 10.17660/ActaHortic.2018.1194.169
20. Association of Official Analytical Chemists (AOAC). The Official Methods of Analysis. Washington, DC: Association of Official Analytical Chemists (2000).
21. Hasan MM, Hossain A, Shamim A, Rahman MM. Phytochemical and pharmacological evaluation of ethanolic extract of Lepisanthes rubiginosa L. leaves. BMC Compl Alter Med. (2017) 17:496. doi: 10.1186/s12906-017-2010-y
22. Choi H-J, Park YS, Kim MG, Kim TK, Yoon NS, Lim YJ. Isolation and characterization of the major colorant in gardenia fruit. Dyes Pigm. (2001) 49:15–20. doi: 10.1016/S0143-7208(01)00007-9
23. Tangpao T, Chung HH, Sommano RS. Aromatic profiles of essential oils from five commonly used Thai basils. Foods. (2018) 7:11. doi: 10.3390/foods7110175
24. Sobolev AP, Carradori S, Capitani D, Vista S, Trella A, Marini F, et al. Saffron samples of different origin: an NMR study of microwave-assisted extracts. Foods. (2014) 3:403–19. doi: 10.3390/foods3030403
25. Posch TN, Martin N, Pütz M, Huhn C. Nonaqueous capillary electrophoresis–mass spectrometry: a versatile, straightforward tool for the analysis of alkaloids from psychoactive plant extracts. Electrophoresis. (2012) 33:1557–66. doi: 10.1002/elps.201100682
26. Chen Y, Zhang H, Tian X, Zhao C, Cai L, Liu Y, et al. Antioxidant potential of crocins and ethanol extracts of Gardenia jasminoides Ellis and Crocus sativus L.: a relationship investigation between antioxidant activity and crocin contents. Food Chem. (2008) 109:484–92. doi: 10.1016/j.foodchem.2007.09.080
27. Han Y, Wen J, Zhou T, Fan G. Chemical fingerprinting of Gardenia jasminoides Ellis by HPLC–DAD–ESI-MS combined with chemometrics methods. Food Chem. (2015) 188:648–57. doi: 10.1016/j.foodchem.2015.05.039
28. Park YS, Choi HJ, Yun SH, Kim SH, Kim TK, Yoon NS, et al. Qualitative analysis of crocetin colorants in gardenias (Gardenia jasminoides Ellis) by LC/DAD/MS. J. Eng. Chem. (2001) 7:375–9. Availabe online at: https://www.cheric.org/research/tech/periodicals/view.php?seq=328620&fbclid=IwAR2k7QOiBGECyPG3xpcB1pz7qScDL3uLFS9bSHqvzv1aZKREBbPac6_0okI
29. Posch TN, Müller A, Schulz W, Pütz M, Huhn C. Implementation of a design of experiments to study the influence of the background electrolyte on separation and detection in non-aqueous capillary electrophoresis–mass spectrometry. Electrophoresis. (2012) 33:583–98. doi: 10.1002/elps.201100381
30. Kesavan K, Gnanasekaran J, Gurunagarajan S, Nayagam AA. Microscopic, physicochemical phytochemical analysis of Gardenia jasminoides Ellis. Int J Phar Pharma Sci. (2018) 10:1. doi: 10.22159/ijpps.2018v10i1.21665
31. Corrêa RCG, Garcia JAA, Correa VG, Vieira TF, Bracht A, Peralta RM. Pigments and vitamins from plants as functional ingredients: current trends and perspectives. Adv Food Nutr Res. (2019) 90:259–303. doi: 10.1016/bs.afnr.2019.02.003
32. Humphrey AM. Chlorophyll as a color functional ingredient. J Food Sci. (2004) 69:C422–5. doi: 10.1111/j.1365-2621.2004.tb10710.x
33. Oliver JR, Blakeney AB, Allen HM. The Colour of flour streams as related to ash and pigment contents. J Cereal Sci. (1993) 17:169–82. doi: 10.1006/jcrs.1993.1017
34. Forgacs E, Cserhati T. Thin-layer chromatography of natural pigments: new advances. J Liq Chrom Rel Technol. (2002) 25:1521–41. doi: 10.1081/JLC-120005702
35. Hynstova V, Sterbova D, Klejdus B, Hedbavny J, Huska D, Adam, et al. Separation, identification and quantification of carotenoids and chlorophylls in dietary supplements containing Chlorella vulgaris and Spirulina platensis using high performance thin layer chromatography. J Phar Biomed Anal. (2018) 148:108–18. doi: 10.1016/j.jpba.2017.09.018
36. Katayama N, Kanaizuka Y, Yokohama Y. An improved method for extraction and separation of photosynthetic pigments. J Biol Educ. (2003) 37:186–9. doi: 10.1080/00219266.2003.9655881
37. Quach HT, Steeper RL, Griffin GW. An improved method for the extraction and thin-layer chromatography of chlorophyll a and b from spinach. J Chem Educ. (2004) 81:385. doi: 10.1021/ed081p385
38. Zongram O, Ruangrungsi N, Palanuvej C, Rungsihirunrat K. Standardization of Gardenia jasminoides fruits and crocin content analysis using UV/visible spectrophotometry. Chiang Mai J Sci. (2017) 44:1453–62. Availabe online at: http://www.thaiscience.info/journals/Article/CMJS/10987590.pdf?fbclid=IwAR0xjX8LQvOO2qjUZR6q1mwNcFWVruy1DoMAjXVzuKtPdBvbqC8Y-nQrrb4
39. Moras B, Loffredo L, Rey S. Quality assessment of saffron (Crocus sativus L.) extracts via UHPLC-DAD-MS analysis and detection of adulteration using gardenia fruit extract (Gardenia jasminoides Ellis). Food Chem. (2018) 257:325–32. doi: 10.1016/j.foodchem.2018.03.025
40. Srivastava R, Ahmed H, Dixit RK, Dharamveer, Saraf SA. Crocus sativus L.: a comprehensive review. Pharmacol Rev. (2010) 4:200–8. doi: 10.4103/0973-7847.70919
41. Singh S. Isolation identification of pigment molecule from leaves of Prosopis juliflora. Int Res J Phar. (2012) 3:150–2. Availabe online at: https://irjponline.com/admin/php/uploads/991_pdf.pdf?fbclid=IwAR39CMePhuVonVaFBsrjWLn94lmzuEZMEypmf_lB0IZ3R6EnKtQMc4gStao
42. Li J, Xu B, Zhang Y, Dai S, Sun F, Shi X, et al. Determination of geniposide in Gardenia jasminoides Ellis fruit by near-infrared spectroscopy and chemometrics. Anal Lett. (2016) 49:2063–76. doi: 10.1080/00032719.2015.1130714
43. Yu Y, Xie ZL, Gao H, Ma WW, Dai Y, Wang Y, et al. Bioactive iridoid glucosides from the fruit of Gardenia jasminoides. J Nat Prod. (2009) 72:1459–64. doi: 10.1021/np900176q
44. Xiao W, Li S, Wang S, Ho CT. Chemistry and bioactivity of Gardenia jasminoides. J Food Drug Anal. (2017) 25:43–61. doi: 10.1016/j.jfda.2016.11.005
45. Yin F, Liu J-H. Research and application progress of Gardenia jasminoides. Chin Herb Med. (2018) 10:362–70. doi: 10.1016/j.chmed.2018.09.001
46. Peng K, Yang L, Zhao S, Chen L, Zhao F, Qiu F, et al. Chemical constituents from the fruit of Gardenia jasminoides and their inhibitory effects on nitric oxide production. Bioorg Med Chem Lett. (2013) 23:1127–31. doi: 10.1016/j.bmcl.2012.11.099
47. Ragasa CY, Pimenta LEN, Rideout JA. Iridoids from Gardenia jasminoides. Nat Prod Res. (2007) 21:1078–84. doi: 10.1080/14786410701589998
48. Song J-L, Wang R, Shi Y-P, Qi H-Y. Iridoids from the flowers of Gardenia jasminoides Ellis and their chemotaxonomic significance. Biochem Syst Ecol. (2014) 56:267–70. doi: 10.1016/j.bse.2014.06.011
49. Zhang LS, Wang YL, Liu Q, Zhou CX, Mo J-X, Lin LG, et al. Three new 3,4-seco-cycloartane triterpenoids from the flower of Gardenia jasminoides. Phytochem Lett. (2018) 23:172–5. doi: 10.1016/j.phytol.2017.11.006
50. Shang YF, Zhang YG, Cao H, Ma YL, Wei ZJ. Comparative study of chemical compositions and antioxidant activities of Zhizi fruit extracts from different regions. Heliyon. (2019) 5:e02853. doi: 10.1016/j.heliyon.2019.e02853
51. Wang C, Gong X, Bo A, Zhang L, Zhang M, Zang E, et al. Iridoids: research advances in their phytochemistry, biological activities, and pharmacokinetics. Molecules. (2020) 25:287. doi: 10.3390/molecules25020287
52. Shan M, Yu S, Yan H, Guo S, Xiao W, Wang, et al. A review on the phytochemistry, pharmacology, pharmacokinetics and toxicology of geniposide, a natural product. Molecules. (2017) 22:1689. doi: 10.3390/molecules22101689
Botanical glossary
Gardenia florida L. (syn. Gardenia jasminoides var. jasminoides)
An evergreen gardening shrub of the family Rubiaceae, bearing white fragrant flowers with a simple corolla. The flower is fertile and the fruit is ellipsoid to ovoid, orange when ripe with fleshy placentae and numerous seeds.
Gardenia jasminoides Ellis
A common tropical landscape plant. It is quite variable morphologically especially in leaf size, calyx lobe size, and corolla size. Several varieties have been recognized but, in this work, the corolla is white to pale yellow and doubled that of var. fortuneana. The flower is sterile and the plant does not set fruit.
Zhizhi or fructus
Fruits of Gardenia florida L.
Keywords: cape jasmine, carotenoids, chromatography, dye, edible flower, natural pigment
Citation: Sommano SR, Suppakittpaisarn P, Sringarm K, Junmahasathien T and Ruksiriwanich W (2020) Recovery of Crocins From Floral Tissue of Gardenia jasminoides Ellis. Front. Nutr. 7:106. doi: 10.3389/fnut.2020.00106
Received: 24 January 2020; Accepted: 10 June 2020;
Published: 02 September 2020.
Edited by:
Edy Sousa De Brito, Embrapa Agroindústria Tropical, BrazilReviewed by:
Sui Kiat Chang, Chinese Academy of Sciences, ChinaEduardo Dellacassa, Universidad de la República, Uruguay
Copyright © 2020 Sommano, Suppakittpaisarn, Sringarm, Junmahasathien and Ruksiriwanich. This is an open-access article distributed under the terms of the Creative Commons Attribution License (CC BY). The use, distribution or reproduction in other forums is permitted, provided the original author(s) and the copyright owner(s) are credited and that the original publication in this journal is cited, in accordance with accepted academic practice. No use, distribution or reproduction is permitted which does not comply with these terms.
*Correspondence: Sarana Rose Sommano, c2FyYW5hLnNAY211LmFjLnRo