- 1ICAR-Indian Institute of Rice Research, Hyderabad, India
- 2ICMR-National Institute of Nutrition, Hyderabad, India
- 3Agricultural Research Station, University of Agricultural Sciences-D, Bangalore, India
- 4Department of Plant Breeding and Genetics, AICRIP (Rice), Rajendra Agricultural University, Samastipur, India
Zinc malnutrition is a major issue in developing countries where polished rice is a staple food. With the existing significant genetic variability for high zinc in polished rice, the development of biofortified rice varieties was targeted in India with support from HarvestPlus, Department of Biotechnology, and Indian Council of Agricultural Research of Government of India. Indian Institute of Rice Research (IIRR) facilitates rice varietal release through All India Coordinated Rice Improvement Project (AICRIP) and also supports rice biofortification program in India. Various germplasm sets of several national institutions were characterized at IIRR for their zinc content in brown rice using energy-dispersive X-ray fluorescence spectroscopy indicating the range of zinc to be 7.3 to 52.7 mg/kg. Evaluation of different mapping populations involving wild germplasm, landraces, and varieties for their zinc content showed the feasibility of favorable recombination of high zinc content and yield. Ninety-nine genotypes from germplasm and 344 lines from mapping populations showed zinc content of ≥28 mg/kg in polished rice meeting the target zinc content set by HarvestPlus. Through AICRIP biofortification trial constituted since 2013, 170 test entries were nominated by various national institutions until 2017, and four biofortified rice varieties were released. Only the test entry with target zinc content, yield, and quality parameters is promoted to the next year; thus, each test entry is evaluated for 3 years across 17 to 27 locations for their performance. Multilocation studies of two mapping populations and AICRIP biofortification trials indicated the zinc content to be highly influenced by environment. The bioavailability of a released biofortified rice variety, viz., DRR Dhan 45 was found to twice that of control IR64. The technology efficacy of the four released varieties developed through conventional breeding ranged from 48 to 75% with zinc intake of 38 to be 47% and 46 to 57% of the RDA for male and female, respectively. The observations from the characterization of germplasm and mapping populations for zinc content and development of national evaluation system for the release of biofortified rice varieties have been discussed in the context of the five criteria set by biofortification program.
Introduction
Among various food crops, rice is the world's most important crop, and more than half of the global population is dependent on rice. Greater than 90% of rice is produced and consumed in Asia (1). India is the second largest producer of rice with a production of 112.76 million metric tons during 2017–2018 (2). Imbalanced supply of essential amino acids, micronutrients, and vitamins leads to their deficiency or accumulation affecting the human metabolism. According to World Health Organization, zinc deficiency is the fifth important factor for illness and diseases in developing countries and 11th in world (3). Worldwide, the prevalence of zinc deficiency has been estimated to be ~20% in soils (4). Zinc deficiency causes diarrhea and respiratory diseases, leading to 400,000 deaths annually across the world (5, 6). Zinc deficiency is also associated with poor growth, loss of appetite, skin lesions, impaired taste acuity, delayed wound healing, hypogonadism, delayed sexual maturation, and impaired immune response (7, 8). Every year 1.31 million disability-adjusted life-years (DALYs) are lost in India because of zinc malnutrition. An ex-ante analysis of zinc biofortification of rice in India revealed that of the 1.31 million DALYs lost, 0.142 and 0.456 million DALYs could be saved in pessimistic and optimistic assumptions, if zinc-biofortified rice is introduced (9).
As a “global public good,” international agriculture research in the form of International Rice Research Institute and Center for Improvement of Wheat and Maize merged into Consultative Group on International Agricultural Research (CGIAR) initially implemented “Green Revolution” during 1960s, successfully leading to enhanced grain production through the development of high-yielding varieties (HYVs). However, grains of HYVs contain lesser amounts of nutrients; in case of rice, polishing further reduces the nutrients content, viz., iron and zinc. Subsequently in 1991, considering concern raised by the international nutrition community about micronutrient deficiency as a global problem, CGIAR initiated studies on developing “micronutrient-dense” staple crops (10) in the name of biofortification. In 2003, CGIAR launched HarvestPlus Challenge Program as a global program, with the objective of developing biofortified staple crops such as wheat, rice, maize, cassava, and so on, through plant breeding (11, 12). Biofortification of rice grain with iron was initiated in 1992 and zinc in 1995 (13). Improving nutrition is also being targeted under 12 of the 17 Sustainable Development Goals set by United Nations (https://www.undp.org/content/undp/…/sustainable-development-goals.html).
The word “biofortification” refers to enhancing bioavailable micronutrient content of food crops through genetic selection via plant breeding. It is a promising strategy to address nutrition security and was initiated with five criteria, viz., (1) crop productivity (i.e., yield) must be maintained or increased to ensure farmer acceptance; (2) the enhanced micronutrient level must have significant impact on human health; (3) the enhanced micronutrient trait must be relatively stable across various edaphic environments and climatic zones; (4) the bioavailability of micronutrients in enriched lines must be tested in humans to ensure that they improve the micronutrient status of people preparing and eating them in traditional ways within normal household environments; and (5) consumer acceptance must be tested (taste and cooking quality must be acceptable to household members) to ensure maximum impact on nutritional health (14).
Although a few independent groups have initiated research on biofortification since 2000 in India, the programs kick-started after HarvestPlus program implementation in India during 2007. Department of Biotechnology (DBT) and Indian Council of Agricultural Research (ICAR) of Government of India have also initiated support to biofortification projects leading to the consorted national and international research efforts toward the development of biofortified rice varieties. With the negative impact of zinc deficiency on human metabolism, especially in countries with rice as a major staple food, development of zinc-biofortified varieties has become one of the important objectives of crop improvement programs. The success of biofortification relies on the existence of diversity for the target trait in the germplasm of crop, successful recombination of the target trait with yield, and identification of suitable recombinants with yield and target trait through multilocation evaluation. To phenotype zinc content of rice grains across thousands of germplasm lines or mapping populations, X-ray fluorescence spectroscopy (XRF) was found to be promising because of its high throughput, cost-effectiveness, and rapid analyses over atomic absorption spectrometry and inductively coupled plasma mass spectrometry (15). HarvestPlus has supported rice biofortification research of several laboratories in India through sponsoring XRF equipment and providing preliminary biofortified rice breeding lines.
ICAR–Indian Institute of Rice Research (IIRR) is a national institute that facilitates rice varietal release through All India Coordinated Rice Improvement Project (AICRIP) (http://www.icar-iirr.org/); IIRR is also translating the benefits of the research on zinc biofortification of rice to society through release of biofortified rice varieties through AICRIP and coordination of a Consortia Research Platform on biofortification of the cereals. In the present study, we summarize our observations from the studies on characterization of germplasm and mapping populations for zinc content and development of national evaluation system, that is, AICRIP, for the release of biofortified rice varieties and its successful implementation in India.
Materials and Methods
Two datasets were analyzed for characterization of zinc content in rice, viz., (1) data of zinc content from the field experiments of genotypes and recombinant inbred lines (RILs) populations developed by our group at IIRR and (2) data from analysis of the zinc content in the germplasm and RIL populations of various national institutions including IIRR at XRF facility of IIRR.
Conduct of Field Experiments at IIRR
The field experimental material included 170 rice genotypes comprising landraces, released varieties, and derivatives of various crosses (Table 1) and 13 RIL populations developed from the six identified donors for high zinc in polished rice (Chittimuthyalu, Jalpriya, BR2655, Type-3, Suraksha, and Ranbir Basmati) and five mega varieties (MTU1010, IR64, Swarna, RPBio226, and PR116) using a single seed descent method (Table 3). The germplasm experiment was laid out in randomized block design with two replications. The 13 mapping populations were grown in 13 blocks in an augmented block design with IR64 and Chittimuthyalu as replicated checks. The germplasm and mapping populations were evaluated at the research farm of IIRR, Hyderabad, during the wet season following the recommended package of rice production and protection practices. The experimental soil characteristics were as follows: pH 8.2; non-saline (EC 0.7l dS/m); calcareous (free CaCO3 5.01%); CEC 44.1 C mol (p+)/kg soil and medium soil organic carbon (0.69%); low soil available nitrogen (228 kg ha−1); high available phosphorus (105 kg P2O5ha−1); high available potassium (530 kg K2O ha−1); and high available zinc (12.5 mg/kg). A RIL population, viz., MTU1010/Jalpriya, was also grown at Agriculture Research Station (ARS), Mugad and Rajendra Agricultural University (RAU), Pusa, and another RIL population, MTU1010/Suraksha was evaluated at ARS, Mugad. For each genotype and RIL, three representative plants from the middle row were harvested at maturity, and single plant yield (g) adjusted to 14% grain moisture content was recorded. The single plant yield from the three plants was pooled and divided into three parts to be analyzed as three replicates for zinc content.
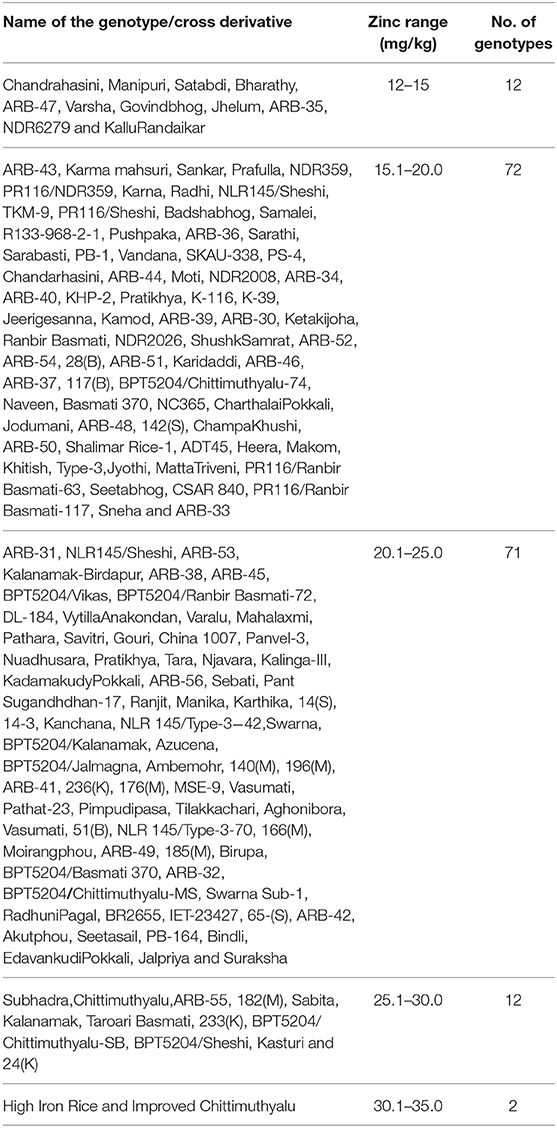
Table 1. Range and classification based on zinc content in brown rice of 170 rice genotypes comprising landraces, released varieties, and derivatives from various crosses.
Evaluation of Rice Germplasm and Mapping Populations at IIRR
Seventeen sets of rice genotypes, several mapping populations, and the selected lines from mapping populations from various national institutions including IIRR were evaluated for their zinc content at the XRF facility of IIRR.
Constitution of Biofortification Trial Under AICRIP
To evaluate the breeding lines developed with high zinc by various institutions of India, a national evaluation study was constituted under AICRIP as Biofortification trial during 2013 with two kinds of check genotypes for zinc content and yield. Initially, during 2013, to identify the promising lines in biofortification trial, a modest threshold/target zinc value of 20 mg/kg was set, as most of the cultivated rice varieties showed grain zinc content of ~15 mg/kg. From 2015, optimum threshold/target content of zinc in polished rice was increased to 24 mg/kg to match the threshold value of international target set earlier by HarvestPlus. The landraces, Kalanamak and Chittimuthyalu, with high zinc are the two check genotypes for zinc content in the trial (Supplementary Table 1). BPT5204, also known as Samba Mahsuri (BPT), popular with farmers and consumers across India because of its high yield and preferred cooking quality was included as yield check genotype. Because of the late crop duration of BPT, a mid–early duration popular variety IR64 was also included as another yield check during 2014. A hybrid (DRRH3) check was also added as another yield check during 2015 to compare the hybrids evaluated under this trial, but discontinued from 2016 because of the lack of hybrids nominated as test entries for evaluation. Under AICRIP, seed of potential lines gets nominated by the developers from various national institutions to IIRR as test entries. IIRR assigns an initial evaluation trial number to each test entry and constitutes Initial Variety Trial (IVT) with the nominated test entries along with check genotypes for yield and zinc content and sends seed along with details of the conduct of experiment to 10 to 30 locations across the country each year. After completion of the harvest, the agro-morphological and yield data of each location were sent to IIRR for compilation and identification of the promising lines with high yield and zinc. The seed is also sent to IIRR for estimation of zinc through XRF. The quality of test entries is also studied at IIRR as 16 traits/parameters (http://www.aicrip-intranet.in/PlantBreeding.aspx). The promising test entries of IVT are promoted to next year as advanced varietal trial 1 (AVT1) and subsequently to AVT2 based on their zinc content, yield, and quality. The consistent promising test entry during the 3 years of evaluation is identified as a biofortified variety.
Zinc Estimation
The seed samples were dehusked and polished as per the standardized protocol for analyzing samples with XRF (16). Seed was cleaned manually and dehusked using JLGJ4.5 rice husker with non-ferrous components (Jingjian Huayuan International Trade Co., Ltd., Jiangsu Sheng, China) sponsored by HarvestPlus. Dehusked brown rice was sieved to remove the broken rice grains, and the full brown rice grains were cleaned with soft tissue paper. Sample was gently rubbed for 1 min against paper in the hands of a trained person wearing gloves to ensure removal of particles other than rice. Each brown rice sample was milled in a specially designed K-710 Non-Ferrous Rice Polisher (Krishi International India Ltd., Hyderabad, India), and the percentage of polishing was calculated based on weight of the polished (white) rice to the brown rice. As polished rice is the most preferred form of consumption, brown rice samples were subjected to polishing for 90 to 120 s until the desirable whiteness was reached. Cleaning of the polished rice was repeated like that of brown rice. Time gap between polishing and cleaning was minimized to avoid sticking of the bran particles to the polished grain. Each sample of brown or polished rice (5 g) was subjected to energy-dispersive XRF (ED-XRF) (OXFORD Instruments X-Supreme 8000, Highwycombe Bucks, England) sponsored by HarvestPlus at IIRR. Samples were scanned against the prestandardized method, which converts the fluorescence intensity of each sample into zinc content (mg/kg). The percentage loss of zinc was calculated using the zinc content in brown and polished rice.
Statistical Analysis
Statistical analysis was done using R software, R foundation for Statistical computing, Vienna, Austria and all the graphs were made using ggplot2. Correlation between single plant yield and zinc content was done by performance analytics package.
Technology Efficacy of Zinc Biofortification in Rice
The current zinc intake from rice with the popular varieties was calculated based on the per-capita rice consumption of 220 g/day (17). Under assumption that the current rice consumption pattern is maintained in India and considering the adoption of biofortified rice varieties as technology, improved intake of zinc through biofortified rice varieties was calculated. The technology efficacy (E) of zinc biofortification in rice was derived as follows:
where IZ is improved zinc intake, CZ is current zinc intake, and RDA is the Recommended Dietary Allowance of zinc.
Results and Discussion
Country-wide food supplies including those from India were found to be zinc-deficient for ~15% to 20% of the world, based on the FAO food balance sheets (18). Biofortification strategy is suggested to be the most feasible and cost-effective means of delivering micronutrients to populations with limited access to diverse diets and other micronutrient interventions (15). Of various strategies for biofortification, development of biofortified varieties is often considered the most sustainable, especially if major staple cereal food crops are targeted. Once developed, the biofortified varieties can easily be adopted by the farmers with minimal cost of cultivation, unlike agronomic biofortification with additional expenditure on external nutrient application. Biofortification of rice with zinc in polished rice is envisaged as a promising approach to address zinc deficiency in countries where rice is the major staple food crop.
Genetic Variability for Zinc in Rice Grains
For developing varieties with high zinc in polished rice through conventional breeding, genetic variability for grain zinc content should exist in the germplasm. Since 1992, various sets of germplasm comprising varieties including indica and japonica, landraces from various countries and wild accessions, have been analyzed, and a wide variability of zinc content was reported (13, 19–21). In our study, zinc content in brown rice of the sample set of 170 rice germplasm ranged from 12 to 31.7 mg/kg (Table 1). Most of the genotypes showed zinc content in the range of 15 to 25 mg/kg, and four promising donors with >25 mg/kg zinc content were selected as donors for the development of mapping populations at IIRR. Zinc content in the germplasm comprising landraces, breeding lines, and varieties from 16 national institutions evaluated at XRF facility of IIRR ranged from 7.3 to 52.7 mg/kg (Table 2). Unlike reported mutants of IR64 with high zinc content in polished rice, higher zinc content was not observed in the mutants of BPT (ICAR-IIRR-SM-MUT) in our study (22). The mean values of zinc content in brown rice of germplasm sets ranged from 15.9 to 27.3 mg/kg. Of 3,177 germplasm evaluated, 3.1% showed zinc content of ≥35 mg/kg, and only 0.9% had zinc content of ≥40 mg/kg. Some of the reported ranges of grain zinc content include 13.5 to 58.4 mg/kg in 1,138 germplasm (13), 24.0 to 35.0 mg/kg in aromatic genotypes (14), 25.5 to 37.0 mg/kg in eight highly cultivated varieties (23), 12 to 27.6 mg/kg in 60 Iranian rice genotypes (24), and 13.3 to 34.2 mg/kg in 18 indigenous cultivars of Tripura state of India (25). In case of polished rice, zinc content ranged from 4.8 to 40.9 mg/kg in 170 rice germplasm of the present study. The reported ranges of grain zinc content in polished rice were 16.0 to 26.5 mg/kg in eight highly cultivated varieties (23), and up to 33 mg/kg zinc content in polished rice of 246 germplasm (26). Across the analyzed samples, a mean percentage loss of zinc content was found to be 19.0%, that is, 1.9 mg/kg loss in every 10 mg/kg of brown rice during polishing. The mean percentage loss of zinc varied from 11.1 to 27.9% in mapping populations and various germplasm sets (Figure 1). The reported losses of zinc content due to polished ranged from 5 to 30% (26, 27). The variation in the polishing could be due to the difference in the thickness of aleurone layer across rice genotypes as reported earlier (23, 28).
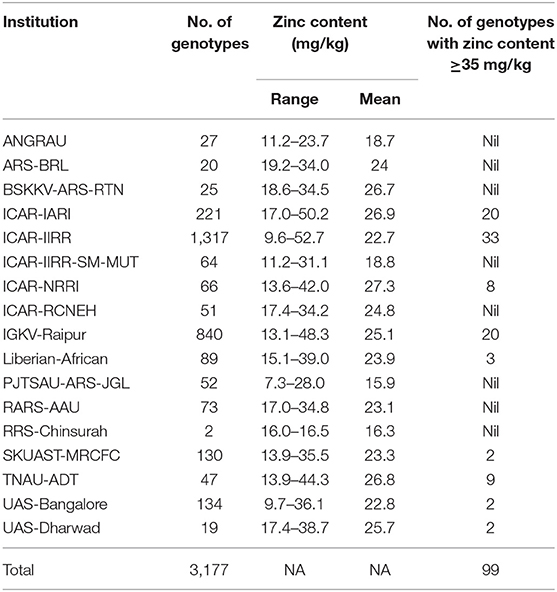
Table 2. Zinc content in brown rice of germplasm sets analyzed at the XRF facility of IIRR and the number of promising lines with zinc ≥35 mg/kg.
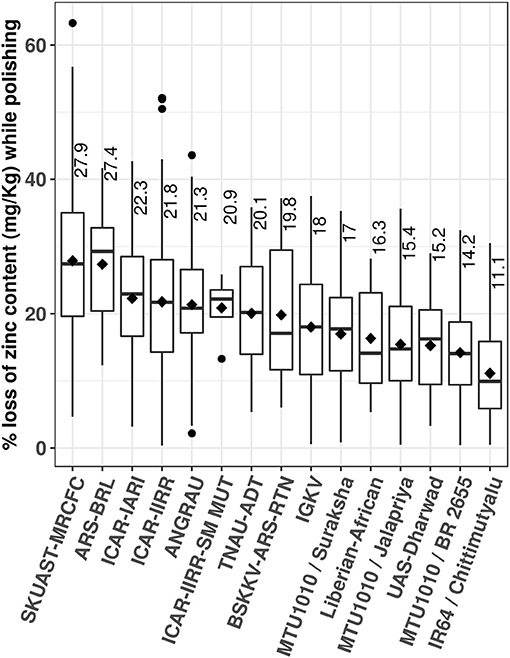
Figure 1. The range of loss in zinc content (%) during polishing in the germplasm sets and mapping populations. ANGRAU, Acharya N. G. Ranga Agricultural University, ARS-BRL, Agricultural Research Station, breeding lines, Shirgoan, BSKKV-ARS-RTN, Dr. BalasahebSawantKonkanKrishiVidyapeeth, Agricultural Research Station, Ratnagiri, ICAR-IARI, Indian Council of Agricultural Research–Indian Agricultural Research Institute, ICAR-IIRR, ICAR–Indian Institute of Rice Research, ICAR-IIRR-SM-MUT, Sambha Mahsuri Mutants, IGKV, Indira Gandhi Krishi Vishwavidyalaya-Raipur, SKUAST-MRCFC, Share e Kashmir University of Agricultural Science & Technology–Mountain Research Center for Field Crops, TNAU-ADT, Tamil Nadu Agricultural University, Aduthurai, UAS-Dharwad, University of Agricultural Sciences–Dharwad.
The zinc content in brown rice of varieties widely grown by the farmers ranged from <5 to 25 mg/kg (16, 20). The zinc content in polished rice of the popular rice varieties widely grown by the farmers ranges from (<12–14 mg/kg). Considering the threshold value of 28 mg/kg by HarvestPlus and 19.0% overall mean loss of zinc during polishing, germplasm with zinc content ≥35 mg/kg in brown rice can be considered as promising as donors to meet the target zinc.
Among 16 mapping populations grown at IIRR, the mean zinc content was least (18.6 mg/kg) in IR64/Jalpriya and highest (36.3 mg/kg) in RP Bio 226/Jalpriya (Figure 2). Of four mapping populations with IR64 as one of the parents, the mean zinc content was least (18.6 mg/kg) in cross with Jalpriya and highest (26.9 mg/kg) in cross with Chittimuthyalu. In the five mapping populations derived from MTU1010 as one of the parents, the mean zinc content was least (20.6 mg/kg) in cross with Chittimuthyalu and highest (29.0 mg/kg) in cross with Suraksha. Of the five mapping populations with Swarna as one of the parents, mean zinc content was least (19.2 mg/kg) in cross with Type-3 and highest (28.3 mg/kg) in cross with Ranbir Basmati followed closely by BR 2655. The mean zinc content of mapping populations and number of promising lines per mapping population varied despite common donors and recipients, suggesting the complexity of the trait and effect of interactions of parents for zinc content in progeny as per the cross combinations. Hence, screening of rice germplasm for higher zinc content should be a continuous process to identify compatible donors. Approximately 389 lines with zinc content ≥35 mg/kg were observed in 16 mapping populations studied (Table 3). Among 2,352 lines of mapping populations from other research groups of various national institutions analyzed using XRF facility at IIRR, 24 lines (1%) showed ≥35 mg/kg zinc content (Table 4). Thus, the development of rice breeding lines with target zinc content appears to be feasible from the recombination and selection, although the frequency of RILs with high zinc content appears to be less.
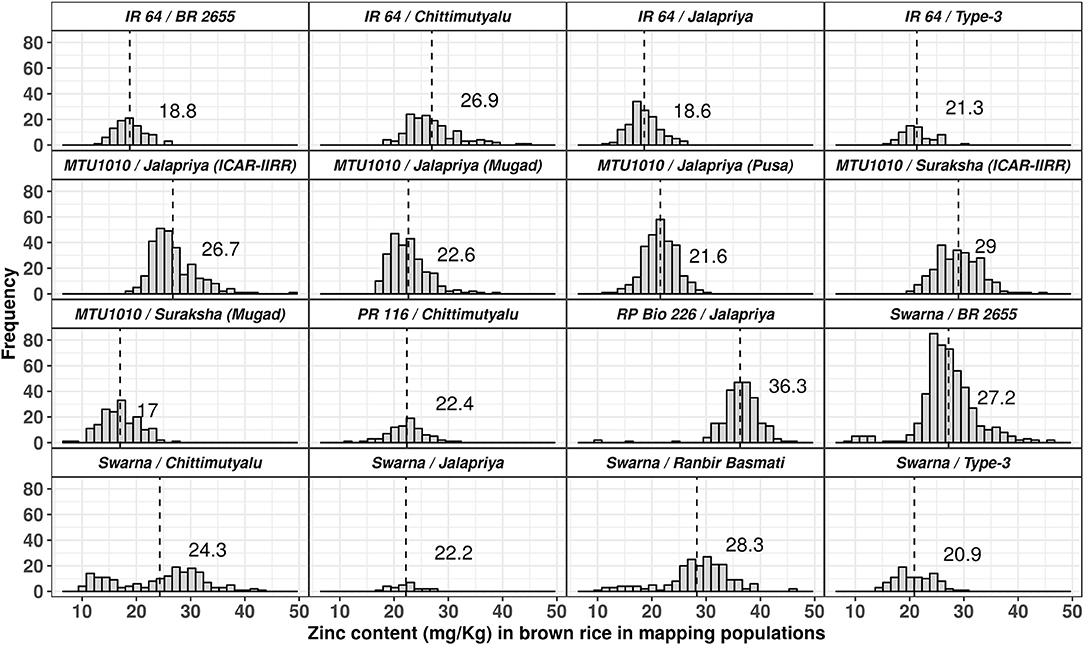
Figure 2. Distribution of zinc content in brown rice of 13 mapping populations evaluated at IIRR. MTU1010/Jalpriya also evaluated at ARS, Mugad, and RAU, Pusa. MTU1010/Suraksha also evaluated at ARS, Mugad.
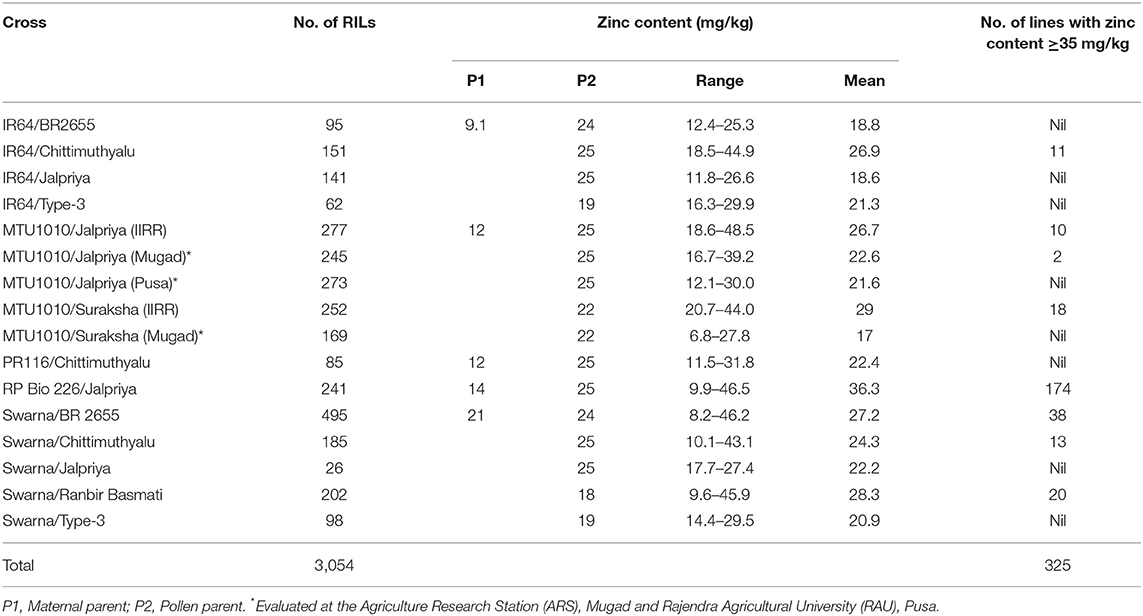
Table 3. Zinc content in brown rice of recombinant inbred lines (RILs) and their parents developed and evaluated at IIRR and the number of promising lines with zinc ≥35 mg/kg.
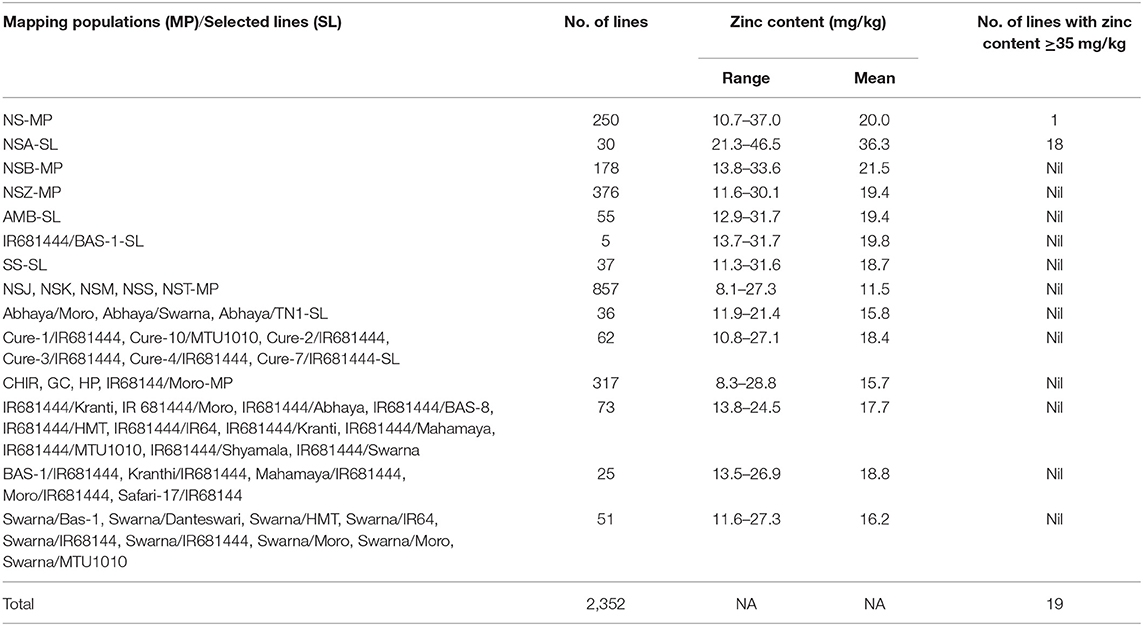
Table 4. Zinc content in brown rice of mapping populations and selected lines of mapping populations of various national institutions analyzed at the XRF facility of IIRR and the number of promising lines with zinc ≥35 mg/kg.
Multilocation studies of two mapping populations, viz., MTU1010/Suraksha and MTU1010/Jalpriya, in two to three locations indicated that the zinc content to be highly influenced by environment. Earlier studies also reported significant environmental variance for zinc in rice germplasm, transgenics developed for high zinc content, and mapping populations (29–31).
Significant negative correlation (P < 0.001) was observed between single plant yield and zinc content in Swarna/Chittimuthyalu and IR64/Chittimuthyalu RILs, whereas no correlation was observed in MTU1010/Suraksha and MTU1010/Jalpriya (Figure 3). Overall, lines with highest zinc content showed poor yield and vice versa. While most studies report negative associations between grain zinc content and yield, a few contradicting results of positive associations were also reported (32). The dilution effect of decrease of nutrient concentrations in plant tissues with the dry matter increase is generally observed in cereals, thus explaining the inverse relationship of zinc content and yield (33). Although negative correlations are observed when the associations were studied for complete set of mapping populations, individual recombinants of high yield and zinc were also obtained. In addition to higher zinc content, the biofortified rice varieties should have yield on a par with the cultivated varieties. Until now, there is no special price for biofortified rice grains in India; thus, there is no incentive for the farmer for growing the biofortified varieties. Thus, the adoption of the biofortified varieties by the farmers is possible only when the yield is on a par with the existing popular cultivated rice varieties. Cooking quality is also important for the rice varieties for their release and adoption. Thus, for biofortified rice varieties to be released and adopted, they should possess zinc content ≥35 mg/kg without yield penalty and desired cooking quality. Nevertheless, this combination happens rarely in germplasm, and thus, conscious breeding efforts are needed to develop biofortified rice varieties with desirable attributes.
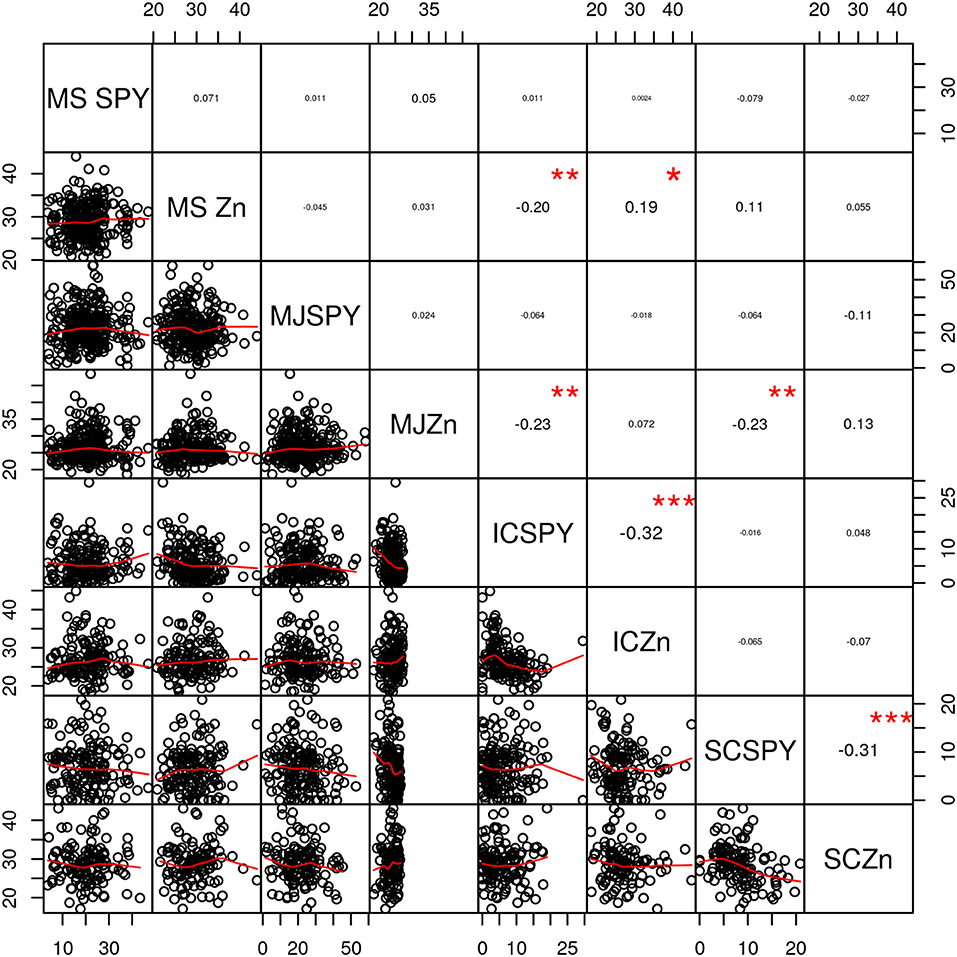
Figure 3. Correlation between zinc content in brown rice and single plant yield (SPY) in RILs. MS, MTU1010/Suraksha; MJ, MTU1010/Jalpriya; IC, IR64/Chittimuthyalu; SC, Swarna/Chittimuthyalu. *, **, and *** significant at the 0.05, 0.01, and 0.001 level.
Evaluation of Zinc Biofortified Rice Under AICRIP
With the breeding lines developed for high zinc in polished rice across the country from various projects sponsored by HarvestPlus, DBT, and ICAR, a biofortification breeding trial was initiated by AICRIP during 2013 to evaluate high zinc breeding lines without compromising yield, that is, yield must be on par the respective yield check or greater (IR64 or BPT5204). Up to 2013, the promising lines in the biofortification trial were identified with a modest threshold/target zinc value of 20 mg/kg. The optimum threshold content of zinc in polished rice since 2015 was increased to 24 mg/kg to match the international target set earlier by HarvestPlus. Considering the baseline zinc content as 16.25 mg/kg, target levels were recently revised to 28 mg/kg by HarvestPlus based on the daily requirement of the women, the consumption of rice along with absorption and retention of zinc in the body (15). However, the threshold level of 28 mg/kg still needs to be adopted by AICRIP. Since 2013, the biofortification trial has completed 5 years by April 2018 with five IVTs, four AVT 1s, and three AVT 2s (Table 5). A total of 170 entries were nominated at IVT level. Among these, 83 entries contain threshold zinc content ≥24 mg/kg, and 40 entries were found to contain desirable zinc and yield and exhibited desirable cooking quality. The 170 nominated lines represented several grain types, viz., short bold, short slender, long slender, long bold, medium slender, and medium bold and scented. From IVT, 32.3% of the test entries were promoted to AVT 1 and from AVT 1, 50.9% of the entries were promoted to AVT 2. As the AICRIP locations were categorized into seven zones, performance of each test entry is checked state-wise, zone-wise, and at country level every year. Thus, of the 170 breeding lines tested for 3 consecutive years across 5 years for their consistency in zinc content, yield, and cooking quality, only four promising test entries were recommended for their release based on their performance in one or more than one state or zones or more than one zone for 2 years to the Central Variety Release Committee (CVRC), India (Table 6, Supplementary Table 1). The promising lines in the state/s for two test entries were recommended to the State Variety Release Committee.
Effect of Environment on Grain Zinc Content of Rice
Although biofortified rice varieties have high grain zinc, the zinc content in the grains is highly variable, depending on the soils, seasons, agronomic practices according to AICRIP, and reported studies in India. The zinc content of two micronutrient check genotypes varied within and across locations and even within the locations across the experimental plots where IVT, AVT 1, and AVT 2 were conducted. The minimum ranges of 1.0 to 17.0 mg/kg for Kalanamak and 1.0 to 21.4 mg/kg for Chittimuthyalu were observed across 27 locations for 5 years of AICRIP studies. And maximum ranges of 9.5 to 21.4 mg/kg for Kalanamak and 12.4 to 36.3 mg/kg for Chittimuthyalu were noted (Supplementary Table 2). The grain zinc content of the four released varieties was also found to be variable with locations, as shown in Figure 4. Therefore, it is essential to identify suitable locations with favorable soils for large-scale seed production of biofortified varieties; otherwise, the potential of biofortified rice variety might not be realized because of zinc deficiency in the soil. Several countries including India, Pakistan, China, Iran, and Turkey come under zinc-deficient soils, and availability of zinc will further decrease if the soil pH is alkaline and if there is less organic matter and less moisture (3). And HYVs also take up zinc from the soil each cropping season, making the soil further deficient in zinc. Therefore, external application of zinc is essential to realize and maintain the nutrient performance potential of a biofortified rice variety.
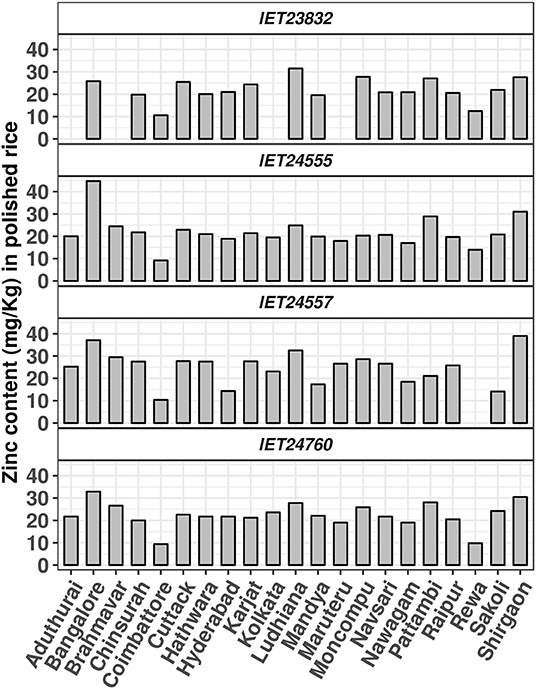
Figure 4. Zinc content mg/kg of zinc-biofortified varieties, released through CVRC evaluated across 21 locations under AICRIP Biofortification trial.
Biofortified Rice Varieties and Their Anticipated Impact
Considering the zinc content of the popular varieties of rice as 13 mg/kg and RDA of zinc as 12 mg/kg for male and 10 mg/kg for female (39), the zinc intake through rice consumption is 2.9 g/day per person. With the consumption of rice of existing popular varieties, the % RDA of zinc intake accounted for 24% for males and 29% for females. On the assumption that the consumption of biofortified varieties with higher zinc in polished rice will result in higher zinc intake, the effect of zinc biofortification through higher zinc intake and the extent of the improved intake to meet the RDA of zinc because of biofortified varieties in comparison to popular varieties are summarized in Table 7. With zinc-biofortified varieties, the zinc intake could account for 38 to 47% of the RDA for male and 46 to 57% of the RDA for female. The technology efficacy of zinc biofortification ranged from 48 to 67% for male and 55 to 75% for female.
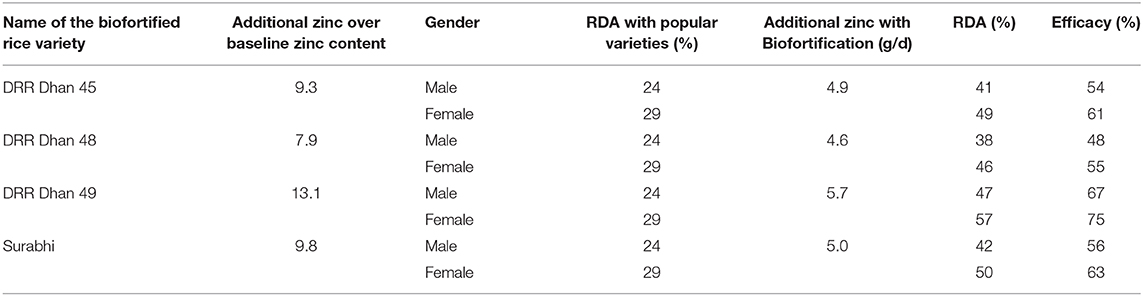
Table 7. Technology efficacy and potential impact of biofortified zinc varieties calculated based on the zinc content of the popular varieties of rice as 13 mg/kg as baseline; RDA of zinc as 12 mg/kg for male and 10 mg/kg for female; zinc intake through rice consumption as 2.9 g/day per person and considering per-capita consumption of rice in India as 220 g/day.
With rice as the sole diet component, in order to meet the RDA of zinc, polished grains should have 54.5 to 68.2 mg/kg zinc (without including bioavailability status) (40). Transgenic rice lines with soybean ferritin gene were developed for enhanced iron content in polished rice grains, but the same lines also accumulated higher amount of zinc ranging from 34.9 to 55.5 mg/kg (41). NASFer-274 event of IR64 transgenic rice with nicotianamine synthase (OsNAS2) and soybean ferritin (SferH-1) genes showed 45.7 mg/kg zinc content in polished rice without yield penalty or altered grain quality (31). Although zinc content of transgenic rice lines can meet the RDA of zinc, the transgenic rice lines are still to be accepted and adopted in India.
Our learning experiences since 2013 from the studies on characterization of germplasm for zinc content, development of national evaluation system for the release of biofortified rice varieties, and evaluation of biofortified rice breeding lines have been consolidated with reference to the five criteria set by the biofortification program (14).
As per criterion 1 (crop productivity, i.e., yield must be maintained or increased to ensure farmer acceptance), the recombination of zinc content and yield appears to be feasible as evidenced from the nominations made by several national research groups to biofortification trials and the release of four biofortified rice varieties through AICRIP.
Criterion 2 (impact of the enhanced micronutrient level on human health) has been indirectly confirmed through in vitro Caco-2 cell model studies of biofortified rice varieties. Because in vivo human clinical tests are expensive, labor-intensive, and subject-dependent response with, results of in vitro bioavailability studies can be explored as a possible alternative as proof of concept for zinc-biofortified rice varieties popularization. Bioavailability of biofortified rice variety with higher zinc in polished rice showed higher absorbed zinc through in vitro Caco-2 cell model and suckling rat pups studies (42). Preliminary studies of Indian Council of Medical Research–National Institute of Nutrition (ICMR-NIN) Hyderabad demonstrated that the zinc content and bioavailability from DRR Dhan 45 rice variety were almost twice those of the control IR64 (43). The clinical trial of one of the released biofortified rice varieties is under consideration by the Government of India.
According to criterion 3 (the enhanced micronutrient trait must be relatively stable across various edaphic environments and climatic zones), only the consistent genotypes across the states/zones and years are being released as biofortified zinc varieties under AICRIP in India. Multilocation data of AICRIP or independent research groups (31) indicated that the zinc content across the locations is highly variable and dependent on soil factors (Figure 4). Considering the wide diversity of agroclimatic regions of India, for the identification of the promising lines and their release as varieties, the data collected under AICRIP are being analyzed as performance state and zone-wise for the identification of stable varieties.
Criterion 4 addresses the bioavailability of micronutrients and is being carried out at ICMR-NIN. The coupled in vitro digestion/Caco-2 cell model studies with extrinsic 65Zn isotopic labeling demonstrated higher absorption of zinc from DRR Dhan 45 rice in intestinal cells. The proximate composition of DRR Dhan 45 and IR64 varieties remained similar, except that DRR Dhan 45 rice had a little higher protein. Detailed studies of zinc absorption of rice genotypes with differential zinc using coupled in vitro digestion/Caco-2 cell model studies are in progress. The overall food composition dictates the fate of intestinal absorption of zinc in diet. For zinc, phytate, fiber (cellulose, hemicellulose, lignin), oxalic acid, lectins, and heavy metals act as antinutrients, whereas organic acids (ascorbate, fumarate, malate, and citrate), long-chain fatty acids, β-carotene, and some of the amino acids act as pronutrients (44). As per criterion 5, consumer acceptance by taste and cooking quality is also taken care through a set of approved laboratory experiments in AICRIP coupled with panel test at IIRR.
Conclusions
Zinc deficiency, especially in the developing countries with rice as a major staple food, is being addressed by the development of biofortified rice varieties with high zinc in polished rice with the national and international support in India. Germplasm screening for their zinc content in brown and polished rice using ED-XRF revealed wide genetic variability, and evaluation of mapping populations indicated the possibility of favorable recombination of high zinc content and yield. Although only 2.4% biofortified varieties were released out of the total test entries until 2017, the rigorous screening through AICRIP biofortification indicated the achievability of combining target zinc content, yield, and quality parameters. A preliminary study of bioavailability of a released biofortified rice variety was found to be twice that of control variety IR64. The technology efficacy and the enhancement of RDA calculated with the four released varieties were found to be promising. Thus, the benefits of biofortification research are being translated to the society as biofortified rice varieties with high zinc, hoping for their adoption and impact in achieving the nutritional security of the needy.
Data Availability Statement
All datasets generated for this study are included in the article/Supplementary Material.
Author Contributions
DS: analyses and writing of the manuscript. CN: planning and writing of the manuscript. PM, PS, and RK: evaluation of mapping populations. BN: economic impact of biofortification. KSum: writing of the manuscript. LR: AICRIP analyses. KSur: editing of the manuscript. PR and TL: bioavailability studies. VB and SV: Coordination of the study.
Funding
This work was supported by ICAR funded project ICAR plan CRP Biofortification, India.
Conflict of Interest
The authors declare that the research was conducted in the absence of any commercial or financial relationships that could be construed as a potential conflict of interest.
Acknowledgments
The authors acknowledge to ICAR-CRP-Biofortification project, ICAR-Indian Institute of Rice Research, Hyderabad for financial support and providing facilities and HarvestPlus for providing ED-XRF. Authors acknowledge AICRIP for the Biofortification trial reports and cooperating centers.
Supplementary Material
The Supplementary Material for this article can be found online at: https://www.frontiersin.org/articles/10.3389/fnut.2020.00026/full#supplementary-material
Abbreviations
ED-XRF, Energy Dispersive X-ray fluorescence spectroscopy; RIL, Recombinant Inbred Line; ICAR, Indian Council of Agricultural Research; ICMR, Indian Council of Medical Research; IIRR, Indian Institute of Rice Research; AICRIP, All India Coordinated Rice Improvement Programme; NIN, National Institute of Nutrition; CRP, Consortia Research Platform; ARS, Agricultural Research Station; IVT, Initial Variety Trial; AVT, Advance Varietal Trial.
References
1. Timsina J, Connor DJ. Productivity and management of rice-wheat cropping systems: issues and challenges. F Crop Res. (2001) 69:93–132. doi: 10.1016/S0378-4290(00)00143-X
2. United States Department of Agriculture (USDA). World Agricultural Production. (2019) Available online at: https://apps.fas.usda.gov/psdonline/circulars/production.pdf (accessed July 2019).
3. Cakmak I. Enrichment of cereal grains with zinc: Agronomic or genetic biofortification? Plant Soil. (2008) 302:1–17. doi: 10.1007/s11104-007-9466-3
4. Hotz C, Brown KH. Contents international zinc nutrition consultative group (IZiNCG) technical document. Food Nutr Bull. (2004) 25:S94–200.
5. Black RE, Allen LH, Bhutta ZA, Caulfield LE, de Onis M, Ezzati M, et al. Maternal and child undernutrition: global and regional exposures and health consequences. Lancet. (2008) 371:243–60. doi: 10.1016/S0140-6736(07)61690-0
6. Tan Z, Ma G, Lin L, Liu C, Liu Y, Jiang J, et al. Prevalence of subclinical vitamin A deficiency and its affecting factors in 8,669 children's of China. Zhonghua Yu Fang Yi XueZaZhi. (2002) 6:161–3.
7. Prasad AS. Metabolism of zinc and its deficiency in human subjects. In: Prasad AS, Thomas CC, editors. Zinc Metabolism. Springfield, IL (1966). p. 250–302.
8. Paul W. Iron and zinc interactions in humans. Am J Clic Nutr. (1998) 68:442S−6S. doi: 10.1093/ajcn/68.2.442S
9. Nirmala B, Babu VR, Neeraja CN, Waris A, Muthuraman P, Rao DS. Linking agriculture and nutrition: an ex-ante analysis of zinc biofortification of rice in india. Agric Econ Res Rev. (2016) 29:171. doi: 10.5958/0974-0279.2016.00044.6
10. Sally Brooks. Is international agricultural research a global public good? The case of rice biofortification. J Peasant Stud. (2011). 38:67–80. doi: 10.1080/03066150.2010.538581
11. HarvestPlus. Breeding Crops for Better Nutrition: Harnessing Agricultural Technology to Improve Micronutrient Deficiencies. Washington, DC: International Food Policy Research Institute. (2004).
12. Bouis HE, Graham RD, Welch RM. The consultative group on international agricultural research (CGIAR) micronutrients project: justification and objectives. Food Nutr Bull. (2000) 21:374–81. doi: 10.1177/156482650002100406
13. Gregorio GB. Progress in breeding for trace minerals in staple crops. Symposium: plant breeding: a new tool for fighting micronutrient malnutrition. J. Nutr. (2002) 132:500S−2S. doi: 10.1093/jn/132.3.500S
14. Welch RM, Graham RD. Breeding for micronutrients in staple food crops from a human nutrition perspective. J Exp Bot. (2004) 55:353–64. doi: 10.1093/jxb/erh064
15. Bouis HE, Saltzman A. Improving nutrition through biofortification: a review of evidence from harvestplus, 2003 through (2016). Glob Food Sec. (2017) 12:49–58. doi: 10.1016/j.gfs.2017.01.009
16. Babu VR, Neeraja CN, SanjeevaRao D, Sundaram RM, Longvah T, Usharani G, et al. Bioforotification in Rice. DRR Technical Bulletin No. 81/2014. Hyderabad: Directorate of Rice Research. (2014) pp. 86.
17. Wailes E, Chavez E. International rice baseline with deterministic and stochastic projections, 2012-2021. Staff Pap. (2012)
18. Wessells KR, Brown KH. Estimating the global prevalence of zinc deficiency: results based on zinc availability in national food supplies and the prevalence of stunting. PLoS ONE. (2012) 7:e50568. doi: 10.1371/journal.pone.0050568
19. Chandel G, Banerjee S, See S, Meena R, Sharma DJ, Verulkar SB. Effects of different nitrogen fertilizer levels and native soil properties on rice grain Fe, Zn and protein contents. Rice Sci. (2010) 17:213–27. doi: 10.1016/S1672-6308(09)60020-2
20. Anandan A, Rajiv G, Eswaran R, Prakash M. Genotypic variation and relationships between quality traits and trace elements in traditional and improved rice (Oryza sativaL.) genotypes. J Food Sci. (2011) 76:H122–30. doi: 10.1111/j.1750-3841.2011.02135.x
21. Nachimuthu VV, Robin S, Sudhakar D, Raveendran M, Rajeswari S, Manonmani S. Evaluation of rice genetic diversity and variability in a population panel by principal component analysis. Indian J Sci Technol. (2014) 7:1555–62.
22. Jeng TL, Lin YW, Wang CS, Sung JM. Comparison and selection of rice mutents with high and zinc contents in their polished grains that were mutated from the indica type cultiver IR64. J Food Compost Anal. (2012) 28:149–54. doi: 10.1016/j.jfca.2012.08.008
23. Sellappan K, Datta K, Parkhi V, Datta SK. Rice caryopsis structure in relation to distribution of micronutrients (iron, zinc, β-carotene) of rice cultivars including transgenic indica rice. Plant Sci. (2009) 177:557–62. doi: 10.1016/j.plantsci.2009.07.004
24. Zazoli MA, Shokrzadeh M, Bazerafshan E, Hazrati M, Tavakkouli A. Investigation of zinc content in Iranian rice (Oryza sativa) and its weekly intake. Am Eurasian J Agric Environ Sci. (2006) 1:156–9.
25. Thongbam PD, Raychaudhury M, Durai A, Das SP, Ramesh T, Ramya KT, et al. Studies on grain and food quality traits of some indigenous rice cultivars of north-eastern hill region of India. J Agric Sci. (2011) 4:259–70. doi: 10.5539/jas.v4n3p259
26. Jeomho L, Kyuseong L, Hunggoo H, Changihn Y, Sangbok L, Younghuran C, et al. Evaluation of iron and zinc content in rice germplasm. Korean J Breed Sci. (2008) 40:101–5.
27. Franz KB, Kennedy BM, Fellers DA. Relative bioavailability of zinc from selected cereals and legumes using rat growth. J Nutr. (1980) 110:2272–83. doi: 10.1093/jn/110.11.2272
28. Gregorio GB, Senadhira D, Htut H, Graham RD. Breeding for trace mineral density in rice. Food Nutr Bull. (2000) 21:382–6. doi: 10.1177/156482650002100407
29. Huang Y, Tong C, Xu F, Chen Y, Zhang C, Bao J. Variation in mineral elements in grains of 20 brown rice accessions in two environments. Food Chem. (2016) 192:873–8. doi: 10.1016/j.foodchem.2015.07.087
30. Hu BL, Huang DR, Xiao YQ, Fan YY, Chen DZ, Zhuang JY. Mapping QTLs for mineral element contents in brown and milled rice using an Oryza sativa × O. rufipogon backcross inbred line population. Cereal Res Commun. (2016) 44:57–68. doi: 10.1556/0806.43.2015.044
31. Trijatmiko KR, Duenãs C, Tsakirpaloglou N, Torrizo L, Arines FM, Adeva C, et al. Biofortified indica rice attains iron and zinc nutrition dietary targets in the field. Sci Rep. (2016) 6:19792. doi: 10.1038/srep19792
32. Swamy BPM, Rahman MA, Inabangan-Asilo MA, Amparado A, Manito C, Chadha-Mohanty P, et al. Advances in breeding for high grain zinc in rice. Rice. (2016) 9:49. doi: 10.1186/s12284-016-0122-5
33. Mcdonald G, Genc Y. A simple method to evaluate genetic variation in grain zinc concentration by correcting for differences in grain yield. Plant Soil. (2008) 306:49–55. doi: 10.1007/s11104-008-9555-y
34. Directorate of Rice Research (DRR). Progress Report 2013. Varietal Improvement. All India Coordinated Rice Improvement Programme (ICAR). Vol. 1. Hyderabad: Directorate of Rice Research (2014).
35. Indian Institute of Rice Research (ICAR). Progress Report 2014. Varietal Improvement All India Coordinated Rice Improvement Programme. Vol. 1. Hyderabad: ICAR- Indian Institute of Rice Research (formerly Directorate of Rice Research) (2015).
36. Indian Institute of Rice Research (ICAR). Progress Report 2015. Varietal Improvement All India Coordinated Rice Improvement Programme. Vol. 1. Hyderabad: ICAR- Indian Institute of Rice Research (formerly Directorate of Rice Research) (2016).
37. Indian Institute of Rice Research (ICAR). Progress Report 2016. Varietal Improvement All India Coordinated Rice Improvement Programme. Vol. 1. Hyderabad: ICAR- Indian Institute of Rice Research (formerly Directorate of Rice Research) (2017). Available online at: http://www.icar-iirr.org/AICRIP/Volume_1Progress%20Report%20Revised-2016.pdf (accessed July 2019).
38. Indian Institute of Rice Research (ICAR). Progress Report-2017, Varietal Improvement All India Coordinated Rice Improvement Programme. Vol. 1. Hyderabad: ICAR- Indian Institute of Rice Research (formerly Directorate of Rice Research) (2018). Available online at: http://www.icariirr.org/AICRIP/Varietal%20Improvement%20Progress%20Report%202017.pdf (accessed July 2019).
39. National Institute of Nutrition (NIN). Nutritional Intake in India. NSS Report No. 560 (2011). Available online at: http://www.indiaenvironmentportal.org.in/files/file/nutritional%20intake%20in%20India%202011-12.pdf
40. Sanjeeva Rao D, Babu PM, Swarnalatha P, Kota S, Bhadana VP, Surekha K, et al. Assessment of grain zinc and iron variability in rice germplasm using energy dispersive X-ray fluorescence spectrophotometer (ED - XRF). J Rice Res. (2014) 7:45–52.
41. Vasconcelos M, Datta K, Oliva N, Khalekuzzaman M, Torrizo L, Krishnan S, et al. Enhanced iron and zinc accumulation in transgenic rice with the ferritin gene. Plant Sci. (2003) 164:371–8. doi: 10.1016/S0168-9452(02)00421-1
42. Jou MY, Du X, Hotz C, Lönnerdal B. Biofortification of rice with zinc: assessment of the relative bioavailability of zinc in a caco-2 cell model and suckling rat pups. J Agric Food Chem. (2012) 60:3650–7. doi: 10.1021/jf202338t
43. Neeraja Voleti CN SR. Consortia Research Plotform (CRP) Biofortification in Selected Crops for Nutritional Security-Status. Vol. 104. Technical bulletin. Hyderabad: ICAR-Indian Institute of Rice Research (2019) . p. 1–84.
Keywords: rice, biofortification, high zinc, germplasm, varieties, AICRIP, RIL's
Citation: Sanjeeva Rao D, Neeraja CN, Madhu Babu P, Nirmala B, Suman K, Rao LVS, Surekha K, Raghu P, Longvah T, Surendra P, Kumar R, Babu VR and Voleti SR (2020) Zinc Biofortified Rice Varieties: Challenges, Possibilities, and Progress in India. Front. Nutr. 7:26. doi: 10.3389/fnut.2020.00026
Received: 27 August 2019; Accepted: 25 February 2020;
Published: 07 April 2020.
Edited by:
Sachiko Narita Isobe, Kazusa DNA Research Institute, JapanReviewed by:
Nimai Prasad Mandal, Indian Council of Agricultural Research, IndiaChikara Kuwata, Chiba Prefectural Agriculture and Forestry Research Center, Japan
Copyright © 2020 Sanjeeva Rao, Neeraja, Madhu Babu, Nirmala, Suman, Rao, Surekha, Raghu, Longvah, Surendra, Kumar, Babu and Voleti. This is an open-access article distributed under the terms of the Creative Commons Attribution License (CC BY). The use, distribution or reproduction in other forums is permitted, provided the original author(s) and the copyright owner(s) are credited and that the original publication in this journal is cited, in accordance with accepted academic practice. No use, distribution or reproduction is permitted which does not comply with these terms.
*Correspondence: C. N. Neeraja, cnneeraja@gmail.com