- 1UO Nefrologia, Azienda Ospedaliero-Universitaria di Parma & Dipartimento di Medicina e Chirurgia, Università di Parma, Parma, Italy
- 2Dipartimento di Medicina e Chirurgia, Università di Parma, Parma, Italy
- 3Diagnostic and Interventional Radiology, IRCCS Istituto Ortopedico Rizzoli, Bologna, Italy
Kidney transplantation is the treatment of choice for most of the patients with end-stage renal disease (ESRD). It improves quality of life, life expectancy, and has a lower financial burden to the healthcare system in comparison to dialysis. Every year more and more older patients are included in the kidney transplant waitlist. Within this patient population, transplanted subjects have better survival and quality of life as compared to those on dialysis. It is therefore crucial to select older patients who may benefit from renal transplantation, as well as those particularly at risk for post-transplant complications. Sarcopenia and frailty are frequently neglected in the evaluation of kidney transplant candidates. Both conditions are interrelated complex geriatric syndromes that are linked to disability, aging, comorbidities, increased mortality, and graft failure post-transplantation. Chronic kidney disease (CKD) and more importantly ESRD are characterized by multiple metabolic complications that contribute for the development of sarcopenia and frailty. In particular, anorexia, metabolic acidosis and chronic low-grade inflammation are the main contributors to the development of sarcopenia, a key component in frail transplant candidates and recipients. Both frailty and sarcopenia are considered to be reversible. Frail patients respond well to multiprofessional interventions that focus on the patients' positive frailty criteria, while physical rehabilitation and oral supplementation may improve sarcopenia. Prospective studies are still needed to evaluate the utility of formally measuring frailty and sarcopenia in the older candidates to renal transplantation as part of the transplant evaluation process.
Introduction
Kidney transplantation is the treatment of choice for most of the patients with end-stage renal disease (ESRD). In fact, it improves quality of life (1, 2), life expectancy (3–5), and has a lower financial burden to the healthcare system in comparison to dialysis (6). The increased prevalence of ESRD in parallel with stable or decreased organ availability has led to an increase in the average waiting time for transplantation. In addition, every year more and more older patients are candidates for the enrollment in the kidney transplant list (7). Although in this case patient and graft survival are lower than those for younger recipients (7), kidney transplant guarantees, also in this category, a survival advantage (4, 8) and better quality of life (9, 10) as compared to dialysis. Therefore, it is crucial to select which older patient may benefit from renal transplantation, and which are at high risk for post-transplant complications.
The suitability for transplantation is based on the evaluation of the balance between the risks faced by the patient undergoing the procedure and the risks associated with staying on dialysis. Sarcopenia and frailty are frequently neglected in the evaluation of kidney transplant candidates. However, these two geriatric syndromes have many overlapping causes and consequences with major impact in this specific clinical setting.
As a matter of fact, sarcopenia, which is characterized by a loss of muscle mass and function, correlates with increased mortality and graft failure (11, 12) while frailty, a measure of reduced functional reserve and increased vulnerability of the organism to stressful events, is associated with an increased incidence of delayed graft function (13), reduced tolerance to immunosuppressive drugs such as mycophenolate mofetil (14), increased risk of early rehospitalization (15) and mortality in patients with kidney transplantation (16–18). In the present review, we will thoroughly discuss the pathogenesis and epidemiology of frailty and sarcopenia in older transplant candidates and recipients.
Frailty Definition and Pathogenesis
Even though the concept of frailty derives from the necessity born in the geriatric field of assessing “biological age” and predicting outcomes in older people (14), now it is spreading across many different clinical contexts. Older people are at increased risk for mortality, morbidities and hospitalization (19). However, the chronological age cannot be considered the only precise and linear measure of the risk of frailty and sarcopenia, and many factors are contributing to increasing the risk of these complications. Moreover, even after taking into consideration the different comorbidities, it is not straightforward to predict the vulnerability to adverse outcomes in the individual elderly subject.
From this gap of knowledge, the concept of frailty was born. The frailty syndrome is characterized by a reduced functional reserve, vulnerability to stressors and increased risk for adverse clinical outcomes (20–24). The main innovation of the frailty concept is the predictive power of developing future complications in response to hypothetic stressors.
It has been suggested that this clinical syndrome is the nonlinear result of multiple dysfunctional biological systems, regardless of the individual dysfunction, chronic disease, and chronological age progressively dysreguling the organism homeostasis (25–33). First of all, chronological age itself has been associated with progressive dysregulation of the organism homeostasis. Mild proinflammatory state (i.e., as revealed by increased IL-6, C-reactive protein [CRP], leukocyte count, and lymphocyte activation pathways), hypercoagulation, anemia, impaired endocrine system, micronutrient deficiencies, neuromuscular deficits have been also associated with the development of frailty (22, 26, 34–37). Moreover, abnormal metabolic systems can interact with the above factors and, increasing the risk for frailty (i.e., abnormal IGF-1, glucose intolerance, DHEA-S and vitamin D) (38–40). Depression and cognitive impairment are additional risk factors for the development of the frailty syndrome (41–44).
Frailty etiology and progression has a spiraling nature (Figure 1), depending on the total number of the dysfunctional systems involved, rather than on the severity of each system dysfunction (37). Therefore, the frail organism has to find a new precarious balance among these systems that can be disrupted easily by stressors leading to adverse outcomes. The major obstacle to the success of such concept was the absence of a simple, reliable and standardized method aimed at screening for frailty (19, 46–48).
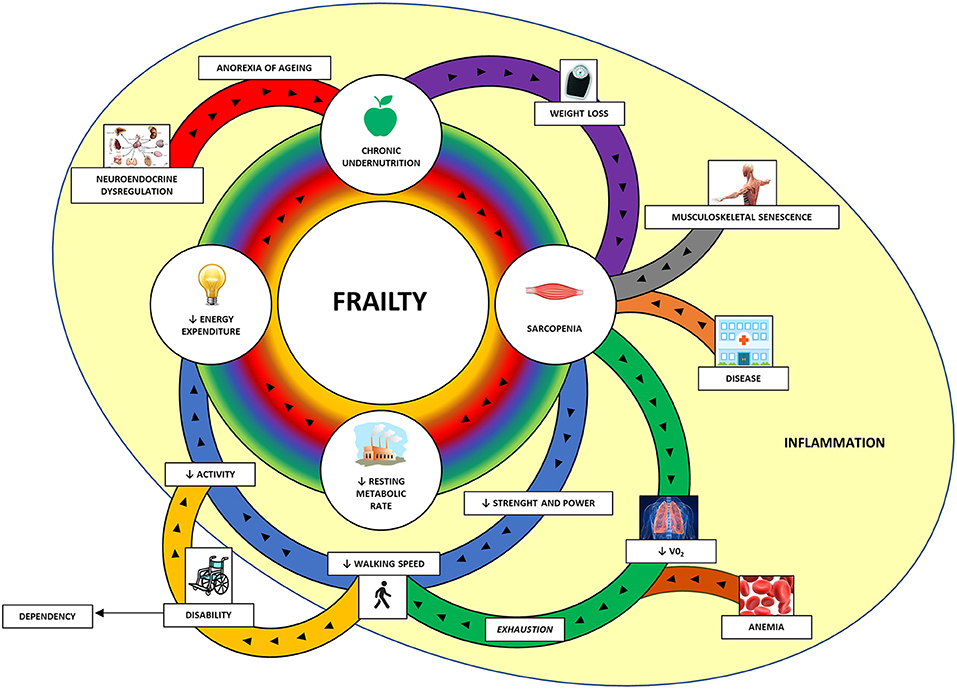
Figure 1. The cycle of frailty (45). The four key factors of frailty (in the central circle) are sarcopenia, lower resting metabolic rate, lower energy expenditure, and chronic undernutrition. These factors are interconnected and amplifying the process and it is hard to distinguish the first factor that started it. In the peripheral area are represented the contributors to these factors. The main contributors to sarcopenia are weight loss (through protein and micronutrient deficit and increased catabolism), musculoskeletal senescence and various diseases. Sarcopenia itself, through the reduction of muscle strength and power, provokes one of the key features of the frailty assessment: the reduced walking speed. The diaphragm and visceral muscle sarcopenia along with anemia are responsible for reduced oxygen uptake (VO2) and subsequent exhaustion, which contribute in slowing the walking speed. Reduced walking speed is associated with lower physical activity and subsequent lower energy expenditure. In some cases, the marked reduced walking capacity can configure a disability with loss of independency and need for assistance. Neuroendocrine dysregulation, frequently observed in older people, can reduce the appetite (anorexia of aging) and contribute to chronic undernutrition along with the lower energy expenditure with subsequent weight loss. A mild and chronic inflammation can have a negative impact in these processes. VO2, oxygen uptake.
Since frail patients frequently have reduced lean body mass, endurance, balance, strength, and walking speed, as well as low physical activity, all of the frailty scores include measurement of self-reported physical activity and tests for directly quantifying physical activity and sarcopenia (37, 45, 49, 50). In particular, the Fried phenotype score, the most well-known frailty assessment, is based on five components of physical impairment in older adults: weakness (measured by grip strength), slowness (measured by walking speed), low level of physical activity in the past 2 weeks, self-reported exhaustion, and unintentional weight loss (measured by a questionnaire) (37, 51, 52). Individuals are considered frail if 3 or more of the listed components are present, pre-frail if at least one of the components is present or non-frail (zero component) (Table 1). This test is therefore able to identify easily the presence and score the degree of frailty, and to predict negative clinical outcomes, such as the incidence of falls, worsening mobility, hospitalization, and death. The robustness of the Fried score have been documented in the large Cardiovascular Health Study (CHS), and the test has been successfully applied to patient cohorts of Women's Health and Aging Studies (40).
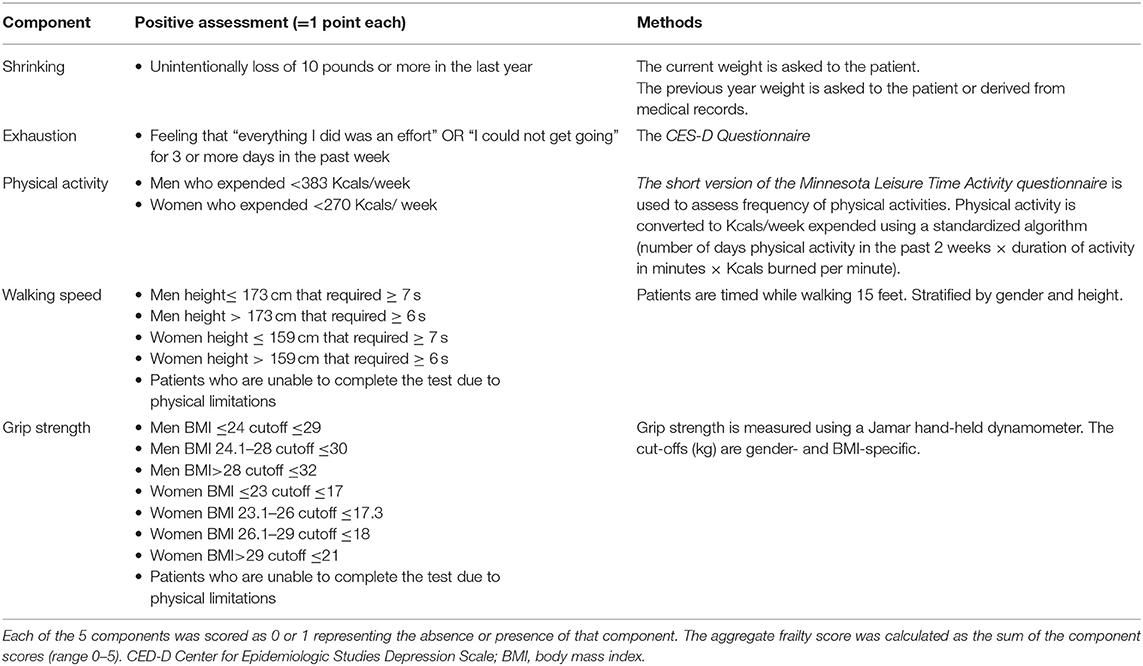
Table 1. Measurement and Definition of Frailty Components using the Fried criteria (45).
In consideration of the relationship between chronic disease and frailty, some authors took into consideration the presence and severity of chronic diseases in their “Frailty Index” (19, 53–57). This score appears more sensitive and precise to estimate biological age, however its clinical application has been hampered by time consumption and the lack of distinguishing frailty from comorbidity and disability. The relationship between chronic disease and frailty is not clear and although they often share the same etiology and biological pathways as well as many chronic conditions contribute to frailty, the presence of chronic disease does not necessarily mean frailty.
Another critical component of frailty is the cognitive impairment, and for this reason, when the Fried phenotype score was combined with the assessment of cognitive impairment and depression symptoms, a significant association with disability and worsening quality of life at 12 months was observed (41–44). Along with the development of the frailty concept, many authors tried to combine more and more elements in order to increase the predictive power of the score, including memory tests (58), comorbidities and malignancies (59), anthropometric measures, laboratory markers and caregiver reports (60). As a consequence, in face of a minimal increased predictive power, these scores are applicable only by trained geriatric teams, being time consuming in the clinical setting (61).
Frailty in Transplant Candidates
Thanks to the increased life expectancy and the improvement in medical and surgical management, an increasing number of patients with CKD (62) over 65 years are evaluated for kidney transplant waiting list (63, 64). A number of studies have, indeed, shown a significant improvement in the overall life expectancy (mortality risk 40–60% lower) for older patients receiving a kidney transplant compared to similar waiting-listed patients remaining on dialysis (65–70), even taking into consideration the significantly higher incidence of early mortality (65, 68, 69, 71) and the use of extreme aged (>75 years) cadaveric donors (70).
It is therefore crucial to evaluate at the time of wait listing evaluation which older kidney transplant candidate will benefit from kidney transplantation, and which are subject to an unacceptable risk of adverse events following transplantation (64).
Frailty as defined by Fried has been proposed as a practical and useful assessment in clinical practice evaluation for kidney transplant waiting list (17). The prevalence of frailty is higher among dialysis patients, and it increases with age: 44% of dialysis patients under 40 years are frail, while this prevalence reach 78% among dialysis patients above 70 years. In addition, functional decline is highly prevalent in older patients during the first 6 months of dialysis, especially in the presence of frailty (72). Patients with CKD (62) typically present many risk factors for frailty (71), such as anemia, osteoporosis, cardiovascular disease and chronic inflammation (72, 73). They also experience neuroendocrine changes, like 25-OH vitamin D deficiency, insulin-resistance (73) and low testosterone levels (74), commonly associated with frailty and sarcopenia (32). Since dietary limitations are also part of the medical prescription to CKD patients, in order to reduce the daily intake of phosphorus, potassium, salt and water, this very fact can exacerbate malnutrition and contribute to sarcopenia (75). Moreover, cognitive impairment is common among ESRD patients, with a prevalence of cognitive decline in older patients on hemodialysis between 16 and 38% (76–79).
The investigation of frailty and its effect on transplant outcomes, could help the clinicians to inform the ESRD patients on treatment options and tailor the pre- and post-transplant follow up strategies (80). In fact, a prospective study of ESRD patients on hemodialysis, has linked frailty to a 2.6-fold increase of mortality risk (95% confidence interval, 1.04–6.49; P = 0.041). Of note, this important risk increase has been independent of age, sex, comorbidities, and disability (16). Also, in kidney transplant candidates, who are generally selected among “healthier” patients on dialysis, frailty has been significantly associated with mortality on the transplant waiting list (hazard ratio [HR]: 6.7, confidence interval [CI]: 1.5–30.1; P = 0.01) independently of age, diabetes, or duration of dialysis (81).
Frail kidney transplant candidates are also less likely to be listed for kidney transplantation compared to non-frail patients (hazard ratio, 0.62; 95% confidence interval, 0.56–0.69; P < 0.001), independently of age and other demographic factors (82), and they are by one third less frequently transplanted than their non-frail counterpart (incidence rate ratio, 0.68; 95% confidence interval, 0.58–0.81; P < 0.001) (82).
Recently it has been shown that almost 50% of candidates experience a frailty phenotype worsening while on waiting list, and that these changes are associated with increased risk of mortality and longer hospitalization. Monitoring these changes can in fact improve the post-transplant risk stratification, and modify the suitability to kidney transplantation (83).
Frailty in Transplant Recipients
Patients that are considered frail at the time of transplant have more than 2-fold adjusted risk of mortality (95% CI: 1.01–4.65, p = 0.047) (17) compared to non-frail patients, independently of recipient age (13). Frailty has been also associated with a 1.94-fold increased risk for delayed graft function within the first post-transplant week (95% CI, 1.13–3.36; P = 0.02) (15), and increased rate of both morbidity and hospital readmission (adjusted RR = 1.61, 95% CI: 1.18-2.19, p = 0.002) in the first month after surgery (15). Frail kidney transplant recipients are more susceptible to drug-related adverse events, like in the case of mycophenolate mofetil use, with a 1.29-times (95%CI:1.01–1.66; P = 0.04) more frequent need for dose reduction (14), being this latter effect independently associated with a substantially increased risk of death-censored graft loss (aHR, 5.24; 95% CI, 1.97–13.98, P = 0.001) (14). Finally, frailty has been recently associated with medium-term cognitive decline (measured by Modified Mini-Mental State Examination) post-kidney transplantation (84).
Despite the increasing evidence of the role of frailty in predicting the pre- and post- transplant outcomes, no current guidelines are indicating a threshold of frailty score at which a patient should be excluded from the waiting list (64).
Notably, after an initial worsening early after kidney transplantation, adult recipients of all ages experience an improvement of the frailty score at 3 months (85). And despite the fact that patients that are considered frail at the moment of transplantation, still present a higher frailty score over time, these patients are more likely to show an improvement after transplantation, showing the reversible state of frailty and the advantage of transplanting this population (85). Moreover, frail patients reported a better improvement in post-transplant health-related quality of life compared to non-frail transplant recipients (frail, 1.35 points/month; 95% confidence interval [CI], 0.65–2.05; non-frail, 0.34 points/month; 95% CI, −0.17–0.85; P = 0.02) (86).
Recent KDIGO guidelines suggest that patients should be evaluated for frailty at the time of listing and while on the waiting list, in order to better define inherent risks and enable optimization strategies (87). Further studies are needed to investigate the role of frailty in predicting specific post-transplant outcomes (88) and define the phenotypes of frail transplant candidates who are expected to benefit the most from transplantation over dialysis, in order to tailor the clinical approach to their unique needs and develop interventions to reverse frailty both pre- and post- transplantation (87).
Sarcopenia Definition and Pathogenesis
Sarcopenia (from the Greek sarx = flesh, penia = scarcity) is a complex geriatric syndrome associated with the loss of muscle mass and reduced muscle strength (Table 2) (89). Sarcopenia can be defined as primary or secondary. In the first case, it is a sole consequence of aging, while secondary sarcopenia has a multifactorial etiology, and include as possible causes the decline in physical activity, alterations of the endocrine system, presence of comorbidities, inflammation, insulin resistance and nutritional inadequacy (90). However, in a clinical setting characterized by prevalent older subjects, both age-related and diseases related factors play a role in the development of sarcopenia. Reduced muscle strength leads to a reduction in muscle performance, and is a major cause of disability, mortality and other adverse outcomes (91) (Figure 1).
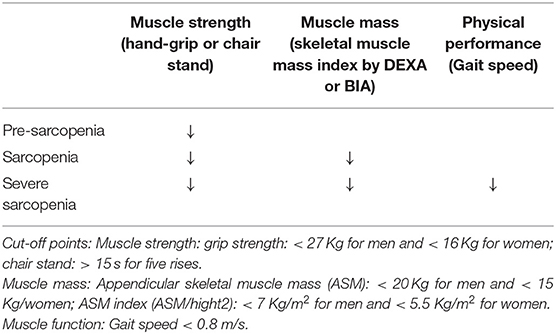
Table 2. Criteria for sarcopenia by the European Working Group for Sarcopenia (EWGSOP2) (88).
The 2010 European Working Group on Sarcopenia in Older People (92) recognized for the first time that muscle strength is also an important component of sarcopenia. The group defined the syndrome as a progressive loss of skeletal muscle mass and strength, increasing the risk for the development of physical disability, poor quality of life and death (92–98).
Despite being primarily considered a natural part of aging, the degree of muscle loss is highly variable and depends on the presence of some risk factors. Muscle homeostasis is maintained thanks to a fine balance between the formation of new myocytes, hypertrophy and protein catabolism. This equilibrium is controlled by the nervous, endocrine and immune systems, and is highly affected by nutrition and physical activity (99).
Lack of exercise is believed to be the most important risk factor for the development of secondary sarcopenia (100). Muscle mass picks at around 30 years of age and starts to decline in a rate of 0.5–1% every year, accelerating after 65 years of age (101, 102). A 30% cumulative loss of muscle mass and a 20% loss in muscle cross-sectional area until reaching 80 years have been described (103), being the reduction in muscle fibers and strength more pronounced in people with a sedentary lifestyle.
Hormonal imbalances, including age-related reduction in growth-hormone (GH), testosterone, thyroid hormones, insulin-resistance, reduced IGF-1, and increased cortisol lead to loss of muscle mass and strength (104). Particularly, the reduction of hormonal anabolic signals and the increase in catabolic signals promoted by glucocorticoids and pro-inflammatory cytokines such as TNF-α and IL-6 both contribute to the loss of muscle mass (104).
Inflammatory cytokines activate muscle ring finger 1 (MURF1), which, like atrogin-1, activates the ubiquitin-proteasome degradation system. In addition, they also cause apoptosis through the activation of NF-kB, and activation of the caspase 8. Increased concentrations of TNF-α and IL-6 have been described in older adults (105).
In parallel to the reduced ability to synthetize muscle fibers, a reduction in energy and protein intake is common in the development of sarcopenia. The lack of protein to sustain muscle mass associates with accumulation of oxidized proteins that are scarcely removed from the muscle via the proteolytic system, leading to an increase of non-contractile dysfunctional protein content in the skeletal muscles. This effect is thought to explain, at least in part, why muscle strength is severely decreased in sarcopenia (106).
Sarcopenia in Kidney Transplant Candidates
Sarcopenia is a frequent finding in kidney transplant candidates (107), in whom muscle loss occurs at a younger age, and more markedly, in comparison to age-matched controls (108, 109). This phenomenon has been associated to nutritional problems, chronic diseases, sedentary lifestyle and drug-related side effects (110, 111), and its prevalence seems to be related with worsening of renal function (112).
Currently available evidence divides the causes of sarcopenia in kidney transplant candidates into two groups: causes related to the kidney disease itself, and causes related to the chronic low-grade inflammatory process typical of patients on dialysis, but also present in earlier stages of the disease (113, 114). Factors related to kidney disease that contribute to the development of sarcopenia include nutritional deficits and consequent malnutrition, vitamin D deficiency, metabolic acidosis, insulin resistance, low physical activity, hyperparathyroidism, and proteinuria (115).
Inadequate nutrient intake is the most important factor that contributes to the development of sarcopenia in these patients. Progressive loss of appetite begins already in the earlier stages of CKD (116), and it worsens in parallel to the loss of renal function. In this clinical setting anorexia can be viewed as the consequence of the complex negative interactions between metabolic signaling, accumulation of uremic toxins, alterations of factors that regulate appetite (such as gastric mediators, adipokines, and cytokines), and altered hypothalamic signaling (116). In addition, CKD patients on dietary nutritional treatment (the so called conservative treatment) usually undergo prolonged restrictions of protein intake, as well as of phosphorus, potassium and sodium; all of these dietetic measures, though aimed at preventing metabolic complications, can set the stage to the development of malnutrition, especially when energy intake is inadequate (117, 118).
Metabolic acidosis is a very frequent complication of ESRD, and represents a powerful stimulus for protein catabolism (119). In fact, metabolic acidosis activates two systems responsible for intracellular protein degradation, the caspase-3 and the ubiquitin-proteasome systems, and may also reduce protein synthesis and promote insulin and GH resistance, thus leading to negative protein balance (120, 121).
Insulin resistance is one of the most important metabolic challenges in kidney transplant candidates. It has been demonstrated that diabetes is a major risk-factor for sarcopenia in hemodialyzed patients, who suffers from increased protein degradation and loss of lean body mass in comparison to non-diabetic patients (122, 123). However, insulin resistance can be observed also in non-diabetic patients on dialysis, and it is associated with increased protein catabolism also mediated by the ubiquitin-proteasome pathway (124). Insulin resistance is also responsible for a decrease in muscle phosphatidylinositol 3 kinase (PI3K), which may explain the overactivation of the ubiquitin-proteasome pathway. Beside of metabolic acidosis, vitamin D deficiency also contributes to the development of insulin resistance by affecting pancreatic insulin secretion (125, 126). Moreover, muscle is also a target organ for vitamin D, increasing calcium influx from cellular membranes and stimulating muscle synthesis when vitamin D binds to its muscular receptor. Low concentrations of vitamin D are associated with muscle atrophy and sarcopenia (127).
Proteinuria as a consequence of glomerular kidney disease could represent an important additional way of protein loss even in the earlier stages of CKD. Chronic inflammation is a frequent finding in ESRD patients; its consequences include an increase in nutritional needs and the development of anorexia through a lack of balance between orexigenic/anorexigenic mechanisms that control the energetic homeostasis of renal patients (128, 129). Many factors are thought to contribute to the pathogenesis of inflammation in ESRD: worsening of renal function by a reduction of the elimination of pro-inflammatory cytokines and uremic toxins, acute and chronic comorbidities, and factors related to the dialytic treatment itself, such as membrane and dialysis fluid bioincompatibility (130).
In fact, recent data show that the duration of hemodialysis before transplantation is highly correlated with the presence of sarcopenia in kidney transplant candidates (131).
Furthermore, evidence suggest a key role for the gastrointestinal tract as a consequence of intestinal dysbiosis and barrier disruption (132–134). The uremic milieu and reduced intake of fibers that are characteristic of CKD/ESRD patients are responsible for negative effects both on the resident microbial population (i.e., dysbiosis,) and in the structure and function of the gastrointestinal tract, enhancing its permeability (107, 132, 134). This dysbiotic environment of CKD/ESRD is characterized by a switch toward a more proteolytic metabolism profile, leading to an increase in protein fermentation (putrefaction), the generation of increased amounts of potentially toxic compounds (i.e., ammonium, thiols, phenols, indoles) that are absorbed into the bloodstream and accumulate in CKD/ESRD patients (107, 134). In addition, the deranged and more permeable intestinal barrier may facilitate bacterial translocation, which is the passage of bacteria or their structural component lipopolysaccharide (LPS) from the lumen to the blood (107, 134), with a final stimulation effect leading to chronic inflammation. Cytokines like TNFα, IL-6, IL-8, and IFN-γ are, in fact, mentioned among the most frequently observed indicators and activators of muscle proteolysis, while CRP seems to be a useful and inexpensive marker of systemic inflammation, despite being nonspecific (135).
Physical activity and function (defined as the capacity to perform activities of daily living), which are key components in the diagnosis of sarcopenia, are markedly reduced in transplant candidates in comparison to age-matched controls (136, 137). Reasons for reduced physical activity are fatigue on dialysis days, lack of time and motivation, physical problems and pain (138–140). In addition, some physical limitations related to the peritoneal dialysis and the presence of central venous catheter may play a role in limiting the enrollment in some sports. In this clinical setting, physical inactivity represents a modifiable risk factor for the development of sarcopenia, and it may cause further increase in the already high cardiovascular risk of these patients (137, 141). Physical exercise, even in dialyzed patients, is able to reduce depressive symptoms, to improve the quality of life, appetite and energy supply (141).
However, despite the aforementioned higher prevalence of sarcopenia among kidney transplant candidates and its correlation with negative post-transplant outcomes, its evaluation is frequently neglected at the time of waiting list.
Sarcopenia in Kidney Transplant Recipients
The presence of sarcopenia at the time of kidney transplant has been associated with increased mortality, graft failure, and postoperative complications such as infections (11, 12, 142, 143).
Successful renal transplantation is able to correct or improve many of the conditions related to CKD that promotes muscle wasting and sarcopenia, such as metabolic acidosis and chronic inflammation. However, the use of glucocorticoids as immunosuppressive therapy and the improved, but still suboptimal, renal function can continue to propitiate negative changes in body composition of renal transplant recipients. In general, an increase in body weight is frequently observed early following kidney transplantation (144, 145). However, it seems to be predominantly due to an increase in fat mass instead of muscle mass (146–148). In addition, low physical activity, a common finding in transplant recipients (149, 150), resulting from a combination of low exercise capacity and exercise intolerance/barriers that starts already in the pre-dialysis phases of CKD (140, 151), may significantly contribute to the reduction of muscle mass and function. Skeletal muscle dysfunction, particularly reduced muscle strength, is an important contributor to exercise intolerance in renal transplant candidates and recipients (151, 152). In summary, it seems that changes in body composition of transplant recipients are mainly characterized by an early gain in adipose tissue, while the restoration of muscle mass and function seems to be incomplete. The excess of fat mass and the presence of reduced muscle mass found in transplant recipients characterize a condition called sarcopenic obesity (153). Many studies have shown that when combined, sarcopenia and obesity (as assessed by excess fat mass or central obesity, but not BMI values only) may act in a complementary way increasing the risk of mortality, disability, cardiovascular disease, and metabolic impairment (154–158).
Sarcopenia and Frailty
Although the pathogenesis of frailty and sarcopenia is not fully understood, these two conditions seem to share risk factors, pathways and often contribute to the same negative outcomes. A growing interest in the frailty and sarcopenia definition and pathogenesis has been observed also in kidney transplant field.
Sarcopenia is one of the most important key components of frailty in kidney transplant candidates, and recognizes as pathogenetic factors aging, physical inactivity, malnutrition, acidosis, metabolic/neuroendocrine dysregulation and mild chronic inflammation (159). Once established, sarcopenia can produce a decrease in resting metabolic rate, contributing to the loss of appetite and malnutrition. The skeletal muscle and the diaphragm can become weaker, leading to increase exhaustion (160), physical inactivity and potential disability in a loop way process (32).
Available evidence regarding the prevalence of frailty components in frail community dwelling adults, revealed that reduced gait speed and weakness were the most common positive Fried criteria (43 and 54% respectively) (41). Increased relative risk for developing weakness and low activity was also reported, in comparison to the risk of developing any other frailty component during 7.5 years of follow up in initially non-frail women (161). This finding suggests that having frailty without sarcopenia is theoretically possible, however it is clinically unlikely.
In the general population sarcopenia has been shown to be twice as common as frailty (162). In kidney transplant candidates, which are selected among the healthier dialyzed patients, the prevalence of these two conditions almost overlaps at around 20% (17), however when the whole dialyzed population is analyzed, the prevalence rises up to 20–44% for sarcopenia and 42% for frailty (163, 164).
Both frailty and sarcopenia are considered reversible conditions. Epidemiological and interventional studies showed that multiprofessional treatments based on individual's frailty positive criteria are successful in improving patients' frailty status (165–167). In addition, other studies specifically have shown that it is possible to improve muscular components of frailty, and consequently sarcopenia (168, 169), confirming that frailty and sarcopenia are linked conditions that correlate to musculoskeletal aging.
Practical Considerations
In order to prevent or identify subjects at risk for sarcopenia, the EWGSOP2 recommends the use of the SARC-F questionnaire in healthy community living older subjects (89). The SARC-F is a self-reported 5-item questionnaire that reports patients' perceptions and experiences regarding strength, walking ability, chair standing, stair climbing and falls (170). Considering that the SARC-F lacks validation in the renal setting and that sarcopenia in renal patients is mostly secondary, with multiple factors influencing its development, a more comprehensive screening shall be recommended. Periodic appetite assessment tools and food diaries are critical, and allows early intervention when nutrient intake is already slightly reduced (<1 g/Kg/day of protein and <30 Kcal/Kg/day in hemodialysis patients, and <0.8 g/Kg/day in transplant recipients with adequate graft function) (115). In fact, in hemodialysis patients, an intake of protein of <0.8 g/Kg/die and/or calories <25 Kcal/Kg/die in associated with increased risk of malnutrition and sarcopenia (11, 128, 171). Calculation of the protein catabolic rate (PCR) is useful to estimate protein intake in stable patients (172). In transplant recipients not on hemodialysis, PCR can be calculated based on 24 h urinary urea nitrogen excretion, or by urea kinetics in patients on dialysis (172, 173). The recommended energy and protein intake for dialysis patients and transplant recipients are described in Table 3. In addition, considering that patients with ESKD frequently perform blood tests, the combined evaluation of serum albumin and CRP might be useful to assess inflammation. Most importantly, the treatment of conditions related to the renal disease itself that contribute to the increase of catabolism (i.e., proteinuria, metabolic acidosis, hyperparathyroidism, vitamin D deficiency, chronic inflammation) is a key component to prevent the development of sarcopenia in renal patients (115). On this premises, in Figure 2 an algorithm is proposed to identify the presence of sarcopenia among transplant candidates and recipients.
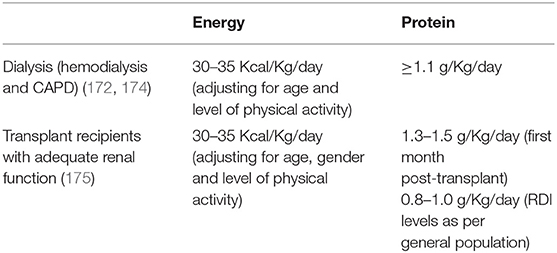
Table 3. Energy and protein intake recommendations for patients on dialysis and transplant recipients.
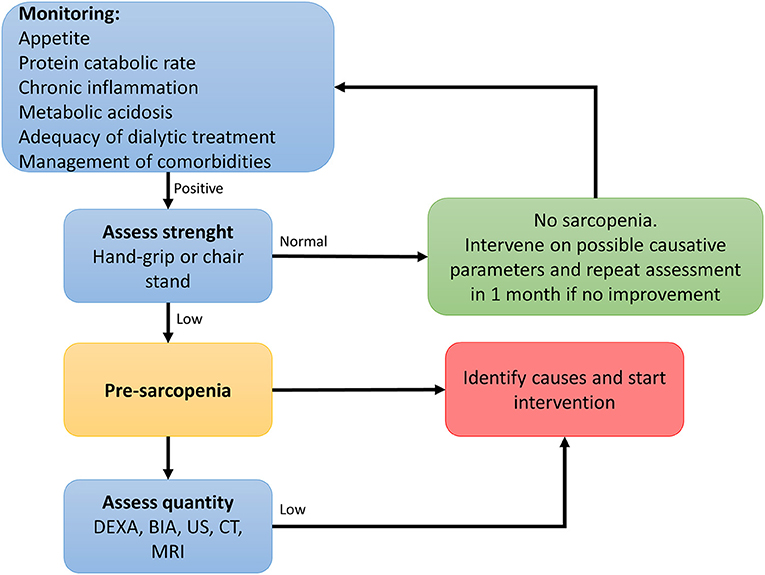
Figure 2. Algorithm to identify cases of sarcopenia in transplant candidates and recipients [adapted from (89)].
Conclusion
Kidney transplantation is the treatment of choice for most of the patients with ESRD. The suitability for transplantation is based on the evaluation of risks faced by the patient undergoing the procedure and the risks associated with staying on dialysis.
Frailty and sarcopenia are interrelated complex geriatric syndromes that are linked to disability, aging and comorbidities. CKD and ESRD are characterized by multiple factors that set the stage for the development of sarcopenia and frailty. Despite the available evidence showing that frailty and sarcopenia correlate to worse outcomes in transplant recipients, both conditions are frequently neglected in the evaluation of kidney transplant candidates. Prospective studies are needed to evaluate the utility of formally measuring frailty and sarcopenia as part of the transplant evaluation process.
Author Contributions
IG and AS wrote the first draft of the paper. All authors read and contributed to the final version of the paper.
Conflict of Interest
The authors declare that the research was conducted in the absence of any commercial or financial relationships that could be construed as a potential conflict of interest.
References
1. Tonelli M, Wiebe N, Knoll G, Bello A, Browne S, Jadhav D, et al. Systematic review: kidney transplantation compared with dialysis in clinically relevant outcomes. Am J Transplant. (2011) 11:2093–109. doi: 10.1111/j.1600-6143.2011.03686.x
2. Gentile S, Beauger D, Speyer E, Jouve E, Dussol B, Jacquelinet C, et al. Factors associated with health-related quality of life in renal transplant recipients: results of a national survey in France. Health Qual Life Outcomes. (2013) 11:88. doi: 10.1186/1477-7525-11-88
3. Port FK, Wolfe RA, Mauger EA, Berling DP, Jiang K. Comparison of survival probabilities for dialysis patients vs cadaveric renal transplant recipients. JAMA. (1993) 270:1339–43. doi: 10.1001/jama.270.11.1339
4. Wolfe RA, Ashby VB, Milford EL, Ojo AO, Ettenger RE, Agodoa LY, et al. Comparison of mortality in all patients on dialysis, patients on dialysis awaiting transplantation, and recipients of a first cadaveric transplant. N Engl J Med. (1999) 341:1725–30. doi: 10.1056/NEJM199912023412303
5. Rabbat CG, Thorpe KE, Russell JD, Churchill DN. Comparison of mortality risk for dialysis patients and cadaveric first renal transplant recipients in Ontario, Canada. J Am Soc Nephrol. (2000) 11:917–22.
6. Karopadi AN, Mason G, Rettore E, Ronco C. Cost of peritoneal dialysis and haemodialysis across the world. Nephrol Dial Transplant. (2013) 28:2553–69. doi: 10.1093/ndt/gft214
7. Saran R, Robinson B, Abbott KC, Agodoa LYC, Bragg-Gresham J, Balkrishnan R, et al. US renal data system 2018 annual data report: epidemiology of kidney disease in the United States. Am J Kidney Dis. (2019) 73:A7–8. doi: 10.1053/j.ajkd.2019.01.001
8. Rao PS, Merion RM, Ashby VB, Port FK, Wolfe RA, Kayler LK. Renal transplantation in elderly patients older than 70 years of age: results from the scientific registry of transplant recipients. Transplantation. (2007) 83:1069–74. doi: 10.1097/01.tp.0000259621.56861.31
9. Weber M, Faravardeh A, Jackson S, Berglund D, Spong R, Matas AJ, et al. Quality of life in elderly kidney transplant recipients. J Am Geriatr Soc. (2014) 62:1877–82. doi: 10.1111/jgs.13065
10. Humar A, Denny R, Matas AJ, Najarian JS. Graft and quality of life outcomes in older recipients of a kidney transplant. Exp Clin Transplant. (2003) 1:69–72.
11. Streja E, Molnar MZ, Kovesdy CP, Bunnapradist S, Jing J, Nissenson AR, et al. Associations of pretransplant weight and muscle mass with mortality in renal transplant recipients. Clin J Am Soc Nephrol. (2011) 6:1463–73. doi: 10.2215/CJN.09131010
12. Englesbe MJ, Patel SP, He K, Lynch RJ, Schaubel DE, Harbaugh C, et al. Sarcopenia and mortality after liver transplantation. J Am Coll Surg. (2010) 211:271–8. doi: 10.1016/j.jamcollsurg.2010.03.039
13. Garonzik-Wang JM, Govindan P, Grinnan JW, Liu M, Ali HM, Chakraborty A, et al. Frailty and delayed graft function in kidney transplant recipients. Arch Surg. (2012) 147:190–3. doi: 10.1001/archsurg.2011.1229
14. McAdams-DeMarco MA, Law A, Tan J, Delp C, King EA, Orandi B, et al. Frailty, mycophenolate reduction, and graft loss in kidney transplant recipients. Transplantation. (2015) 99:805–10. doi: 10.1097/TP.0000000000000444
15. McAdams-DeMarco MA, Law A, Salter ML, Chow E, Grams M, Walston J, et al. Frailty and early hospital readmission after kidney transplantation. Am J Transplant. (2013) 13:2091–5. doi: 10.1111/ajt.12300
16. McAdams-DeMarco MA, Law A, Salter ML, Boyarsky B, Gimenez L, Jaar BG, et al. Frailty as a novel predictor of mortality and hospitalization in individuals of all ages undergoing hemodialysis. J Am Geriatr Soc. (2013) 61:896–901. doi: 10.1111/jgs.12266
17. McAdams-DeMarco MA, Law A, King E, Orandi B, Salter M, Gupta N, et al. Frailty and mortality in kidney transplant recipients. Am J Transplant. (2015) 15:149–54. doi: 10.1111/ajt.12992
18. McAdams-DeMarco MA, Tan J, Salter ML, Gross A, Meoni LA, Jaar BG, et al. Frailty and cognitive function in incident hemodialysis patients. Clin J Am Soc Nephrol. (2015) 10:2181–9. doi: 10.2215/CJN.01960215
19. Rockwood K, Stadnyk K, MacKnight C, McDowell I, Hebert R, Hogan DB. A brief clinical instrument to classify frailty in elderly people. Lancet. (1999) 353:205–6. doi: 10.1016/S0140-6736(98)04402-X
20. Campbell AJ, Buchner DM. Unstable disability and the fluctuations of frailty. Age Ageing. (1997) 26:315–8. doi: 10.1093/ageing/26.4.315
21. Buchner DM, Wagner EH. Preventing frail health. Clin Geriatr Med. (1992) 8:1–17. doi: 10.1016/S0749-0690(18)30494-4
22. Bortz WM 2nd. The physics of frailty. J Am Geriatr Soc. (1993) 41:1004–8. doi: 10.1111/j.1532-5415.1993.tb06769.x
23. Lipsitz LA, Goldberger AL. Loss of ‘complexity' and aging. Potential applications of fractals and chaos theory to senescence. JAMA. (1992) 267:1806–9. doi: 10.1001/jama.267.13.1806
24. Hamerman D. Toward an understanding of frailty. Ann Intern Med. (1999) 130:945–50. doi: 10.7326/0003-4819-130-11-199906010-00022
25. Chen X, Mao G, Leng SX. Frailty syndrome: an overview. Clin Interv Aging. (2014) 9:433–41. doi: 10.2147/CIA.S45300
26. Fried LP, Hadley EC, Walston JD, Newman AB, Guralnik JM, Studenski S, et al. From bedside to bench: research agenda for frailty. Sci Aging Knowledge Environ. (2005) 2005:pe24. doi: 10.1126/sageke.2005.31.pe24
27. Santos-Eggimann B, Cuenoud P, Spagnoli J, Junod J. Prevalence of frailty in middle-aged and older community-dwelling Europeans living in 10 countries. J Gerontol A Biol Sci Med Sci. (2009) 64:675–81. doi: 10.1093/gerona/glp012
28. Alvarado BE, Zunzunegui MV, Beland F, Bamvita JM. Life course social and health conditions linked to frailty in Latin American older men and women. J Gerontol A Biol Sci Med Sci. (2008) 63:1399–406. doi: 10.1093/gerona/63.12.1399
29. Searle SD, Mitnitski A, Gahbauer EA, Gill TM, Rockwood K. A standard procedure for creating a frailty index. BMC Geriatr. (2008) 8:24. doi: 10.1186/1471-2318-8-24
30. Rockwood K, Mitnitski A. Frailty defined by deficit accumulation and geriatric medicine defined by frailty. Clin Geriatr Med. (2011) 27:17–26. doi: 10.1016/j.cger.2010.08.008
31. Rockwood K, Mitnitski A. Frailty in relation to the accumulation of deficits. J Gerontol A Biol Sci Med Sci. (2007) 62:722–7. doi: 10.1093/gerona/62.7.722
32. Fried LP, Xue QL, Cappola AR, Ferrucci L, Chaves P, Varadhan R, et al. Nonlinear multisystem physiological dysregulation associated with frailty in older women: implications for etiology and treatment. J Gerontol A Biol Sci Med Sci. (2009) 64:1049–57. doi: 10.1093/gerona/glp076
33. Leng S, Chaves P, Koenig K, Walston J. Serum interleukin-6 and hemoglobin as physiological correlates in the geriatric syndrome of frailty: a pilot study. J Am Geriatr Soc. (2002) 50:1268–71. doi: 10.1046/j.1532-5415.2002.50315.x
34. Walston J, Hadley EC, Ferrucci L, Guralnik JM, Newman AB, Studenski SA, et al. Research agenda for frailty in older adults: toward a better understanding of physiology and etiology: summary from the American Geriatrics Society/National Institute on Aging Research Conference on Frailty in Older Adults. J Am Geriatr Soc. (2006) 54:991–1001. doi: 10.1111/j.1532-5415.2006.00745.x
35. Clegg A, Young J, Iliffe S, Rikkert MO, Rockwood K. Frailty in elderly people. Lancet. (2013) 381:752–62. doi: 10.1016/S0140-6736(12)62167-9
36. Lipsitz LA. Dynamics of stability: the physiologic basis of functional health and frailty. J Gerontol A Biol Sci Med Sci. (2002) 57:B115–25. doi: 10.1093/gerona/57.3.B115
37. Pedone C, Costanzo L, Cesari M, Bandinelli S, Ferrucci L, Antonelli Incalzi R. Are performance measures necessary to predict loss of independence in elderly people? J Gerontol A Biol Sci Med Sci. (2016) 71:84–9. doi: 10.1093/gerona/glv096
38. Romero-Ortuno R, Walsh CD, Lawlor BA, Kenny RA. A frailty instrument for primary care: findings from the Survey of Health, Ageing and Retirement in Europe (SHARE). BMC Geriatr. (2010) 10:57. doi: 10.1186/1471-2318-10-57
39. Fried LP, Ferrucci L, Darer J, Williamson JD, Anderson G. Untangling the concepts of disability, frailty, and comorbidity: implications for improved targeting and care. J Gerontol A Biol Sci Med Sci. (2004) 59:255–63. doi: 10.1093/gerona/59.3.M255
40. Bandeen-Roche K, Xue QL, Ferrucci L, Walston J, Guralnik JM, Chaves P, et al. Phenotype of frailty: characterization in the women's health and aging studies. J Gerontol A Biol Sci Med Sci. (2006) 61:262–6. doi: 10.1093/gerona/61.3.262
41. Rothman MD, Leo-Summers L, Gill TM. Prognostic significance of potential frailty criteria. J Am Geriatr Soc. (2008) 56:2211–16. doi: 10.1111/j.1532-5415.2008.02008.x
42. Murray A, McNeil C, Salarirad S, Deary I, Phillips L, Whalley L, et al. Brain hyperintensity location determines outcome in the triad of impaired cognition, physical health and depressive symptoms: a cohort study in late life. Arch Gerontol Geriatr. (2016) 63:49–54. doi: 10.1016/j.archger.2015.10.004
43. Aguilar-Navarro SG, Mimenza-Alvarado AJ, Anaya-Escamilla A, Gutierrez-Robledo LM. Frailty and vascular cognitive impairment: mechanisms behind the link. Rev Invest Clin. (2016) 68:25–32.
44. Buigues C, Padilla-Sanchez C, Garrido JF, Navarro-Martinez R, Ruiz-Ros V, Cauli O. The relationship between depression and frailty syndrome: a systematic review. Aging Ment Health. (2015) 19:762–72. doi: 10.1080/13607863.2014.967174
45. Fried LP, Tangen CM, Walston J, Newman AB, Hirsch C, Gottdiener J, et al. Frailty in older adults: evidence for a phenotype. J Gerontol A Biol Sci Med Sci. (2001) 56:M146–56. doi: 10.1093/gerona/56.3.M146
46. Speechley M, Tinetti M. Falls and injuries in frail and vigorous community elderly persons. J Am Geriatr Soc. (1991) 39:46–52. doi: 10.1111/j.1532-5415.1991.tb05905.x
47. Winograd CH. Targeting strategies: an overview of criteria and outcomes. J Am Geriatr Soc. (1991) 39(9 Pt 2):25s–35. doi: 10.1111/j.1532-5415.1991.tb05930.x
48. Rubenstein LZ, Josephson KR, Wieland GD, English PA, Sayre JA, Kane RL. Effectiveness of a geriatric evaluation unit. A randomized clinical trial. N Engl J Med. (1984) 311:1664–70. doi: 10.1056/NEJM198412273112604
49. Gill TM, Baker DI, Gottschalk M, Peduzzi PN, Allore H, Byers A. A program to prevent functional decline in physically frail, elderly persons who live at home. N Engl J Med. (2002) 347:1068–74. doi: 10.1056/NEJMoa020423
50. Saliba D, Elliott M, Rubenstein LZ, Solomon DH, Young RT, Kamberg CJ, et al. The Vulnerable elders survey: a tool for identifying vulnerable older people in the community. J Am Geriatr Soc. (2001) 49:1691–9. doi: 10.1046/j.1532-5415.2001.49281.x
51. Fried LP, Borhani NO, Enright P, Furberg CD, Gardin JM, Kronmal RA, et al. The Cardiovascular Health Study: design and rationale. Ann Epidemiol. (1991) 1:263–76. doi: 10.1016/1047-2797(91)90005-W
52. Makary MA, Segev DL, Pronovost PJ, Syin D, Bandeen-Roche K, Patel P, et al. Frailty as a predictor of surgical outcomes in older patients. J Am Coll Surg. (2010) 210:901–8. doi: 10.1016/j.jamcollsurg.2010.01.028
53. Mitnitski AB, Mogilner AJ, Rockwood K. Accumulation of deficits as a proxy measure of aging. ScientificWorldJournal. (2001) 1:323–36. doi: 10.1100/tsw.2001.58
54. Mitnitski AB, Graham JE, Mogilner AJ, Rockwood K. Frailty, fitness and late-life mortality in relation to chronological and biological age. BMC Geriatr. (2002) 2:1. doi: 10.1186/1471-2318-2-1
55. Rockwood K, Song X, MacKnight C, Bergman H, Hogan DB, McDowell I, et al. A global clinical measure of fitness and frailty in elderly people. Cmaj. (2005) 173:489–95. doi: 10.1037/t19726-000
56. Abellan van Kan G, Rolland Y, Bergman H, Morley JE, Kritchevsky SB, Vellas B. The I.A.N.A Task Force on frailty assessment of older people in clinical practice. J Nutr Health Aging. (2008) 12:29–37. doi: 10.1007/BF02982161
57. Hyde Z, Flicker L, Almeida OP, Hankey GJ, McCaul KA, Chubb SA, et al. Low free testosterone predicts frailty in older men: the health in men study. J Clin Endocrinol Metab. (2010) 95:3165–72. doi: 10.1210/jc.2009-2754
58. Kennedy CC, Ioannidis G, Rockwood K, Thabane L, Adachi JD, Kirkland S, et al. A Frailty Index predicts 10-year fracture risk in adults age 25 years and older: results from the Canadian Multicentre Osteoporosis Study (CaMos). Osteoporos Int. (2014) 25:2825–32. doi: 10.1007/s00198-014-2828-9
59. Choi JY, Yoon SJ, Kim SW, Jung HW, Kim KI, Kang E, et al. Prediction of postoperative complications using multidimensional frailty score in older female cancer patients with american society of anesthesiologists physical status class 1 or 2. J Am Coll Surg. (2015) 221:652–60.e2. doi: 10.1016/j.jamcollsurg.2015.06.011
60. Goldstein J, Hubbard RE, Moorhouse P, Andrew MK, Mitnitski A, Rockwood K. The validation of a care partner-derived frailty index based upon comprehensive geriatric assessment (CP-FI-CGA) in emergency medical services and geriatric ambulatory care. Age Ageing. (2015) 44:327–30. doi: 10.1093/ageing/afu161
61. Khezrian M, Myint PK, McNeil C, Murray AD. A review of frailty syndrome and its physical, cognitive and emotional domains in the elderly. Geriatrics. (2017) 2:36. doi: 10.3390/geriatrics2040036
62. Levey AS, Stevens LA, Schmid CH, Zhang YL, Castro AF III, Feldman HI, et al. A new equation to estimate glomerular filtration rate. Ann Intern Med. (2009) 150:604–12. doi: 10.7326/0003-4819-150-9-200905050-00006
63. Stevens KK, Woo YM, Clancy M, McClure JD, Fox JG, Geddes CC. Deceased donor transplantation in the elderly–are we creating false hope? Nephrol Dial Transplant. (2011) 26:2382–6. doi: 10.1093/ndt/gfq826
64. Ponticelli C, Podesta MA, Graziani G. Renal transplantation in elderly patients. How to select the candidates to the waiting list? Transplant Rev. (2014) 28:188–92. doi: 10.1016/j.trre.2014.07.001
65. Lloveras J, Arcos E, Comas J, Crespo M, Pascual J. A paired survival analysis comparing hemodialysis and kidney transplantation from deceased elderly donors older than 65 years. Transplantation. (2015) 99:991–6. doi: 10.1097/TP.0000000000000474
66. Macrae J, Friedman AL, Friedman EA, Eggers P. Live and deceased donor kidney transplantation in patients aged 75 years and older in the United States. Int Urol Nephrol. (2005) 37:641–8. doi: 10.1007/s11255-004-0010-6
67. Massie AB, Luo X, Chow EK, Alejo JL, Desai NM, Segev DL. Survival benefit of primary deceased donor transplantation with high-KDPI kidneys. Am J Transplant. (2014) 14:2310–6. doi: 10.1111/ajt.12830
68. Merion RM, Ashby VB, Wolfe RA, Distant DA, Hulbert-Shearon TE, Metzger RA, et al. Deceased-donor characteristics and the survival benefit of kidney transplantation. JAMA. (2005) 294:2726–33. doi: 10.1001/jama.294.21.2726
69. Ojo AO, Hanson JA, Meier-Kriesche H, Okechukwu CN, Wolfe RA, Leichtman AB, et al. Survival in recipients of marginal cadaveric donor kidneys compared with other recipients and wait-listed transplant candidates. J Am Soc Nephrol. (2001) 12:589–97.
70. Perez-Saez MJ, Arcos E, Comas J, Crespo M, Lloveras J, Pascual J. Survival benefit from kidney transplantation using kidneys from deceased donors aged ≥75 years: a time-dependent analysis. Am J Transplant. (2016) 16:2724–33. doi: 10.1111/ajt.13800
71. Gill JS, Schaeffner E, Chadban S, Dong J, Rose C, Johnston O, et al. Quantification of the early risk of death in elderly kidney transplant recipients. Am J Transplant. (2013) 13:427–32. doi: 10.1111/j.1600-6143.2012.04323.x
72. Goto NA, van Loon IN, Boereboom FTJ, Emmelot-Vonk MH, Willems HC, Bots ML, et al. Association of initiation of maintenance dialysis with functional status and caregiver burden. Clin J Am Soc Nephrol. (2019) 14:1039–47. doi: 10.2215/CJN.13131118
73. de Boer IH, Zelnick L, Afkarian M, Ayers E, Curtin L, Himmelfarb J, et al. Impaired glucose and insulin homeostasis in moderate-severe CKD. J Am Soc Nephrol. (2016) 27:2861–71. doi: 10.1681/ASN.2015070756
74. Khurana KK, Navaneethan SD, Arrigain S, Schold JD, Nally JV Jr, Shoskes DA. Serum testosterone levels and mortality in men with CKD stages 3–4. Am J Kidney Dis. (2014) 64:367–74. doi: 10.1053/j.ajkd.2014.03.010
75. Piotti G, Gandolfini I, Palmisano A, Maggiore U. Metabolic risk profile in kidney transplant candidates and recipients. Nephrol Dial Transplant. (2019) 34:388–400. doi: 10.1093/ndt/gfy151
76. Kurella Tamura M, Wadley V, Yaffe K, McClure LA, Howard G, Go R, et al. Kidney function and cognitive impairment in US adults: the Reasons for Geographic and Racial Differences in Stroke (REGARDS) Study. Am J Kidney Dis. (2008) 52:227–34. doi: 10.1053/j.ajkd.2008.05.004
77. Feng L, Yap KB, Yeoh LY, Ng TP. Kidney function and cognitive and functional decline in elderly adults: findings from the Singapore longitudinal aging study. J Am Geriatr Soc. (2012) 60:1208–14. doi: 10.1111/j.1532-5415.2012.04043.x
78. Kurella Tamura M, Muntner P, Wadley V, Cushman M, Zakai NA, Bradbury BD, et al. Albuminuria, kidney function, and the incidence of cognitive impairment among adults in the United States. Am J Kidney Dis. (2011) 58:756–63. doi: 10.1053/j.ajkd.2011.05.027
79. Buchman AS, Tanne D, Boyle PA, Shah RC, Leurgans SE, Bennett DA. Kidney function is associated with the rate of cognitive decline in the elderly. Neurology. (2009) 73:920–7. doi: 10.1212/WNL.0b013e3181b72629
80. Cheng XS, Lentine KL, Koraishy FM, Myers J, Tan JC. Implications of frailty for peritransplant outcomes in kidney transplant recipients. Curr Transplant Rep. (2019) 6:16–25. doi: 10.1007/s40472-019-0227-z
81. Lorenz EC, Cosio FG, Bernard SL, Bogard SD, Bjerke BR, Geissler EN, et al. The relationship between frailty and decreased physical performance with death on the kidney transplant waiting list. Prog Transplant. (2019) 29:108–14. doi: 10.1177/1526924819835803
82. Haugen CE, Chu NM, Ying H, Warsame F, Holscher CM, Desai NM, et al. Frailty and access to kidney transplantation. Clin J Am Soc Nephrol. (2019) 14:576–82. doi: 10.2215/CJN.12921118
83. Chu NM, Deng A, Ying H, Haugen CE, Garonzik Wang JM, Segev DL, et al. Dynamic frailty before kidney transplantation-time of measurement matters. Transplantation. (2019) 103:1700–4. doi: 10.1097/TP.0000000000002563
84. Chu NM, Gross AL, Shaffer AA, Haugen CE, Norman SP, Xue QL, et al. Frailty and changes in cognitive function after kidney transplantation. J Am Soc Nephrol. (2019) 30:336–45. doi: 10.1681/ASN.2018070726
85. McAdams-DeMarco MA, Isaacs K, Darko L, Salter ML, Gupta N, King EA, et al. Changes in frailty after kidney transplantation. J Am Geriatr Soc. (2015) 63:2152–7. doi: 10.1111/jgs.13657
86. McAdams-DeMarco MA, Olorundare IO, Ying H, Warsame F, Haugen CE, Hall R, et al. Frailty and postkidney transplant health-related quality of life. Transplantation. (2018) 102:291–9. doi: 10.1097/TP.0000000000001943
87. KDIGO. KDIGO Clinical Practice Guideline on the Evaluation and Management of Candidates for Kidney Transplantation. KDIGO (2018).
88. Exterkate L, Slegtenhorst BR, Kelm M, Seyda M, Schuitenmaker JM, Quante M, et al. Frailty and Transplantation. Transplantation. (2016) 100:727–33. doi: 10.1097/TP.0000000000001003
89. Cruz-Jentoft AJ, Bahat G, Bauer J, Boirie Y, Bruyere O, Cederholm T, et al. Sarcopenia: revised European consensus on definition and diagnosis. Age Ageing. (2019) 48:16–31. doi: 10.1093/ageing/afy169
90. Fielding RA, Vellas B, Evans WJ, Bhasin S, Morley JE, Newman AB, et al. Sarcopenia: an undiagnosed condition in older adults. Current consensus definition: prevalence, etiology, and consequences. International working group on sarcopenia. J Am Med Dir Assoc. (2011) 12:249–56. doi: 10.1016/j.jamda.2011.01.003
91. Dhillon RJ, Hasni S. Pathogenesis and Management of Sarcopenia. Clin Geriatr Med. (2017) 33:17–26. doi: 10.1016/j.cger.2016.08.002
92. Cruz-Jentoft AJ, Baeyens JP, Bauer JM, Boirie Y, Cederholm T, Landi F, et al. Sarcopenia: European consensus on definition and diagnosis: report of the European Working Group on sarcopenia in older people. Age Ageing. (2010) 39:412–23. doi: 10.1093/ageing/afq034
93. Beaudart C, Rizzoli R, Bruyere O, Reginster JY, Biver E. Sarcopenia: burden and challenges for public health. Arch Public Health. (2014) 72:45. doi: 10.1186/2049-3258-72-45
94. Rizzoli R, Reginster JY, Arnal JF, Bautmans I, Beaudart C, Bischoff-Ferrari H, et al. Quality of life in sarcopenia and frailty. Calcif Tissue Int. (2013) 93:101–20. doi: 10.1007/s00223-013-9758-y
95. Lauretani F, Russo CR, Bandinelli S, Bartali B, Cavazzini C, Di Iorio A, et al. Age-associated changes in skeletal muscles and their effect on mobility: an operational diagnosis of sarcopenia. J Appl Physiol. (1985). (2003) 95:1851–60. doi: 10.1152/japplphysiol.00246.2003
96. Visser M, Schaap LA. Consequences of sarcopenia. Clin Geriatr Med. (2011) 27:387–99. doi: 10.1016/j.cger.2011.03.006
97. Lang T, Streeper T, Cawthon P, Baldwin K, Taaffe DR, Harris TB. Sarcopenia: etiology, clinical consequences, intervention, and assessment. Osteoporos Int. (2010) 21:543–59. doi: 10.1007/s00198-009-1059-y
98. Landi F, Liperoti R, Russo A, Giovannini S, Tosato M, Capoluongo E, et al. Sarcopenia as a risk factor for falls in elderly individuals: results from the ilSIRENTE study. Clin Nutr. (2012) 31:652–8. doi: 10.1016/j.clnu.2012.02.007
99. Wilson D, Jackson T, Sapey E, Lord JM. Frailty and sarcopenia: the potential role of an aged immune system. Ageing Res Rev. (2017) 36:1–10. doi: 10.1016/j.arr.2017.01.006
100. Abate M, Di Iorio A, Di Renzo D, Paganelli R, Saggini R, Abate G. Frailty in the elderly: the physical dimension. Eura Medicophys. (2007) 43:407–15.
101. Kyle UG, Genton L, Hans D, Karsegard L, Slosman DO, Pichard C. Age-related differences in fat-free mass, skeletal muscle, body cell mass and fat mass between 18 and 94 years. Eur J Clin Nutr. (2001) 55:663–72. doi: 10.1038/sj.ejcn.1601198
102. Melton LJ 3rd, Riggs BL, Achenbach SJ, Amin S, Camp JJ, Rouleau PA, et al. Does reduced skeletal loading account for age-related bone loss? J Bone Miner Res. (2006) 21:1847–55. doi: 10.1359/jbmr.060908
103. Frontera WR, Hughes VA, Fielding RA, Fiatarone MA, Evans WJ, Roubenoff R. Aging of skeletal muscle: a 12-yr longitudinal study. J Appl Physiol. (2000) 88:1321–6. doi: 10.1152/jappl.2000.88.4.1321
104. Ryall JG, Schertzer JD, Lynch GS. Cellular and molecular mechanisms underlying age-related skeletal muscle wasting and weakness. Biogerontology. (2008) 9:213–28. doi: 10.1007/s10522-008-9131-0
105. Bruunsgaard H, Ladelund S, Pedersen AN, Schroll M, Jorgensen T, Pedersen BK. Predicting death from tumour necrosis factor-alpha and interleukin-6 in 80-year-old people. Clin Exp Immunol. (2003) 132:24–31. doi: 10.1046/j.1365-2249.2003.02137.x
106. Marcell TJ. Sarcopenia: causes, consequences, and preventions. J Gerontol A Biol Sci Med Sci. (2003) 58:M911–6. doi: 10.1093/gerona/58.10.M911
107. Cosola C, Rocchetti MT, Sabatino A, Fiaccadori E, Di Iorio BR, Gesualdo L. Microbiota issue in CKD: how promising are gut-targeted approaches? J Nephrol. (2019) 32:27–37. doi: 10.1007/s40620-018-0516-0
108. Domanski M, Ciechanowski K. Sarcopenia: a major challenge in elderly patients with end-stage renal disease. J Aging Res. (2012) 2012:754739. doi: 10.1155/2012/754739
109. Ozkayar N, Altun B, Halil M, Kuyumcu ME, Arik G, Yesil Y, et al. Evaluation of sarcopenia in renal transplant recipients. Nephrourol Mon. (2014) 6:e20055. doi: 10.5812/numonthly.20055
110. Kim HY. Nutritional intervention for a patient with diabetic nephropathy. Clin Nutr Res. (2014) 3:64–8. doi: 10.7762/cnr.2014.3.1.64
112. Foley RN, Wang C, Ishani A, Collins AJ, Murray AM. Kidney function and sarcopenia in the United States general population: NHANES III. Am J Nephrol. (2007) 27:279–86. doi: 10.1159/000101827
113. Raj DS, Sun Y, Tzamaloukas AH. Hypercatabolism in dialysis patients. Curr Opin Nephrol Hypertens. (2008) 17:589–94. doi: 10.1097/MNH.0b013e32830d5bfa
114. Honda H, Qureshi AR, Axelsson J, Heimburger O, Suliman ME, Barany P, et al. Obese sarcopenia in patients with end-stage renal disease is associated with inflammation and increased mortality. Am J Clin Nutr. (2007) 86:633–8. doi: 10.1093/ajcn/86.3.633
115. Sabatino A, Regolisti G, Karupaiah T, Sahathevan S, Sadu Singh BK, Khor BH, et al. Protein-energy wasting and nutritional supplementation in patients with end-stage renal disease on hemodialysis. Clin Nutr. (2017) 36:663–71. doi: 10.1016/j.clnu.2016.06.007
116. Bossola M, Tazza L, Giungi S, Luciani G. Anorexia in hemodialysis patients: an update. Kidney Int. (2006) 70:417–22. doi: 10.1038/sj.ki.5001572
117. Hahn D, Hodson EM, Fouque D. Low protein diets for non-diabetic adults with chronic kidney disease. Cochrane Database Syst Rev. (2018) 10:Cd001892. doi: 10.1002/14651858.CD001892.pub4
118. Kovesdy CP, Kopple JD, Kalantar-Zadeh K. Management of protein-energy wasting in non-dialysis-dependent chronic kidney disease: reconciling low protein intake with nutritional therapy. Am J Clin Nutr. (2013) 97:1163–77. doi: 10.3945/ajcn.112.036418
119. Abramowitz MK, Hostetter TH, Melamed ML. Association of serum bicarbonate levels with gait speed and quadriceps strength in older adults. Am J Kidney Dis. (2011) 58:29–38. doi: 10.1053/j.ajkd.2010.12.021
120. Kraut JA, Madias NE. Consequences and therapy of the metabolic acidosis of chronic kidney disease. Pediatr Nephrol. (2011) 26:19–28. doi: 10.1007/s00467-010-1564-4
121. Siew ED, Ikizler TA. Insulin resistance and protein energy metabolism in patients with advanced chronic kidney disease. Semin Dial. (2010) 23:378–82. doi: 10.1111/j.1525-139X.2010.00763.x
122. Pupim LB, Heimburger O, Qureshi AR, Ikizler TA, Stenvinkel P. Accelerated lean body mass loss in incident chronic dialysis patients with diabetes mellitus. Kidney Int. (2005) 68:2368–74. doi: 10.1111/j.1523-1755.2005.00699.x
123. Pupim LB, Flakoll PJ, Majchrzak KM, Aftab Guy DL, Stenvinkel P, Ikizler TA. Increased muscle protein breakdown in chronic hemodialysis patients with type 2 diabetes mellitus. Kidney Int. (2005) 68:1857–65. doi: 10.1111/j.1523-1755.2005.00605.x
124. Siew ED, Pupim LB, Majchrzak KM, Shintani A, Flakoll PJ, Ikizler TA. Insulin resistance is associated with skeletal muscle protein breakdown in non-diabetic chronic hemodialysis patients. Kidney Int. (2007) 71:146–52. doi: 10.1038/sj.ki.5001984
125. Remuzzi A. Vitamin D, insulin resistance, and renal disease. Kidney Int. (2007) 71:96–8. doi: 10.1038/sj.ki.5002047
126. Norman AW, Frankel JB, Heldt AM, Grodsky GM. Vitamin D deficiency inhibits pancreatic secretion of insulin. Science. (1980) 209:823–5. doi: 10.1126/science.6250216
127. Bischoff Ferrari HA. Validated treatments and therapeutic perspectives regarding nutritherapy. J Nutr Health Aging. (2009) 13:737–41. doi: 10.1007/s12603-009-0207-6
128. Obi Y, Qader H, Kovesdy CP, Kalantar-Zadeh K. Latest consensus and update on protein-energy wasting in chronic kidney disease. Curr Opin Clin Nutr Metab Care. (2015) 18:254–62. doi: 10.1097/MCO.0000000000000171
129. Wang XH, Mitch WE. Mechanisms of muscle wasting in chronic kidney disease. Nat Rev Nephrol. (2014) 10:504–16. doi: 10.1038/nrneph.2014.112
130. Pupim LB, Ikizler TA. Uremic malnutrition: new insights into an old problem. Semin Dial. (2003) 16:224–32. doi: 10.1046/j.1525-139X.2003.16046.x
131. Takamoto D, Kawahara T, Mochizuki T, Makiyama K, Teranishi J, Uemura H. A longer history of hemodialysis can lead to sarcopenia in renal transplantation patients. Transplant Proc. (2018) 50:2447–50. doi: 10.1016/j.transproceed.2018.02.192
132. Ramezani A, Raj DS. The gut microbiome, kidney disease, and targeted interventions. J Am Soc Nephrol. (2014) 25:657–70. doi: 10.1681/ASN.2013080905
133. Mafra D, Fouque D. Gut microbiota and inflammation in chronic kidney disease patients. Clin Kidney J. (2015) 8:332–4. doi: 10.1093/ckj/sfv026
134. Sabatino A, Regolisti G, Brusasco I, Cabassi A, Morabito S, Fiaccadori E. Alterations of intestinal barrier and microbiota in chronic kidney disease. Nephrol Dial Transplant. (2015) 30:924–33. doi: 10.1093/ndt/gfu287
135. Carrero JJ, Chmielewski M, Axelsson J, Snaedal S, Heimburger O, Barany P, et al. Muscle atrophy, inflammation and clinical outcome in incident and prevalent dialysis patients. Clin Nutr. (2008) 27:557–64. doi: 10.1016/j.clnu.2008.04.007
136. Heiwe S, Jacobson SH. Exercise training for adults with chronic kidney disease. Cochrane Database Syst Rev. (2011) 2011:Cd003236. doi: 10.1002/14651858.CD003236.pub2
137. Johansen KL, Chertow GM, Kutner NG, Dalrymple LS, Grimes BA, Kaysen GA. Low level of self-reported physical activity in ambulatory patients new to dialysis. Kidney Int. (2010) 78:1164–70. doi: 10.1038/ki.2010.312
138. Regolisti G, Maggiore U, Sabatino A, Gandolfini I, Pioli S, Torino C, et al. Interaction of healthcare staff's attitude with barriers to physical activity in hemodialysis patients: a quantitative assessment. PLoS ONE. (2018) 13:e0196313. doi: 10.1371/journal.pone.0196313
139. Fiaccadori E, Sabatino A, Schito F, Angella F, Malagoli M, Tucci M, et al. Barriers to physical activity in chronic hemodialysis patients: a single-center pilot study in an Italian dialysis facility. Kidney Blood Press Res. (2014) 39:169–75. doi: 10.1159/000355793
140. Sabatino A, Regolisti G, Delsante M, Di Motta T, Cantarelli C, Pioli S, et al. Noninvasive evaluation of muscle mass by ultrasonography of quadriceps femoris muscle in End-Stage Renal Disease patients on hemodialysis. Clin Nutr. (2019) 38:1232–9. doi: 10.1016/j.clnu.2018.05.004.
141. Aucella F, Battaglia Y, Bellizzi V, Bolignano D, Capitanini A, Cupisti A. Physical exercise programs in CKD: lights, shades and perspectives [corrected]. J Nephrol. (2015) 28:143–50. doi: 10.1007/s40620-014-0169-6
142. Oterdoom LH, van Ree RM, de Vries AP, Gansevoort RT, Schouten JP, van Son WJ, et al. Urinary creatinine excretion reflecting muscle mass is a predictor of mortality and graft loss in renal transplant recipients. Transplantation. (2008) 86:391–8. doi: 10.1097/TP.0b013e3181788aea
143. Barone M, Viggiani MT, Avolio AW, Iannone A, Rendina M, Di Leo A. Obesity as predictor of postoperative outcomes in liver transplant candidates: review of the literature and future perspectives. Dig Liver Dis. (2017) 49:957–66. doi: 10.1016/j.dld.2017.07.004
144. Verran D, Munn S, Collins J, Hill G. Impact of renal allograft implantation and immunosuppression on body composition using in vivo neutron activation analysis. Transplant Proc. (1992) 24:173–4.
145. Gonyea JE, Anderson CF. Weight change and serum lipoproteins in recipients of renal allografts. Mayo Clin Proc. (1992) 67:653–7. doi: 10.1016/S0025-6196(12)60720-4
146. van den Ham EC, Kooman JP, Christiaans MH, Leunissen KM, van Hooff JP. Posttransplantation weight gain is predominantly due to an increase in body fat mass. Transplantation. (2000) 70:241–2.
147. Steiger U, Lippuner K, Jensen EX, Montandon A, Jaeger P, Horber FF. Body composition and fuel metabolism after kidney grafting. Eur J Clin Invest. (1995) 25:809–16. doi: 10.1111/j.1365-2362.1995.tb01689.x
148. Isiklar I, Akin O, Demirag A, Niron EA. Effects of renal transplantation on body composition. Transplant Proc. (1998) 30:831–2. doi: 10.1016/S0041-1345(98)00067-0
149. van den Ham EC, Kooman JP, Christiaans MH, van Hooff JP. Relation between steroid dose, body composition and physical activity in renal transplant patients. Transplantation. (2000) 69:1591–8. doi: 10.1097/00007890-200004270-00013
150. Yanishi M, Tsukaguchi H, Kimura Y, Koito Y, Yoshida K, Seo M, et al. Evaluation of physical activity in sarcopenic conditions of kidney transplantation recipients. Int Urol Nephrol. (2017) 49:1779–84. doi: 10.1007/s11255-017-1661-4
151. van den Ham EC, Kooman JP, Schols AM, Nieman FH, Does JD, Franssen FM, et al. Similarities in skeletal muscle strength and exercise capacity between renal transplant and hemodialysis patients. Am J Transplant. (2005) 5:1957–65. doi: 10.1111/j.1600-6143.2005.00944.x
152. van den Ham EC, Kooman JP, Schols AM, Nieman FH, Does JD, Akkermans MA, et al. The functional, metabolic, and anabolic responses to exercise training in renal transplant and hemodialysis patients. Transplantation. (2007) 83:1059–68. doi: 10.1097/01.tp.0000259552.55689.fd
153. López-Medrano F, Fernández-Ruiz M, Silva JT, Carver PL, van Delden C, Merino E, et al. Multinational case-control study of risk factors for the development of late invasive pulmonary aspergillosis following kidney transplantation. Clin Microbiol Infect. (2018) 24:192–8. doi: 10.1016/j.cmi.2017.06.016
154. Zamboni M, Rubele S, Rossi AP. Sarcopenia and obesity. Curr Opin Clin Nutr Metab. Care. (2019) 22:13–9. doi: 10.1097/MCO.0000000000000519
155. Rossi AP, Bianchi L, Volpato S, Bandinelli S, Guralnik J, Zamboni M, et al. Dynapenic Abdominal Obesity as a Predictor of Worsening Disability, Hospitalization, and Mortality in Older Adults: Results From the InCHIANTI Study. J Gerontol A Biol Sci Med Sci. (2017) 72:1098–104. doi: 10.1093/gerona/glw203
156. Mikkola TM, von Bonsdorff MB, Salonen MK, Simonen M, Pohjolainen P, Osmond C, et al. Body composition as a predictor of physical performance in older age: a ten-year follow-up of the Helsinki Birth Cohort Study. Arch Gerontol Geriatr. (2018) 77:163–8. doi: 10.1016/j.archger.2018.05.009
157. Ozturk ZA, Turkbeyler IH, Abiyev A, Kul S, Edizer B, Yakaryilmaz FD, et al. Health-related quality of life and fall risk associated with age-related body composition changes; sarcopenia, obesity and sarcopenic obesity. Intern Med J. (2018) 48:973–81. doi: 10.1111/imj.13935
158. Tolea MI, Chrisphonte S, Galvin JE. Sarcopenic obesity and cognitive performance. Clin Interv Aging. (2018) 13:1111–9. doi: 10.2147/CIA.S164113
159. Fried LP, Darer J, Walston J. Frailty. Geriatric Medicine. New York, NY: Springer (2003). p. 1067–76.
160. Ting SM, Iqbal H, Hamborg T, Imray CH, Hewins S, Banerjee P, et al. Reduced functional measure of cardiovascular reserve predicts admission to critical care unit following kidney transplantation. PLoS ONE. (2013) 8:e64335. doi: 10.1371/journal.pone.0064335
161. Xue QL, Bandeen-Roche K, Varadhan R, Zhou J, Fried LP. Initial manifestations of frailty criteria and the development of frailty phenotype in the Women's Health and Aging Study II. J Gerontol A Biol Sci Med Sci. (2008) 63:984–90. doi: 10.1093/gerona/63.9.984
162. von Haehling S, Morley JE, Anker SD. An overview of sarcopenia: facts and numbers on prevalence and clinical impact. J Cachexia Sarcopenia Muscle. (2010) 1:129–33. doi: 10.1007/s13539-010-0014-2
163. Musso CG, Jauregui JR, Macias Nunez JF. Frailty phenotype and chronic kidney disease: a review of the literature. Int Urol Nephrol. (2015) 47:1801–7. doi: 10.1007/s11255-015-1112-z
164. Molfino A, Chiappini MG, Laviano A, Ammann T, Bollea MR, Alegiani F, et al. Effect of intensive nutritional counseling and support on clinical outcomes of hemodialysis patients. Nutrition. (2012) 28:1012–5. doi: 10.1016/j.nut.2012.01.008
165. Lee JS, Auyeung TW, Leung J, Kwok T, Woo J. Transitions in frailty states among community-living older adults and their associated factors. J Am Med Dir Assoc. (2014) 15:281–6. doi: 10.1016/j.jamda.2013.12.002
166. Espinoza SE, Jung I, Hazuda H. Frailty transitions in the San Antonio Longitudinal Study of Aging. J Am Geriatr Soc. (2012) 60:652–60. doi: 10.1111/j.1532-5415.2011.03882.x
167. Cameron ID, Fairhall N, Langron C, Lockwood K, Monaghan N, Aggar C, et al. A multifactorial interdisciplinary intervention reduces frailty in older people: randomized trial. BMC Med. (2013) 11:65. doi: 10.1186/1741-7015-11-65
168. Fiatarone MA, O'Neill EF, Ryan ND, Clements KM, Solares GR, Nelson ME, et al. Exercise training and nutritional supplementation for physical frailty in very elderly people. N Engl J Med. (1994) 330:1769–75. doi: 10.1056/NEJM199406233302501
169. Liu-Ambrose T, Khan KM, Eng JJ, Janssen PA, Lord SR, McKay HA. Resistance and agility training reduce fall risk in women aged 75 to 85 with low bone mass: a 6-month randomized, controlled trial. J Am Geriatr Soc. (2004) 52:657–65. doi: 10.1111/j.1532-5415.2004.52200.x
170. Malmstrom TK, Miller DK, Simonsick EM, Ferrucci L, Morley JE. SARC-F: a symptom score to predict persons with sarcopenia at risk for poor functional outcomes. J Cachexia Sarcopenia Muscle. (2016) 7:28–36. doi: 10.1002/jcsm.12048
171. Ikizler TA, Cano NJ, Franch H, Fouque D, Himmelfarb J, Kalantar-Zadeh K, et al. Prevention and treatment of protein energy wasting in chronic kidney disease patients: a consensus statement by the International Society of Renal Nutrition and Metabolism. Kidney Int. (2013) 84:1096–107. doi: 10.1038/ki.2013.147
172. Cano NJ, Fouque D, Roth H, Aparicio M, Azar R, Canaud B, et al. Intradialytic parenteral nutrition does not improve survival in malnourished hemodialysis patients: a 2-year multicenter, prospective, randomized study. J Am Soc Nephrol. (2007) 18:2583–91. doi: 10.1681/ASN.2007020184
173. Kopple JD. The National Kidney Foundation K/DOQI clinical practice guidelines for dietary protein intake for chronic dialysis patients. Am J Kidney Dis. (2001) 38(4 Suppl 1):S68–73. doi: 10.1053/ajkd.2001.27578
174. Cano N, Fiaccadori E, Tesinsky P, Toigo G, Druml W, Kuhlmann M, et al. ESPEN guidelines on enteral nutrition: adult renal failure. Clin Nutr. (2006) 25:295–310. doi: 10.1016/j.clnu.2006.01.023
Keywords: frailty, sarcopenia, kidney transplant, elderly, disability, malnutrition
Citation: Gandolfini I, Regolisti G, Bazzocchi A, Maggiore U, Palmisano A, Piotti G, Fiaccadori E and Sabatino A (2019) Frailty and Sarcopenia in Older Patients Receiving Kidney Transplantation. Front. Nutr. 6:169. doi: 10.3389/fnut.2019.00169
Received: 16 July 2019; Accepted: 23 October 2019;
Published: 12 November 2019.
Edited by:
Alessio Molfino, Sapienza University of Rome, ItalyReviewed by:
Maria Teresa Viggiani, University of Bari Aldo Moro, ItalyAlessandro Laviano, Sapienza University of Rome, Italy
Copyright © 2019 Gandolfini, Regolisti, Bazzocchi, Maggiore, Palmisano, Piotti, Fiaccadori and Sabatino. This is an open-access article distributed under the terms of the Creative Commons Attribution License (CC BY). The use, distribution or reproduction in other forums is permitted, provided the original author(s) and the copyright owner(s) are credited and that the original publication in this journal is cited, in accordance with accepted academic practice. No use, distribution or reproduction is permitted which does not comply with these terms.
*Correspondence: Alice Sabatino, YWxpY2Uuc2FiYXRpbm84NiYjeDAwMDQwO2dtYWlsLmNvbQ==