- 1Department of Clinical Chemistry and Laboratory Medicine, Saarland University Hospital, Homburg, Germany
- 2Department of Clinical Chemistry, Erasmus MC University Medical Center Rotterdam, Rotterdam, Netherlands
- 3Department of Rheumatology and Clinical Immunology, UMC Utrecht, Utrecht, Netherlands
- 4FrieslandCampina, Amersfoort, Netherlands
- 5Division of Human Nutrition, Wageningen University, Wageningen, Netherlands
- 6Department of Epidemiology, CARIM School for Cardiovascular Diseases Maastricht University, Maastricht, Netherlands
The EAT-Lancet commission recently suggested that transformation to healthy diets by 2050 will require a reduction of at least 50% in consumption of foods such as red meat and sugar, and a doubling in the global consumption of fruits, vegetables, nuts, and legumes. A diet rich in plant-based foods and with fewer animal source foods confers both improved health and environmental benefits. Notably, the risk of vitamin B12 deficiency increases when consuming a diet low in animal products. Humans are dependent on animal foods such as dairy products, meat, fish and eggs. Vitamin B12 deficiency is common worldwide, especially in populations with low consumption of animal foods because of low socioeconomic status, ethical reasons, or because of their lifestyle (i.e., vegans). According to the European Food Safety Authoroty, the recommended adequate intake of vitamin B12 is 4.0 μg/d for adults, and vitamin B12 requirements are higher during pregnancy and lactation. Infants and children from deficient mothers and elderly people are at risk for vitamin B12 deficiency. Diagnosis of vitamin B12 deficiency is hampered by low specificity of available biomarkers, and there is no consensus yet regarding the optimal definition of low vitamin B12 status. In general, a combination of at least two biomarkers is recommended. Therefore, this review presents an overview of vitamin B12 biochemistry and its biomarkers. We further summarize current recommendations of vitamin B12 intake, and evidence on the associations of vitamin B12 intake from different nutrient-dense animal foods with vitamin B12 status markers. Finally, potential consequences of low vitamin B12 status on different health outcomes for pregnant women, infants and elderly are presented.
Vitamin B12
Food Sources of Vitamin B12
Vitamin B12 (cobalamin) is an essential water-soluble micronutrient of microbial origin (1). It is naturally found in animal food products, including meat, poultry, (shell)fish, eggs, milk, and other dairy products (2). Vitamin B12 is generally not present in plant foods, but fortified breakfast cereals are a readily available source of vitamin B12 with high bioavailability (3, 4). Some nutritional yeast products also contain vitamin B12. This paper will only focus on vitamin B12 intake from natural food products, e.g. animal foods. According to recent results of the 2012-2016 Dutch Food Consumption Survey, the contribution of dairy, meat, (shell) fish, supplements, and eggs to total vitamin B12 intake is 38.5, 30, 8.5, 8.4, and 4.6%, respectively1. The nutrient dense animal food products rich in vitamin B12 are also rich in other nutrients such as zinc, iron, vitamin D, and proteins. To the best of our knowledge, interactions of vitamin B12 with these nutrients are not fully established, and it is not unlikely that associations of low vitamin B12 with health outcomes may be modified by deficiency of these nutrients or the presence of disorders that affect the digestive system. Notably, vitamin B12 and folate act together within the one-carbon metabolism, and paragraph 4.3.1 will elaborate on a potential role of high folate and low vitamin B12 status in health.
Uptake of Vitamin B12
Vitamin B12 plays an important role in one-carbon metabolism. Dietary vitamin B12 is, once ingested, bound to haptocorin (an animal protein), which carries vitamin B12 to the stomach. In the stomach, HCl and pepsin are released which release vitamin B12 from animal proteins. Free vitamin B12 then binds to haptocorrin in the stomach after which it is transported into the intestine, where vitamin B12 is released by pancreatic enzymes after which vitamin B12 binds to intrinsic factor (IF) (5). Vitamin B12 -IF complex binds to the cubulin receptor in the distal ileum, which takes up vitamin B12 through receptor-mediated endocytosis (5). Once taken up, vitamin B12 is released to the plasma where it is bound to its transport proteins; haptocorrin (HC) and trancobalamin (TC) (6, 7). In the circulation, 20–25% of vitamin B12 is bound to TC (called holo-TC or active B12), which is taken up and used by the cells. The other 75–80% of vitamin B12 is bound to HC, which is stored in the liver (6–8).
Causes of Acquired Vitamin B12 Deficiency
Vitamin B12 deficiency increased with age and is mostly due to malabsorption of the vitamin. In addition, low intake of animal food products—as outlined in chapter 3–and use of certain drugs may also result in vitamin B12 deficiency (Table 1). Absorption of vitamin B12 is dependent upon several processes including IF production. If gastric IF production is impaired, like when gastric parietal cells are destructed in case of gastritis or when less gastric parietal cells are strongly reduced in case of a gastric bypass less, this will result in reduced absorption of vitamin B12. Uptake of vitamin B12 takes place in the distal ileum, and in case of an ileal dissection, bacterial overgrowth or intestinal diseases such as Crohn's disease less vitamin B12 can be taken up by the ileal cells resulting in lower intake of vitamin B12. Drugs that regulate secretion of gastric acid production such as proton-pomp inhibitors can also lead to vitamin B12 deficiency due to in impaired release of vitamin B12 from food proteins. In addition, metformin, a drug that is used to lower glucose levels, has been shown to results in lower vitamin B12 levels in serum most likely due to interfering with calcium-related binding of IF-B12 complex to the cubulin receptor (9). Considering drugs the party drug nitrous oxide has gained a lot of attention recently as it has been shown that high intake of nitrous oxide can result in vitamin B12 deficiency due to irreversible oxidation of the cobalt ion of MeCbl and AdoCbl, which makes both coenzymes inactive resulting in increased levels of methylmalonic acid (MMA) and homocysteine (10). Vitamin B12 and active B12 levels are mostly not low in serum in case of nitrous oxide overabuse and functional markers such as MMA and homocysteine should be used for laboratory diagnosis (10).
Biochemistry of Vitamin B12
Different derivatives of cobalamin exist of which methylcobalamin (MeCbl) and adenosylcobalamin (AdoCbl) are the physiological co-enzyme forms. MeCbl is a cofactor in the methionine-synthase dependent remethylation of homocysteine into methionine, which takes place into the cytosol. This remethylation reaction is an important step of the one-carbon metabolism, in which also reduction of folate derivatives takes place, which are important for DNA synthesis. In addition, methionine is an essential amino acid which is involved in formation of the universal methyl donor S-adenosylmethionine. Low dietary intake of vitamin B12 results in elevated homocysteine levels and might affect DNA synthesis and DNA methylation. AdoCbl is involved in the l-methylmalonyl-CoA-mutase-dependent conversion of methylmalonyl-CoA into succinyl-CoA, which takes place in the mitochondrium (11). Low dietary intake of vitamin B12 results in accumulation of methylmalonyl-CoA that converts to MMA. Increasing levels of MMA are observed in plasma in case of vitamin B12 deficiency.
Biomarkers of Vitamin B12
Several biomarkers (Table 2) exist to evaluate vitamin B12 status in blood. The most used biomarker is total vitamin B12, which measures vitamin B12 bound to both transport proteins (HC and TC), which gives a generally estimation of the vitamin B12 status in the blood (7, 11). In addition, holoTC (active B12), which is the transcobalamin-bound vitamin B12 has been suggested to be an early marker of vitamin B12 status. HoloTC can be used as an initial test to measure vitamin B12 status in blood (12–14). Both total vitamin B12 and holoTC are applied in laboratory diagnostics and functional tests such as homocysteine and MMA are used to confirm diagnosis in case of low-normal vitamin B12 status. No consensus exists about which cut-off values should be applied and which is the best marker or combination of markers to assess vitamin B12 status (15). In general, reference intervals are used or alternative cut-off values are chose based upon sensitivity and specificity (14). However, these cut-off values are not generally applicable, as total vitamin B12 and active vitamin B12 tests are not harmonized, which hampers interpretation difficult for general practitioners.
Consideration
Regarding biomarkers of vitamin B12 there is a need to establish reference intervals of total vitamin B12 in pregnancy as these levels decrease during pregnancy. In addition, better biomarkers are necessary to determin vitamin B12 deficiency as total B12 and active B12 hamper diagnostic specificity.
Present Recommended Dietary Intake of Vitamin b12 and Their Limitations
Dietary Reference Values for Vitamin B12
Several organizations have followed different approaches to set the dietary reference values for vitamin B12 (Table 3). The dietary reference values for adult men and women aged >18 years range between 2 and 4 μg/d depending on the judgments used. Generally, the increased requirements for vitamin B12 in women during pregnancy and lactation have been acknowledged and translated into higher reference values compared with non-pregnant women. No special intake recommendations exist for elderly people, despite the evidence that vitamin B12 malabsorption and deficiency are common in the elderly. Furthermore, the intake recommendations for infants were mainly based on outdated observational studies and on vitamin B12 content in human breastmilk. Measurement of vitamin B12 in breastmilk has been hampered by methodological problems due to the high milk haptocorrin that interferes with most available assays. In general, the European Food Safety Authority (EFSA) panel defined the Adequate Intake of vitamin B12 based on three indicators of vitamin B12 requirements (16):
1- Maintenance of heamatological markers in patients with pernicious anemia in a remission phase (i.e., correcting hemoglobin, mean corpuscular volume, and reticulocyte).
2- Maintenance of the total body stores of vitamin B12 (≈2–3 mg) by adjusting the daily requirements for the daily loss of the vitamin. The absorption efficacy of vitamin B12 from foods is assumed to be 40% and the daily loss is between 2 and 6 μg/d (biliary loss or transfer to the fetus or infant (via the placenta or the breastmilk).
3- Maintenance of normal serum levels of vitamin B12 markers (total vitamin B12, MMA, holoTC, and Hcy).
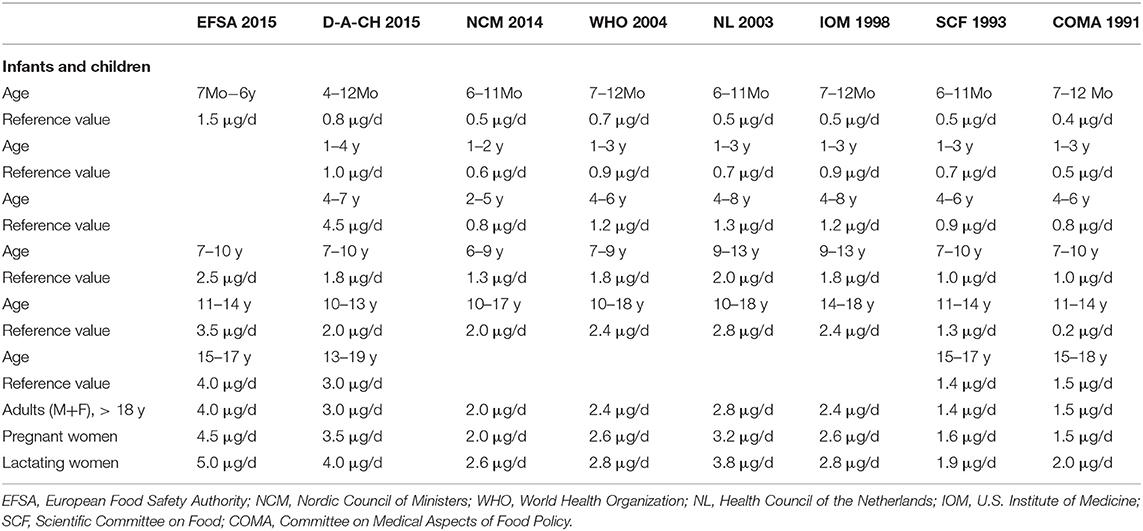
Table 3. Dietary reference values for vitamin B12 (in μg/d) in different age and sex groups as suggested by different organizations.
Vitamin B12 Intake Necessary for Maintenance of Normal Vitamin B12 Biomarkers
Approximately 1% of a high oral vitamin B12 dose (i.e., derived from supplemental cyanocobalamin) crosses the intestinal barrier into the blood via simple diffusion (17), after saturation of IF (max. 5 μg/meal is absorbed via IF) (18). Vitamin B12 intake shows a dose-response relationship with blood vitamin B12 markers. We focused here on studies on vitamin B12 biomarkers in relation to vitamin B12 intake in the range below 50 μg/d. Studies using therapeutic doses of vitamin B12 are beyond the scope of this review.
In a study among 98 Danish post-menopausal women Bor et al. (19)suggested that an intake of 6 μg/d vitamin B12 (determined from 7-d weighed food records) is sufficient to maintain highest concentrations of vitamin B12 and holoTC, and lowest concentrations of MMA and Hcy with median (25–75th percentiles) of 380 (270–480) pmol/L for vitamin B12, 119 (92–162) pmol/L for holoTC, 0.12 (0.14–0.17) μmol/L for MMA, and 9.8 (8.3–11.4) μmol/L for Hcy compared to intakes lower than 6 μg/d. A similar study in 299 healthy US adults found that mean levels of vitamin B12 and holoTC were highest in the intake range between 4.2 and 7.0 μg/d, while plasma MMA and Hcy reached lowest levels in subjects who achieved an intake of ≥7.0 μg/d (20).
The association between vitamin B12 markers and intake was generally weaker in studies in elderly people (21) compared to those in younger people, in studies considering only dietary intake compared to studies on vitamin B12 intake from diet plus supplements, and in studies considering vitamin B12 intake up to 100 μg/d than those using larger doses (21). This could be due to a better absorption of free vitamin B12 from supplements compared to protein-bound vitamin B12 from foods. Van Asselt et al., reported a median vitamin B12 intake (from diet plus supplements) of 6.3 μg/d in elderly Dutch people (mean age 76 years) with normal vitamin B12 markers (vitamin B12 >260 pmol/L and MMA <320 nmol/L) (22). Subjects with mild vitamin B12 deficiency (vitamin B12 <260 pmol/L and MMA>320 nmol/L) had a median intake of 4.9 μg/d, and those with a possible deficiency (either low B12 or elevated MMA) had a median intake of 5.1 μg/d (22). The deficiency in elderly people could be better explained by malabsorption disorders instead of by minor variations in intakes (22). In line with this, associations of plasma concentrations of vitamin B12, MMA, and Hcy with vitamin B12 intake was not present in some studies (23, 24) possibly due to age- and disease- related malabsorption.
A meta-analysis on the association between vitamin B12 intake and biomarkers Dullemeijer et al. estimated that doubling the intake of vitamin B12 is associated with 11.0% (95% CI: 9.4%, 12.5%) higher serum vitamin B12 concentration (21). The association between vitamin B12 intake and biomarkers was stronger in studies conducted in elderly people than in adult populations, which could be related to low baseline concentrations of vitamin B12 in the elderly (21). The slope of the change of plasma vitamin B12 in relation to vitamin B12 intake flattened when vitamin B12 intake was >100 μg/d (21), which could reflect the limited proportional absorption of vitamin B12 from high dose supplements. Compared to plasma vitamin B12, the changes of serum MMA (mean −7%; 95% CI = −10 to −4%) in response to doubling vitamin B12 intake were smaller (21), which could be due to the short observational time of most studies and to the influence of renal function on MMA levels.
The increase in plasma vitamin B12 and the decrease in functional markers appear to depend on population characteristics (mainly age and accompanying diseases), duration of the intervention, starting plasma concentrations of the vitamin, and the administered dose of crystalized cyanocobalamin, even in non-therapeutic ranges. In general, a daily intake of free cyanocobalamin as low as 1.5–2.5 μg provided for approximately 4–6 months may increase plasma vitamin B12 by 50–100 pmol/L.
Consideration
A total intake of vitamin B12 from the diet between 4 and 7 μg/d is associated with normal plasma vitamin B12 and MMA and thus appears to be adequate to maintain body vitamin B12 status in adults. This intake might be insufficient if people have difficulties in chewing foods, releasing the vitamin from its food binding, and/or absorbing it due to disorders as shown in Table 1 (25, 26). Elderly people with H-pylori infection (26), or food-cobalamin malabsorption (25, 27, 28) may be at risk for vitamin B12 deficiency despite sufficient dietary intake. It is unclear if elderly people would generally benefit from higher vitamin B12 intake recommendations.
The Association of Animal Food Products Containing Vitamin b12 With Circulating Vitamin B12 Biomarkers From Observational Studies
In addition to supplements or fortified cereals as potential sources of vitamin B12, this paper focusses on vitamin B12 intake from natural food products, e.g., animal foods. In total, 19 observational studies were identified addressing associations of vitamin B12 containing aminal food items with plasma or serum vitamin B12 biomarkers. These studies were performed among infants (n = 1 study) (29), children (n = 5 studies) (30–35), pregnant women (n = 1 study) (36), adults (n = 7 studies) (3, 19, 20, 37–45), and elderly (n = 5 studies) (22–24, 43, 46–49). The majority of these studies had a cross-sectional design, except for some case-control study conducted among infants (29), children (30), and elderly (24), and a prospective study (3). The observational studies were heterogenous with respect to dietary assessment of animal food items or dietary patterns, usage of different vitamin B12 biomarkers, and statistical analyses, which hampers the direct comparison between studies (Table 4). Therefore, this section summarizes main findings from individual studies by different age categories.
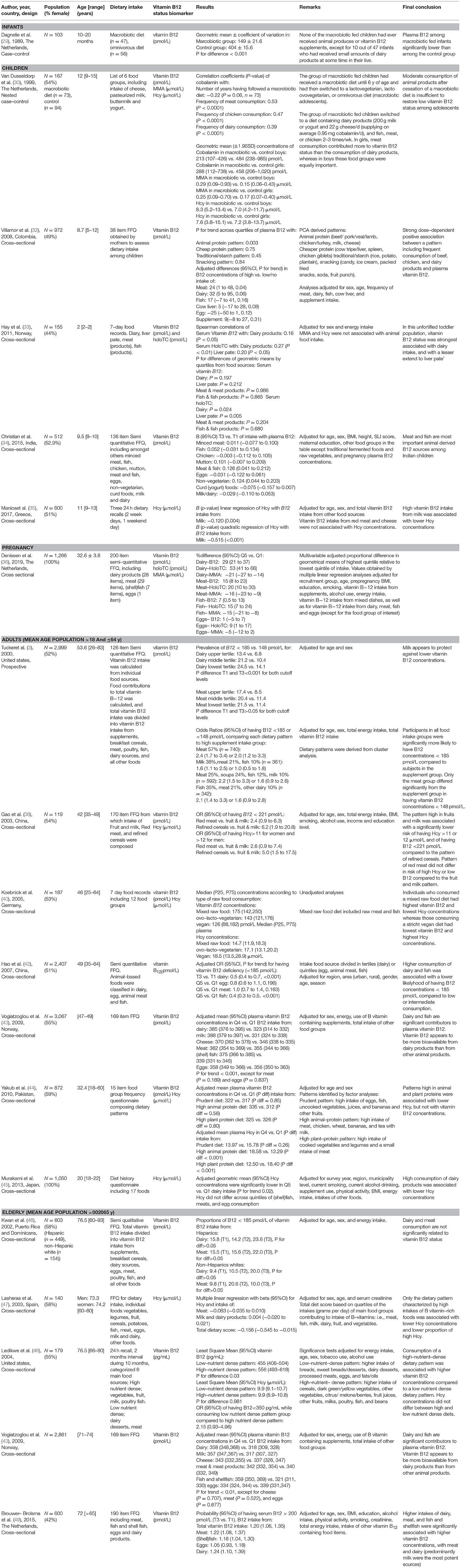
Table 4. Main characteristics and results of observational studies addressing the relation between dietary intake and vitamin B12 status biomarkers among different age categories.
Infants and Children
Two case-control studies among infants (29) and children (30) investigated the effects of a macrobiotic dietary regime (no animal foods) on vitamin B12 biomarkers. Plasma vitamin B12 concentrations were significantly lower among macro-biotic fed infants (n = 47) as compared to their omnivorous fed controls (n = 56) (29). In another study, adolescents who had received a macrobiotic diet until 6 y of age and had then switched a diet containing animal products (n = 73) still had significantly lower vitamin B12 concentrations and higher concentrations MMA, but comparable Hcy concentrations, as compared to their age-matched controls who consumed an omnivorous diet from birth onwards. These results suggest that switching from a macrobiotic diet to moderate consumption of animal food products is inadequate to restore vitamin B12 status among children with a low vitamin B12 in early childhood (30). A Swedish study among adolescents [mean (SD) age: 17.5 (1.0) year] compared vitamin B12 intake between 30 vegans (15 males and 15 females) and 30 sex-, age-, and height-matched omnivores. This study revealed significant differences in vitamin B12 intake between vegans and omnivores, with vitamin B12 intakes of 0.0 and 0.1 μg/day for vegan females and males, respectively, and intakes of 5.0 and 5.9 μg/day for omnivorous females and males, respectively (P for differences < 0.001)(50).
In contrast to following a well-defined dietary regime, a Colombian study identified 4 dietary patterns derived from an 28-item FFQ based on principal component analysis. Patterns included diets rich in (1) animal protein (e.g., beef/pork/veal/lamb, chicken/turkey, milk, cheese), (2) cheaper protein (e.g., cow tripe/liver, spleen, chicken giblets), (3) traditional/starch (e.g., rice, potato, plantain), and (4) snacking products (e.g., candy, ice cream, packed fried snacks, soda, fruit punch). Only the pattern rich in animal protein was significantly positively associated with plasma vitamin B12 (P for trend = 0.003). This study also studied individual animal food groups, and fully adjusted differences in plasma vitamin B12 for low vs. high consumers were significant only for meat, but not for dairy, fish, cows liver, and eggs (32). In line with these findings, a study conducted in India (n = 512) also showed statistically significant positive associations of the meat and fish group with plasma vitamin B12 in fully adjusted models, but not for other animal products (34). Others observed inverse associations between vitamin B12 intake from milk with plasma Hcy, but not for vitamin B12 intake from red meat or cheese (35). One study measured multiple biomarkers for vitamin B12 status. Serum MMA and Hcy concentrations were not correlated with animal food groups, whereas correlation coefficients of serum vitamin B12 and holoTC with dairy intake were 0.16 (P < 0.05) and 0.27 (P < 0.01), respectively. In addition, intake of liver pate correlated with holoTC (r = 0.20, P < 0.05). None of the vitamin B12 biomarkers were associated with fish or eggs intakes (33).
When considering different animal products within individual studies among children, differences in vitamin B12 concentrations were most pronounced when comparing high vs. low intake of dairy products, followed by meat and fish intake (32), and dairy products showed stronger correlations with vitamin B12 and holoTC concentrations compared to liver pate, meat and fish (33). In another study, only a combined group of meat and fish was associated with vitamin B12 concentrations, whereas the individual components fish, chicken, eggs, and dairy were not related to plasma vitamin B12 (34).
Pregnancy
Only one study among pregnant women (n = 1266) was identified that addressed the association of vitamin B12 intake from dairy, meat, (shell)fish, and eggs with circulating levels of vitamin B12 biomarkers, and presence of vitamin B12 deficiency in week 34–36 of pregnancy. Results showed that vitamin B12 from dairy, meat and fish, but not eggs, independently contributed to plasma concentrations of total vitamin B12, holoTC and MMA, as shown by statistically significant dose-response relationships. Vitamin B12 intake from each of these products groups was also independently associated with a reduced odds of vitamin B12 deficiency (holoTC <35 pmol/L and MMA >0.45 μmol/L). Egg-derived vitamin B12 was negatively associated with holoTC but not associated with other vitamin B12 biomarkers (36).
Adults
Those studies addressing specific animal products revealed that high dairy consumption was associated with significantly lower prevalences of vitamin B12 concentrations <185 pmol/L (3, 42) and 148 pmol/L (3) compared to low dairy consumption(3), significantly higher vitamin B12 concentrations among high milk and cheese consumers compared to low consumers (43), and significantly lower Hcy concentrations with high dairy intake compared to low dairy intake (45). Similarly, those with a fish consumption in the highest quintile had a significantly lower odds of having vitamin B12 deficiency compared to adults who had a fish consumption in the lowest quintile (42), and plasma vitamin B12 concentrations were significantly higher in those consuming high amounts (fourth quartile) compared to low fish consumers (first quartile) (43). In contrast, analyses on meat consumption did not show any relation of meat consumption with vitamin B12 deficiency (3, 42). Moreover, plasma vitamin B12 concentrations (43) and serum Hcy (45) did not differ between high and low meat consumers. Egg consumption was also not related to plasma vitamin B12 status (42, 43, 45). None of the studies investigated the link between animal food products with MMA or holoTC concentrations in adults.
A number of studies described vitamin B12 intake or vitamin B12 biomarkers among omnivores, vegetarians and vegans. All studies consistently observed that vitamin intake was lowest, intermediate and highest among vegans, vegetarians and meat-eaters, respectively (51–55). Similarly, studies also observed lowest, intermediate and highest vitamin B12 concentrations among vegans, vegetarians and meat-eaters, respectively (51, 54–56), or with holoTC concentrations(51). In line with this, prevalences of vitamin B12 deficiency were highest among vegans and lowest among omnivorous (52, 54, 56), although it should be noted that these studies used different criteria to define vitamin B12 deficiency. Other studies addressing dietary patterns in relation to vitamin B12 status have used different approaches to define patterns. Tucker et al derived patterns by cluster analysis. Food groups that contributed to vitamin B12 were entered into the analysis as percentages of total individual vitamin B12 intake. The cluster procedure assigns individuals to predetermined numbers of clusters in a manner that maximizes the difference across groups for the included variables. Factor analyses reveal that 6 patterns led to the clearest separation of vitamin B12 sources, being (1) supplements (61% Supplements, 11% meat), (2) meat (57% meat), (3) milk (38% milk, 21% meat, 10% fish), (4) cereal (37% Cereal, 17% meat, 12% milk, (5) meat and soups (25% Meat, 24% soups, 12% fish, 10% milk), and (6) fish (35% Fish, 21% meat, 10% other dairy). Plasma vitamin B12 concentrations were significantly lower in the meat pattern than in the cereal and milk patterns, despite similar average vitamin B12 intakes in these 3 groups. Subjects in all food intake groups were significantly more likely to have plasma vitamin B-12 concentrations <185 pmol/L compared to subjects in the supplement group, with odds ratios ranging from 1.6 for the milk group to 2.4 for the meat group. For the likelihood of plasma vitamin B-12 concentrations <148 pmol/L, the meat group was the only group that differed significantly from the supplement group [OR (95%CI) = 2.0 (1.2–3.3)] (3). Another study also used factor analyses to identify major dietary patterns. Three patterns were defined as (1) prudent diet (high intake of eggs, fish, uncooked vegetables, juices, bananas, and other fruits), (2) high animal-protein diet (high intake of meat, chicken, wheat, bananas, and tea with milk), and (3) high plant-protein diet (large intake of cooked vegetables and legumes and a small intake of meat). High intakes of the prudent dietary pattern and the plant protein dietary pattern (quartile 4) compared with lowest intake (quartile 1) were associated with a reduced odds of hyperhomocysteinemia (Hcy > 15 μmol/L), with OR (95% CI) of 0.52 (0.30–0.90) and 0.42 (0.25–0.69), respectively. In contrast, a high consumption of the animal-protein diet was positively associated with hyperhomocysteinemia [OR (95% CI) quartile 4 vs. quartile 1 = 2.10 (1.22–3.60). Vitamin B12 concentrations did not differ across quartiles of any of the diets (44). Finally, another study investigated if vitamin B12 and Hcy concentrations differed across different degrees of vegetariarism (vegan, ovo-lacto-vegetarian, and mixed raw food diet including raw meat and fish). This study revealed that consumption of a vegan diet had lowest median vitamin B12 concentrations and highest Hcy concentrations and consumption of a pattern with mixed raw foods had highest vitamin B12 and lowest Hcy concentrations (40).
Elderly
Five observational studies were identified among elderly (average age population >65 y), out of which 4 focussed on specific animal products (43, 46, 47, 49) and two on dietary patterns (47, 48). A study investigating vitamin B12 intake from supplements, breakfast cereals, dairy and meat consumption revealed that only cereal, but not dairy or meat consumption was related to vitamin B12 concentrations and proportions of B12 concentrations <185 pmol/L. In line with this, meat and milk and dairy products were not associated with Hcy concentrations (47). However, other studies showed that high consumption of dairy and fish were accompanied by higher plasma vitamin B12 concentrations compared to low consumption of these food groups(43), and that high intakes (T3 vs. T1) of meat, (shell)fish, and dairy were associated with an increased odds of having vitamin B12 concentrations >200 pmol/L (49).
A dietary pattern characterized by high intakes of foods rich in B-vitamins, such as meat, fish, milk, dairy, fruit, and vegetables, was associated with lower mean Hcy concentrations (47). Another study comparing consumption of high vs. low nutrient-dense dietary pattern revealed higher vitamin B12 concentrations in those consuming a high nutrient dense pattern compared to those consuming a low nutrient dense dietary pattern. Hcy concentrations did not differ between these the high and low nutrient dense patterns. In this study, a high nutrient-dense pattern was defined as higher intake of cereals, dark green/yellow vegetables, other vegetables, citrus/ melons/berries, fruit juices, other fruits, milks, poultry, fish, and beans, whereas a low-nutrient-dense pattern consisted of higher intake of breads, sweet breads/desserts, dairy desserts, processed meats, eggs, and fats/oils (48).
Summary of General Findings and Considerations
Dairy consumption seems to be the strongest determinant of vitamin B12 concentrations. However, when comparing the magnitude of the relation of dairy, meat, fish or egg consumption with vitamin B12 status it is essential to adjust statistical analyses for vitamin B12 intake from other animal food products, which was done in 5 studies (3, 34, 35, 43, 49). In addition, the specific individual food items representing dairy, meat, and fish consumption could not always be derived from the individual studies, which hampers direct comparison between studies. Finally, nutrient-density of different dairy (milk, yogurt, cheese, curd cheese), meat (chicken, pork, veal), and fish (lean vs. fatty) differs considerably. There is a knowledge gap regarding the bioavailability of vitamin B12 from these different animal food products. In addition, associations of different animal product groups with MMA and holoTC remain largely unknown.
Vitamin B12 Intake or Status and Health Outcomes
This section addresses the associations between low vitamin B12 intake or status (defined by abnormal biomarkers) and several health outcomes from epidemiological studies performed in vulnerable population groups.
Maternal Vitamin B12 Status and Pregnancy
Vitamin B12 deficiency can cause megaloblastic anemia/pernicious anemia (57). Women with untreated pernicious anemia have often infertility problems or repeated abortions. When women were diagnosed with vitamin B12 deficiency and had received vitamin B12, pregnancy occurred (58–60).
The metabolisms of vitamin B12 and folate interact. Supplementation of folic acid before pregnancy and in the first pregnancy trimester reduces the risk of neural tube defects (NTDs) in the child. An inverse association has been reported between NTDs risk and vitamin B12 status or polymorphisms in vitamin B12 metabolizing enzymes (61–64). Ray et al. have shown that a low serum holotranscobalamin (<55.3 pmol/L) at 15–22 weeks of gestation was associated with the risk of NTDs in a study that was done in Ontario after the fortification with folic acid (65). There is a general agreement that vitamin B12 concentrations >250 pmol/L in women entering pregnancy is associated with low risk for NTDs compared to when vitamin B12 is below this level (63). These findings may suggest that supplementation with vitamin B12 may reduce the risk for NTD. However, it is unknown if supplementation with both folic acid and vitamin B12 decreases the number of births with a NTD compared to supplementation with folic acid alone.
Maternal vitamin B12 status determines vitamin B12 status of the child at birth and thereafter. Vitamin B12 in neonates at birth is higher than that in plasma of the mother, but it generally declines in the infants after birth. In a nested case-control study, concentrations of vitamin B12, Hcy, and MMA were assessed in healthy pregnant women (n = 114) from week 18 of pregnancy through 6 mo postpartum and related to infant cobalamin status at 6 mo, and compared with healthy, never-pregnant women aged 18–40 y controls (n = 123). Compared to controls, vitamin B12, Hcy and MMA were lower in pregnant women at 18 w of pregnancy. Vitamin B12 significantly decreased from week 18 to week 36 of pregnancy and increased again by 6 wk postpartum, whereas Hcy and MMA concentrations increased from week 18 of pregnancy to 6 wk postpartum. Infant vitamin B12 concentration at 6 months correlated with maternal vitamin B12 concentration during pregnancy and postpartum (rho = 0.36–0.55, P < 0.001). A maternal vitamin B12 concentration <394 pmol/L during week 18 of pregnancy was associated with an increased risk (OR: 4.2; 95% CI: 1.5, 11.5) of infant vitamin B12 deficiency at 6 mo (defined as tHcy ≥6.5 μmol/L) (66). Breastfed infants are at risk for deficiency in this period if their depleted mothers are not taking vitamin B12-containing supplements (67). Most cases of infantile vitamin B12 deficiency become manifested between 6 and 11 months of age. Neuromuscular and growth or developmental disorders or cerebral atrophy can occur. Symptoms such as irritation, feeding difficulties, stunting, or anemia have been reported in deficient neonates. Vitamin B12 deficiency may leave residual neurological abnormalities (68).
Breastfeeding
Prolonged breastfeeding is related to food insecurity and represent a problem in many parts of the world where the mothers have multiple micronutrient deficiencies. In Indian children (mean age 16 months) from families of low to middle socioeconomic status, prolonged breastfeeding was associated with stunting, anemia, low weight, or wasting (low weight-for-length) in the child (69, 70). A causal role for vitamin B12 deficiency in childhood stunting is possible, but not well-investigated due to possible confounding by intestinal infections, protein deficient diet or multiple nutrient deficiencies that can affect stunting (71–73). Maternal and/or infant vitamin B12 status has been related to infant physical growth (74), anemia, and cognitive and mental function (75). However, it is unknown if requirements for vitamin B12 in pregnant and lactating women should be increased, and if improving maternal or child vitamin B12 status can improve the outcome such as anemia (76) and cognitive development in the child. There are currently some studies ongoing on this topic (77).
Elderly
Several epidemiological studies reported associations between vitamin B12 biomarkers and brain health, cognitive function, or bone health [reviewed in (78)]. In addition, some evidence appears to suggest that lower B12 status is related to increased pro-oxidant and decreased antioxidant status (79). In a 5-y follow up study among dementia-free elderly people, vitamin B12 in the lowest tertile (<308 pmol/L) or holoTC (< 54 pmol/L) was negatively associations with accelerated brain volume loss, compared with those with higher levels (80). No such association was observed for MMA and Hcy with brain volume loss. In contrast, Smith et al., observed an association between high plasma Hcy and MMA and the risk of cognitive impairment in elderly people who were free of dementia at baseline, while higher holoTC and vitamin B12 showed a negative association with the risk of cognitive impairment (81). Most intervention studies to lower tHy have used multivitamins containing folic acid and vitamin B12 among other vitamins, and studies have shown a protective effect of multivitamins containing vitamin B12 on global cognition (82), brain shrinkage (83), or quality of life scores (84).
In a 6-y follow up study in Swedish elderly men (n = 790; age range 70–81 years), lowered holoTC was associated with an increased risk of fracture (hazard risk for the lowest tertile of holoTC = 1.74; 95% CI 1.12–2.69) (85), while Hcy and MMA were not associated with bone mineral density or fracture risk. In a nationally representative cross-sectional study in U.S. women ≥50 years, Baily et al., have reported an association between elevated Hcy and MMA and the risk of lumbar spine osteoporosis, but B12 and MMA were not associated with bone mineral density (86). In an update meta-analysis including RCTs on the association between homocysteine-lowering trials and fracture risk in elderly people (87)., Garcia Lopez et al., found no association between lowering Hcy (using folic acid and vitamin B12) and the risk of fractures (87).
In general, the evidence from homocysteine-lowering trails by B-vitamins on cognition or bone fracture is mixed and there are several negative studies (78). There is some evidence that vitamin B12 supplementation could have positive effects on health in elderly people who are vitamin B12-deficient. Nevertheless, more research in this group of elderly people is still warranted.
Special Considerations
The Health Significance of High Folate and Low Vitamin B12
There is some concern about supplementing high doses of folic acid to women of reproductive age with low vitamin B12 intake. Supplementing folic acid >1 mg/d is common in many parts of the world where low vitamin B12 is endemic, thus causing unbalanced intake and status of the two B-vitamins. Vitamin B12 deficiency is common in pregnant women from many countries such as Colombia (88), Brazil (89), or India (90). Using multivitamin supplements before pregnancy is not common and is related to education and income level (91). Imbalanced levels of folate and vitamin B12 (i.e., high folate and low B12) have raised some concern, although this topic is not well-investigated yet.
Due to low animal source foods, Indian women are a good example of a population with imbalanced folate-to-B12 ratio. A small observational study including Indian women at 36 weeks of gestation and their newborn children within 24 h after birth reported a negative association between folate-to-B12 ratio and birth weight, birth length, and head and chest circumferences (92). It has been reported that neonates born to Indian women with low B12 intake, consuming >1 mg/d of folic acid, and those with a low B12-to-total folate intake ratio are at increased risk of being born small for gestational age (93). Moreover, high red blood cell (RBC)-folate in Indian pregnant women has been related to adiposity in their children (94) and to increased risk of insulin resistance in the children if maternal plasma B12 was also low (94, 95). The risk of gestational diabetes was higher in vitamin B12-deficient Indian women (95), and the risk of persistent diabetes in the deficient women with gestational diabetes was higher in those women with higher folate status (95).
In UK pregnant women, both folate and vitamin B12 status showed inverse associations with maternal BMI (96). Vitamin B12 insufficiency was also associated with insulin resistance in those women (96). Women with a combination of low plasma vitamin B12 (<170 pmol/L) and folate (<10.3 nmol/L) had the highest BMI, while those with high vitamin B12 (>238 pmol/L) and folate status (>18.3 nmol/L) had the lowest BMI (96). Another study in UK pregnant women (beginning of the 3rd trimester) observed a negative association between maternal vitamin B12 and the risk of obesity and gestational diabetes (97). In pregnant women with gestational diabetes, the risk for fetal macrosomia was higher in the highest folate quartile and lowest vitamin B12 quartile (97). In a study among Spanish women, maternal folate was negatively associated with insulin sensitivity (HOMA-IR test), while low vitamin B12 was associated with insulin resistance (98). Given these close interactions between vitamin B12 and folate, it is not unlikely that other nutrient-nutrient interactions occur, for example with n-3 fatty acids. As such, future studies may also take nutrient-density of specific animal food products or dietary patterns into account.
The Health Significance of High Vitamin B12 Intake and Status
High dietary intake of vitamin B12 has not been shown to be disadvantageous. Supplemental forms of vitamin B12 are considered safe and there is no evidence-based Tolerable Upper Intake Level for vitamin B12. However, elevated plasma concentrations of vitamin B12 (often defined as plasma vitamin B12 levels >600, >800, or >1,000 pmol/L) in individuals not receiving supplemental vitamin B12 have been described in studies in patients with different cancers, liver diseases, or type 2 diabetes (99) that were later attributed to renal dysfunction (100, 101). The clearance of a single dose of radiolabeled vitamin B12 has been shown to be delayed in patients with renal dysfunction (101). Studies conducted in hospital settings have shown that elevated plasma vitamin B12 is associated with elevated plasma levels of liver enzymes and creatinine or albuminurea (102) and several clinical conditions such as chronic kidney disease, diabetes, liver disorders (of any etiology), alcoholism, or malignancies (100, 103, 104).
Elevated plasma vitamin B12 has been shown to predict future cancer (105), cardiovascular mortality in cohort studies (106), and levels above 400 pmol/L may predict short term mortality (within 90 days) irrespective of the cause of death in hospitalized elderly patients (107). A prospective study on 161 patients with different cancers investigated serum vitamin B12 concentrations and the time of death (108). The global median survival time was 45 days (CI 95%: 32–56 days). The highest mortality corresponded to the highest vitamin B12 levels. A significant link was found between elevated vitamin B12 (>600 pmol/l) and the presence of metastasis, a tumor or liver problems (108). In a recent study based on primary care database, the incidence rate ratio for cancer was 4.72 (95% confidence interval: 3.99–5.58) in persons with vitamin B12 >1,000 pmol/L compared to those with a low vitamin B12 levels after multivariate adjustments (109).
Notably, a causal role for vitamin B12 in future diseases or mortality cannot be assumed based on the presence of elevated plasma vitamin B12 levels. High vitamin B12 test results could be due to supplementation (i.e., long storage time), release from damaged tissues, or reduced kidney excretion. In all instances high plasma vitamin B12 levels are likely to be too unspecific to be used as a screening test for existing tumors or to predict future health outcomes. The likelihood of detecting cancer in patients with high vitamin B12 test has not been studied. Considering the high rate of false positive results (with seriously negative impact on patients), there is currently no evidence to initiation of further cancer diagnostic tests in subjects with plasma vitamin B12 levels >600 pmol/L.
Consideration
Studies reporting on the relationship between vitamin B12 intake and health outcomes have limitations due to methodological variations related to quantifying the intake, differences in population characteristics and to the fact that clinical outcomes such as anemia or neuropathy are late manifestations of the deficiency and are not specific for vitamin B12 deficiency.
Overall Summary
The current recommendation is to decrease consumption of animal foods and increase consumption of plant foods, as recently suggested by the EAT-Lancet commission (110). However, a major concern of diets low or without animal products is the risk of vitamin B12 deficiency. This review showed that a total intake of vitamin B12 from the diet between 4 and 7 μg/d is associated with normal plasma vitamin B12 and MMA and thus appears to be adequate to maintain body vitamin B12 status in adults. However, this intake might not be sufficient if people have difficulties in chewing foods, releasing the vitamin from its food binding, and/or absorbing it due to intrinsic factor antibodies or medications (25, 26). It is currently unknown if vitamin B12 requirements should be age-specific, since vitamin B12 deficiency is common in elderly.
When considering specific animal food products, dairy consumption seemed to be a stronger determinant of vitamin B12 concentrations than meat, fish and eggs. However, nutritional composition of different dairy (milk, yogurt, cheese, curd cheese), meat (chicken, pork, veal), and fish (lean vs. fatty) differs considerably, and bioavailability of vitamin B12 from these different animal food products together with potential interactions between vitamin B12 and other nutrients from these nutrient-dense animal products are unclear. Therefore, nutrient-density or well-known interactions between nutrients, such as folate and vitamin B12, should also be considered when studying the relations of intake on status or health.
Author Contributions
SE, RO, SH, and MV substantial contributions to the conception or design of the work. All authors drafting the work or revising it critically for important intellectual content. All authors provide approval for publication of the content. All authors agree to be accountable for all aspects of the work in ensuring that questions related to the accuracy or integrity of any part of the work are appropriately investigated and resolved. SE, RO, SH, and MV selected extracted relevant papers of this manuscript. SE, RO, and SH wrote the manuscript. SE had primary responsibility for final content. All authors read and approved the final manuscript.
Conflict of Interest Statement
EvdH was employed by company FrieslandCampina.
The remaining authors declare that the research was conducted in the absence of any commercial or financial relationships that could be construed as a potential conflict of interest.
Abbreviations
IF, intrinsic factor; holoTC, holotranscobalamin; MMA, methylmalonic acid; Hcy, total homocysteine; NTD, neural tube defects.
Footnotes
References
1. Martens JH, Barg H, Warren MJ, Jahn D. Microbial production of vitamin B12. Appl Microbiol Biotechnol. (2002) 58:275–85. doi: 10.1007/s00253-001-0902-7
2. Watanabe F. Vitamin B12 sources and bioavailability. Exp Biol Med. (2007) 232:1266–74. doi: 10.3181/0703-MR-67
3. Tucker KL, Rich S, Rosenberg I, Jacques P, Dallal G, Wilson PW, et al. Plasma vitamin B-12 concentrations relate to intake source in the Framingham Offspring study. Am J Clin Nutr. (2000) 71:514–22. doi: 10.1093/ajcn/71.2.514
4. Institute of Medicine Standing Committee on the Scientific Evaluation of Dietary Reference I its Panel on Folate OBV Choline: The National Academies Collection: Reports funded by National Institutes of Health. In Dietary Reference Intakes for Thiamin, Riboflavin, Niacin, Vitamin B6, Folate, Vitamin B12, Pantothenic Acid, Biotin, and Choline. Washington, DC: National Academies Press (US) National Academy of Sciences (1998).
5. Nielsen MJ, Rasmussen MR, Andersen CB, Nexo E, Moestrup SK. Vitamin B12 transport from food to the body's cells–a sophisticated, multistep pathway. Nat Rev Gastroenterol Hepatol. (2012) 9:345–54. doi: 10.1038/nrgastro.2012.76
6. Miller JW, Green R. Vitamin B12. In Zempleni J, editor. Handbook of Vitamins. Boca Raton, FL: Taylor & Francis (2014). p. 447–89.
7. Stabler SP. Clinical practice. Vitamin B12 deficiency. N Engl J Med. (2013) 368:149–60. doi: 10.1056/NEJMcp1113996
8. Nexo E, Hoffmann-Lucke E. Holotranscobalamin, a marker of vitamin B-12 status: analytical aspects and clinical utility. Am J Clin Nutr. (2011) 94:359S−65S. doi: 10.3945/ajcn.111.013458
9. Bauman WA, Shaw S, Jayatilleke E, Spungen AM, Herbert V. Increased intake of calcium reverses vitamin B12 malabsorption induced by metformin. Diabetes Care. (2000) 23:1227–31. doi: 10.2337/diacare.23.9.1227
10. Fu X, Francisco C, Pattengale P, O'Gorman MR, Mitchell WG. An adolescent with increased plasma methylmalonic acid and total homocysteine. Clin Chem. (2017) 63:1069–72. doi: 10.1373/clinchem.2016.260695
11. Green R, Allen LH, Bjorke-Monsen AL, Brito A, Gueant JL, Miller JW, et al. Vitamin B12 deficiency. Nat Rev Dis Primers. (2017) 3:17040. doi: 10.1038/nrdp.2017.41
12. Miller JW, Garrod MG, Rockwood AL, Kushnir MM, Allen LH, Haan MN, et al. Measurement of total vitamin B12 and holotranscobalamin, singly and in combination, in screening for metabolic vitamin B12 deficiency. Clin Chem. (2006) 52:278–85. doi: 10.1373/clinchem.2005.061382
13. Clarke R, Sherliker P, Hin H, Nexo E, Hvas AM, Schneede J, et al. Detection of vitamin B12 deficiency in older people by measuring vitamin B12 or the active fraction of vitamin B12, holotranscobalamin. Clin Chem. (2007) 53:963–70. doi: 10.1373/clinchem.2006.080382
14. Heil SG, de Jonge R, de Rotte MC, van Wijnen M, Heiner-Fokkema RM, Kobold AC, et al. Screening for metabolic vitamin B12 deficiency by holotranscobalamin in patients suspected of vitamin B12 deficiency: a multicentre study. Ann Clin Biochem. (2012) 49:184–9. doi: 10.1258/acb.2011.011039
15. Hannibal L, Lysne V, Bjorke-Monsen AL, Behringer S, Grunert SC, Spiekerkoetter U, et al. Biomarkers and algorithms for the diagnosis of vitamin B12 Deficiency. Front Mol Biosci. (2016) 3:27. doi: 10.3389/fmolb.2016.00027
16. EFSA Panel on Dietetic Products Nutrition and Allergies (NDA). Scientific opinion on dietary reference values for cobalamin (vitamin B12). EFSA J. (2015) 13:4150. doi: 10.2903/j.efsa.2015.4150
17. Berlin H, Berlin R, Brante G. Oral treatment of pernicious anemia with high doses of vitamin B12 without intrinsic factor. Acta Med Scand. (1968) 184:247–58. doi: 10.1111/j.0954-6820.1968.tb02452.x
18. Greibe E, Mahalle N, Bhide V, Heegaard CW, Naik S, Nexo E. Increase in circulating holotranscobalamin after oral administration of cyanocobalamin or hydroxocobalamin in healthy adults with low and normal cobalamin status. Eur J Nutr. (2018) 57:2847–55. doi: 10.1007/s00394-017-1553-5
19. Bor MV, Lydeking-Olsen E, Moller J, Nexo E. A daily intake of approximately 6 microg vitamin B-12 appears to saturate all the vitamin B-12-related variables in Danish postmenopausal women. Am J Clin Nutr. (2006) 83:52–8. doi: 10.1093/ajcn/83.1.52
20. Bor MV, von Castel-Roberts KM, Kauwell GP, Stabler SP, Allen RH, Maneval DR, et al. Daily intake of 4 to 7 microg dietary vitamin B-12 is associated with steady concentrations of vitamin B-12-related biomarkers in a healthy young population. Am J Clin Nutr. (2010) 91:571–7. doi: 10.3945/ajcn.2009.28082
21. Dullemeijer C, Souverein OW, Doets EL, van der Voet H, van Wijngaarden JP, de Boer WJ, et al. Systematic review with dose-response meta-analyses between vitamin B-12 intake and European Micronutrient Recommendations Aligned's prioritized biomarkers of vitamin B-12 including randomized controlled trials and observational studies in adults and elderly persons. Am J Clin Nutr. (2013) 97:390–402. doi: 10.3945/ajcn.112.033951
22. van Asselt DZ, de Groot LC, van Staveren WA, Blom HJ, Wevers RA, Biemond I, et al. Role of cobalamin intake and atrophic gastritis in mild cobalamin deficiency in older Dutch subjects. Am J Clin Nutr. (1998) 68:328–34. doi: 10.1093/ajcn/68.2.328
23. Bermejo LM, Aparicio A, Andres P, Lopez-Sobaler AM, Ortega RM. The influence of fruit and vegetable intake on the nutritional status and plasma homocysteine levels of institutionalised elderly people. Public Health Nutr. (2007) 10:266–72. doi: 10.1017/S1368980007246580
24. Howard JM, Azen C, Jacobsen DW, Green R, Carmel R. Dietary intake of cobalamin in elderly people who have abnormal serum cobalamin, methylmalonic acid and homocysteine levels. Eur J Clin Nutr. (1998) 52:582–7. doi: 10.1038/sj.ejcn.1600610
25. Andres E, Affenberger S, Vinzio S, Kurtz JE, Noel E, Kaltenbach G, et al. Food-cobalamin malabsorption in elderly patients: clinical manifestations and treatment. Am J Med. (2005) 118:1154–9. doi: 10.1016/j.amjmed.2005.02.026
26. Henoun Loukili N, Noel E, Ben Abdelghani M, Locatelli F, Blickle JF, Andres E. Cobalamin deficiency due to non-immune atrophic gastritis in elderly patients. A report of 25 cases. J Nutr Health Aging. (2005) 9:462.
27. Andres E, Goichot B, Schlienger JL. Food cobalamin malabsorption: a usual cause of vitamin B12 deficiency. Arch Intern Med. (2000) 160:2061–2. doi: 10.1001/archinte.160.13.2061
28. Carmel R, Aurangzeb I, Qian D. Associations of food-cobalamin malabsorption with ethnic origin, age, Helicobacter pylori infection, and serum markers of gastritis. Am J Gastroenterol. (2001) 96:63–70. doi: 10.1111/j.1572-0241.2001.03453.x
29. Dagnelie PC, van Staveren WA, Vergote FJ, Dingjan PG, van den Berg H, Hautvast JG. Increased risk of vitamin B-12 and iron deficiency in infants on macrobiotic diets. Am J Clin Nutr. (1989) 50:818–24. doi: 10.1093/ajcn/50.4.818
30. van Dusseldorp M, Schneede J, Refsum H, Ueland PM, Thomas CM, de Boer E, et al. Risk of persistent cobalamin deficiency in adolescents fed a macrobiotic diet in early life. Am J Clin Nutr. (1999) 69:664–71. doi: 10.1093/ajcn/69.4.664
31. Gibson S. Micronutrient intakes, micronutrient status and lipid profiles among young people consuming different amounts of breakfast cereals: further analysis of data from the National Diet and Nutrition Survey of Young People aged 4 to 18 years. Public Health Nutr. (2003) 6:815–20. doi: 10.1079/PHN2003493
32. Villamor E, Mora-Plazas M, Forero Y, Lopez-Arana S, Baylin A. Vitamin B-12 status is associated with socioeconomic level and adherence to an animal food dietary pattern in Colombian school children. J Nutr. (2008) 138:1391–8. doi: 10.1093/jn/138.7.1391
33. Hay G, Trygg K, Whitelaw A, Johnston C, Refsum H. Folate and cobalamin status in relation to diet in healthy 2-y-old children. Am J Clin Nutr. (2011) 93:727–35. doi: 10.3945/ajcn.110.003426
34. Christian AM, Krishnaveni GV, Kehoe SH, Veena SR, Khanum R, Marley-Zagar E, et al. Contribution of food sources to the vitamin B12 status of South Indian children from a birth cohort recruited in the city of Mysore. Public Health Nutr. (2015) 18:596–609. doi: 10.1017/S1368980014000974
35. Manios Y, Moschonis G, Dekkers R, Mavrogianni C, Grammatikaki E, van den Heuvel E. Vitamin B2, vitamin B12 and total homocysteine status in children and their associations with dietary intake of B-vitamins from different food groups: the healthy growth study. Eur J Nutr. (2017) 56:321–31. doi: 10.1007/s00394-015-1082-z
36. Denissen KFM, Heil SG, Eussen S, Heeskens JPJ, Thijs C, Mommers M, et al. Intakes of vitamin B-12 from dairy food, meat, and fish and shellfish are independently and positively associated with vitamin b-12 biomarker status in pregnant dutch women. J Nutr. (2019) 149:131–8. doi: 10.1093/jn/nxy233
37. Mennen LI, de Courcy GP, Guilland JC, Ducros V, Bertrais S, Nicolas JP, et al. Homocysteine, cardiovascular disease risk factors, and habitual diet in the French Supplementation with Antioxidant Vitamins and Minerals Study. Am J Clin Nutr. (2002) 76:1279–89. doi: 10.1093/ajcn/76.6.1279
38. Gao X, Yao M, McCrory MA, Ma G, Li Y, Roberts SB, et al. Dietary pattern is associated with homocysteine and B vitamin status in an urban Chinese population. J Nutr. (2003) 133:3636–42. doi: 10.1093/jn/133.11.3636
39. Nagata C, Shimizu H, Takami R, Hayashi M, Takeda N, Yasuda K. Soy product intake is inversely associated with serum homocysteine level in premenopausal Japanese women. J Nutr. (2003) 133:797–800. doi: 10.1093/jn/133.3.797
40. Koebnick C, Garcia AL, Dagnelie PC, Strassner C, Lindemans J, Katz N, et al. Long-term consumption of a raw food diet is associated with favorable serum LDL cholesterol and triglycerides but also with elevated plasma homocysteine and low serum HDL cholesterol in humans. J Nutr. (2005) 135:2372–8. doi: 10.1093/jn/135.10.2372
41. Song WO, Chung CE, Chun OK, Cho S. Serum homocysteine concentration of US adults associated with fortified cereal consumption. J Am Coll Nutr. (2005) 24:503–9. doi: 10.1080/07315724.2005.10719496
42. Hao L, Ma J, Zhu J, Stampfer MJ, Tian Y, Willett WC, et al. Vitamin B-12 deficiency is prevalent in 35- to 64-year-old Chinese adults. J Nutr. (2007) 137:1278–85. doi: 10.1093/jn/137.5.1278
43. Vogiatzoglou A, Smith AD, Nurk E, Berstad P, Drevon CA, Ueland PM, et al. Dietary sources of vitamin B-12 and their association with plasma vitamin B-12 concentrations in the general population: the Hordaland Homocysteine study. Am J Clin Nutr. (2009) 89:1078–87. doi: 10.3945/ajcn.2008.26598
44. Yakub M, Iqbal MP, Iqbal R. Dietary patterns are associated with hyperhomocysteinemia in an urban Pakistani population. J Nutr. (2010) 140:1261–6. doi: 10.3945/jn.109.120477
45. Murakami K, Sasaki S, Uenishi K. Higher intake of vitamin B-6 and dairy products and lower intake of green and oolong tea are independently associated with lower serum homocysteine concentration in young Japanese women. Nutr Res. (2013) 33:653–60. doi: 10.1016/j.nutres.2013.05.010
46. Kwan LL, Bermudez OI, Tucker KL. Low vitamin B-12 intake and status are more prevalent in hispanic older adults of caribbean origin than in neighborhood-matched non-hispanic whites. J Nutr. (2002) 132:2059–64. doi: 10.1093/jn/132.7.2059
47. Lasheras C, Huerta JM, Gonzalez S, Prada M, Braga S, Fernandez S, et al. Diet score is associated with plasma homocysteine in a healthy institutionalised elderly population. Nutr Metab Cardiovasc Dis. (2003) 13:384–90. doi: 10.1016/S0939-4753(03)80008-X
48. Ledikwe JH, Smiciklas-Wright H, Mitchell DC, Miller CK, Jensen GL. Dietary patterns of rural older adults are associated with weight and nutritional status. J Am Geriatr Soc. (2004) 52:589–95. doi: 10.1111/j.1532-5415.2004.52167.x
49. Brouwer-Brolsma EM, Dhonukshe-Rutten RA, van Wijngaarden JP, Zwaluw NL, Velde N, de Groot LC. Dietary sources of vitamin B-12 and their association with vitamin B-12 status markers in healthy older adults in the B-PROOF study. Nutrients. (2015) 7:7781–97. doi: 10.3390/nu7095364
50. Larsson CL, Johansson GK. Dietary intake and nutritional status of young vegans and omnivores in Sweden. Am J Clin Nutr. (2002) 76:100–6. doi: 10.1093/ajcn/76.1.100
51. Damayanti D, Jaceldo-Siegl K, Beeson WL, Fraser G, Oda K, Haddad EH. Foods and Supplements Associated with Vitamin B12 Biomarkers among Vegetarian and Non-Vegetarian Participants of the Adventist Health Study-2 (AHS-2) Calibration Study. Nutrients. (2018) 10:722. doi: 10.3390/nu10060722
52. Alles B, Baudry J, Mejean C, Touvier M, Peneau S, Hercberg S, et al. Comparison of sociodemographic and nutritional characteristics between self-reported vegetarians, vegans, and meat-eaters from the nutrinet-sante study. Nutrients. (2017) 9:1023. doi: 10.3390/nu9091023
53. Davey GK, Spencer EA, Appleby PN, Allen NE, Knox KH, Key TJ. EPIC-Oxford: lifestyle characteristics and nutrient intakes in a cohort of 33 883 meat-eaters and 31 546 non meat-eaters in the UK. Public Health Nutr. (2003) 6:259–69. doi: 10.1079/PHN2002430
54. Majchrzak D, Singer I, Manner M, Rust P, Genser D, Wagner KH, et al. B-vitamin status and concentrations of homocysteine in Austrian omnivores, vegetarians and vegans. Ann Nutr Metab. (2006) 50:485–91. doi: 10.1159/000095828
55. Schupbach R, Wegmuller R, Berguerand C, Bui M, Herter-Aeberli I. Micronutrient status and intake in omnivores, vegetarians and vegans in Switzerland. Eur J Nutr. (2017) 56:283–93. doi: 10.1007/s00394-015-1079-7
56. Gilsing AM, Crowe FL, Lloyd-Wright Z, Sanders TA, Appleby PN, Allen NE, et al. Serum concentrations of vitamin B12 and folate in British male omnivores, vegetarians and vegans: results from a cross-sectional analysis of the EPIC-Oxford cohort study. Eur J Clin Nutr. (2010) 64:933–9. doi: 10.1038/ejcn.2010.142
57. Carmel R. Nutritional anemias and the elderly. Semin Hematol. (2008) 45:225–34. doi: 10.1053/j.seminhematol.2008.07.009
58. Adams JF. Pregnancy and Addisonian pernicious anaemia. Scott Med J. (1958) 3:21–5. doi: 10.1177/003693305800300103
59. Armstrong BK, Davis RE, Martin JD, Woodliff HJ. Pregnancy and untreated Addisonian pernicious anemia. Br Med J. (1968) 4:158–9. doi: 10.1136/bmj.4.5624.158
60. Hall M, Davidson RJ. Prophylactic folic acid in women with pernicious anaemia pregnant after periods of infertility. J Clin Pathol. (1968) 21:599–602. doi: 10.1136/jcp.21.5.599
61. Afman LA, Van Der Put NM, Thomas CM, Trijbels JM, Blom HJ. Reduced vitamin B12 binding by transcobalamin II increases the risk of neural tube defects. QJM. (2001) 94:159–66. doi: 10.1093/qjmed/94.3.159
62. Gaber KR, Farag MK, Soliman SE, El-Bassyouni HT, El-Kamah G. Maternal vitamin B12 and the risk of fetal neural tube defects in Egyptian patients. Clin Lab. (2007) 53:69–75.
63. Molloy AM. Should vitamin B12 status be considered in assessing risk of neural tube defects? Ann N Y Acad Sci. (2018) 1414:109–25. doi: 10.1111/nyas.13574
64. Zhang T, Xin R, Gu X, Wang F, Pei L, Lin L, et al. Maternal serum vitamin B12, folate and homocysteine and the risk of neural tube defects in the offspring in a high-risk area of China. Public Health Nutr. (2009) 12:680–6. doi: 10.1017/S1368980008002735
65. Ray JG, Wyatt PR, Thompson MD, Vermeulen MJ, Meier C, Wong PY, et al. Vitamin B12 and the risk of neural tube defects in a folic-acid-fortified population. Epidemiology. (2007) 18:362–6. doi: 10.1097/01.ede.0000257063.77411.e9
66. Varsi K, Ueland PM, Torsvik IK, Bjorke-Monsen AL. Maternal serum cobalamin at 18 weeks of pregnancy predicts infant cobalamin status at 6 months-a prospective, observational study. J Nutr. (2018) 148:738–45. doi: 10.1093/jn/nxy028
67. Chandyo RK, Ulak M, Kvestad I, Hysing M, Shrestha M, Ranjitkar S, et al. Cobalamin and Folate Status among Breastfed Infants in Bhaktapur, Nepal. Nutrients. (2018) 10:639. doi: 10.3390/nu10050639
68. Guez S, Chiarelli G, Menni F, Salera S, Principi N, Esposito S. Severe vitamin B12 deficiency in an exclusively breastfed 5-month-old Italian infant born to a mother receiving multivitamin supplementation during pregnancy. BMC Pediatr. (2012) 12:85. doi: 10.1186/1471-2431-12-85
69. Kvestad I, Taneja S, Kumar T, Hysing M, Refsum H, Yajnik CS, et al. Vitamin B12 and folic acid improve gross motor and problem-solving skills in young north indian children: a randomized placebo-controlled trial. PLoS ONE. (2015) 10:e0129915. doi: 10.1371/journal.pone.0129915
70. Pasricha SR, Shet AS, Black JF, Sudarshan H, Prashanth NS, Biggs BA. Vitamin B-12, folate, iron, and vitamin A concentrations in rural Indian children are associated with continued breastfeeding, complementary diet, and maternal nutrition. Am J Clin Nutr. (2011) 94:1358–70. doi: 10.3945/ajcn.111.018580
71. McLean ED, Allen LH, Neumann CG, Peerson JM, Siekmann JH, Murphy SP, et al. Low plasma vitamin B-12 in Kenyan school children is highly prevalent and improved by supplemental animal source foods. J Nutr. (2007) 137:676–82. doi: 10.1093/jn/137.3.676
72. Rogers LM, Boy E, Miller JW, Green R, Sabel JC, Allen LH. High prevalence of cobalamin deficiency in Guatemalan schoolchildren: associations with low plasma holotranscobalamin II and elevated serum methylmalonic acid and plasma homocysteine concentrations. Am J Clin Nutr. (2003) 77:433–40. doi: 10.1093/ajcn/77.2.433
73. Siekmann JH, Allen LH, Bwibo NO, Demment MW, Murphy SP, Neumann CG. Kenyan school children have multiple micronutrient deficiencies, but increased plasma vitamin B-12 is the only detectable micronutrient response to meat or milk supplementation. J Nutr. (2003) 133:3972s−80s. doi: 10.1093/jn/133.11.3972S
74. Strand TA, Ulak M, Kvestad I, Henjum S, Ulvik A, Shrestha M, et al. Maternal and infant vitamin B12 status during infancy predict linear growth at 5 years. Pediatr Res. (2018) 84:611–8. doi: 10.1038/s41390-018-0072-2
75. Strand TA, Taneja S, Ueland PM, Refsum H, Bahl R, Schneede J, et al. Cobalamin and folate status predicts mental development scores in North Indian children 12–18 mo of age. Am J Clin Nutr. (2013) 97:310–7. doi: 10.3945/ajcn.111.032268
76. Kumar T, Taneja S, Sachdev HPS, Refsum H, Yajnik CS, Bhandari N, et al. Supplementation of vitamin B12 or folic acid on hemoglobin concentration in children 6–36 months of age: A randomized placebo controlled trial. Clin Nutr. (2017) 36:986–91. doi: 10.1016/j.clnu.2016.07.002
77. Winje BA, Kvestad I, Krishnamachari S, Manji K, Taneja S, Bellinger DC, et al. Does early vitamin B12 supplementation improve neurodevelopment and cognitive function in childhood and into school age: a study protocol for extended follow-ups from randomised controlled trials in India and Tanzania. BMJ Open. (2018) 8:e018962. doi: 10.1136/bmjopen-2017-018962
78. Porter K, Hoey L, Hughes CF, Ward M, McNulty H. Causes, consequences and public health implications of low B-vitamin status in ageing. Nutrients. (2016) 8:725. doi: 10.3390/nu8110725
79. van de Lagemaat EE, de Groot L, van den Heuvel E. Vitamin B12 in relation to oxidative stress: a systematic review. Nutrients. (2019) 11:482. doi: 10.3390/nu11020482
80. Vogiatzoglou A, Refsum H, Johnston C, Smith SM, Bradley KM, de Jager C, et al. Vitamin B12 status and rate of brain volume loss in community-dwelling elderly. Neurology. (2008) 71:826–32. doi: 10.1212/01.wnl.0000325581.26991.f2
81. Smith AD, Refsum H. Vitamin B-12 and cognition in the elderly. Am J Clin Nutr. (2009) 89:707s−11s. doi: 10.3945/ajcn.2008.26947D
82. van der Zwaluw NL, Dhonukshe-Rutten RA, van Wijngaarden JP, Brouwer-Brolsma EM, van de Rest O, In 't Veld PH, et al. Results of 2-year vitamin B treatment on cognitive performance: secondary data from an RCT. Neurology. (2014) 83:2158–66. doi: 10.1212/WNL.0000000000001050
83. Douaud G, Refsum H, de Jager CA, Jacoby R, Nichols TE, Smith SM, et al. Preventing Alzheimer's disease-related gray matter atrophy by B-vitamin treatment. Proc Natl Acad Sci USA. (2013) 110:9523–8. doi: 10.1073/pnas.1301816110
84. de Koning EJ, van der Zwaluw NL, van Wijngaarden JP, Sohl E, Brouwer-Brolsma EM, van Marwijk HW, et al. Effects of two-year vitamin B12 and folic acid supplementation on depressive symptoms and quality of life in older adults with elevated homocysteine concentrations: additional results from the B-PROOF study, an RCT. Nutrients. (2016) 8:748. doi: 10.3390/nu8110748
85. Lewerin C, Nilsson-Ehle H, Jacobsson S, Johansson H, Sundh V, Karlsson MK, et al. Low holotranscobalamin and cobalamins predict incident fractures in elderly men: the MrOS Sweden. Osteoporos Int. (2014) 25:131–40. doi: 10.1007/s00198-013-2527-y
86. Bailey RL, Looker AC, Lu Z, Fan R, Eicher-Miller HA, Fakhouri TH, et al. B-vitamin status and bone mineral density and risk of lumbar osteoporosis in older females in the United States. Am J Clin Nutr. (2015) 102:687–94. doi: 10.3945/ajcn.115.108787
87. Garcia Lopez M, Baron JA, Omsland TK, Sogaard AJ, Meyer HE. Homocysteine-lowering treatment and the risk of fracture: secondary analysis of a randomized controlled trial and an updated meta-analysis. JBMR Plus. (2018) 2:295–303. doi: 10.1002/jbm4.10045
88. Ramirez-Velez R, Correa-Bautista JE, Martinez-Torres J, Meneses-Echavez JF, Lobelo F. Vitamin B12 concentrations in pregnant Colombian women: analysis of nationwide data 2010. BMC Preg Childbirth. (2016) 16:26. doi: 10.1186/s12884-016-0820-4
89. Barnabe A, Alessio AC, Bittar LF, de Moraes Mazetto B, Bicudo AM, de Paula EV, et al. Folate, vitamin B12 and Homocysteine status in the post-folic acid fortification era in different subgroups of the Brazilian population attended to at a public health care center. Nutr J. (2015) 14:19. doi: 10.1186/s12937-015-0006-3
90. Finkelstein JL, Kurpad AV, Thomas T, Srinivasan K, Duggan C. Vitamin B12 status in pregnant women and their infants in South India. Eur J Clin Nutr. (2017) 71:1046–53. doi: 10.1038/ejcn.2017.29
91. Ramirez-Velez R, Correa-Bautista JE, Triana-Reina HR, Gonzalez-Jimenez E, Schmidt-RioValle J, Gonzalez-Ruiz K. Use of dietary supplements by pregnant women in Colombia. BMC Preg Childbirth. (2018) 18:117. doi: 10.1186/s12884-018-1758-5
92. Gadgil M, Joshi K, Pandit A, Otiv S, Joshi R, Brenna JT, et al. Imbalance of folic acid and vitamin B12 is associated with birth outcome: an Indian pregnant women study. Eur J Clin Nutr. (2014) 68:726–9. doi: 10.1038/ejcn.2013.289
93. Dwarkanath P, Barzilay JR, Thomas T, Thomas A, Bhat S, Kurpad AV. High folate and low vitamin B-12 intakes during pregnancy are associated with small-for-gestational age infants in South Indian women: a prospective observational cohort study. Am J Clin Nutr. (2013) 98:1450–8. doi: 10.3945/ajcn.112.056382
94. Yajnik CS, Deshpande SS, Jackson AA, Refsum H, Rao S, Fisher DJ, et al. Vitamin B12 and folate concentrations during pregnancy and insulin resistance in the offspring: the Pune maternal nutrition study. Diabetologia. (2008) 51:29–38. doi: 10.1007/s00125-007-0793-y
95. Krishnaveni GV, Veena SR, Karat SC, Yajnik CS, Fall CH. Association between maternal folate concentrations during pregnancy and insulin resistance in Indian children. Diabetologia. (2014) 57:110–21. doi: 10.1007/s00125-013-3086-7
96. Knight BA, Shields BM, Brook A, Hill A, Bhat DS, Hattersley AT, et al. Lower circulating B12 is associated with higher obesity and insulin resistance during pregnancy in a non-diabetic white british population. PLoS ONE. (2015) 10:e0135268. doi: 10.1371/journal.pone.0135268
97. Sukumar N, Venkataraman H, Wilson S, Goljan I, Selvamoni S, Patel V, et al. Vitamin B12 Status among Pregnant Women in the UK and Its Association with Obesity and Gestational Diabetes. Nutrients. (2016) 8:768. doi: 10.3390/nu8120768
98. Sole-Navais P, Salat-Batlle J, Cavalle-Busquets P, Fernandez-Ballart J, Ueland PM, Ballesteros M, et al. Early pregnancy folate-cobalamin interactions and their effects on cobalamin status and hematologic variables throughout pregnancy. Am J Clin Nutr. (2018) 107:173–82. doi: 10.1093/ajcn/nqx041
99. Chow BF, Rosen DA, Lang CA. Vitamin B12 serum levels and diabetic retinopathy. Proc Soc Exp Biol Med. (1954) 87:38–9. doi: 10.3181/00379727-87-21277
100. Halsted JA, Carroll J, Rubert S. Serum and tissue concentration of vitamin B12 in certain pathologic states. N Engl J Med. (1959) 260:575–80. doi: 10.1056/NEJM195903192601202
102. McMahon GM, Hwang SJ, Tanner RM, Jacques PF, Selhub J, Muntner P, et al. The association between vitamin B12, albuminuria and reduced kidney function: an observational cohort study. BMC Nephrol. (2015) 16:7. doi: 10.1186/1471-2369-16-7
103. Brah S, Chiche L, Mancini J, Meunier B, Arlet JB. Characteristics of patients admitted to internal medicine departments with high serum cobalamin levels: results from a prospective cohort study. Eur J Intern Med. (2014) 25:e57–58. doi: 10.1016/j.ejim.2014.01.014
104. Carmel R, Vasireddy H, Aurangzeb I, George K. High serum cobalamin levels in the clinical setting–clinical associations and holo-transcobalamin changes. Clin Lab Haematol. (2001) 23:365–71. doi: 10.1046/j.1365-2257.2001.00134.x
105. Arendt JF, Farkas DK, Pedersen L, Nexo E, Sorensen HT. Elevated plasma vitamin B12 levels and cancer prognosis: a population-based cohort study. Cancer Epidemiol. (2016) 40:158–165. doi: 10.1016/j.canep.2015.12.007
106. Mendonca N, Jagger C, Granic A, Martin-Ruiz C, Mathers JC, Seal CJ, et al. Elevated total homocysteine in all participants and plasma vitamin B12 concentrations in women are associated with all-cause and cardiovascular mortality in the very old: the newcastle 85+ study. J Gerontol A Biol Sci Med Sci. (2018) 73:1258–64. doi: 10.1093/gerona/gly035
107. Salles N, Herrmann F, Sieber C, Rapin C. High vitamin B12 level and mortality in elderly inpatients. J Nutr Health Aging. (2008) 12:219–21. doi: 10.1007/BF02982624
108. Geissbuhler P, Mermillod B, Rapin CH. Elevated serum vitamin B12 levels associated with CRP as a predictive factor of mortality in palliative care cancer patients: a prospective study over five years. J Pain Symptom Manage. (2000) 20:93–103. doi: 10.1016/S0885-3924(00)00169-X
109. Arendt JF, Sorensen HT, Horsfall LJ, Petersen I. Elevated vitamin B12 levels and cancer risk in UK primary care: a THIN database cohort study. Cancer Epidemiol Biomarkers Prev. (2019) 28:1136. doi: 10.1158/1055-9965.EPI-17-1136
Keywords: vitamin B12 (cobalamin), intake, animal food products, health, infants, pregnancy, elderly
Citation: Obeid R, Heil SG, Verhoeven MMA, van den Heuvel EGHM, de Groot LCPGM and Eussen SJPM (2019) Vitamin B12 Intake From Animal Foods, Biomarkers, and Health Aspects. Front. Nutr. 6:93. doi: 10.3389/fnut.2019.00093
Received: 15 March 2019; Accepted: 30 May 2019;
Published: 28 June 2019.
Edited by:
José M. Alvarez-Suarez, University of the Americas, EcuadorReviewed by:
Ella H. Haddad, Loma Linda University, United StatesMona Elena Popa, University of Agronomic Sciences and Veterinary Medicine, Romania
Copyright © 2019 Obeid, Heil, Verhoeven, van den Heuvel, de Groot and Eussen. This is an open-access article distributed under the terms of the Creative Commons Attribution License (CC BY). The use, distribution or reproduction in other forums is permitted, provided the original author(s) and the copyright owner(s) are credited and that the original publication in this journal is cited, in accordance with accepted academic practice. No use, distribution or reproduction is permitted which does not comply with these terms.
*Correspondence: Simone J. P. M. Eussen, simone.eussen@maastrichtuniversity.nl