- 1Department of Internal Medicine, Endocrinology Section, Amsterdam University Medical Centers, Vrije Universiteit Amsterdam, Amsterdam Movement Sciences, Amsterdam, Netherlands
- 2Department of Radiology and Nuclear Medicine, Amsterdam University Medical Centers, Vrije Universiteit Amsterdam, Amsterdam Movement Sciences, Amsterdam, Netherlands
- 3Department of Anesthesiology, Amsterdam University Medical Centers, Vrije Universiteit Amsterdam, Amsterdam, Netherlands
- 4Department of Urology, Amsterdam University Medical Centers, Vrije Universiteit Amsterdam, Amsterdam, Netherlands
- 5Regeneron Pharmaceuticals, Inc., Tarrytown, NY, United States
Background: Fibrodysplasia Ossificans Progressiva (FOP) is a rare, genetic disease in which heterotopic bone is formed in muscles, tendons and ligaments throughout the body. Disease progression is variable over time and between individuals. 18F-fluoride uptake in newly formed bone can be evaluated using [18F]NaF (i.e., sodiumfluoride) PET/CT, identifying active areas of bone formation in FOP. The purpose of this study was to assess the performance of various semi-quantitative methods with full kinetic analysis.
Results: Seven patients (age range: 20–31 years) with FOP underwent dynamic [18F]NaF scans at baseline and after one year. [18F]NaF uptake was measured in aorta descendens, vertebrae, heterotopic bone lesions and metabolically active regions on PET, and quantified using nonlinear regression (NLR) analysis together with standardized uptake value (SUV) and target-to-blood ratio (TBR). SUV was on measured the 40–45 min frame of the dynamic sequence (SUV40–45) and on the subsequent static sweep (SUVStatic). Correlations between and SUV40–45 and NLR-derived Ki were comparable when normalized to body weight (r = 0.81, 95% CI 0.64–0.90), lean body mass (r = 0.79, 95% CI 0.61–0.89) and body surface area (r = 0.84, 95% CI 0.70–0.92). Correlation between TBR40–45 and NLR-derived Ki (r = 0.92, 95% CI 0.85–0.96) was higher than for SUV40–45. Correlation between TBR40–45 and NLR-derived Ki was similar at baseline and after one year (r = 0.93 and 0.94). The change in TBR40–45 between baseline measurement and after one year correlated best with the change in NLR-derived Ki in the PET-active lesions (r = 0.87).
Conclusion: The present data supports the use of TBR for assessing fluoride uptake in PET-active lesions in FOP.
Clinical trial registration: Sub-study of the Lumina-1 trial (clinicaltrials.gov, NCT03188666, registered 13-06-2017).
Introduction
Fibrodysplasia ossificans progressiva (FOP) is a rare genetic disease affecting 0.88 per million individuals worldwide (1). Slowly but steadily, bone is formed in connective tissues such as muscles, ligaments and tendons throughout the body, leading to progressive immobility and ultimately an early death (2). In 2006, it was discovered that a single nucleotide substitution encoding the activin receptor type 1 (ACVR1)/activin receptor-like kinase 2 (ALK2) was the cause of FOP, and further genetic analyses revealed that nearly 97% of FOP cases are caused by the same mutation (c.617G>A, R206H), although other mutations have been described (3). Despite the fact that most patients share the same mutation, severity and rate of disease progression vary greatly and can be difficult to predict (4).
Heterotopic ossification in FOP is thought to take place in two separate manners. Classically, heterotopic bone in FOP is formed during so-called “flare-ups”, i.e., acute episodes of painful soft tissue swelling triggered by a minor trauma or an infection, but sometimes also occurring spontaneously (5). The initial local inflammatory response in muscle tissue is followed by endochondral ossification of the muscles and connective tissues (6). Recently, when analysing a series of MRI and PET/CT scans over time, areas were identified where heterotopic bone was formed without any typical flare-up symptoms, suggesting that a lower grade type of bone formation can also take place in patients with FOP, which is unrelated to the flare-ups (7). This was also confirmed in an ongoing natural history study in FOP, in which it was noted that nearly half of the patients reported instances of new heterotopic ossification (HO) without having experienced a flare-up (8). Treatment for FOP focuses on preventing soft tissue injury that can provoke a flare-up. Anti-inflammatory medication is often used empirically to reduce further bone formation once a flare-up has started, although there is little scientific evidence for this approach. Nevertheless, several therapeutic targets are being explored in multiple ongoing clinical trials, which will hopefully lead to better treatment and management of this devastating disease (9, 10).
Multiple tools have been developed to assess disease activity and progression of FOP. Assessment of joint mobility by goniometer measurements or cumulative scores such as the CAJIS (cumulative analogue joint involvement scale) reflect overall mobility and disease burden of a patient with FOP over time (11), but only moderately (r = 0.57) reflects the amount of heterotopic bone being formed (2). In clinical practice, however, it usually is more common to determine disease activity at the time of assessment for clinical decision making, rather than that it is based on changes over time. Clinical symptoms of a flare-up, such as pain, swelling, erythema and warmth, are non-specific, and it is difficult to predict whether the acute phase of the flare-up will result in HO formation or whether it will resolve itself (2). A multitude of potential biomarkers involved in inflammatory, chondrogenic and osteogenic processes have been investigated in patients with FOP, both during and in the absence of flare-up activity, and although some were markedly elevated in patients with FOP, none have shown to be capable of consistently reflecting disease activity and adequately predicting HO formation (12, 13).
Imaging techniques can be of great value in characterising disease activity and progression in FOP. Computed tomography (CT) scans can measure heterotopic bone volume over time, making it possible to evaluate disease progression. MRI (magnetic resonance imaging) and ultrasonography are able to detect soft tissue oedema associated with the inflammatory stage of HO, but they are less useful for detecting early bone formation and for accurately quantifying bone volume. Positron emission tomography (PET) is emerging as a new promising tool in FOP, as [18F]sodium fluoride [18F]NaF) PET can detect bone formation before it is visible on conventional CT (14).
Several methods exist to quantify [18F]NaF kinetics. A compartment model together with non-linear regression (NLR), as proposed by Hawkins et al., is considered to be the most accurate method for quantification of [18F]NaF uptake, but is also the most complex one (15, 16). The standardized uptake value (SUV) provides a simpler method towards assessing [18F]NaF uptake at any given time. SUV is the ratio of the image derived tissue radioactivity concentration divided by the whole body concentration based on the known dose of injected radioactivity, normalised to an anthropomorphic factor such body weight (BW). Though SUV is relatively easy to measure, it is prone to bias due to changes in blood flow and cannot assess the full range of [18F]NaF kinetics. Use of SUV to measure [18F]NaF uptake has already been validated against full kinetic NLR analysis in normotopic bone formation (17). Whether this simpler method can also be used to evaluate heterotopic bone formation and metabolism in FOP is unknown. Uptake in FOP may be markedly different from other metabolic diseases, given the genetic mutation interfering in the osteogenic pathways. In addition, drug therapies aimed at altering these pathways as part of a treatment for FOP may affect the rate of bone metabolism and, therefore, [18F]NaF kinetics even further. Potentially even more problematic, is that instead of altering bone metabolism, these drugs may also alter perfusion which, in turn, could give misleading results. For example, if metabolism does not change after therapy, but blood flow changes with 50%, SUV will also show an (erroneous) change of about 50%.
To assess various quantitative parameters reflecting [18F]NaF uptake in FOP, dynamic [18F]NaF PET/CT scans were performed in seven FOP patients who participated in the LUMINA-1 trial, evaluating [18F]NaF kinetics at baseline (T0) and after one year (T1) when all patients were receiving the trial drug garetosmab (an anti-activin A antibody).
Material and methods
Medical ethics
Ethical consent for this study was obtained from the Medical Ethics Review Committee of the VU University Medical Center. Patients were asked to participate in this study, and all patients provided written consent after being fully informed about the study purpose and any potential risks.
Patient inclusion
Seven adults with the classic R206H FOP mutation underwent a dynamic [18F]NaF PET scan as part of the baseline measurements of the LUMINA-1 study. One year later, a second dynamic [18F]NaF PET scan was performed, at which point patients had received garetosmab for either 6 or 12 months.
PET/CT data acquisition
All dynamic [18F]NaF PET scans were performed at the Amsterdam UMC, location VUmc. Scans were acquired using the Ingenuity PET/CT scanner (Philips Healthcare, Cleveland, OH, USA), which records planes over an axial field of view of 18.4 cm with voxels of 4 × 4 × 4 mm. First, a low dose CT scan was performed for attenuation and localisation purposes. Next, a bolus injection of 102 ± 3 (mean ± SD) MBq [18F]NaF was administered in the cephalic vein. Simultaneously, a 45 min dynamic (listmode) PET scan was started over the thoracic area, followed by a whole body scanning sweep (2 min per bed position, 10 bed positions). Venous blood samples were drawn at 10, 20, 30 and 45 min after injection. The dynamic acquisition was reconstructed in 36 consecutive time frames: 6 × 5, 6 × 10, 3 × 20, 5 × 30, 5 × 60, 8 × 150 and 3 × 300 s. The Philips Ingenuity system uses an iterative reconstruction algorithm, 3D-row action maximum likelihood algorithm (RAMLA), for the dynamic scans and rotationally symmetric volume elements ordered subsets time-of-flight (blob-os-tf) for the whole body scans (18). The combined CT and PET scans resulted in an estimated radiation dose of 4.7 mSv.
Regional assessments
For the dynamic scans, the same volumes of interest (VOIs) were delineated at baseline and one year later by one reviewer (RDR). Independently, a second reviewer (BT, musculoskeletal radiologist) randomly segmented a third of the manually defined VOIs to assess inter-observer variability. Three VOIs of heterotopic bone were defined manually on the CT images, maintaining a cut-off of 80 Hounsfield units (HU) to distinguish between heterotopic bone and soft tissue, in line with previous studies (7). Based on an earlier PET/CT study on FOP, PET active lesions were identified using a SUVpeak (average SUV normalised for body weight within a 1 cm3 region of interest centred around the hottest voxel) >8.4 on the 40–45 frame at the end of the dynamic sequence, and then delineating three of these per scan using a semi-automatic tool. The semi-automatic tool included 50% of the SUVpeak in the designated area with adaptation for the local lesion to background contrast. As a reference for muscle tissue, one fixed-size VOI of 7.8 cm3 was defined in the triceps. As a reference for bone tissue 5 axial slices of the 8th thoracic vertebra were included, again maintaining a cut-off of 80 HU to distinguish between bone and soft tissue. An aorta VOI was manually delineated on 5 axial slices in the aorta descendens on the CT images for deriving an image derived input function and calculating target-to-blood ratios.
Kinetic analysis
Analysis of [18F]NaF uptake was performed using the irreversible 2-tissue (2T3k) compartment model with 3 rate constants and an additional parameter for blood volume (Vb), i.e., the preferred model for evaluation of [18F]NaF uptake in bone (17). Based on this model, the net influx rate constant Ki was derived, reflecting the net rate of influx of [18F]NaF into bone. Image derived input functions were generated by projecting the aorta descendens VOIs on all frames. A multi-exponential function was then fitted based on the mean of the whole blood samples obtained at 20, 30 and 45 min. The plasma input function was determined assuming a fixed plasma-to-whole blood ratio of 1.21. The fixed value was based on an in-house unpublished data set for the same tracer from different earlier studies and is in line with plasma-to-whole blood ratios already published (19).
Simplified measures
In addition to full kinetic analysis, 18F uptake was also analysed using simplified methods. SUV normalised for bodyweight (BW), body surface area (BSA) (20) and lean body mass (LBM) (21) were calculated using the final frame of the dynamic scan (40–45 min) and also using the static scan performed 45 min after injection with SUVmean being the mean SUV of an VOI. Target to blood ratio (TBR) was calculated by dividing SUVmean by SUVmean of the aorta. Pearson correlation was used to compare the correlations of SUVmean and TBRmean with the gold standard, NLR-derived Ki, at baseline and after 1 year. The change in uptake between baseline and after 1 year measured by SUVmean and TBRmean was also correlated with the change measured by NLR-derived Ki.
Statistical analysis
IBM SPSS statistics (IBM version 28) was used to perform statistical analyses. Correlations between various uptake values were tested through the Pearsońs correlation test. Inter-observer variability towards determining VOIs was evaluated through the intraclass correlation coefficient. GraphPad Prism 10 (GraphPad Software, San Diego, CA, USA) was used to perform linear interpolation. Solid lines in the graphs represent the mean interpolation lines with dotted lines representing the 95% confidence interval.
Results
Patient characteristics
After inclusion in the LUMINA-1 study, seven patients were scanned at baseline (T0) and after one year (T1) of trial participation. One scan could not be analysed, as no venous samples could be obtained through the venous catheter and, therefore, no calibration of the input function was possible. One scan failed, as a piece of heterotopic bone impinged on the vena basilica during the scan, obstructing the tracer from dispersing until the patient changed position after the scan. One scan could not be analysed due to movement of the patient during the scan, resulting in an unreliable image-derived input function. Thus, eleven scans were available for further analysis, six at baseline and five after one year of trial participation.
Ultimately, six patients (M/F: 2/4, age range: 20–31 years old) were included in these analyses. The CAJIS score (11) at baseline was mean (± SD) 11.3 ± 7.0 and after 1 year 11.3 ± 4.5, indicating that disease progression in all patients had already affected multiple joints (Table 1).
Regional assessment
After manual segmentation of heterotopic bone on the CT images by one reviewer (RDR), a second reviewer (BT, musculoskeletal radiologist) manually segmented 12 of 36 (33.3%) randomly selected HO structures. A comparison of obtained volumes, TBRs and SUVs of the heterotopic bone lesions showed a near-perfect correlation between both observers (intraclass correlation coefficient = 0.99).
Blood kinetics
Radioactivity concentrations of venous blood samples are shown in Figure 1. These samples were used to calibrate the image-derived input function (IDIF). A multi-exponential function was fitted based on the mean of the whole blood samples with exclusion of the venous sample obtained at 10 min. The radioactivity concentration in the whole blood samples showed little intra-individual variability at baseline and after one year, indicating that garetosmab has little effect on tracer clearance.
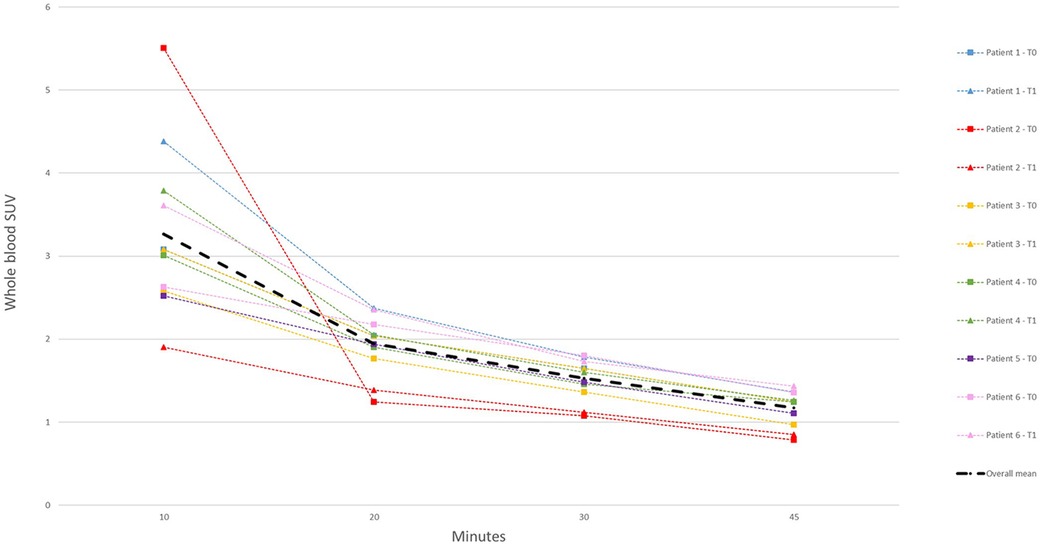
Figure 1 SUV of whole blood venous samples at various time points in all scans. The mean of all samples is represented by the thick line. T0, baseline; T1, after 1 year.
[18F]NaF uptake parameters
The correlation between SUVmean and NLR-derived Ki showed little dependency on the actual body normalisation factor (BW, LBM and BSA) being used, with Pearson's r values varying from 0.79 to 0.87 (Table 2).
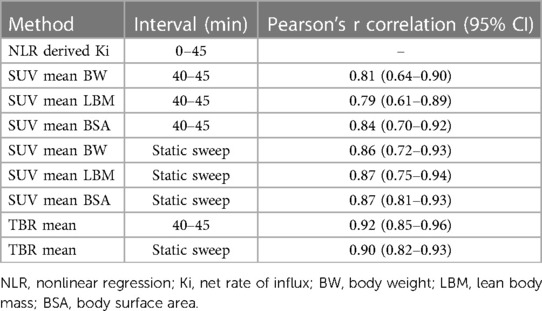
Table 2 Scan intervals and correlation with NLR-derived Ki for all (semi)quantitative parameters at baseline.
At baseline and after one year, SUVmean40–45 correlated with NLR-derived Ki with a Pearson's r of 0.85 and 0.95, respectively, and TBRmean40–45 correlated with a Pearson's r of 0.93 and 0.94 at baseline and after 1 year (Figure 2A). The change in SUVmean40–45 and TBRmean40–45 measured in heterotopic bone, correlated poorly with changes in NLR-derived Ki, with Pearson's of r = 0.42 and 0.57. In contrast, when examining PET-active regions, the change in SUVmean40–45 and TBRmean40–45 had a good correlation with NLR-derived Ki with Pearson's r of 0.82 and 0.91, respectively (Figure 2B). Correlations between SUVmean and TBRmean, derived from the static sweep with NLR-derived Ki were similar. At baseline and after one year, SUVmeanstatic correlated with NLR-derived Ki with a Pearson's r of 0.85 and 0.91, respectively, and TBRmeanstatic correlated with a Pearson's r of 0.88 and 0.93 at baseline and after 1 year (Supplementary Figure S1A). The change in SUVmeanstatic and TBRmeanstatic measured in heterotopic bone did not correlate with the change in the NLR-derived Ki. In contrast, when examining PET-active regions, the change in SUVmeanstatic and TBRmeanstatic had a good correlation with NLR-derived Ki with Pearson's r of 0.92 and 0.93, respectively (Supplementary Figure S1B).
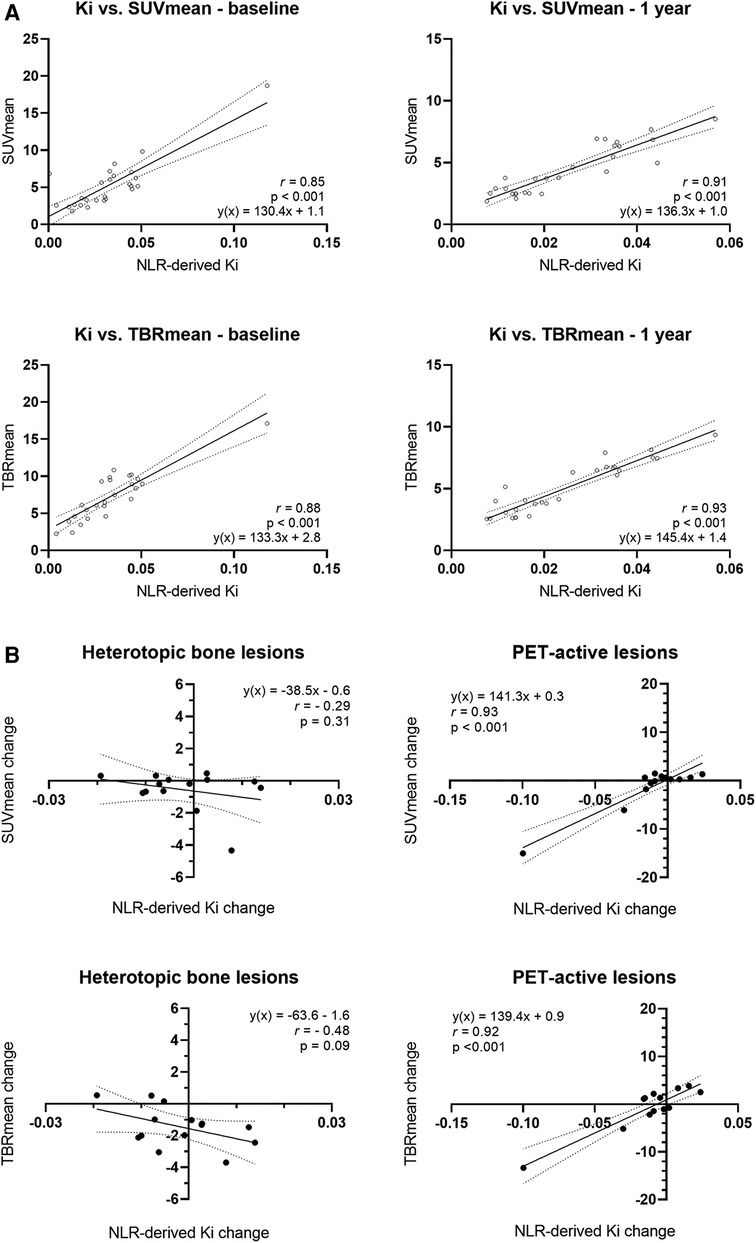
Figure 2 Relationship between F18 uptake as measured through NLR-derived Ki and the simplified parameters SUVmean and TBRmean. (A) Correlation between NLR-derived Ki and simplified parameters derived from the 40–45 min frame (SUVmean40–45 and TBRmean40–45) at baseline and after one year in all areas of interest. (B) Correlation between the change in NLR-derived Ki at baseline and after 1 year and the change SUVmean and TBRmean at baseline and after 1 year. Subanalyses were performed for the heterotopic bone lesions and the PET-active lesions. The solid black line represents the mean agreement between the separate parameters, while the dotted black lines denote the boundaries of the agreement within a 95% confidence interval.
Discussion
Quantification of 18F-fluoride uptake may extend the scope of [18F]NaF PET/CT imaging in FOP from early detection of areas of increased heterotopic bone formation to monitoring disease activity and response to therapeutic interventions. Simplified scanning protocols, without the need for arterial or venous sampling could make it feasible to use [18F]NaF PET/CT scanning for routine assessment of FOP patients. In a cross-sectional analysis, both SUVmean and TBRmean showed a strong correlation with NLR-derived Ki, both before and during treatment with garetosmab. When evaluating the same lesions over time, changes in fluoride uptake as measured by TBRmean best reflected the change in fluoride uptake as measured by NLR-derived Ki.
To the best of our knowledge, this is the first study performing dynamic [18F]NaF scans in patients with FOP. Due to the nature of the disease, there are a few considerations that need to be mentioned. Obtaining an image-derived input function through continuous arterial sampling, the preferred option for dynamic scanning, has an unacceptable high risk of causing heterotopic ossification in FOP. We therefore opted for venous sampling, but were restricted to 1 ml per sample, to do as little harm as possible. In 1 ml of whole blood it is possible to accurately measure the [18F]NaF concentration in MBq/ml, though for conversion to plasma levels a fixed value of 1.21 was assumed based on an in-house unpublished data set for the same tracer from different earlier studies, though other studies have found similar plasma-to whole blood ratios (19). Secondly, most PET/CT scanners currently in use have axial fields of view ranging from 15 to 30 cm, limiting the body area that can be scanned at any given time. By moving the scanner bed during the scan, tracer uptake throughout the body can be visualized, providing a so-called (static) whole body PET/CT scan. In dynamic PET/CT scans, the position of the scanner bed is fixed, as tracer uptake needs to be monitored continuously over the same position. As a consequence, the field of view available for assessment of lesions in dynamic PET/CT scans is limited. To investigate the rest of the body, often a whole static sweep is performed after the dynamic sequence, making it a necessity to assess the performance of simplified parameters such as SUV and TBR. Fortunately, new total body PET/CT scanners have been developed, expanding the field of view to one to two meters. This is of particular interest for conditions where lesions are distributed throughout the body, such as in FOP, and this generation of scanners should therefore be considered for use in future studies when possible.
Despite the limited field of view in our study, we were still able to identify multiple active PET lesions and heterotopic bone in all participants, making it possible to evaluate the correlation between [18F]NaF kinetics and various semi-quantitative parameters. FOP is a heterogeneous disease spread throughout the musculature of the body and which progresses at an unpredictable pace (8). A piece of heterotopic bone may be growing in one location, whilst remaining stable elsewhere. In the present study, various volumes of interest were defined, i.e., CT-based VOIs, in which heterotopic bone was delineated, and PET-based VOIs in which active lesions were defined based on [18F]NaF uptake. From a clinical perspective, it makes more sense to follow the PET-active lesions rather than existing stable heterotopic bone with regard to disease activity. These are the active areas that are not bone yet, but may rapidly be so. Moreover, the changes in TBRmean and SUVmean better reflected the changes in the net rate of [18F]NaF uptake, in these PET-active lesions. We recommend future studies to focus on measuring uptake in PET active regions and monitor them over time by measuring TBR. Following (systemic) therapy, the correlation between semi-quantitative measures and true [18F]NaF kinetics should be re-evaluated, as therapy may affect the underlying biology (e.g., perfusion) in which case simple baseline and follow-up uptake measurements may not be comparable with each other.
The better performance of TBR is likely due to variation in the blood background activity, which is accounted for in the TBR calculation, but not in the SUV calculation. TBR is a semi-quantitative parameter frequently used in PET studies of vascular inflammation and atherosclerotic plaques. Blood activity of the tracer can vary between patients due to biological factors affecting its distribution, uptake and excretion (22). Uptake of an irreversible tracer is directly proportional to perfusion, so both SUV and TBR will be affected by changes in perfusion. Locally, vascularization varies throughout the endochondral ossification process. Initially, a hypoxic poorly vascularized area is present during the cartilaginous phase. This hypoxia, in turn, promotes neovascularization of the cartilage as it transitions to the ossifying phase (6, 23, 24). The normalization of [18F]NaF uptake to blood activity, being part of TBR, corrects for any variation in [18F]NaF blood supply towards the target tissue.
In a few cases, [18F]NaF PET/CT has already been used to evaluate ossifying activity in FOP. Elevated SUV was reported in a FOP patient after jaw surgery, preceding subsequent heterotopic ossification (14). Later, a series of 13 [18F]NaF PET/CT scans in 5 different patients were examined, and areas with increased 18F-fluoride uptake were identified, which subsequently corresponded with radiological progression of HO on a later scan (7). Areas with increased fluoride uptake, measured by SUV, were found to be associated with an increase in heterotopic ossification as measured by CT. A SUVpeak higher than 8.4 was found to be indicative of new HO on a later scan, though the increment of the SUV did not appear to correlate with the amount of bone volume formed. The higher correlation of TBR with full kinetic analysis in the present study, suggests that TBR may be better suited as a semi-quantitative parameter for predicting heterotopic bone formation. Ideally, longitudinal studies with multiple [18F]NaF PET/CT scans need to be performed to determine the optimum cut-off of TBR with regard to sensitivity and specificity for predicting heterotopic ossification in FOP. Most trials in FOP have used the change in total HO volume, as assessed by low-dose whole body CT, as the primary outcome measure for reflecting disease activity and thus drug effectiveness. In addition to the measured HO volume on CT, PET active lesions can be followed over time, visualizing and quantifying disease activity before they are seen on CT images. Other outcome measures reflecting disease activity in FOP include the number of new HO lesions as assessed by CT, number of body regions with HO, flare-up incidence, quality of life questionnaires and clinical measurements, such as CAJIS and pulmonary function tests (NCT03312634, NCT02190747, NCT03188666, NCT04307953). Two trials have also included the number of active PET lesions and lesion activity as outcome measures (NCT03188666, NCT04307953).
Conclusions
Our present study supports TBR as the most suitable semi-quantitative parameter to measure change in 18F-fluoride uptake in patients with FOP, given the high correlation with NLR-derived Ki and the short scanning time required. In addition, changes in uptake are best evaluated in small PET active regions rather than over larger areas of heterotopic bone. Separate studies with dynamic scanning should be performed if there is reason to believe that 18F-fluoride kinetics may be altered by the therapy itself, rather than as a result of a change in bone metabolism reflecting disease activity.
Data availability statement
Qualified researchers may request access to study documents that support the methods and findings reported in this manuscript. Individual anonymized patient data will be considered for sharing once the product and indication has been approved by major health authorities (for example, US FDA, European Medicines Agency and the Pharmaceuticals and Medical Devices Agency), if there is legal authority to share the data and there is not a reasonable likelihood of patient re-identification. Requests should be submitted to https://vivli.org/ (the typical response time is 6-12 months).
Ethics statement
The studies involving humans were approved by Medical Ethics Committee of the Vrije Universiteit medical centre in Amsterdam. The studies were conducted in accordance with the local legislation and institutional requirements. The participants provided their written informed consent to participate in this study.
Author contributions
RD: Formal Analysis, Methodology, Writing – original draft, Writing – review & editing. EB: Data curation, Formal Analysis, Methodology, Writing – original draft, Writing – review & editing. BT: Formal Analysis, Supervision, Writing – original draft, Writing – review & editing. AL: Conceptualization, Investigation, Methodology, Supervision, Writing – original draft, Writing – review & editing. RB: Conceptualization, Data curation, Investigation, Methodology, Software, Writing – original draft, Writing – review & editing. PR: Formal Analysis, Methodology, Supervision, Writing – original draft, Writing – review & editing. LS: Data curation, Methodology, Writing – original draft, Writing – review & editing. JN: Data curation, Methodology, Writing – original draft, Writing – review & editing. DG: Conceptualization, Data curation, Investigation, Methodology, Project administration, Writing – original draft, Writing – review & editing. EE: Conceptualization, Data curation, Formal Analysis, Funding acquisition, Investigation, Methodology, Project administration, Resources, Supervision, Writing – original draft, Writing – review & editing. MY: Data curation, Formal Analysis, Investigation, Methodology, Software, Supervision, Writing – original draft, Writing – review & editing.
Funding
The author(s) declare financial support was received for the research, authorship, and/or publication of this article.
Acknowledgments
We thank the patients and their families for their participation in this study, as well as the healthcare professionals from the VUmc nuclear medicine department who assisted in the scan acquisitions.
Conflict of interest
DGT was employed by Regeneron Pharmaceuticals, Inc. EE reports that Amsterdam UMC receives subsidies/financing FOP research from the Dutch FOP Patient Foundation, IFOPA, Regeneron Pharmaceuticals Inc., EU-IMI (AstraZeneca), and Clementia/ Ipsen; non-paid board memberships for the International Clinical Council on FOP, the IFOPA registry advisory board, and the Dutch Society for Endocrinology Bone; chair of the Rare Bone Expert Center and member of the steering committee of Amsterdam Bone Center, European FOP consortium; and being a member of European Reference Network on rare bone diseases (ERN BOND) and of an American Society for Bone and Mineral Research committee.
The remaining authors declare that the research was conducted in the absence of any commercial or financial relationships that could be construed as a potential conflict of interest.
The authors declare that this study received funding from Regeneron Pharmaceuticals, Inc. The funder had the following involvement in the study: study design and collection, analysis and interpretation of data. The funder was not involved in the writing of this article, or the decision to submit it for publication.
The author(s) declared that they were an editorial board member of Frontiers, at the time of submission. This had no impact on the peer review process and the final decision.
Publisher's note
All claims expressed in this article are solely those of the authors and do not necessarily represent those of their affiliated organizations, or those of the publisher, the editors and the reviewers. Any product that may be evaluated in this article, or claim that may be made by its manufacturer, is not guaranteed or endorsed by the publisher.
Supplementary material
The Supplementary Material for this article can be found online at: https://www.frontiersin.org/articles/10.3389/fnume.2024.1406947/full#supplementary-material
References
1. Pignolo RJ, Hsiao EC, Baujat G, Lapidus D, Sherman A, Kaplan FS. Prevalence of fibrodysplasia ossificans progressiva (FOP) in the United States: estimate from three treatment centers and a patient organization. Orphanet J Rare Dis. (2021) 16(1):350. doi: 10.1186/s13023-021-01983-2
2. Pignolo RJ, Baujat G, Brown MA, De Cunto C, Di Rocco M, Hsiao EC, et al. Natural history of fibrodysplasia ossificans progressiva: cross-sectional analysis of annotated baseline phenotypes. Orphanet J Rare Dis. (2019) 14(1):98. doi: 10.1186/s13023-019-1068-7
3. Shen Q, Little SC, Xu M, Haupt J, Ast C, Katagiri T, et al. The fibrodysplasia ossificans progressiva R206H ACVR1 mutation activates BMP-independent chondrogenesis and zebrafish embryo ventralization. J Clin Invest. (2009) 119(11):3462–72. doi: 10.1172/jci37412
4. Al Kaissi A, Kenis V, Ben Ghachem M, Hofstaetter J, Grill F, Ganger R, et al. The diversity of the clinical phenotypes in patients with fibrodysplasia ossificans progressiva. J Clin Med Res. (2016) 8(3):246–53. doi: 10.14740/jocmr2465w
5. Kaplan FS, Xu M, Seemann P, Connor JM, Glaser DL, Carroll L, et al. Classic and atypical fibrodysplasia ossificans progressiva (FOP) phenotypes are caused by mutations in the bone morphogenetic protein (BMP) type I receptor ACVR1. Hum Mutat. (2009) 30(3):379–90. doi: 10.1002/humu.20868
6. de Ruiter RD, Smilde BJ, Pals G, Bravenboer N, Knaus P, Schoenmaker T, et al. Fibrodysplasia ossificans progressiva: what have we achieved and where are we now? Follow-up to the 2015 Lorentz workshop. Front Endocrinol (Lausanne). (2021) 12:732728. doi: 10.3389/fendo.2021.732728
7. Botman E, Raijmakers PGHM, Yaqub M, Teunissen B, Netelenbos C, Lubbers W, et al. Evolution of heterotopic bone in fibrodysplasia ossificans progressiva: an [18F]NaF PET/CT study. Bone. (2019) 124:1–6. doi: 10.1016/j.bone.2019.03.009
8. Pignolo RJ, Bedford-Gay C, Liljesthröm M, Durbin-Johnson BP, Shore EM, Rocke DM, et al. The natural history of flare-ups in fibrodysplasia ossificans progressiva (FOP): a comprehensive global assessment. J Bone Miner Res. (2016) 31(3):650–6. doi: 10.1002/jbmr.2728
9. Shaikh U, Khan A, Kumari P, Ishfaq A, Ekhator C, Yousuf P, et al. Novel therapeutic targets for fibrodysplasia ossificans progressiva: emerging strategies and future directions. Cureus. (2023) 15(7):e42614. doi: 10.7759/cureus.42614
10. Smilde BJ, Botman E, de Ruiter RD, Smit JM, Teunissen BP, Lubbers WD, et al. Monitoring and management of fibrodysplasia ossificans progressiva: current perspectives. Orthop Res Rev. (2022) 14:113–20. doi: 10.2147/ORR.S337491
11. Kaplan FS, Al Mukaddam M, Pignolo RJ. A cumulative analogue joint involvement scale (CAJIS) for fibrodysplasia ossificans progressiva (FOP). Bone. (2017) 101:123–8. doi: 10.1016/j.bone.2017.04.015
12. Pignolo RJ, McCarrick-Walmsley R, Wang H, Qiu S, Hunter J, Barr S, et al. Plasma-soluble biomarkers for fibrodysplasia ossificans progressiva (FOP) reflect acute and chronic inflammatory states. J Bone Miner Res. (2022) 37(3):475–83. doi: 10.1002/jbmr.4492
13. Hildebrand L, Gaber T, Kühnen P, Morhart R, Unterbörsch H, Schomburg L, et al. Trace element and cytokine concentrations in patients with fibrodysplasia ossificans progressiva (FOP): a case control study. J Trace Elem Med Biol. (2017) 39:186–92. doi: 10.1016/j.jtemb.2016.10.001
14. Eekhoff EMW, Botman E, Coen Netelenbos J, de Graaf P, Bravenboer N, Micha D, et al. [18f]NaF PET/CT scan as an early marker of heterotopic ossification in fibrodysplasia ossificans progressiva. Bone. (2018) 109:143–6. doi: 10.1016/j.bone.2017.08.012
15. Hawkins RA, Choi Y, Huang SC, Hoh CK, Dahlbom M, Schiepers C, et al. Evaluation of the skeletal kinetics of fluorine-18-fluoride ion with PET. J Nucl Med. (1992) 33(5):633–42.1569473
16. Puri T, Blake GM, Siddique M, Frost ML, Cook GJ, Marsden PK, et al. Validation of new image-derived arterial input functions at the aorta using 18F-fluoride positron emission tomography. Nucl Med Commun. (2011) 32(6):486–95. doi: 10.1097/MNM.0b013e3283452918
17. Raijmakers P, Temmerman OP, Saridin CP, Heyligers IC, Becking AG, van Lingen A, et al. Quantification of 18F-fluoride kinetics: evaluation of simplified methods. J Nucl Med. (2014) 55(7):1122–7. doi: 10.2967/jnumed.113.135269
18. Popescu LM, Matej S, Lewitt RM. Iterative image reconstruction using geometrically ordered subsets with list-mode data. IEEE Symposium Conference Record Nuclear Science 2004. IEEE (2004). p. 3536–40.
19. Schiepers C, Nuyts J, Bormans G, Dequeker J, Bouillon R, Mortelmans L, et al. Fluoride kinetics of the axial skeleton measured in vivo with fluorine-18-fluoride PET. J Nucl Med. (1997) 38(12):1970–6.9430479
20. Du Bois D, Du Bois EF. A formula to estimate the approximate surface area if height and weight be known. 1916. Nutrition. (1989) 5(5):303–11; discussion 312–3.2520314
21. Janmahasatian S, Duffull SB, Ash S, Ward LC, Byrne NM, Green B. Quantification of lean bodyweight. Clin Pharmacokinet. (2005) 44(10):1051–65. doi: 10.2165/00003088-200544100-00004
22. Chen W, Dilsizian V. PET assessment of vascular inflammation and atherosclerotic plaques: SUV or TBR? J Nucl Med. (2015) 56(4):503–4. doi: 10.2967/jnumed.115.154385
23. Wang H, Lindborg C, Lounev V, Kim JH, McCarrick-Walmsley R, Xu M, et al. Cellular hypoxia promotes heterotopic ossification by amplifying BMP signaling. J Bone Miner Res. (2016) 31(9):1652–65. doi: 10.1002/jbmr.2848
Keywords: fibrodysplasia ossificans progressiva, [18F]NaF, sodiumfluoride, PET, positron emission tomography, dynamic imaging
Citation: de Ruiter RD, Botman E, Teunissen BP, Lammertsma AA, Boellaard R, Raijmakers PG, Schwarte LA, Nieuwenhuijzen JA, Gonzalez Trotter D, Eekhoff EMW and Yaqub M (2024) Performance of simplified methods for quantification of [18F]NaF uptake in fibrodysplasia ossificans progressiva. Front. Nucl. Med. 4: 1406947. doi: 10.3389/fnume.2024.1406947
Received: 25 March 2024; Accepted: 2 July 2024;
Published: 22 July 2024.
Edited by:
Mario Petretta, IRCCS SYNLAB SDN, ItalyReviewed by:
Adriana D'Antonio, University of Naples Federico II, ItalyRoberta Green, Federico II University Hospital, Italy
© 2024 de Ruiter, Botman, Teunissen, Lammertsma, Boellaard, Raijmakers, Schwarte, Nieuwenhuijzen, Gonzalez Trotter, Eekhoff and Yaqub. This is an open-access article distributed under the terms of the Creative Commons Attribution License (CC BY). The use, distribution or reproduction in other forums is permitted, provided the original author(s) and the copyright owner(s) are credited and that the original publication in this journal is cited, in accordance with accepted academic practice. No use, distribution or reproduction is permitted which does not comply with these terms.
*Correspondence: Elisabeth Marelise W. Eekhoff, emw.eekhoff@amsterdamumc.nl
†These authors have contributed equally to this work and share last authorship
‡ORCID:
Ruben Daniel de Ruiter
orcid.org/0000-0002-5035-9199
Esmée Botman
orcid.org/0000-0002-5001-4748
Bernd P. Teunissen
orcid.org/0000-0002-1122-155X
Adriaan Anthonius Lammertsma
orcid.org/0000-0003-1237-2891
Ronald Boellaard
orcid.org/0000-0002-0313-5686
Pieter G. Raijmakers
orcid.org/0000-0002-5225-3240
Lothar A. Schwarte
orcid.org/0000-0001-6119-7810
Jakko A. Nieuwenhuijzen
orcid.org/0000-0002-8977-5501
Elisabeth Marelise W. Eekhoff
orcid.org/0000-0001-5399-676X
Maqsood Yaqub
orcid.org/0000-0003-2122-740X