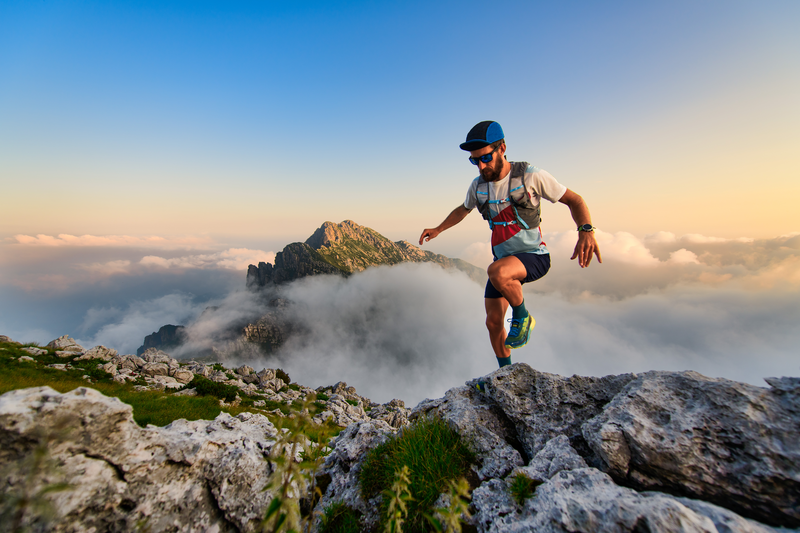
95% of researchers rate our articles as excellent or good
Learn more about the work of our research integrity team to safeguard the quality of each article we publish.
Find out more
REVIEW article
Front. Neurosci. , 29 January 2025
Sec. Neuropharmacology
Volume 19 - 2025 | https://doi.org/10.3389/fnins.2025.1518967
Water serves as the primary substance in all living cells and is an essential molecule involved in numerous biological processes critical for maintaining homeostasis in the central nervous system (CNS). Disruptions in water balance can occur in conditions such as cerebral edema, where fluid accumulation results in increased intracranial pressure (ICP). Aquaporins (AQPs) are transmembrane proteins that play a vital role in the rapid transport of water across cell membranes. Various subtypes of AQPs (AQP1, AQP3, AQP4, AQP5, AQP6, AQP7, AQP8, AQP9, and AQP11) have been identified in brain tissue. This review summarizes the latest advancements in our understanding of the critical role of AQPs in regulating water transport in brain edema. Abundant evidence indicates that AQP4, the most prevalent AQP in the CNS, regulates brain water transport and contributes to both cytotoxic and vasogenic edema, suggesting that AQP4 may serve as a potential therapeutic target for brain edema. Additionally, some studies have indicated that AQP1 plays a significant role in the formation of cerebrospinal fluid (CSF) and the maintenance of steady-state ICP. However, to date, these findings have not been translated into clinical practice. There is an urgent need to develop specific AQP inhibitors and activators to explore the potential benefits of modulating the functions of AQP1 and AQP4 in the context of brain edema.
Water is the most abundant component of all living cells and organisms, and the effective regulation of water balance is crucial for numerous biochemical processes. Approximately 80% of the brain is composed of water, which circulates among various compartments, including blood, cerebrospinal fluid (CSF), and the intracellular and interstitial spaces of brain parenchyma. It traverses the blood-brain and the blood-CSF interfaces. Disturbances in water homeostasis can have severe detrimental effects on brain function and significantly contribute to the pathophysiology of traumatic brain injury (TBI), stroke, and various cerebral disorders (Fishman, 1975).
Cerebral edema is defined as the excessive accumulation of water within the brain parenchyma, which is associated with various cerebral injuries, including ischemic injury, TBI, and brain tumors. This condition ultimately leads to an increase in intracranial pressure (ICP) (Chen et al., 2024). Elevated ICP can further impair the regulation of cerebral blood flow (CBF), eventually resulting in additional brain injury and potentially death. The regulation of brain water balance is of clinically significant. However, the exact mechanisms involved in water accumulation and clearance in the brain remain unclear. Currently, the treatment of cerebral edema mainly focuses on removing excess water from the brain parenchyma and reducing ICP, which helps to limit the damage but fails to address the underlying causes of brain edema. Therefore, the development of novel therapeutic drugs that directly target the molecular key players in the mechanisms of edema formation will contribute to adjusting current therapies and establishing new treatment strategies.
Aquaporins (AQPs) are a recently identified family of transmembrane water channel proteins that facilitate water transport and play a significant role in regulating water homeostasis across various tissues, including the nervous system (Zhou et al., 2022). Numerous recent studies have shown that AQP water channels are crucial for maintaining brain water balance and for the development and resolution of edema. This review specifically examines the role of AQPs in brain water transport and cerebral edema.
To date, a total of 13 isoforms (AQP0–12) (Figure 1) are included in the AQP superfamily across numerous mammalian tissues, such as the kidney, nervous system, lung, liver, gastrointestinal tract, eye, heart, skin, and adipose tissue (Czyżewski et al., 2024). AQP monomers weigh approximately 28 kDa and typically encompass six highly hydrophobic transmembrane spanning domains and a central water pore (Eriksson et al., 2013) (Figure 2). These proteins are currently classified into three principal subfamilies based on their pore selectivity and sequence homology (da Silva et al., 2022): (a) conventional water-selective AQPs or aquaporins (AQP0,−1,−2,−4,−5,−6, and−8) are primarily permeable to water at a high flow rate. Nevertheless, AQP1, AQP6, and AQP8 also transport volatile solutes such as CO2, anions, and ammonia, respectively (Galli et al., 2021); (b) aquaglyceroporins (AQP3,−7,−9, and−10) are permeable to glycerol, urea, and other small solutes in addition to water; and (c) superaquaporins or subcellular aquaporins (AQP11 and 12) are localized within the cytosol and are involved in intracellular homeostasis. Additionally, a fourth subgroup was reported relatively recently, which included paralogs belonging to the aforementioned three subgroups (AQP0, 1, 3, 5, 8, 9, and 11), named peroxiporins. These AQPs have high permeability to hydrogen peroxide (H2O2) and play a significant role in eliminating excessive reactive oxygen species (ROS) (Varadaraj and Kumari, 2020; Montiel et al., 2020; Silva et al., 2022; Krüger et al., 2021; Zhang et al., 2022; Sorrentino et al., 2022). Table 1 summarizes the subdivision of the mammalian AQPs and their basic properties.
Figure 2. A secondary structure and topology of AQP molecule. AQP monomer has six membranespanning regions (1–6), five loops (A-E) with intracellular amino and carboxy termini as well as internal tandem repeats (top). Each monomer has a water pore (bottom).
To date, nine subtypes of AQP (AQP1, AQP3, AQP4, AQP5, AQP6, AQP7, AQP8, AQP9, and AQP11) have been recognized in various types of brain-resident cells, such as the cerebral cortex, cerebellum, choroid plexus, brain cells, and neural stem cells (as illustrated in Figure 3 and Table 2). However, it is important to note that only AQP1, AQP4, and AQP9 possess significant physiological functions and pathological implications. AQP4 is the most predominant and well-characterized aquaporin identified in the brain, with the brain being the primary site of AQP4 expression in mammals (Jung et al., 1994). In brain tissue, AQP4 is predominantly expressed in glia cells, particularly at the interface between the brain and major water-containing compartments (Rash et al., 1998), indicating its role in the movement of water into and out of the brain. AQP1 is expressed in the ventricular-facing cell plasma membrane of epithelial cells in the choroid plexus, suggesting a role of AQP1 in CSF secretion (Nielsen et al., 1993). AQP9 is the sole aquaglyceroporin expressed in astrocytes of the glia limitans and white matter tracts (Badaut et al., 2004). The function of AQP9 in the brain remains unclear, but its permeability to glycerol suggests that its likely involvement in brain metabolism. Although the transcriptional abundances of AQP1 and AQP9 are extremely low in human and mouse brain cells under physiological conditions, certain upregulations may occur under pathological circumstances (Misawa et al., 2008; Geistlinger et al., 2022).
Figure 3. Brain aquaporin expression. Astrocytes express AQP1, AQP3, AQP4, AQP5, AQP8, and AQP9. Oligodendrocytes express AQP8. Neuron express AQP1, AQP3, AQP5, AQP8, AQP9, and AQP11. Neural stem cells express AQP4, AQP6, AQP8, and AQP9. AQP1, AQP3, AQP5, AQP7, AQP8, and AQP11 are expressed in the choroid plexus. AQP1, AQP3, AQP4, AQP5, AQP6, AQP8, AQP9, and AQP11 are found in the cerebellum. AQP1, AQP3, AQP4, AQP5, AQP8, AQP9, and AQP11 are expressed in the cerebral cortex.
In the typical adult brain, water migrates between various compartments (CSF, blood, and both intracellular and interstitial brain parenchyma) in response to differences in hydrostatic and osmotic pressure. Water enters the brain through the blood-brain barrier (BBB) or the choroid plexus and exits through the arachnoid granulations into the venous system (Abbott, 2004). The significant expression of AQP4 at the BBB and in ependymal cells lining the ventricles has promoted initial studies on the crucial role of AQP4 in determining the water permeability of the BBB. AQP4 knockout mice exhibited greater ventricular enlargement and elevated ICP compared to wild-type mice in an experimental model of obstructive hydrocephalus, suggesting a function for AQP4 in CSF absorption (Bloch et al., 2006). Transgenic mice overexpressing AQP4 demonstrated an accelerated progression of brain swelling and poorer outcomes in water intoxication (Yang et al., 2008a). The deletion of AQP1 reduces osmotic water permeability in the choroid plexus by a factor of five. However, CSF production is only reduced by approximately 25% in AQP1 knockout mice compared to wild-type mice, indicating that only a portion of CSF secretion is AQP1-dependent and that there is a substantial contribution from extrachoroidal fluid production by the brain parenchyma (Oshio et al., 2005). Moreover, a study involving AQP1-deficient and AQP4-deficient mice concluded that AQP4, rather than AQP1, aids in mediating water flow into CSF, suggesting that AQP4 plays a vital role in CSF production (Igarashi et al., 2014). These phenotypic studies of AQP4 and AQP1 transgenic mice offer evidence for the roles of these water channels in the physiology of brain water homeostasis under normal and abnormal circumstances.
Klatzo classified edema into cytotoxic and vasogenic types (Klatzo, 1967). Fishman later added another type, termed interstitial (or hydrocephalic) edema, observed in patients with hydrocephalus (Fishman, 1975). Cytotoxic edema represents the intracellular accumulation of water reliant on ionic imbalances and affects glial, neuronal, and endothelial cells within the brain. The typical cytotoxic edema occurs in the early stages of ischemia or hypoxia, cerebral malaria, and hyponatremia, when brain cells are impaired but the BBB remains intact. In contrast, vasogenic edema emerges due to the disruption of the BBB, leading to an enhanced permeability of capillary endothelial cells to albumin and other plasma proteins (Hu et al., 2023). Nevertheless, in most instances, these two types of edema coexist, and one type of edema gradually transforms into the other (Pasantes-Morales and Cruz-Rangel, 2010). Interstitial cerebral edema refers to the outflow of CSF from the ventricles into the interstitial space of the brain. Patients with hydrocephalus or meningitis are affected by this etiology. The elevated pressure on the brain and CSF forces fluid into the brain parenchyma. The accumulation of fluid in the extracellular space, primarily in the white matter, leads to cerebral edema.
Since astrocytes are the major cell type that swells in cytotoxic brain edema (Kimelberg, 1995) and are the predominant sites of AQP4 expression in the brain (González-Marrero et al., 2022), it can be logically inferred that AQP4 plays a crucial role in the development of cytotoxic brain edema (Figure 4). Manley et al. (2000) demonstrated that AQP4-deficient mice performed better than their wild-type counterparts in cytotoxic brain edema, employing two models of cytotoxic edema, namely acute water intoxication and early cerebral ischemia. Additionally, Papadopoulos and Verkman (2005) reported that AQP4-deficient mice had significantly less brain water accumulation in meningitis-induced brain edema, another cytotoxic edema model. Recently, Sucha et al. (2022) discovered that simultaneous deletion of AQP4 and Transient Receptor Potential Vanilloid 4 mitigated the cytotoxic edema following ischemic injury. AQP4 molecules are linked to the cell membrane by α-syntrophin, dystrophin, and other proteins, which are crucial for the localization of AQP4 to the astrocytic end-feet. Comparable studies have validated the role of AQP4 in cytotoxic edema using the dystrophin null mdx-βgeo transgenic mice and the α-syntrophin null mice, which are considered as alternative models for the AQP4-null genotype (Moëlo et al., 2023; Vajda et al., 2002). Furthermore, AQP5 is also implicated in cytotoxic brain edema. Yamamoto et al. (2001) indicated that hypoxia elicited a significant decrease in AQP5 mRNA in rat astrocytes, suggesting that AQP5 might be one of the candidates for inducing cytotoxic edema in the central nervous system after ischemic injury.
Figure 4. Role of AQP4 in cytotoxic edema. The swelling of the endfeet of astrocytes after water enters through the AQP4 water channel is a characteristic of cytotoxic edema.
AQP4 may not only facilitate water entry into the brain but also its exit (Figure 5). Overexpression of AQP4 has been observed in meningiomas, which is associated with the response to vasogenic edema of meningiomas (Faropoulos et al., 2021). In relation to vasogenic edema, Papadopoulos et al. (2004) noted that AQP4 deletion had the opposite effect (increased brain swelling) in three vasogenic edema models: intraparenchymal fluid infusion, focal cortical freeze injury, and brain tumor cell implantation. Following continuous intracerebral fluid infusion, AQP4-deficient mice exhibited higher ICP and brain water content compared to wild-type controls. Cortical freeze injury gives rise to a significantly greater brain water content and higher ICP in AQP4-deficient mice. In a brain tumor edema model involving stereotactic implantation of melanoma cells, AQP4-deficient mice showed higher ICP and accelerated neurological deterioration (Papadopoulos et al., 2004). A similar outcome was observed with the injection of Staphylococcus aureus to generate brain abscesses in the striatum of mice. AQP4-deficient mice exhibited more than twice the increase in brain water content, along with significantly higher ICP than wild-type control mice (Bloch et al., 2005). These findings suggest that AQP4 activators might alleviate vasogenic brain edema in humans. Additionally, AQP5 also plays a role in water homeostasis in vasogenic brain edema. Lambertz et al. (2013) demonstrated that the occurrence and severity of peritumoral edema in meningiomas were associated with AQP5 polymorphism A(-1364)C.
Figure 5. Different role of AQP4 in cytotoxic and vasogenic edema. In cytotoxic edema the water accumulation in astrocyte is AQP4 dependent. Contrary to cytotoxic edema, AQP4 plays a favorable role in water elimination in vasogenic edema.
Given the crucial roles of AQP1 in CSF production and AQP4 in CSF absorption, it is proposed that they are involved in hydrocephalic brain edema resulting from the increase in CSF pressure and disruption of the BBB. The increased expression of AQP1 highlights the importance of excessive CSF production and an intact blood-CSF barrier during the development of hydrocephalic edema (Kim and Jung, 2011). Experiments have demonstrated that AQP1-null mice exhibit a fivefold reduction in osmotic water permeability in the choroid plexus compared to wild-type mice, indicating that reducing the function of AQP1 decreases the occurrence of non-obstructive hydrocephalus (Oshio et al., 2003). Several studies have reported elevated expression of AQP4 in connection with hydrocephalus, including congenital hydrocephalus in Texas rats (Paul et al., 2011), idiopathic communicating internal hydrocephalus in dogs (Schmidt et al., 2016), and inflammatory communicating hydrocephalus in rats (Tourdias et al., 2009). In patients with congenital hydrocephalus, the expression of AQP4 is elevated in both CSF and brain parenchyma (Castañeyra-Ruiz et al., 2013). In a model of obstructive hydrocephalus induced by kaolin injection, AQP4-deficient mice exhibited significant ventriculomegaly and increased ICP, which was suggested to result from impaired transependymal water clearance (Bloch et al., 2006). These discoveries suggest a compensatory function of AQPs in the water dynamics of hydrocephalic edema.
Vasopressin (VP) plays a crucial role in the development of brain injury and has been shown to regulate AQP4 expression in cases of brain edema. Studies indicate that astrocyte AQP4 levels were reduced by vasopressin 1a receptor (V1AR) antagonist treatment in a model of TBI, concurrently with the prevention of cell swelling and a reduction in edema (Marmarou et al., 2014; Taya et al., 2008). Additionally, V1AR has been found to upregulated cerebreal AQP1 expression, suggesting that V1AR may worsen brain edema formation post-TBI (Rauen et al., 2020). There is a substantial evidence suggesting that V1AR antagonists help reduce the development of cytotoxic brain edema by decreasing the upregulation of AQP4 (Kleindienst et al., 2013). In a stroke model induced by middle cerebral artery occlusion followed by reperfusion, it was observed that administering a V1AR antagonist decreased AQP4 expression in cytotoxic brain edema (Okuno et al., 2008).
Dopamine has been demonstrated to curtail the proliferation of striatal astrocytes in vitro and downregulate AQP4 expression in primary cultured astrocytes (Küppers et al., 2008). It has been reported that AQP4 expression is augmented in the substantia nigra following tissue-type plasminogen activator-induced degeneration of dopaminergic neurons (Villarán et al., 2009). Collectively, these studies imply that dopamine might exert a persistent suppressive effect on AQP4 expression. In a model of TBI, it has been reported that dopamine deteriorates cerebral edema formation shortly after impact (Beaumont et al., 2000). This effect has been attributed to the vasopressor action of dopamine, which facilitated vasogenic edema.
In recent years, a potent neuroprotective agent, erythropoietin (EPO), has been demonstrated to enhance the permeability of astrocyte AQP4 and might directly lower the risk of astrocyte swelling in stroke and other brain disorders (Gunnarson et al., 2009). In an animal hydrocephalus model, EPO treatment significantly mitigated the dilation of the cerebral ventricles in obstructive hydrocephalus by augmenting the expression of AQP4 (Rizwan Siddiqui et al., 2018). The administration of EPO post-TBI inhibited the reduction of AQP4, alleviated early cytotoxic brain edema, and maintained the structural and functional characteristics of the BBB, thereby weakening the vasogenic edema response (Blixt et al., 2018).
Thyroid transcription factor-1 (TTF-1), a transcriptional regulator containing a homeodomain and is coexpressed with AQP1 in the rat brain choroid plexus, has been reported to enhance the transcription of the AQP1 gene (Kim et al., 2007). Consequently, inhibiting the synthesis of TTF-1 increased the survival rate of animals with acute water intoxication-induced brain edema by suppressing AQP1 expression.
Astrocytes-mediated potassium (K+) homeostasis is critically important for regulating neuronal excitability. An early study revealed that AQP4 is co-localized with the inward rectifier potassium channel Kir4.1 in the end-feet of retinal Müller cells, suggesting a functional interaction between the two (Nagelhus et al., 1999). The uncoupled expression of AQP4 and Kir4.1 on astrocytic end-feet contributes to the development of cytotoxic edema in the brain following subarachnoid hemorrhage (Yan et al., 2012).
Calcium (Ca2+) signaling mediates of bidirectional interactions between neurons and astrocytes. Impaired Ca2+ signaling plays a critical role in the progression of brain edema. Recent evidence suggests that AQP4 is involved in Ca2+ signaling in astrocytes. The deletion of AQP4 reduces Ca2+ signaling induced by hypo-osmotic stress in these cells (Thrane et al., 2011). This finding indicates that Ca2+ signals may result from AQP4-induced astrocyte swelling rather than being directly induced by AQP4 itself. Edema leads to astrocyte swelling, and the presence of AQP4 exacerbates this condition.
Connexin 43 (Cx43) is a prominent gap junction protein that is extensively expressed in the end-feet of astrocytes. It mediates intracellular interactions by facilitating the transport of ionic and molecules. Recent studies have linked Cx43 to abnormal neuro-differentiation associated with brain injury (Samarasinghe et al., 2014). Both Cx43 and AQP4 play crucial roles in the development of brain edema, with Cx43 potentially acting as a downstream effector of AQP4 (Li et al., 2015). The coordinated action of Cx43 and AQP4, along with K+ channels (Kir4.1/Kir5.1), ensures the efficient clearance of K+ from astrocytes into the vascular system (Lichter-Konecki et al., 2008).
The glymphatic system (GS) is a recently discovered microscopic fluid clearance system composed of astrocytic perivascular tunnels that eliminate metabolic wastes from the brain. In this system, the CSF and interstitial fluid (ISF) continuously exchange. It comprises three key compartments: a periarterial CSF influx pathway, a perivenous ISF efflux pathway, and a parenchymal exchange pathway that relies on astrocytic AQP4 (Iliff et al., 2012). Since this discovery, increasing evidence indicates that the GS dysfunction is associated with the formation of brain edema, as it involves AQP4, which plays a crucial role in regulating water homeostasis. The water flow through AQP4 is bidirectional. AQP4 might potentially contribute to astrocytic swelling and cytotoxic edema by facilitating water influx into astrocytes during the early stages of ischemic stroke. However, in the later stages of ischemic stroke, when vasogenic edema becomes the primary cause of brain swelling due to the BBB breakdown, AQP4 could serve the purpose of alleviating brain edema by mediating the transport of excess water from the interstitial space to the GS. By promoting the AQP4 polarization to improve the GS function, it might facilitate the inflow of edema fluids, but it can also significantly enhance downstream drainage function, thereby reducing brain edema (Zhu et al., 2024).
The management of brain edema involves sedation and the prevention of hypercapnia to avoid elevated intracranial pressure. This includes the administration of intravenous hyperosmolar solutions, such as mannitol and hypertonic saline. Corticosteroids are utilized for treating brain tumors. Surgical resection of the underlying lesion may be performed, and in severe cases, decompressive craniectomy is indicated. For critically ill patients, invasive monitoring of intracranial pressure and cerebral perfusion pressure is conducted to optimize treatment. However, many of these therapies for reducing brain swelling were introduced in the early to mid-20th century, and their efficacy remains limited.
Water transport is a crucial process that contributes to human cerebral edema. Consequently, the utilization of AQP-targeted drugs to regulate water transport would offer novel prospects for therapeutic approaches to brain edema. Nevertheless, at present, there are currently a limited number of AQP-specific pharmacological modulators. Despite being non-specific, certain drugs that enhance or suppress AQP4 activity provide effective therapy in both the formation and resolution of edema. Bumetanide has been demonstrated to prevent edema formation following brain ischemia by reducing AQP4 expression (Migliati et al., 2010; Chen et al., 2023). However, bumetanide is a loop diuretic that inhibits the Na+/K+/2Cl− cotransporter isoform (Boyarko et al., 2023), and its benefits on cerebral edema might also be attributed to the aberration of Na+/K+/2Cl− cotransporter 1 expression in endothelial cells (Yang et al., 2019; O'Donnell et al., 2004). Therefore, it is challenging to comprehend the specific impact of AQP4 on edema formation. Acetazolamide (AZA), one of the most prevalently used drugs for lowering ICP and reducing CSF secretion, was also regarded as an inhibitor of AQP1 and AQP4 (Uldall et al., 2017; Gao et al., 2006). However, contrary to the aforementioned results, Yang et al. (2008b) found no significant inhibition of AQP4 water permeability by AZA. Similarly, methazolamide (MZA), another sulfonamide carbonic anhydrase inhibitor, also exhibited no significant effect on water permeability (Tanimura et al., 2009). Instead of a specific pharmacological drug to suppress AQP4, small interfering RNA (siRNA) technology has been employed to silence AQP4 in primary cultured astrocytes to prevent glutamate-induced astrocyte swelling (Lu et al., 2022). AQP4-siRNA was used to mitigate TBI induced edema by modifying post-traumatic AQP4 polarity reversal in rats (Lu et al., 2020). Similar findings have been documented by Guan et al. (2020), Chen et al. (2016), Fukuda et al. (2013), and others. While the siRNA approach is unlikely to be utilized as a specific drug for preventing edema formation, research on AQP4 inhibition by siRNA in animals will be valuable for validating the hypothesis that inhibiting AQP4 activity is a potential therapeutic target for managing brain edema.
In this review, data from cellular and in vivo animal studies strongly support the idea that AQPs play a functional role in brain water transport. The modulation of water transport is an integral component of controlling edema. Increasing evidence indicates that AQP4 has been shown to influence the accumulation and elimination of edema fluid. The careful modulation of AQP4 in different types of edema is particularly significant because AQP4 has dual roles in brain edema. In the early cytotoxic edema phase, AQP4 promotes the formation of edema fluid. In vasogenic brain edema, AQP4 enhances the rate of edema fluid elimination. Therefore, AQP4 inhibitors would be necessary to protect the brain in cytotoxic edema, while AQP4 activators are anticipated to facilitate the elimination of vasogenic brain edema. Unfortunately, as of now, no effective drugs for altering AQP4 expression or function have been identified. Additionally, AQP4 is expressed in many human tissues involved in crucial cellular and organ functions, such as urinary concentration, exocrine glandular secretion, and metabolism. Therefore, AQP4-selective therapeutic is required, which is a challenge since there are conserved amino acid sequences in the pore regions of various AQP subtypes. Furthermore, there are other brain-AQPs (AQP1 and 9) whose expression changes in brain disorders, but little is known about their pathophysiological effects. It is not clear whether there is an interaction between the different subtypes during edema and whether the other AQPs present in the brain also play roles in brain edema.
YL: Conceptualization, Writing – original draft, Writing – review & editing. YW: Investigation, Visualization, Writing – original draft. XH: Investigation, Writing – original draft. HZ: Investigation, Writing – original draft. YG: Conceptualization, Writing – review & editing. XZ: Conceptualization, Writing – review & editing.
The author(s) declare financial support was received for the research, authorship, and/or publication of this article. This work was supported by the National Key R&D Program of China 2020YFC2005000, the National Natural Science Foundation of China Grants 81970606 (to XZ), 81970595 (to YG), and the General Project of the Department of Education of Liaoning Province JYTMS20230566 (to YL). This work was also supported by the Youth Talent Cultivation Fund Project of Dalian Medical University (to YL).
The authors declare that the research was conducted in the absence of any commercial or financial relationships that could be construed as a potential conflict of interest.
The author(s) declare that no Gen AI was used in the creation of this manuscript.
All claims expressed in this article are solely those of the authors and do not necessarily represent those of their affiliated organizations, or those of the publisher, the editors and the reviewers. Any product that may be evaluated in this article, or claim that may be made by its manufacturer, is not guaranteed or endorsed by the publisher.
CSF, cerebrospinal fluid; TBI, traumatic brain injury; ICP, intracranial pressure; CFB, cerebral blood flow; AQPs, Aquaporins; ROS, reactive oxygen species; BBB, blood-brain barrier; VP, vasopressin; V1AR, vasopressin 1a receptor; EPO, erythropoietin; TTF-1, thyroid transcription factor-1; GS, glymphatic system; ISF, interstitial fluid; AZA, acetazolamide; MZA, methazolammide; siRNA, small interfering RNA.
Abbott, N. J. (2004). Evidence for bulk flow of brain interstitial fluid: significance for physiology and pathology. Neurochem. Int. 45, 545–552. doi: 10.1016/j.neuint.2003.11.006
Alishahi, M., and Kamali, R. (2019). A novel molecular dynamics study of CO2 permeation through aquaporin-5. Eur. Phys. J. E Soft Matter. 42:151. doi: 10.1140/epje/i2019-11912-x
Antequera, D., Carrero, L., Cunha Alves, V., Ferrer, I., Hernández-Gallego, J., Municio, C., et al. (2022). Differentially aquaporin 5 expression in submandibular glands and cerebral cortex in Alzheimer's disease. Biomedicines 10:1645. doi: 10.3390/biomedicines10071645
Arena, S., Arena, F., Maisano, D., Di Benedetto, V., Romeo, C., Nicòtina, P. A., et al. (2011). Aquaporin-9 immunohistochemistry in varicocele testes as a consequence of hypoxia in the sperm production site. Andrologia 43, 34–37. doi: 10.1111/j.1439-0272.2009.01009.x
Assentoft, M., Kaptan, S., Schneider, H. P., Deitmer, J. W., de Groot, B. L., MacAulay, N., et al. (2016). Aquaporin 4 as a NH3 channel. J. Biol. Chem. 291, 19184–19195. doi: 10.1074/jbc.M116.740217
Badaut, J., Petit, J. M., Brunet, J. F., Magistretti, P. J., Charriaut-Marlangue, C., Regli, L., et al. (2004). Distribution of Aquaporin 9 in the adult rat brain: preferential expression in catecholaminergic neurons and in glial cells. Neuroscience 128, 27–38. doi: 10.1016/j.neuroscience.2004.05.042
Beaumont, A., Hayasaki, K., Marmarou, A., Barzo, P., Fatouros, P., Corwin, F., et al. (2000). The effects of dopamine on edema formation in two models of traumatic brain injury. Acta Neurochir. Suppl. 76, 147–151. doi: 10.1007/978-3-7091-6346-7_30
Benga, G., Popescu, O., Borza, V., Pop, V. I., Muresan, A., Mocsy, I., et al. (1986). Water permeability in human erythrocytes: identification of membrane proteins involved in water transport. Eur. J. Cell Biol. 41, 252–262.
Blixt, J., Gunnarson, E., and Wanecek, M. (2018). Erythropoietin attenuates the brain edema response after experimental traumatic brain injury. J. Neurotrauma. 35, 671–680. doi: 10.1089/neu.2017.5015
Bloch, O., Auguste, K. I., Manley, G. T., and Verkman, A. S. (2006). Accelerated progression of kaolin-induced hydrocephalus in aquaporin-4-deficient mice. J. Cereb. Blood Flow Metab. 26, 1527–1537. doi: 10.1038/sj.jcbfm.9600306
Bloch, O., Papadopoulos, M. C., Manley, G. T., and Verkman, A. S. (2005). Aquaporin-4 gene deletion in mice increases focal edema associated with staphylococcal brain abscess. J. Neurochem. 95, 254–262. doi: 10.1111/j.1471-4159.2005.03362.x
Bogner, B., Schroedl, F., Trost, A., Kaser-Eichberger, A., Runge, C., Strohmaier, C., et al. (2016). Aquaporin expression and localization in the rabbit eye. Exp. Eye Res. 147, 20–30. doi: 10.1016/j.exer.2016.04.013
Borsani, E., Bernardi, S., Albertini, R., Rezzani, R., and Rodella, L. F. (2009). Alterations of AQP2 expression in trigeminal ganglia in a murine inflammation model. Neurosci. Lett. 449, 183–188. doi: 10.1016/j.neulet.2008.11.014
Boyarko, B., Podvin, S., Greenberg, B., Momper, J. D., Huang, Y., Gerwick, W. H., et al. (2023). Evaluation of bumetanide as a potential therapeutic agent for Alzheimer's disease. Front. Pharmacol. 14:1190402. doi: 10.3389/fphar.2023.1190402
Castañeyra-Ruiz, L., González-Marrero, I., González-Toledo, J. M., Castañeyra-Ruiz, A., de Paz-Carmona, H., Castañeyra-Perdomo, A., et al. (2013). Aquaporin-4 expression in the cerebrospinal fluid in congenital human hydrocephalus. Fluids Barriers CNS 10:18. doi: 10.1186/2045-8118-10-18
Cavazzin, C., Ferrari, D., Facchetti, F., Russignan, A., Vescovi, A. L., La Porta, C. A., et al. (2006). Unique expression and localization of aquaporin-4 and aquaporin-9 in murine and human neural stem cells and in their glial progeny. Glia 53, 167–181. doi: 10.1002/glia.20256
Chai, R. C., Jiang, J. H., Wong, A. Y., Jiang, F., Gao, K., Vatcher, G., et al. (2013). AQP5 is differentially regulated in astrocytes during metabolic and traumatic injuries. Glia 61, 1748–1765. doi: 10.1002/glia.22555
Chen, C., Fan, P., Zhang, L., Xue, K., Hu, J., Huang, J., et al. (2023). Bumetanide rescues aquaporin-4 depolarization via suppressing β-dystroglycan cleavage and provides neuroprotection in rat retinal ischemia-reperfusion injury. Neuroscience 510, 95–108. doi: 10.1016/j.neuroscience.2022.11.033
Chen, J. Q., Zhang, C. C., Jiang, S. N., Lu, H., and Wang, W. (2016). Effects of aquaporin 4 knockdown on brain edema of the uninjured side after traumatic brain injury in rats. Med. Sci. Monit. 22, 4809–4819. doi: 10.12659/MSM.898190
Chen, X., Józsa, T. I., Cardim, D., Robba, C., Czosnyka, M., Payne, S. J., et al. (2024). Modelling midline shift and ventricle collapse in cerebral oedema following acute ischaemic stroke. PLoS Comput. Biol. 20:e1012145. doi: 10.1371/journal.pcbi.1012145
Chepelinsky, A. B. (2009). Structural function of MIP/aquaporin 0 in the eye lens; genetic defects lead to congenital inherited cataracts. Handb. Exp. Pharmacol. 190, 265–297. doi: 10.1007/978-3-540-79885-9_14
Chung, S. W., Kim, J. Y., Yoon, J. P., Suh, D. W., Yeo, W. J., Lee, Y. S., et al. (2020). Atrogin1-induced loss of aquaporin 4 in myocytes leads to skeletal muscle atrophy. Sci. Rep. 10:14189. doi: 10.1038/s41598-020-71167-8
Czyżewski, W., Litak, J., Sobstyl, J., Mandat, T., Torres, K., Staśkiewicz, G., et al. (2024). Aquaporins: gatekeepers of fluid dynamics in traumatic brain injury. Int. J. Mol. Sci. 25:6553. doi: 10.3390/ijms25126553
da Silva, I. V., Garra, S., Calamita, G., and Soveral, G. (2022). The multifaceted role of aquaporin-9 in health and its potential as a clinical biomarker. Biomolecules 12:897. doi: 10.3390/biom12070897
Dai, C., Charlestin, V., Wang, M., Walker, Z. T., Miranda-Vergara, M. C., Facchine, B. A., et al. (2020). Aquaporin-7 regulates the response to cellular stress in breast cancer. Cancer Res. 80, 4071–4086. doi: 10.1158/0008-5472.CAN-19-2269
Delporte, C., Virreira, M., Crutzen, R., Louchami, K., Sener, A., Malaisse, W. J., et al. (2009). Functional role of aquaglyceroporin 7 expression in the pancreatic beta-cell line BRIN-BD11. J. Cell. Physiol. 221, 424–429. doi: 10.1002/jcp.21872
Ehring, G. R., Zampighi, G., Horwitz, J., Bok, D., and Hall, J. E. (1990). Properties of channels reconstituted from the major intrinsic protein of lens fiber membranes. J. Gen. Physiol. 96, 631–664. doi: 10.1085/jgp.96.3.631
Eide, P. K. (2022). Cellular changes at the glia-neuro-vascular interface in definite idiopathic normal pressure hydrocephalus. Front. Cell. Neurosci. 16:981399. doi: 10.3389/fncel.2022.981399
Elsherbini, D. M. A., Ghoneim, F. M., El-Mancy, E. M., Ebrahim, H. A., El-Sherbiny, M., El-Shafey, M., et al. (2022). Astrocytes profiling in acute hepatic encephalopathy: Possible enrolling of glial fibrillary acidic protein, tumor necrosis factor-alpha, inwardly rectifying potassium channel (Kir 4.1) and aquaporin-4 in rat cerebral cortex. Front. Cell. Neurosci. 16:896172. doi: 10.3389/fncel.2022.896172
Eriksson, U. K., Fischer, G., Friemann, R., Enkavi, G., Tajkhorshid, E., Neutze, R., et al. (2013). Subangstrom resolution X-ray structure details aquaporin-water interactions. Science 340, 1346–1349. doi: 10.1126/science.1234306
Faropoulos, K., Polia, A., Tsakona, C., Pitaraki, E., Moutafidi, A., Gatzounis, G., et al. (2021). Evaluation of AQP4/TRPV4 channel co-expression, microvessel density, and its association with peritumoral brain edema in intracranial meningiomas. J. Mol. Neurosci. 71, 1786–1795. doi: 10.1007/s12031-021-01801-1
Frühbeck, G., Balaguer, I., Méndez-Giménez, L., Valent,í, V., Becerril, S., Catalán, V., et al. (2020). Aquaporin-11 contributes to TGF-β1-induced endoplasmic reticulum stress in human visceral adipocytes: role in obesity-associated inflammation. Cells 9:1403. doi: 10.3390/cells9061403
Fukuda, A. M., Adami, A., Pop, V., Bellone, J. A., Coats, J. S., Hartman, R. E., et al. (2013). Posttraumatic reduction of edema with aquaporin-4 RNA interference improves acute and chronic functional recovery. J. Cereb. Blood Flow Metab. 33, 1621–1632. doi: 10.1038/jcbfm.2013.118
Fukuhara, S., Matsuzaki, J., Tsugawa, H., Masaoka, T., Miyoshi, S., Mori, H., et al. (2014). Mucosal expression of aquaporin-4 in the stomach of histamine type 2 receptor knockout mice and Helicobacter pylori-infected mice. J. Gastroenterol. Hepatol. 29, 53–59. doi: 10.1111/jgh.12771
Fushimi, K., Uchida, S., Hara, Y., Hirata, Y., Marumo, F., Sasaki, S., et al. (1993). Cloning and expression of apical membrane water channel of rat kidney collecting tubule. Nature 361, 549–552. doi: 10.1038/361549a0
Galli, M., Hameed, A., Żbikowski, A., and Zabielski, P. (2021). Aquaporins in insulin resistance and diabetes: more than channels! Redox Biol. 44:102027. doi: 10.1016/j.redox.2021.102027
Gao, J., Wang, X., Chang, Y., Zhang, J., Song, Q., Yu, H., et al. (2006). osmotic water permeability by interaction with aquaporin-1. Anal. Biochem. 350, 165–170. doi: 10.1016/j.ab.2006.01.003
Geistlinger, K., Schmidt, J. D. R., and Beitz, E. (2022). Lactic acid permeability of aquaporin-9 enables cytoplasmic lactate accumulation via an ion trap. Life 12:120. doi: 10.3390/life12010120
González-Marrero, I., Hernández-Abad, L. G., González-Gómez, M., Soto-Viera, M., Carmona-Calero, E. M., Castañeyra-Ruiz, L., et al. (2022). Altered expression of AQP1 and AQP4 in brain barriers and cerebrospinal fluid may affect cerebral water balance during chronic hypertension. Int. J. Mol. Sci. 23:12277. doi: 10.3390/ijms232012277
Gorelick, D. A., Praetorius, J., Tsunenari, T., Nielsen, S., and Agre, P. (2006). Aquaporin-11: a channel protein lacking apparent transport function expressed in brain. BMC Biochem. 7:14. doi: 10.1186/1471-2091-7-14
Gorin, M. B., Yancey, S. B., Cline, J., Revel, J. P., and Horwitz, J. (1984). The major intrinsic protein (MIP) of the bovine lens fiber membrane: characterization and structure based on cDNA cloning. Cell 39, 49–59. doi: 10.1016/0092-8674(84)90190-9
Guan, Y., Li, L., Chen, J., and Lu, H. (2020). Effect of AQP4-RNAi in treating traumatic brain edema: Multi-modal MRI and histopathological changes of early stage edema in a rat model. Exp. Ther. Med. 19, 2029–2036. doi: 10.3892/etm.2020.8456
Gunnarson, E., Song, Y., Kowalewski, J. M., Brismar, H., Brines, M., Cerami, A., et al. (2009). Erythropoietin modulation of astrocyte water permeability as a component of neuroprotection. Proc. Natl. Acad. Sci. USA. 106, 1602–1607. doi: 10.1073/pnas.0812708106
Hermo, L., Krzeczunowicz, D., and Ruz, R. (2004). Cell specificity of aquaporins 0, 3, and 10 expressed in the testis, efferent ducts, and epididymis of adult rats. J. Androl. 25, 494–505. doi: 10.1002/j.1939-4640.2004.tb02820.x
Hirt, B., Gleiser, C., Eckhard, A., Mack, A. F., Müller, M., Wolburg, H., et al. (2011). All functional aquaporin-4 isoforms are expressed in the rat cochlea and contribute to the formation of orthogonal arrays of particles. Neuroscience 189, 79–92. doi: 10.1016/j.neuroscience.2011.05.037
Hirt, L., Price, M., Mastour, N., Brunet, J. F., Barrière, G., Friscourt, F., et al. (2018). Increase of aquaporin 9 expression in astrocytes participates in astrogliosis. J. Neurosci. Res. 96, 194–206. doi: 10.1002/jnr.24061
Holm, L. M., Klaerke, D. A., and Zeuthen, T. (2004). Aquaporin 6 is permeable to glycerol and urea. Pflugers Arch. 448, 181–186. doi: 10.1007/s00424-004-1245-x
Hu, S., Exner, C., Sienel, R. I., Wehn, A., Seker, B., Magdane Boldoczki, F., et al. (2023). Characterization of vasogenic and cytotoxic brain edema formation after experimental TBI by free water diffusion MRI. J. Neurotrauma. 41, 393–406. doi: 10.1089/neu.2023.0222
Huang, Y. D., Xia, S. W., Dai, P., and Han, D. Y. (2011). Role of AQP1 in inner ear in motion sickness. Physiol. Behav. 104, 749–753. doi: 10.1016/j.physbeh.2011.07.031
Iena, F. M., Kalucka, J., Nielsen, L., Søndergaard, E., Nielsen, S., Lebeck, J., et al. (2022). Localization of aquaglyceroporins in human and murine white adipose tissue. Histochem. Cell Biol. 157, 623–639. doi: 10.1007/s00418-022-02090-4
Iena, F. M., and Lebeck, J. (2018). Implications of Aquaglyceroporin 7 in Energy Metabolism. Int. J. Mol. Sci. 19:154. doi: 10.3390/ijms19010154
Igarashi, H., Tsujita, M., Kwee, I. L., and Nakada, T. (2014). Water influx into cerebrospinal fluid is primarily controlled by aquaporin-4, not by aquaporin-1: 17O JJVCPE MRI study in knockout mice. Neuroreport 25, 39–43. doi: 10.1097/WNR.0000000000000042
Ikaga, R., Namekata, I., Kotiadis, V. N., Ogawa, H., Duchen, M. R., Tanaka, H., et al. (2015). Knockdown of aquaporin-8 induces mitochondrial dysfunction in 3T3-L1 cells. Biochem. Biophys. Rep. 4, 187–195. doi: 10.1016/j.bbrep.2015.09.009
Iliff, J. J., Wang, M., Liao, Y., Plogg, B. A., Peng, W., Gundersen, G. A., et al. (2012). A paravascular pathway facilitates CSF flow through the brain parenchyma and the clearance of interstitial solutes, including amyloid β. Sci. Transl. Med. 4:147ra.111. doi: 10.1126/scitranslmed.3003748
Ishibashi, K., Morinaga, T., Kuwahara, M., Sasaki, S., and Imai, M. (2002). Cloning and identification of a new member of water channel (AQP10) as an aquaglyceroporin. Biochim. Biophys. Acta. 1576, 335–340. doi: 10.1016/S0167-4781(02)00393-7
Ishibashi, K., Sasaki, S., Fushimi, K., Uchida, S., Kuwahara, M., Saito, H., et al. (1994). Molecular cloning and expression of a member of the aquaporin family with permeability to glycerol and urea in addition to water expressed at the basolateral membrane of kidney collecting duct cells. Proc. Natl. Acad. Sci. USA. 91, 6269–6273. doi: 10.1073/pnas.91.14.6269
Ishibashi, K., Tanaka, Y., and Morishita, Y. (2014). The role of mammalian superaquaporins inside the cell. Biochim. Biophys. Acta. 1840, 1507–1512. doi: 10.1016/j.bbagen.2013.10.039
Jung, J. S., Bhat, R. V., Preston, G. M., Guggino, W. B., Baraban, J. M., Agre, P., et al. (1994). Molecular characterization of an aquaporin cDNA from brain: candidate osmoreceptor and regulator of water balance. Proc. Natl. Acad. Sci. USA. 91, 13052–13056. doi: 10.1073/pnas.91.26.13052
Kim, J., and Jung, Y. (2011). Different expressions of AQP1, AQP4, eNOS, and VEGF proteins in ischemic versus non-ischemic cerebropathy in rats: potential roles of AQP1 and eNOS in hydrocephalic and vasogenic edema formation. Anat. Cell Biol. 44, 295–303. doi: 10.5115/acb.2011.44.4.295
Kim, J. G., Son, Y. J., Yun, C. H., Kim, Y. I., Nam-Goong, I. S., Park, J. H., et al. (2007). Thyroid transcription factor-1 facilitates cerebrospinal fluid formation by regulating aquaporin-1 synthesis in the brain. J. Biol. Chem. 282, 14923–14931. doi: 10.1074/jbc.M701411200
Kimball, E., Schaub, J., Quillen, S., Keuthan, C., Pease, M. E., Korneva, A., et al. (2021). The role of aquaporin-4 in optic nerve head astrocytes in experimental glaucoma. PLoS ONE 16:e0244123. doi: 10.1371/journal.pone.0244123
Kimelberg, H. K. (1995). Current concepts of brain edema: review of laboratory investigations. J. Neurosurg. 83, 1051–1059. doi: 10.3171/jns.1995.83.6.1051
Kirscht, A., Sonntag, Y., Kjellbom, P., and Johanson, U. (2018). A structural preview of aquaporin 8 via homology modeling of seven vertebrate isoforms. BMC Struct. Biol. 18:2. doi: 10.1186/s12900-018-0081-8
Klatzo, I. (1967). Presidental address. Neuropathological aspects of brain edema. J. Neuropathol. Exp. Neurol. 26, 1–14. doi: 10.1097/00005072-196701000-00001
Kleindienst, A., Dunbar, J. G., Glisson, R., and Marmarou, A. (2013). The role of vasopressin V1A receptors in cytotoxic brain edema formation following brain injury. Acta Neurochir. 155, 151–164. doi: 10.1007/s00701-012-1558-z
Koike, S., Tanaka, Y., Matsuzaki, T., Morishita, Y., and Ishibashi, K. (2016). Aquaporin-11 (AQP11) expression in the mouse brain. Int. J. Mol. Sci. 17:861. doi: 10.3390/ijms17060861
Kong, H., Fan, Y., Xie, J., Ding, J., Sha, L., Shi, X., et al. (2008). AQP4 knockout impairs proliferation, migration and neuronal differentiation of adult neural stem cells. J. Cell Sci. 121, 4029–4036. doi: 10.1242/jcs.035758
Krüger, C., Jörns, A., Kaynert, J., Waldeck-Weiermair, M., Michel, T., Elsner, M., et al. (2021). The importance of aquaporin-8 for cytokine-mediated toxicity in rat insulin-producing cells. Free Radic. Biol. Med. 174, 135–143. doi: 10.1016/j.freeradbiomed.2021.08.003
Küppers, E., Gleiser, C., Brito, V., Wachter, B., Pauly, T., Hirt, B., et al. (2008). AQP4 expression in striatal primary cultures is regulated by dopamine–implications for proliferation of astrocytes. Eur. J. Neurosci. 28, 2173–2182. doi: 10.1111/j.1460-9568.2008.06531.x
La Porta, C. A., Gena, P., Gritti, A., Fascio, U., Svelto, M., Calamita, G., et al. (2006). Adult murine CNS stem cells express aquaporin channels. Biol. Cell 98, 89–94. doi: 10.1042/BC20040153
Lambertz, N., Hindy, N. E., Adler, C., Rump, K., Adamzik, M., Keyvani, K., et al. (2013). Expression of aquaporin 5 and the AQP5 polymorphism A(-1364)C in association with peritumoral brain edema in meningioma patients. J. Neurooncol. 112, 297–305. doi: 10.1007/s11060-013-1064-z
Lee, J. S., Cho, W. J., Shin, L., and Jena, B. P. (2010). Involvement of cholesterol in synaptic vesicle swelling. Exp. Biol. Med. 235, 470–477. doi: 10.1258/ebm.2010.009259
Lei, L., Wang, W., Jia, Y., Su, L., Zhou, H., Verkman, A. S., et al. (2017). Aquaporin-3 deletion in mice results in renal collecting duct abnormalities and worsens ischemia-reperfusion injury. Biochim. Biophys. Acta Mol. Basis Dis. 1863, 1231–1241. doi: 10.1016/j.bbadis.2017.03.012
Li, G., Liu, X., Liu, Z., and Su, Z. (2015). Interactions of connexin 43 and aquaporin-4 in the formation of glioma-induced brain edema. Mol. Med. Rep. 11, 1188–1194. doi: 10.3892/mmr.2014.2867
Liao, S., Gan, L., Lv, L., and Mei, Z. (2020). The regulatory roles of aquaporins in the digestive system. Genes Dis. 8, 250–258. doi: 10.1016/j.gendis.2019.12.011
Lichter-Konecki, U., Mangin, J. M., Gordish-Dressman, H., Hoffman, E. P., and Gallo, V. (2008). Gene expression profiling of astrocytes from hyperammonemic mice reveals altered pathways for water and potassium homeostasis in vivo. Glia 56, 365–377. doi: 10.1002/glia.20624
Litman, T., Søgaard, R., and Zeuthen, T. (2009). Ammonia and urea permeability of mammalian aquaporins. Handb. Exp. Pharmacol. 190, 327–358. doi: 10.1007/978-3-540-79885-9_17
Liu, J. Y., Chen, X. X., Chen, H. Y., Shi, J., Leung, G. P., Tang, S. C., et al. (2018). Downregulation of aquaporin 9 exacerbates beta-amyloid-induced neurotoxicity in Alzheimer's disease models in vitro and in vivo. Neuroscience 394, 72–82. doi: 10.1016/j.neuroscience.2018.09.016
Liu, Y. L., Matsuzaki, T., Nakazawa, T., Murata, S., Nakamura, N., Kondo, T., et al. (2007). Expression of aquaporin 3 (AQP3) in normal and neoplastic lung tissues. Hum. Pathol. 38, 171–178. doi: 10.1016/j.humpath.2006.07.015
Liu, Z., Shen, J., Carbrey, J. M., Mukhopadhyay, R., Agre, P., Rosen, B. P., et al. (2002). Arsenite transport by mammalian aquaglyceroporins AQP7 and AQP9. Proc. Natl. Acad. Sci. USA. 99, 6053–6058. doi: 10.1073/pnas.092131899
Lu, H., Zhan, Y., Ai, L., Chen, H., and Chen, J. (2020). AQP4-siRNA alleviates traumatic brain edema by altering post-traumatic AQP4 polarity reversal in TBI rats. J. Clin. Neurosci. 81, 113–119. doi: 10.1016/j.jocn.2020.09.015
Lu, Q., Xiong, J., Yuan, Y., Ruan, Z., Zhang, Y., Chai, B., et al. (2022). Minocycline improves the functional recovery after traumatic brain injury via inhibition of aquaporin-4. Int. J. Biol. Sci. 18, 441–458. doi: 10.7150/ijbs.64187
Manley, G. T., Fujimura, M., Ma, T., Noshita, N., Filiz, F., Bollen, A. W., et al. (2000). Aquaporin-4 deletion in mice reduces brain edema after acute water intoxication and ischemic stroke. Nat. Med. 6, 159–163. doi: 10.1038/72256
Marmarou, C. R., Liang, X., Abidi, N. H., Parveen, S., Taya, K., Henderson, S. C., et al. (2014). Selective vasopressin-1a receptor antagonist prevents brain edema, reduces astrocytic cell swelling and GFAP, V1aR and AQP4 expression after focal traumatic brain injury. Brain Res. 1581, 89–102. doi: 10.1016/j.brainres.2014.06.005
Matsushima, A., Ogura, H., Koh, T., Shimazu, T., and Sugimoto, H. (2014). Enhanced expression of aquaporin 9 in activated polymorphonuclear leukocytes in patients with systemic inflammatory response syndrome. Shock 42, 322–326. doi: 10.1097/SHK.0000000000000218
Medina, Y., Acosta, L., Reppetti, J., Corominas, A., Bustamante, J., Szpilbarg, N., et al. (2021). Lactic acid transport mediated by aquaporin-9: implications on the pathophysiology of preeclampsia. Front. Physiol. 12:774095. doi: 10.3389/fphys.2021.774095
Merves, M., Krane, C. M., Dou, H., Greinwald, J. H., Menon, A. G., Choo, D., et al. (2003). Expression of aquaporin 1 and 5 in the developing mouse inner ear and audiovestibular assessment of an Aqp5 null mutant. J. Assoc. Res. Otolaryngol. 4, 264–275. doi: 10.1007/s10162-002-3033-7
Migliati, E. R., Amiry-Moghaddam, M., Froehner, S. C., Adams, M. E., Ottersen, O. P., Bhardwaj, A., et al. (2010). Na(+)-K (+)-2Cl (-) cotransport inhibitor attenuates cerebral edema following experimental stroke via the perivascular pool of aquaporin-4. Neurocrit. Care. 13, 123–131. doi: 10.1007/s12028-010-9376-8
Mints, M., Hildenbrand, A., Lalitkumar, L. P., Andersson, S., Nielsen, S., Gemzell-Danielsson, K., et al. (2007). Expression of aquaporin-1 in endometrial blood vessels in menorrhagia. Int. J. Mol. Med. 19, 407–411. doi: 10.3892/ijmm.19.3.407
Misawa, T., Arima, K., Mizusawa, H., and Satoh, J. (2008). Close association of water channel AQP1 with amyloid-beta deposition in Alzheimer disease brains. Acta Neuropathol. 116, 247–260. doi: 10.1007/s00401-008-0387-x
Mobasheri, A., Wray, S., and Marples, D. (2005). Distribution of AQP2 and AQP3 water channels in human tissue microarrays. J. Mol. Histol. 36, 1–14. doi: 10.1007/s10735-004-2633-4
Moëlo, C., Quillévéré, A., Le Roy, L., and Timsit, S. (2023). (S)-roscovitine, a CDK inhibitor, decreases cerebral edema and modulates AQP4 and α1-syntrophin interaction on a pre-clinical model of acute ischemic stroke. Glia 72, 322–337. doi: 10.1002/glia.24477
Molinas, A., Turkina, M. V., Magnusson, K. E., Mirazimi, A., and Vikström, E. (2017). Perturbation of wound healing, cytoskeletal organization and cellular protein networks during hazara virus infection. Front. Cell Dev. Biol. 5:98. doi: 10.3389/fcell.2017.00098
Molinas, S. M., Trumper, L., and Marinelli, R. A. (2012). Mitochondrial aquaporin-8 in renal proximal tubule cells: evidence for a role in the response to metabolic acidosis. Am. J. Physiol. Renal Physiol. 303, F458–F466. doi: 10.1152/ajprenal.00226.2012
Montiel, V., Bella, R., Michel, L. Y. M., Esfahani, H., De Mulder, D., Robinson, E. L., et al. (2020). Inhibition of aquaporin-1 prevents myocardial remodeling by blocking the transmembrane transport of hydrogen peroxide. Sci. Transl. Med. 12:eaay2176. doi: 10.1093/ehjci/ehaa946.3665
Mori, S., Kurimoto, T., Miki, A., Maeda, H., Kusuhara, S., Nakamura, M., et al. (2020). Aqp9 gene deletion enhances retinal ganglion cell (RGC) death and dysfunction induced by optic nerve crush: evidence that aquaporin 9 acts as an astrocyte-to-neuron lactate shuttle in concert with monocarboxylate transporters to support rgc function and survival. Mol. Neurobiol. 57, 4530–4548. doi: 10.1007/s12035-020-02030-0
Muroi, S. I., and Isohama, Y. (2021). C-terminal domain of aquaporin-5 is required to pass its protein quality control and ensure its trafficking to plasma membrane. Int. J. Mol. Sci. 22:13461. doi: 10.3390/ijms222413461
Musa-Aziz, R., Chen, L. M., Pelletier, M. F., and Boron, W. F. (2009). Relative CO2/NH3 selectivities of AQP1, AQP4, AQP5, AmtB, and RhAG. Proc. Natl. Acad. Sci. USA. 106, 5406–5411. doi: 10.1073/pnas.0813231106
Nagase, H., Agren, J., Saito, A., Liu, K., Agre, P., Hazama, A., et al. (2007). Molecular cloning and characterization of mouse aquaporin 6. Biochem. Biophys. Res. Commun. 352, 12–16. doi: 10.1016/j.bbrc.2006.10.110
Nagelhus, E. A., Horio, Y., Inanobe, A., Fujita, A., Haug, F. M., Nielsen, S., et al. (1999). Immunogold evidence suggests that coupling of K+ siphoning and water transport in rat retinal Müller cells is mediated by a coenrichment of Kir4.1 and AQP4 in specific membrane domains. Glia 26, 47–54. doi: 10.1002/(sici)1098-1136(199903)26:1<47::aid-glia5>3.0.co;2-5
Nielsen, S., Frøkiaer, J., Marples, D., Kwon, T. H., Agre, P., Knepper, M. A., et al. (2002). Aquaporins in the kidney: from molecules to medicine. Physiol. Rev. 82, 205–244. doi: 10.1152/physrev.00024.2001
Nielsen, S., Smith, B. L., Christensen, E. I., and Agre, P. (1993). Distribution of the aquaporin CHIP in secretory and resorptive epithelia and capillary endothelia. Proc. Natl. Acad. Sci. USA. 90, 7275–7279. doi: 10.1073/pnas.90.15.7275
Öberg, F., Sjöhamn, J., Fischer, G., Moberg, A., Pedersen, A., Neutze, R., et al. (2011). Glycosylation increases the thermostability of human aquaporin 10 protein. J. Biol. Chem. 286, 31915–31923. doi: 10.1074/jbc.M111.242677
O'Donnell, M. E., Tran, L., Lam, T. I., Liu, X. B., and Anderson, S. E. (2004). Bumetanide inhibition of the blood-brain barrier Na-K-Cl cotransporter reduces edema formation in the rat middle cerebral artery occlusion model of stroke. J. Cereb. Blood Flow Metab. 24, 1046–1056. doi: 10.1097/01.WCB.0000130867.32663.90
Ohshiro, K., Yaoita, E., Yoshida, Y., Fujinaka, H., Matsuki, A., Kamiie, J., et al. (2001). Expression and immunolocalization of AQP6 in intercalated cells of the rat kidney collecting duct. Arch. Histol. Cytol. 64, 329–338. doi: 10.1679/aohc.64.329
Ohta, E., Itoh, T., Nemoto, T., Kumagai, J., Ko, S. B., Ishibashi, K., et al. (2009). Pancreas-specific aquaporin 12 null mice showed increased susceptibility to caerulein-induced acute pancreatitis. Am. J. Physiol. Cell Physiol. 297, C1368–C1378. doi: 10.1152/ajpcell.00117.2009
Okuno, K., Taya, K., Marmarou, C. R., Ozisik, P., Fazzina, G., Kleindienst, A., et al. (2008). The modulation of aquaporin-4 by using PKC-activator (phorbol myristate acetate) and V1a receptor antagonist (SR49059) following middle cerebral artery occlusion/reperfusion in the rat. Acta Neurochir. Suppl. 102, 431–436. doi: 10.1007/978-3-211-85578-2_84
Oshio, K., Song, Y., Verkman, A. S., and Manley, G. T. (2003). Aquaporin-1 deletion reduces osmotic water permeability and cerebrospinal fluid production. Acta Neurochir. Suppl. 86, 525–528. doi: 10.1007/978-3-7091-0651-8_107
Oshio, K., Watanabe, H., Song, Y., Verkman, A. S., and Manley, G. T. (2005). Reduced cerebrospinal fluid production and intracranial pressure in mice lacking choroid plexus water channel Aquaporin-1. FASEB J. 19, 76–78. doi: 10.1096/fj.04-1711fje
Papadopoulos, M. C., Manley, G. T., Krishna, S., and Verkman, A. S. (2004). Aquaporin-4 facilitates reabsorption of excess fluid in vasogenic brain edema. FASEB J. 18, 1291–1293. doi: 10.1096/fj.04-1723fje
Papadopoulos, M. C., and Verkman, A. S. (2005). Aquaporin-4 gene disruption in mice reduces brain swelling and mortality in pneumococcal meningitis. J. Biol. Chem. 280, 13906–13912. doi: 10.1074/jbc.M413627200
Park, J., Madan, M., Chigurupati, S., Baek, S. H., Cho, Y., Mughal, M. R., et al. (2021). Neuronal aquaporin 1 inhibits amyloidogenesis by suppressing the interaction between beta-secretase and amyloid precursor protein. J. Gerontol. A Biol. Sci. Med. Sci. 76, 23–31. doi: 10.1093/gerona/glaa068
Pasantes-Morales, H., and Cruz-Rangel, S. (2010). Brain volume regulation: osmolytes and aquaporin perspectives. Neuroscience 168, 871–884. doi: 10.1016/j.neuroscience.2009.11.074
Paul, L., Madan, M., Rammling, M., Chigurupati, S., Chan, S. L., Pattisapu, J. V., et al. (2011). Expression of aquaporin 1 and 4 in a congenital hydrocephalus rat model. Neurosurgery 68, 462–473. doi: 10.1227/NEU.0b013e3182011860
Qiu, B., Li, X., Sun, X., Wang, Y., Jing, Z., Zhang, X., et al. (2014). Overexpression of aquaporin-1 aggravates hippocampal damage in mouse traumatic brain injury models. Mol. Med. Rep. 9, 916–922. doi: 10.3892/mmr.2014.1899
Rash, J. E., Yasumura, T., Hudson, C. S., Agre, P., and Nielsen, S. (1998). Direct immunogold labeling of aquaporin-4 in square arrays of astrocyte and ependymocyte plasma membranes in rat brain and spinal cord. Proc. Natl. Acad. Sci. USA. 95, 11981–11986. doi: 10.1073/pnas.95.20.11981
Rauen, K., Pop, V., Trabold, R., Badaut, J., and Plesnila, N. (2020). Vasopressin V1a receptors regulate cerebral aquaporin 1 after traumatic brain injury. J. Neurotrauma. 37, 665–674. doi: 10.1089/neu.2019.6653
Rizwan Siddiqui, M., Attar, F., Mohanty, V., Kim, K. S., Shekhar Mayanil, C., Tomita, T., et al. (2018). Erythropoietin-mediated activation of aquaporin-4 channel for the treatment of experimental hydrocephalus. Childs. Nerv. Syst. 34, 2195–2202. doi: 10.1007/s00381-018-3865-z
Sadashima, S., Honda, H., Suzuki, S. O., Shijo, M., Aishima, S., Kai, K., et al. (2020). Accumulation of astrocytic aquaporin 4 and aquaporin 1 in prion protein plaques. J. Neuropathol. Exp. Neurol. 79, 419–429. doi: 10.1093/jnen/nlaa010
Saito, K., Kageyama, Y., Okada, Y., Kawakami, S., Kihara, K., Ishibashi, K., et al. (2004). Localization of aquaporin-7 in human testis and ejaculated sperm: possible involvement in maintenance of sperm quality. J. Urol. 172, 2073–2076. doi: 10.1097/01.ju.0000141499.08650.ab
Samarasinghe, R. A., Kanuparthi, P. S., Timothy Greenamyre, J., DeFranco, D. B., and Di Maio, R. (2014). Transient muscarinic and glutamatergic stimulation of neural stem cells triggers acute and persistent changes in differentiation. Neurobiol. Dis. 70, 252–261. doi: 10.1016/j.nbd.2014.06.020
Schmidt, M. J., Rummel, C., Hauer, J., Kolecka, M., Ondreka, N., McClure, V., et al. (2016). Increased CSF aquaporin-4, and interleukin-6 levels in dogs with idiopathic communicating internal hydrocephalus and a decrease after ventriculo-peritoneal shunting. Fluids Barriers CNS. 13:12. doi: 10.1186/s12987-016-0034-1
Seyahian, E. A., Cacciagiu, L., Damiano, A. E., and Zotta, E. (2020). AQP1 expression in the proximal tubule of diabetic rat kidney. Heliyon 6:e03192. doi: 10.1016/j.heliyon.2020.e03192
Shannonhouse, J. L., Urbanski, H. F., Woo, S. L., Fong, L. A., Goddard, S. D., Lucas, W. F., et al. (2014). Aquaporin-11 control of testicular fertility markers in Syrian hamsters. Mol. Cell. Endocrinol. 391, 1–9. doi: 10.1016/j.mce.2014.04.011
Shin, I., Kim, H. J., Lee, J. E., and Gye, M. C. (2006). Aquaporin7 expression during perinatal development of mouse brain. Neurosci. Lett. 409, 106–111. doi: 10.1016/j.neulet.2006.09.075
Silva, P. M., da Silva, I. V., Sarmento, M. J., Silva, Í. C., Carvalho, F. A., Soveral, G., et al. (2022). Aquaporin-3 and aquaporin-5 facilitate migration and cell-cell adhesion in pancreatic cancer by modulating cell biomechanical properties. Cells 11:1308. doi: 10.3390/cells11081308
Skauli, N., Savchenko, E., Ottersen, O. P., Roybon, L., and Amiry-Moghaddam, M. (2022). Canonical bone morphogenetic protein signaling regulates expression of aquaporin-4 and its anchoring complex in mouse astrocytes. Front. Cell. Neurosci. 16:878154. doi: 10.3389/fncel.2022.878154
Sorrentino, I., Galli, M., Medraño-Fernandez, I., and Sitia, R. (2022). Transfer of H2O2 from Mitochondria to the endoplasmic reticulum via Aquaporin-11. Redox Biol. 55:102410. doi: 10.1016/j.redox.2022.102410
Sosa, C., Guillén, N., Lucea, S., and Sorribas, V. (2020). Effects of oral exposure to arsenite on arsenic metabolism and transport in rat kidney. Toxicol. Lett. 333, 4–12. doi: 10.1016/j.toxlet.2020.07.029
Srisook, C., Glaharn, S., Punsawad, C., and Viriyavejakul, P. (2022). Apoptotic changes and aquaporin-1 expression in the choroid plexus of cerebral malaria patients. Malar. J. 21:43. doi: 10.1186/s12936-022-04044-6
Sucha, P., Hermanova, Z., Chmelova, M., Kirdajova, D., Camacho Garcia, S., Marchetti, V., et al. (2022). The absence of AQP4/TRPV4 complex substantially reduces acute cytotoxic edema following ischemic injury. Front. Cell. Neurosci. 16:1054919. doi: 10.3389/fncel.2022.1054919
Sugiyama, Y., Yamazaki, K., Kusaka-Kikushima, A., Nakahigashi, K., Hagiwara, H., Miyachi, Y., et al. (2014). Analysis of aquaporin 9 expression in human epidermis and cultured keratinocytes. FEBS Open Bio. 4, 611–616. doi: 10.1016/j.fob.2014.06.004
Sveinsdottir, S., Gram, M., Cinthio, M., Sveinsdottir, K., Mörgelin, M., Ley, D., et al. (2014). Altered expression of aquaporin 1 and 5 in the choroid plexus following preterm intraventricular hemorrhage. Dev. Neurosci. 36, 542–551. doi: 10.1159/000366058
Takata, T., Hamada, S., Mae, Y., Iyama, T., Ogihara, R., Seno, M., et al. (2022). Uromodulin regulates murine aquaporin-2 activity via thick ascending limb-collecting duct cross-talk during water deprivation. Int. J. Mol. Sci. 23:9410. doi: 10.3390/ijms23169410
Tanimura, Y., Hiroaki, Y., and Fujiyoshi, Y. (2009). Acetazolamide reversibly inhibits water conduction by aquaporin-4. J. Struct. Biol. 166, 16–21. doi: 10.1016/j.jsb.2008.11.010
Taya, K., Gulsen, S., Okuno, K., Prieto, R., Marmarou, C. R., Marmarou, A., et al. (2008). Modulation of AQP4 expression by the selective V1a receptor antagonist, SR49059, decreases trauma-induced brain edema. Acta Neurochir. Suppl. 102, 425–429. doi: 10.1007/978-3-211-85578-2_83
Thrane, A. S., Rappold, P. M., Fujita, T., Torres, A., Bekar, L. K., Takano, T., et al. (2011). Critical role of aquaporin-4 (AQP4) in astrocytic Ca2+ signaling events elicited by cerebral edema. Proc. Natl. Acad. Sci. USA. 108, 846–851. doi: 10.1073/pnas.1015217108
Tourdias, T., Dragonu, I., Fushimi, Y., Deloire, M. S., Boiziau, C., Brochet, B., et al. (2009). Aquaporin 4 correlates with apparent diffusion coefficient and hydrocephalus severity in the rat brain: a combined MRI-histological study. Neuroimage 47, 659–666. doi: 10.1016/j.neuroimage.2009.04.070
Trillo-Contreras, J. L., Ramírez-Lorca, R., Villadiego, J., and Echevarría, M. (2022). Cellular distribution of brain aquaporins and their contribution to cerebrospinal fluid homeostasis and hydrocephalus. Biomolecules 12:530. doi: 10.3390/biom12040530
Uldall, M., Botfield, H., Jansen-Olesen, I., Sinclair, A., and Jensen, R. (2017). Acetazolamide lowers intracranial pressure and modulates the cerebrospinal fluid secretion pathway in healthy rats. Neurosci. Lett. 645, 33–39. doi: 10.1016/j.neulet.2017.02.032
Vajda, Z., Pedersen, M., Füchtbauer, E. M., Wertz, K., Stødkilde-Jørgensen, H., Sulyok, E., et al. (2002). Delayed onset of brain edema and mislocalization of aquaporin-4 in dystrophin-null transgenic mice. Proc. Natl. Acad. Sci. USA. 99, 13131–13136. doi: 10.1073/pnas.192457099
Varadaraj, K., and Kumari, S. S. (2020). Lens aquaporins function as peroxiporins to facilitate membrane transport of hydrogen peroxide. Biochem. Biophys. Res. Commun. 524, 1025–1029. doi: 10.1016/j.bbrc.2020.02.031
Verkerk, A. O., Lodder, E. M., and Wilders, R. (2019). Aquaporin channels in the heart-physiology and pathophysiology. Int. J. Mol. Sci. 20:2039. doi: 10.3390/ijms20082039
Villandre, J., White, V., Lear, T. B., Chen, Y., Tuncer, F., Vaiz, E., et al. (2022). A repurposed drug screen for compounds regulating aquaporin 5 stability in lung epithelial cells. Front. Pharmacol. 13:828643. doi: 10.3389/fphar.2022.828643
Villarán, R. F., de Pablos, R. M., Argüelles, S., Espinosa-Oliva, A. M., Tomás-Camardiel, M., Herrera, A. J., et al. (2009). The intranigral injection of tissue plasminogen activator induced blood-brain barrier disruption, inflammatory process and degeneration of the dopaminergic system of the rat. Neurotoxicology 30, 403–413. doi: 10.1016/j.neuro.2009.02.011
Wang, Q., Li, Y., Wu, C., Wang, T., and Wu, M. (2022). Aquaporin-1 inhibition exacerbates ischemia-reperfusion-induced lung injury in mice. Am. J. Med. Sci. 5:S0002. doi: 10.1016/j.amjms.2022.08.017
Wiegman, M. J., Bullinger, L. V., Kohlmeyer, M. M., Hunter, T. C., and Cipolla, M. J. (2008). Regional expression of aquaporin 1, 4, and 9 in the brain during pregnancy. Reprod. Sci. 15, 506–516. doi: 10.1177/1933719107311783
Wu, Y., Pan, C. Y., Guo, C. Z., Dong, Z. J., Wu, Q., Dong, H. M., et al. (2015). Expression of aquaporin 1 and 4 in rats with acute hypoxic lung injury and its significance. Genet. Mol. Res. 14, 12756–12764. doi: 10.4238/2015.October.19.19
Yamamoto, N., Yoneda, K., Asai, K., Sobue, K., Tada, T., Fujita, Y., et al. (2001). Alterations in the expression of the AQP family in cultured rat astrocytes during hypoxia and reoxygenation. Brain Res. Mol. Brain Res. 90, 26–38. doi: 10.1016/S0169-328X(01)00064-X
Yan, J. H., Khatibi, N. H., Han, H. B., Hu, Q., Chen, C. H., Li, L., et al. (2012). p53-induced uncoupling expression of aquaporin-4 and inwardly rectifying K+ 4.1 channels in cytotoxic edema after subarachnoid hemorrhage. CNS Neurosci. Ther. 18, 334–342. doi: 10.1111/j.1755-5949.2012.00299.x
Yang, B., Song, Y., Zhao, D., and Verkman, A. S. (2005). Phenotype analysis of aquaporin-8 null mice. Am. J. Physiol. Cell Physiol. 288, C1161–C1170. doi: 10.1152/ajpcell.00564.2004
Yang, B., Zador, Z., and Verkman, A. S. (2008a). Glial cell aquaporin-4 overexpression in transgenic mice accelerates cytotoxic brain swelling. J. Biol. Chem. 283, 15280–15286. doi: 10.1074/jbc.M801425200
Yang, B., Zhang, H., and Verkman, A. S. (2008b). Lack of aquaporin-4 water transport inhibition by antiepileptics and arylsulfonamides. Bioorg. Med. Chem. 16, 7489–7493. doi: 10.1016/j.bmc.2008.06.005
Yang, M., Gao, F., Liu, H., Yu, W. H., He, G. Q., Zhuo, F., et al. (2011). Immunolocalization of aquaporins in rat brain. Anat. Histol. Embryol. 40, 299–306. doi: 10.1111/j.1439-0264.2011.01070.x
Yang, X. L., Zeng, M. L., Shao, L., Jiang, G. T., Cheng, J. J., Chen, T. X., et al. (2019). NFAT5 and HIF-1α coordinate to regulate NKCC1 expression in hippocampal neurons after hypoxia-ischemia. Front. Cell Dev. Biol. 7:339. doi: 10.3389/fcell.2019.00339
Yu, B., Zhang, J., Li, H., and Sun, X. (2020). Silencing of aquaporin1 activates the Wnt signaling pathway to improve cognitive function in a mouse model of Alzheimer's disease. Gene 755:144904. doi: 10.1016/j.gene.2020.144904
Zhang, B., Lv, D., Chen, Y., Nie, W., Jiao, Y., Zhang, J., et al. (2022). Aquaporin-9 facilitates liver regeneration following hepatectomy. Redox Biol. 50:102246. doi: 10.1016/j.redox.2022.102246
Zhang, H., Cai, W., and Shao, X. (2021). Regulation of aquaporin-3 water permeability by hyaluronan. Phys. Chem. Chem. Phys. 23, 25706–25711. doi: 10.1039/D1CP02867G
Zhao, L., Luo, Z., Qiu, S., Jia, Y., Zhong, S., Chen, G., et al. (2020). Abnormalities of aquaporin-4 in the cerebellum in bipolar II disorder: an ultra-high b-values diffusion weighted imaging study. J. Affect. Disord. 274, 136–143. doi: 10.1016/j.jad.2020.05.035
Zhou, Z., Zhan, J., Cai, Q., Xu, F., Chai, R., Lam, K., et al. (2022). The water transport system in astrocytes-aquaporins. Cells 11:2564. doi: 10.3390/cells11162564
Zhu, C., Chen, Z., and Jiang, Z. (2016). Expression, distribution and role of aquaporin water channels in human and animal stomach and intestines. Int. J. Mol. Sci. 17:1399. doi: 10.3390/ijms17091399
Keywords: aquaporins, water channels, water transport, brain edema, cytotoxic, vasogenic, hydrocephalus
Citation: Li Y, Wang Y, Huang X, Zhang H, Guan Y and Zhang X (2025) Role of aquaporins in brain water transport and edema. Front. Neurosci. 19:1518967. doi: 10.3389/fnins.2025.1518967
Received: 29 October 2024; Accepted: 13 January 2025;
Published: 29 January 2025.
Edited by:
Paola Bagnoli, University of Pisa, ItalyReviewed by:
Marco Mainardi, University of Padua, ItalyCopyright © 2025 Li, Wang, Huang, Zhang, Guan and Zhang. This is an open-access article distributed under the terms of the Creative Commons Attribution License (CC BY). The use, distribution or reproduction in other forums is permitted, provided the original author(s) and the copyright owner(s) are credited and that the original publication in this journal is cited, in accordance with accepted academic practice. No use, distribution or reproduction is permitted which does not comply with these terms.
*Correspondence: Youfei Guan, guanyf@dmu.edu.cn; Xiaoyan Zhang, xyzhang@hsc.ecnu.edu.cn
†These authors have contributed equally to this work
Disclaimer: All claims expressed in this article are solely those of the authors and do not necessarily represent those of their affiliated organizations, or those of the publisher, the editors and the reviewers. Any product that may be evaluated in this article or claim that may be made by its manufacturer is not guaranteed or endorsed by the publisher.
Research integrity at Frontiers
Learn more about the work of our research integrity team to safeguard the quality of each article we publish.