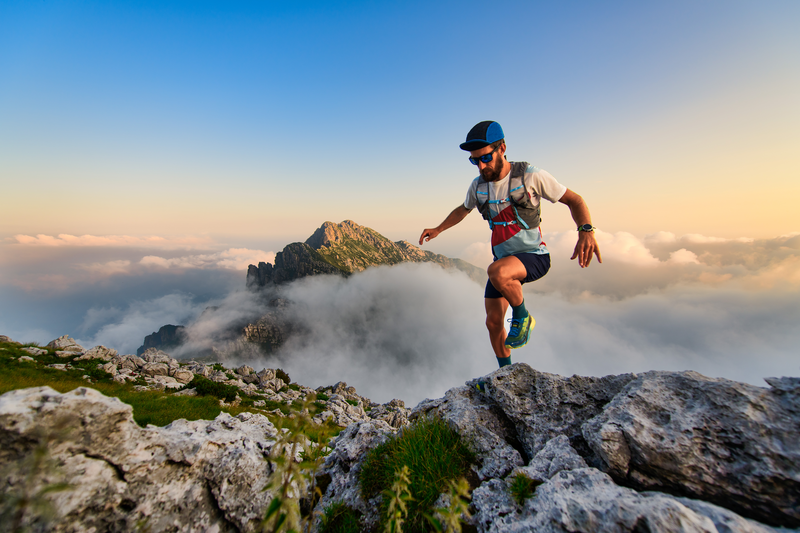
94% of researchers rate our articles as excellent or good
Learn more about the work of our research integrity team to safeguard the quality of each article we publish.
Find out more
ORIGINAL RESEARCH article
Front. Neurosci. , 17 February 2025
Sec. Gut-Brain Axis
Volume 19 - 2025 | https://doi.org/10.3389/fnins.2025.1503176
This article is part of the Research Topic Gut-Brain Axis Correlates, Mediators, and Moderators of Stress Resilience or Vulnerability View all 5 articles
Night shift is a prevalent workstyle in medical hospitals, demanding continuous health monitoring and rapid decision making of medical professionals. Night shifts may cause serious health problems to medical staff, including cognitive impairments, poor sleep, and inflammatory responses, leading to the altered gut-brain axis. However, how night shifts impact gut-brain axis and how long the impact lasts remain to be studied. Hence, we investigated the dynamic changes of brain-microbiota relations following night shifts and subsequent recovery days among medical shift workers. Young medical staffs were recruited for the 3-session assessments over the scheduled night shifts (pre-shift, post-shift, and recovery) by measuring (a) sleep metrics, (b) brain functions, (c) gut bacteriome compositions, and (d) cognitive assessments. Participants experienced partial sleep deprivation only during the 5-day night shifts but rapidly returned to baseline after the 4-day recovery, so as the elevated brain fluctuations in the superior frontal gyrus after night shifts. Meanwhile, the night shifts caused elongated connectivity changes of default-mode and dorsal attention networks without recovery. Nevertheless, we did not find prevailing night-shift effects on cognition and gut bacteriome compositions, except the Gemellaceae concentration and the multi-task performance. Collectively, night shifts may induce prolonged alterations on brain connectivity without impacts on gut bacteriome, suggesting the vulnerable brain functions and the resilient gut bacteriome to the short-term night shifts among medical shift workers.
Shift work, the irregularly arranged work schedule, is prevailing among the specific job types requiring consecutive surveillance and swift decision making, such as the road constructor, medical caregiver, and aircraft traffic controller (Machi et al., 2012; Mélan and Cascino, 2022). It was reported that 15 million American and 1.5 million Australian are employed in a working style of night shifts or rotating shifts (Rajaratnam et al., 2013; Rogers et al., 2021), and the shift workers are generally suffered from the circadian misalignment, poor sleep quality, chronic fatigue and higher risk of dementia (Bokenberger et al., 2018; Gilavand, 2022). In Taiwan, the Taiwan Society of Sleep Medicine (TSSM) reported the prevalence of chronic insomnia among the Taiwanese shift workers was about 23.3%, about 2.18 times higher than the daytime workers (TSSM, 2019). In the Taiwanese medical field, the consecutive rotation of 3–5-night shifts in a row are a common schedule across medical specialties (Chang et al., 2017); however, the prolonged wakefulness and diminished vigilance during the circadian trough easily led the medical staffs to poor performances or even medical errors (Kalmbach et al., 2017; Kang et al., 2020; Qiu et al., 2020). The most prominent and subject drawback of the night shift is the attention impairment, and other domains of cognitive functions are also affected, such as the decision making, emotion and memory (Kecklund and Axelsson, 2016). During the COVID-19 outbreak, the bursts of workloads further elevated the shift-work burdens to the clinical staffs, causing inevitable burnout and fatigue as the results (Xia et al., 2021; Gilavand et al., 2023). These aversive effects of medical shift work not only impact the quality of patient healthcare, but also a risk factor of traffic accident and work-life balance for the medical staffs.
Underlying the cognitive impairments and behavioral risks, previous literature has addressed that the night shift causes various detrimental effects on biology, such as the melatonin depletion, impaired glucose homeostasis, increased pro-inflammatory cytokines and oxidative stress (Kecklund and Axelsson, 2016; Wu et al., 2021). However, such biomarkers could cause the aversive effect on physical health, but indirect to the cognitive impairments following night shifts. The direct cause of the cognitive decline after night shifts is speculated as the neurophysiological factors, such as the brain functions and gut microbial functions. From the angle of brain functions, the shift work may induce abnormal brain functionality (Wu et al., 2021; Tian et al., 2022), regional cerebral blood flow (Park et al., 2019), and decreased cerebrospinal fluid (CSF) volume, leading to the alterations of plasma β-amyloid and tau protein (Ye et al., 2023). For medical staffs, shift works associated with the reduction of long-range functional connectivity in the medial frontal gyrus (part of default-mode network, DMN) (Ye et al., 2022), and lower brain activity/connectivity in the right dorsal attention network (DAN) (Dong et al., 2024). Meanwhile, the gut microbiota and their corresponding metabolites are regarded as the second central nervous system involved in the cognitive performances as well (Mashaqi and Gozal, 2020). In the animal model, abundant evidence disclosed that the circadian-rhythm misalignment could lead to the microbial malfunctions, such as the decreased proportion of Firmicutes and the elevated abundance of Proteobacteria at the phylum level (Hu et al., 2022), involved in dysfunctional glucose homeostasis (Altaha et al., 2022). However, even though literature discloses the key role of the gut-brain axis in cognition and circadian rhythms (Cryan and Dinan, 2012; Teichman et al., 2020; Cai et al., 2021), the quantifiable relationship between brain functions and gut microbial compositions remains to be investigated for shift workers.
Rather than taking place as a switch, the medical staffs usually undergo night shifts in a long-term rotation, which lead to worse cognitive performances (Machi et al., 2012; Sun et al., 2021), and the cognitive decline might not return to the baseline after years (Marquié et al., 2015). Such chronic effect of shift work induces additional question: How many resting days after the night shifts would be sufficient to alleviate the aversive effects of cognitive and neurophysiological impairments? Previously, Belenky et al. (2003) exhibited that the 3 days of normal sleep following a 7-day sleep restriction were insufficient to restore the cognitive impairments, and Brown et al. (2020) also showed that the reduced sleep efficiency during the night shifts might return to normal after 5 days of recovery. From such evidence, it is reasonable to speculate the aversive effects of night shift could prolong as an inertia effect for the consecutive few days with normal circadian rhythms, which awaits to be investigated as well.
Altogether, the research questions intrigued the following two hypotheses: (1) night shifts in medical professionals can lead to alterations of brain functions, gut microbial compositions, and their mutual relations; (2) night-shift-induced changes of brain function and gut microbiota may not quickly recover after few days of normal circadian rhythms. To probe the gut-brain relationship, we conducted a functional magnetic resonance imaging (fMRI) protocol to assess the brain functionality, collected the fecal sample for assessing the gut bacteriome composition and their metabolites, short-chain fatty acids (SCFA), and probed the cognitive performances and sleep metrics among Taiwanese medical staffs in a repeated-measure design (3 time points: pre-shift, post-shift, recovery).
We recruited 15 medical personnel from hospitals in the Taipei metropolitan area, including registered nurses, radiologists, and pharmacists. Participants eligible for the study should meet the following inclusion criteria: (1) age between 20 and 65 years; (2) without any neurological or psychiatric disorders; (3) no history of addictive drug use or habitual alcohol consumption; (4) no antibiotics, probiotics, prebiotics, or antifungal medications for 3 months prior to experiment; (5) not taking any sleeping aids in the past 2 weeks; (6) without pregnancy; (7) without any metal implants incompatible with Magnetic Resonance Imaging (MRI), such as pacemakers, metal pins, or cardiac stents; (8) without claustrophobia; and (9) without any type of intestinal pathogens. The participants were recruited for having a normal schedule of consecutive shift work for at least 4 days with a subsequent three-day resting period after shifts, and all participants provided written informed consents. Procedures in this experiment were approved by the Taipei Medical University - Joint Institutional Review Board (TMU-JIRB N202105081), and we confirmed that all methods in this study were performed in accordance with the relevant guidelines and regulations.
All participants were instructed to visit the MRI center for three times to assess the effects of shift work in a repeated-measure design. The three time points contained (a) before starting their shift work (pre-shift), (b) after working in night shift for at least 4 days (post-shift), and (c) at least 3 days of back-to-normal-sleep after shift days (recovery). We held an experimental briefing 7 days before each participant’s first fMRI experiment. All participants were required to wear actigraphy devices (Philips Respironics Inc., Pittsburgh, Pennsylvania) to measure their sleep metrics, including total sleep time (TST), sleep efficiency (SE), and wake after sleep onset (WASO).
The MRI experiments were scheduled before 2 pm to fulfill the participants’ off-work times and to avoid circadian trough. On the scanning date, participants were requested to avoid consuming any beverages containing caffeine or alcohol 24 h prior to the experiment. In the morning before the scheduled MRI scanning, participants were instructed to collect fecal samples (1 g) properly using the DNA/RNA ShieldTM Fecal Collection Tube (PANGEA laboratory, USA). Fecal samples were stored in tubes containing DNA stabilization buffer to prevent DNA degradation at room temperature. We conducted the MRI experiments at National Taiwan University with a 3T PRISMA scanner (Siemens, Erlangen, Germany) using a 20-channel birdcage head coil. The scanning protocol included one 3D-MPRAGE T1-weighted anatomical image and a resting-state fMRI scan. Parameters for the anatomical 3D-MPRAGE sequence: image dimensions = 256 × 256 × 192; voxel size = 1 × 1 × 1 mm3; repetition time (TR) = 2 s, echo time (TE) = 2.3 msec; flip angle (FA) = 8°; total scan time = 6 min 24 s. The fMRI scans were using single-shot gradient-echo-based echo-planar imaging (GE-EPI) sequence with the following imaging parameters: image dimensions = 64 × 64 × 33; voxel size = 3.44 × 3.44 × 3.4 mm3; TR = 2 s, TE = 32 msec, FA = 77°. The total scan time for resting-state fMRI was 7 min.
After the fMRI experiment, we administered the Cambridge Neuropsychological Test Automated Battery (CANTAB) on an iPad (Apple Inc., California, US) to investigate the overall cognitive performances. We examined four cognitive domains of the CANTAB tasks, including (A) Memory function, including 3 tasks: delayed matching sample (DMS), paired associates learning (PAL), and spatial span (SSP); (B) Psychomotor ability, including reaction time (RTI) task; (C) Emotion and social recognition function, including emotion recognition task (ERT); and (D) Executive function, including multitasking test (MTT), spatial working memory (SWM), and stop signal task (SST). The time to complete the entire CANTAB examinations was approximately 1 h.
SPM 12 and CONN toolbox were used for image preprocessing, seed-based connectivity analysis, and region of interest (ROI) analysis. Functional and anatomical data were preprocessed using a flexible preprocessing pipeline including smoothing. Anatomical data were normalized into standard MNI space, segmented into grey matter, white matter, and CSF tissue classes, and resampled to 1-mm isotropic voxels using SPM unified segmentation and normalization algorithm with the default IXI-549 tissue probability template. Functional data were smoothed using spatial convolution with a Gaussian kernel of 6 mm full-width half maximum (FWHM). In addition, functional data were denoised using a standard denoising pipeline including the regression of potential confounding effects characterized by white matter, CSF, motion parameters and their first order derivatives, outlier scans, and linear trends (2 factors) within each functional run, followed by bandpass frequency filtering of the BOLD time series between 0.008 Hz and 0.09 Hz. From the number of noise terms included in this denoising strategy, the average degrees of freedom of the BOLD signal after denoising were estimated to be 482.3 (range from 182.4 to 549.7) across all subjects.
Two indices of brain functionality were chosen to estimate the shift-work effects: amplitude of low-frequency fluctuation (ALFF) and seed-based connectivity (SBC) analysis to estimate the patterns of spontaneity fluctuations and functional connectivity (FC), respectively, within the 164 ROIs of HPC-ICA and Harvard-Oxford templates (Whitfield-Gabrieli and Nieto-Castanon, 2012). FC strength was represented by Fisher-transformed bivariate correlation coefficients from a weighted general linear model (GLM), defined separately for each pair of seed areas, modeling the association between their BOLD signal time series. Targeting on the two brain networks, we prescribed the two seed regions, posterior cingulate cortex (PCC) and left intraparietal sulcus (IPS), for DMN and DAN, respectively. Group analysis was carried out using a second-level GLM, in which voxel-level hypotheses were evaluated using multivariate parametric statistics with random effects across subjects and sample covariance estimation. Inferences were performed at the level of individual clusters (groups of contiguous voxels), based on parametric statistics from Random Field theory. Results were presented using an FDR-corrected p < 0.05 with cluster-size (k) thresholds (kALFF ≥ 77 mm3; kDMN ≥ 108 mm3; kDAN ≥ 100 mm3).
Within 3 h following the stool sample collection, bacterial DNA was extracted using the Qiagen DNA Mini Prep kit (Qiagen, Hilden, Germany) and stored at −80°C until further processing. Next, in the Joint Human Biobank at Taipei Medical University, the 16S rRNA gene was analyzed following Illumina’s recommended protocol (Illumina, San Diego, CA, USA). The v3–v4 region of the bacterial 16S rRNA gene was amplified to construct a DNA library. Dual-index tags and Nextera XT sequencing adapters (Illumina, San Diego, CA, USA) were added to the amplicons. The DNA quality and quantity were assessed using a QSep 100 analyzer (BiOptic, Taipei, Taiwan), and high-throughput sequencing was performed on an Illumina MiSeq 2000 sequencer. OTU identification and taxonomy assignment followed the methodology described by Callahan et al. (2016). Sequence resolution and accuracy were improved using the DADA2 R package (v1.14.1). Taxonomy was assigned based on the SILVA database (v138) with a minimum bootstrap confidence level of 80 (Quast et al., 2013). Multiple sequence alignments were performed using DECIPHER (v2.14.0), and a phylogenetic tree was constructed using phangorn (v2.5.5) (Schliep, 2010). The resulting count table, taxonomy assignments, and phylogenetic tree were integrated and visualized using phyloseq (v1.30.0) (McMurdie and Holmes, 2013).
Since the gene sequencing did not encompass other microbes (archaea, fungi, viruses), we refer our findings as gut bacteriome instead of gut microbiome. Gut bacterial community diversity across the three repeated measures (pre-shift, post-shift, and recovery) was assessed using the phyloseq and vegan (v2.5.6) R packages. Alpha diversity indices, including observed OTUs, Shannon diversity index, Simpson diversity index, and Chao1 richness estimator, were calculated to measure richness, evenness, and overall diversity. Statistical comparisons of alpha diversity between groups were conducted using t-tests and Wilcoxon tests. For beta diversity, principal coordinate analysis (PCoA) based on UniFrac distances was performed using Adonis from the vegan package to assess differences in OTU composition across the time points. Differential abundance analyses for individual OTUs were conducted using Kruskal–Wallis and Wilcoxon tests with UniFrac (v1.1), with significance set at p < 0.05 (Chen et al., 2012).
For SCFA, we homogenized 50 mg of human fecal sample in 0.5 mL of phosphate-buffered saline (0.5%) and extracted the supernatant. Subsequently, we added an equal volume of ethyl acetate (EA) and mixed thoroughly, followed by centrifugation at 14,800 rpm for 15 min. We injected 1 μL the supernatant into Gas Chromatography–Mass Spectrometry (GC/MS) for SCFA analysis, leading to determination of the contents, including acetate, propionate, and butyrate.
R software (4.3.3) and R Studio (2023.12.1 + 402) were used for data analysis after the ROI extraction. We performed one-way repeated-measure analysis of variance (ANOVA) for between-timepoint comparison on cognition, brain functions, and gut bacteriome with post-hoc tests for pairwise comparison, where the statistical significance was set as p < 0.05 with Bonferroni correction. Furthermore, to investigate the gut-brain relationship, the Pearson correlation analysis across the 3 repeated measures were conducted between the indices of brain function and gut bacteriome, with false discovery rate correction (FDR-corrected p < 0.05).
Ten out of 15 recruited participants completed the entire procedure of three repeated measures and achieved all the requirements in night shifts. The averaged time spans of the shift-work and recovery days were listed in Table 1. The sleep metrics during the experimental procedure was shown in Figure 1. After the shift work, it was prominent that the TST reduced from the original 420.6 ± 123.6 min to 350.5 ± 111.1 min, and rebounded back to 440.7 ± 185.4 min in recovery days, indicating a consequence of partial sleep deprivation during the shift work (F2,145 = 5.12, p = 0.007). However, the SE did not show significant changes along the three measures (F2,145 = 0.53, p = 0.59), so as the WASO (F2,145 = 0.21, p = 0.81). Most of the assessed cognitive functions (i.e., memory function, psychomotor ability, and emotion recognition) did not show significant time effects (F2,27 < 2.61, p > 0.10) except the MTT task in the executive function. The MTT exhibited the reduced reaction time in recovery session as compared with that in pre-shift (Bonferroni-corrected p < 0.002, Supplementary Figure S1).
Figure 1. Sleep metrics over the consecutive night shifts (before, after and recovery) in medical staff (n = 10). (A) total sleep time; (B) sleep efficiency; (C) wake after sleep onset (WASO). One-way repeated-measure ANOVA and post-hoc tests with Bonferroni correction are used for cross-session comparisons (*p < 0.05 in post-hoc tests).
To evaluate the night-shift effects on brain functionality, we specifically assessed the ALFF across the 3 repeated measures (Figure 2A). The superior frontal gyrus (SFG) exhibited a significant time effect on ALFF (F1.2,16.5 = 19.16, p < 0.001, ηp2 = 0.36), where the post hoc tests, Bonferroni adjusted, revealed that the medical staff had elevated ALFF after shift work (p = 0.022) but returned to the baseline after recovery (p < 0.001), while no significant differences was found between the pre-shift and recovery sessions (p = 0.21). Figures 2B,C exhibit the FC changes across the 3 measures over the shift work, targeting on the two networks: DMN and DAN, respectively. In DMN (Figure 2B), we observed the time effect on the FC between PCC and thalamus (FCPCC-thalamus, F2,27 = 16.63, p < 0.001, ηp2 = 0.53), indicating that a significant reduction of FCPCC-thalamus in the post-shift session (pre-shift vs. post-shift: p < 0.001) remained low in the recovery days (post-shift vs. recovery: p = 0.65). In DAN (Figure 2C), the time effect was found on the FC between the IPS and the precentral gyrus (PreCG) (F2,27 = 24.79, p < 0.001, ηp2 = 0.31), indicating an elevated FCIPS-PreCG in the post-shift session (pre-shift vs. post-shift: p < 0.01).
Figure 2. Brain functionality over the consecutive night shifts (before, after and recovery) in medical staff. (A) ALFF; (B) FC of default-mode network (DMN, seeding at posterior cingulate cortex) connecting to bilateral thalamus; (C) FC of dorsal attention network (DAN, seeding at left intra-parietal sulcus) connecting to the precentral gyrus. One-way repeated-measure ANOVA and post-hoc tests with Bonferroni correction are used for cross-session comparisons. (*p < 0.05, **p < 0.01, and ***p < 0.001 in post-hoc tests).
We estimated the gut bacterial composition across the repeated measures through the analysis of alpha and beta diversity. Figure 3A exhibits that no significant time effect was presented among the number of Observed species (F2,27 = 0.54, p = 0.59), the Chao1 index (F2,27 = 0.79, p = 0.47), and the Simpson diversity (F2,27 = 2.58, p = 0.10). Only the Shannon diversity exhibited significant variation (F2,27 = 4.95, p = 0.019), indicating a lowered diversity after night shifts and recovery; however, no significant difference was shown in the follow-up post hoc tests with Bonferroni correction. In beta diversity, the principal coordinate analysis (PCoA) based on variance adjusted with weighted UniFrac index (Figure 3B) exhibited no significant difference in gut bacterial composition across repeated measures, and the relative abundance of the top 200 in both Phylum and Family levels also exhibited insignificant difference across sessions (Supplementary Figure S3). We subsequently estimated bacterial phyla abundances across three time points, but still no significant time effects were observed in all tested bacterial phyla, including Actinobacteriota, Bacteroidota, Campilobacterota, Desulfobacterota, Firmicutes, Fusobacteriota, Patescibacteria, Proteobacteria, Synergistota, and Verrucomicrobiota (Supplementary Figure S2). Notably, the Firmicutes/Bacteroidota ratio (F/B ratio) also demonstrated non-significant time effect (F2,27 = 1.58, p = 0.23, and ηp2 = 0.03). Across the 56 families, only the Gemellaceae showed significant time effect (F2,27 = 4.40, p = 0.028) with significant post-hoc comparison (pre-shift vs. recovery: p = 0.023, Figure 3C). In SCFA, Supplementary Figure S4 shows the boxplots of SCFAs across the 3 repeated measures, and no significant changes was found for all 3 types of SCFA (F2,24 < 1.92, p > 0.18).
Figure 3. Diversity of gut bacteriome over the consecutive night shifts (before, after and recovery) in medical staff. (A) Alpha diversity; (B) Beta diversity based on weighted UniFrac PCoA plots; (C) Concentration of Gemellaceae in the Family level. One-way repeated-measure ANOVA and post-hoc tests with Bonferroni correction are used for cross-session comparisons. (NS: none significance, and *p < 0.05 in post-hoc tests).
The correlation between the FC and gut bacteriome composition were utilized to represent the gut-brain associations across the 3 measures over the shift-work period. No significant difference was found between brain FC and alpha diversity indices in the correlation analysis with FDR correction (Z < 1.96, p > 0.05). In assessing the bacterial phyla abundance, we noticed that the original positive relationship between FCPCC-thalamus and Bacteroidota (r = 0.43) turned into a negative correlation during the post-shift (r = −0.62), and returned back to a positive correlation (r = 0.22) in the recovery session (Figure 4A). Similarly, the relationship between the FCPCC-thalamus and Proteobacteria also exhibited the positive–negative–positive associations across the 3 time points (rpre-shift: rpost-shift: rpost-rest = 0.17: −0.68: 0.22) (Figure 4B).
Figure 4. Relationship between brain FC and gut bacteriome over the consecutive night shifts in medical staff. (A) Association between FCPCC-thalamus and Bacteroidota; (B) Association between FCPCC-thalamus and Proteobacteria. The three repeated measures are marked in different colors—pre-shift (green), post-shift (red), and recovery (yellow). Pearson correlation analysis is conducted across the 3 sessions with false discovery rate correction (*FDR-corrected p < 0.05).
We investigated whether the night-shift workstyle impacts the cognition, brain function, and gut bacteriome among Taiwanese medical professionals along the 3 repeated measures (pre-shift, post-shift, and recovery). Partial sleep deprivation was noticed after the 5-day night shifts (Figure 1). The fMRI results disclosed that the brain functionality (ALFF, DAN and DMN) was changed after the night-shift work. However, the assessments in the gut bacteriome (alpha/beta diversity, phyla abundance and SCFA) did not show significant changes along the 3 time points, so as most the cognition domains (except executive function, Supplementary Figure S1). These observations imply that the circadian misalignments due to night shifts showed profound and immediate impacts on the brain functionality, but the majority of cognition and gut bacteriome were not swiftly affected among the medical professionals. At last, after recovery from the night-shift works, the ALFF indeed recovered back to the pre-shift state in the recovery session (Figure 2A), and so as the gut-brain relationships (Figure 4). The only index remained impaired in the recovery session was the FCPCC-thalamus of DMN (Figure 2B), implying that more than 4 days of circadian realignment was insufficient to provide a full recovery on specific brain functionality.
ALFF is one frequency-specific index to represent the amplitude of spontaneous brain activity (Yang et al., 2007), through which a recent study disclosed a frontal ALFF reduction among nurses working long-term shifts (Dong et al., 2024). Previous studies also disclosed that ALFF or fractional ALFF (fALFF) in the frontal lobe could change with the sleep deprivation or insomnia. For example, Wang et al. demonstrated reduced ALFF in the middle frontal gyrus under a 24-h total sleep deprivation in healthy young adults (Wang et al., 2016), but Nechifor et al. exhibited an elevated fALFF in the SFG after a partial sleep deprivation (Nechifor et al., 2020). In the previous work of paradoxical insomnia, we reported an elevated ALFF in the SFG for those who had sleep-state misperception (Hsiao et al., 2018), denoting a hyperarousal in SFG. In the current study, we detected another scenario of enhanced ALFF under the night-shift workstyle, which may link to the frontal hyperarousal under the involuntary sustenance of wakefulness during night shifts. However, different from Wang’s observation of reduced ALFF, we speculated that the ALFF changes in the partial sleep deprivation (e.g., after night shifts in this study) was distinctive to those in the total sleep deprivation. An additional correlation analysis (Supplementary Figure S5) illustrated that the ALFFSFG exhibited positive correlations with PSQI total scores (r > 0.31) across the 3 measures, especially significant in the recovery session (r = 0.66, p = 0.038). Further investigations are warranted to disclose the ALFF alterations in sleep deprivation and shift work.
FCDMN and FCDAN are associated with the network functions of self-referential and sustained attention, respectively. Literature reported that the shift workers had dysfunctions of both networks, leading to cognitive impairments, especially in the sustained attention (Chellappa et al., 2018; Zhao et al., 2023; Dong et al., 2024). The rationale of network selection leads us to the current findings that the inter-network connections of DMN and DAN were indeed impacted after the consecutive night shifts and they did not return to the baseline even after 4 recovery days to their normal circadian rhythms. Compared with the quick response of ALFF, the FCIPS-PreCG of DAN in the recovery session might be half way returning to the baseline, leading to insignificant results compared to the other two sessions (Figure 2C). Relatively, the recovery speed of FCPCC-thalamus (DMN) was even more sluggish where the aversive impact by the night-shift work sustained after multiple recovery days (Figure 2B). Specifically, the functionality of FCPCC-thalamus was referred to the self-awareness. Boveroux et al. conducted the propofol-induced anesthesia associated with the reduction of FCPCC-thalamus (Boveroux et al., 2010), and our previous work also illustrated the relation between the reduced FCPCC-thalamus and the pre-sleep fatigue (Tsai et al., 2014). Meanwhile, the elevated FCIPS-PreCG could be associated with a higher anxiety level (Huang et al., 2021) or with a sleep deprivation condition (Kong et al., 2018), where it could be intuitively linked to a higher vigilance on their motor function during night shifts. Altogether, the dynamic pattern of DAN/DMN connectivity in medical professionals could imply an enhanced anxiety condition, ready to handle plausible medical emergencies, while the consciousness is dissipating during the night-shift work, and such intense mental process did not return even after 4 days of rest.
One concern is raised that the changes of brain functional indices may originate from the instability over the three consecutive MRI measures. To evaluate the stability of the three functional indices without the circadian misalignment, we applied the same imaging protocol to additional seven young participants with regular sleep–wake schedule over 10-day time span. Based on repeated-measure ANOVA, the three functional indices of the control group exhibited stability over the observable time window. Please see Supplementary Figure S6 for statistical details.
Most of the cognitive tasks tested remained unaffected across the three time points, in contrast to reports in the literature (Rajaratnam et al., 2013; Kecklund and Axelsson, 2016). The unusual observations imply that the Taiwanese medical professionals adapted to bear burden to prevent from medical error, even under consecutive night shifts. One study with a similar design of consecutive 4-night shifts disclosed that the cognitive impairments occurred on the first night of the night shifts, but the nurses gradually regained their cognitive performances in the nocturnal worktime (Chang et al., 2017). This performance adaptation could be one reason for the resilience to the circadian misalignment among the medical personnel. On the other hand, the other possible reason would be the chronic effect of shift work on cognition, especially for the medical personnel working in a repetitive shift schedule. Marquié et al. (2015) reported that the recovery of cognitive function occurs around 5 years after the cessation of any form of shift work, which implies that the medical shift workers may experience consistent and sustained cognitive decline due to their workstyle. Of course, the last influencing factor in the current study would be the limited sample size; therefore, further studies are warranted to prove whether the cognitive functions are affected by shift works.
Additional issue beyond our expectation would be the unchanged gut bacteriome composition across repeated measures. Literature has shown that circadian dysrhythmia, such as the night shift, negatively influences microbiota communities in the gastrointestinal tract, potentially disrupting energy homeostasis, activating pro-inflammatory pathways, and inducing systemic metabolic syndrome in high-risk populations (Bishehsari et al., 2020). Nevertheless, inconsistent with the speculation, the unaffected gut bacteriome in the current work could be due to insufficient time duration to induce the biological dysbiosis of gut bacteriome. Previously, Liu et al. (2020) conducted similar repeated-measure design on healthy adults and found that acute sleep–wake cycle shift did not cause influences on the alpha and beta diversity of the gut microbiota. The finding of no overt changes in gut microbial abundance and diversity following circadian or sleep shifts was consistent with previous studies (Benedict et al., 2016; Zhang et al., 2017), indicating a resilience of microbial eubiosis in human studies. Interestingly, in rodent models, the sleep deprivation could cause detrimental effects on the gut microbiota compositions. Altaha et al. (2022) conducted a 24-h central clock disruption in mice to mimic shift work and found the dysfunctional arrhythmicity of microbial functions. We conducted a 72-h total sleep deprivation in C57BL/6 J mice and found the dramatic reduction of alpha and beta diversity of microbiota, along with the body-weight decline and increased anxiety behavior (Yang et al., 2023). However, while we conducted a partial sleep deprivation (10 h per day, 8 am to 6 pm) extended to 28 consecutive days, the alpha diversity index of Shannon and Simpson did not show much disparities as compared to the control group (Tung et al., 2024), indicating different mechanisms affecting microbiota between the chronic and acute sleep deprivation.
The Gemellaceae is the only family that showed time effect among the 56-family analysis (Figure 3C). Level of Gemellaceae significantly decreased after the recovery session compared with the pre-shift session. Komanduri et al. (2021) reported that the concentration of Gemellaceae positively correlated with speed of memory and power of attention in a human aging study, and significant reduction of Gemellaceae were found with frailty in neurodegenerative aging (Borrego-Ruiz and Borrego, 2024). Considering the Gemellaceae is a family member of Firmicutes, a dominant phylum in most human gut environment, it may play an important role in mediating metabolism through regulating SCFA production (Houtman et al., 2022). However, singular family changes seemed unable to change the equilibrium status in the phylum level, based on the insignificant distinctions in Firmicutes after the night shifts.
This is a pioneer study presenting the dynamic neuroimaging changes of gut-brain association. Although we did not find the significant associations between microbial diversity and brain connectivity across the 3 measures of shift work, the correlation alterations between the FCDMN and Bacteroidota and Proteobacteria (Figure 4) demonstrated the gut-brain association could change after the circadian realignments within the time span of a week. Since FCPCC-thalamus possibly associates with the declined consciousness, the negative correlation in post-shift (red line in Figure 4A) indicates a higher abundance of Bacteroidota and Proteobacteria in response to the progressively decreased FCPCC-thalamus. This could be a mechanism originated from the brain malfunction after consecutive night shifts, propagating to alter the gut bacteriome as a compensatory role in the gut-brain axis. However, this effect is supposed to be a one-way direction from brain to gut, because the Bacteroidota and Proteobacteria might not accumulate sufficiently over the shift-work duration to feedback to the brain in the current design, evidenced by the unchanged SCFA (Supplementary Figure S4). In the recovery session, the brain-bacteriome association returned back to a positive correlation, even though the FCPCC-thalamus remained in a low level.
In the current study, the inability to control the food habit was the major confounds affecting our results. Literature has shown that various dietary choices have significant impacts on the stability and functionality of the microbial community (Ross et al., 2024), so the control of dietary habit may alleviate the confounding factor. However, under the high-stress workload in night shifts, the recruited participants declined to comply with the request of maintaining consistent dietary habit. Considering an enormous between-subject variability in food habits, it is suggested to further record the food diary as the covariates for further investigations.
This study contained several limitations. First, the insignificant findings of cognition and gut bacteria may come from the small sample size in statistics, because the arduous procedures in fMRI, fecal-sample collection and neuropsychological assessments refrained the participating willingness of medical shift workers. We plan to enlarge the sample size in the near future to further illustrate the relationship between gut and brain. Second, from a perspective of clinical practices, it is essential to consider the length of shift work experience, given the diversity of shift work schedules and individual experiences. During our data collection, we observed large variability in shift patterns across different hospitals and departments around Taipei area. Distinctive combination of day shifts, night shifts, and days off can present varying levels of fatigue and recovery. This variability may contribute to discrepancies between our results and those of previous rodent trials conducted under strict control. Third, the senior medical staff members are not required to undertake numerous overnight shifts in Taiwan, stemming from a collegial understanding of their family responsibilities. Therefore, most late-night shifts are typically assigned to less experienced, junior, unmarried medical personnel, preserving more daytime working hours for senior team members. Such medical culture led to a potential difficulty for recruiting senior medical staff as the participant in the repeated-measure design over a night-shift workstyle.
Medical professionals in Taiwan medical fields commonly experience a regular night-shift rotations, where such regular circadian misalignment may deteriorate cognitive performances but their biological mechanism remained elusive. Hence, we hypothesized that the night shift work may perturb the medical shift workers’ brain function, gut bacteriome composition and the gut-brain association. The three repeated measures disclosed that the brain DAN/DMN connectivity changed dynamically, implying the enhanced anxiety at the consciousness dissipation during the night-shift work periods in medical staff. However, the evaluations of the gut bacteriome composition and majority of cognitive functions did not change significantly before and after the night shifts, even after couple days with normal sleep–wake pattern. The unaffected cognition may indicate a good resilience in medical personnel to the night-shift workstyle, and the unchanged gut bacteriome composition may indicate that the five-day night shifts with partial sleep deprivation was insufficient to induce microbial dysbiosis.
The raw data supporting the conclusions of this article will be made available by the authors, without undue reservation.
The studies involving humans were approved by the Taipei Medical University – Joint Institutional Review Board (TMU-JIRB N202105081). The studies were conducted in accordance with the local legislation and institutional requirements. The participants provided their written informed consent to participate in this study.
TY: Data curation, Formal analysis, Methodology, Writing – review & editing, Writing – original draft. Y-PC: Investigation, Supervision, Validation, Writing – review & editing. C-MH: Investigation, Supervision, Validation, Writing – review & editing. H-CL: Funding acquisition, Project administration, Writing – review & editing. C-YL: Project administration, Writing – review & editing. K-WL: Data curation, Writing – review & editing. A-LH: Writing – review & editing. Y-TT: Investigation, Methodology, Project administration, Supervision, Validation, Writing – review & editing. CW: Conceptualization, Formal analysis, Funding acquisition, Methodology, Resources, Supervision, Visualization, Writing – original draft, Writing – review & editing.
The author(s) declare that financial support was received for the research, authorship, and/or publication of this article. This research was supported by National Health Research Institutes (NHRI-EX113-11129EI), National Science and Technology Council (NSTC 113-2813-C-038-074-B) and Higher Education Sprout Project by the Ministry of Education (MOE) in Taiwan (DP2-TMU-113-N-06); the Center for Intelligent Drug Systems and Smart Bio-devices (IDS2B) from The Featured Areas Research Center Program.
The authors would like to thank the Imaging Center for Integrated Body, Mind and Culture Research, National Taiwan University in support of MRI facilities.
The authors declare that the research was conducted in the absence of any commercial or financial relationships that could be construed as a potential conflict of interest.
The authors declare that no Gen AI was used in the creation of this manuscript.
All claims expressed in this article are solely those of the authors and do not necessarily represent those of their affiliated organizations, or those of the publisher, the editors and the reviewers. Any product that may be evaluated in this article, or claim that may be made by its manufacturer, is not guaranteed or endorsed by the publisher.
The Supplementary material for this article can be found online at: https://www.frontiersin.org/articles/10.3389/fnins.2025.1503176/full#supplementary-material
Altaha, B., Heddes, M., Pilorz, V., Niu, Y., Gorbunova, E., Gigl, M., et al. (2022). Genetic and environmental circadian disruption induce weight gain through changes in the gut microbiome. Mol. Metab. 66:101628. doi: 10.1016/j.molmet.2022.101628
Belenky, G., Wesensten, N. J., Thorne, D. R., Thomas, M. L., Sing, H. C., Redmond, D. P., et al. (2003). Patterns of performance degradation and restoration during sleep restriction and subsequent recovery: a sleep dose-response study. J. Sleep Res. 12, 1–12. doi: 10.1046/j.1365-2869.2003.00337.x
Benedict, C., Vogel, H., Jonas, W., Woting, A., Blaut, M., Schürmann, A., et al. (2016). Gut microbiota and glucometabolic alterations in response to recurrent partial sleep deprivation in normal-weight young individuals. Mol. Metab. 5, 1175–1186. doi: 10.1016/j.molmet.2016.10.003
Bishehsari, F., Voigt, R. M., and Keshavarzian, A. (2020). Circadian rhythms and the gut microbiota: from the metabolic syndrome to cancer. Nat. Rev. Endocrinol. 16, 731–739. doi: 10.1038/s41574-020-00427-4
Bokenberger, K., Sjölander, A., Aslan, A. K. D., Karlsson, I. K., Åkerstedt, T., and Pedersen, N. L. (2018). Shift work and risk of incident dementia: a study of two population-based cohorts. Eur. J. Epidemiology 33, 977–987. doi: 10.1007/s10654-018-0430-8
Borrego-Ruiz, A., and Borrego, J. J. (2024). Influence of human gut microbiome on the healthy and the neurodegenerative aging. Exp. Gerontol. 194:112497. doi: 10.1016/j.exger.2024.112497
Boveroux, P., Vanhaudenhuyse, A., Bruno, M.-A., Noirhomme, Q., Lauwick, S., Luxen, A., et al. (2010). Breakdown of within- and between-network resting state functional magnetic resonance imaging connectivity during propofol-induced loss of consciousness. Anesthesiology 113, 1038–1053. doi: 10.1097/aln.0b013e3181f697f5
Brown, C., Abdelrahman, T., Lewis, W., Pollitt, J., Egan, R., Abdulal, S., et al. (2020). To bed or not to bed: the sleep question? Postgrad. Méd. J. 96, 520–524. doi: 10.1136/postgradmedj-2018-135795
Cai, H., Wang, C., Qian, Y., Zhang, S., Zhang, C., Zhao, W., et al. (2021). Large-scale functional network connectivity mediate the associations of gut microbiota with sleep quality and executive functions. Hum. Brain Mapp. 42, 3088–3101. doi: 10.1002/hbm.25419
Callahan, B. J., McMurdie, P. J., Rosen, M. J., Han, A. W., Johnson, A. J. A., and Holmes, S. P. (2016). DADA2: high-resolution sample inference from Illumina amplicon data. Nat. Methods 13, 581–583. doi: 10.1038/nmeth.3869
Chang, Y.-S., Wu, Y.-H., Chen, H.-L., and Hsu, C.-Y. (2017). Four night shifts have a degree of performance adaptation. Hum. Factors. J. Hum. Factors Ergon. Soc. 59, 925–936. doi: 10.1177/0018720817711285
Chellappa, S. L., Morris, C. J., and Scheer, F. A. J. L. (2018). Daily circadian misalignment impairs human cognitive performance task-dependently. Sci. Rep. 8:3041. doi: 10.1038/s41598-018-20707-4
Chen, J., Bittinger, K., Charlson, E. S., Hoffmann, C., Lewis, J., Wu, G. D., et al. (2012). Associating microbiome composition with environmental covariates using generalized UniFrac distances. Bioinformatics 28, 2106–2113. doi: 10.1093/bioinformatics/bts342
Cryan, J. F., and Dinan, T. G. (2012). Mind-altering microorganisms: the impact of the gut microbiota on brain and behaviour. Nat. Publ. Group 13, 701–712. doi: 10.1038/nrn3346
Dong, Y., Wu, X., Zhou, Y., and Qiu, K. (2024). Differences in functional activity and connectivity in the right Frontoparietal network between nurses working Long-term shifts and fixed day shifts. J. Integr. Neurosci. 23:9. doi: 10.31083/j.jin2301009
Gilavand, A. (2022). The effect of shift work on burnout and occupational fatigue among clinical faculty members during the COVID-19 pandemic. Front. Public Heal. 10:973690. doi: 10.3389/fpubh.2022.973690
Gilavand, A., Jafarian, N., and Zarea, K. (2023). Evaluation of medication errors in nursing during the COVID-19 pandemic and their relationship with shift work at teaching hospitals: a cross-sectional study in Iran. Front. Med. 10:1200686. doi: 10.3389/fmed.2023.1200686
Houtman, T. A., Eckermann, H. A., Smidt, H., and Weerth, C. (2022). Gut microbiota and BMI throughout childhood: the role of firmicutes, bacteroidetes, and short-chain fatty acid producers. Sci. Rep. 12:3140. doi: 10.1038/s41598-022-07176-6
Hsiao, F.-C., Tsai, P. J., Wu, C. W., Yang, C.-M., Lane, T. J., Lee, H.-C., et al. (2018). The neurophysiological basis of the discrepancy between objective and subjective sleep during the sleep onset period: an EEG-fMRI study. Sleep 41, 231–210. doi: 10.1093/sleep/zsy056
Hu, L., Li, G., Shu, Y., Hou, X., Yang, L., and Jin, Y. (2022). Circadian dysregulation induces alterations of visceral sensitivity and the gut microbiota in light/dark phase shift mice. Front. Microbiol. 13:935919. doi: 10.3389/fmicb.2022.935919
Huang, F.-Y., Hsu, A.-L., Chao, Y.-P., Shang, C. M.-H., Tsai, J.-S., and Wu, C. W. (2021). Mindfulness-based cognitive therapy on bereavement grief: alterations of resting-state network connectivity associate with changes of anxiety and mindfulness. Hum. Brain Mapp. 42, 510–520. doi: 10.1002/hbm.25240
Kalmbach, D. A., Arnedt, J. T., Song, P. X., Guille, C., and Sen, S. (2017). Sleep disturbance and short sleep as risk factors for depression and perceived medical errors in first-year residents. Sleep 40:zsw073. doi: 10.1093/sleep/zsw073
Kang, J., Noh, W., and Lee, Y. (2020). Sleep quality among shift-work nurses: a systematic review and meta-analysis. Appl. Nurs. Res. 52:151227. doi: 10.1016/j.apnr.2019.151227
Kecklund, G., and Axelsson, J. (2016). Health consequences of shift work and insufficient sleep. BMJ 355:i5210. doi: 10.1136/bmj.i5210
Komanduri, M., Savage, K., Lea, A., McPhee, G., Nolidin, K., Deleuil, S., et al. (2021). The relationship between gut microbiome and cognition in older Australians. Nutrients 14:64. doi: 10.3390/nu14010064
Kong, D., Liu, R., Song, L., Zheng, J., Zhang, J., and Chen, W. (2018). Altered Long- and short-range functional connectivity density in healthy subjects after sleep deprivations. Front. Neurol. 9:546. doi: 10.3389/fneur.2018.00546
Liu, Z., Wei, Z.-Y., Chen, J., Chen, K., Mao, X., Liu, Q., et al. (2020). Acute sleep-wake cycle shift results in community alteration of human gut microbiome. mSphere 5, e00914–e00919. doi: 10.1128/msphere.00914-19
Machi, M. S., Staum, M., Callaway, C. W., Moore, C., Jeong, K., Suyama, J., et al. (2012). The relationship between shift work, sleep, and cognition in career emergency physicians. Acad. Emerg. Med. 19, 85–91. doi: 10.1111/j.1553-2712.2011.01254.x
Marquié, J.-C., Tucker, P., Folkard, S., Gentil, C., and Ansiau, D. (2015). Chronic effects of shift work on cognition: findings from the VISAT longitudinal study. Occup. Environ. Med. 72, 258–264. doi: 10.1136/oemed-2013-101993
Mashaqi, S., and Gozal, D. (2020). “Circadian misalignment and the gut microbiome. A bidirectional relationship triggering inflammation and metabolic disorders”- a literature review. Sleep Med. 72, 93–108. doi: 10.1016/j.sleep.2020.03.020
McMurdie, P. J., and Holmes, S. (2013). Phyloseq: an R package for reproducible interactive analysis and graphics of microbiome census data. PLoS One 8:e61217. doi: 10.1371/journal.pone.0061217
Mélan, C., and Cascino, N. (2022). Effects of a modified shift work organization and traffic load on air traffic controllers’ sleep and alertness during work and non-work activities. Appl. Ergon. 98:103596. doi: 10.1016/j.apergo.2021.103596
Nechifor, R. E., Ciobanu, D., Vonica, C. L., Popita, C., Roman, G., Bala, C., et al. (2020). Social jetlag and sleep deprivation are associated with altered activity in the reward-related brain areas: an exploratory resting-state fMRI study. Sleep Med. 72, 12–19. doi: 10.1016/j.sleep.2020.03.018
Park, Y. K., Kim, J.-H., Choi, S. J., Kim, S. T., and Joo, E. Y. (2019). Altered regional cerebral blood flow associated with mood and sleep in shift workers: cerebral perfusion magnetic resonance imaging study. J. Clin. Neurol. 15, 438–447. doi: 10.3988/jcn.2019.15.4.438
Qiu, D., Yu, Y., Li, R.-Q., Li, Y.-L., and Xiao, S.-Y. (2020). Prevalence of sleep disturbances in Chinese healthcare professionals: a systematic review and meta-analysis. Sleep Med. 67, 258–266. doi: 10.1016/j.sleep.2019.01.047
Quast, C., Pruesse, E., Yilmaz, P., Gerken, J., Schweer, T., Yarza, P., et al. (2013). The SILVA ribosomal RNA gene database project: improved data processing and web-based tools. Nucleic Acids Res. 41, D590–D596. doi: 10.1093/nar/gks1219
Rajaratnam, S. M. W., Howard, M. E., and Grunstein, R. R. (2013). Sleep loss and circadian disruption in shift work: health burden and management. Méd. J. Aust. 199, S11–S15. doi: 10.5694/mja13.10561
Rogers, A. E., Hu, Y. J., Yue, Y., Wissel, E. F. III, and Jarrett, S. (2021). Shiftwork, functional bowel symptoms, and the microbiome. PeerJ 9:e11406. doi: 10.7717/peerj.11406
Ross, F. C., Patangia, D., Grimaud, G., Lavelle, A., Dempsey, E. M., Ross, R. P., et al. (2024). The interplay between diet and the gut microbiome: implications for health and disease. Nat. Rev. Microbiol. 22, 671–686. doi: 10.1038/s41579-024-01068-4
Schliep, K. P. (2010). Phangorn: phylogenetic analysis in R. Bioinformatics 27, 592–593. doi: 10.1093/bioinformatics/btq706
Sun, J., Stewart, P., Chiew, A., Becker, T., Siu, W., Varndell, W., et al. (2021). Association between shift work and cognitive performance on the trail making test in emergency department health officers. Emerg. Med. Australas. 33, 711–717. doi: 10.1111/1742-6723.13753
Teichman, E. M., O’Riordan, K. J., Gahan, C. G. M., Dinan, T. G., and Cryan, J. F. (2020). When rhythms meet the blues: circadian interactions with the microbiota-gut-brain Axis. Cell Metab. 31, 448–471. doi: 10.1016/j.cmet.2020.02.008
Tian, F., Li, H., Tian, S., Shao, J., and Tian, C. (2022). Effect of shift work on cognitive function in Chinese coal mine workers: a resting-state fNIRS study. Int. J. Environ. Res. Public Heal. 19:4217. doi: 10.3390/ijerph19074217
Tsai, P. J., Chen, S. C. J., Hsu, C. Y., Wu, C. W., Wu, Y. C., Hung, C. S., et al. (2014). Local awakening: regional reorganizations of brain oscillations after sleep. NeuroImage 102, 894–903. doi: 10.1016/j.neuroimage.2014.07.032
TSSM Investigation of Taiwan Society of Sleep Medicine (TSSM): Prevalence of chronic insomnia and sleep problems among Taiwan shift workers (2019). Available at: https://tssm.org.tw/file/1563864454.pdf (Accessed Aprtl 15, 2024).
Tung, C.-L., Wu, J.-H., Chang, H.-C., Xu, J.-W., Yang, Y.-C. S. H., Wu, C. W., et al. (2024). Effects of black soybean seed coat (BSSC) crude extract on the immune regulation, gut microbiota, and brain function of mice with sleep deprivation. J. Funct. Foods 119:106335. doi: 10.1016/j.jff.2024.106335
Wang, L., Chen, Y., Yao, Y., Pan, Y., and Sun, Y. (2016). Sleep deprivation disturbed regional brain activity in healthy subjects: evidence from a functional magnetic resonance-imaging study. Neuropsychiatr. Dis. Treat. 12, 801–807. doi: 10.2147/ndt.s99644
Whitfield-Gabrieli, S., and Nieto-Castanon, A. (2012). Conn: a functional connectivity toolbox for correlated and Anticorrelated brain networks. Brain Connect. 2, 125–141. doi: 10.1089/brain.2012.0073
Wu, X., Bai, F., Wang, Y., Zhang, L., Liu, L., Chen, Y., et al. (2021). Circadian rhythm disorders and corresponding functional brain abnormalities in young female nurses: a preliminary study. Front. Neurol. 12:664610. doi: 10.3389/fneur.2021.664610
Xia, L., Chen, C., Liu, Z., Luo, X., Guo, C., Liu, Z., et al. (2021). Prevalence of sleep disturbances and sleep quality in Chinese healthcare workers during the COVID-19 pandemic: a systematic review and Meta-analysis. Front. Psych. 12:646342. doi: 10.3389/fpsyt.2021.646342
Yang, D. F., Huang, W. C., Wu, C. W., Huang, C. Y., Yang, Y.-C. S. H., and Tung, Y.-T. (2023). Acute sleep deprivation exacerbates systemic inflammation and psychiatry disorders through gut microbiota dysbiosis and disruption of circadian rhythms. Microbiol. Res. 268:127292. doi: 10.1016/j.micres.2022.127292
Yang, H., Long, X.-Y., Yang, Y., Yan, H., Zhu, C.-Z., Zhou, X.-P., et al. (2007). Amplitude of low frequency fluctuation within visual areas revealed by resting-state functional MRI. NeuroImage 36, 144–152. doi: 10.1016/j.neuroimage.2007.01.054
Ye, H., Ji, M., Wang, C., Wang, C., Li, Y., Chen, Y., et al. (2022). Integrated functional neuroimaging, monoamine neurotransmitters, and behavioral score on depressive tendency in intensive care unit medical staffs induced by sleep deprivation after night shift work. Front. Psych. 13:848709. doi: 10.3389/fpsyt.2022.848709
Ye, H., Lu, C., Wang, C., Zhang, D., Li, Y., Feng, X., et al. (2023). Plasma Aβ level alterations after sleep deprivation correspond to brain structural remodeling in medical night shift workers. Brain Res. Bull. 203:110776. doi: 10.1016/j.brainresbull.2023.110776
Zhang, S. L., Bai, L., Goel, N., Bailey, A., Jang, C. J., Bushman, F. D., et al. (2017). Human and rat gut microbiome composition is maintained following sleep restriction. Proc. Natl. Acad. Sci. USA 114, E1564–E1571. doi: 10.1073/pnas.1620673114
Keywords: shift work, attention, circadian rhythm, functional MRI, brain connectivity, gut microbiota, gut-brain axis
Citation: Yao T, Chao Y-P, Huang C-M, Lee H-C, Liu C-Y, Li K-W, Hsu A-L, Tung Y-T and Wu CW (2025) Impacts of night shift on medical professionals: a pilot study of brain connectivity and gut microbiota. Front. Neurosci. 19:1503176. doi: 10.3389/fnins.2025.1503176
Received: 28 September 2024; Accepted: 27 January 2025;
Published: 17 February 2025.
Edited by:
Amy Mackos, The Ohio State University, United StatesReviewed by:
Valentina Caputi, University College Cork, IrelandCopyright © 2025 Yao, Chao, Huang, Lee, Liu, Li, Hsu, Tung and Wu. This is an open-access article distributed under the terms of the Creative Commons Attribution License (CC BY). The use, distribution or reproduction in other forums is permitted, provided the original author(s) and the copyright owner(s) are credited and that the original publication in this journal is cited, in accordance with accepted academic practice. No use, distribution or reproduction is permitted which does not comply with these terms.
*Correspondence: Changwei W. Wu, sleepbrain@tmu.edu.tw; Yu-Tang Tung, peggytung@nchu.edu.tw
Disclaimer: All claims expressed in this article are solely those of the authors and do not necessarily represent those of their affiliated organizations, or those of the publisher, the editors and the reviewers. Any product that may be evaluated in this article or claim that may be made by its manufacturer is not guaranteed or endorsed by the publisher.
Research integrity at Frontiers
Learn more about the work of our research integrity team to safeguard the quality of each article we publish.