- Faculty of Basic Sciences, Department of Biology, Lorestan University, Khorramabad, Iran
Central nervous system disorders impact over 1.5 billion individuals globally, with neurodegenerative diseases such as Alzheimer’s, Parkinson’s, and Huntington’s diseases being particularly prominent. These conditions, often associated with aging, present debilitating symptoms including memory loss and movement difficulties. The growing incidence of neurological disorders, alongside a scarcity of effective anti-amyloidogenic therapies, highlights an urgent need for innovative treatment methodologies. Nanoparticles (NPs), derived from medicinal plants and characterized by their favorable pharmacological properties and minimal side effects, offer a promising solution. Their inherent attributes allow for successful traversal of the blood–brain barrier (BBB), enabling targeted delivery to the brain and the modulation of specific molecular pathways involved in neurodegeneration. NPs are crucial in managing oxidative stress, apoptosis, and neuroinflammation in ND. This study reviews the efficacy of green-synthesized nanoparticles in conjunction with various medicinal plants for treating neurodegenerative diseases, advocating for further research to refine these formulations for enhanced clinical applicability and improved patient outcomes.
1 Introduction
Neurodegenerative diseases (ND) is characterized by the gradual loss of neurons, structural and functional impairments in the brain and spinal cord, as well as cognitive and physical decline, ultimately leading to the direct and indirect demise of patients. The World Health Organization estimates that 50 million people worldwide are affected by neurodegenerative disorders – primarily characterized by motor neuron dysfunction and loss – and that number is expected to rise as our population ages (Scatena et al., 2007; Yavarpour-Bali et al., 2019; Bhattacharya et al., 2022). Numerous factors contribute to the development of ND, including the accumulation of amyloid proteins, intracellular or extracellular protein misfolding within the CNS, neuroinflammation, oxidative stress, neurotransmitter depletion such as butyrylcholine (BCh) and acetylcholine (ACh), and disruption of the blood–brain barrier (BBB) (Rasool et al., 2014; Maiti and Dunbar, 2018). Genetic predisposition plays a significant role in ND, alongside factors like excessive brain accumulation of metals such as copper (Cu), zinc (Zn), lead (Pb), and iron (Fe), mitochondrial dysfunction, and impaired redox reactions (Masoudi Asil et al., 2020; Sriramcharan et al., 2022). Additionally, certain chemicals like monoamine oxidase and cholinesterase contribute to the breakdown of dopaminergic and cholinergic synapses (Teibo John et al., 2020).
Conditions like Alzheimer’s diseases (AD), Parkinson’s diseases (PD), and Huntington’s diseases (HD) are closely linked to the aging process, manifesting symptoms such as memory loss, movement impairments, and speech and breathing difficulties. (Gitler et al., 2017; Bhattacharya et al., 2022). AD represents the most prevalent form of dementia, with the number of AD patients reaching 50 million by 2017. The disease has multifaceted causes, with abnormal amyloid β (Aβ) accumulation being a key factor (Kalimuthu et al., 2020; Huang et al., 2021). AD prevalence rises notably after age 65, with a marked exponential increase with advancing age. AD risk factors encompass familial history, head injuries, genetic factors (apolipoprotein E), gender (female), vascular conditions, and environmental influences. In familial AD cases, mutations in presenilin 1 and 2 genes are observed, accounting for 2-3% of AD instances and affecting individuals under 65 (Singh et al., 2010; Castellani et al., 2010). Loss of synapses in AD is associated with the accumulation of low-solubility Aβ species. Amygdala regions in AD patients have many plaques and neurofibrillary. Also, two types of Aβ plaques are seen in the brain parenchyma along with tau inclusions. Most of these patients also have amyloid angiopathy (Castellani et al., 2010; Dugger and Dickson, 2017).
Oxidative damage triggered by mitochondrial dysfunction (induced by Aβ42) and glial activation leads to cytotoxicity and calcium overload (Emerit et al., 2004). The integrity of brain cell support and transport systems relies on the tau protein’s proper structure and function. In AD, the abnormal twisting of tau strands results in dysfunctional tangles within brain cells, disrupting the transport system and culminating in cell death (Agarwal et al., 2013). PD represents the most prevalent motor ND, affecting at least 1% of individuals over 70 years of age. Approximately 80% of PD patients develop dementia within two decades. Parkinson’s disease dementia (PDD) is characterized by deficits in short-term memory and decision-making functions, stemming from the degeneration of subcortical nuclei like the medial substantia nigra and the cholinergic nucleus basalis of Meynert. Dopamine deficiency in the striatum due to the loss of dopamine-producing cells is a hallmark of PD (Savitt et al., 2006; Edison et al., 2013; Irwin et al., 2013).
Oxidative stress and neuroinflammation are primary contributors to the death of dopaminergic neurons (Khazdair et al., 2020). The etiology of PD includes the accumulation of intracellular α-synuclein aggregates, reduced activity of mitochondrial complex 1, and telomere shortening (Hou et al., 2019). Additionally, PD is characterized by factors such as iron accumulation in the zona, elevated nitrogen levels in Lewy bodies, activation of the caspase cascade, and increased apoptosis (Emerit et al., 2004). Aβ is present in both PD and PDD, with significant Aβ levels observed in 40% of PDD cases (Edison et al., 2013).
HD is an autosomal dominant disorder affecting 4-10 individuals per 100,000 people. While typically manifesting between ages 30 and 50, HD can also onset as early as two or as late as over 80 in rare cases (Sandhir et al., 2014). HD leads to degeneration of the striatum, hypothalamus, and cerebral cortex, resulting in motor, cognitive, and behavioral impairments, weight loss, disruptions in circadian sleep rhythms, and autonomic nervous system dysfunction (Popovic and Brundin, 2006; Roos, 2010). HD is caused by misfolding of the huntingtin protein into its β form and post-translational modifications like phosphorylation. This abnormal protein disrupts cellular metabolism and mitochondrial function, generating atypical metabolites and markers of oxidative stress. Neuronal death in HD is associated with movement disorders, with disease progression influenced by environmental and genetic factors (Ross et al., 2014).
This study addresses the critical relevance of central nervous system disorders affecting over 1.5 billion individuals worldwide, particularly highlighting neurodegenerative diseases such as Alzheimer’s and Parkinson’s. While current literature acknowledges the urgent need for effective treatments, significant gaps remain in the application of nanoparticle (NP) technology derived from medicinal plants. Specifically, there is a lack of comprehensive research on the optimization of NP formulations for targeted delivery across the blood–brain barrier and their specific mechanisms in mitigating neurodegeneration. This study aims to address these gaps by systematically reviewing existing data and proposing refined NP strategies to enhance therapeutic efficacy. The main contributions of this research include advancing the understanding of green-synthesized nanoparticles in neuroprotection and offering a pathway for developing innovative treatment options with minimal side effects for affected patients.
2 Amyloids
Amyloids are fibrous, insoluble proteins resistant to protease degradation, forming aggregates in the cytoplasm of neurons, glia, parenchyma, and blood vessel walls as plaques or amyloid angiopathy. Amyloid plaques can manifest in various forms, including diffuse, dense-cored, classical, and cotton wool, which vary based on the type of amyloid, disease stage, and deposition site (Dugger and Dickson, 2017; Girigoswami et al., 2019). These amyloid deposits can lead to significant tissue damage and cell death (Ghosh et al., 2021). By binding to cell membranes, amyloid accumulations can disrupt membrane integrity, leading to increased cellular damage, oxidative stress, cytoskeletal alterations, organ dysfunction, and apoptosis (Ghosh and De, 2020). This membrane disruption is a key driver of amyloid-induced cytotoxicity (Wang et al., 2017).
Human amyloidosis involves over 20 amyloidogenic peptides and pathogenic proteins like Aβ, α-synuclein, Tau, and serum amyloid protein. Amyloidosis is categorized into systemic and local forms, with systemic involvement across multiple organs and local involvement in specific tissues (Wang et al., 2017; Ghosh and De, 2020). Amyloid deposition is common in individuals with a genetic disorder associated with apolipoprotein E (Dugger and Dickson, 2017).
3 Oxidative stress
Oxidative stress inflicts damage on cell proteins and lipid membranes, primarily driven by free radicals such as reactive oxygen species (ROS) and reactive nitrogen species (RNS) (Ríos et al., 2016; Chandran and Abrahamse, 2020). The nervous system is particularly susceptible to ROS due to its high oxygen consumption, limited antioxidant capacity, abundance of steroid lipids, and metal catalyst content (Sharifi-Rad et al., 2022). ROS plays a pivotal role in neurodegeneration in AD, PD, and HD, leading to mitochondrial dysfunction, neuroinflammation, and elevated levels of nuclear factor κB (NFκB) and insulin-like growth factor (IGF) (Ríos et al., 2016; Chandran and Abrahamse, 2020; Sharifi-Rad et al., 2022).
Edible oyster mushrooms are notable for their rich composition of bioactive compounds, such as phenolics, flavonoids, ascorbic acid, glycosides, tocopherols, polysaccharides, ergothioneine, and carotenoids. These compounds possess robust antioxidant properties that effectively combat free radicals, thereby playing a significant role in mitigating oxidative stress (Gupta et al., 2017). The administration of medicinal mushroom extracts has demonstrated potential in treating patients and offering protection against a variety of diseases, including neurodegenerative disorders (Chaturvedi et al., 2020).
Mitochondrial superoxide radicals impair movement within the brain, resulting in DNA damage and the onset of neurodegenerative diseases. Hydrogen peroxide (H2O2) is linked to excessive oxidation in nerve cells, with peroxisomes typically responsible for controlling superoxide radicals and H2O2 enzymatically. In AD, hyperphosphorylated tau proteins lead to catalase (CAT) and peroxidase depletion from peroxisomes, exacerbating oxidative stress (Chandran and Abrahamse, 2020; Sharifi-Rad et al., 2022).
4 Neuroinflammation
Neuroinflammation is a common feature of ND, characterized by elevated levels of cytokines and inflammatory markers in AD, PD, and HD. In neuroinflammation, the brain’s innate immune response triggers an increase in chemokine concentrations like interleukin-6 (IL-6), IL-1β, CC-motif ligand-2 and 5 (CCL-2 and 5), and CXC-motif ligand-1 (CXCL-1), promoting ROS and RNS production and enhancing BBB permeability (Fakhri et al., 2022).
Microglial cells, part of the brain’s mononuclear phagocyte system, play a crucial role in neuroinflammation. Inflammatory responses by microglia contribute to the demise of dopamine-producing cells. Microglia induce cell damage by releasing IL-1, 6, and 12, tumor necrosis factor (TNF-α), and nitric oxide (NO), stimulating amyloid precursor protein production, and elevating Aβ levels. Conversely, Aβ enhances microglial activation (Klegeris and McGeer, 2005; Edison et al., 2013). Cytokines released by microglia bind to neuronal receptors, activating apoptotic pathways (Sharifi-Rad et al., 2022).
5 Metal accumulation in the brain
Excessive accumulation of metals in the brain leads to oxidative damage, mitochondrial dysfunction, protein misfolding, impaired autophagy, neuroinflammation, and neuronal death (Yan et al., 2022).
In AD, calcium release from the endoplasmic reticulum disrupts memory, while the Cu, Zn, and Fe buildup enhances Aβ accumulation and triggers oxidative stress. Manganese accumulation inhibits glycolysis, causing toxicity and cytoskeletal disruption in HD, while abnormal copper-protein interactions contribute to HD development by affecting the huntingtin structure. Elevated Fe levels in PD lead to ferroptosis and the loss of dopaminergic neurons (Yan et al., 2022).
6 Acetylcholinesterase (AChE) and butyrylcholinesterase (BChE) enzymes
ACh is the essential natural brain substance that affects memory, speech, concentration, and logical reasoning. BCh is also effective on memory. BChE and AChE are in the group of serine hydrolases. BChE is mainly found in the white matter and areas effective in cognition and behavior. The main action of BChE and AChE is the simultaneous regulation of ACh. An increase in the level of AChE and BChE leads to the breakdown of ACh and BCH and reduces their stimulatory effects. In AD, the number of neurons expressing BChE is increased and is associated with the formation of amyloid plaques. Inhibition of AChE and BChE can reduce the accumulation of Aβ and the formation of nerve fibrils and increase the level of ACh (Darvesh, 2016; Gul et al., 2021).
7 Available treatments for AD, PD, and HD
Memantine enhances cholinergic signaling and inhibits glutamate overactivation through N-methyl-D-aspartate receptor inhibition in AD. Cholinesterase inhibitors like tacrine, donepezil, rivastigmine, and galantamine manage AD symptoms (Casey et al., 2010; Tang et al., 2023). Levodopa is a common PD treatment that stimulates dopamine receptors (Schapira, 2005).
The drugs olanzapine and haloperidol help to manage Huntington’s disease by decreasing chorea symptoms, while tetrabenazine works by reducing dopamine levels in the brain. These medications are crucial in alleviating motor symptoms and improving the quality of life for individuals with HD (Bonelli et al., 2004). The rising prevalence of neurological disorders, coupled with the limited availability of anti-amyloidogenic drugs, underscores the urgent need for innovative treatment approaches (Ghosh and De, 2020). In addition, given the side effects associated with existing treatments (Figure 1), the utilization of medicinal plants for treating AD and other diseases has been under consideration (Ovais et al., 2018).
8 Medicinal plants
Medicinal plants harbor bioactive compounds with potent pharmacological properties and minimal side effects (Table 1) (Hassan et al., 2022; Mishrikoti et al., 2022). Different plant parts, such as flowers, seeds, fruits, roots, leaves, and bark, are used in disease treatment, with extraction methods varying based on compound characteristics. For this purpose, polar and nonpolar solvents and methods such as sonication, Soxhlet extraction, and heating under reflux are utilized (Chandran and Abrahamse, 2020; Mishrikoti et al., 2022). Secondary metabolites like sterols, polyphenols, lignans, flavonoids, alkaloids, triterpenes, and tannins from medicinal plants show efficacy in combating CNS-related diseases by targeting AChE, BChE, oxidative stress, and neuroinflammation (Gul et al., 2021; Bakrim et al., 2022).
8.1 Flavonoids
Flavonoids are low molecular weight compounds obtained from various parts of plants such as roots, stems, and flowers, with over 6,000 types identified. They exhibit therapeutic properties for ND by reducing cell death caused by inflammation through the modulation of MAPK pathways, Akt, and NF-κB. Flavonoids inhibit the production of inflammatory cytokines, chemokines (Solanki et al., 2016; Martano et al., 2022), ROS and RNS, and inhibition of lipid peroxidation. These compounds have been shown to improve memory, increase neurogenesis, and suppress cytochrome c oxidase activity (Solanki et al., 2016; Prasanna and Upadhyay, 2021; Martano et al., 2022). Additionally, flavonoids have protective effects on PD and HD, preventing the spread of Aβ peptides and neurotoxic aggregations (Ramirez-Nuñez et al., 2018).
Specific flavonoids like baicalein, catechin, epigallocatechin-3-gallate (EGCG), fisetin, genistein, quercetin, and wogonin modulate neuroinflammation and reduce prostanoids levels. Genistein and silibinin inhibit AChE and BChE (Prasanna and Upadhyay, 2021).
Quercetin, in particular, has therapeutic properties for AD by removing free radicals and reducing inflammation. Quercetin is also a competitive inhibitor of AChE and BChE and inhibits them in a dose-dependent manner. Quercetin reduces the level of AChE in the hippocampus and increases it in the synaptic space by preventing the degradation of ACh (Testa et al., 2014; Khan et al., 2019; Liao et al., 2022).
Naringin is a member of the flavonoid group that is derived from various citrus fruits and Artemisia selengensis and it decreases the levels of IL-1β, TNF-α, malondialdehyde (MDA) and AChE and increases the levels of CAT, superoxide dismutase (SOD) and glutathione (GSH). This flavonoid improves mitochondrial and redox activity in the cerebral cortex and hippocampus (Sachdeva et al., 2014).
8.2 Phenols
Phenols are characterized by one or more aromatic rings with hydroxyl groups (Chandran and Abrahamse, 2020) and are part of a group of natural compounds known as polyphenols. These compounds possess anti-inflammatory, antioxidant, and anti-amyloid properties superior to synthetic compounds as part of a healthy diet (Velander et al., 2017). Phenolic compounds inhibit the secretion of IL-1β and TNF-α, induction of iNOS, production of NO, NADPH oxidase, and ROS while regulating the inhibition of pro-inflammatory transcription factors like NF-κB (Sharifi-Rad et al., 2022). Ellagic acid, a polyphenol derived from various plants (such as Rosa rugosa, Rubus chamaemorus, Rubus ursinus × Rubus idaeus, Rubus allegheniensis, and Rubus fruticosus) increases SOD levels, improves memory, inhibits tau hyperphosphorylation, and prevents Aβ toxicity (Ahmadi and Javid, 2023). It also reduces Aβ plaques in the cingulate cortex, hippocampus, and entorhinal cortex (Rezai-Zadeh et al., 2008). Curcumin, another polyphenol, improves memory, prevents the progression of AD, and exhibits anti-Aβ and anti-inflammatory properties, reducing the inflammatory response caused by Aβ in microglia. This polyphenol inhibits the oxidative stress caused by Aβ by increasing SOD and CAT, maintaining the level of GSH and reducing MDA. In addition, Curcumin inhibits AChE in the cortex and striatum with a mechanism similar to AD drugs (Hamaguchi et al., 2010; Tang and Taghibiglou, 2017; Chen et al., 2018).
8.3 Alkaloids
Alkaloids are compounds found in plants, mainly flowering plants, containing carbon, hydrogen, nitrogen, and, in most cases, oxygen. These compounds have anti-amyloid, anti-inflammatory, antioxidant, and neuroprotective properties, making them suitable and safe for treating neurodegenerative diseases (Hussain et al., 2018; Bakrim et al., 2022).
The alkaloid galantamine derived from Amaryllidaceae plants suppresses cytotoxicity and Aβ accumulation while stimulating ACh receptors. Juliflorine alkaloid derived from Prosopis juliflora leaves inhibits AChE and BChE (Hussain et al., 2018; Bakrim et al., 2022).
Berberine derived from the plants Argemone Mexicana, Berberis aquifolium, Berberis vulgaris, improves cognitive and motor skills and reduces levels of mutant protein huntingtin (Htt), NF-κB, α, IL 6, IL-8 and oxidative stress caused by ROS and RNS in HD patients (Singh et al., 2022).
Alkaloid huperzine-A decreases the levels of mutant protein Htt, ROS, MDA, TNF-α and AChE and increases SOD, CAT and Glutathione Peroxidase (Gpx) in HD patients (Subaraja et al., 2024).
Alkaloid derived from Piper longum, improves motor skills, increases dopaminergic neurons, SOD and GSH and decreases MDA level in patients with PD (Bi et al., 2015).
Alkaloids derived from the Crossyne flava, plant improve the morphology of neurons, inhibit ROS and apoptosis, and increase the level of adenosine triphosphate activity (ATP) in patients with PD (Omoruyi et al., 2021).
8.4 Terpenes
Terpenes represent the largest and most diverse group of secondary metabolites, consisting of simple hydrocarbons with multiple isoprene units. They exhibit various beneficial properties such as anti-cancer, anti-hyperglycemic, anti-inflammatory, antioxidant, immune-modulating, and anti-cholinesterase effects. Terpenes are known for their neuroprotective properties (Lai Shi Min et al., 2022; Bakrim et al., 2022). The terpene derived from Alpinia oxyphylla Miq has a neuroprotective effect and aids in synthesizing and releasing neurotransmitters from neurons. The terpene derived from G. repens has an inhibitory effect on AChE and BChE. Terpenes derived from Nepeta obtusicrena inhibit AChE and exhibit therapeutic properties for AD (Bakrim et al., 2022).
9 Problems of using medicinal plants
The poor uptake of certain herbal compounds has posed challenges in their treatment applications. For instance, the polyphenol curcumin exhibits limited absorption and bioavailability in AD treatment (Abhishek et al., 2023). The BBB is a semipermeable boundary consisting of endothelial cells, pericytes, astrocytes, and the basement membrane, serving as a protective interface between the CNS and peripheral circulation (Sahni et al., 2011; Nguyen et al., 2021; Bhattacharya et al., 2022). This barrier impedes the delivery of treatments to brain neurons (Shabbir et al., 2020).
Suitable approaches to enhance bioavailability and traverse the BBB should be explored. To this end, employing innovative techniques like combining plant compounds with nanoparticles proves viable (Abhishek et al., 2023).
10 Nanoparticles
Nanoparticles (NPs) are sizes ranging from 10 to 100 nm, categorized into ceramic, metal, semiconductor, carbon-based, lipid-based, and polymer groups based on their properties and structures. NPs possess small size, high reactivity, and a substantial surface-to-volume ratio (Saratale et al., 2018; Fakhri et al., 2022). They exhibit stability in the body, efficient cellular uptake, and the ability to neutralize superoxide anion and H2O2, and are applications in wound dressing (Xing et al., 2014; Ko et al., 2022). Among the most utilized NPs in AD diagnosis and treatment are gadolinium NPs, Selenium NPs (SeNPs), AuNPs, polymeric NPs, and protein- and polysaccharide-based NPs (Gupta et al., 2019).
The attributes of NPs, such as extensive surface area, high cellular uptake capability, and prolonged circulation in the bloodstream, facilitate their passage through the BBB, enabling effective drug delivery to the brain. NPs target specific molecular mechanisms based on the type of disease, addressing cells or intracellular and extracellular molecules like Aβ plaques (Zhang et al., 2013; Shabbir et al., 2020; Martano et al., 2022). NPs are crucial in managing oxidative stress, apoptosis, and neuroinflammation in ND (Fakhri et al., 2022; Woon et al., 2022) (Figure 2).
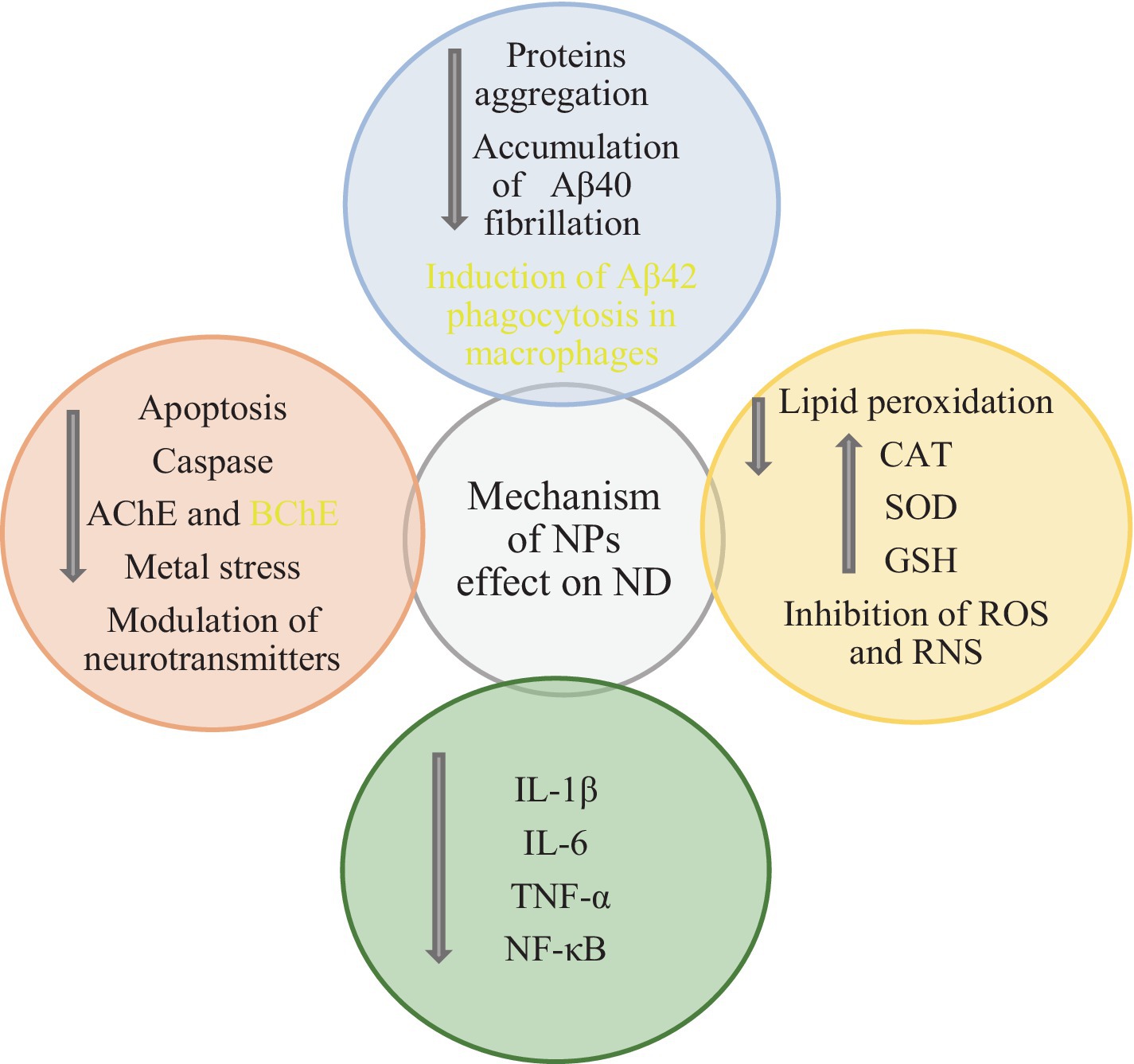
Figure 2. Anti-apoptotic, antioxidant, anti-inflammatory, anti-cholinesterase effects and other mechanisms of NPs in ND (Ovais et al., 2018; Fakhri et al., 2022; Woon et al., 2022; Behera et al., 2023).
NPs impact amyloid fibrillation and the degradation of mature protein fibrils through six mechanisms: 1. Enhancing bioavailability, leading to the dispersion of insoluble molecules in water and enhancing the stability of unstable chemical molecules; 2. They are inhibiting protein aggregation during synthesis, 3. Facilitating high cellular uptake, 4. Leveraging the multivalence of NPs to enhance binding to protein aggregates, 5, mitigating toxicity induced by protein fibrils, 6 and ensuring precise targeting within the brain (Pradhan et al., 2018). Cationic NPs are internalized by cells through uptake-mediated endocytosis, highly hydrophilic NPs through receptor-mediated endocytosis, small hydrophilic NPs through the paracellular pathway, and small lipophilic NPs through passive diffusion via intercellular pathways (Shabbir et al., 2020). NPs can be absorbed through nerve terminals in the airway epithelium and transported to CNS axons. Additionally, they can reach the CNS via the olfactory bulb nerves (Sahni et al., 2011).
Various methods exist for synthesizing NPs. Physical methods encompass mechanical milling, laser ablation, sputtering, plasma arching, and chemical etching. In contrast, chemical methods include the sol–gel method, electrolytic deposition, chemical vapor deposition, microemulsion route pyrolysis, and green synthesis methods involving microorganisms, enzymes, and plant extracts (Garg et al., 2021).
11 Green synthesis
Synthesizing NPs via chemical methods can be toxic, while physical methods require high energy consumption. Both physical and chemical methods raise environmental concerns, making the green synthesis method appealing due to its safety, environmental friendliness, and low toxicity advantages (Rai et al., 2013; Saratale et al., 2018; Mikhailova, 2021). Biogenic nanoparticles can be readily functionalized with targeting ligands or therapeutic agents, facilitating precise targeting and delivery to cancer cells (Chaturvedi et al., 2023).
Key factors like temperature, time, reactant concentration, environmental conditions, pore size, and pH significantly influence the morphological properties of NPs. For instance, physical and chemical methods typically operate at high temperatures (> 350°C for physical methods and < 350°C for chemical methods), whereas the green synthesis method occurs at lower temperatures (≤ 100°C) (Bahramikia and Izadi, 2023). In green synthesis, photoautotrophic eukaryotes like microbes, algae, and plants can be utilized. Employing microorganisms for green synthesis is viable due to its safety and cost-effectiveness. Microorganisms exhibit selective metal ion absorption and can function under various ionic, temperature, and pH conditions. Different microorganisms, including fungi, bacteria, and yeasts, can be us for green synthesis (Saratale et al., 2018).
Algae, serving as blue photoautotrophs, are primary producers in green synthesis environments. They have been instrumental in synthesizing AuNPs, AgNPs, CuNPs, ZnNPs, ZnONPs, and CuONPs. For instance, Sargassum wightii algae has been utilized for extracellular AuNP synthesis (Saratale et al., 2018).
Using plant extracts for green synthesis of metal and metal oxide NPs is one of the simplest methods for green synthesis. Plant extracts containing ketones, aldehydes, flavonoids, amides, terpenoids, phenols, carboxylic acids and ascorbic acids are used for green synthesis (Bhattacharya et al., 2022). NPs synthesized through green methods find applications in treating various diseases like cancer, diabetes, and bacterial infections, showcasing antioxidant and anti-inflammatory properties (Bahramikia and Izadi, 2023) Ag NPs and Au NPs showed promising results against a human colon cancer cell line. These NPs reduced the proliferation of a cancer cell line by generating a large amount of intracellular ROS (Chaturvedi et al., 2020). Oyster mushroom mediated bimetallic Au-Pt nanoparticles exhibited apoptotic activity on the human colon cancer cell line in a dose-dependent manner (Chaturvedi et al., 2021a). In addition, oyster mushroom mediated Au–Pt–Ag trimetallic nanoparticles successfully killed triple-negative breast cancer cells with superior IC50 values (Chaturvedi et al., 2020).
11.1 Metal NPs and metal oxide synthesized with medicinal plants and their compounds
Numerous metal NPs have been developed for drug delivery, with AuNPs and AgNPs being the most prevalent in biomedicine (Jabbar et al., 2018). Metal NPs and metal oxides exhibit anti-inflammatory properties (Xing et al., 2014; Ko et al., 2022). Notably, AuNPs, AgNPs, CuNPs, SeNPs, ZnONPs, magnesium oxide NPs (MgONPs), Cerium oxide NPs (CeONPs), and FeONPs possess anti-inflammatory attributes and demonstrate effectiveness in AD treatment (Fakhri et al., 2022). AuNPs inhibit tau hyperphosphorylation, alter the secondary structure of Aβ, enhance memory, reduce H2O2, and elevate CAT, SOD, and GSH levels (Lee et al., 2014; dos Santos Tramontin et al., 2020). Silver, gold, and many other nanoparticles effectively prevent progressive neurodegeneration in PD (Chaturvedi et al., 2021a). Various studies have demonstrated the potential benefits of NPs synthesized with natural extracts in treating neurodegenerative diseases (Table 2).
11.2 Polymeric NPs synthesized with medicinal plants and their compounds
Polymeric drug carriers with nanometer sizes are being explored for their advantageous properties, including high drug-carrying capacity, stability, solubility, targeted tissue absorption, controlled drug release, and suitability for hydrophilic and hydrophobic compounds (Naseri et al., 2022). These polymeric NPs can traverse the BBB and hold promise for treating neurodegenerative diseases such as AD (Gupta et al., 2019). Various studies have investigated the application of polymeric NPs for treating neurological diseases, including AD (Table 3).
11.3 Other NPs synthesized with medicinal plants and their compounds
Other NPs synthesized using medicinal plants and their compounds have shown promising effects in various studies (Table 4).
12 Conclusion
Bioactive molecules such as sterols, polyphenols, lignans, flavonoids, alkaloids, triterpenes, and tannins, which are abundant secondary metabolites in the diet, have been utilized to treat CNS-related diseases. These secondary metabolites have demonstrated inhibitory effects on Aβ, toxicity induced by AChE, BChE, oxidative stress, and neuroinflammation (Gul et al., 2021; Bakrim et al., 2022). The unique characteristics of NPs, including their large surface area, high cellular uptake capacity, and prolonged presence in the bloodstream, enable them to traverse the BBB and efficiently deliver drugs to the brain. NPs can target specific molecular mechanisms based on the type of disease, addressing cellular, intracellular, or extracellular targets such as Aβ plaques (Zhang et al., 2013; Shabbir et al., 2020; Martano et al., 2022). Using plant extracts for green synthesis of metal and metal oxide NPs is one of the simplest methods for green synthesis. Plant extracts containing ketones, aldehydes, flavonoids, amides, terpenoids, phenols, carboxylic acids and ascorbic acids are used for green synthesis (Bhattacharya et al., 2022). These green-synthesized NPs have been employed in treating various diseases, including cancer, diabetes, and bacterial infections, and have demonstrated antioxidant and anti-inflammatory properties (Bahramikia and Izadi, 2023). Additionally, metal NPs and metal oxides exhibit anti-inflammatory properties (Xing et al., 2014; Ko et al., 2022). Polymeric NPs, known for their ability to cross the BBB, hold the potential for treating neurodegenerative diseases such as AD (Gupta et al., 2019). Furthermore, NPs synthesized via the green synthesis method offer diverse mechanisms for treating neurodegenerative diseases (Figure 3).
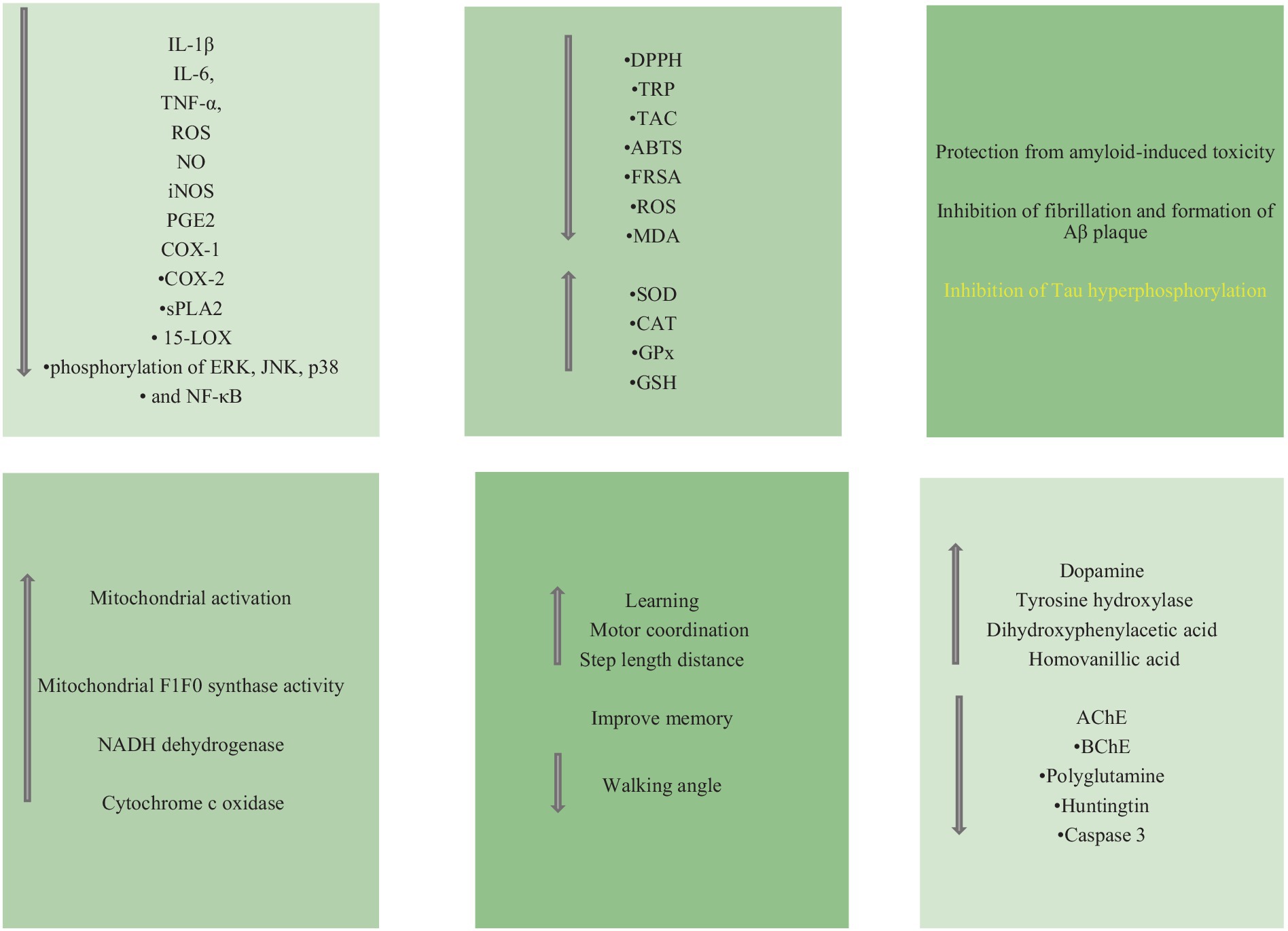
Figure 3. The most important events occurring in ND after treatment with NPs synthesized by green method.
Author contributions
RI: Investigation, Writing – original draft. SB: Writing – review & editing, Project administration, Supervision. VA: Visualization, Writing – review & editing.
Funding
The author(s) declare that no financial support was received for the research, authorship, and/or publication of this article.
Conflict of interest
The authors declare that the research was conducted in the absence of any commercial or financial relationships that could be construed as a potential conflict of interest.
Publisher’s note
All claims expressed in this article are solely those of the authors and do not necessarily represent those of their affiliated organizations, or those of the publisher, the editors and the reviewers. Any product that may be evaluated in this article, or claim that may be made by its manufacturer, is not guaranteed or endorsed by the publisher.
References
Abhishek, K., Saksham, R., Deepti, K., Rao NGR, R., Surya, P., Vinay, K., et al. (2023). Medicinal plants and herbal formulations ameliorating neurodegeneration: Remedies combating Parkinson’s and Alzheimer’s disease. J. Young Pharm. 15, 194–200. doi: 10.5530/jyp.2023.15.28
Agarwal, P., Alok, S., Fatima, A., and Singh, P. P. (2013). Herbal remedies for neurodegenerative disorder (alzheimer’s disease): a review. Int. J. Pharm. Sci. Res. 4, 3328–3340. doi: 10.13040/IJPSR.0975-8232.4(9).3328-3340
Ahmadi, S., and Javid, H. (2023). Nano Micro Biosystems novel formulations of ellagic acid for the improvement of antimicrobial, antioxidant, anticancer, antidiabetic, and neuroprotective applications. Nano Micro Biosystems 2023, 31–35. doi: 10.22034/nmbj.2023.388479.1015
Akram, M., and Nawaz, A. (2017). Effects of medicinal plants on Alzheimer’s disease and memory deficits. Neural Regen. Res. 12:660. doi: 10.4103/1673-5374.205108
Ayeni, E. A., Gong, Y., Yuan, H., Hu, Y., Bai, X., and Liao, X. (2022). Medicinal plants for anti-neurodegenerative diseases in West Africa. J. Ethnopharmacol. 285:114468. doi: 10.1016/j.jep.2021.114468
Bahramikia, S., and Izadi, R. (2023). Plant-based green synthesis of nanoparticles as an effective and safe treatment for gastric ulcer. Inflammopharmacology 31, 2843–2855. doi: 10.1007/s10787-023-01367-x
Bakrim, S., Aboulaghras, S., El Menyiy, N., El Omari, N., Assaggaf, H., Lee, L.-H., et al. (2022). Phytochemical compounds and nanoparticles as phytochemical delivery Systems for Alzheimer’s disease management. Molecules 27:9043. doi: 10.3390/molecules27249043
Barbara, R., Belletti, D., Pederzoli, F., Masoni, M., Keller, J., Ballestrazzi, A., et al. (2017). Novel curcumin loaded nanoparticles engineered for blood-brain barrier crossing and able to disrupt Abeta aggregates. Int. J. Pharm. 526, 413–424. doi: 10.1016/j.ijpharm.2017.05.015
Behera, A., Sa, N., Pradhan, S. P., Swain, S., and Sahu, P. K. (2023). Metal nanoparticles in Alzheimer’s disease. J. Alzheimers Dis. Rep. 7, 791–810. doi: 10.3233/ADR-220112
Bhattacharya, T., Soares, G. A. B., Chopra, H., Rahman Md, M., Hasan, Z., Swain, S. S., et al. (2022). Applications of Phyto-nanotechnology for the treatment of neurodegenerative disorders. Materials 15:804. doi: 10.3390/ma15030804
Bi, Y., Qu, P.-C., Wang, Q.-S., Zheng, L., Liu, H.-L., Luo, R., et al. (2015). Neuroprotective effects of alkaloids from Piper longum in a MPTP-induced mouse model of Parkinson’s disease. Pharm. Biol. 53, 1516–1524. doi: 10.3109/13880209.2014.991835
Bonelli, R. M., Wenning, G. K., and Kapfhammer, H. P. (2004). Huntington’s disease: present treatments and future therapeutic modalities. Int. Clin. Psychopharmacol. 19, 51–62. doi: 10.1097/01.yic.0000115715.96925.3d
Casey, D. A., Antimisiaris, D., and O’Brien, J. (2010). Drugs for Alzheimer’s disease: are they effective? Pharm. Therap. 35, 208–211.
Castellani, R. J., Rolston, R. K., and Smith, M. A. (2010). Alzheimer Disease. Dis. Mon. 56, 484–546. doi: 10.1016/j.disamonth.2010.06.001
Chandran, R., and Abrahamse, H. (2020). Identifying plant-based natural medicine against oxidative stress and neurodegenerative disorders. Oxidative Med. Cell. Longev. 2020, 1–9. doi: 10.1155/2020/8648742/
Chaturvedi, V. K., Sharma, B., Tripathi, A. D., Yadav, D. P., Singh, K. R., Singh, J., et al. (2023). Biosynthesized nanoparticles: a novel approach for cancer therapeutics. Frontiers in Medical Technology 5:1236107. doi: 10.3389/fmedt.2023.1236107
Chaturvedi, V. K., Singh, P., and Singh, M. (2021a). Recent advancement in the nanoparticles mediated therapeutics of Parkinson’s disease. Recent Adv. Treat Neurodegener Disord 26:208. doi: 10.2174/9781681087726121010014
Chaturvedi, V. K., Yadav, N., Rai, N. K., Bohara, R. A., Rai, S. N., Aleya, L., et al. (2021b). Two birds with one stone: oyster mushroom mediated bimetallic Au-Pt nanoparticles for agro-waste management and anticancer activity. Environmental Science and Pollution Research 28, 13761–13775. doi: 10.1007/s11356-020-11435-2
Chaturvedi, V. K., Yadav, N., Rai, N. K., Ellah, N. H. A., Bohara, R. A., Rehan, I. F., et al. (2020). Pleurotus sajor-caju-mediated synthesis of silver and gold nanoparticles active against colon cancer cell lines: a new era of herbonanoceutics. Molecules 25:3091. doi: 10.3390/molecules25133091
Chen, M., Du, Z.-Y., Zheng, X., et al. (2018). Use of curcumin in diagnosis, prevention, and treatment of Alzheimer’s disease. Neural Regen Res 13:742. doi: 10.4103/1673-5374.230303
Darvesh, S. (2016). Butyrylcholinesterase as a diagnostic and therapeutic target for Alzheimer’s disease. Curr. Alzheimer Res. 13, 1173–1177. doi: 10.2174/1567205013666160404120542
de Rus Jacquet, A., Timmers, M., Ma, S. Y., Thieme, A., McCabe, G. P., Vest, J. H. C., et al. (2017). Lumbee traditional medicine: neuroprotective activities of medicinal plants used to treat Parkinson’s disease-related symptoms. J. Ethnopharmacol. 206, 408–425. doi: 10.1016/j.jep.2017.02.021
Debnath, K., Shekhar, S., Kumar, V., Jana, N. R., and Jana, N. R. (2016). Efficient inhibition of protein aggregation, disintegration of aggregates, and lowering of cytotoxicity by green tea polyphenol-based self-assembled polymer nanoparticles. ACS Appl. Mater. Interfaces 8, 20309–20318. doi: 10.1021/acsami.6b06853
dos Santos Tramontin, N., da Silva, S., Arruda, R., Ugioni, K. S., Canteiro, P. B., de Bem Silveira, G., et al. (2020). Gold nanoparticles treatment reverses brain damage in Alzheimer’s disease model. Mol. Neurobiol. 57, 926–936. doi: 10.1007/s12035-019-01780-w
Dugger, B. N., and Dickson, D. W. (2017). Pathology of neurodegenerative diseases. Cold Spring Harb. Perspect. Biol. 9:a028035. doi: 10.1101/cshperspect.a028035
Edison, P., Ahmed, I., Fan, Z., Hinz, R., Gelosa, G., Ray Chaudhuri, K., et al. (2013). Microglia, amyloid, and glucose metabolism in Parkinson’s disease with and without dementia. Neuropsychopharmacology 38, 938–949. doi: 10.1038/npp.2012.255
El-Hawwary, S. S., Abd Almaksoud, H. M., Saber, F. R., Elimam, H., Sayed, A. M., El Raey, M. A., et al. (2021). Green-synthesized zinc oxide nanoparticles, anti-Alzheimer potential and the metabolic profiling of Sabal blackburniana grown in Egypt supported by molecular modelling. RSC Adv. 11, 18009–18025. doi: 10.1039/D1RA01725J
Emerit, J., Edeas, M., and Bricaire, F. (2004). Neurodegenerative diseases and oxidative stress. Biomed. Pharmacother. 58, 39–46. doi: 10.1016/j.biopha.2003.11.004
Fakhri, S., Abdian, S., Zarneshan, S. N., Moradi, S. Z., Farzaei, M. H., and Abdollahi, M. (2022). Nanoparticles in combating neuronal dysregulated signaling pathways: recent approaches to the Nanoformulations of phytochemicals and synthetic drugs against neurodegenerative diseases. Int. J. Nanomedicine 17, 299–331. doi: 10.2147/IJN.S347187
Garg, R., Rani, P., Garg, R., and Eddy, N. O. (2021). Study on potential applications and toxicity analysis of green synthesized nanoparticles. Turk. J. Chem. 45, 1690–1706. doi: 10.3906/kim-2106-59
Ghosh, P., Bera, A., Bhadury, P., and De, P. (2021). From small molecules to synthesized polymers: potential role in combating Amyloidogenic disorders. ACS Chem. Neurosci. 12, 1737–1748. doi: 10.1021/acschemneuro.1c00104
Ghosh, P., and De, P. (2020). Modulation of amyloid protein fibrillation by synthetic polymers: recent advances in the context of neurodegenerative diseases. ACS Appl. Bio. Mater. 3, 6598–6625. doi: 10.1021/acsabm.0c01021
Girigoswami, A., Ramalakshmi, M., Akhtar, N., Metkar, S. K., and Girigoswami, K. (2019). ZnO Nanoflower petals mediated amyloid degradation - an in vitro electrokinetic potential approach. Mater. Sci. Eng. C 101, 169–178. doi: 10.1016/j.msec.2019.03.086
Gitler, A. D., Dhillon, P., and Shorter, J. (2017). Neurodegenerative disease: models, mechanisms, and a new hope. Dis. Model. Mech. 10, 499–502. doi: 10.1242/dmm.030205
Goswami, S., Kumar, N., Thawani, V., Tiwari, M., and Thawani, M. (2011). Effect of Bacopa monnieri on cognitive functions in Alzheimer’s disease patients. Int. J. Collab. Res. Intern. Med. Public Health 3, 285–293.
Gul, R., Jan, H., Lalay, G., Andleeb, A., Usman, H., Zainab, R., et al. (2021). Medicinal plants and biogenic metal oxide nanoparticles: A paradigm shift to treat Alzheimer’s disease. Coatings 11:717. doi: 10.3390/coatings11060717
Gupta, K., Agarwal, S., Kushwaha, A., Maurya, S., Chaturvedi, V., Pathak, R., et al. (2017). Oyster mushroom: A rich source of antioxidants. Incredible World Biotechnol. 1, 43–57.
Gupta, J., Fatima, M. T., Islam, Z., Khan, R. H., Uversky, V. N., and Salahuddin, P. (2019). Nanoparticle formulations in the diagnosis and therapy of Alzheimer’s disease. Int. J. Biol. Macromol. 130, 515–526. doi: 10.1016/j.ijbiomac.2019.02.156
Hamaguchi, T., Ono, K., and Yamada, M. (2010). REVIEW: curcumin and Alzheimer’s disease. CNS Neurosci. Ther. 16, 285–297. doi: 10.1111/j.1755-5949.2010.00147.x
Hassan, N. A., Alshamari, A. K., Hassan, A. A., Elharrif, M. G., Alhajri, A. M., Sattam, M., et al. (2022). Advances on therapeutic strategies for Alzheimer’s disease: from Medicinal plant to nanotechnology. Molecules 27:4839. doi: 10.3390/molecules27154839
Hassan, M. A., Balasubramanian, R., Masoud, A. D., Burkan, Z. E., Sughir, A., and Kumar, R. S. (2014). Role of Medicinal Plants in Neurodegenerative Diseases with Special Emphasis to Alzheimer’s. Phytomedicine 5, 454–462.
Hassan, T., Saeed, S., Hassan, M., Naseem, S., and Siddique, S. (2021). ETHNOMEDICINAL plants in the treatment of neurodegenerative diseases: A narrative review. Gomal J. Med. Sci. 19, 35–44. doi: 10.46903/gjms/19.01.958
Hou, Y., Dan, X., Babbar, M., Wei, Y., Hasselbalch, S. G., Croteau, D. L., et al. (2019). Ageing as a risk factor for neurodegenerative disease. Nat. Rev. Neurol. 15, 565–581. doi: 10.1038/s41582-019-0244-7
Huang, Y., Chang, Y., Liu, L., and Wang, J. (2021). Nanomaterials for modulating the aggregation of β-amyloid peptides. Molecules 26:4301. doi: 10.3390/molecules26144301
Hussain, G., Rasul, A., Anwar, H., Aziz, N., Razzaq, A., Wei, W., et al. (2018). Role of plant derived alkaloids and their mechanism in neurodegenerative disorders. Int. J. Biol. Sci. 14, 341–357. doi: 10.7150/ijbs.23247
Irwin, D. J., Lee, V. M.-Y., and Trojanowski, J. Q. (2013). Parkinson’s disease dementia: convergence of α-synuclein, tau and amyloid-β pathologies. Nat. Rev. Neurosci. 14, 626–636. doi: 10.1038/nrn3549
Jabbar, A. H., Hamzah, M. Q., Mezan, S. O., Binti Ameruddin, A. S., and Agam, M. A. (2018). Green synthesis of silver/ polystyrene Nano composite (ag/PS NCs) via plant extracts beginning a new era in drug delivery. Indian J. Sci. Technol. 11, 1–9. doi: 10.17485/ijst/2018/v11i22/121154
Jan, H., Shah, M., Andleeb, A., Faisal, S., Khattak, A., Rizwan, M., et al. (2021a). Plant-based synthesis of zinc oxide nanoparticles (ZnO-NPs) using aqueous leaf extract of Aquilegia pubiflora: their Antiproliferative activity against HepG2 cells inducing reactive oxygen species and other in vitro properties. Oxidative Med. Cell. Longev. 2021, 1–14. doi: 10.1155/2021/4786227
Jan, H., Zaman, G., Usman, H., Ansir, R., Drouet, S., Gigliolo-Guivarch, N., et al. (2021b). Biogenically proficient synthesis and characterization of silver nanoparticles (ag-NPs) employing aqueous extract of Aquilegia pubiflora along with their in vitro antimicrobial, anti-cancer and other biological applications. J. Mater. Res. Technol. 15, 950–968. doi: 10.1016/j.jmrt.2021.08.048
Jivad, N., and Rabiei, Z. (2014). A review study on medicinal plants used in the treatment of learning and memory impairments. Asian Pac. J. Trop. Biomed. 4, 780–789. doi: 10.12980/APJTB.4.2014APJTB-2014-0412
John, T., Samuel, B., Abolaji, O., Folashade, O., Oyetooke, A., and Oluwatosin, F. (2020). Functional foods and bioactive compounds: roles in the prevention, treatment and management of neurodegenerative diseases. GSC Biological and Pharmaceutical Sciences 11, 297–313. doi: 10.30574/gscbps.2020.11.2.0143
Jyoti, A., and Sharma, D. (2006). Neuroprotective role of Bacopa monniera extract against aluminium-induced oxidative stress in the hippocampus of rat brain. Neurotoxicology 27, 451–457. doi: 10.1016/j.neuro.2005.12.007
Kalimuthu, K., Cha, B. S., Kim, S., and Park, K. S. (2020). Eco-friendly synthesis and biomedical applications of gold nanoparticles: A review. Microchem. J. 152:104296. doi: 10.1016/j.microc.2019.104296
Khan, H., Ullah, H., Aschner, M., Cheang, W. S., and Akkol, E. K. (2019). Neuroprotective effects of quercetin in Alzheimer’s disease. Biomol. Ther. 10:59. doi: 10.3390/biom10010059
Khazdair, M. R., Kianmehr, M., and Anaeigoudari, A. (2020). Effects of Medicinal plants and flavonoids on Parkinson’s disease, a review on basic and clinical evidences. Adv. Pharm Bull. 11:224.doi: 10.34172/apb.2021.026
Khuwaja, G., Khan Mohd, M., Ishrat, T., Ahmad, A., Raza, S. S., Ashafaq, M., et al. (2011). Neuroprotective effects of curcumin on 6-hydroxydopamine-induced parkinsonism in rats: behavioral, neurochemical and immunohistochemical studies. Brain Res. 1368, 254–263. doi: 10.1016/j.brainres.2010.10.023
Klegeris, A., and McGeer, P. (2005). Non-steroidal anti-inflammatory drugs (NSAIDs) and other anti- inflammatory agents in the treatment of neurodegenerative disease. Curr. Alzheimer Res. 2, 355–365. doi: 10.2174/1567205054367883
Ko, W.-C., Wang, S.-J., Hsiao, C.-Y., Hung, C.-T., Hsu, Y.-J., Chang, D.-C., et al. (2022). Pharmacological role of functionalized gold nanoparticles in disease applications. Molecules 27:1551. doi: 10.3390/molecules27051551
Kuang, Y., Zhang, J., Xiong, M., Zeng, W., Lin, X., Yi, X., et al. (2020). A novel Nanosystem realizing curcumin delivery based on Fe3O4@carbon dots nanocomposite for Alzheimer’s disease therapy. Front. Bioeng. Biotechnol. 8:614906. doi: 10.3389/fbioe.2020.614906
Lai Shi Min, S., Liew, S. Y., Chear, N. J. Y., Goh, B. H., Tan, W.-N., and Khaw, K. Y. (2022). Plant Terpenoids as the promising source of cholinesterase inhibitors for anti-AD therapy. Biology 11:307. doi: 10.3390/biology11020307
Lee, H., Kim, Y., Park, A., and Nam, J. (2014). Amyloid-β aggregation with gold nanoparticles on brain lipid bilayer. Small 10, 1779–1789. doi: 10.1002/smll.201303242
Li, X., Smid, S. D., Lin, J., Gong, Z., Chen, S., You, F., et al. (2019). Neuroprotective and anti-amyloid β effect and Main chemical profiles of white tea: comparison against green. Oolong Black Tea Mol. 24:1926. doi: 10.3390/molecules24101926
Liao, Y., Mai, X., Wu, X., Hu, X., Luo, X., and Zhang, G. (2022). Exploring the inhibition of quercetin on acetylcholinesterase by multispectroscopic and in silico approaches and evaluation of its neuroprotective effects on PC12 cells. Molecules 27:7971. doi: 10.3390/molecules27227971
Maiti, P., and Dunbar, G. (2018). Use of curcumin, a natural polyphenol for targeting molecular pathways in treating age-related neurodegenerative diseases. Int. J. Mol. Sci. 19:1637. doi: 10.3390/ijms19061637
Malik, J., Choudhary, S., and Kumar, P. (2013). Plants and phytochemicals for Huntington′s disease. Pharmacogn. Rev. 7:81. doi: 10.4103/0973-7847.120505
Martano, S., De Matteis, V., Cascione, M., and Rinaldi, R. (2022). Inorganic nanomaterials versus polymer-based nanoparticles for overcoming neurodegeneration. Nano 12:2337. doi: 10.3390/nano12142337
Masoudi Asil, S., Ahlawat, J., Guillama Barroso, G., and Narayan, M. (2020). Nanomaterial based drug delivery systems for the treatment of neurodegenerative diseases. Biomater. Sci. 8, 4109–4128. doi: 10.1039/D0BM00809E
Mathew, A., Fukuda, T., Nagaoka, Y., Hasumura, T., Morimoto, H., Yoshida, Y., et al. (2012). Curcumin loaded-PLGA nanoparticles conjugated with Tet-1 peptide for potential use in Alzheimer’s disease. PLoS One 7:e32616. doi: 10.1371/journal.pone.0032616
Mikhailova, E. O. (2021). Gold nanoparticles: biosynthesis and potential of biomedical application. J. Funct. Biomater 12:70. doi: 10.3390/jfb12040070
Mishra, S., and Palanivelu, K. (2008). The effect of curcumin (turmeric) on Alzheimer′s disease: an overview. Ann. Indian Acad. Neurol. 11:13. doi: 10.4103/0972-2327.40220
Mishrikoti, S. M., Isaq, F., Kamble, G. R., and Kulkarni, B. D. (2022). Management of Neurodegenerative Diseases using Medicinal plants – A review. Res. J. Biotechnol. 17, 145–152. doi: 10.25303/1709rjbt1450152
Mulik, R. S., Mönkkönen, J., Juvonen, R. O., Mahadik, K. R., and Paradkar, A. R. (2010). ApoE3 mediated poly(butyl) cyanoacrylate nanoparticles containing curcumin: study of enhanced activity of curcumin against Beta amyloid induced cytotoxicity using in vitro cell culture model. Mol. Pharm. 7, 815–825. doi: 10.1021/mp900306x
Naseri, M., Delfani, H., Mirzaeei, S., Bahrami, G., Farzaei, M. H., and Mohammadi Pour, P. (2022). Protective effect of nanoparticles of oleoresin of Pistacia atlantica var. mutica against acetic acid-induced ulcerative colitis in rats. Tradit. Integ. Med. doi: 10.18502/tim.v7i2.9915
Nellore, J., Pauline, C., and Amarnath, K. (2013). Bacopa monnieri phytochemicals mediated synthesis of platinum nanoparticles and its Neurorescue effect on 1-methyl 4-phenyl 1,2,3,6 Tetrahydropyridine-induced experimental parkinsonism in zebrafish. J. Neurodegener Dis. 2013, 1–8. doi: 10.1155/2013/972391
Nguyen, T. T., Nguyen, T. T. D., Nguyen, T. K. O., Vo, T. K., and Vo, V. G. (2021). Advances in developing therapeutic strategies for Alzheimer’s disease. Biomed. Pharmacother. 139:111623. doi: 10.1016/j.biopha.2021.111623
Omoruyi, S. I., Ibrakaw, A. S., Ekpo, O. E., Boatwright, J. S., Cupido, C. N., and Hussein, A. A. (2021). Neuroprotective activities of Crossyne flava bulbs and Amaryllidaceae alkaloids: implications for Parkinson’s disease. Molecules 26:3990. doi: 10.3390/molecules26133990
Ovais, M., Zia, N., Ahmad, I., Khalil, A. T., Raza, A., Ayaz, M., et al. (2018). Phyto-therapeutic and Nanomedicinal approaches to cure Alzheimer’s disease: present status and future opportunities. Front. Aging Neurosci. 10:284. doi: 10.3389/fnagi.2018.00284
Pérez-Hernández, J., Zaldívar-Machorro, V. J., Villanueva-Porras, D., Vega-Ávila, E., and Chavarría, A. (2016). A Potential Alternative against Neurodegenerative Diseases: Phytodrugs. (2016). Potent. Alternat. Neurodegen. Dis. :2016.
Poka, L. P., Mohan, G. K., Rao, K. V., and Shanker, K. (2017). Neuroprotective effect of green synthesized Iron oxide nanoparticles using aqueous extract of Convolvulus Pluricaulis Plant in the Management of Alzheimer’s disease. Int. J. Pharmacognosy Phytochem. Res. 9. doi: 10.25258/phyto.v9i5.8152
Popovic, N., and Brundin, P. (2006). Therapeutic potential of controlled drug delivery systems in neurodegenerative diseases. Int. J. Pharm. 314, 120–126. doi: 10.1016/j.ijpharm.2005.09.040
Pradeep, S., Prabhuswaminath, S. C., Reddy, P., Srinivasa, S. M., Shati, A. A., Alfaifi, M. Y., et al. (2022). Anticholinesterase activity of Areca Catechu: in vitro and in silico green synthesis approach in search for therapeutic agents against Alzheimer’s disease. Front. Pharmacol. 13:1044248. doi: 10.3389/fphar.2022.1044248
Pradhan, N., Debnath, K., Mandal, S., Jana, N. R., and Jana, N. R. (2018). Antiamyloidogenic chemical/biochemical-based designed nanoparticle as artificial chaperone for efficient inhibition of protein aggregation. Biomacromolecules 19, 1721–1731. doi: 10.1021/acs.biomac.8b00671
Prasanna, P., and Upadhyay, A. (2021). Flavonoid-based nanomedicines in Alzheimer’s disease therapeutics: promises made, a long way to go. ACS Pharmacol. Transl. Sci. 4, 74–95. doi: 10.1021/acsptsci.0c00224
Rai, M., Ingle, A., Gupta, I., Birla, S., Yadav, A., and Abd-Elsalam, K. (2013). Potential role of biological Systems in Formation of nanoparticles: mechanism of synthesis and biomedical applications. Curr. Nanosci. 9, 576–587. doi: 10.2174/15734137113099990092
Rajakumar, G., Gomathi, T., Thiruvengadam, M., Devi Rajeswari, V., Kalpana, V. N., and Chung, I.-M. (2017). Evaluation of anti-cholinesterase, antibacterial and cytotoxic activities of green synthesized silver nanoparticles using from Millettia pinnata flower extract. Microb. Pathog. 103, 123–128. doi: 10.1016/j.micpath.2016.12.019
Rajamanickam, G., and Manju, S. L. (2022). A review on phyto-nanotechnology for therapy of alzheimer’s disease. Indian J. Biochem. Biophys. 59, 867–872. doi: 10.56042/ijbb.v59i9.57758
Ramirez-Nuñez, A. L., Jimenez-Garcia, L. F., Goya, G. F., Sanz, B., and Santoyo-Salazar, J. (2018). In vitro magnetic hyperthermia using polyphenol-coated Fe 3 O 4 @ Fe2 O 3 nanoparticles from Cinnamomun verum and Vanilla planifolia: the concert of green synthesis and therapeutic possibilities. Nanotechnology 29:074001. doi: 10.1088/1361-6528/aaa2c1
Rasool, M., Malik, A., Qureshi, M. S., Manan, A., Pushparaj, P. N., Asif, M., et al. (2014). Recent updates in the treatment of neurodegenerative disorders using natural compounds. Evid. Based Complement. Alternat. Med. 2014, 1–7. doi: 10.1155/2014/979730
Rauf, M. A., Alam, M. T., Ishtikhar, M., Ali, N., Alghamdi, A., and AlAsmari, A. F. (2022). Investigating chaperone like activity of green silver nanoparticles: possible implications in drug development. Molecules 27:944. doi: 10.3390/molecules27030944
Rezai-Zadeh, K., Arendash, G. W., Hou, H., et al. (2008). Green tea epigallocatechin-3-gallate (EGCG) reduces β-amyloid mediated cognitive impairment and modulates tau pathology in Alzheimer transgenic mice. Brain Res. 1214, 177–187. doi: 10.1016/j.brainres.2008.02.107
Ríos, J.-L., Onteniente, M., Picazo, D., and Montesinos, M.-C. (2016). Medicinal plants and natural products as potential sources for Antiparkinson drugs. Planta Med. 82, 942–951. doi: 10.1055/s-0042-107081
Roos, R. A. (2010). Huntington’s disease: a clinical review. Orphanet J. Rare Dis. 5:40. doi: 10.1186/1750-1172-5-40
Ross, C. A., Aylward, E. H., Wild, E. J., Langbehn, D. R., Long, J. D., Warner, J. H., et al. (2014). Huntington disease: natural history, biomarkers and prospects for therapeutics. Nat. Rev. Neurol. 10, 204–216. doi: 10.1038/nrneurol.2014.24
Sachdeva, A. K., Kuhad, A., and Chopra, K. (2014). Naringin ameliorates memory deficits in experimental paradigm of Alzheimer’s disease by attenuating mitochondrial dysfunction. Pharmacol. Biochem. Behav. 127, 101–110. doi: 10.1016/j.pbb.2014.11.002
Sahni, J. K., Doggui, S., Ali, J., Baboota, S., Dao, L., and Ramassamy, C. (2011). Neurotherapeutic applications of nanoparticles in Alzheimer’s disease. J. Control. Release 152, 208–231. doi: 10.1016/j.jconrel.2010.11.033
Sandhir, R., Yadav, A., Mehrotra, A., Sunkaria, A., Singh, A., and Sharma, S. (2014). Curcumin nanoparticles attenuate neurochemical and neurobehavioral deficits in experimental model of Huntington’s disease. NeuroMolecular Med. 16, 106–118. doi: 10.1007/s12017-013-8261-y
Saratale, R. G., Karuppusamy, I., Saratale, G. D., Pugazhendhi, A., Kumar, G., Park, Y., et al. (2018). A comprehensive review on green nanomaterials using biological systems: recent perception and their future applications. Colloids Surf. B Biointerfaces 170, 20–35. doi: 10.1016/j.colsurfb.2018.05.045
Savitt, J. M., Dawson, V. L., and Dawson, T. M. (2006). Diagnosis and treatment of Parkinson disease: molecules to medicine. J. Clin. Invest. 116, 1744–1754. doi: 10.1172/JCI29178
Saxena, G., Singh, S. P., Pal, R., Singh, S., Pratap, R., and Nath, C. (2007). Gugulipid, an extract of Commiphora whighitii with lipid-lowering properties, has protective effects against streptozotocin-induced memory deficits in mice. Pharmacol. Biochem. Behav. 86, 797–805. doi: 10.1016/j.pbb.2007.03.010
Scatena, R., Martorana, G. E., Bottoni, P., Botta, G., Pastore, P., and Giardina, B. (2007). An update on pharmacological approaches to neurodegenerative diseases. Expert Opin. Investig. Drugs 16, 59–72. doi: 10.1517/13543784.16.1.59
Schapira, A. H. V. (2005). Present and future drug treatment for Parkinson’s disease. J. Neurol. Neurosurg. Psychiatry 76, 1472–1478. doi: 10.1136/jnnp.2004.035980
Shabbir, U., Rubab, M., Tyagi, A., and Oh, D.-H. (2020). Curcumin and its derivatives as Theranostic agents in Alzheimer’s disease: the implication of nanotechnology. Int. J. Mol. Sci. 22:196. doi: 10.3390/ijms22010196
Sharifi-Rad, J., Rapposelli, S., Sestito, S., Herrera-Bravo, J., Arancibia-Diaz, A., Salazar, L. A., et al. (2022). Multi-target mechanisms of phytochemicals in Alzheimer’s disease: effects on oxidative stress, Neuroinflammation and protein aggregation. J. Pers. Med. 12:1515. doi: 10.3390/jpm12091515
Singh, A., Gupta, A., Mishra, A., Gupta, V., Bansal, P., and Kumar, S. (2010). Medicinal Plant for Curing Alzheimer’s disease. International Journal of Pharmaceutical & Biological Archives 1, 108–114.
Singh, P., Mishra, G., Molla, M., Shumet Yimer, Y., Sisay, W., Andargie, Y., et al. (2022). Dietary and nutraceutical-based therapeutic approaches to combat the pathogenesis of Huntington’s disease. J. Funct. Foods 92:105047. doi: 10.1016/j.jff.2022.105047
Solanki, I., Parihar, P., and Parihar, M. S. (2016). Neurodegenerative diseases: from available treatments to prospective herbal therapy. Neurochem. Int. 95, 100–108. doi: 10.1016/j.neuint.2015.11.001
Sriramcharan, P., Natarajan, J., Raman, R., Nagaraju, G., Justin, A., and Senthil, V. (2022). A review on green-synthesis of cerium oxide nanoparticles: focus on central nervous system disorders. Int. J. Appl. Pharm. 14:94. doi: 10.22159/ijap.2022v14i4.44487
Subaraja, M., Arokiyaraj, S., and Mathew, P. (2024). Neuroprotective effect of huperzine-A against cadmium chloride-induced Huntington’s disease in Drosophila melanogaster model. J. King Saud Univ. Sci. 36:103319. doi: 10.1016/j.jksus.2024.103319
Suganthy, N., Sri Ramkumar, V., Pugazhendhi, A., Benelli, G., and Archunan, G. (2018). Biogenic synthesis of gold nanoparticles from Terminalia arjuna bark extract: assessment of safety aspects and neuroprotective potential via antioxidant, anticholinesterase, and antiamyloidogenic effects. Environ. Sci. Pollut. Res. 25, 10418–10433. doi: 10.1007/s11356-017-9789-4
Tang, M., and Taghibiglou, C. (2017). The mechanisms of action of curcumin in Alzheimer’s disease. J. Alzheimers Dis. 58, 1003–1016. doi: 10.3233/JAD-170188
Tang, B. C., Wang, Y. T., and Ren, J. (2023). Basic information about memantine and its treatment of Alzheimer’s disease and other clinical applications. Ibrain 9, 340–348. doi: 10.1002/ibra.12098
Testa, G., Gamba, P., Badilli, U., Gargiulo, S., Maina, M., Guina, T., et al. (2014). Loading into nanoparticles improves Quercetin’s efficacy in preventing Neuroinflammation induced by oxysterols. PLoS One 9:e96795. doi: 10.1371/journal.pone.0096795
Veerendra Kumar, M., and Gupta, Y. (2003). Effect of Centella asiatica on cognition and oxidative stress in an intracerebroventricular streptozotocin model of Alzheimer’s disease in rats. Clin. Exp. Pharmacol. Physiol. 30, 336–342. doi: 10.1046/j.1440-1681.2003.03842.x
Velander, P., Wu, L., Henderson, F., Zhang, S., Bevan, D. R., and Xu, B. (2017). Natural product-based amyloid inhibitors. Biochem. Pharmacol. 139, 40–55. doi: 10.1016/j.bcp.2017.04.004
Venuto, C. S., McGarry, A., Ma, Q., and Kieburtz, K. (2012). Pharmacologic approaches to the treatment of Huntington’s disease. Mov. Disord. 27, 31–41. doi: 10.1002/mds.23953
Wang, B., Pilkington, E. H., Sun, Y., Davis, T. P., Ke, P. C., and Ding, F. (2017). Modulating protein amyloid aggregation with nanomaterials. Environ. Sci. Nano 4, 1772–1783. doi: 10.1039/C7EN00436B
Woon, C. K., Hui, W. K., Abas, R., Haron, M. H., Das, S., and Lin, T. S. (2022). Natural product-based nanomedicine: recent advances and issues for the treatment of Alzheimer’s disease. Curr. Neuropharmacol. 20, 1498–1518. doi: 10.2174/1570159X20666211217163540
Xing, M., Ge, L., Wang, M., Li, Q., Li, X., and Ouyang, J. (2014). Nanosilver particles in medical applications: synthesis, performance, and toxicity. Int. J. Nanomedicine 2399–2407. doi: 10.2147/IJN.S55015
Xue, J., Liu, T., Liu, Y., Jiang, Y., Seshadri, V. D. D., Mohan, S. K., et al. (2019). Neuroprotective effect of biosynthesised gold nanoparticles synthesised from root extract of Paeonia moutan against Parkinson disease – In vitro & In vivo model. J. Photochem. Photobiol. B 200:111635. doi: 10.1016/j.jphotobiol.2019.111635
Yadav, E., Singh, D., Yadav, P., and Verma, A. (2018). Comparative Evaluation of Prosopis cineraria (L.) Druce and Its ZnO Nanoparticles on Scopolamine Induced Amnesia. Front Pharmacol :9. doi: 10.3389/fphar.2018.00549
Yan, L., Guo, M.-S., Zhang, Y., Yu, L., Wu, J.-M., Tang, Y., et al. (2022). Dietary plant polyphenols as the potential drugs in neurodegenerative diseases: current evidence, advances, and opportunities. Oxidative Med. Cell. Longev. 2022, 1–40. doi: 10.1155/2022/5288698
Yang, R., Zheng, Y., Wang, Q., and Zhao, L. (2018). Curcumin-loaded chitosan–bovine serum albumin nanoparticles potentially enhanced Aβ 42 phagocytosis and modulated macrophage polarization in Alzheimer’s disease. Nanoscale Res. Lett. 13:330. doi: 10.1186/s11671-018-2759-z
Yassin, N. A. Z., El-Shenawy, S. M. A., Mahdy, K. A., Gouda, N. A., Marrie, A. E.-F. H., Farrag, A. R. H., et al. (2013). Effect of Boswellia serrata on Alzheimer’s disease induced in rats. J. Arab Soci. Med. Res. 8, 1–11. doi: 10.7123/01.JASMR.0000429323.25743.cc
Yavarpour-Bali, H., Pirzadeh, M., and Ghasemi-Kasman, M. (2019). Curcumin-loaded nanoparticles: A novel therapeutic strategy in treatment of central nervous system disorders. Int. J. Nanomedicine 14, 4449–4460. doi: 10.2147/IJN.S208332
Youssif, K. A., Haggag, E. G., Elshamy, A. M., Rabeh, M. A., Gabr, N. M., Seleem, A., et al. (2019). Anti-Alzheimer potential, metabolomic profiling and molecular docking of green synthesized silver nanoparticles of Lampranthus coccineus and Malephora lutea aqueous extracts. PLoS One 14:e0223781. doi: 10.1371/journal.pone.0223781
Zhang, X., Li, Y., and Hu, Y. (2020). Green synthesis of silver nanoparticles and their preventive effect in deficits in recognition and spatial memory in sporadic Alzheimer’s rat model. Colloids Surf. Physicochem. Eng. Asp. 605:125288. doi: 10.1016/j.colsurfa.2020.125288
Keywords: amyloid, green synthesis, nanoparticles, neurodegenerative diseases, medicinal plants
Citation: Izadi R, Bahramikia S and Akbari V (2024) Green synthesis of nanoparticles using medicinal plants as an eco-friendly and therapeutic potential approach for neurodegenerative diseases: a comprehensive review. Front. Neurosci. 18:1453499. doi: 10.3389/fnins.2024.1453499
Edited by:
Suman Chowdhury, The State University of New Jersey, United StatesReviewed by:
Vivek K. Chaturvedi, Banaras Hindu University, IndiaShivani Kumar, Guru Gobind Singh Indraprastha University, India
Copyright © 2024 Izadi, Bahramikia and Akbari. This is an open-access article distributed under the terms of the Creative Commons Attribution License (CC BY). The use, distribution or reproduction in other forums is permitted, provided the original author(s) and the copyright owner(s) are credited and that the original publication in this journal is cited, in accordance with accepted academic practice. No use, distribution or reproduction is permitted which does not comply with these terms.
*Correspondence: Seifollah Bahramikia, YmFocmFtaWtpYS5zQGx1LmFjLmly