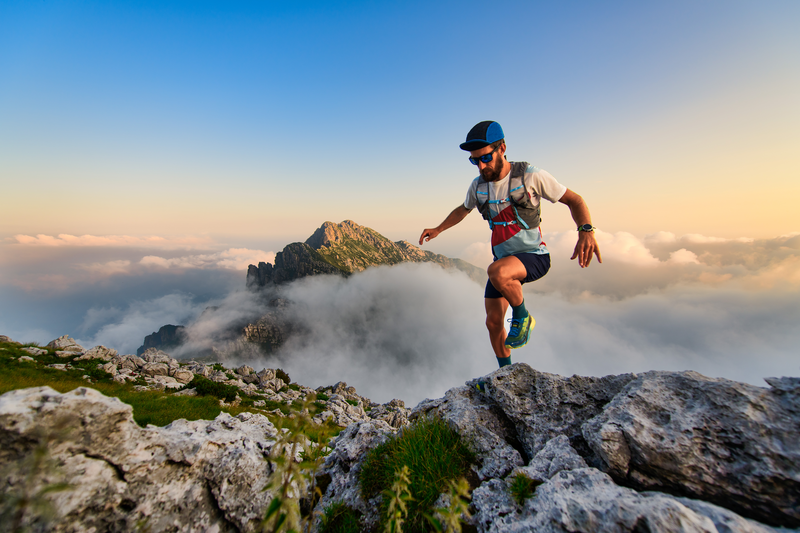
94% of researchers rate our articles as excellent or good
Learn more about the work of our research integrity team to safeguard the quality of each article we publish.
Find out more
REVIEW article
Front. Neurosci. , 06 October 2023
Sec. Neural Technology
Volume 17 - 2023 | https://doi.org/10.3389/fnins.2023.1247021
During the past decades, the potential effects of extremely low-frequency electromagnetic fields (ELF-EMFs) on human health have gained great interest all around the world. Though the International Commission on Non-Ionizing Radiation Protection recommended a 100 μT, and then a 200 μT magnetic field limit, the long-term effects of ELF-EMFs on organisms and systems need to be further investigated. It was reported that both electrotherapy and possible effects on human health could be induced under ELF-EM radiation with varied EM frequencies and fields. This present article intends to systematically review the in vivo experimental outcome and the corresponding mechanisms to shed some light on the safety considerations of ELF-EMFs. This will further advance the subsequent application of electrotherapy in human health.
Extremely low-frequency electromagnetic fields (ELF-EMFs) usually refer to alternating electromagnetic fields ranging from 1 to 100 Hz (Raggi et al., 2008) or 300 Hz (Sang-Kon et al., 2016), among which the power transmission frequency of 50 or 60 Hz is closely associated with our working and living environments. As shown in Figure 1, electrical fields can be produced by a high-voltage power transmission line, and induced current and magnetic fields would be generated inside biological systems, which might have a potential impact on the human body. During the past decades, the evaluation of the effects of ELF-EMFs on biological and human health has received significant attention (Carlberg et al., 2017; Turner et al., 2017; Consales et al., 2018; Jalilian et al., 2018; Zhang et al., 2018; Lee et al., 2020; Carnecka et al., 2022).
Figure 1. Illustration of biological effects of extremely low-frequency electromagnetic fields (ELF-EMFs) from power transmission lines, electrical appliances, etc.
The International Commission on Non-Ionizing Radiation Protection (ICNIRP) recommended a magnetic induction limit of 100 μT for power frequency magnetic fields but further reassessed and revised the limitation to 200 μT in 2010 (ICNIRP, 2010) based on epidemiologic, in vitro, and in vivo studies of ELF-EMFs' health effects. Among all the research, experimental studies offer advantages over epidemiological ones, such as controllable variables, high repeatability, and the ability to support causal inferences. In the past few decades, experimental research on the biological effects of ELF-EMFs has made significant contributions at multiple levels, including genes, cells, tissues, and biological systems.
On the cellular level, biological activities include cell responses such as cell proliferation, differentiation, and apoptosis and resulting functional alterations, which can lead to either beneficial or adverse outcomes depending on the specific cell type and characteristics of the electromagnetic fields. Among them, cell proliferation is one of the most basic and crucial activities for cell growth, development, tissue repair, and structural and functional maintenance in organisms. On the one hand, some studies showed that exposure to ELF-EMFs had an inhibitory effect on cell proliferation. For instance, Manni et al. (2004) pointed out that human oral keratinocyte (HOK) cells exposed to an ELF-EMF of 2 mT at 50 Hz produced smaller clones than the control group and exhibited a decreased cell growth rate. In addition to normal cells, the findings from Lu et al. (2019) also confirmed this negative effect on tumor cell proliferation, possibly due to the induction of intracellular reactive oxygen species (ROS) under exposure to a combined static magnetic field and ELF-EMF of 5.1 mT at 50 Hz for 2h/day. Fathi and Farahzadi (2018) investigated the impact of ELF-EMF exposure on rat adipose tissue-derived mesenchymal stem cells (rADSCs) and observed a reduction in the proliferation rate of these cells. The same reduction was also validated by Cho et al. (2012) in human bone marrow-derived mesenchymal stem cells (hBM-MSCs) under a 12-day exposure to ELF-EMF. On the other hand, some other studies suggested that exposure to ELF-EMFs could promote cell proliferation, which further enhanced the therapeutic effects of low-frequency EMF on various diseases, including skin wounds (Manni et al., 2002; Huo et al., 2010; Lv et al., 2021) and orthopedic diseases (Li et al., 2018). Human keratinocytes (HaCaT) were found to have an increase in cellular growth and proliferation under ELF-EMFs through in vitro experiments (Manni et al., 2002; Cricenti et al., 2008; Vianale et al., 2008). The proliferation of rat calvarial osteoblasts and the formation of bone-like nodules were confirmed in Bodamyali et al. (1998) study under pulsed electromagnetic fields at 15 Hz for 6 h. The experiment conducted by Zhang et al. (2006) further manifested that a moderate-intensity magnetic field (1, 5, 10, and 20 mT) at 15 Hz with an exposure time of 30 min per day for 2 days further validated the significant increase of rat calvarial osteoblasts. In vitro experiments were further conducted on human epidermal stem cells, and the results supported the promotive effects of ELF-EMFs on cell proliferation in a frequency-dependent manner, with the most preferred frequency being 50 Hz (Zhang et al., 2013). In addition to cell proliferation, other cellular responses were also studied. An in vitro study by Kula et al. (2002) showed no obvious changes in the peroxidation of membrane structures in fibroblasts exposed to a static 0.49 T magnetic field or an ELF-EMF of 20 mT at 50 Hz. It was also reported that obese mice can lose weight under the treatment of 7.5 Hz, 0.4 T ELF-EMF, possibly due to the inhibition of adipogenic differentiation of mesenchymal stem cells, but there is no effect on osteogenic differentiation with ELF-EMF (Du et al., 2014).
The ELF-EMFs can affect the expression of some genes while exerting no obvious influence on others. For inhibitory effects, Mahaki et al. (2019a) showed that the expression levels of transcription factor Maf (c-Maf), signal transducer and activator of transcription 6 (STAT6), and retinoid-related orphan receptor alpha (RORa) in the spleen of rats decreased significantly after 2 months of exposure to the ELF-EMFs. Sun et al. (2009) showed that the frequency and exposure duration of the electromagnetic field had a great influence on the expression of the cell apoptosis-related proteins Bax and Bcl-2, and EMF would gradually reduce the expression of Bcl-2. Hu et al. (2006) showed that the adherence-related OPN gene expression level in vascular smooth muscle cells in ELF-EMF-exposed experimental groups was lower than that in the control group, and the inhibition effect was enhanced with the increase in EMF intensity. On the other hand, Ebrahimi et al. (2019) indicated that the expression levels of PROK and Cyp 17 increased, but the difference was not statistically significant. Mahaki et al. (2019a) showed that after 2 months of exposure to ELF-EMF, the expression of c-Maf, STAT6, and RORa in the thymus of rats did not decrease. Studies conducted by Reyes-Guerrero et al. (2010) indicated that there was no significant difference in the expression of estrogen receptor-α (ER-α) and estrogen receptor-β (ER-β) genes. Exposure to ELF-EMF during both prenatal and postnatal phases results in heightened IL-17A levels within the spleen and bloodstream of juvenile female rats. This exposure also triggered an increase in the expression of the IL-17 gene in the spleen, leading to the proliferation of CD4+ cells and an inflammatory response (Ozturk et al., 2022).
In the aforementioned studies on the cellular and gene level effects, although various studies have yielded either positive or negative findings, in vitro experiments alone cannot fully capture the interplay of diverse bodily systems in response to external stimuli. Therefore, in vivo studies are more suitable for comprehensively assessing the overall impact of experiments on living subjects. In this review, we aimed to systematically review in vivo experimental studies investigating the systemic effects of EMF and provide a comprehensive overview of the underlying mechanisms through selected in vitro experiments. In this way, we hope to address knowledge gaps and provide direction for future investigations.
In experimental research on the effects of ELF-EMFs, the accurate construction of controlled and reproducible environments is of paramount importance. The process of constructing appropriate ELF-EMF environments involves various considerations, including the choice of experimental apparatus, field strength, coil size, and turns per coil. Understanding the different methods and approaches used to generate ELF-EMF environments is essential for researchers seeking to design rigorous experiments and contribute to the advancement of knowledge in this field.
Experimentally, there are two main methods to generate the ELF-EMF: either a single solenoid or coils parallel to each other, as shown in Figure 2. In the cell experiment of Zhang et al. (2006), the electromagnetic emission apparatus consisted of a tightly wound solenoid coil with an inner diameter of 5 cm and a length of 50 cm, ensuring a relatively uniform magnetic field along the axis near the center of the coil. Mahaki et al. (2019a) used solenoids with a diameter of 40 cm and a length of 2 m to explore the effects of ELF-EMFs on certain gene expression in the rat spleen and thymus. During the experiment, changeable field intensities of 1, 100, 500, and 2000 μT were generated by combining different turns of wire and voltage. However, the solenoid's excessive length of 2 m made it challenging to assess spatial homogeneity. The magnetic field generator built by Mao et al. (2018) was composed of a pair of copper coils with a vertical distance of 70 mm, and each coil had 160 turns with an inner diameter of 440 mm and a height of 70 mm. A voltage-regulating transformer was used to modulate the current added to the coil, and an AC voltage ammeter was utilized to detect the current in the coil. The results showed that the magnetic field was uniform and stable, with the frequency fixed at 50 Hz, and the magnetic induction intensity was continuously adjustable from 0 to 0.5 mT. As for the device constructed by Lu et al. (2019), the two 30 cm-diameter coils were placed 11 cm apart and could generate a static magnetic field and ELF-EMF at a frequency of 50 Hz by direct current. In addition to self-built copper coils, Helmholtz coils were also utilized by some groups (Griefahn et al., 2001; Jeong et al., 2005; Zhang et al., 2013) to generate ELF-EMF. In the experiment of Zhang et al. (2013), the two coils were 15 ± 0.3 cm in diameter and were placed 15 ± 0.5 cm apart. The results showed that ELF-EMF with a magnetic field intensity of 5 mT and a frequency of 1 to 50 Hz could be produced. To ensure sufficient living space for the animals in the experiment, large-diameter copper coils were employed in Zhang Y. et al.'s (2016) and Liu et al.'s (2018) studies. The device's size was 2 m in length, 1 m in width, and 2 m in height, with a distance between the adjacent coils of 0.35 m. Among the coils, the ones on the top and bottom were wrapped with 400 turns of copper wire, while the three coils in the middle were wrapped with 200 turns. After being connected to the voltage-regulating transformer and powered by the voltage-regulating power supply, a magnetic field with an intensity of 0–2 mT was generated.
Figure 2. Construction of an extremely low-frequency electromagnetic field environment with a solenoid or coils. (A) Schematic diagram of generating a magnetic field with current passing through the solenoid based on the right-hand screw rule. (B) The reproduction of the magnetic field within the coils. (C) Schematic diagram illustrating the generation of a magnetic field in the animals' living space after the coils are energized.
In summary, in vitro experiments have lower spatial requirements and can achieve a uniformly distributed magnetic field using small-sized coils/solenoids. On the other hand, for animal experiments, where a uniform magnetic field is required in a larger space, coils are more preferred than solenoids.
The impact of EMF on the immune system has garnered significant attention in recent years. The immune system comprises intricately structured cells, tissues, and organs. The functions of the thymus, spleen, and macrophage phagocytosis; serum levels of antibodies and cytokines; and the number of immune cells are common indicators for evaluating the immune system's function. Among them, the thymus, spleen, and macrophage phagocytosis indexes are closely associated with non-specific immune function, while the serum levels of antibody IgG can be measured in relation to humoral immune function. Existing reviews have summarized the cellular variations induced by EMF exposure as observed in epidemiological studies and in vitro experiments (Boscolo et al., 2007; Santini et al., 2009). However, to comprehensively evaluate the impact on the immune system's functionality, it remains crucial to consolidate conclusions derived from in vivo experiments. Table 1 shows that exposure to ELF-EMFs can have a positive or negative influence on biological immune function.
For immune organ function, Quaglino et al. (2004) conducted long-term in vivo exposure to 50-Hz ELF-EMF on Sprague-Dawley (SD) rats for 8 months, and the results demonstrated gradual atrophy in the rat thymus caused by collagen deposition and fat substitution. For studies in BALB/c mice, Zhu (2010) found that the low-dose-treatment mice induced an increase in thymus, spleen, and macrophage phagocytosis indexes at 1 mT and 4.5 mT. By comparison, all these indexes and levels of antibody IgG significantly decreased in the high-dose treatment group at 9 mT. This study indicated that low-dose ELF-EMFs were likely to contribute to human health, while a strong magnetic field could weaken the immune system. Zhang H. et al. (2016) denoted that an ELF-EMF of 8 mT at 50 Hz could decrease the concentration of thymus and spleen indexes and weaken the proliferation of T- and B-type lymphocytes, which implied that high-dose magnetic fields would have certain adverse effects on the biological immune system.
Immune cells such as white blood cells, lymphocytes, and natural killer (NK) cells play a vital role in the immune system. de Kleijn et al. (2016) exposed BalB/c mice to the ELF-EMF of 10 μT for 1 week and 15 weeks, respectively and observed a significant increase in leukocyte counts under short-term exposure. Furthermore, Zhang Y. et al. (2016) exerted a 100-μT magnetic field at 50 Hz on SD rats for 12 consecutive weeks, and the outcome showed no significant effects on the peripheral hematopoietic system without influencing the number of white blood cells, the neutrophil ratio, or the lymphocyte ratio. Canseven et al. (2006) applied a 50-Hz 2-mT EMF for 5 days to guinea pigs and found a decrease in NK cell cytotoxic activity in the spleens of the exposed animals.
Recently, researchers have shown increased interest in investigating alterations in cytokines in serum. Mahaki et al. (2020) exposed rats to 50 Hz EMFs at intensities of 1 and 100 μT and observed the reduction of pro-inflammatory cytokines such as IL-9 and TNF-α, alongside the increase of the anti-inflammatory cytokine IL-10, which could potentially trigger an anti-inflammatory response. The results from Wyszkowska et al. (2018) revealed that a single uninterrupted 24-h exposure to 50 Hz, 7 mT ELF-EMF resulted in notable elevations in plasma IL-1β, IL-6, and IL-2 levels, as well as an increase in blood parameters such as white blood cells, lymphocytes, hemoglobin, and hematocrit levels. Conversely, when rats were subjected to repetitive 1-h daily exposures to ELF-EMF over 7 days, there were no discernible alterations.
Based on the results from existing in vitro experiments, the mechanisms underlying the interaction between EMF and the immune system may involve calcium ion regulation, modulation of heat shock proteins, increased production of reactive oxygen species (ROS), cell signal transduction related to free radicals, and DNA damage and repair (Mahaki et al., 2019b). Certainly, some studies have investigated the immunomodulatory mechanisms of EMF and explored its potential therapeutic effects on certain diseases, such as wound healing (Rosado et al., 2018). However, on the whole, these mechanisms interplay, and impacts on the immune system can vary depending on factors such as exposure duration, frequency, and intensity, as well as the specific experiment subject types involved.
On the whole, in assessing immune organ function, in vivo experiments suggest that low-dose EMF exposure may be beneficial to human immune function, while high-dose exposure might have a weakening effect. The effects of EMF on immune cells and possible mechanisms depend on cell types and factors such as the intensity and duration of EMF exposure. The serum level of cytokines exhibited a possible anti-inflammatory effect to promote wound healing, but the impact depended on EMF parameters and might adapt to the changes with a longer exposure duration.
The effects of ELF-EMFs on reproduction can be quite concerning. In daily life, people pay special attention to the effect of electromagnetic radiation on fetal development. However, the adverse effects from epidemiological studies exhibited controversial results regarding both female and male reproductive functions as shown in Table 2. For instance, some studies showed no association between maternal or paternal exposure to ELF-EMFs and harmful reproductive outcomes either at 50 or 60 Hz (Sang-Kon et al., 2016). On the other hand, some cohort studies and case–control studies declared that women exposed to ELF-EMF during pregnancy might have a high risk of miscarriage (Shamsi Mahmoudabadi et al., 2013; Wang et al., 2013).
In vivo experiments offer advantages over epidemiological studies in assessing the effects of EMF on the reproductive system due to controlled exposure and direct mechanistic insights (Karimi et al., 2020). Furthermore, in vivo experiments provide a method for exploring the effects of environmental factors on different compositions of the reproduction system in different periods of pregnancy (Asghari et al., 2016). Considering the weight effects of pregnant rats exposed to electromagnetic radiation, Cao et al. (2006) reported that the weight increase in pregnant mice in the exposed group was significantly lower than that of the control group in the late gestation period after 21 days of exposure to the ELF-EMF of 1.2 mT at 50 Hz. In addition, the delivery rate of pregnant mice in the exposed group was significantly lower than that of the control group, with the occurrence of preterm birth, stillbirth, and teratogenesis. Field exposure to SD rats showed a significant decrease in sex hormones, including luteinizing hormone, progesterone, and estrogen, and ovarian weight, but the progesterone level was partly reversed after removing the exposure (Al-Akhras, 2008). However, Ruan et al. (2020) found through animal experiments that there was no significant difference in the mass or the number of stillbirths among pregnant rats in each group after 30 μT, 100 μT, and 500 μT magnetic field exposure. The evaluation of hormonal changes, including progesterone level and 17-beta estradiol level, also showed no difference between the exposed group and the control group (Aydin et al., 2009). The mentioned studies confirmed the change in hormones due to exposure to EMFs, which might further influence the reproduction and fertility systems of animals, but the mechanism behind this needs to be explored. For male mice, ELF-EMF exposure led to lower sperm counts but did not alter germ cell morphological characteristics (Heredia-Rojas et al., 2018). Earlier rat models exhibited a reduction in reproductive outcomes after exposure to EMF for a short time but recovered in 90 days (Al-Akhras et al., 2001). Furthermore, a reduction in the level of serum testosterone was detected as a result of the reduced functionality of the seminal vesicles and preputial glands (Al-Akhras et al., 2006).
According to Santini et al. (2018) review of the effect of ELF-EMF on reproductive function, in vivo experiments since the early 2000s have exhibited complex results due to different experiment environments or animal species. For the mechanism, an increasing amount of research indicates that EMF exposure could lead to the overproduction of ROS by mitochondria in both male and female mice, which might explain its effect on the reproductive system (Santini et al., 2018), but further specific research is still required to delve into this matter due to the lack of clear mechanisms.
In 2002, the International Agency for Research on Cancer (IARC) classified ELF-EMF as one of the possible carcinogenic factors for humans. In the past several decades, epidemiological studies have been conducted worldwide (Wertheimer and Leeper, 1979; Pedersen et al., 2014), but no concrete conclusion has been drawn regarding the relationship between ELF-EMF and various cancers (Carpenter, 2019). Therefore, to establish the causation between ELF-EMF and tumors, in vivo experiments were conducted to support the causal findings as shown in Table 3.
In vivo experiments have shown mixed results. Galloni and Marino (2000) investigated the influence of a 50-Hz, 2 mT EMF on mammary murine adenocarcinoma-bearing mice, and no association was observed. For a 60-Hz, 2 mT EMF, the same results were observed in the incidence of both benign and malignant tumors (McLean et al., 2003). For rats, Salim et al. (2008) explored the effects of a 1 mT EMF on chemically induced colon cancer and revealed that the influence was neither carcinogenic nor cancer-promoting. The Ramazzini Institute had committed to filling the gap in long-term in vivo experiments with the use of more than 7000 SD rats, and no evidence showing the carcinogenic effects of ELF-EMF was detected (Soffritti and Giuliani, 2019). For breast cancer, Fedrowitz and Löscher (2007) exposed 344 Fischer rats to a 100 μT, 50 Hz EMF for 26 weeks and detected a significant increase in the incidence of adenocarcinomas. In conclusion, the results from in vivo tests can be controversial due to the animal species, cancer model types, and EMF parameters.
The carcinogenic mechanisms of ELF-EMF have been hypothesized to involve various factors, including the induction of DNA damage, cellular repair process impairment (Lai and Levitt, 2023), and altered hormone secretion. In acute experiments, Lai and Singh observed a dose-dependent increase in DNA strand breaks in brain cells in rats exposed to 60 Hz EMF, which could be related to carcinogenesis and cell death (Lai and Singh, 1997). However, in McNamee et al.'s (2002, 2005) experiments, adult rats, adult mice, and immature mice were exposed to 60 Hz EMFs, and no evidence supporting the hypothesis that the exposure might cause DNA damage in the cerebellum or brain was found. In long-term animal experiments, Yokus et al. (2005) measured the level of 8OHdG in DNA, a predominant form of radical-induced DNA lesions, and the results confirmed that an EMF of 0.97 mT at 50 Hz induced oxidative DNA damage. In conclusion, in vivo experiment results showed a difference in the effect of EMF on DNA damage. This could be due to the fact that the systems had diverse genetic characteristics (Santini et al., 2009), and in vitro experiments had already confirmed that the EMF effect was sensitive to cell types (Ivancsits et al., 2005). A hypothesis was put forward that the reduction of the hormone melatonin in the pineal gland might contribute to an increase in breast cancer (Loomis et al., 1994). To verify the hypothesis, Fedrowitz et al. (2002) utilized SD rats to observe the development and growth of mammary gland tumors and found that EMF increased the cell proliferation of mammary epithelium cells but did not influence melatonin levels.
Since some tumor cells go into apoptosis in response to ELF-EMFs (Yuan et al., 2020; Sołek et al., 2022), EMF has also been used for therapeutic purposes. This therapeutic effect on various cancers has long been demonstrated through in vivo experiments (De Seze et al., 2000; Tofani et al., 2002; Novikov et al., 2009; Mansourian et al., 2020; Chen et al., 2022). Several in vitro studies showed cell-type inhibition selectivity of EMF toward specific tumor cells other than normal cells (Koh et al., 2008; Crocetti et al., 2013), but the potential mechanism was still unknown, which necessitated further investigations to uncover the intricate workings at play. Recently, in a study by Barati et al. (2021), BALB/c mice with MC-4L2 tumors were exposed to a 10-Hz 100-mT ELF-EMF for 2 h daily over a period of 28 days. The research revealed a significant augmentation of proinflammatory reactions alongside the inhibition of tumor growth, and further verified through in vitro experiments that the phenomenon was partly caused by ROS accumulation and Ca2+ overload-induced necroptosis (Barati et al., 2021). In addition to the in vivo test, an in vitro experiment further proved that the inhibition mechanism was closely linked with the contact between cells and changes in membrane potential (Sun et al., 2023).
The cardiovascular system is directly related to heartbeat and bioelectrical activities, which could potentially be affected by surrounding magnetic fields through inducing electric currents in biological body under the electromagnetic effects. A survey based on power company workers showed that there was a positive correlation between the duration of occupational electromagnetic field exposure and the incidence of arrhythmias and acute myocardial infarction (Savitz et al., 1999). On the whole, current epidemiological study findings identified limited evident cardiovascular risks associated with either short-term or prolonged exposures to ELF-EMFs within exposure limits set by current standards (Jauchem, 1997; McNamee et al., 2009).
Certain metrics, such as heart rate variability, heart rate, and blood pressure, are employed for the assessment and prediction of specific cardiovascular conditions. Since heartbeat and blood pressure are two key factors leading to cardiovascular disease, the effects of ELF-EMFs on heartbeat and blood pressure were mainly included in this review as summarized in Table 4. In short-term exposure, the results indicated that ELF-EMF might influence ventricular repolarization, leading to a suppression in heart rate elevation (Jeong et al., 2005). Other effects, including the reduction of glutathione content in the heart, were also detected in a 2-h exposure (Martínez-Sámano et al., 2010). For rat heart activity, no effect was observed under the exposure of a 50-Hz, 1-μT EMF (Elmas et al., 2012). To explore the mechanisms, Wei et al. (2015) exerted a 2 mT, 15 Hz, 50 Hz, 75 Hz, and 100 Hz EMF on cardiomyocytes isolated from neonatal SD rats and confirmed the modulation of calcium-related activities, including the increase in calcium concentration baseline level and the decrease in the amplitude of calcium transients in the sarcoplasmic reticulum. However, long-term experiments of 24 weeks on rats indicated that 50 Hz EMF had no obvious effects on the cardiovascular system through the detection of blood pressure, pulse rate, heart rate, and cardiac rhythm (Zhou et al., 2016) or based on the results of echocardiography, cardiac catheterization detection, HE staining of cardiomyocytes, and mRNA levels of related genes (Liu et al., 2018; Zhang et al., 2020).
In recent years, EMF therapy has gained significant interest due to its potential protective impact on cardiovascular functions, and various in vivo experiments on humans have been conducted. For instance, a pilot randomized controlled trial on healthy adults showed that a magnetic field of 50 Hz had therapeutic effects to stimulate parasympathetic activity, resulting in enhanced vasodilation and blood flow through a nitric oxide-dependent mechanism (Okano et al., 2021). A double-blind, repeated study explored the effect of ELF-EMFs on heart rate (HR) and HR variability and found a reduction in HR caused by the possible enhancement of parasympathetic predominance by short-term EMF (Binboga et al., 2021). Another study exposed mild-to-moderate hypertension patients to 1 μT ELF-EMF and observed a blood pressure-lowering effect (Nishimura et al., 2011). The phenomenon of EMF-related vasodilation and microcirculation was also detected clinically (Kondo et al., 2019).
Apart from the observed phenomenon, the mechanism behind was further studied. The industry generally believed that despite the lack of a complete understanding of the precise mechanisms, the main mechanisms of action involved the modulation of the autonomic nervous system (ANS), promotion of angiogenesis, enhancement of nitric oxide (NO) synthesis, and exhibition of antioxidant and anti-inflammatory properties (Soltani et al., 2023). Based on that, EMF-related medical devices have been subsequently developed to treat cardiovascular diseases such as hypertension and heart failure non-invasively. Undoubtedly, the emergence of these therapeutic devices has bridged gaps in conventional treatments, yet the substantial benefits of such therapeutic approaches and the specific mechanisms of these modulations still require validation through both in vivo and in vitro experiments.
Many diseases are concerned with the malfunction of the biological neural system, and thus the association between ELF-EMFs and neural function has attracted some interest. In vitro experiments exhibited the potential for cellular behavior modulation by EMF. For instance, immature cerebellar granule neurons (CGNs) of postnatal rats were saved from apoptosis by exposure to the ELF-EMF of 300 mT at 50 Hz, compared with no survival of CGNs without exposure (Oda and Koike, 2004). Ma et al. (2016) found that the proliferation of embryonic neural stem cells (eNSCs) was enhanced under the exposure of 1 mT, 50 Hz ELF-EMF. Furthermore, EMF has the potential to facilitate the differentiation of neural lineages and enhance the maturation of neurons (Ho et al., 2019).
In addition to the cellular-level effects, in vivo experiments were conducted to explore the system-level impacts as summarized in Table 5. Dong et al. (2023) described that it is insufficient to directly activate neuronal action potentials by exposing them to ELF-EMF on the order of milliTesla. However, the ELF-EMFs were able to regulate neural excitability contributing to learning and memory by modulating synaptic plasticity. The effect of ELF-EMF on the spatial memory of mice and rats was complex: Under a short period of exposure, the effect could be ineffective or positive, while EMF could have devastating effects with an increase in frequency, intensity, and exposure duration (Abkhezr et al., 2023). An enhancement of hippocampal neurogenesis in adult mice was also detected after exposure to EMF (Ma et al., 2016). The study conducted by Zheng et al. (2021) explored the impact of EMF stimulation patterns on long-term potentiation (LTP) related to learning and memory at synapses, revealing a consistent decrease in LTP across various waveforms that could be possibly induced by the closure of some calcium channels in the membrane. Rezaei-Tavirani et al. (2018) adopted rat models for the analysis of the proteome. The results indicated that cytoskeletal protein expression increased with the increase in EMF intensity and exposure time, which was possibly induced by the impairment in the short memory of the hippocampus under exposure.
Under conditions of cerebral ischemia, EMF has been found to promote neural regeneration in the hippocampus to possibly repair brain tissue through animal behavior experiments, which could be induced by the modulation of the Notch signaling pathway (Gao et al., 2021). For stroke, Cichoń et al. (2017) denoted that oxidative stress can be modulated by the ELF-EMF, which significantly advanced the functional and mental status of patients with a field of 7 mT at 40 Hz. In a follow-up study on post-stroke patients, they found that exposure to ELF-EMF led to a significant elevation in the levels of growth factors and cytokines associated with neuroplasticity and facilitated a notable improvement in functional recovery (Cichoń et al., 2018). In animal models of transient stroke, the results indicated that exposure to ELF-EMF enhanced the neurological assessment and behavioral outcomes, exhibited a positive impact on neuronal viability, and contributed to a reduction in glial reactivity within the hippocampus, which confirmed the potential of EMF as a clinical therapy (Moya-Gómez et al., 2023).
EMFs have demonstrated promising applications in the regulation of neural activities so as to provide a new means for the treatment of nervous system diseases. Compared with traditional therapeutic methods of electrical stimulation, EMFs in this field have advantages such as indirect contact with tissues to reduce potential damage, induction of focal neural activity, and achievement of selective activation of certain neuron populations. For instance, transcranial magnetic stimulation (TMS) is widely applied in treating neurological disorders, notably depression and anxiety (Fregni and Pascual-Leone, 2007; Klomjai et al., 2015; Cappon et al., 2022). Within intricate systems such as the retina and visual cortex, EMFs were able to offer a less-invasive approach to modulating neuronal behavior. Bonmassar et al.'s (2012) and Lee and Fried's (2015) studies introduced sub-millimeter magnetic coils to activate or suppress neuronal tissue through in vitro experiments, which offered an effective alternative for deeper and sub-cortical targets. Lee and Fried (2017) further utilized the micromagnetic stimulation on layer V pyramidal neurons (PNs) and showed that the coils produced asymmetric fields and activated the corresponding focal region, which was promising for selective stimulation of local neurons. Park et al. (2013) applied this technology in the central nervous systems of hamsters and activated inferior colliculus neurons in the dorsal cochlear nucleus. In vagus nerve stimulation (VNS), Jeong et al. (2022) adopted micro-magnetic stimulation of the rat vagus nerve and found this way of stimulation to be an effective alternative to VNS with fewer heart-related side effects.
Regarding the underlying mechanisms of these effects, the activities of neuronal ion channels were crucial and basic. According to Bertagna et al. (2021), the most frequently observed outcome of EMF exposure appears to be alterations in calcium balance, primarily associated with voltage-gated calcium channels. Under both acute and chronic exposure to ELF-EMF, an elevation of calcium ion concentration was observed intracellularly, which should be the foundation for neural activity modulation. A more intricate network of causal elements could range from interactions at the cell membrane that initiate signaling pathways to the involvement of various mechanisms such as ROS, nitric oxide, growth factors, cryptochromes, and processes related to epigenetic and genetic alterations (Funk and Fähnle, 2021; Dong et al., 2023). This comprehensive interplay within the neural environment underlines the multifaceted nature of the mechanisms that underlie the effects of EMF on the nervous system.
Currently, human lives are surrounded by various kinds of electromagnetic fields from power transmission lines, home appliances, transformers, and mobile phones. People are paying much attention to the potential health problems associated with ELF-EMFs. Most of these studies are cohort studies, and there may be confounding factors such as age, sex, magnetic induction, and frequency. The ICNIRP also denotes that there are no fixed thresholds for possible adverse biological effects due to inadequate consistent scientific evidence. Consequently, by reviewing the existing literature about the effects of ELF-EMFs on systems, this study aimed to present an overview of biological effects related to ELF-EMFs from an in vivo perspective.
It is well apprehensible that electromagnetic field intensity plays a significant role in humans on a system level. In terms of the immune system, ELF-EMFs at 1 mT and 4.5 mT increased thymus, spleen, and macrophage phagocytosis indexes, contributing to biological immune function. The decrease of these indexes happened at a higher intensity of 9 mT, which indicated potential damage to immune function. In terms of the influence on fertility, it was found that an electromagnetic field strength of 1.2 mT decreased the delivery rate of pregnant rats and also led to preterm birth, stillbirth, and teratogenesis. No connection has been detected in mammals after exposure to ELF-EMFs. Studies of their offspring also exposed to EMFs have shown that exposure to extremely low-frequency magnetic fields had no harmful effect on their growth or reproduction. On the one hand, EMF intensity lower than 500 μT showed no obvious influence on delivery rate or mass. On the other hand, ELF-EMFs such as 30 μT, 100 μT, and 500 μT also showed no effect on the heart rhythm, cardiac histology, or blood pressure of SD rats. In the nervous system, EMF had positive effects on some neural cells, such as CGNs and eNSCs. The intensity of milliTesla was not able to activate single neurons but was able to regulate some neural activities. Based on this, studies have been carried out to explore the positive and regulable effects of EMF on hippocampus-related synaptic and neural plasticity and on post-stroke rehabilitation. TMS and millimeter magnetic coils were also adopted to treat nervous diseases and modulate the retina and visual cortex.
In vivo experiments, investigating the effects of EMF exposure on cancer in animals have produced mixed results, with varying outcomes reported depending on factors such as the EMF parameters, animal species, and cancer models used. Some studies observed no significant association between EMF exposure and cancer incidence (e.g., mammary murine adenocarcinoma in mice and colon cancer in rats), while others detected increased incidence of specific tumors, indicating the complex and context-dependent nature of EMF–cancer interactions (e.g., adenocarcinoma in rats). The potential carcinogenic mechanisms of ELF-EMF involve factors such as DNA damage, impaired cellular repair processes, altered hormone secretion, and oxidative stress. Discrepancies in in vivo outcomes about DNA damage could be attributed to genetic variations and cell sensitivity. The hypothesis of EMF's impact on melatonin reduction in the pineal gland was not consistently supported by experimental results. Since ELF-EMFs have demonstrated a potential for inducing apoptosis in tumor cells, EMFs were studied for therapeutic uses. In vivo research on mice highlighted a significant increase in proinflammatory responses and inhibition of tumor growth, which was attributed to ROS accumulation and Ca2+ overload-induced necroptosis.
From the review of the literature, ELF-EMFs on the order of mT could probably affect the organism cellularly or systematically. Sometimes, the influence is helpful and therapeutic. Accumulating evidence demonstrates that ELF-EMFs are capable of modifying neuronal function with magnetic fields on the order of mT. For low-dose magnetic field exposure, such as those weaker than 500 μT, especially 100 μT or 200 μT, there are no obvious effects on biological tissue, whether in vitro or in vivo. Gutiérrez-Mercado et al. (2013) noted that biological systems exposed to EMFs of 0.66 mT can produce health effects such as tissue recovery in wounds, blood circulation re-establishment after tissue ischemia, and increasing vascular permeability of circumventricular organs in the brain. Since the ELF-EMFs surrounding our occupational and living environment are only several μT or <0.1 μT (McCurdy et al., 2001), these findings further give us confidence that biological health would not be harmed by ELF-EMFs in the surroundings from power transmission lines, electrical appliances, base stations, mobile phones, etc. with low-dose radiation exposure.
In addition, this review further sheds light on the application of ELF-EMFs to modulate or treat health problems clinically. High-frequency EMF primarily interacts with biological tissues through thermal effects, where energy is absorbed and converted into heat. This can lead to tissue heating and potential thermal damage, which is also the basis for applications such as medical diathermy and microwave ablation. ELF-EMF's interactions with biological tissues are more complex and are often attributed to non-thermal mechanisms. These include the induction of electric currents in tissues due to their conductive nature, which can affect cellular processes or recruit excitable tissues. After conducting literature research, it becomes evident that EMFs have a significant impact on neural functions such as neuronal electrical signaling. Additionally, there is a promising avenue for further exploration into the applications of electromagnetic fields in neural modulation.
In conclusion, ELF-EMF's effects are diverse and have been proposed to influence various physiological and biochemical processes, such as ion channel activities, DNA damage, hormone secretion, and oxidative stress reactions. In order to fully harness the therapeutic potential of EMF, it is essential to conduct comprehensive research into the underlying mechanisms of its effects on biological tissues so as to lay the foundation for precise modulation and control of EMF therapies, enabling their optimal utilization.
To sum up, the ELF-EMFs of different frequencies and intensities will have various effects on biological activities, either microscopically or macroscopically. Due to inconsistent model establishment and instability in the frequency and exposure time of ELF-EMFs, the research on the health effects of ELF-EMFs cannot come to a consistent conclusion. However, under weak magnetic fields, most of the research supported the fact that there are no obvious adverse effects of ELF-EMFs on biological organisms. In particular, more and more interest is being paid to adopt ELF-EMFs for disease treatment. Consequently, within the daily-life environment, ELF-EMFs would not affect human health, and they can be further applied to treat specific diseases under some medium magnetic fields.
HT contributed to design, drafting, and revision of this paper. HZ actively contributed to the original drafting of this paper. CG, MS, DY, and MJ contributed to figure drawing and manuscript revision. FW and XS contributed to design and revision of this paper. All authors contributed to the article and approved the submitted version.
This study was funded by the Science and Technology project of the State Grid Shanghai Municipal Electric Power Company (52094022000U).
The authors would like to thank Mr. Yunfeng Fan for helping in drawing Figure 1 and they also thank Master Xintong Wang for improving their English writing styles.
HT and MJ were employed by State Grid Shanghai Municipal Electric Power Company.
The remaining authors declare that the research was conducted in the absence of any commercial or financial relationships that could be construed as a potential conflict of interest.
All claims expressed in this article are solely those of the authors and do not necessarily represent those of their affiliated organizations, or those of the publisher, the editors and the reviewers. Any product that may be evaluated in this article, or claim that may be made by its manufacturer, is not guaranteed or endorsed by the publisher.
Abkhezr, H., Mohaddes, G., Nikniaz, Z., Abbasalizad Farhangi, M., Heydari, H., Nikniaz, L., et al. (2023). The effect of extremely low frequency electromagnetic field on spatial memory of mice and rats: a systematic review. Learn. Motiv. 81, 101873. doi: 10.1016/j.lmot.2023.101873
Al-Akhras, M'd.-A. (2008). Influence of 50 Hz magnetic field on sex hormones and body, uterine, and ovarian weights of adult female rats. Electromagn. Biol. Med. 27, 155–163. doi: 10.1080/15368370802072125
Al-Akhras, M'd.-A., Darmani, H., and Elbetieha, A. (2006). Influence of 50 Hz magnetic field on sex hormones and other fertility parameters of adult male rats. Bioelectromagnetics 27, 127–131. doi: 10.1002/bem.20186
Al-Akhras, M'd.-A., Elbetieha, A., Hasan, M.-K., Al-Omari, I., Darmani, H., and Albiss, B. (2001). Effects of extremely low frequency magnetic field on fertility of adult male and female rats. Bioelectromagnetics 22, 340–344. doi: 10.1002/bem.59
Asghari, A., Khaki, A. A., Rajabzadeh, A., and Khaki, A. (2016). A review on electromagnetic fields (EMFs) and the reproductive system. Electr. Phys. 8, 2655–2662. doi: 10.19082/2655
Aydin, M., Cevik, A., Kandemir, F. M., Yuksel, M., and Apaydin, A. M. (2009). Evaluation of hormonal change, biochemical parameters, and histopathological status of uterus in rats exposed to 50-Hz electromagnetic field. Toxicol. Ind. Health 25, 153–158. doi: 10.1177/0748233709102717
Barati, M., Javidi, M. A., Darvishi, B., Shariatpanahi, S. P., Mesbah Moosavi, Z. S., Ghadirian, R., et al. (2021). Necroptosis triggered by ROS accumulation and Ca(2+) overload, partly explains the inflammatory responses and anti-cancer effects associated with 1Hz, 100 mT ELF-MF in vivo. Free Radic. Biol. Med. 169, 84–98. doi: 10.1016/j.freeradbiomed.2021.04.002
Bertagna, F., Lewis, R., Silva, S. R. P., Mcfadden, J., and Jeevaratnam, K. (2021). Effects of electromagnetic fields on neuronal ion channels: a systematic review. Annal. Acad. Sci. 1499, 82–103. doi: 10.1111/nyas.14597
Binboga, E., Tok, S., and Munzuroglu, M. (2021). The short-term effect of occupational levels of 50 Hz electromagnetic field on human heart rate variability. Bioelectromagnetics 42, 60–75. doi: 10.1002/bem.22308
Bodamyali, T., Bhatt, B., Hughes, F. J., Winrow, V. R., Kanczler, J. M., Simon, B., et al. (1998). Pulsed electromagnetic fields simultaneously induce osteogenesis and upregulate transcription of bone morphogenetic proteins 2 and 4 in rat osteoblasts in vitro. Biochem. Biophys. Res. Commun. 250, 458–461. doi: 10.1006/bbrc.1998.9243
Bonmassar, G., Lee, S. W., Freeman, D. K., Polasek, M., Fried, S. I., Gale, J. T., et al. (2012). Microscopic magnetic stimulation of neural tissue. Nat. Commun. 3, 921. doi: 10.1038/ncomms1914
Boscolo, P., Gioacchino, D., Di Giampaolo, M., Antonucci, L. A., and Di Luzio, S. (2007). Combined effects of electromagnetic fields on immune and nervous responses. Int. J. Immunopathol. Pharmacol. 20, 59–63. doi: 10.1177/03946320070200S212
Canseven, A. G., Seyhan, N., Mirshahidi, S., and Imir, T. (2006). Suppression of natural killer cell activity on Candida stellatoidea by a 50 Hz magnetic field. Electr. Biol. Med. 25, 79–85. doi: 10.1080/15368370600697453
Cao, Y., Zhang, Y., and Liu, Y. (2006). Effects of exposure to extremely low frequency electromagnetic fields on reproduction of female mice and development of offsprings. Chin. J. Ind. Hyg. Occup. Dis. 24, 468–470. Available online at: https://kns.cnki.net/kcms2/article/abstract?v=KiAZ1SjiA-7g6mAEB541HcCdmMFn0IxeyAyNGrVUS-sXin1JfMx7VbYgMoM3n29gAH6vqQ2QgJdr-q0BjLDFGZyMvnkynP2JZGPrXDeoV-M29zjigVW3Qxc7YuAjVWcqO6LIroN4phMzhVFcz42hYT5MY0WcZ2jg&uniplatform=NZKPT&language=CHS
Cappon, D., De Boer, N., Jordan, T., Yu, C., Metzger, W. E., and Pascual-Leone, A. (2022). Transcranial magnetic stimulation (TMS) for geriatric depression. Ageing Res. Rev. 74, 101531. doi: 10.1016/j.arr.2021.101531
Carlberg, M., Koppel, T., Ahonen, M., and Hardell, L. (2017). Case-control study on occupational exposure to extremely low-frequency electromagnetic fields and glioma risk. Am. J. Ind. Med. 60, 494–503. doi: 10.1002/ajim.22707
Carnecka, L., Bajtos, M., Judakova, Z., Radil, R., and Janousek, L. (2022). Proliferative activity of eukaryotic cells affected by extremely low-frequency electromagnetic field. ELEKTRO 2022, 1–5. doi: 10.1109/ELEKTRO53996.2022.9803049
Carpenter, D. O. (2019). Extremely low frequency electromagnetic fields and cancer: how source of funding affects results. Environ. Res. 178, 108688. doi: 10.1016/j.envres.2019.108688
Chen, M. Y., Li, J., Zhang, N., Waldorff, E. I., Ryaby, J. T., Fedor, P., et al. (2022). In vitro and in vivo study of the effect of osteogenic pulsed electromagnetic fields on breast and lung cancer cells. Technol. Cancer Res. Treat 21, 15330338221124658. doi: 10.1177/15330338221124658
Cho, H., Seo, Y. K., Yoon, H. H., Kim, S. C., Kim, S. M., Song, K. Y., et al. (2012). Neural stimulation on human bone marrow-derived mesenchymal stem cells by extremely low frequency electromagnetic fields. Biotechnol. Prog. 28, 1329–1335. doi: 10.1002/btpr.1607
Cichoń, N., Bijak, M., Czarny, P., Miller, E., Synowiec, E., Sliwinski, T., et al. (2018). Increase in blood levels of growth factors involved in the neuroplasticity process by using an extremely low frequency electromagnetic field in post-stroke patients. Front. Aging Neurosci. 10, 294. doi: 10.3389/fnagi.2018.00294
Cichoń, N., Bijak, M., Miller, E., and Saluk, J. (2017). Extremely low frequency electromagnetic field (ELF-EMF) reduces oxidative stress and improves functional and psychological status in ischemic stroke patients. Bioelectromagnetics 38, 386–396. doi: 10.1002/bem.22055
Consales, C., Cirotti, C., Filomeni, G., Panatta, M., Butera, A., Merla, C., et al. (2018). Fifty-hertz magnetic field affects the epigenetic modulation of the miR-34b/c in neuronal cells. Mol. Neurobiol. 55, 5698–5714. doi: 10.1007/s12035-017-0791-0
Cricenti, A., Generosi, R., Luce, M., Perfetti, P., Sanghera, J. S., Aggarwal, I. D., et al. (2008). Low-frequency electromagnetic field effects on functional groups in human skin keratinocytes cells revealed by IR-SNOM. J. Microsc. 229, 551–554. doi: 10.1111/j.1365-2818.2008.01942.x
Crocetti, S., Beyer, C., Schade, G., Egli, M., Fröhlich, J., Franco-Obregón, A., et al. (2013). Low intensity and frequency pulsed electromagnetic fields selectively impair breast cancer cell viability. PLoS ONE 8, e72944. doi: 10.1371/journal.pone.0072944
de Kleijn, S., Ferwerda, G., Wiese, M., Trentelman, J., Cuppen, J., Kozicz, T., et al. (2016). A short-term extremely low frequency electromagnetic field exposure increases circulating leukocyte numbers and affects HPA-axis signaling in mice. Bioelectromagnetics 37, 433–443. doi: 10.1002/bem.21998
De Seze, D., Tuffet, R., Moreau, S. J.-M., and Veyret, B. (2000). Effects of 100 mT time varying magnetic fields on the growth of tumors in mice. Bioelectromagnetics 21, 107–111. doi: 10.1002/(SICI)1521-186X(200002)21:2<107::AID-BEM5>3.0.CO;2-6
Dong, L., Xia, P., Tian, L., Tian, C., Zhao, W., Zhao, L., et al. (2023). A review of aspects of synaptic plasticity in hippocampus via mT extremely low-frequency magnetic fields. Bioelectromagnetics. 44, 63–70. doi: 10.1002/bem.22437
Du, L., Fan, H., Miao, H., Zhao, G., and Hou, Y. (2014). Extremely low frequency magnetic fields inhibit adipogenesis of human mesenchymal stem cells. Bioelectromagnetics 35, 519–530. doi: 10.1002/bem.21873
Ebrahimi, M. R., Seraj, M., Majidzadeh, A. K., Khoshdel, A., and Shahmiri, H. (2019). The effects of extremely low-frequency electromagnetic field on depression, anxiety, thyroid hormones, and gene expression in mice. Activitas Nervosa Superior Red. 61, 2019.
Elmas, O., Comlekci, S., and Koylu, H. (2012). Effects of short-term exposure to powerline-frequency electromagnetic field on the electrical activity of the heart. Arch. Environ. Occup. Health 67, 65–71. doi: 10.1080/19338244.2011.578680
Fathi, E., and Farahzadi, R. (2018). Zinc sulphate mediates the stimulation of cell proliferation of rat adipose tissue-derived mesenchymal stem cells under high intensity of EMF exposure. Biol. Trace Elem. Res. 184, 529–535. doi: 10.1007/s12011-017-1199-4
Fedrowitz, M., and Löscher, W. (2007). Exposure of Fischer 344 rats to a weak power frequency magnetic field facilitates mammary tumorigenesis in the DMBA model of breast cancer. Carcinogenesis 29, 186–193. doi: 10.1093/carcin/bgm217
Fedrowitz, M., Westermann, J. R., and LöScher, W. (2002). Magnetic field exposure increases cell proliferation but does not affect melatonin levels in the mammary gland of female sprague dawley rats1. Cancer Res. 62, 1356–1363.
Fregni, F., and Pascual-Leone, A. (2007). Technology insight: noninvasive brain stimulation in neurology—perspectives on the therapeutic potential of rTMS and tDCS. Nat. Clin. Prac. Neurology 3, 383–393. doi: 10.1038/ncpneuro0530
Funk, R. H. W., and Fähnle, M. (2021). A short review on the influence of magnetic fields on neurological diseases. FBS 13, 181–189. doi: 10.52586/S561
Galloni, P., and Marino, C. (2000). Effects of 50 Hz magnetic field exposure on tumor experimental models. Bioelectromagnetics 21, 608–614. doi: 10.1002/1521-186X(200012)21:8<608::AID-BEM7>3.0.CO;2-Z
Gao, Q., Leung, A., Yang, Y. H., Lau, B. W., Wang, Q., Liao, L. Y., et al. (2021). Extremely low frequency electromagnetic fields promote cognitive function and hippocampal neurogenesis of rats with cerebral ischemia. Neural. Regen. Res. 16, 1252–1257. doi: 10.4103/1673-5374.301020
Griefahn, B., Künemund, C., Blaszkewicz, M., Golka, K., Mehnert, P., Degen, G., et al. (2001). Experiments on the effects of a continuous 16.7 Hz magnetic field on melatonin secretion, core body temperature, and heart rates in humans. J. Bioelectromag. Soc. Soc. Phys. Reg. Biol. Med. 22, 581–588. doi: 10.1002/bem.87
Gutiérrez-Mercado, Y. K., Cañedo-Dorantes, L., Gómez-Pinedo, U., Serrano-Luna, G., Bañuelos-Pineda, J., and Feria-Velasco, A. (2013). Increased vascular permeability in the circumventricular organs of adult rat brain due to stimulation by extremely low frequency magnetic fields. Bioelectromagnetics 34, 145–155. doi: 10.1002/bem.21757
Heredia-Rojas, J. A., Rodríguez-De La Fuente, A. O., Gomez-Flores, R., Heredia-Rodríguez, O., Rodríguez-Flores, L. E., Beltcheva, M., et al. (2018). In vivo Cytotoxicity induced by 60 Hz electromagnetic fields under a high-voltage substation environment. Sustainability 10, 2789. doi: 10.3390/su10082789
Ho, S.-Y., Chen, I. C., Chen, Y.-J., Lee, C.-H., Fu, C.-M., Liu, F. C., et al. (2019). Static magnetic field induced neural stem/progenitor cell early differentiation and promotes maturation. Stem Cells Int. 2019, 8790176. doi: 10.1155/2019/8790176
Hu, T., Jia, G. L., Wang, H.-C., Guo, W. Y., Cao, Y. J., Wang, X. Y., et al. (2006). Effects of low frequency electromagnetic field on the expression of osteopontin gene of the aortic vascular smooth muscle cells in rats. Chin. J. Phys. Med. Rehab. 28, 91–94. Available online at: https://kns.cnki.net/kcms2/article/abstract?v=KiAZ1SjiA-4IfZjRSfDhwsaTDi_OtlnAXA6BeTZc5iFsNma34al2MN1OcKQsWhcvvXjjgFTm8yZPPH_kzrevlJ0hc1p0HVXVBQjSENyaGYa_KgVnM2DExFxsORr2Q07OTWZ7E-vdgnt_4noOP-no27AwV8TNACka&uniplatform=NZKPT&language=CHS
Huo, R., Ma, Q., Wu, J. J., Chin-Nuke, K., Jing, Y., Chen, J., et al. (2010). Noninvasive electromagnetic fields on keratinocyte growth and migration. J. Surg. Res. 162, 299–307. doi: 10.1016/j.jss.2009.02.016
ICNIRP (2010). Guidelines for limiting exposure to time-varying electric and magnetic fields (1 Hz to 100 kHz). Health Phys. 99, 818–836. doi: 10.1097/HP.0b013e3181f06c86
Ivancsits, S., Pilger, A., Diem, E., Jahn, O., and Rüdiger, H. W. (2005). Cell type-specific genotoxic effects of intermittent extremely low-frequency electromagnetic fields. Mutat. Res. 583, 184–188. doi: 10.1016/j.mrgentox.2005.03.011
Jalilian, H., Teshnizi, S. H., Röösli, M., and Neghab, M. (2018). Occupational exposure to extremely low frequency magnetic fields and risk of Alzheimer disease: a systematic review and meta-analysis. Neurotoxicology 69, 242–252. doi: 10.1016/j.neuro.2017.12.005
Jauchem, J. R. (1997). Exposure to extremely-low-frequency electromagnetic fields and radiofrequency radiation: cardiovascular effects in humans. Int. Arch. Occup. Environ. Health 70, 9–21. doi: 10.1007/s004200050181
Jeong, H., Cho, A., Ay, I., and Bonmassar, G. (2022). Short-pulsed micro-magnetic stimulation of the vagus nerve. Front. Physiol. 13, 938101. doi: 10.3389/fphys.2022.938101
Jeong, J., Kim, J., Lee, B., Min, Y., Kim, D., Ryu, J., et al. (2005). Influence of exposure to electromagnetic field on the cardiovascular system. Autonomic Autacoid Pharmacol. 25, 17–23. doi: 10.1111/j.1474-8673.2004.00328.x
Karimi, A., Ghadiri Moghaddam, F., and Valipour, M. (2020). Insights in the biology of extremely low-frequency magnetic fields exposure on human health. Mol. Biol. Rep. 47, 5621–5633. doi: 10.1007/s11033-020-05563-8
Klomjai, W., Katz, R., and Lackmy-Vallée, A. (2015). Basic principles of transcranial magnetic stimulation (TMS) and repetitive TMS (rTMS). Ann. Phys. Rehabil. Med. 58, 208–213. doi: 10.1016/j.rehab.2015.05.005
Koh, E. K., Ryu, B. K., Jeong, D. Y., Bang, I. S., Nam, M. H., Chae, K. S., et al. (2008). A 60-Hz sinusoidal magnetic field induces apoptosis of prostate cancer cells through reactive oxygen species. Int. J. Rad. Biol. 84, 945–955. doi: 10.1080/09553000802460206
Kondo, T., Okano, H., Ishiwatari, H., and Watanuki, K. (2019). “Evaluation of the Hemodynamic Effects of AC Magnetic Field Exposure by Measurement of an FMD and a Microscope,” in, Advances in Human Factors and Ergonomics in Healthcare and Medical Devices, ed N. J. Lightner (Cham: Springer International Publishing), 68-79.
Kula, B., Sobczak, A., and Kuśka, R. (2002). A study of the effects of static and extremely low frequency magnetic fields on lipid peroxidation products in subcellular fibroblast fractions. Electromag. Biol. Med. 21, 161–168. doi: 10.1081/JBC-120006789
Lai, H., and Levitt, B. B. (2023). Cellular and molecular effects of non-ionizing electromagnetic fields. Rev. Environ. Health. doi: 10.1515/reveh-2023-0023 Available online at: degruyter.com
Lai, H., and Singh, N. P. (1997). Acute exposure to a 60 Hz magnetic field increases DNA strand breaks in rat brain cells. Bioelectromagnetics 18, 156–165. doi: 10.1002/(SICI)1521-186X(1997)18:2<156::AID-BEM8>3.0.CO;2-1
Lee, C. Y., Tang, J. Y., Chen, P. J., Jang, L. S., and Chuang, H. L. (2020). “The effect of extremely low frequency electromagnetic field on weight gain of preterm babies,” in 2020 IEEE International Conference on Consumer Electronics-Taiwan (ICCE-Taiwan), 2020. Taiwan: IEEE, 1–2.
Lee, S. W., and Fried, S. I. (2015). Suppression of subthalamic nucleus activity by micromagnetic stimulation. IEEE Trans. Neural. Syst. Rehabil. Eng. 23, 116–127. doi: 10.1109/TNSRE.2014.2348415
Lee, S. W., and Fried, S. I. (2017). Enhanced control of cortical pyramidal neurons with micromagnetic stimulation. IEEE Trans. Neural. Syst. Rehabil. Eng. 25, 1375–1386. doi: 10.1109/TNSRE.2016.2631446
Li, S., Jiang, H., Wang, B., Gu, M., Bi, X., Yin, Y., et al. (2018). Magnetic resonance spectroscopy for evaluating the effect of pulsed electromagnetic fields on marrow adiposity in postmenopausal women with osteopenia. J. Comput. Assis. Tomography 42, 792–797. doi: 10.1097/RCT.0000000000000757
Liu, X., Tang, Y., Hai, J., He, W., Chen, C., Zhang, Y., et al. (2018). Exposure experiment research of the power frenquency magnetic field on the cardiovascular system in SD Rats. High Voltage Eng. 44, 599–609. doi: 10.13336/j.1003-6520.hve.20180131034
Loomis, D. P., Savitz, D. A., and Ananth, C. V. (1994). Breast cancer mortality among female electrical workers in the United States. JNCI 86, 921–925. doi: 10.1093/jnci/86.12.921
Lu, D., Yuan, L., Wang, C., Tan, L., and Chen, X. (2019). Inhibitory effect of extremely low frequency-electromagnetic field combined with static magnetic field on tumor cell proliferation and the possible mechanism. Tumor 39, 973–984. Available online at: https://kns.cnki.net/kcms2/article/abstract?v=KiAZ1SjiA-4-apF7DDQOExE1OmSPGnwrIZ4ojjiaqLmmStgTAIRew0nGqA4kG-bT9OFDyEyHHJf__z8dCWfH5ISiYYd4Gh-iceGEg4dbW_lDjJW0VS8-T5TeUhcq2sNSLTveUDVFWOlc1GPFZKTHZbkc8gdQ4A5s&uniplatform=NZKPT&language=CHS
Lv, H., Liu, J., Zhen, C., Wang, Y., Wei, Y., Ren, W., et al. (2021). Magnetic fields as a potential therapy for diabetic wounds based on animal experiments and clinical trials. Cell Prolif. 54, 982. doi: 10.1111/cpr.12982
Ma, Q., Chen, C., Deng, P., Zhu, G., Lin, M., Zhang, L., et al. (2016). Extremely low-frequency electromagnetic fields promote in vitro neuronal differentiation and neurite outgrowth of embryonic neural stem cells via up-regulating TRPC1. PloS One 11, e0150923. doi: 10.1371/journal.pone.0150923
Mahaki, H., Jabarivasal, N., Sardanian, K., and Zamani, A. (2020). Effects of various densities of 50 Hz electromagnetic field on serum IL-9, IL-10, and TNF-α Levels. Int. J. Occup. Environ. Med. 11, 24–32. doi: 10.15171/ijoem.2020.1572
Mahaki, H., Jabarivasal, N., Sardarian, K., and Zamani, A. (2019a). The effects of extremely low-frequency electromagnetic fields on c-Maf, STAT6, and RORα expressions in spleen and thymus of rat. Electromagnetic Biol. Med. 38, 177–183. doi: 10.1080/15368378.2019.1608832
Mahaki, H., Tanzadehpanah, H., Jabarivasal, N., Sardanian, K., and Zamani, A. (2019b). A review on the effects of extremely low frequency electromagnetic field (ELF-EMF) on cytokines of innate and adaptive immunity. Electromag. Biol. Med. 38, 84–95. doi: 10.1080/15368378.2018.1545668
Manni, V., Lisi, A., Pozzi, D., Rieti, S., Serafino, A., Giuliani, L., et al. (2002). Effects of extremely low frequency (50 Hz) magnetic field on morphological and biochemical properties of human keratinocytes. Bioelectromagnetics 23, 298–305. doi: 10.1002/bem.10023
Manni, V., Lisi, A., Rieti, S., Serafino, A., Ledda, M., Giuliani, L., et al. (2004). Low electromagnetic field (50 Hz) induces differentiation on primary human oral keratinocytes (HOK). Bioelectromagnetics 25, 118–126. doi: 10.1002/bem.10158
Mansourian, M., Firoozabadi, M., and Hassan, Z. M. (2020). The role of 217-Hz ELF magnetic fields emitted from GSM mobile phones on electrochemotherapy mechanisms. Electromag. Biol. Med. 39, 239–249. doi: 10.1080/15368378.2020.1762635
Mao, Y., Liu, H., Wu, X., and Gao, B. (2018). Effects of extremely low frequency electromagnetic fields exposure on function and morphology of liver, kidney and spleen of SD rats. J. Univ. 44, 1120–1123. doi: 10.13481/j.1671-587x.20180602
Martínez-Sámano, J., Torres-Durán, P. V., Juárez, O. R., Elías-Viñas, D., and Verdugo-Díaz, L. (2010). Effects of acute electromagnetic field exposure and movement restraint on antioxidant system in liver, heart, kidney and plasma of Wistar rats: a preliminary report. Int. J. Radiat. Biol. 86, 1088–1094. doi: 10.3109/09553002.2010.501841
McCurdy, A. L., Wijnberg, L., Loomis, D., Savitz, D., and Nylander-French, L. A. (2001). Exposure to extremely low frequency magnetic fields among working women and homemakers. Annal. Occup. Hyg. 45, 643–650. doi: 10.1016/S0003-4878(01)00034-5
McLean, J. R., Thansandote, A., Mcnamee, J. P., Tryphonas, L., Lecuyer, D., Gajda, G., et al. (2003). A 60 Hz magnetic field does not affect the incidence of squamous cell carcinomas in SENCAR mice. Bioelectromagnetics 24, 75–81. doi: 10.1002/bem.10067
McNamee, D. A., Legros, A. G., Krewski, D. R., Wisenberg, G., Prato, F. S., Thomas, A. W., et al. (2009). A literature review: the cardiovascular effects of exposure to extremely low frequency electromagnetic fields. Int. Arch. Occup. Environ. Health 82, 919–933. doi: 10.1007/s00420-009-0404-y
McNamee, J. P., Bellier, P. V., Chauhan, V., Gajda, G. B., Lemay, E., Thansandote, A., et al. (2005). Evaluating DNA damage in rodent brain after acute 60 Hz magnetic-field exposure. Radiation Res. 164, 791–797. doi: 10.1667/RR3465.1
McNamee, J. P., Bellier, P. V., Mclean, J. R. N., Marro, L., Gajda, G. B., Thansandote, A., et al. (2002). DNA damage and apoptosis in the immature mouse cerebellum after acute exposure to a 1 mT, 60 Hz magnetic field. Mutat. Res. Gen. Toxicol. Environ. Mutag. 513, 121–133. doi: 10.1016/S1383-5718(01)00302-3
Moya-Gómez, A., Font, L. P., Burlacu, A., Alpizar, Y. A., Cardonne, M. M., Brône, B., et al. (2023). Extremely low-frequency electromagnetic stimulation (ELF-EMS) improves neurological outcome and reduces microglial reactivity in a rodent model of global transient stroke. Int. J. Mol. Sci. 24, 11117. doi: 10.3390/ijms241311117
Nishimura, T., Tada, H., Guo, X., Murayama, T., Teramukai, S., Okano, H., et al. (2011). A 1-μT extremely low-frequency electromagnetic field vs. sham control for mild-to-moderate hypertension: a double-blind, randomized study. Hypertension Res. 34, 372–377. doi: 10.1038/hr.2010.246
Novikov, V. V., Novikov, G. V., and Fesenko, E. E. (2009). Effect of weak combined static and extremely low-frequency alternating magnetic fields on tumor growth in mice inoculated with the Ehrlich ascites carcinoma. Bioelectromagnetics 30, 343–351. doi: 10.1002/bem.20487
Oda, T., and Koike, T. (2004). Magnetic field exposure saves rat cerebellar granule neurons from apoptosis in vitro. Neurosci. Lett. 365, 83–86. doi: 10.1016/j.neulet.2004.04.068
Okano, H., Fujimura, A., Kondo, T., Laakso, I., Ishiwatari, H., Watanuki, K., et al. (2021). A 50 Hz magnetic field affects hemodynamics, ECG and vascular endothelial function in healthy adults: a pilot randomized controlled trial. PLoS ONE 16, e0255242. doi: 10.1371/journal.pone.0255242
Ozturk, H., Saribal, D., Gelmez, Y. M., Deniz, G., Yilmaz, A., Kirectepe, A., et al. (2022). Extremely low frequency electromagnetic fields exposure during the prenatal and postnatal periods alters pro-inflammatory cytokines levels by gender. Electromag. Biol. Med. 41, 163–173. doi: 10.1080/15368378.2022.2046045
Park, H. J., Bonmassar, G., Kaltenbach, J. A., Machado, A. G., Manzoor, N. F., Gale, J. T., et al. (2013). Activation of the central nervous system induced by micro-magnetic stimulation. Nat. Commun. 4, 2463. doi: 10.1038/ncomms3463
Pedersen, C., Raaschou-Nielsen, O., Rod, N. H., Frei, P., Poulsen, A. H., Johansen, C., et al. (2014). Distance from residence to power line and risk of childhood leukemia: a population-based case-control study in Denmark. Cancer Causes Control 25, 171–177. doi: 10.1007/s10552-013-0319-5
Quaglino, D., Capri, M., Zecca, L., Franceschi, C., and Ronchetti, I. P. (2004). The effect on rat thymocytes of the simultaneous in vivo exposure to 50-Hz electric and magnetic field and to continuous light. Sci. World J. 4, 91–99. doi: 10.1100/tsw.2004.183
Raggi, F., Vallesi, G., Rufini, S., Gizzi, S., Ercolani, E., Rossi, R., et al. (2008). ELF magnetic therapy and oxidative balance. Electromag. Biol. Med. 27, 325–339. doi: 10.1080/15368370802493271
Reyes-Guerrero, G., Guzmán, C., García, D. E., Camacho-Arroyo, I., and Vázquez-García, M. (2010). Extremely low-frequency electromagnetic fields differentially regulate estrogen receptor-α and-β expression in the rat olfactory bulb. Neurosci. Lett. 471, 109–113. doi: 10.1016/j.neulet.2010.01.021
Rezaei-Tavirani, M., Hasanzadeh, H., Seyyedi, S., Ghoujeghi, F., Semnani, V., Zali, H., et al. (2018). Proteomic analysis of extremely low-frequency electromagnetic field (ELF-EMF) with different intensities in rats hippocampus. Arch. Neurosci. 5, e62954. doi: 10.5812/archneurosci.62954
Rosado, M. M., Simko, M., Mattsson, M. O., and Pioli, C. (2018). Immune-modulating perspectives for low frequency electromagnetic fields in innate immunity. Front. Pub. Health 6, 85. doi: 10.3389/fpubh.2018.00085
Ruan, G., Liu, X., Zhang, Y., Liu, H., He, M., Lai, J., et al. (2020). Effects of extremely low frequency electromagnetic fields on reproductive system and development of mice. High Voltage Eng. 46, 287–293. doi: 10.13336/j.1003-6520.hve.20190496
Salim, E. I., Omar, K. M., Abou-Hattab, H. A., and Abou-Zaid, F. A. (2008). Pituitary toxicity but lack of rat colon carcinogenicity of a DC-magnetic field in a medium-term bioassay. Asian Pac. J. Cancer Prev 9, 131–140.
Sang-Kon, L., Sungman, P., and Yoon-Won, K. (2016). The Effects of Extremely Low-Frequency Magnetic Fields on Reproductive Function in Rodents. Insights from Animal Reproduction. Rijeka: IntechOpen.
Santini, M. T., Rainaldi, G., and Indovina, P. L. (2009). Cellular effects of extremely low frequency (ELF) electromagnetic fields. Int. J. Rad. Biol. 85, 294–313. doi: 10.1080/09553000902781097
Santini, S. J., Cordone, V., Falone, S., Mijit, M., Tatone, C., Amicarelli, F., et al. (2018). Role of mitochondria in the oxidative stress induced by electromagnetic fields: focus on reproductive systems. Oxidative Med. Cell. Longevity 2018, 5076271. doi: 10.1155/2018/5076271
Savitz, D. A., Liao, D., Sastre, A., Kleckner, R. C., and Kavet, R. (1999). Magnetic field exposure and cardiovascular disease mortality among electric utility workers. Am. J. Epidemiol. 149, 135–142. doi: 10.1093/oxfordjournals.aje.a009779
Shamsi Mahmoudabadi, F., Ziaei, S., Firoozabadi, M., and Kazemnejad, A. (2013). Exposure to extremely low frequency electromagnetic fields during pregnancy and the risk of spontaneous abortion: a case-control study. J. Res. Health Sci. 13, 131–134. doi: 10.1186/s40201-015-0193-z
Soffritti, M., and Giuliani, L. (2019). The carcinogenic potential of non-ionizing radiations: the cases of S-50 Hz MF and 1.8 GHz GSM radiofrequency radiation. Basic Clin. Pharmacol. Toxicol. 125, 58–69. doi: 10.1111/bcpt.13215
Sołek, P., Mytych, J., Łannik, E., Majchrowicz, L., Koszła, O., Koziorowska, A., et al. (2022). Cancer on-target: selective enhancement of 3-bromopyruvate action by an electromagnetic field in vitro. Free Rad. Biol. Med. 180, 153–164. doi: 10.1016/j.freeradbiomed.2022.01.011
Soltani, D., Samimi, S., Vasheghani-Farahani, A., Shariatpanahi, S. P., Abdolmaleki, P., Madjid Ansari, A., et al. (2023). Electromagnetic field therapy in cardiovascular diseases: a review of patents, clinically effective devices, and mechanism of therapeutic effects. Trends Cardiovas. Med. 33, 72–78. doi: 10.1016/j.tcm.2021.10.006
Sun, J., Tong, Y., Jia, Y., Jia, X., Wang, H., Chen, Y., et al. (2023). Effects of extremely low frequency electromagnetic fields on the tumor cell inhibition and the possible mechanism. Sci. Rep. 13, 6989. doi: 10.1038/s41598-023-34144-5
Sun, X., Duan, F., Kou, S., Liu, J., and Ma, Y. (2009). Effect of extremely low frequency electromagnetic fields on Bax and Bcl-2 expression in spermatogenic cells of rats. Chin. J. Pub. Health 25, 989–990. Available online at: https://kns.cnki.net/kcms2/article/abstract?v=KiAZ1SjiA-5MHcxXX3KEW0jzLP4vwRLA-7IimSKs6Wub9vsL_xEdjKv3_dmSn251Ibtmy72XUDGDqpFRM4HvUvThzk-g0aM1SPxqFN-1eLT8RXsnDHCWcAboHdx8I6SWBPZgpYKAb_RPSW3uMu7Rd_6aYBm2zQiY&uniplatform=NZKPT&language=CHS
Tofani, S., Cintorino, M., Barone, D., Berardelli, M., Santi, D., Ferrara, M. M., et al. (2002). Increased mouse survival, tumor growth inhibition and decreased immunoreactive p53 after exposure to magnetic fields. Bioelectromagnetics 23, 230–238. doi: 10.1002/bem.10010
Turner, M. C., Benke, G., Bowman, J. D., Figuerola, J., Fleming, S., Hours, M., et al. (2017). Interactions between occupational exposure to extremely low frequency magnetic fields and chemicals for brain tumour risk in the INTEROCC study. Occup. Environ. Med. 74, 802–809. doi: 10.1136/oemed-2016-104080
Vianale, G., Reale, M., Amerio, P., Stefanachi, M., Luzio, D., Muraro, S., et al. (2008). Extremely low frequency electromagnetic field enhances human keratinocyte cell growth and decreases proinflammatory chemokine production. Br. J. Dermatol. 158, 1189–1196. doi: 10.1111/j.1365-2133.2008.08540.x
Wang, Q., Cao, Z., Qu, Y., Peng, X., Guo, S., Chen, L., et al. (2013). Residential exposure to 50 Hz magnetic fields and the association with miscarriage risk: a 2-year prospective cohort study. PLoS ONE 8, e82113. doi: 10.1371/journal.pone.0082113
Wei, J., Sun, J., Xu, H., Shi, L., Sun, L., Zhang, J., et al. (2015). Effects of extremely low frequency electromagnetic fields on intracellular calcium transients in cardiomyocytes. Electromagn. Biol. Med. 34, 77–84. doi: 10.3109/15368378.2014.881744
Wertheimer, N., and Leeper, E. (1979). Electrical wiring configurations and childhood cancer. Am. J. Epidemiol. 109, 273–284. doi: 10.1093/oxfordjournals.aje.a112681
Wyszkowska, J., Jedrzejewski, T., Piotrowski, J., Wojciechowska, A., Stankiewicz, M., Kozak, W., et al. (2018). Evaluation of the influence of in vivo exposure to extremely low-frequency magnetic fields on the plasma levels of pro-inflammatory cytokines in rats. Int. J. Rad. Biol. 94, 909–917. doi: 10.1080/09553002.2018.1503428
Yokus, B., Cakir, D. U., Akdag, M. Z., Sert, C., and Mete, N. (2005). Oxidative DNA damage in rats exposed to extremely low frequency electro magnetic fields. Free Rad. Res. 39, 317–323. doi: 10.1080/10715760500043603
Yuan, L. Q., Wang, C., Lu, D. F., Zhao, X. D., Tan, L. H., Chen, X., et al. (2020). Induction of apoptosis and ferroptosis by a tumor suppressing magnetic field through ROS-mediated DNA damage. Aging 12, 3662–3681. doi: 10.18632/aging.102836
Zhang, H., Cheng, Y., Luo, X., and Duan, Y. (2016). Protective effect of procyanidins extracted from the lotus seedpod on immune function injury induced by extremely low frequency electromagnetic field. Biomed. Pharmacother. 82, 364–372. doi: 10.1016/j.biopha.2016.05.021
Zhang, M., Li, X., Bai, L., Uchida, K., Bai, W., Wu, B., et al. (2013). Effects of low frequency electromagnetic field on proliferation of human epidermal stem cells: an in vitro study. Bioelectromagnetics 34, 74–80. doi: 10.1002/bem.21747
Zhang, N., Wang, S., Wang, S., and Ning, S. (2018). “The design and analysis of a static and extremely low-frequency magnetic field stimulation platform for cell prolifation inhibition,” in 2018 International Applied Computational Electromagnetics Society Symposium-China (ACES). Piscataway, NJ: IEEE, 1–2.
Zhang, X., Zhang, J., Long, K., Wen, J., and Qu, X. (2006). The effect of extremely low frequency pulsed electromagnetic fields on neonatal rat osetoblasts. Chin. J. Med. Phys. 23, 462–464. Available online at: https://kns.cnki.net/kcms2/article/abstract?v=KiAZ1SjiA-5xOpDKCVpqXpY3fhEMbPW44DmPmIEPINIXOFB_t3XN2pq7cD6B1Nw9FZQZ0JeHBvYii7zV9cDnm3wKI_sg7Uxi5qSrw6NgtNDXo8PPUy3-ar5MuVRXEIrURrGMGG-miPuExsG-LT5qms0TPOK5Xa2C&uniplatform=NZKPT&language=CHS
Zhang, Y., Lai, J., Zhang, J., Zhao, J., Wan, H., Chen, C., et al. (2016). Effects of extremely low frequency electromagnetic fields on the hematopoietic system of the SD rats. High Voltage Eng. 42, 2519–2527. doi: 10.13336/j.1003-6520.hve.20160812010
Zhang, Y., Li, L., Liu, X., Ding, L., Wu, X., Wang, J., et al. (2020). Examination of the effect of a 50-Hz electromagnetic field at 500 μT on parameters related with the cardiovascular system in rats. Front. Pub. Health 8, 87. doi: 10.3389/fpubh.2020.00087
Zheng, Y., Zhao, L., Dong, L., Tian, C., Xia, P., Jin, Z., et al. (2021). The time-dependence of three different modes of ELF-EMF stimulation on LTP at schaffer collateral-CA1 synapses. Bioelectromagnetics 42, 538–549. doi: 10.1002/bem.22369
Zhou, L., Wan, B., Liu, X., Zhang, Y., Lai, J., Ruan, G., et al. (2016). The effects of a 50-Hz magnetic field on the cardiovascular system in rats. J. Radiat Res. 57, 627–636. doi: 10.1093/jrr/rrw090
Keywords: ELF-EMF, health effect, electromagnetic radiation, power transmission line, work frequency
Citation: Tian H, Zhu H, Gao C, Shi M, Yang D, Jin M, Wang F and Sui X (2023) System-level biological effects of extremely low-frequency electromagnetic fields: an in vivo experimental review. Front. Neurosci. 17:1247021. doi: 10.3389/fnins.2023.1247021
Received: 25 June 2023; Accepted: 12 September 2023;
Published: 06 October 2023.
Edited by:
Ravi L. Hadimani, Virginia Commonwealth University, United StatesReviewed by:
Tianruo Guo, University of New South Wales, AustraliaCopyright © 2023 Tian, Zhu, Gao, Shi, Yang, Jin, Wang and Sui. This is an open-access article distributed under the terms of the Creative Commons Attribution License (CC BY). The use, distribution or reproduction in other forums is permitted, provided the original author(s) and the copyright owner(s) are credited and that the original publication in this journal is cited, in accordance with accepted academic practice. No use, distribution or reproduction is permitted which does not comply with these terms.
*Correspondence: Xiaohong Sui, c3VpeGhvbmdAc2p0dS5lZHUuY24=; Fenghua Wang, Zmh3YW5nNzcyM0BzanR1LmVkdS5jbg==
Disclaimer: All claims expressed in this article are solely those of the authors and do not necessarily represent those of their affiliated organizations, or those of the publisher, the editors and the reviewers. Any product that may be evaluated in this article or claim that may be made by its manufacturer is not guaranteed or endorsed by the publisher.
Research integrity at Frontiers
Learn more about the work of our research integrity team to safeguard the quality of each article we publish.