- 1Department of Emergency Medicine, Affiliated Kunshan Hospital of Jiangsu University, Kunshan, China
- 2School of Computer Science and Technology, Harbin Institute of Technology, Harbin, China
Clusterin (CLU) is an extracellular chaperone involved in reducing amyloid beta (Aβ) toxicity and aggregation. Although previous genome-wide association studies (GWAS) have reported a potential protective effect of CLU on Alzheimer’s disease (AD) patients, how intron-located rs11136000 (CLU) affects AD risk by regulating CLU expression remains unknown. In this study, we integrated multiple omics data to construct the regulated pathway of rs11136000-CLU-AD. In step 1, we investigated the effects of variant rs11136000 on AD risk with different genders and diagnostic methods using GWAS summary statistics for AD from International Genomics of Alzheimer’s Project (IGAP) and UK Biobank. In step 2, we assessed the regulation of rs11136000 on CLU expression in AD brain samples from Mayo clinic and controls from Genotype-Tissue Expression (GTEx). In step 3, we investigated the differential gene/protein expression of CLU in AD and controls from four large cohorts. The results showed that rs11136000 T allele reduced AD risk in either clinically diagnosed or proxy AD patients. By using expression quantitative trait loci (eQTL) analysis, rs11136000 variant downregulated CLU expression in 13 normal brain tissues, but upregulated CLU expression in cerebellum and temporal cortex of AD samples. Importantly, CLU was significantly differentially expressed in temporal cortex, dorsolateral prefrontal cortex and anterior prefrontal cortex of AD patients compared with normal controls. Together, rs11136000 may reduce AD risk by regulating CLU expression, which may provide important information about the biological mechanism of rs9848497 in AD progress.
Introduction
Alzheimer’s disease (AD) is a neurodegenerative disease of the central nervous system characterized by progressive cognitive dysfunction and behavioral impairment (Scheltens et al., 2016; Van Cauwenberghe et al., 2016; Pimenova et al., 2018; Hu et al., 2021b). It is estimated that at least 40 million middle-aged and elderly people worldwide suffer from AD (Van Cauwenberghe et al., 2016. Among all the AD susceptibility genes, Apolipoprotein E (APOE), which mediates the binding, internalization, and catabolism of lipoprotein particles, is considered to be the major risk factor (Namba et al., 1991; Belloy et al., 2019). APOE not only co-deposits with beta-amyloid (Aβ) through protein-protein interaction, but also directly leads to secretion and impaired clearance of Aβ (Namba et al., 1991; Huynh et al., 2017; Belloy et al., 2019).
Other susceptibility genes, such as CLU, also affect the occurrence and progression of AD through the accumulation and clearance of Aβ, nerve inflammation, and lipid metabolism (Foster et al., 2019; Uddin et al., 2020a). Previous genome-wide association studies (GWAS) have shown that rs11136000 (CLU) is a protective locus for AD risk, and several case-control association studies replicate this result (Harold et al., 2009; Lancaster et al., 2015; Balcar et al., 2021). However, some of other studies report no statistically significant association of rs11136000 on AD or no association in non-European populations (Carrasquillo et al., 2010; Seshadri et al., 2010; Seripa et al., 2018; Zhu et al., 2018). The conflicting results of these studies made us interested in investigating the effect of rs11136000 on AD. Moreover, how rs11136000 regulates CLU expression and leads to AD needs further evaluation (Hu et al., 2020, 2021a).
In this study, we integrated multiple omics data, including genome-wide association study (GWAS), expression quantitative trait loci (eQTLs), transcriptome and proteome data, to investigate whether rs11136000 regulates CLU expression and thereby contribute to AD. In addition, we identified the different effects of rs11136000 on AD patients of different genders.
Materials and methods
Genome-wide association studies datasets
Genome-wide association studies uses single nucleotide polymorphisms (SNPs) in the human genome as molecular genetic markers to analyze the correlation between genotype and phenotype, aiming to discover genetic risk variants that affect phenotype (Tam et al., 2019). A total of five large-scale GWAS datasets for AD were included in the statistical analysis of this study. First, we obtained two GWAS datasets for AD patients with clinical or autopsy diagnosis from International Genomics of Alzheimer’s Project (IGAP), including 17,008 AD cases and 37,154 controls, and 21,982 AD cases and 41,944 controls, respectively (Table 1; Lambert et al., 2013; Kunkle et al., 2019). In addition, we obtained three large GWAS cohorts for AD proxy from UK Biobank, including family history of maternal AD (27,696 cases and 260,980 controls), family history of patrilineal AD (14,338 cases and 245,941 controls), and family history of all AD patients (Marioni et al., 2018). All of the participants were of European descent.
Expression quantitative trait loci datasets
Expression quantitative trait loci are genetic variants that control the expression levels of quantitative trait genes. In particular, variants located in non-coding regions may cause disease by modulating gene expression. In this study, we obtained datasets that rs11136000 regulates gene expression in AD patients and controls, respectively. The eQTL data of AD and non-AD samples were obtained from Mayo clinic and Genotype-Tissue Expression (GTEx) project, respectively (Table 2; Allen et al., 2012; GTEx Consortium., 2017). The Mayo dataset contained gene expression data for temporal cortex (TCX) in 186 AD subjects and 170 normal subjects, and cerebellar tissue (CER) in 191 AD subjects and 181 normal subjects (Allen et al., 2012). In addition, eQTL data of 13 brain tissues, including amygdala, anterior cingulate cortex, caudate, cerebellar hemisphere, cerebellum, cortex, frontal cortex, hippocampus, hypothalamus, nucleus accumbens, putamen, spinal cord, and substantia nigra were obtained from GTEx (version 8) as controls (GTEx Consortium., 2017). The donors in GTEx were of multiple descents including European (85.3%), African (12.3%), Asian (1.4%), etc., (GTEx Consortium., 2017).
RNA expression datasets
RNA-seq data for AD versus controls was generated from over 2,100 samples from post-mortem brains of more than 1,100 individuals from seven distinct brain regions from three human cohort studies, including Religious Orders Study and Memory and Aging Project (ROSMAP), Mayo RNAseq (MAYO), and Mount Sinai Brain Bank (MSBB) (Bennett et al., 2012a,b; Zou et al., 2012; Ng et al., 2017). The seven brain regions contained dorsolateral prefrontal cortex (DLPFC), CER, TCX, frontal pole (FP), inferior frontal gyrus (IFG), parahippocampal gyrus (PHG), and superior temporal gyrus (STG).
Proteomics datasets
Proteomic data was generated from post-mortem brains of more than 500 individuals from four human cohort studies, including Banner Sun Health Research Institute (Banner), Baltimore Longitudinal Study on Aging (BLSA), MAYO and MSBB. Brain samples consisted of four different brain regions [DLPFC, Middle Frontal Gyrus (MFG), TCX and Anterior Prefrontal Cortex (AntPFC)]. Protein abundance was quantified using liquid-free quantification (LFQ). The proteomic data was adjusted for age, sex, and post mortem interval (PMI).
The effect of genetic variant rs11136000 on Alzheimer’s disease risk
We investigated the effect of rs11136000 T allele on AD risk in GWAS summary statistics for AD of clinically diagnosed or autopsy and first-degree relative proxies, respectively. In addition, we explored the effect of rs11136000 on AD patients with different genders using GWAS by proxy (GWAX) from UK Biobank. The statistically significant association is defined to be P < 5E-08 after adjusting for multiple testing.
The effect of rs11136000 on clusterin expression in Alzheimer’s disease and controls
We investigated the potential differential cis-regulated effect of rs11136000 on CLU in AD versus controls using an additive model eQTL analysis (Hu et al., 2020, 2021b; Qiu et al., 2022). According to the additive model, each allele has an independent effect on the trait. Here, we coded the possible genotypes of rs11136000 (TT = 2, TC = 1, CC = 0), where T is an effect allele and C is a non-effect allele. Thus, the differential regulation of CLU expression in rs11136000 T allele carriers of AD and controls can be calculated using linear regression models. The statistically significant association is defined to be P < 0.05/(number of brain tissues) = 0.05/17 = 0.00294 after multiple testing.
Differential expression of clusterin between Alzheimer’s disease and normal individuals
We evaluated the differential mRNA expression of CLU in seven brain regions between AD and controls from ROSMAP, MAYO and MSBB. Meanwhile, we investigated the differential protein expression of CLU in four brain regions of AD versus controls from DLPFC, MFG, TCX, and AntPFC. The differential expression was determined via ANOVA. The significance level of differential expression was defined as P < 0.05/7 = 0.00714 and P < 0.05/4 = 0.0125 after multiple testing.
Results
rs11136000 T allele reduced Alzheimer’s disease risk
rs11136000 T allele significantly reduced AD risk in both clinically diagnosed Alzheimer’s cohorts from IGAP (OR: 0.92, 95%CI: 0.91-0.94, P = 1.38E-24; OR: 0.88, 95%CI: 0.86-0.91, P = 4.90E-16) (Figure 1). In the UK Biobank cohort (using participants whose parents suffered from AD as a proxy for cases), rs11136000 T allele was suggestively protective against AD (OR: 0.95, 95%CI: 0.93-0.97, P = 1.88E-07) (Figure 1). However, rs11136000 only potentially affected female individuals with AD (OR: 0.94, 95%CI: 0.92-0.96, P = 3.96E-07).
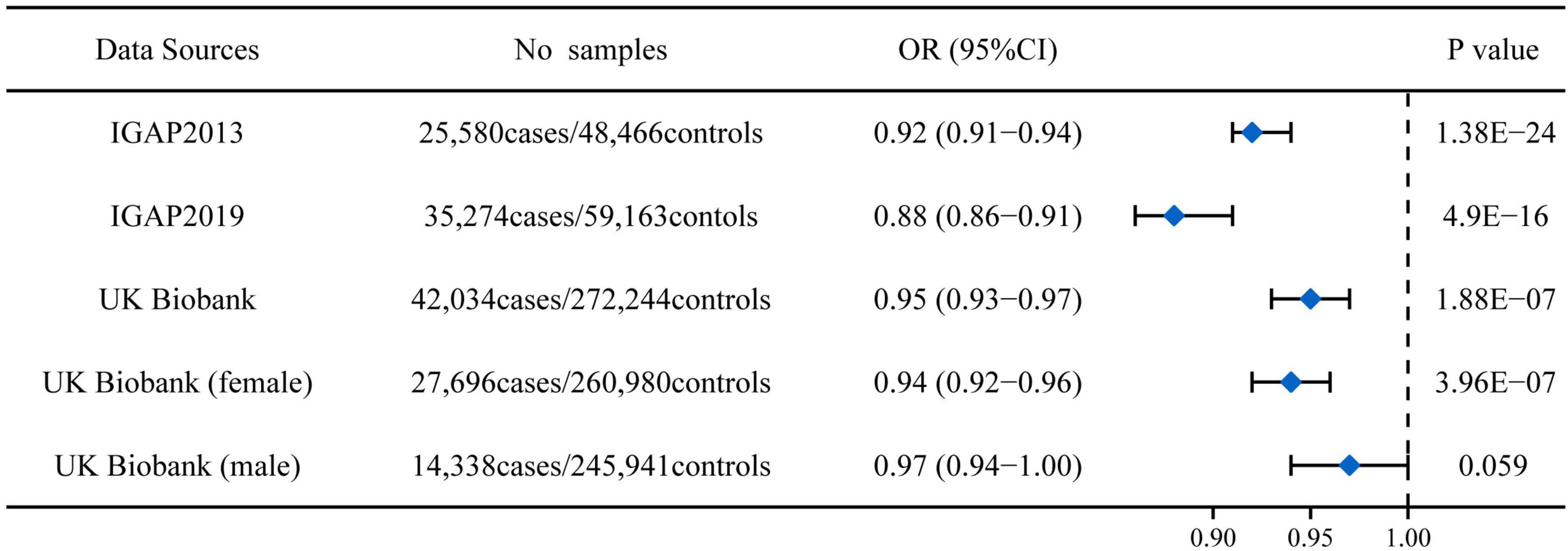
Figure 1. Association between rs11136000 variant T allele and AD. IGAP, International Genomics of Alzheimer’s Project. The statistically significant association is defined to be P < 5E-08.
rs11136000 upregulated clusterin expression in Alzheimer’s disease
rs11136000 downregulated CLU expression in all 13 normal brain tissues from GTEx, two of which passed multiple testing (Pnucleusaccumbens = 0.00023 and Pputamen = 0.00082) (Table 2). However, rs11136000 suggestively upregulated CLU expression in cerebellum (β = 0.0635, P = 0.23) and temporal cortex samples of AD (β = 0.0588, P = 0.031) (Table 2).
Clusterin differentially expressed in Alzheimer’s disease versus controls
To further determine the effect of CLU in AD patients, we investigated the differential expression of CLU between AD and controls in various brain regions at the level of gene expression and protein expression, respectively. The CLU mRNA expression in temporal cortex region of AD patients significantly differed from controls regardless of gender ( = 0.83, PTCX = 2.96E-10) (Table 3). However, CLU was only significantly differentially expressed in parahippocampal gyrus region of female AD patients compared to controls ( = 0.34, PPHG = 0.00032). Furthermore, CLU protein was detected in DLPFC and AntPFC, and was significantly differentially expressed in both two brain tissues ( = 0.29, PAntPFC = 0.00022; = 0.23, PDLPFC = 5.09E-06) (Figure 2).
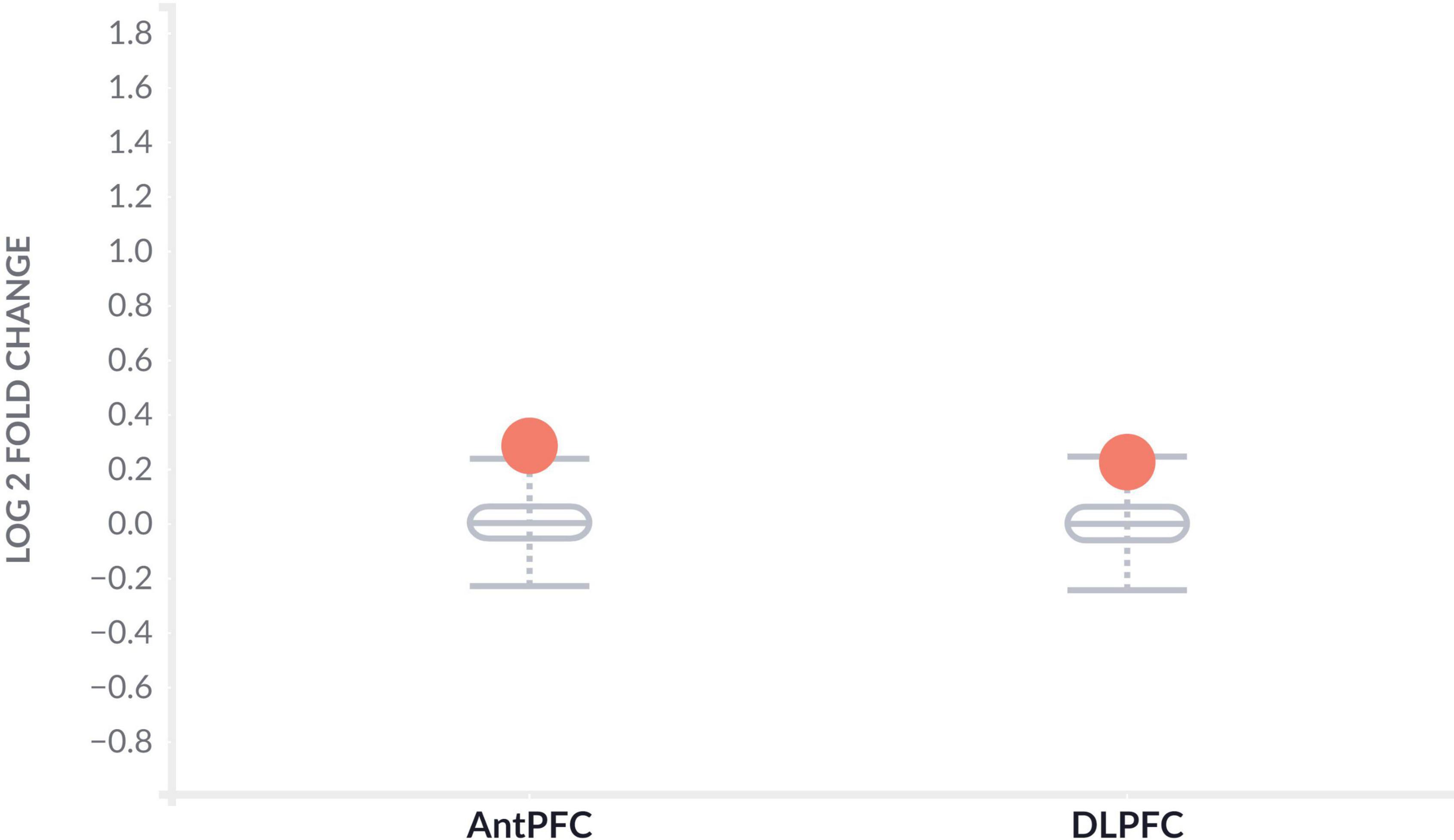
Figure 2. Differential protein expression of CLU between AD and normal samples. The gray boxplots represent the expression levels of CLU protein in the brain tissues of healthy participants. The orange dots represent the expression levels of CLU protein in the brain tissues of AD patients. AntPFC, anterior prefrontal cortex; DLPFC, dorsolateral prefrontal cortex.
Discussion
Large-scale GWAS in recent years have identified substantial genetic variants and genes associated with AD risk (Jansen et al., 2019; Kunkle et al., 2019; Schwartzentruber et al., 2021). Susceptibility loci including APOE have been confirmed by numerous studies (Al Mamun et al., 2020; Uddin et al., 2020b). CLU, also known as apolipoprotein J (APOJ) protein, is identified as the third-highest risk gene for late-onset AD (LOAD), contributing approximately 9% of AD risk (Bertram et al., 2007; Foster et al., 2019; Uddin et al., 2020a). Previous studies have shown that elevated CLU levels have been detected in the brain and plasma of AD individuals and are involved in neuroinflammation, lipid metabolism, and Aβ clearance in AD patients (Lidstrom et al., 1998; Bu, 2009; Thambisetty et al., 2010; Uddin et al., 2020a). However, some studies have also reported that CLU incorporation into amyloid aggregates is more harmful than Aβ42 aggregates alone (Uddin et al., 2020a). The ambiguous and complex role of CLU in AD prevents it from becoming a therapeutic target for AD.
In this study, we integrated GWAS, eQTL, gene expression and protein expression data to investigate whether rs11136000 (CLU) affects AD risk by regulating CLU expression. We successfully explained the pathway of rs11136000-CLU-AD. The results showed that rs11136000 significantly reduced AD risk in both clinically diagnosed AD and AD proxy. The effects of rs11136000 on AD risk with different genders and different diagnostic modalities were slightly different. In addition, previous meta-analyses and systematic reviews suggested that the heterogeneity of rs11136000 on AD risk was also reflected by race. Both Han et al. (2018) and Zhu et al. (2018) believed that rs11136000 only reduced the risk of AD in the European population, while the association was weak in the East Asian population. Subsequent eQTL analysis revealed heterogeneity of rs11136000 expression in various brain tissues. Significant difference of the regulation of rs11136000 on CLU expression was only showed in temporal cortex region between AD patients versus controls. Interestingly, CLU-immunopositive Aβ deposits were found in the temporal cortex of AD patients, and 29% of Aβ in brain tissue was associated with CLU protein (Martin-Rehrmann et al., 2005; Uddin et al., 2020a).
The study has some advantages. The multiple omics data used in this study were all from European populations, avoiding the bias associated with population stratification. Multiple omics data constructed a complete pathway that genetic variants regulate gene expression and then affect disease phenotype, which better explains the role of rs11136000 in the brain of AD patients than previous studies. However, this study also has certain limitations. It is difficult for us to obtain gender- and ethnic-specific multi-omics data, which limits the further disclosure of the specific regulatory role of rs11136000 on AD patients in different populations.
In conclusion, this study highlights the potential role of the variant rs11136000 on AD risk by regulating CLU expression. These findings reveal the importance of a better understanding of CLU function and dysfunction in the context of normal and AD individuals.
Data availability statement
The original contributions presented in this study are included in the article/supplementary material, further inquiries can be directed to the corresponding author.
Author contributions
SQ analyzed the data and drafted the manuscript. JM designed the study, revised the manuscript, and supervised this work. Both authors reviewed and approved the final version.
Acknowledgments
We acknowledge the consortiums that provided the data in this study. The results published here are in part based on data obtained from Agora, a platform initially developed by the NIA-funded AMP-AD consortium that shares evidence in support of AD target discovery. We thank the International Genomics of Alzheimer’s Project (IGAP) and UK Biobank (https://www.ukbiobank.ac.uk) for providing GWAS summary data. The investigators within IGAP provided data but did not participate in analysis or writing of this report. IGAP was made possible by the generous participation of the control subjects, the patients, and their families. The i-Select chips was funded by the French National Foundation on AD and related disorders. EADI was supported by the LABEX (laboratory of excellence program investment for the future) DISTALZ grant, Inserm, Institut Pasteur de Lille, Université de Lille 2, and the Lille University Hospital. GERAD was supported by the Medical Research Council (Grant n° 503480), Alzheimer’s Research UK (Grant n° 503176), the Wellcome Trust (Grant n° 082604/2/07/Z), and German Federal Ministry of Education and Research (BMBF): Competence Network Dementia (CND) grant n° 01GI0102, 01GI0711, 01GI0420. CHARGE was partly supported by the NIH/NIA grant R01 AG033193, the NIA AG081220, and AGES contract N01-AG-12100, the NHLBI grant R01 HL105756, the Icelandic Heart Association, and the Erasmus Medical Center and Erasmus University. ADGC was supported by the NIH/NIA grants: U01 AG032984, U24 AG021886, U01 AG016976, and the Alzheimer’s Association grant ADGC-10-196728. This research has been conducted using the UK Biobank resource.
Conflict of interest
The authors declare that the research was conducted in the absence of any commercial or financial relationships that could be construed as a potential conflict of interest.
Publisher’s note
All claims expressed in this article are solely those of the authors and do not necessarily represent those of their affiliated organizations, or those of the publisher, the editors and the reviewers. Any product that may be evaluated in this article, or claim that may be made by its manufacturer, is not guaranteed or endorsed by the publisher.
References
Al Mamun, A., Uddin, M. S., Bin Bashar, M. F., Zaman, S., Begum, Y., Bulbul, I. J., et al. (2020). Molecular insight into the therapeutic promise of targeting APOE4 for Alzheimer’s disease. Oxid. Med. Cell. Longev. 2020:5086250. doi: 10.1155/2020/5086250
Allen, M., Zou, F., Chai, H. S., Younkin, C. S., Crook, J., Pankratz, V. S., et al. (2012). Novel late-onset Alzheimer disease loci variants associate with brain gene expression. Neurology 79, 221–228. doi: 10.1212/WNL.0b013e3182605801
Alzheimer’s Association (2021). 2021 Alzheimer’s disease facts and figures. Alzheimers Dement. 17, 327–406. doi: 10.1002/alz.12328
Balcar, V. J., Zeman, T., Janout, V., Janoutova, J., Lochman, J., and Sery, O. (2021). Single nucleotide polymorphism rs11136000 of CLU gene (Clusterin, ApoJ) and the risk of late-onset Alzheimer’s disease in a central European population. Neurochem. Res. 46, 411–422. doi: 10.1007/s11064-020-03176-y
Belloy, M. E., Napolioni, V., and Greicius, M. D. (2019). A quarter century of APOE and Alzheimer’s disease: progress to date and the path forward. Neuron 101, 820–838. doi: 10.1016/j.neuron.2019.01.056
Bennett, D. A., Schneider, J. A., Arvanitakis, Z., and Wilson, R. S. (2012a). Overview and findings from the religious orders study. Curr. Alzheimer Res. 9, 628–645. doi: 10.2174/156720512801322573
Bennett, D. A., Schneider, J. A., Buchman, A. S., Barnes, L. L., Boyle, P. A., and Wilson, R. S. (2012b). Overview and findings from the rush memory and aging project. Curr. Alzheimer Res. 9, 646–663. doi: 10.2174/156720512801322663
Bertram, L., Mcqueen, M. B., Mullin, K., Blacker, D., and Tanzi, R. E. (2007). Systematic meta-analyses of Alzheimer disease genetic association studies: the AlzGene database. Nat. Genet. 39, 17–23. doi: 10.1038/ng1934
Bu, G. J. (2009). Apolipoprotein E and its receptors in Alzheimer’s disease: pathways, pathogenesis and therapy. Nat Rev Neurosci. 10, 333–344. doi: 10.1038/nrn2620
Carrasquillo, M. M., Belbin, O., Hunter, T. A., Ma, L., Bisceglio, G. D., Zou, F., et al. (2010). Replication of CLU, CR1, and PICALM associations with alzheimer disease. Arch Neurol. 67, 961–964. doi: 10.1001/archneurol.2010.147
Foster, E. M., Dangla-Valls, A., Lovestone, S., Ribe, E. M., and Buckley, N. J. (2019). Clusterin in Alzheimer’s disease: mechanisms, genetics, and lessons from other pathologies. Front Neurosci. 13:164. doi: 10.3389/fnins.2019.00164
GTEx Consortium. (2017). Genetic effects on gene expression across human tissues. Nature 550, 204–213.
Han, Z. J., Qu, J. J., Zhao, J. H., and Zou, X. (2018). Analyzing 74,248 samples confirms the association between CLU rs11136000 polymorphism and Alzheimer’s disease in caucasian but not Chinese population. Sci. Rep. 8:11062. doi: 10.1038/s41598-018-29450-2
Harold, D., Abraham, R., Hollingworth, P., Sims, R., Gerrish, A., Hamshere, M. L., et al. (2009). Genome-wide association study identifies variants at CLU and PICALM associated with Alzheimer’s disease. Nat. Genet. 41, 1088–1093.
Hu, Y., Sun, J. Y., Zhang, Y., Zhang, H., Gao, S., Wang, T., et al. (2021b). rs1990622 variant associates with Alzheimer’s disease and regulates TMEM106B expression in human brain tissues. BMC Med. 19:11. doi: 10.1186/s12916-020-01883-5
Hu, Y., Qiu, S., and Cheng, L. (2021a). Integration of multiple-omics data to analyze the population-specific differences for coronary artery disease. Comput. Math. Methods Med. 2021:7036592. doi: 10.1155/2021/7036592
Hu, Y., Zhang, H., Liu, B., Gao, S., Wang, T., Han, Z., et al. (2020). rs34331204 regulates TSPAN13 expression and contributes to Alzheimer’s disease with sex differences. Brain 143:e95.
Huynh, T. V., Davis, A. A., Ulrich, J. D., and Holtzman, D. M. (2017). Apolipoprotein E and Alzheimer’s disease: the influence of apolipoprotein E on amyloid-beta and other amyloidogenic proteins. J. Lipid Res. 58, 824–836.
Jansen, I. E., Savage, J. E., Watanabe, K., Bryois, J., Williams, D. M., Steinberg, S., et al. (2019). Genome-wide meta-analysis identifies new loci and functional pathways influencing Alzheimer’s disease risk. Nat. Genet. 51, 404–413.
Kunkle, B. W., Grenier-Boley, B., Sims, R., Bis, J. C., Damotte, V., Naj, A. C., et al. (2019). Genetic meta-analysis of diagnosed Alzheimer’s disease identifies new risk loci and implicates Abeta, tau, immunity and lipid processing. Nat. Genet. 51, 414–430.
Lambert, J. C., Ibrahim-Verbaas, C. A., Harold, D., Naj, A. C., Sims, R., Bellenguez, C., et al. (2013). Meta-analysis of 74,046 individuals identifies 11 new susceptibility loci for Alzheimer’s disease. Nat. Genet. 45, 1452–1458. doi: 10.1038/ng.2802
Lancaster, T. M., Brindley, L. M., Tansey, K. E., Sims, R. C., Mantripragada, K., Owen, M. J., et al. (2015). Alzheimer’s disease risk variant in CLU is associated with neural inefficiency in healthy individuals. Alzheimers Dement. 11, 1144–1152. doi: 10.1016/j.jalz.2014.10.012
Lidstrom, A. M., Bogdanovic, N., Hesse, C., Volkman, I., Davidsson, P., and Blennow, K. (1998). Clusterin (apolipoprotein J) protein levels are increased in hippocampus and in frontal cortex in Alzheimer’s disease. Exp. Neurol. 154, 511–521.
Marioni, R. E., Harris, S. E., Zhang, Q., Mcrae, A. F., Hagenaars, S. P., Hill, W. D., et al. (2018). GWAS on family history of Alzheimer’s disease. Transl. Psychiatry 8:99.
Martin-Rehrmann, M. D., Hoe, H. S., Capuani, E. M., and Rebeck, G. W. (2005). Association of apolipoprotein J-positive beta-amyloid plaques with dystrophic neurites in Alzheimer’s disease brain. Neurotox. Res. 7, 231–241. doi: 10.1007/BF03036452
Namba, Y., Tomonaga, M., Kawasaki, H., Otomo, E., and Ikeda, K. (1991). Apolipoprotein E immunoreactivity in cerebral amyloid deposits and neurofibrillary tangles in Alzheimer’s disease and kuru plaque amyloid in Creutzfeldt-Jakob disease. Brain Res. 541, 163–166.
Ng, B., White, C. C., Klein, H. U., Sieberts, S. K., Mccabe, C., Patrick, E., et al. (2017). An xQTL map integrates the genetic architecture of the human brain’s transcriptome and epigenome. Nat. Neurosci. 20, 1418–1426. doi: 10.1038/nn.4632
Pimenova, A. A., Raj, T., and Goate, A. M. (2018). Untangling genetic risk for Alzheimer’s disease. Biol. Psychiatry 83, 300–310.
Qiu, S., Hu, Y., and Cheng, L. (2022). BIN1 rs744373 located in enhancers of brain tissues upregulates BIN1 mRNA expression, thereby leading to Alzheimer’s disease. Alzheimers Dement. [Epub ahead of print]. doi: 10.1002/alz.12548
Scheltens, P., Blennow, K., Breteler, M. M., De Strooper, B., Frisoni, G. B., Salloway, S., et al. (2016). Alzheimer’s disease. Lancet 388, 505–517.
Schwartzentruber, J., Cooper, S., Liu, J. Z., Barrio-Hernandez, I., Bello, E., Kumasaka, N., et al. (2021). Genome-wide meta-analysis, fine-mapping and integrative prioritization implicate new Alzheimer’s disease risk genes. Nat. Genet. 53, 392–402.
Seripa, D., Panza, F., Paroni, G., D’onofrio, G., Bisceglia, P., Gravina, C., et al. (2018). Role of CLU, PICALM, and TNK1 genotypes in aging with and without Alzheimer’s disease. Mol. Neurobiol. 55, 4333–4344. doi: 10.1007/s12035-017-0547-x
Seshadri, S., Fitzpatrick, A. L., Ikram, M. A., Destefano, A. L., Gudnason, V., Boada, M., et al. (2010). Genome-wide analysis of genetic loci associated with Alzheimer disease. JAMA 303, 1832–1840. doi: 10.1001/jama.2010.574
Tam, V., Patel, N., Turcotte, M., Bosse, Y., Pare, G., and Meyre, D. (2019). Benefits and limitations of genome-wide association studies. Nat. Rev. Genet. 20, 467–484. doi: 10.1038/s41576-019-0127-1
Thambisetty, M., Simmons, A., Velayudhan, L., Hye, A., Campbell, J., Zhang, Y., et al. (2010). Association of plasma clusterin concentration with severity, pathology, and progression in Alzheimer disease. Arch Gen Psychiatry. 67, 739–748. doi: 10.1001/archgenpsychiatry.2010.78
Uddin, M. S., Kabir, M. T., Begum, M. M., Islam, M. S., Behl, T., and Ashraf, G. M. (2020a). Exploring the role of CLU in the pathogenesis of Alzheimer’s disease. Neurotox. Res. 39, 2108–2119. doi: 10.1007/s12640-020-00271-4
Uddin, M. S., Kabir, M. T., Rahman, M. S., Behl, T., Jeandet, P., Ashraf, G. M., et al. (2020b). Revisiting the amyloid cascade hypothesis: from anti-a beta therapeutics to auspicious new ways for Alzheimer’s disease. Int. J. Mol. Sci. 21:5858. doi: 10.3390/ijms21165858
Van Cauwenberghe, C., Van Broeckhoven, C., and Sleegers, K. (2016). The genetic landscape of Alzheimer disease: clinical implications and perspectives. Genet. Med. 18, 421–430. doi: 10.1038/gim.2015.117
Zhu, R. X., Liu, X., and He, Z. Y. (2018). Association between CLU gene rs11136000 polymorphism and Alzheimer’s disease: an updated meta-analysis. Neurol. Sci. 39, 679–689. doi: 10.1007/s10072-018-3259-8
Keywords: Alzheimer’s disease, genetic variant, CLU, genome-wide association study, rs11136000, eQTL
Citation: Ma J and Qiu S (2022) Genetic variant rs11136000 upregulates clusterin expression and reduces Alzheimer’s disease risk. Front. Neurosci. 16:926830. doi: 10.3389/fnins.2022.926830
Received: 23 April 2022; Accepted: 11 July 2022;
Published: 10 August 2022.
Edited by:
Zhijie Han, Chongqing Medical University, ChinaReviewed by:
Sandra Rebelo, University of Aveiro, PortugalSteven Estus, University of Kentucky, United States
Copyright © 2022 Ma and Qiu. This is an open-access article distributed under the terms of the Creative Commons Attribution License (CC BY). The use, distribution or reproduction in other forums is permitted, provided the original author(s) and the copyright owner(s) are credited and that the original publication in this journal is cited, in accordance with accepted academic practice. No use, distribution or reproduction is permitted which does not comply with these terms.
*Correspondence: Jin Ma, MTAzMTE4OTAwQHFxLmNvbQ==