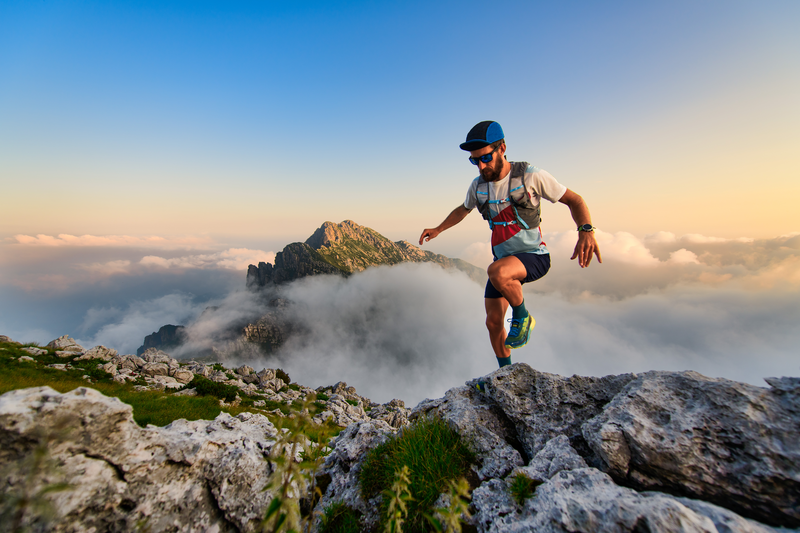
94% of researchers rate our articles as excellent or good
Learn more about the work of our research integrity team to safeguard the quality of each article we publish.
Find out more
SYSTEMATIC REVIEW article
Front. Neurol.
Sec. Dementia and Neurodegenerative Diseases
Volume 16 - 2025 | doi: 10.3389/fneur.2025.1556535
This article is part of the Research Topic Cellular Senescence and its Impact on Neurological Disorders View all articles
The final, formatted version of the article will be published soon.
You have multiple emails registered with Frontiers:
Please enter your email address:
If you already have an account, please login
You don't have a Frontiers account ? You can register here
Objective: This systematic review and meta-analysis aimed to evaluate the association between the methylenetetrahydrofolate reductase (5,10-methylenetetrahydrofolate reductase, MTHFR) cytosine (C)677thymine (T) polymorphism and cerebral small vessel disease (CSVD), addressing potential sources of heterogeneity and publication bias.Methods: An extensive search of databases, including PubMed, the Excerpta Medical Database, and The Cochrane Database of Systematic Reviews, was conducted to identify studies assessing the prevalence of the MTHFR C677T variant associated with CSVD subtypes in humans. Random or fixed effects models were used to accommodate heterogeneity across the study results. Odds ratios (ORs) and weighted mean differences with 95% confidence intervals (CIs) were used for pooled analyses of the relationships between the MTHFR C677T variant associated and CSVD subtypes. Subgroup analyses and assessments of publication bias were performed using Stata software.Results: Nineteen studies involving 12,441 participants were included. Significant associations were observed across all genetic models: recessive (OR=1.33; 95%CI=
Keywords: MTHFR 677CT polymorphism, Cerebral small vessel disease, metaanalysis, white matter hyperintensities, lacunar infarction, microbleeds
Received: 07 Jan 2025; Accepted: 28 Feb 2025.
Copyright: © 2025 Zheng, Lai, Wang, Kong, Cai, Lin, Wang, Cai, Pi and 秦. This is an open-access article distributed under the terms of the Creative Commons Attribution License (CC BY). The use, distribution or reproduction in other forums is permitted, provided the original author(s) or licensor are credited and that the original publication in this journal is cited, in accordance with accepted academic practice. No use, distribution or reproduction is permitted which does not comply with these terms.
* Correspondence:
Min Pi, Shenzhen Traditional Chinese Medicine Hospital, Shenzhen ,Guangdong, China
秀德 秦, Shenzhen Traditional Chinese Medicine Hospital, Shenzhen ,Guangdong, China
Disclaimer: All claims expressed in this article are solely those of the authors and do not necessarily represent those of their affiliated organizations, or those of the publisher, the editors and the reviewers. Any product that may be evaluated in this article or claim that may be made by its manufacturer is not guaranteed or endorsed by the publisher.
Research integrity at Frontiers
Learn more about the work of our research integrity team to safeguard the quality of each article we publish.