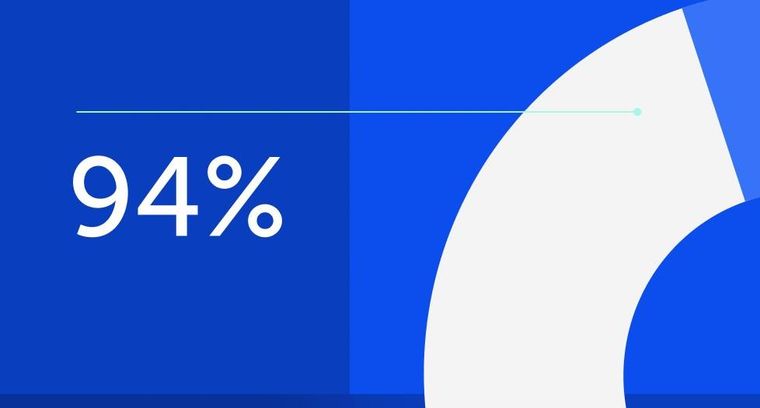
94% of researchers rate our articles as excellent or good
Learn more about the work of our research integrity team to safeguard the quality of each article we publish.
Find out more
OPINION article
Front. Neurol., 12 March 2025
Sec. Neuroinfectious Diseases
Volume 16 - 2025 | https://doi.org/10.3389/fneur.2025.1553594
Anti-retroviral treatment (ART) has transformed HIV infection into a manageable chronic disease. It restores immune functionality and eliminates or reduces many AIDS-defining co-morbidities. In particular, the severity of HIV-associated neurocognitive disorders (HAND) has been greatly reduced, significantly decreasing the prevalence of HIV-associated dementia (1). However, the overall prevalence of milder forms of HAND remains comparable to the pre-ART era (2–4). Neurocognitive impairment affects nearly 50% of people living with HIV (PLWH), yet the HIV-specific factors responsible for this co-morbidity remain poorly understood. A role for viral proteins such as gp120, Tat, and Nef has been suggested (5–9). Two recent articles have provided evidence that Nef is the key HIV protein responsible for neuroinflammation, myelin impairment, and neuronal injury.
Both studies underscore the detrimental effects of Nef on the CNS, particularly in the context of HIV-1 infection. The first study (10) demonstrates the Nef's key role in neuroinflammation and myelin damage in the context of mouse brain infection with EcoHIV, a hybrid virus carrying HIV core and envelope of murine leukemia virus (11). This model is consistent with ART-suppressed HIV infection in people, as EcoHIV establishes latent infection of the murine brain (12). Considering brain inflammation, expression of inflammatory cytokines in mice infected with Nef-deficient EcoHIV was between the levels observed in mock-infected mice and mice infected with Nef-positive EcoHIV, and significantly differed from both (10), indicating that other HIV factors besides Nef contributed to neuroinflammation. This conclusion is in line with proposed role of Tat in neuroinflammation (8, 9). Interestingly, the effects of Nef and the other HIV factors (presumably Tat) on neuroinflammation appear additive, suggesting that they may work throw different mechanisms. Indeed, Tat was suggested to disrupt the BBB and activate NF-kB on monocytes and microglia, promoting migration of activated cells into the brain and production of inflammatory cytokines (13, 14), whereas Nef induces inflammation in myeloid cells by affecting cholesterol homeostasis and lipid rafts (15).
While Nef appears to cooperate with other HIV factors to promote inflammation, the observed effects on myelin impairment and neuronal injury in this model were attributed exclusively to Nef, as no such defects were detected in mice infected with Nef-deficient EcoHIV (10). However, this conclusion may be influenced by the relatively short post-infection observation period in this study (2–3 weeks), as chronic inflammation is expected to contribute to neuronal damage over time. Consequently, the potential contribution of other HIV proteins, such as Tat, to neurotoxicity via the induction of neuroinflammation may have been overlooked. These findings also argue against the direct acute cytotoxicity of Tat proposed in previous studies (16). This discrepancy may arise because the Tat concentrations required to induce cytotoxicity in vitro [~400 nM (17)] are likely not achieved in the EcoHIV model, although Tat concentrations in the brain have not been directly measured in this context. Notably, Tat concentrations in the cerebrospinal fluid (CSF) of ART-treated PLWH range from 0.5 to 6.5 ng/mL (36–465 pM) (18), which is significantly lower than the neurotoxic concentrations used in in vitro studies. Collectively, these results suggest that Nef is a primary driver of acute neuroinflammation, myelin impairment and neuronal damage in the EcoHIV model of HAND. Further studies are needed to explore the potential long-term contributions of other HIV proteins, such as Tat, to neuropathogenesis in this model.
The second study (19) provides a more in-depth analysis of Nef-mediated brain damage and introduces the concept of Nef-containing EVs as key mediators of myelin impairment and oligodendrocyte injury. Instead of injecting HIV directly, in this study mice were injected in the brain with Nef EVs, a strategy informed by growing evidence of EVs' roles in neurological diseases (20). Nef is efficiently incorporated into EVs, and these vesicles have been detected in the blood of PLWH with undetectable HIV loads (21). Since EVs can routinely cross the blood-brain barrier (22), they may serve as a vehicle for Nef to reach and affect the central nervous system. Furthermore, Nef has been identified in the brains of ART-treated PLWH (23) and SIV-infected monkeys (24), and it can be taken up by neighboring cells, including neurons, leading to neuronal damage (25). This mechanism is particularly significant because it offers a novel explanation for how Nef may propagate neurotoxic effects throughout the brain without requiring direct viral infection of every affected cell. Importantly, even when HIV replication is suppressed to undetectable levels by ART, Nef EVs continue to be produced (26) and remain capable of exerting neurotoxic effects.
This study demonstrated that Nef-containing EVs disrupted myelin sheaths and inflicted damage upon glial cells, in particular oligodendrocytes, within the murine CNS. The damage to oligodendrocytes was partially prevented by agents that blocked Nef-mediated inhibition of the activity of cellular cholesterol transporter, ABCA1, suggesting that the effects of Nef EVs on myelination were mediated by alterations in cholesterol homeostasis, a known feature of Nef EVs (15). In addition, Nef EVs promoted inflammatory responses by significantly increasing the number of activated microglial cells at the sites of injection.
Both studies identified similar neurotoxic effects of Nef, including pro-inflammatory activity and myelin impairment. Since Nef EVs were not detected in the EcoHIV study, it remains unclear whether the observed effects were driven solely by EcoHIV infection of microglial cells or by a combination of direct infection and EV-mediated toxicity. A more likely scenario is that both mechanisms contribute, with infected microglia triggering inflammation while Nef EVs induce demyelination. This is supported by the low levels of myelin impairment observed in that study (10), which are consistent with Nef EV levels falling below the limit of detection.
When related to HIV infection and HAND, the findings from these two studies support the following model: Under ART treatment, HIV persists in brain-resident cells, particularly microglia and astrocytes, which produce Nef EVs. Additionally, Nef EVs originating from peripheral sources enter the brain via the bloodstream. HIV-infected microglia adopt a pro-inflammatory phenotype, releasing inflammatory cytokines, while Nef EVs further exacerbate inflammation and disrupt myelin integrity. Together, these effects contribute to neuronal damage and impaired synaptic communication, ultimately leading to cognitive deficits.
The two studies reviewed here have several limitations. They did not specifically assess Nef's direct neurotoxicity, though prior research suggests Nef may induce neuronal injury via caspase activation and free radical production (27). However, neuronal death is not a defining feature of the mild forms of HAND that are most prevalent in the ART era (28). Beyond its role in neuroinflammation and myelin impairment, Nef may also disrupt the blood-brain barrier (29), potentially exacerbating the pathogenic mechanisms discussed in this review. Additionally, Nef-driven inflammation could be amplified by its stimulation of CCL5 in microglia (30), creating a feedback loop that sustains neuroinflammatory damage. These aspects, along with the role of Nef EVs in the EcoHIV model, remain unexamined.
Clarifying Nef's role in HAND pathogenesis not only deepens our understanding of the disease but also identifies promising therapeutic targets for preventing neurocognitive decline in PLWH.
The relevance of the EcoHIV model to HAND in PLWH remains incompletely understood. A key limitation of this model is the absence of gp120, a viral protein implicated in neuropathogenesis, which restricts investigations into its role and likely reduces the overall pathological impact. Additionally, unlike HIV-1, which enters cells via CD4/CCR5 or CD4/CXCR4, EcoHIV utilizes mCAT-1, a receptor broadly expressed across various mouse tissues, including the brain. Despite this difference, EcoHIV primarily infects CD4+ T cells and monocytes/macrophages in the periphery (11), while in the brain, it predominantly resides in myeloid cells (31, 32).
Notably, EcoHIV-infected mice develop neurocognitive impairment (NCI) resembling that seen in ART-treated HIV-infected individuals (32, 33). In these mice, infected microglia play a central role in NCI pathogenesis, mirroring findings in HIV-infected humans (34). Specifically, hippocampus- and amygdala-dependent deficits in memory consolidation and recall observed in EcoHIV-infected mice (32) closely parallel cognitive impairments in ART-treated PLWH (35). Additionally, the selective loss of dopaminergic neurons—without significant damage to non-dopaminergic neurons—in the substantia nigra and subventricular zones of EcoHIV-infected mice (36) mirrors aspects of HIV-associated neurotoxicity (37). Crucially, NCI in EcoHIV-infected mice depends on the persistent replication of the virus within brain microglia, which continues despite immune responses and ART (32), resembling the persistence of HIV in the brains of PLWH with mild forms of HAND (38). While differences exist between HIV infection in the human brain and EcoHIV infection in the mouse brain, the resulting neurological impairments share striking similarities. Given these parallels, it is reasonable to infer that the underlying mechanisms of neuropathology in both infections are also closely related.
Neuroinflammation is a hallmark of HAND and is widely recognized as a primary driver of neuronal injury and cognitive decline (39–42). The pro-inflammatory effects of Nef on human myeloid cells (15, 43, 44), along with its presence in post-mortem brain tissues of individuals (23) and monkeys (24) with HAND, highlight its potential role in driving neuroinflammation.
Beyond Nef, other HIV proteins, including Tat and gp120, have also been shown to exert pro-inflammatory effects (45, 46). Additionally, systemic inflammation resulting from the leakage of bacterial products through the gut due to incomplete restoration of the gut mucosa (“leaky gut”) has been implicated as a contributing factor (47). The relative contributions of these factors to neuroinflammation in PLWH remain unclear and require further investigation.
Less is known about Nef's role in myelin impairment. However, myelin loss and disruption are consistently observed in the brains of individuals with HAND (28, 48) and SIV-infected monkeys (49, 50). Notably, Nef has been detected in brain regions critical for cognition and motor function, where demyelination is evident (48). Together with previous findings demonstrating Nef's pathogenic effects on oligodendrocytes (51) and the attenuation of these effects in monkeys infected with Nef-attenuated SIV (52), these studies reinforce the significance of Nef-dependent demyelination observed in our research. The damaging impact of Nef on oligodendrocytes and myelin may contribute to the motor deficits and cognitive impairments that characterize HAND.
The reviewed studies suggest several potential therapeutic strategies for mitigating the neurotoxic effects of Nef and improving the management of HAND. Beyond directly targeting Nef with small-molecule inhibitors (53), blocking Nef's interference with cholesterol efflux presents a promising approach to protecting oligodendrocytes (19) and preserving myelin integrity. This can be achieved through inducers of expression of ABCA1, the main cellular effector of cholesterol efflux, by such agents as LXR agonists (54), or inhibitors that disrupt the interaction between Nef and calnexin, thereby preventing Nef-mediated impairment of ABCA1 maturation (55). Notably, this strategy may also help reduce Nef-driven inflammation (5).
Furthermore, the recent discovery that Nef is exposed on Nef EVs (56) opens the possibility of specifically targeting these pathogenic entities using monoclonal antibodies. Monoclonal antibody therapies have revolutionized the treatment of various diseases by providing precise and effective interventions. Examples include autoimmune diseases such as rheumatoid arthritis, treated with anti-TNF antibodies (57); infectious diseases like COVID-19, managed with SARS-CoV-2 spike protein-targeting antibodies (58); and cancers treated with anti-PD-1 antibodies (59). To apply this approach to neutralizing Nef EVs, it will be essential to identify a high-affinity antibody targeting a conserved region of Nef, ensuring broad efficacy against all Nef variants.
The current studies contribute to the growing body of evidence supporting Nef's central role in the pathogenesis of HAND. Its involvement in neuroinflammation, oligodendrocyte damage, and myelin impairment highlights Nef as a promising therapeutic target in HIV-1 infection. The identification of extracellular vesicles carrying Nef as mediators of neuronal injury further reinforces the potential of targeting Nef EVs for intervention. A key novel insight from these studies is the demyelination effect of Nef EVs, offering a potential explanation for myelin impairment in HAND. Future research should focus on delineating the precise molecular pathways through which Nef exerts its neurotoxic effects, as well as exploring therapeutic strategies to block these mechanisms and prevent or reverse CNS damage. A critical priority will be distinguishing the respective contributions of HIV-infected brain cells and the Nef EVs they produce to neurocognitive impairment. Additionally, the mechanisms underlying Nef-mediated myelin damage remain to be fully elucidated. Advancing our understanding in these areas will be crucial for accelerating the development of effective treatments targeting Nef-driven neurotoxicity. Given the persistent challenges in managing HAND, targeted interventions that neutralize Nef's neurotoxic effects could provide a much-needed strategy to improve neurological outcomes in individuals living with HIV.
MB: Writing – original draft, Writing – review & editing.
The author(s) declare that financial support was received for the research and/or publication of this article. This work was supported by NIH grants R01 NS124477 and P30 AI117970.
The author declares that the research was conducted in the absence of any commercial or financial relationships that could be construed as a potential conflict of interest.
The author(s) declare that no Gen AI was used in the creation of this manuscript.
All claims expressed in this article are solely those of the authors and do not necessarily represent those of their affiliated organizations, or those of the publisher, the editors and the reviewers. Any product that may be evaluated in this article, or claim that may be made by its manufacturer, is not guaranteed or endorsed by the publisher.
1. Bandera A, Taramasso L, Bozzi G, Muscatello A, Robinson JA, Burdo TH, et al. HIV-associated neurocognitive impairment in the modern ART era: are we close to discovering reliable biomarkers in the setting of virological suppression? Front Aging Neurosci. (2019) 11:187. doi: 10.3389/fnagi.2019.00187
2. Sacktor N, Skolasky RL, Seaberg E, Munro C, Becker JT, Martin E, et al. Prevalence of HIV-associated neurocognitive disorders in the multicenter AIDS cohort study. Neurology. (2016) 86:334–40. doi: 10.1212/WNL.0000000000002277
3. Heaton RK, Franklin DR, Ellis RJ, McCutchan JA, Letendre SL, Leblanc S, et al. HIV-associated neurocognitive disorders before and during the era of combination antiretroviral therapy: differences in rates, nature, and predictors. J Neurovirol. (2011) 17:3–16. doi: 10.1007/s13365-010-0006-1
4. Tozzi V, Balestra P, Bellagamba R, Corpolongo A, Salvatori MF, Visco-Comandini U, et al. Persistence of neuropsychologic deficits despite long-term highly active antiretroviral therapy in patients with HIV-related neurocognitive impairment: prevalence and risk factors. J Acquir Immune Defic Syndr. (2007) 45:174–82. doi: 10.1097/QAI.0b013e318042e1ee
5. Sviridov D, Bukrinsky M. Neuro-HIV-new insights into pathogenesis and emerging therapeutic targets. FASEB J. (2023) 37:e23301. doi: 10.1096/fj.202301239RR
6. Shrestha J, Santerre M, Allen CN, Arjona SP, Hooper R, Mukerjee R, et al. HIV-1 gp120 protein promotes HAND through the calcineurin pathway activation. Mitochondrion. (2023) 70:31–40. doi: 10.1016/j.mito.2023.03.003
7. Marino J, Maubert ME, Mele AR, Spector C, Wigdahl B, Nonnemacher MR. Functional impact of HIV-1 tat on cells of the CNS and its role in HAND. Cell Mol Life Sci. (2020) 77:5079–99. doi: 10.1007/s00018-020-03561-4
8. Muvenda T, Williams AA, Williams ME. Transactivator of transcription (Tat)-induced neuroinflammation as a key pathway in neuronal dysfunction: a scoping review. Mol Neurobiol. (2024) 61:9320–46. doi: 10.1007/s12035-024-04173-w
9. Thompson LJ, Genovese J, Hong Z, Singh MV, Singh VB. HIV-associated neurocognitive disorder: a look into cellular and molecular pathology. Int J Mol Sci. (2024) 25:4697. doi: 10.3390/ijms25094697
10. Schenck JK, Clarkson-Paredes C, Pushkarsky T, Wang Y, Miller RH, Bukrinsky MI. Nef mediates neuroimmune response, myelin impairment, and neuronal injury in EcoHIV-infected mice. Life Sci Alliance. (2025) 8:e202402879. doi: 10.26508/lsa.202402879
11. Potash MJ, Chao W, Bentsman G, Paris N, Saini M, Nitkiewicz J, et al. mouse model for study of systemic HIV-1 infection, antiviral immune responses, and neuroinvasiveness. Proc Natl Acad Sci USA. (2005) 102:3760–5. doi: 10.1073/pnas.0500649102
12. Kim BH, Chao W, Hadas E, Borjabad A, Potash MJ, Volsky DJ. EcoHIV infection of primary murine brain cell cultures to model HIV replication and neuropathogenesis. Viruses. (2024) 16:693. doi: 10.3390/v16050693
13. Sui Z, Sniderhan LF, Schifitto G, Phipps RP, Gelbard HA, Dewhurst S, et al. Functional synergy between CD40 ligand and HIV-1 Tat contributes to inflammation: implications in HIV type 1 dementia. J Immunol. (2007) 178:3226–36. doi: 10.4049/jimmunol.178.5.3226
14. Toborek M, Lee YW, Pu H, Malecki A, Flora G, Garrido R, et al. HIV-Tat protein induces oxidative and inflammatory pathways in brain endothelium. J Neurochem. (2003) 84:169–79. doi: 10.1046/j.1471-4159.2003.01543.x
15. Mukhamedova N, Hoang A, Dragoljevic D, Dubrovsky L, Pushkarsky T, Low H, et al. Exosomes containing HIV protein Nef reorganize lipid rafts potentiating inflammatory response in bystander cells. PLoS Pathog. (2019) 15:e1007907. doi: 10.1371/journal.ppat.1007907
16. King JE, Eugenin EA, Buckner CM, Berman JW. HIV tat and neurotoxicity. Microbes Infect. (2006) 8:1347–57. doi: 10.1016/j.micinf.2005.11.014
17. Kruman II, Nath A, Mattson MP. HIV-1 protein tat induces apoptosis of hippocampal neurons by a mechanism involving caspase activation, calcium overload, oxidative stress. ExpNeurol. (1998) 154:276–88. doi: 10.1006/exnr.1998.6958
18. Henderson LJ, Johnson TP, Smith BR, Reoma LB, Santamaria UA, Bachani M, et al. Presence of tat and transactivation response element in spinal fluid despite antiretroviral therapy. AIDS. (2019) 33:S145–57. doi: 10.1097/QAD.0000000000002268
19. Schenck JK, Karl MT, Clarkson-Paredes C, Bastin A, Pushkarsky T, Brichacek B, et al. Extracellular vesicles produced by HIV-1 Nef-expressing cells induce myelin impairment and oligodendrocyte damage in the mouse central nervous system. J Neuroinflammation. (2024) 21:127. doi: 10.1186/s12974-024-03124-5
20. Ghosh M, Pearse DD. The Yin and Yang of microglia-derived extracellular vesicles in CNS injury and diseases. Cells. (2024) 13:1834. doi: 10.3390/cells13221834
21. Ferdin J, Goricar K, Dolzan V, Plemenitas A, Martin JN, Peterlin BM, et al. Viral protein Nef is detected in plasma of half of HIV-infected adults with undetectable plasma HIV RNA. PLoS ONE. (2018) 13:e0191613. doi: 10.1371/journal.pone.0191613
22. Banks WA, Sharma P, Bullock KM, Hansen KM, Ludwig N, Whiteside TL. Transport of extracellular vesicles across the blood-brain barrier: brain pharmacokinetics and effects of inflammation. Int J Mol Sci. (2020) 21:4407. doi: 10.3390/ijms21124407
23. Pushkarsky T, Ward A, Ivanov A, Lin X, Sviridov D, Nekhai S, et al. Abundance of Nef and p-Tau217 in brains of individuals diagnosed with HIV-associated neurocognitive disorders correlate with disease severance. Mol Neurobiol. (2022) 59:1088–97. doi: 10.1007/s12035-021-02608-2
24. Yarandi SS, Robinson JA, Vakili S, Donadoni M, Burdo TH, Sariyer IK. Characterization of Nef expression in different brain regions of SIV-infected macaques. PLoS ONE. (2020) 15:e0241667. doi: 10.1371/journal.pone.0241667
25. Sami Saribas A, Cicalese S, Ahooyi TM, Khalili K, Amini S, Sariyer IK. HIV-1 Nef is released in extracellular vesicles derived from astrocytes: evidence for Nef-mediated neurotoxicity. Cell Death Dis. (2017) 8:e2542. doi: 10.1038/cddis.2016.467
26. Lee JH, Schierer S, Blume K, Dindorf J, Wittki S, Xiang W, et al. HIV-Nef and ADAM17-containing plasma extracellular vesicles induce and correlate with immune pathogenesis in chronic HIV infection. EBioMedicine. (2016) 6:103–13. doi: 10.1016/j.ebiom.2016.03.004
27. Trillo-Pazos G, McFarlane-Abdulla E, Campbell IC, Pilkington GJ, Everall IP. Recombinant Nef HIV-IIIB protein is toxic to human neurons in culture. Brain Res. (2000) 864:315–26. doi: 10.1016/S0006-8993(00)02213-7
28. Gelman BB. Neuropathology of HAND with suppressive antiretroviral therapy: encephalitis and neurodegeneration reconsidered. Curr HIV/AIDS Rep. (2015) 12:272–9. doi: 10.1007/s11904-015-0266-8
29. Raymond AD, Diaz P, Chevelon S, Agudelo M, Yndart-Arias A, Ding H, et al. Microglia-derived HIV Nef+ exosome impairment of the blood-brain barrier is treatable by nanomedicine-based delivery of Nef peptides. J Neurovirol. (2016) 22:129–39. doi: 10.1007/s13365-015-0397-0
30. Liu X, Shah A, Gangwani MR, Silverstein PS, Fu M, Kumar A. HIV-1 Nef induces CCL5 production in astrocytes through p38-MAPK and PI3K/Akt pathway and utilizes NF-kB, CEBP and AP-1 transcription factors. Sci Rep. (2014) 4:4450. doi: 10.1038/srep04450
31. He H, Sharer LR, Chao W, Gu CJ, Borjabad A, Hadas E, et al. Enhanced human immunodeficiency virus type 1 expression and neuropathogenesis in knockout mice lacking type I interferon responses. J Neuropathol Exp Neurol. (2014) 73:59–71. doi: 10.1097/NEN.0000000000000026
32. Kelschenbach J, He H, Kim BH, Borjabad A, Gu CJ, Chao W, et al. Efficient expression of HIV in immunocompetent mouse brain reveals a novel nonneurotoxic viral function in hippocampal synaptodendritic injury and memory impairment. MBio. (2019) 10:e00591–19. doi: 10.1128/mBio.00591-19
33. Gu CJ, Borjabad A, Hadas E, Kelschenbach J, Kim BH, Chao W, et al. EcoHIV infection of mice establishes latent viral reservoirs in T cells and active viral reservoirs in macrophages that are sufficient for induction of neurocognitive impairment. PLoS Pathog. (2018) 14:e1007061. doi: 10.1371/journal.ppat.1007061
34. Rappaport J, Volsky DJ. Role of the macrophage in HIV-associated neurocognitive disorders and other comorbidities in patients on effective antiretroviral treatment. J Neurovirol. (2015) 21:235–41. doi: 10.1007/s13365-015-0346-y
35. Carroll A, Brew B. HIV-associated neurocognitive disorders: recent advances in pathogenesis, biomarkers, and treatment. F1000Res. (2017) 6:312. doi: 10.12688/f1000research.10651.1
36. Olson KE, Bade AN, Namminga KL, Potash MJ, Mosley RL, Poluektova LY, et al. Persistent EcoHIV infection induces nigral degeneration in 1-methyl-4-phenyl-1,2,3,6-tetrahydropyridine-intoxicated mice. J Neurovirol. (2018) 24:398–410. doi: 10.1007/s13365-018-0629-1
37. Everall IP, Heaton RK, Marcotte TD, Ellis RJ, McCutchan JA, Atkinson JH, et al. Cortical synaptic density is reduced in mild to moderate human immunodeficiency virus neurocognitive disorder. HNRC group HIV neurobehavioral research center. Brain Pathol. (1999) 9:209–17. doi: 10.1111/j.1750-3639.1999.tb00219.x
38. Schlachetzki JC, Gianella S, Ouyang Z, Lana AJ, Yang X, O'Brien S, et al. Gene expression and chromatin conformation of microglia in virally suppressed people with HIV. Life Sci Alliance. (2024) 7:e202402736. doi: 10.26508/lsa.202402736
39. Williams ME, Naude PJW. The relationship between HIV-1 neuroinflammation, neurocognitive impairment and encephalitis pathology: a systematic review of studies investigating post-mortem brain tissue. Rev Med Virol. (2024) 34:e2519. doi: 10.1002/rmv.2519
40. Hong S, Banks WA. Role of the immune system in HIV-associated neuroinflammation and neurocognitive implications. Brain Behav Immun. (2015) 45:1–12. doi: 10.1016/j.bbi.2014.10.008
41. Sreeram S, Ye F, Garcia-Mesa Y, Nguyen K, El Sayed A, Leskov K, et al. The potential role of HIV-1 latency in promoting neuroinflammation and HIV-1-associated neurocognitive disorder. Trends Immunol. (2022) 43:630–9. doi: 10.1016/j.it.2022.06.003
42. Strain JF, Burdo TH, Song SK, Sun P, El-Ghazzawy O, Nelson B, et al. Diffusion basis spectral imaging detects ongoing brain inflammation in virologically well-controlled HIV+ patients. J Acquir Immune Defic Syndr. (2017) 76:423–30. doi: 10.1097/QAI.0000000000001513
43. Dubrovsky L, Brichacek B, Prashant NM, Pushkarsky T, Mukhamedova N, Fleetwood AJ, et al. Extracellular vesicles carrying HIV-1 Nef induce long-term hyperreactivity of myeloid cells. Cell Rep. (2022) 41:111674. doi: 10.1016/j.celrep.2022.111674
44. Jadhav S, Nema V. HIV-associated neurotoxicity: the interplay of host and viral proteins. Mediators Inflamm. (2021) 2021:1267041. doi: 10.1155/2021/1267041
45. Ellis R, Langford D, Masliah E. HIV and antiretroviral therapy in the brain: neuronal injury and repair. Nat Rev Neurosci. (2007) 8:33–44. doi: 10.1038/nrn2040
46. Ben Haij N, Planes R, Leghmari K, Serrero M, Delobel P, Izopet J, et al. HIV-1 tat protein induces production of proinflammatory cytokines by human dendritic cells and monocytes/macrophages through engagement of TLR4-MD2-CD14 complex and activation of NF-kappaB pathway. PLoS ONE. (2015) 10:e0129425. doi: 10.1371/journal.pone.0129425
47. Hu A, Zaongo SD, Harypursat V, Wang X, Ouyang J, Chen Y. HIV-associated neurocognitive disorder: key implications of the microbiota-gut-brain axis. Front Microbiol. (2024) 15:1428239. doi: 10.3389/fmicb.2024.1428239
48. D'Amico D, Barone R, Di Felice V, Ances B, Prideaux B, Eugenin EA. Chronic brain damage in HIV-infected individuals under antiretroviral therapy is associated with viral reservoirs, sulfatide release, and compromised cell-to-cell communication. Cell Mol Life Sci. (2023) 80:116. doi: 10.1007/s00018-023-04757-0
49. Marcario JK, Manaye KF, SantaCruz KS, Mouton PR, Berman NE, Cheney PD. Severe subcortical degeneration in macaques infected with neurovirulent simian immunodeficiency virus. J Neurovirol. (2004) 10:387–99. doi: 10.1080/13550280490521131
50. Pope TW, Raymond L, Foresman L, Pinson D, Joag SV, Marcario J, et al. Texture analysis of cerebral white matter in SIV-infected macaque monkeys. J Neurosci Methods. (1997) 74:53–64. doi: 10.1016/S0165-0270(97)02257-7
51. Radja F, Kay DG, Albrecht S, Jolicoeur P. Oligodendrocyte-specific expression of human immunodeficiency virus type 1 Nef in transgenic mice leads to vacuolar myelopathy and alters oligodendrocyte phenotype in vitro. J Virol. (2003) 77:11745–53. doi: 10.1128/JVI.77.21.11745-11753.2003
52. Clarke S, Berry N, Ham C, Alden J, Almond N, Ferguson D. Neuropathology of wild-type and Nef-attenuated T cell tropic simian immunodeficiency virus (SIVmac32H) and macrophage tropic neurovirulent SIVmac17E-Fr in cynomolgus macaques. J Neurovirol. (2012) 18:100–12. doi: 10.1007/s13365-012-0084-3
53. Emert-Sedlak LA, Shi H, Tice CM, Chen L, Alvarado JJ, Shu ST, et al. Antiretroviral drug discovery targeting the HIV-1 Nef virulence factor. Viruses. (2022) 14:2025. doi: 10.3390/v14092025
54. Morrow MP, Grant A, Mujawar Z, Dubrovsky L, Pushkarsky T, Kiselyeva Y, et al. Stimulation of the liver X receptor pathway inhibits HIV-1 replication via induction of ATP-binding cassette transporter A1. Mol Pharmacol. (2010) 78:215–25. doi: 10.1124/mol.110.065029
55. Adzhubei AA, Anashkina AA, Tkachev YV, Kravatsky YV, Pushkarsky T, Kulkarni A, et al. Modelling interaction between HIV-1 Nef and calnexin. AIDS. (2018) 32:2103–11. doi: 10.1097/QAD.0000000000001951
56. Vanpouille C, Brichacek B, Pushkarsky T, Dubrovsky L, Fitzgerald W, Mukhamedova N, et al. HIV-1 Nef is carried on the surface of extracellular vesicles. J Extracell Vesicles. (2024) 13:e12478. doi: 10.1002/jev2.12478
57. Smolen JS, Landewe RBM, Bijlsma JWJ, Burmester GR, Dougados M, Kerschbaumer A, et al. EULAR recommendations for the management of rheumatoid arthritis with synthetic and biological disease-modifying antirheumatic drugs: 2019 update. Ann Rheum Dis. (2020) 79:685–99. doi: 10.1136/annrheumdis-2019-216655
58. Weinreich DM, Sivapalasingam S, Norton T, Ali S, Gao H, Bhore R, et al. REGN-COV2, a neutralizing antibody cocktail, in outpatients with COVID-19. N Engl J Med. (2021) 384:238–51. doi: 10.1056/NEJMoa2035002
Keywords: human immunodeficiency virus (HIV), Nef + exosomes, myelin alteration, inflammation, neuroHIV
Citation: Bukrinsky MI (2025) Nef is a key player in neuroinflammation and myelin impairment associated with neuroHIV. Front. Neurol. 16:1553594. doi: 10.3389/fneur.2025.1553594
Received: 31 December 2024; Accepted: 27 February 2025;
Published: 12 March 2025.
Edited by:
Walter Royal III, Morehouse School of Medicine, United StatesReviewed by:
Palsamy Periyasamy, University of Nebraska Medical Center, United StatesCopyright © 2025 Bukrinsky. This is an open-access article distributed under the terms of the Creative Commons Attribution License (CC BY). The use, distribution or reproduction in other forums is permitted, provided the original author(s) and the copyright owner(s) are credited and that the original publication in this journal is cited, in accordance with accepted academic practice. No use, distribution or reproduction is permitted which does not comply with these terms.
*Correspondence: Michael Ilya Bukrinsky, bWJ1a3JpbnNAZ3d1LmVkdQ==
Disclaimer: All claims expressed in this article are solely those of the authors and do not necessarily represent those of their affiliated organizations, or those of the publisher, the editors and the reviewers. Any product that may be evaluated in this article or claim that may be made by its manufacturer is not guaranteed or endorsed by the publisher.
Research integrity at Frontiers
Learn more about the work of our research integrity team to safeguard the quality of each article we publish.