- 1Department of Nephrology, Xuanwu Hospital, Capital Medical University, Beijing, China
- 2Department of Neurology and Innovation Center for Neurological Disorders, Xuanwu Hospital, Capital Medical University, National Center for Neurological Disorders, Beijing, China
- 3Department of Radiology and Nuclear Medicine, Xuanwu Hospital, Capital Medical University, Beijing, China
- 4Department of Radiology, Fuxing Hospital, Capital Medical University, Beijing, China
- 5Beijing Key Laboratory of Magnetic Resonance Imaging and Brain Informatics, Beijing, China
- 6National Clinical Research Center for Geriatric Disorders, Xuanwu Hospital, Capital Medical University, Beijing, China
Background: Cognitive impairment and brain atrophy are common in chronic kidney disease patients. It remains unclear whether differences in renal function, even within normal levels, influence hippocampal volume (HCV) and cognition. We aimed to investigate the association between estimated glomerular filtration rate (eGFR), HCV and cognition in outpatients.
Methods: This single-center retrospective study enrolled 544 nonrenal outpatients from our hospital. All participants underwent renal function assessment and 3.0 T magnetic resonance imaging (MRI) in the same year. HCV was also measured, and cognitive assessments were obtained. The correlations between eGFR, HCV, and cognitive function were analyzed. Logistic regression analysis was performed to identify the risk factors for hippocampal atrophy and cognitive impairment. Receiver-operator curves (ROCs) were performed to find the cut-off value of HCV that predicts cognitive impairment.
Results: The mean age of all participants was 66.5 ± 10.9 years. The mean eGFR of all participants was 88.5 ± 15.1 mL/min/1.73 m2. eGFR was positively correlated with HCV and with Mini-Mental State Examination (MMSE) and Montreal Cognitive Assessment (MoCA) scores. Univariate and multivariate logistic regression analysis showed Age ≥ 65 years, eGFR < 75 mL/min/1.73 m2, Glucose ≥6.1 mmol/L and combined cerebral microvascular diseases were independent risk factors for hippocampal atrophy and Age ≥ 65 years, left hippocampal volume (LHCV) <2,654 mm3 were independent risk factors for cognitive impairment in outpatients. Although initial unadjusted logistic regression analysis indicated that a lower eGFR (eGFR < 75 mL/min/1.73 m2) was associated with poorer cognitive function, this association was lost after adjusting for confounding variables. ROC curve analysis demonstrated that LHCV <2,654 mm3 had the highest AUROC [(0.842, 95% CI: 0.808–0.871)], indicating that LHCV had a credible prognostic value with a high sensitivity and specificity for predicting cognitive impairment compared with age in outpatients.
Conclusion: Higher eGFR was associated with higher HCV and better cognitive function. eGFR < 75 mL/min/1.73 m2 was an independent risk factor for hippocampal atrophy after adjusting for age. It is suggested that even eGFR < 75 mL/min/1.73 m2, lower eGFR may still be associated with hippocampal atrophy, which is further associated with cognitive impairment. LHCV was a favorable prognostic marker for predicting cognitive impairment rather than age.
Introduction
Cognitive impairment is common in chronic kidney disease (CKD) (1, 2), and poor renal function has been associated with cognitive impairment in CKD patients (3). Patients with CKD are significantly more likely to have disproportionate levels of cerebrovascular disease, particularly small-vessel cerebrovascular disease, suggesting that these may be important factors in the development of CKD-related cognitive impairment (4). A study of 1,527 community-based participants showed that kidney function measures are significantly associated with markers of neurodegeneration and small vessel disease visible on brain MRI (5). Current studies believe that CKD-related microvascular injury may be the main factor leading to cognitive impairment (4). There are multifaceted evidence supporting this hypothesis. First, individuals with CKD have a high prevalence of cardiovascular disease (CVD) and CVD risk factors, including diabetes, hypertension, and dyslipidemia, frequently severe enough to lead to renal failure. Second, those with CKD are more susceptible to clinical cerebrovascular disease, including stroke and transient ischemic attack, as well as have subclinical cerebrovascular disease on imaging such as small-vessel infarcts, lacunes, and white matter disease. Third, the cognitive impairment associated with cerebrovascular disease primarily affect processing and executive function, cognitive domains that affect planning and carrying out tasks, and most studies found that processing speed and executive function are the domains most affected in individuals with CKD. Fourth, CVD and its risk factors are associated with worse executive function. Moreover, in earlier stages of CKD, the presence of albuminuria, possible representative of systemic vascular injury, is associated with worse executive function and incident dementia (4). Cognitive impairment in CKD is influenced by multifactor and is accompanied by brain atrophy, including hippocampal atrophy (6, 7). Reduced hippocampal volume (HCV) is associated with late-life cognitive decline (8). HCV is usually defined as an imaging marker of cognitive impairment in general population (8). Most previous studies on the relationship between eGFR and HCV paid attention to patients with clinically significant renal injury (e.g., eGFR < 60 mL/min/1.73 m2) (9, 10). A study found that eGFR < 60 mL/min/1.73 m2 was significantly associated with cortical atrophy in nondisabled adults (11). A three-year observation in elderly diabetic patients found that changes in eGFR during follow-up were independent risk factors for hippocampal atrophy (mean eGFR decreased from 65 mL/min/1.73 m2 to 59 mL/min/1.73 m2) (12). However, is there a relationship between renal function and HCV in nonrenal disease population? Reports about this topic are scarce, and the results are controversial (13, 14). Two studies from the ADNI database came to different conclusions: a cohort study composed of all participants with eGFR > 60 mL/min/1.73 m2 found that higher renal function was associated with slower hippocampal atrophy and cognitive decline even within the normal range of renal function (13). Another cross-sectional study found that a mildly to moderately reduced eGFR (eGFR > 45 mL/min/1.73 m2) was not associated with brain atrophy in ADNI participants (14). Dementia and mild cognitive impairment (MCI) are highly prevalent in China (15). There are fewer studies on the relationship between GFR, HCV, and cognitive function in the real world. In this study, we will explore the association between eGFR, HCV, and cognitive function in outpatients who are not from a renal clinic.
Materials and methods
Study population
This was a cross-sectional study of the majority of outpatients with normal renal function recruited from the nonrenal clinic of Xuanwu Hospital, Capital Medical University. Participants who were undergoing 3.0 T MRI with oblique coronal thin sections oriented perpendicular to the hippocampal long axis were consecutively recruited from Jan 2016 to Dec 2019. The inclusion criteria were as follows: (1) patients with renal function results in the same year (5). The exclusion criteria were as follows: (1) patients with apparent brain lesions, such as cerebral hemorrhage, cerebral infarction, brain tumor, and other brain damage; (2) patients with poor image quality; and (3) patients without MMSE and MoCA scores. A total of 662 outpatients met the inclusion criteria, among whom 118 were excluded from this estimate, including 10 with cerebral hemorrhage, 33 subjects with cerebral infarction, 5 with brain tumors, 7 with other brain damage, 24 with poor image quality, 21 without MMSE and MoCA scores and 18 with missing clinical data. Thus, a total of 544 subjects were recruited (Figure 1). Among them, 325 cases were diagnosed with cognitive impairment, including 8 cases were diagnosed with AD, 31 cases were diagnosed with dementia, 17 cases were diagnosed with vascular dementia. 219 cases were cognitively normal.
Clinical characteristics and laboratory data
Patient characteristics included age, sex, clinical diagnosis, MRI diagnosis, and laboratory data including serum urea, serum creatinine, total protein, albumin, and serum uric acid (SUA), glucose, total cholesterol, low density lipoprotein, triglyceride, homocysteine, potassium, phosphate, alanine aminotransferase (ALT), leukocytes, hemoglobin, platelets. Serum creatinine concentrations were measured by an automatic biochemical analyzer (BioTek Instrument, Inc., Beijing, China) and used to compute eGFR based on the CKD-Epidemiology Collaboration (CKD-EPI) equation (16). We chose the CKD-EPI equation as it is more accurate in the elderly and at higher levels of kidney function (eGFR > 60 mL/min/1.73 m2) and is a better predictor of mortality when compared to the Modification of Diet in Renal Disease Study equation (17–19). The CKD-EPI equation (16) was calculated as recommended: For women with a plasma creatinine ≤0.7 mg/dL,(plasma creatinine/0.7)−0.329 × (0.993)age (×166 if black; ×144 if white or other); for women with a plasma creatinine >0.7 mg/dL, (plasma creatinine/0.7)−1.209 × (0.993)age (×166 if black; ×144 if white or other); for men with a plasma creatinine ≤0.9 mg/dL, (plasma creatinine/0.9)−0.411 × (0.993)age (×163 if black; ×141 if white or other),for men with a plasma creatinine >0.9 mg/dL, (plasma creatinine/0.9)−1.209 (×0.993)age (×163 if black; ×141 if white or other).
MRI acquisition and analysis
All MR images were obtained on a 3.0 T MR scanner (GE DISCOVERY 750, America) with a 20-channel coil. All participants underwent MRI with oblique coronal thin sections oriented perpendicular to the hippocampal long axis. The scanning parameters were as follows: For Cor T1 FSPGR scanning, we used Fast Spoiled Gradient Recalled sequence, repetition time (TR) = 115 ms, echo time (TE): 2.1 ms, Flip Angle:70°, layer thickness = 3 mm, matrix size = 2,256 × 192, and layer number = 23.
All MRI images were evaluated by two experienced radiologists to exclude patients with obvious brain lesions and poor image quality.
Hippocampal volume calculation
T1-weighted structural images were analyzed for the measurement of hippocampal volume. First, we manually segmented the hippocampus and identified the boundaries of the hippocampus through the sagittal and coronal plane and drew an outline of the structure of the hippocampus (20, 21). The critical point of hippocampal volume measurement is to accurately calibrate the oblique coronal section perpendicular to the long axis of the hippocampus and the oblique coronal section from the head to the tail of the hippocampus as the main measurement section (20, 21). Then, the ITK-SNAP software was used to delineate the boundary of the hippocampal structure, with oblique coronal images serving as the reference level and horizontal and sagittal images aiding in boundary identification (Figure 2). Subsequently, the software automatically computed the volume of the hippocampus (22). Finally, we computed the intracranial volume (ICV) and obtained standardization via dividing hippocampal volume by total brain volume (23). Two proficient investigators independently measured bilateral hippocampal volumes and cross-validated their findings.
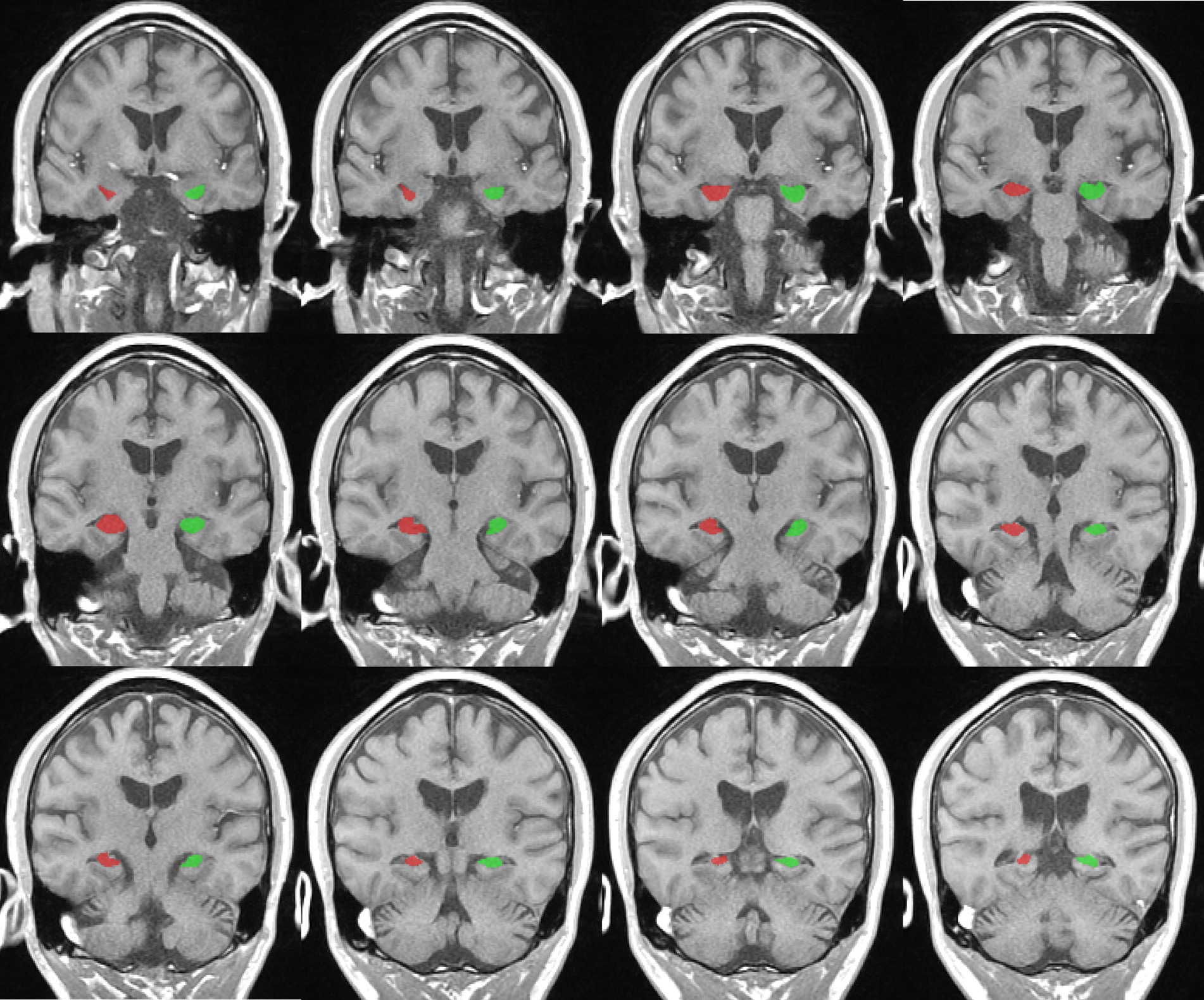
Figure 2. Representative example of right (red) and left (green) hippocampus segmentation and volume calculation on T1-weighted images in ITK-snap software.
Cognitive function
All patients had a medical history in the outpatient department of neurology, and MMSE and MoCA scores were recorded. MoCA score < 26 was defined as cognitive impairment (24).
Cerebral microvascular diseases
The presence of cerebral microvascular diseases was defined as microbleeds, microinfarcts, vascular spaces, and increased white matter lesions in MRI reports.
Statistical analysis
Statistical analysis was done by using SPSS version 23.0 (IBM) and Med Calc version 20.116. The Kolmogorov–Smirnov test was used to evaluate the distribution of the variables. For continuous variables, the data was expressed as mean ± SD or median (interquartile range). Categorical variables were displayed as percentages or ratios. The Student t-test, Mann–Whitney U test or Chi-square test was performed for comparisons between variables at the right time. Spearman correlation analysis was performed between eGFR and HCV as well as eGFR and cognitive function. ROCs were utilized to evaluate the predictive sensitivity and specificity of different markers for cognitive impairment, and the areas under the curves (AUCs) were computed. All analyses were two-tailed, and statistical significance was accepted at p < 0.05.
Results
Comparison of clinical characteristics and HCV between hippocampal atrophy patients and nonhippocampal atrophy patients
MTA visual scale was used to make the imaging diagnosis of hippocampal atrophy in the Radiology department of our hospital. A total of 544 participants were divided into two groups: 226 in the hippocampal atrophy group and 318 in the nonhippocampal atrophy group. The baseline demographic and clinical characteristics are shown in Table 1. There were no significant differences in sex, combined hypertension, combined diabetes, total protein, SUA, total cholsterol, low density lipoprotein, triglycerides, potassium, phosphate, leukocytes, platelet counts between the two groups. Compared with patients in the nonhippocampal atrophy group, patients in the hippocampal atrophy group were older [70.8 ± 9.6 years vs. 62.4 ± 10.8 years, (p < 0.001)], and were vulnerable to cerebral microvascular diseases [67.3% vs. 33.6%, (p < 0.001)]. In terms of laboratory findings, patients in the hippocampal atrophy group had higher levels of serum urea, serum creatinine, glucose, and homocysteine, but lower levels of eGFR, albumin, ALT and hemoglobin. Notably, mean eGFR of both groups were within the normal levels [84.7 ± 15.3 mL/min/1.73 m2 vs. 91.2 ± 14.4 mL/min/1.73 m2, (p < 0.001)].
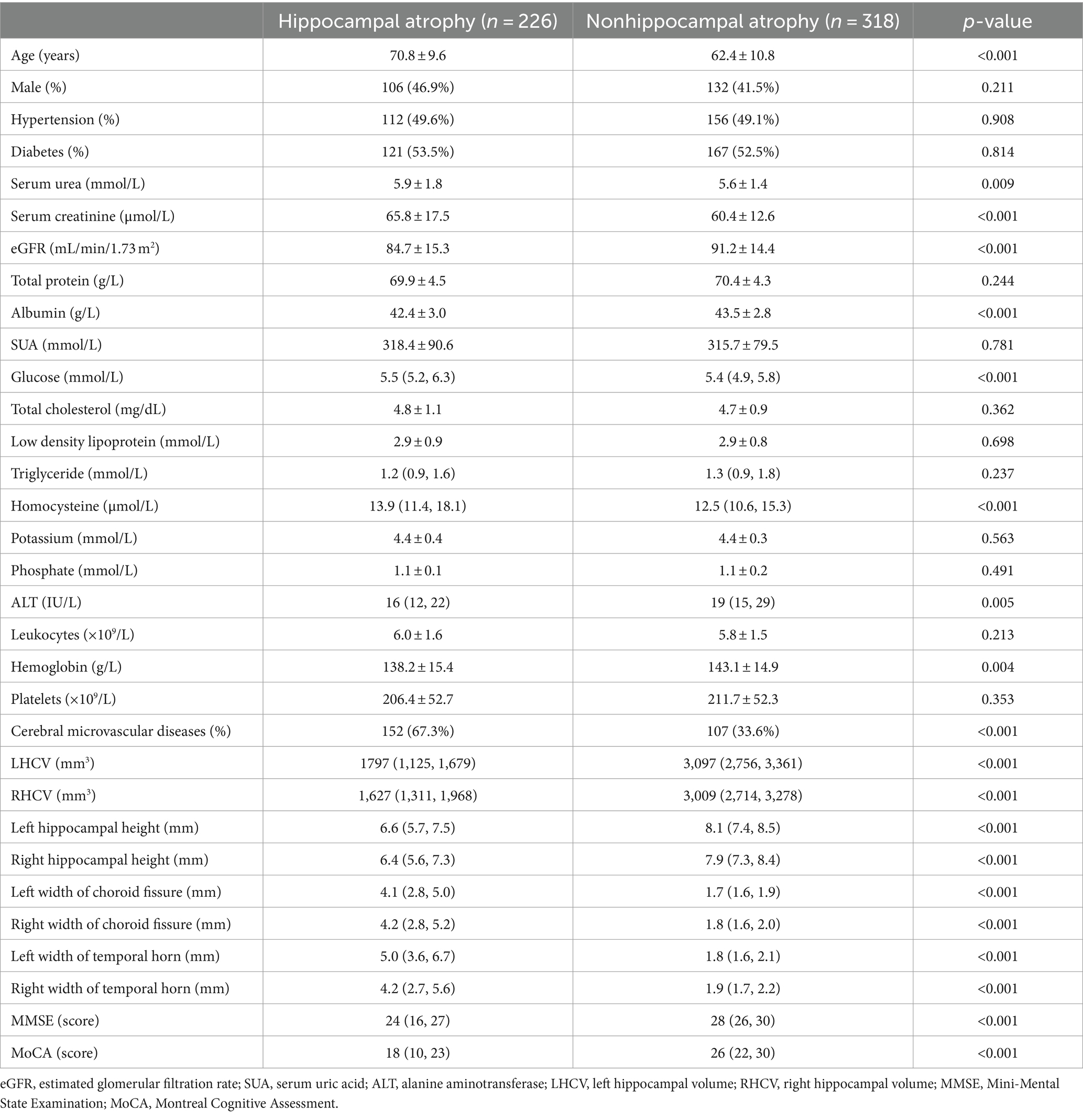
Table 1. Comparison of baseline demographic, clinical characteristics, and combined cerebral microvascular diseases between hippocampal atrophy patients and nonhippocampal atrophy patients.
Risk factors for hippocampal atrophy
Univariate logistic regression demonstrated that age ≥ 65 years, eGFR < 75 mL/min/1.73 m2, Glucose≥6.1 mmol/L, homocysteine≥15 μmol/L, combined cerebral microvascular diseases were the risk factors for hippocampal atrophy; whereas multivariate logistic regression analysis showed that age ≥ 65 years, eGFR < 75 mL/min/1.73 m2, Glucose≥6.1 mmol/L and combined cerebral microvascular diseases were independent risk factors for hippocampal atrophy (Table 2).
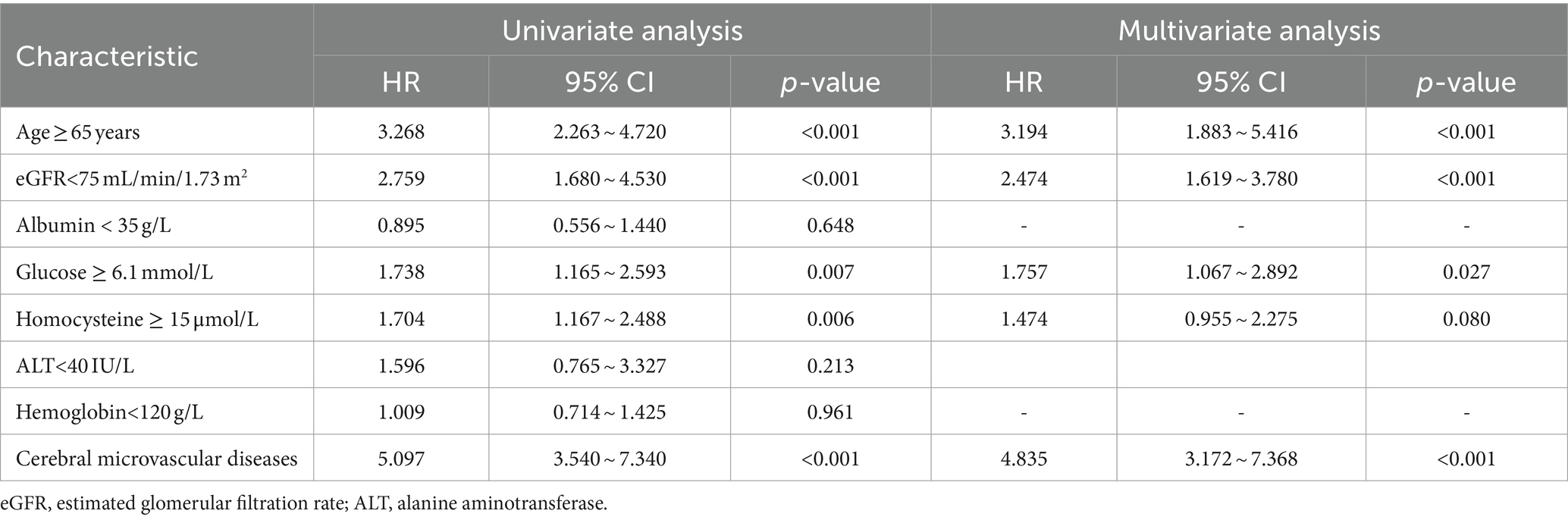
Table 2. Univariate analysis and multivariate analysis of prognostic factors for hippocampal atrophy.
Comparison of clinical characteristics and HCV between cognitive impairment patients and cognitively normal patients
MoCA score < 26 was defined as the threshold of cognitive impairment and 544 outpatients were divided into two groups: the cognitive impairment group and cognitively normal group. As shown in Table 3, patients with cognitive impairment had lower eGFR than patients without cognitive impairment[87.3 ± 12.7 mL/min/1.73 m2 vs. 92.2 ± 15.3 mL/min/1.73 m2, (p < 0.001)]. Patients with cognitive impairment had significantly smaller HCV, lower hippocampal height, wider choroid fissure and temporal horn than patients without cognitive impairment (p < 0.001); they showed older age and lower albumin level than those without cognitive impairment (p < 0.001). There were significant differences in SUA, glucose, triglyceride, homocysteine, ALT and hemoglobin between the two groups (p < 0.05). There were more patients with cerebral microvascular diseases in the cognitive impairment group than in the cognitively normal group (p < 0.05). There were no significant differences in sex, combined hypertension, combined diabetes, serum urea, serum creatinine, total protein, total cholesterol, low density lipoprotein, potassium, phosphate, leukocytes and platelets between the two groups (Table 3).
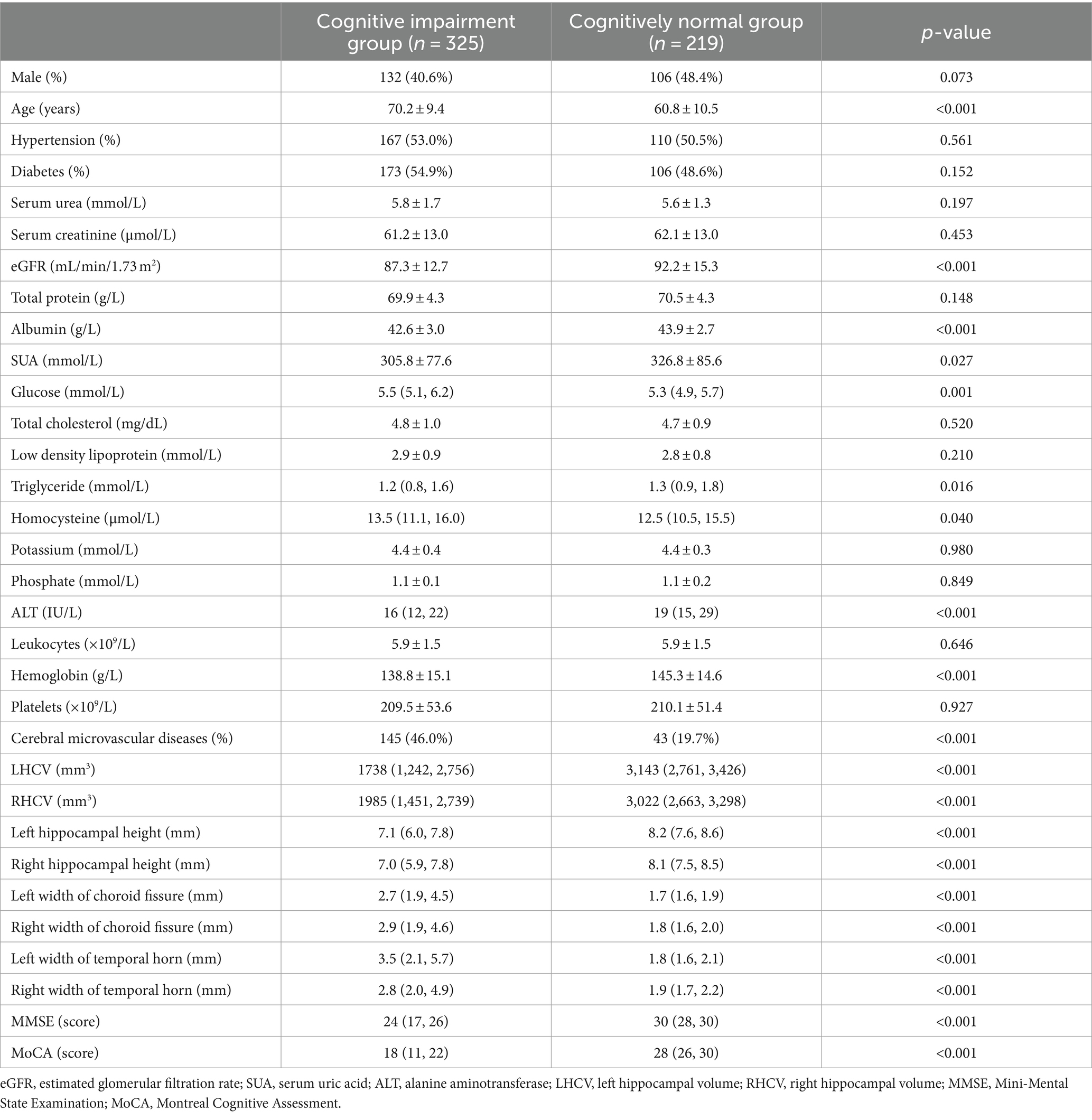
Table 3. Comparison of baseline demographic, clinical characteristics, combined cerebral microvascular diseases, and hippocampal measurements between cognitive impairment patients and cognitively normal patients.
Association between eGFR and HCV with cognitive function
HCV were measured and cognitive assessment were obtained in 544 patients. The medians of LHCV and right hippocampal volume (RHCV) were 2,587 mm3 and 2,600 mm3, respectively. eGFR was positively correlated with HCV and cognitive function (Table 4; Figure 3).
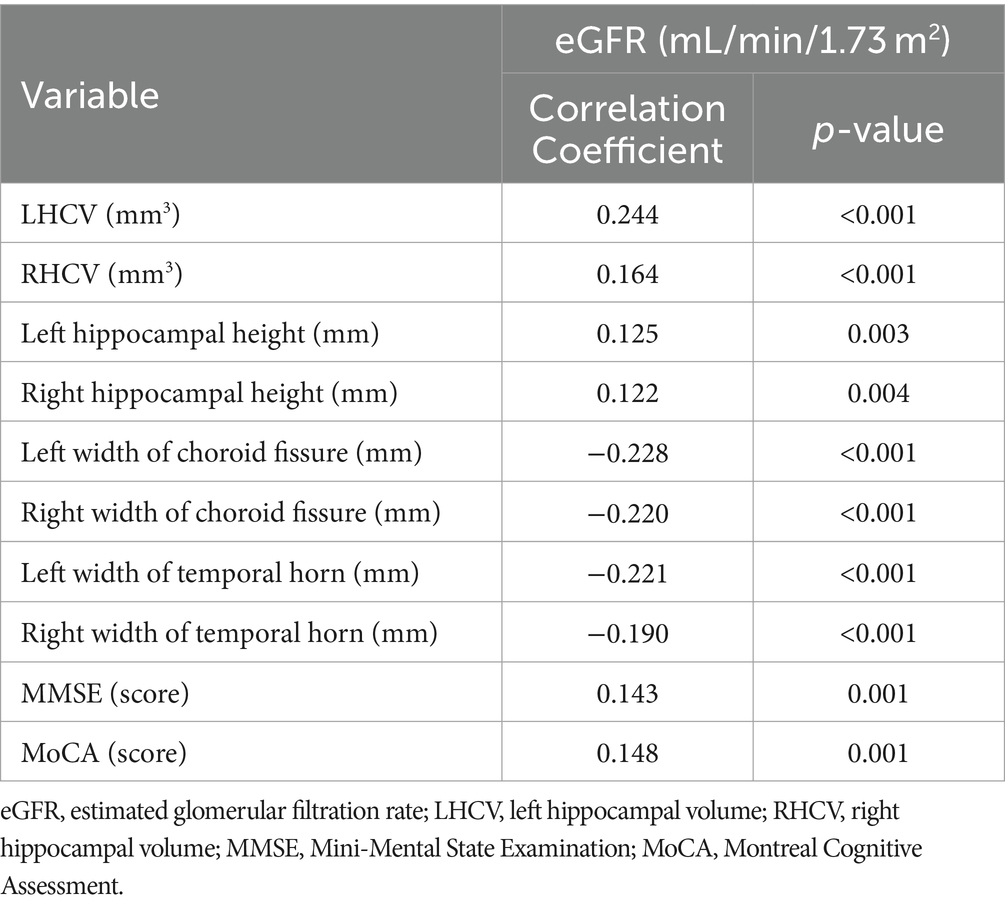
Table 4. Spearman correlation analysis of eGFR with hippocampal measurements and cognitive function.
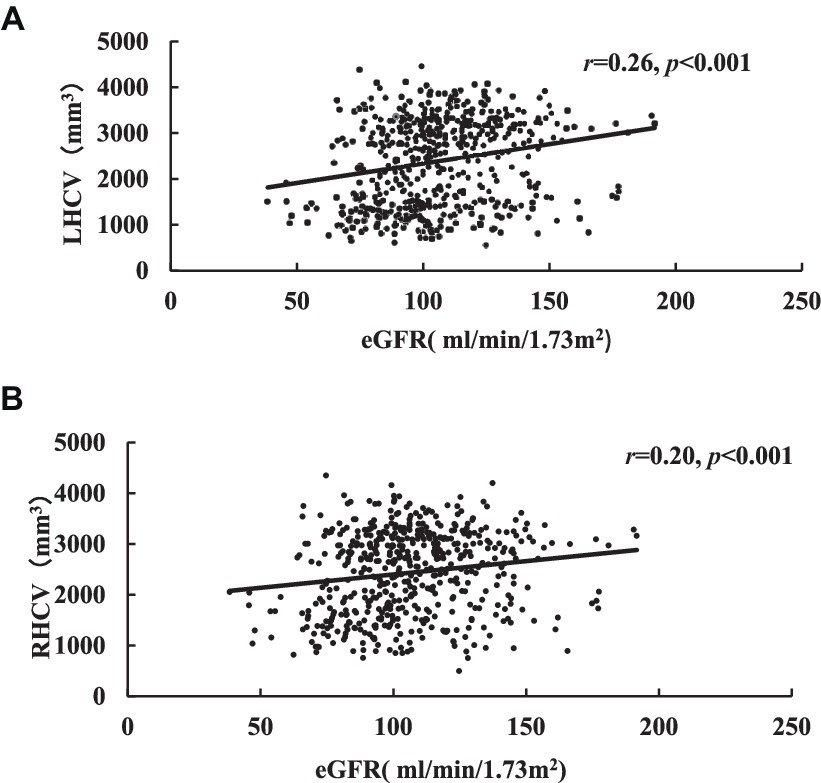
Figure 3. Correlation analysis of eGFR related to LHCV (A) and RHCV (B). eGFR, estimated glomerular filtration rate; LHCV, left hippocampal volume; RHCV, right hippocampal volume.
Risk factors for cognitive impairment
Although univariate logistic regression suggested that eGFR < 75 mL/min/1.73 m2, glucose ≥6.1 mmol/L, combined cerebral microvascular diseases, LHCV < 2,654 mm3 and RHCV < 2,225 mm3 were risk factors for cognitive impairment, but multivariate logistic regression analysis showed that age ≥ 65 years, LHCV < 2,654 mm3 were independent risk factors for cognitive impairment (Table 5).
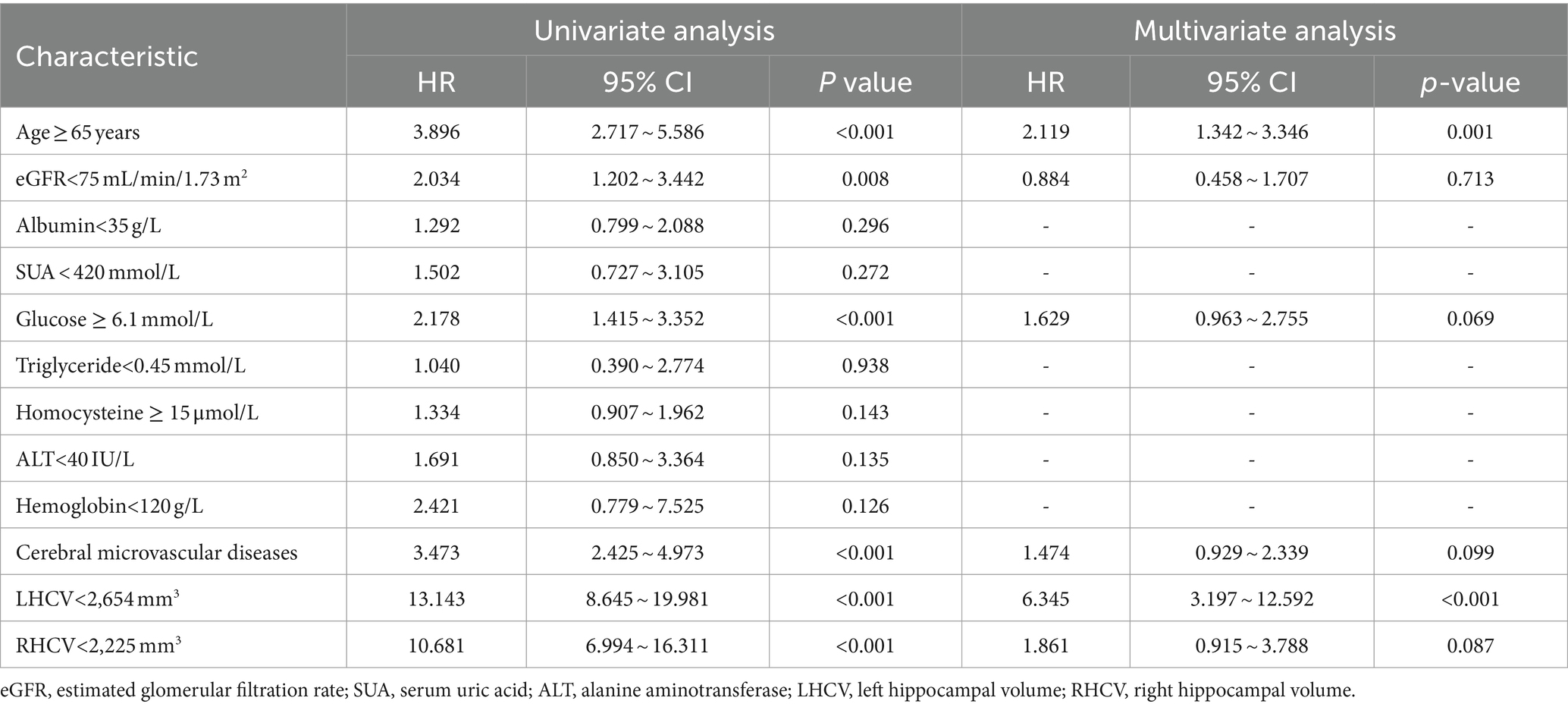
Table 5. Univariate analysis and multivariate analysis of prognostic factors for cognitive impairment patients.
Predictive accuracy of different markers for cognitive impairment in outpatients
In Figure 4, ROCs were obtained from the risk factors that were statistically significant in our previous analysis. LHCV showed the largest AUC of 0.842 and demonstrated more significant diagnostic value than age (p < 0.001). The optimal LHCV threshold was 2,654 mm3 for predicting cognitive impairment in outpatients.
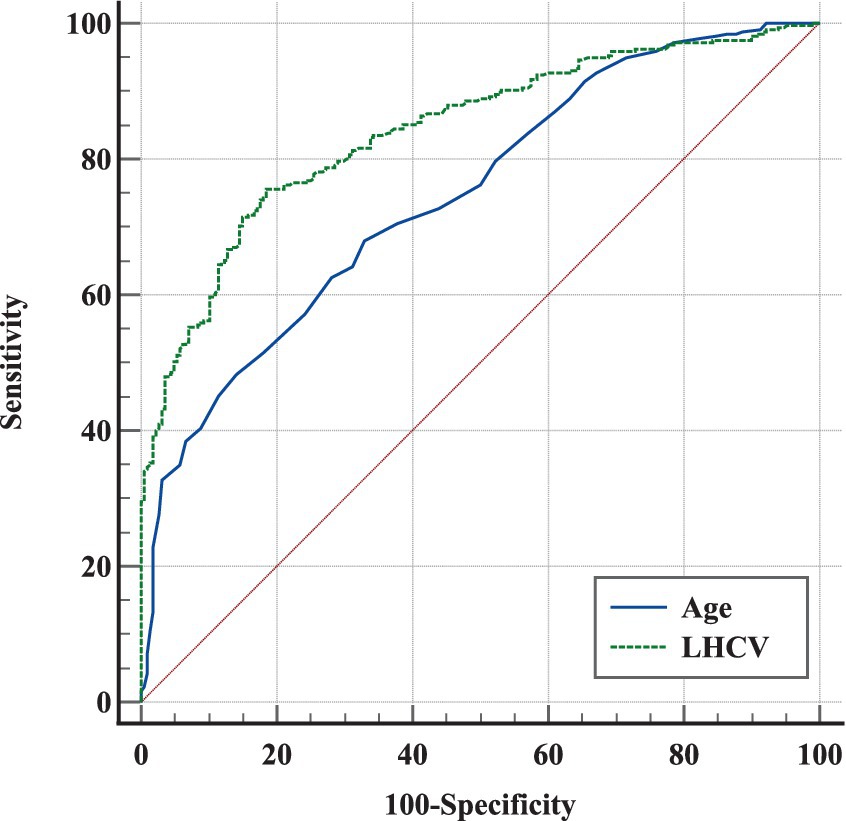
Figure 4. ROC analysis of different markers in predicting cognitive impairment in outpatients. The ROCs of different markers showed that LHCV had the largest AUC and a credible prognostic value with a high sensitivity and specificity for predicting cognitive impairment in outpatients. LHCV, left hippocampal volume.
Discussion
In contrast to other studies, our study was a retrospective cross-sectional study of the relationship between renal function, HCV, and cognitive function in outpatients who were not from the nephrology department. We found that eGFR was positively correlated with HCV and cognitive function. The results are consistent with other studies (9, 25). We found that eGFR <75 mL/min/1.73 m2 was an independent risk factor for hippocampal atrophy. Although univariate logistic regression suggested that eGFR <75 mL/min/1.73 m2 was a risk factor for cognitive impairment, the link disappeared after multivariate analysis. Our study found that a smaller LHCV was an independent risk factor for cognitive impairment. LHCV <2,654 mm3 can predict cognitive impairment in outpatients (Figure 4).
HCV is a recognized imaging marker of cognitive dysfunction (8). The faster volume loss of the hippocampus suggested a higher risk of disease progression in mild cognitive impairment patients (26). Compared with the normal population, patients with CKD frequently had hippocampal atrophy (9), The possible cause is the damage of hippocampal cells caused by uraemic toxin (27). But, most previous studies concerned about participants with eGFR <60 mL/min/1.73 m2. However, in the majority participants with normal renal function, there are few studies on the relationship between renal function, HCV and cognitive function (12–14).
We found that eGFR was positively correlated with HCV and cognitive function, which was consistent with most studies (9, 28). We found age ≥ 65 years was an independent risk factor for cognitive impairment and hippocampal atrophy. The strong association between age and brain volume as well as cognitive function is not unexpected (29). We found hyperglycemia was an independent risk factor for hippocampal atrophy, which was similar to other studies (30, 31). Additionally, we also found combined cerebral microvascular diseases was another independent risk factor for hippocampal atrophy (32).
In our current study, we found that eGFR <75 mL/min/1.73 m2 was an independent risk factor for hippocampal atrophy although it is within defined normal renal function after adjusting for age. Studies on the relationship between eGFR and HCV in people within normal renal function are few and results are controversial (13, 14). For example, two studies from the same database (ADNI) at different times produced two different conclusions: An H, et al. reported that a longitudinal study including 1,269 patients with eGFR >60 mL/min/1.73 m2 found that higher renal function was associated with slower cognitive decline and hippocampal atrophy even within the range of normal renal function (13). However, another cross-sectional study including 1,596 patients (73% of patients with eGFR >60 mL/min/1.73 m2) found that a mild to moderately reduced eGFR (eGFR >45 mL/min/1.73 m2) was not associated with hippocampal atrophy (14). However, even in the study (14) also found that eGFR >90 mL/min 1.73 m2 was associated with larger brain volumes including HCV. A decline in eGFR accelerated age-related changes in brain volume (33). Only 2.0% of the patients in our study with GFR < 60 mL/min/1.73 m2, and the basic composition was similar to An. H’s study (13).
Although the mechanisms of the pathological association between kidney function and hippocampal atrophy remain unclear, several hypotheses might explain this correlation. First, a path analysis model suggested that vascular load play an important role in hippocampal atrophy even within normal ranges of renal function (13). Additionally, vascular burden induced amyloid deposition, which also linked with hippocampal atrophy (13). Most participants in our study had hypertension, diabetes or both diseases, which could generate vascular burden (34). A reasonable explanation is endothelial injury and neuroinflammation (35). Second, GFR estimations based on serum creatinine may be affected by muscle mass, creatine dietary supplements, or meat-based diet, and older people are more likely to have less than average muscle mass due to chronic disease, leading to low creatinine levels and underlying overestimation of GFR. Finally, albuminuria is an important risk factor for the development of both AD and mild cognitive impairment (MCI) (36). Moreover, albuminuria was also associated with temporal lobe atrophy and cortical thinning, independent of eGFR (37, 38). The mechanisms by which albuminuria is associated with brain atrophy may simply represent systemic endothelial dysfunction (39). Because most participants in our study were not from renal clinics, most of them lacked urinary albumin/creatinine ratio (UACR) data, and the prevalence of CKD may have been greatly underestimated.
We did not find eGFR <75 mL/min/1.73 m2 to be an independent risk factor for cognitive impairment although eGFR <75 mL/min/1.73 m2 was a risk factor for hippocampal atrophy. The possible reasons are as follows: First, the eGFR of the participants in our study is higher than other studies; second, hippocampal atrophy may precede cognitive impairment in the progression of the disease. Maybe it needs time for hippocampal atrophy to develop into cognitive impairment, and perhaps further follow-up will see the relationship between them.
Our study found LHCV <2,654 mm3 not RHCV was an independent risk factor for cognitive impairment and had the largest ROC value in ROC curve compared with age (Figure 4). HCV is a strong predictor of memory decline in MCI (40). A study found the involvement of white matter hyperintensities (WMH) and medial temporal lobe atrophy (MTA) were indicatives of AD pathology in cognitive dysfunction (41). So our study included white matter lesions as a covariate in the analysis of cognitive impairment and still found that decreased hippocampal volume was an independent risk factor for cognitive impairment. Wolf et al. (42) also found left-sided and posterior hippocampal measures were more responsible for cognitive impairment discrimination than right-sided and anterior measures. A study suggested that an increased APOE ɛ4 dose is associated with decreased effective brain-waste clearance, such as iron and β-amyloid, worsening the pathology of AD (43). These results suggest that APOE ε4 allele may lead to left hippocampal atrophy in patients with early-onset mild cognitive impairment, and the atrophy in some hippocampal subregions is more obvious (44). Logistic regression revealed that left hippocampal subiculum volume was a significant predictor of MCI conversion (45). Our study was in consistence with them. However, the cut-off value of LHCV was lower than other studies in China (46), may related to larger proportion of the elderly in our study.
This study has several strengths. There are few studies investigating the association of renal function, HCV, and cognitive function, especially in patients with normal renal function. Our study was a scarce clinical research in the nonrenal clinic population and had relatively large samples of nonrenal outpatients. Our results show that when eGFR <75 mL/min/1.73 m2, hippocampal atrophy may occur. Additionally, measurement of HCV provides a threshold value for the diagnosis of cognitive impairment, which is conducive to further research in the future.
Our study also had some limitations. First, due to the cross-sectional nature of this study, a future study with a larger cohort size is needed to determine causality or whether there is a causal relationship. Second, our hospital is a tertiary hospital with neurology as its dominant discipline. The participants were mostly from the outpatient department of neurology, which may have led to selection bias. Finally, the UACR test is lacking, which is another marker of kidney function in most outpatients in our study.
Conclusion
We demonstrated that lower eGFR, even within normal renal function, are associated with HCV and cognition. LHCV <2,654 mm3 can predict cognitive impairment in outpatients.
Data availability statement
The original contributions presented in the study are included in the article/supplementary material, further inquiries can be directed to the corresponding author.
Ethics statement
The Research Ethics Boards at Xuanwu Hospital of Capital Medical University approved the study protocol (approval number: CTR-IPR-2019130). The studies were conducted in accordance with the local legislation and institutional requirements. Written informed consent for participation in this study was provided by the participants’ legal guardians/next of kin.
Author contributions
L-YW: Data curation, Funding acquisition, Investigation, Software, Writing – original draft. Y-YL: Conceptualization, Formal analysis, Investigation, Methodology, Project administration, Supervision, Writing – review & editing. S-SZ: Data curation, Methodology, Project administration, Resources, Supervision, Validation, Writing – review & editing. Y-DC: Data curation, Formal analysis, Methodology, Project administration, Supervision, Writing – review & editing. JL: Supervision, Validation, Visualization, Writing – review & editing. A-HZ: Methodology, Supervision, Validation, Visualization, Writing – review & editing.
Funding
The author(s) declare that financial support was received for the research, authorship, and/or publication of this article. This study was supported by Xuanwu Hospital Science Program for Fostering Young Scholars (QNPY2020019).
Acknowledgments
The authors thank the participants for their willingness to contribute to the present work.
Conflict of interest
The authors declare that this study was conducted in the absence of any commercial or financial relationships that could be interpreted as a potential conflict of interest.
The author(s) declared that they were an editorial board member of Frontiers, at the time of submission. This had no impact on the peer review process and the final decision.
Publisher’s note
All claims expressed in this article are solely those of the authors and do not necessarily represent those of their affiliated organizations, or those of the publisher, the editors and the reviewers. Any product that may be evaluated in this article, or claim that may be made by its manufacturer, is not guaranteed or endorsed by the publisher.
References
1. Kurella, MCG, Fried, LF, Cummings, SR, Harris, T, Simonsick, E, Satterfield, S, et al. Chronic kidney disease and cognitive impairment in the elderly: the health, aging, and body composition study. J Am Soc Nephrol. (2005) 16:2127–33. doi: 10.1681/ASN.2005010005
2. Etgen, TCM, Forstl, H, and Sander, D. Chronic kidney disease and cognitive impairment: a systematic review and meta-analysis. Am J Nephrol. (2012) 35:474–82. doi: 10.1159/000338135
3. Yaffe, K, Ackerson, L, Kurella Tamura, M, Le Blanc, P, Kusek, JW, Sehgal, AR, et al. Chronic renal insufficiency cohort I. Chronic kidney disease and cognitive function in older adults: findings from the chronic renal insufficiency cohort cognitive study. J Am Geriatr Soc. (2010) 58:338–45. doi: 10.1111/j.1532-5415.2009.02670.x
4. Drew, DA, Weiner, DE, and Sarnak, MJ. Cognitive impairment in CKD: pathophysiology, management, and prevention. Am J Kidney Dis. (2019) 74:782–90. doi: 10.1053/j.ajkd.2019.05.017
5. Scheppach, JB, Wu, A, Gottesman, RF, Mosley, TH, Arsiwala-Scheppach, LT, Knopman, DS, et al. Association of Kidney Function Measures with Signs of neurodegeneration and small vessel disease on brain magnetic resonance imaging: the atherosclerosis risk in communities (ARIC) study. Am J Kidney Dis. (2023) 81:261–269.e1. doi: 10.1053/j.ajkd.2022.07.013
6. Hartung, EA, Erus, G, Jawad, AF, Laney, N, Doshi, JJ, Hooper, SR, et al. Brain magnetic resonance imaging findings in children and young adults with CKD. Am J Kidney Dis. (2018) 72:349–59. doi: 10.1053/j.ajkd.2017.11.024
7. Tsuruya, K, and Yoshida, H. Brain atrophy and cognitive impairment in chronic kidney disease. Contrib Nephrol. (2018) 196:27–36. doi: 10.1159/000485694
8. Dawe, RJ, Yu, L, Arfanakis, K, Schneider, JA, Bennett, DA, and Boyle, PA. Late-life cognitive decline is associated with hippocampal volume, above and beyond its associations with traditional neuropathologic indices. Alzheimers Dement. (2020) 16:209–18. doi: 10.1002/alz.12009
9. Chang, CYLC, Tsai, CF, Yang, WC, Wang, SJ, Lin, FH, and Fuh, JL. Cognitive impairment and hippocampal atrophy in chronic kidney disease. Acta Neurol Scand. (2017) 136:477–85. doi: 10.1111/ane.12753
10. Auriel, EKE, Shenhar-Tsarfaty, S, Molad, J, Berliner, S, Shapira, I, Ben-Bashat, D, et al. Impaired renal function is associated with brain atrophy and poststroke cognitive decline. Neurology. (2016) 86:1996–2005. doi: 10.1212/WNL.0000000000002699
11. Yakushiji, Y, Nanri, Y, Hirotsu, T, Nishihara, M, Hara, M, Nakajima, J, et al. Marked cerebral atrophy is correlated with kidney dysfunction in nondisabled adults. Hypertens Res. (2010) 33:1232–7. doi: 10.1038/hr.2010.171
12. Kawamura, T, Umemura, T, Umegaki, H, Imamine, R, Kawano, N, Mase, H, et al. Factors associated with changes in brain atrophy during a three-year observation in elderly diabetic patients: effect of renal impairment on hippocampal atrophy. Dement Geriatr Cogn Dis Extra. (2016) 6:55–67. doi: 10.1159/000443497
13. An, H, Choi, B, Son, SJ, Cho, EY, Kim, SO, Cho, S, et al. Alzheimer's disease neuroimaging I. Renal function affects hippocampal volume and cognition: the role of vascular burden and amyloid deposition. Geriatr Gerontol Int. (2017) 17:1899–906. doi: 10.1111/ggi.12985
14. Grasing, M, Sharma, P, Lepping, RJ, Honea, R, Burns, JM, Brooks, WM, et al. Association between the estimated glomerular filtration rate and brain atrophy in older adults. Am J Nephrol. (2022) 53:176–81. doi: 10.1159/000521892
15. Jia, L, Du, Y, Chu, L, Zhang, Z, Li, F, Lyu, D, et al. Prevalence, risk factors, and management of dementia and mild cognitive impairment in adults aged 60 years or older in China: a cross-sectional study. Lancet Public Health. (2020) 5:e661–71. doi: 10.1016/S2468-2667(20)30185-7
16. Andrew, S, Levey, LAS, Schmid, CH, Zhang, YL, Castro 3rd, AF, Feldman, HI, et al. CKD-EPI (chronic kidney disease epidemiology collaboration). A new equation to estimate glomerular filtration rate. Ann Intern Med. (2009) 150:604–12. doi: 10.7326/0003-4819-150-9-200905050-00006
17. Kilbride, HS, Stevens, PE, Eaglestone, G, Knight, S, Carter, JL, Delaney, MP, et al. Accuracy of the MDRD (modification of diet in renal disease) study and CKD-EPI (CKD epidemiology collaboration) equations for estimation of GFR in the elderly. Am J Kidney Dis. (2013) 61:57–66. doi: 10.1053/j.ajkd.2012.06.016
18. Stevens, LA, Schmid, CH, Greene, T, Zhang, Y(L), Beck, GJ, Froissart, M, et al. Comparative performance of the CKD epidemiology collaboration (CKD-EPI) and the modification of diet in renal disease (MDRD) study equations for estimating GFR levels above 60 mL/min/1.73 m2. Am J Kidney Dis. (2010) 56:486–95. doi: 10.1053/j.ajkd.2010.03.026
19. Shafi, T, Matsushita, K, Selvin, E, Sang, Y, Astor, BC, Inker, LA, et al. Comparing the association of GFR estimated by the CKD-EPI and MDRD study equations and mortality: the third national health and nutrition examination survey (NHANES III). BMC Nephrol. (2012) 13:42. doi: 10.1186/1471-2369-13-42
20. Pruessner, JC, Collins, DL, Pruessner, M, and Evans, AC. Age and gender predict volume decline in the anterior and posterior hippocampus in early adulthood. J Neurosci. (2001) 21:194–200. doi: 10.1523/JNEUROSCI.21-01-00194.2001
21. Pruessner, JC, Li, LM, Serles, W, Pruessner, M, Collins, DL, Kabani, N, et al. Volumetry of hippocampus and amygdala with high-resolution MRI and three-dimensional analysis software: minimizing the discrepancies between laboratories. Cereb Cortex. (2000) 10:433–42. doi: 10.1093/cercor/10.4.433
22. Yushkevich, PA, and Gerig, G. ITK-SNAP: An Intractive medical image segmentation tool to meet the need for expert-guided segmentation of complex medical images. IEEE Pulse. (2017) 8:54–7. doi: 10.1109/MPUL.2017.2701493
23. Free, SL, Bergin, PS, Fish, DR, Cook, MJ, Shorvon, SD, and Stevens, JM. Methods for normalization of hippocampal volumes measured with MR. AJNR Am J Neuroradiol. (1995) 16:637–43.
24. Mai, LM, Oczkowski, W, Mackenzie, G, Shuster, A, Wasielesky, L, Franchetto, A, et al. Screening for cognitive impairment in a stroke prevention clinic using the MoCA. Can J Neurol Sci. (2013) 40:192–7. doi: 10.1017/S031716710001372X
25. Oh, YS, Kim, JS, Park, JW, An, JY, Park, SK, Shim, YS, et al. Arterial stiffness and impaired renal function in patients with Alzheimer's disease. Neurol Sci. (2016) 37:451–7. doi: 10.1007/s10072-015-2434-4
26. Zeng, Q, Li, K, Luo, X, Wang, S, Xu, X, Li, Z, et al. The Alzheimer's disease neuroimaging I. Distinct atrophy pattern of hippocampal subfields in patients with progressive and stable mild cognitive impairment: a longitudinal MRI study. J Alzheimers Dis. (2021) 79:237–47. doi: 10.3233/JAD-200775
27. Watanabe, K, Sato, E, Mishima, E, Watanabe, M, Abe, T, Takahashi, N, et al. Effect of uremic toxins on hippocampal cell damage: analysis in vitro and in rat model of chronic kidney disease. Heliyon. (2021) 7:e06221. doi: 10.1016/j.heliyon.2021.e06221
28. Ben Assayag, E, Eldor, R, Korczyn, AD, Kliper, E, Shenhar-Tsarfaty, S, Tene, O, et al. Type 2 diabetes mellitus and impaired renal function are associated with brain alterations and Poststroke cognitive decline. Stroke. (2017) 48:2368–74. doi: 10.1161/STROKEAHA.117.017709
29. Gupta, A, and Burns, JM. A single point-in-time eGFR is not associated with increased risk of dementia in the elderly. J Am Soc Nephrol. (2020) 31:2965. doi: 10.1681/ASN.2020081119
30. Hirabayashi, N, Hata, J, Ohara, T, Mukai, N, Nagata, M, Shibata, M, et al. Association between diabetes and hippocampal atrophy in elderly Japanese: the Hisayama study. Diabetes Care. (2016) 39:1543–9. doi: 10.2337/dc15-2800
31. Bao, C, Liu, X, Li, Y, Yang, J, Wei, F, Gong, Y, et al. Region-specific hippocampal atrophy is correlated with poor glycemic control in type 2 diabetes: a cross-sectional study. Endocr J. (2023) 70:1131–40. doi: 10.1507/endocrj.EJ23-0211
32. Kril, JJ, Patel, S, Harding, AJ, and Halliday, GM. Patients with vascular dementia due to microvascular pathology have significant hippocampal neuronal loss. J Neurol Neurosurg Psychiatry. (2002) 72:747–51. doi: 10.1136/jnnp.72.6.747
33. Freedman, BI, Sink, KM, Hugenschmidt, CE, Hughes, TM, Williamson, JD, Whitlow, CT, et al. Associations of early kidney disease with brain magnetic resonance imaging and cognitive function in African Americans with type 2 diabetes mellitus. Am J Kidney Dis. (2017) 70:627–37. doi: 10.1053/j.ajkd.2017.05.006
34. Georgakis, MK, Ntanasi, E, Ramirez, A, Grenier-Boley, B, Lambert, J-C, Sakka, P, et al. Vascular burden and genetic risk in association with cognitive performance and dementia in a population-based study. Cereb Circ Cogn Behav. (2022) 3:100145. doi: 10.1016/j.cccb.2022.100145
35. Miranda, AS, Cordeiro, TM, Soares, TMDSL, Ferreira, RN, and Silva, ACSE. Kidney-brain axis inflammatory cross-talk: from bench to bedside. Clin Sci (Lond). (2017) 131:1093–105. doi: 10.1042/CS20160927
36. Takae, K, Hata, J, Ohara, T, Yoshida, D, Shibata, M, Mukai, N, et al. Albuminuria increases the risks for both Alzheimer disease and vascular dementia in community-dwelling Japanese elderly: the Hisayama study. J Am Heart Assoc. (2018) 7:6693. doi: 10.1161/JAHA.117.006693
37. Vemuri, P, Knopman, DS, Jack, CR Jr, Lundt, ES, Weigand, SD, Zuk, SM, et al. Association of Kidney Function Biomarkers with brain MRI findings: the BRINK study. J Alzheimers Dis. (2017) 55:1069–82. doi: 10.3233/JAD-160834
38. Cho, EB, Shin, HY, Park, SE, Chun, P, Jang, HR, Yang, JJ, et al. Albuminuria, cerebrovascular disease and cortical atrophy: among cognitively Normal elderly individuals. Sci Rep. (2016) 6:20692. doi: 10.1038/srep20692
39. Stehouwer, CD, and Smulders, YM. Microalbuminuria and risk for cardiovascular disease: analysis of potential mechanisms. J Am Soc Nephrol. (2006) 17:2106–11. doi: 10.1681/ASN.2005121288
40. Gorbach, T, Pudas, S, Lundquist, A, Oradd, G, Josefsson, M, Salami, A, et al. Longitudinal association between hippocampus atrophy and episodic-memory decline. Neurobiol Aging. (2017) 51:167–76. doi: 10.1016/j.neurobiolaging.2016.12.002
41. Yamanaka, T, Uchida, Y, Sakurai, K, Kato, D, Mizuno, M, Sato, T, et al. Anatomical links between white matter Hyperintensity and medial temporal atrophy reveal impairment of executive functions. Aging Dis. (2019) 10:711–8. doi: 10.14336/AD.2018.0929
42. Wolf, H, Grunwald, M, Kruggel, F, Riedel-Heller, SG, Angerhofer, S, Hojjatoleslami, A, et al. Hippocampal volume discriminates between normal cognition; questionable and mild dementia in the elderly. Neurobiol Aging. (2001) 22:177–86. doi: 10.1016/S0197-4580(00)00238-4
43. Uchida, Y, Kan, H, Sakurai, K, Horimoto, Y, Hayashi, E, Iida, A, et al. APOE varepsilon4 dose associates with increased brain iron and beta-amyloid via blood-brain barrier dysfunction. J Neurol Neurosurg Psychiatry. (2022) 93:772–8. doi: 10.1136/jnnp-2021-328519
44. Moon, SW, Lee, B, and Choi, YC. Changes in the hippocampal volume and shape in early-onset mild cognitive impairment. Psychiatry Investig. (2018) 15:531–7. doi: 10.30773/pi.2018.02.12
45. Abraham, M, Seidenberg, M, Kelly, DA, Nielson, KA, Woodard, JL, Carson Smith, J, et al. Episodic memory and hippocampal volume predict 5-year mild cognitive impairment conversion in healthy apolipoprotein epsilon4 carriers. J Int Neuropsychol Soc. (2020) 26:733–8. doi: 10.1017/S1355617720000181
Keywords: renal function, hippocampus volume, estimated glomerular filtration, cognitive impairment, magnetic resonance imaging
Citation: Wu L-Y, Lu Y-Y, Zheng S-S, Cui Y-D, Lu J and Zhang A-H (2024) Associations between renal function, hippocampal volume, and cognitive impairment in 544 outpatients. Front. Neurol. 15:1347682. doi: 10.3389/fneur.2024.1347682
Edited by:
Giulia Sprugnoli, University Hospital of Parma, ItalyReviewed by:
Yuto Uchida, Johns Hopkins Medicine, United StatesChristine Fernandez, University of Poitiers, France
Copyright © 2024 Wu, Lu, Zheng, Cui, Lu and Zhang. This is an open-access article distributed under the terms of the Creative Commons Attribution License (CC BY). The use, distribution or reproduction in other forums is permitted, provided the original author(s) and the copyright owner(s) are credited and that the original publication in this journal is cited, in accordance with accepted academic practice. No use, distribution or reproduction is permitted which does not comply with these terms.
*Correspondence: Ai-Hua Zhang, cm9zZXpoYW5nOTk4QGhvdG1haWwuY29t; Jie Lu, aW1hZ2luZ2x1QGhvdG1haWwuY29t