- 1Department of Neurology, West China Hospital of Sichuan University, Chengdu, Sichuan, China
- 2Department of Radiology, West China Hospital of Sichuan University, Chengdu, Sichuan, China
Background: Brain gray matter alterations in patients with trigeminal neuralgia (TN) have been detected in prior neuroimaging studies, but the results are heterogeneous. The current study conducted coordinate-based meta-analyses across neuroimaging studies, aiming to find the pattern of brain anatomic and functional alterations in patients with TN.
Methods: We performed a systematic literature search of PubMed, Embase, and Web of Science to identify relevant publications. A multimodal meta-analysis for whole-brain voxel-based morphometry (VBM) studies and functional imaging studies in TN was performed using anisotropic effect size-based signed differential mapping.
Results: The meta-analysis comprised 10 VBM studies with 398 TN patients and 275 healthy controls, and 13 functional magnetic resonance imaging studies with 307 TN patients and 264 healthy controls. The multimodal meta-analysis showed conjoint structural and functional brain alterations in the right fusiform gyrus and inferior temporal gyrus, bilateral thalamus, left superior temporal gyrus, left insula, and inferior frontal gyrus. The unimodal meta-analysis showed decreased gray matter volume alone in the left putamen, left postcentral gyrus, and right amygdala as well as only functional abnormalities in the left cerebellum, bilateral precuneus, and left middle temporal gyrus.
Conclusion: This meta-analysis revealed overlapping anatomic and functional gray matter abnormalities in patients with TN, which may help provide new insights into the neuropathology and potential treatment biomarkers of TN.
Introduction
Trigeminal neuralgia (TN) is a common cranial neuralgia, which is featured by recurrent severe paroxysmal, electric shock-like, lancinating pain limited to one or more of the trigeminal nerve branches, lasting from a fraction of a second to 2 min per episode and is triggered by innocuous stimuli (1). TN can be classified into secondary TN which is caused by a neurological disease other than neurovascular compression, and primary TN which includes classical TN (cTN) which develops without apparent cause other than neurovascular compression and idiopathic TN (iTN) without significant abnormalities in either electrophysiological tests or conventional magnetic resonance imaging (MRI) (1). The annual incidence of TN is estimated to be four to five per 100,000 and affects one in 15,000–20,000 people worldwide with a female preponderance (2). Increased depression, anxiety, and poor sleep were also reported in patients with TN, indicating that TN is a debilitating neuropathic pain condition that affects human physiological and psychological activities (3). Although the origin of TN is considered to be at the trigeminal root (4), the peripheral mechanisms alone could not entirely explain the pathophysiology of TN, especially iTN.
By changing the flow and integration of information through many brain regions, chronic pain including TN could influence brain function and behavior (5). Central facilitation and central allodynic mechanisms that engage the nociceptive neurons at the trigeminal nucleus, thalamic, and cortical levels were suggested to be related to this persistent pain (6). Growing evidence with advanced neuroimaging techniques has demonstrated that both the brain’s structural and functional changes may also contribute to the development of TN. Voxel-based morphometry (VBM) is a hypothesis-free technique that allows voxel-wise comparisons of the whole brain tissue volume between groups in vivo (7). VBM studies have reported decreased or increased gray matter volume (GMV) in various brain regions in patients with TN, including the secondary somatosensory cortex, insula, thalamus, anterior cingulate cortex, cerebellum, caudate nucleus, amygdala, postcentral gyrus, and precuneus compared to healthy controls (HCs) (8–15), while functional studies using function MRI also detected increased or decreased brain activation in the thalamus, postcentral gyrus, cerebellum, anterior cingulate cortex, and other cortical and subcortical brain regions (10, 11, 16, 17). However, these structural and functional studies often reported different or even contradictory results. The reason for these inconsistencies might be associated with differences in design, imaging methodology, and sample sizes, as well as heterogeneities in demographic and clinical characteristics across studies.
A better understanding of the brain alterations in subjects with TN may provide new insight into the pathophysiology of primary TN, which may contribute to facilitating advances in treatment. In this study, we conducted a multimodal meta-analysis to identify the conjoint and dissociated brain changes in the structure and function of subjects with primary TN. Although there was one meta-analysis that included anatomic and functional neuroimaging studies in subjects with TN published before August 2018, it did not conduct a multimodal meta-analysis to examine the overlapping regions between anatomic and functional brain alterations, and many related studies have been published since then (18). In this study, we integrated the recently published VBM and functional studies on primary TN and conducted unimodal and multimodal meta-analyses by using anisotropic effect size-based signed differential mapping (AES-SDM), which is a powerful coordinate-based meta-analytic tool widely applied in neurological diseases (19). In addition, meta-regression analyses were performed to evaluate potential relationships between brain GMV and functional changes and clinical features.
Materials and methods
Search strategy and study selection
The review process was based on the Preferred Reporting Items for Systematic Reviews and Meta-Analyses (PRISMA) statement. A systematic search was conducted in the Web of Science, PubMed, and Embase up to October 3rd, 2022, using the following keywords (“trigeminal neuralgia” OR “trifacial neuralgia”) AND (“magnetic resonance imaging” OR “MRI” OR “functional MRI” OR “fMRI”). We also searched the reference lists of included studies to obtain potential studies.
Inclusion/exclusion criteria
The inclusion criteria for the candidate studies were: (a) published in English with peer review; (b) employed whole-brain functional imaging or structural imaging using VBM to explore gray matter changes in primary TN compared to HCs; (c) localized effects using Montreal Neurological Institute (MNI) or Talairach coordinates; (d) used thresholds for significance corrected for multiple comparisons or uncorrected with spatial extent thresholds. These studies were considered ineligible: (a) review articles, case reports, and meta-analyses; (b) studies that exclusively used a region of interest approach; (c) studies where the whole-brain results in three-dimensional coordinates could not be retrieved after contacting corresponding authors by emails. Group coordinates were regarded as separate datasets if a study contains more than one independent patient sample. Figure 1 summarizes the study selection procedures.
Data extraction
Study selection and data extraction were conducted in a standard form by two investigators separately and any disagreements were assessed by the third author. Information, such as peak coordinates (x, y, z) and effect sizes (t-, z-, or p-values) of structural or functional differences between subjects with primary TN and HCs were extracted from each dataset according to the SDM tutorial.
Quality assessment
To better evaluate the quality of included studies, a 10-point checklist (Supplementary Table S1) was applied according to prior meta-analysis (20). This assessment evaluated both the demographic and clinical features of each study’s sample and the imaging methodology applied in each study. Each item on the checklist received a score of 0, 0.5, or 1 according to the criteria that were not met, partially met, or fully met, respectively. The checklist paid attention to rating the completeness of the included studies, not to criticize the investigators or the work itself.
Meta-analyses of structural and functional studies
Voxel-wise meta-analyses of regional brain differences in gray matter structure and functional activity were performed independently using the AES-SDM software package (version 5.15, www.sdmproject.com). The procedure has been described in detail in a previous study (21) and briefly summarized here. Using an anisotropic non-normalized Gaussian kernel, AES-SDM recreates an effect-size map and an effect-size variance map of the signed structural or functional activity differences between groups from peak coordinates and effect sizes for each study. All maps were then combined as a mean map with a standard-effects model weighted by the sample size, intra-study variability, and inter-study heterogeneity. Default SDM kernel size and statistical thresholds [full width at half maximum (FWHM) = 20 mm, voxel p = 0.005, peak height z = 1, and cluster extent = 10 voxels] were used (22).
Multimodal analysis
Both structural and functional findings were summarized in a single meta-analytic map as described in a previous study (23, 24) to detect the brain regions showing functional and structural changes. A multimodal meta-analysis in AES-SDM was conducted to assess brain regions of overlapping functional and anatomic alterations between primary TN subjects and HCs by computing the value of p overlap within each voxel from the original meta-analytic maps accounting for error (details in Supplementary materials). A more stringent probability threshold was applied for this multimodal analysis (p < 0.0025) than that used in unimodal meta-analyses (23).
Heterogeneity, publication bias, and sensitivity analysis
To detect the between-study heterogeneity of individual clusters in the meta-analytical results, Q-statistic with a random-effects model and tested for significance with a permutation approach (uncorrected p < 0.005) was applied; thresholds (voxel threshold p < 0.005, peak Z > 1, and cluster extent of 10 voxels) indicated significant heterogeneity (22). We also applied the I2 statistic tests to quantify the degree of heterogeneity. Funnel plots were created by Egger’s tests for visual inspection, in which any result with p < 0.05 indicated obvious publication bias. Jackknife sensitivity analyses were performed to assess the robustness of the main meta-analytical findings by iteratively repeating analyses excluding one dataset each time. Additionally, subgroup analyses of those studies applying same-strength MRI scanners, same smoothing kernel, and statistical parametric mapping (SPM) software were performed. These findings are considered well replicable if the results are still significant in all or majority of the combinations of datasets.
Meta-regression analyses
To explore the potential effects of clinical variables (e.g., age and disease duration) on regional brain anatomic or functional alterations in subjects with primary TN, meta-regression analyses were conducted using a stringent threshold (p < 0.0005 and extent threshold = 10 voxels).
Results
Literature results
Twenty studies (8–14, 16, 17, 25–35) were finally included in the current meta-analyses. Among these studies, three studies (11, 13, 33) reported both structural and functional results. Three fMRI studies (16, 17, 29) shared the same sample but reported results with different imaging measures, while another two pairs of fMRI studies (13, 26, 27, 31) examined another same dataset with different imaging measures. One fMRI study and one VBM study divided patients into two subgroups and conducted two comparisons respectively, so two sets of data were obtained from each of these two studies (11, 12). Another VBM study divided patients into three groups according to different symptoms and conducted three comparisons (34). In the end, we obtained 14 datasets from the 10 VBM studies comprising 398 subjects with primary TN and 275 HCs and 10 datasets from the 13 fMRI studies comprising subjects with 307 primary TN and 264 HCs. The details of demographic and clinical features and quality assessments of the included studies are presented in Table 1. Supplementary Table S2 shows the detailed quality assessment scores of the included studies, all of which got relatively high scores.
Regional alterations in gray matter volume and functional activity
Compared to HCs, subjects with primary TN showed increased GMV in the right inferior temporal gyrus and fusiform gyrus, while they showed decreased GMV in the left insula, left superior temporal gyrus, left putamen, left postcentral gyrus, left inferior frontal gyrus, right amygdala, bilateral thalamus, and left striatum (Table 2; Figures 2, 3).
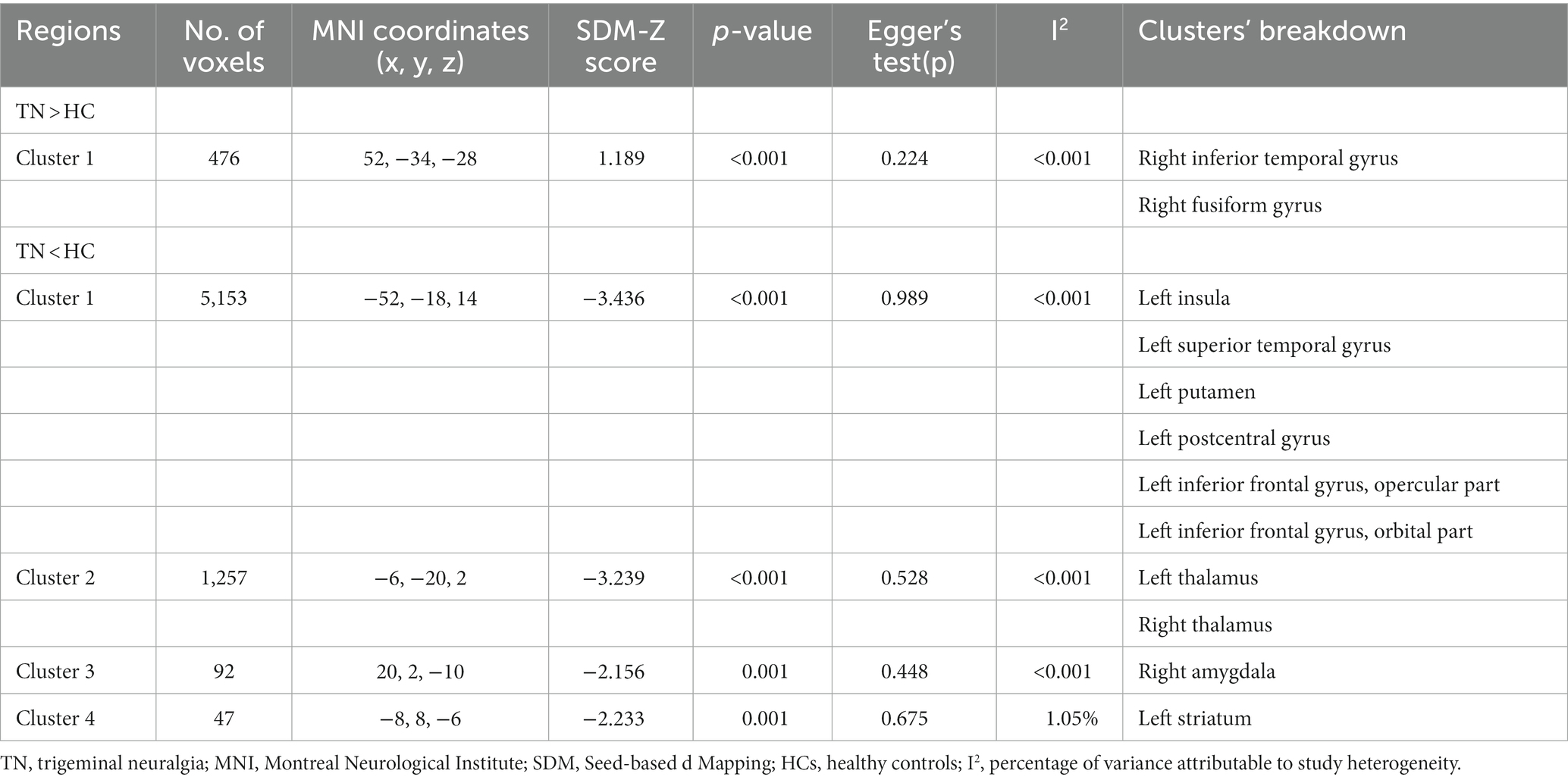
Table 2. Gray matter volume alterations in patients with primary trigeminal neuralgia compared with healthy controls.
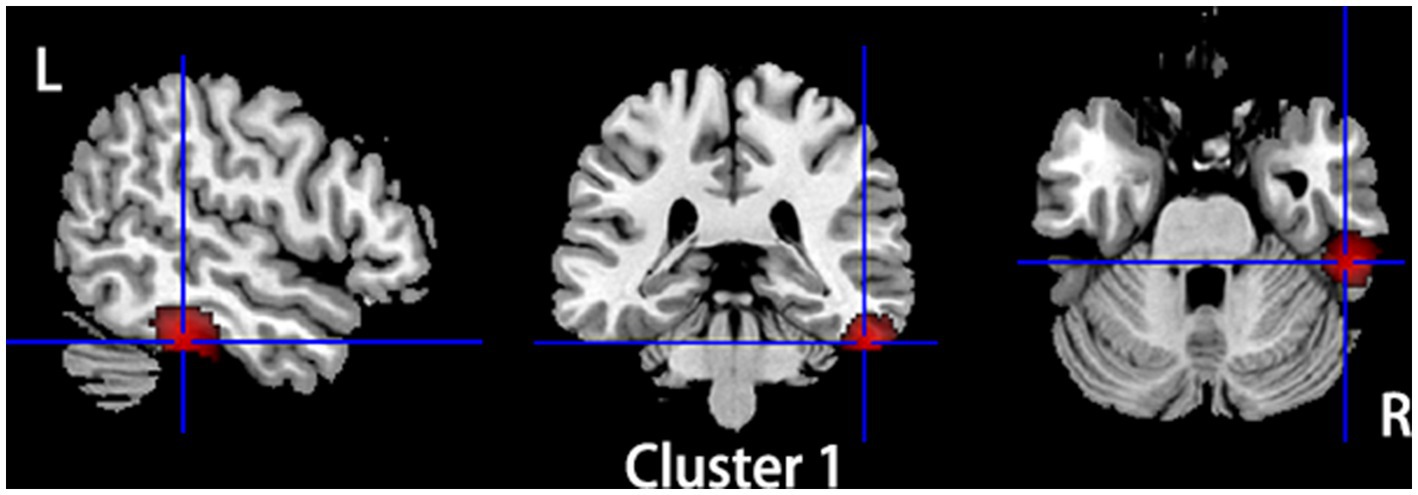
Figure 2. Regions of gray matter volume increase in patients with primary TN compared with healthy controls. Compared to healthy controls, patients with primary TN showed increased gray matter volume in the right inferior temporal gyrus and fusiform gyrus.
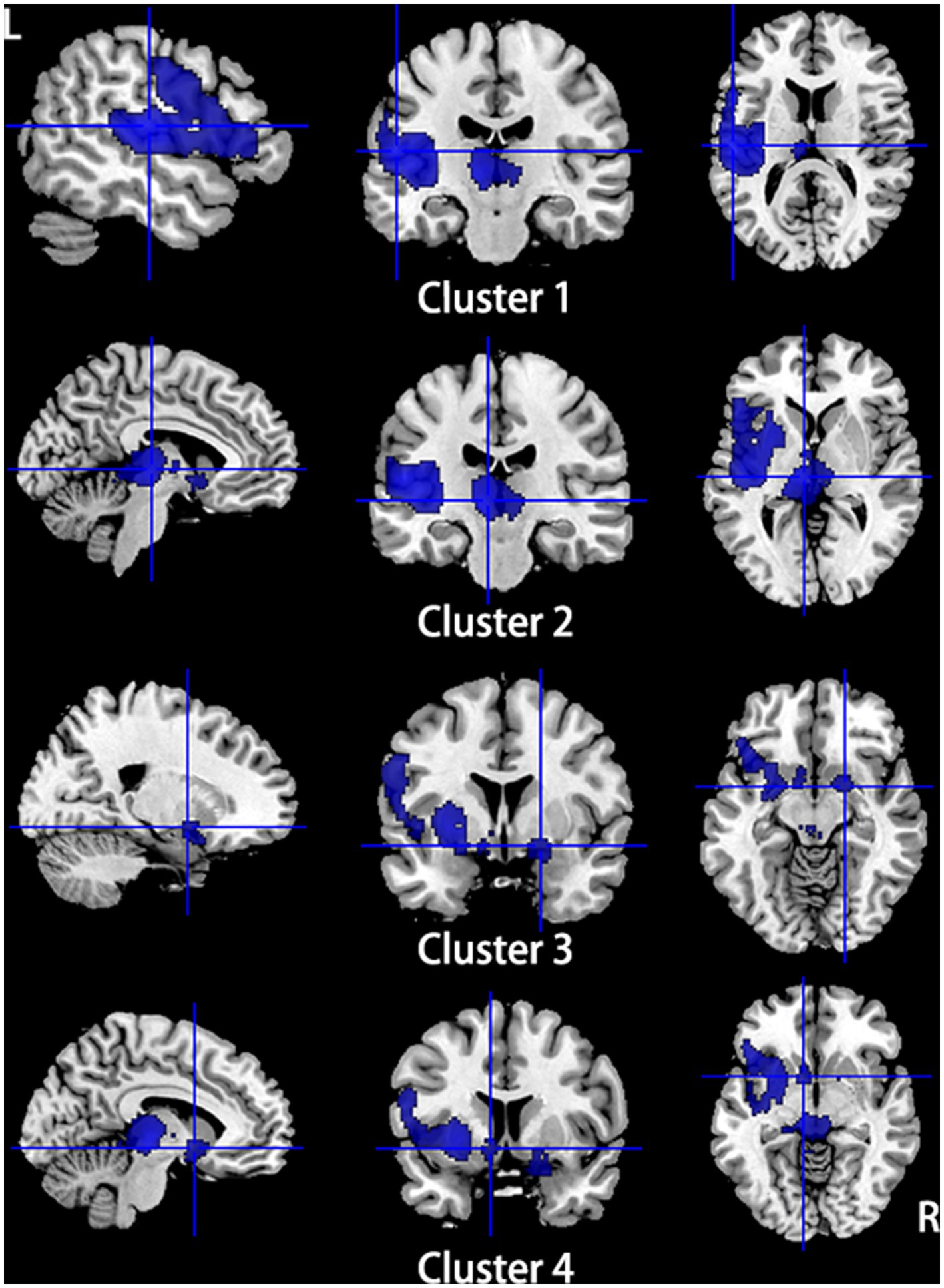
Figure 3. Regions of gray matter volume decrease in patients with primary TN compared with healthy controls. Compared to healthy controls, patients with primary TN showed decreased gray matter volume in the left insula, left superior temporal gyrus, left putamen, left postcentral gyrus, left inferior frontal gyrus (Cluster 1), bilateral thalamus (Cluster 2), right amygdala (Cluster 3), and left striatum (Cluster 4).
Subjects with primary TN showed hyperactivation in the left cerebellum, hemispheric lobule VIII, and bilateral thalamus, while they showed hypoactivation in the bilateral precuneus, left middle temporal gyrus, and superior temporal gyrus (Table 3; Figures 4, 5).
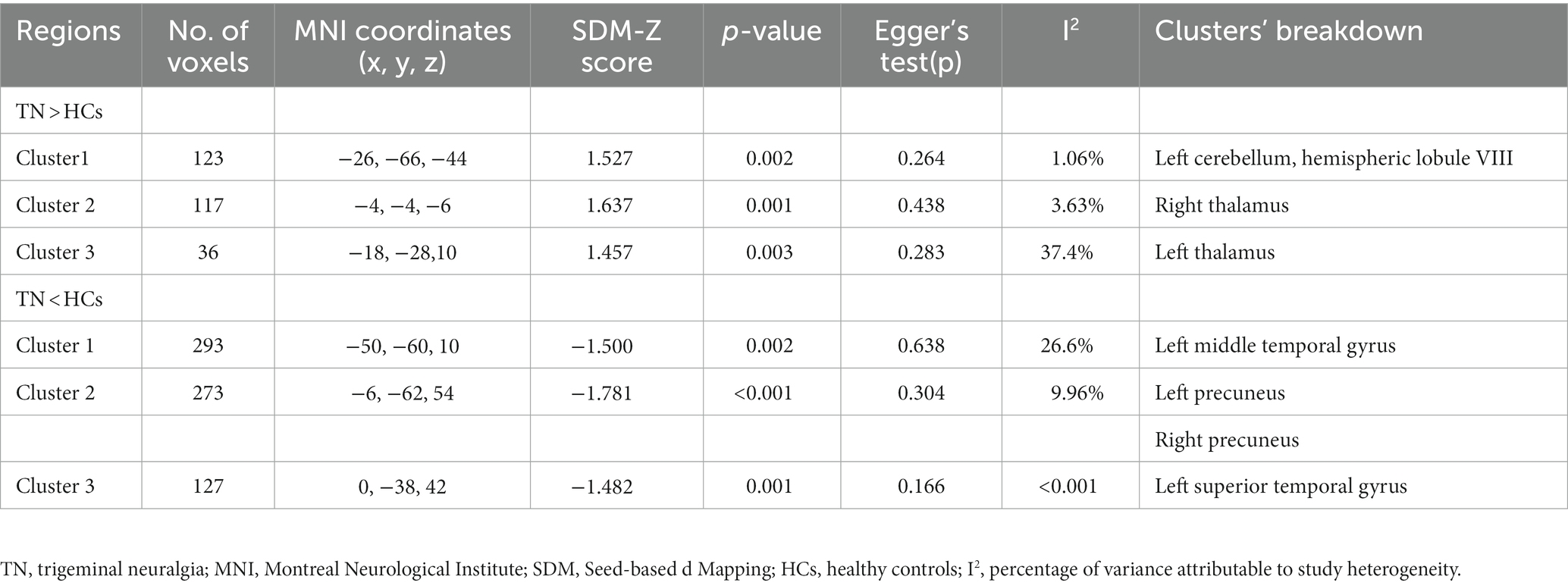
Table 3. Brain functional alterations in patients with primary trigeminal neuralgia compared with healthy controls.
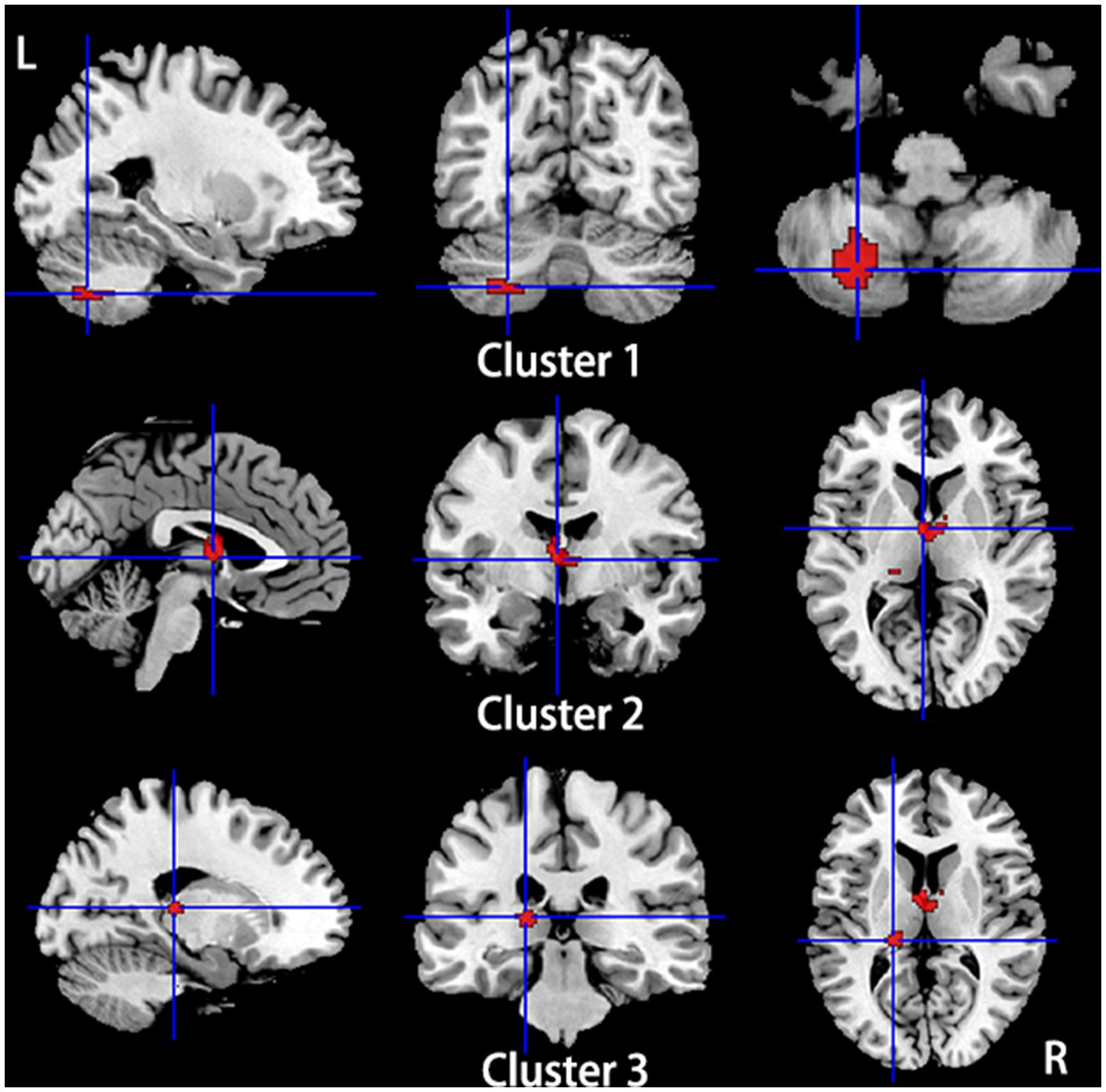
Figure 4. Regions of hyperactivity in patients with primary TN compared with healthy controls. Compared to healthy controls, patients with primary TN showed hyperactivity in the Left cerebellum, hemispheric lobule VIII (cluster 1), right thalamus (cluster 2), and left thalamus (cluster 3).
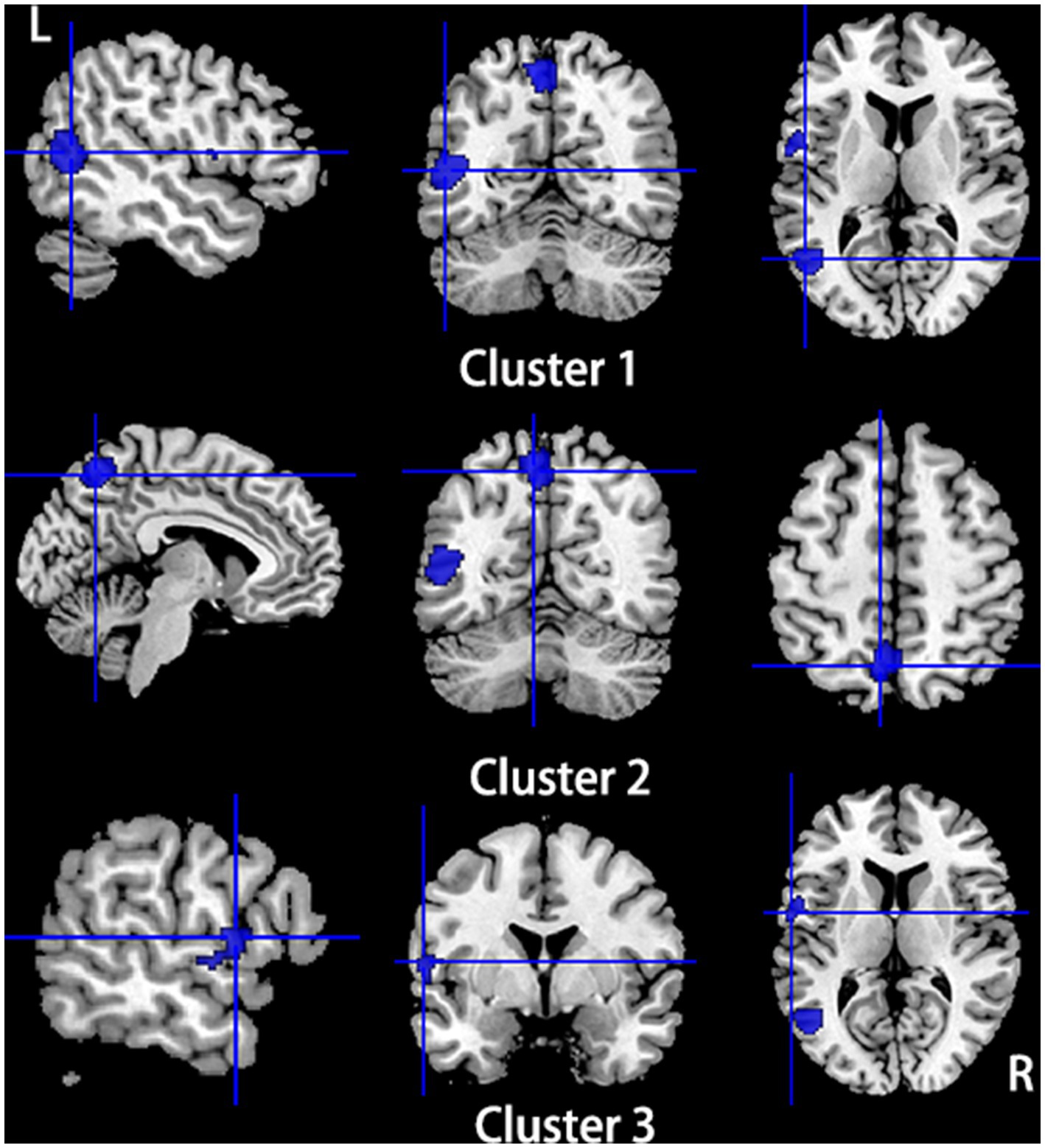
Figure 5. Regions of hypoactivity in patients with primary TN compared with healthy controls. Compared to healthy controls, patients with primary TN showed hypoactivity in the left middle temporal gyrus (cluster 1), bilateral precuneus (cluster 2), and left superior temporal gyrus (Cluster 3).
Multimodal analysis of brain gray matter volume and functional activity
Compared to HCs, subjects with primary TN showed a conjoint increase of GMV and functional activity in the right fusiform gyrus and right inferior temporal gyrus and a decrease of GMV and functional activity in the left superior temporal gyrus, left insula, and left inferior frontal gyrus. The bilateral thalamus showed gray matter hypotrophy with hyperactivity in subjects with primary TN compared to HCs (Table 4; Figure 6).
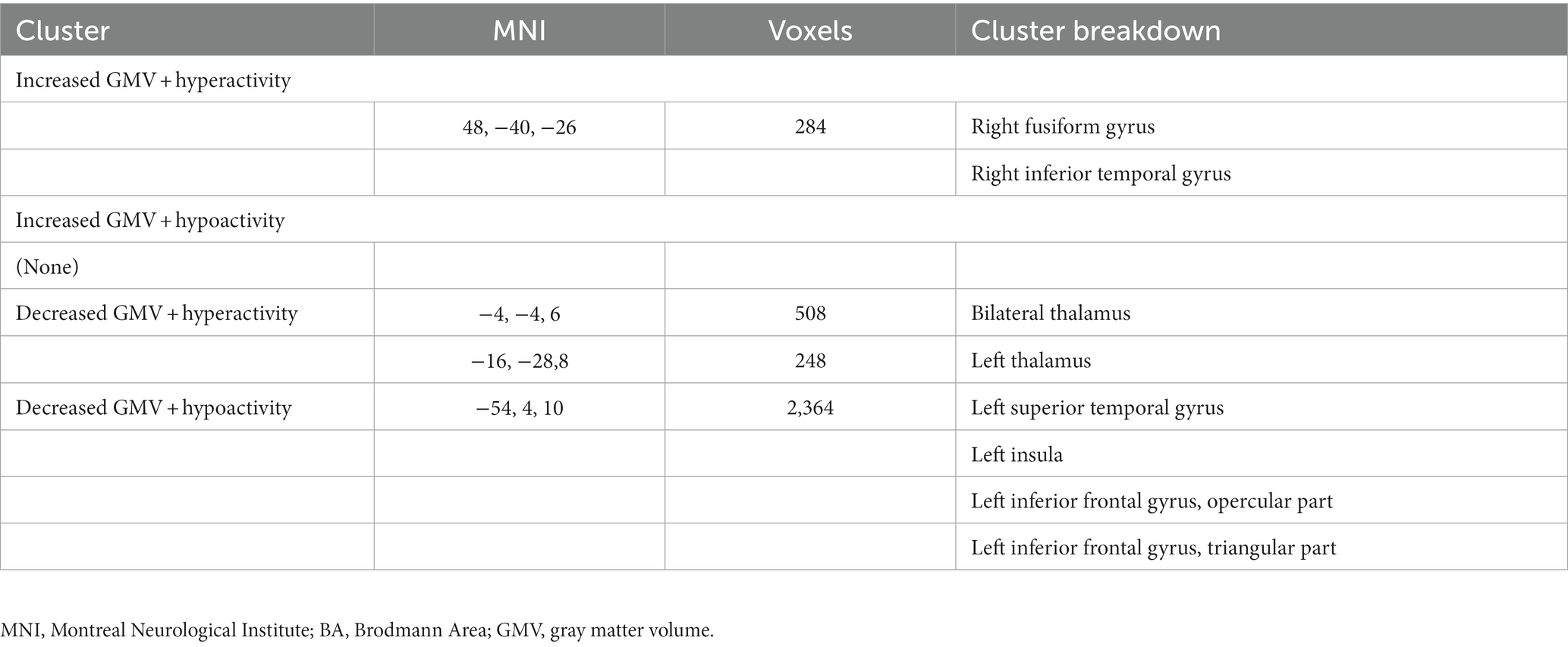
Table 4. Multimodal structural and functional abnormalities in patients with primary trigeminal neuralgia compared with healthy controls.
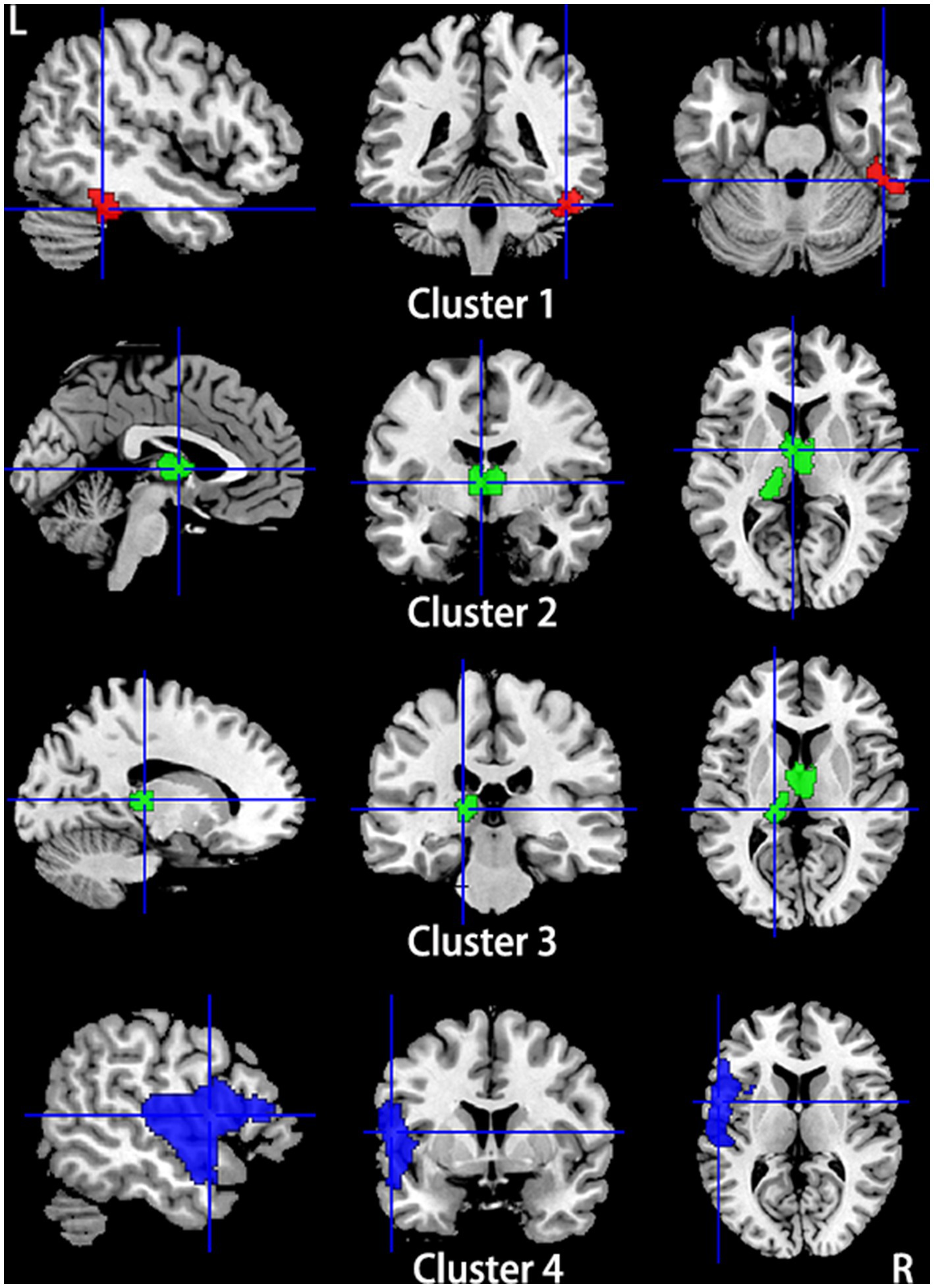
Figure 6. Regions showed conjoint gray matter structural and functional alterations in patients with primary TN compared with healthy controls from the multimodal meta-analysis. Compared to healthy controls, patients with primary TN showed increased gray matter volume and hyperactivity in the right inferior temporal gyrus and fusiform gyrus (Cluster 1), decreased gray matter volume and hyperactivity in the bilateral thalamus (Cluster 2) and left thalamus (cluster 3), and decreased gray matter volume and hypoactivity in left superior temporal gyrus and inferior frontal gyrus and left insula (Cluster 4).
Sensitivity analysis, heterogeneity, and publication Bias analysis
The results from both VBM and functional meta-analyses were highly replicable according to the jackknife sensitivity analyses and subgroup analyses limited to methodologically homogenous groups of studies (Supplementary Tables S3, S4). Heterogeneity analysis using Q statistics indicated that there was no significant variability between studies for meta-analysis of VBM studies and meta-analysis of functional studies. The I2 statistics indicated substantial heterogeneity with an I2 of 37.4 and 26.6% for left thalamus and left middle temporal gyrus, respectively, in the functional meta-analysis, and low heterogeneity for all the other findings in both structural and functional meta-analysis (<10%; Tables 2, 3). No obvious asymmetry of all significant brain regions was unveiled from the funnel plots (Supplementary Figures S1–S11). Egger’s tests revealed no publication bias from the quantitative assessment measured (Tables 2, 3).
Meta-regression results
The meta-regression analysis showed that age at scanning time was positively associated with decreased GMV in the left superior temporal gyrus and left striatum, while disease duration was positively associated with hypoactivation in the left thalamus.
Discussion
To the best of our current knowledge, this is the largest multimodal neuroimaging meta-analytic study investigating overlapping and dissociated gray matter structural and functional changes in subjects with primary TN. We identified conjoint gray matter anatomic and functional alterations in the right fusiform gyrus and inferior temporal gyrus, bilateral thalamus, left superior temporal gyrus, left insula, and inferior frontal gyrus in subjects with primary TN compared to HCs. In addition, several brain regions including the left putamen, left postcentral gyrus, and right amygdala exhibited only gray matter atrophy, while the left cerebellum, bilateral precuneus, and left middle temporal gyrus showed only functional alterations. The current meta-analysis emphasized a central role in the pathophysiology of primary TN with both consistent anatomic and functional alterations mainly in brain regions associated with perception and the processing of pain and regulation of emotion.
An increase in GMV with hyperactive function was found in the right fusiform gyrus and inferior temporal gyrus in subjects with TN relative to HCs. The fusiform gyrus plays a role in sensory integration and cognitive processing (36). The right fusiform gyrus is also one of the visual processing centers of the mammalian brain, which works in integrating visual information and forming conscious perception (29). A previous study found that painful electrical shock may activate the fusiform cortex, indicating that pain in TN may also activate the fusiform gyrus (37). The structural and functional abnormalities in the right fusiform gyrus found in the current study emphasized the role of the fusiform cortex involved in pain perception and modulation in patients with TN. The inferior temporal gyrus has the projection to the amygdala and hippocampus, which plays a crucial role in emotional processing, regulation, and self-cognition (17, 38). Increased functional activity within this region may be relative to the inhibition of pain caused by negative emotions and cognitive degradation (17).
Gray matter atrophy with hyperactivation was detected in the bilateral thalamus in subjects with TN. The thalamus is a pivotal relay station for sensory information. Evidence from neuroimaging studies, physiological studies, and lesioning studies indicated that the thalamus may hold the key to pain consciousness and understanding of spontaneous and evoked pain in chronic pain conditions (39–41). Altered GMV and functional activity in the thalamus were also found in neuroimaging studies in other chronic pain conditions including migraine, chronic back pain, and fibromyalgia (41–43). Using multiple brain imaging techniques, significant volume loss in the somatosensory thalamus was found to be associated with hypoactivity in the thalamic reticular nucleus and primary somatosensory cortex in patients with TN. These findings suggested that altered thalamic structure and activity in chronic neuropathic pain may result in disturbed thalamocortical circuits (44). Neuropathic-specific hyperactivity in the thalamic neurons was found several days after injuring the trigeminal ganglion neurons in the animal model, indicating that these may contribute to neuroplastic changes in the thalamocortical circuits and produce long-lasting neuropathic pain in the orofacial region (45). The decreased GMV of the thalamus identified in the meta-analysis may be related to impaired processing and modulation of neuropathic pain signals, and the hyperactivation in the thalamus may be secondary to the gray matter atrophy and suggest increased sensory load in patients with TN.
A conjoint decrease in GMV and functional activity was found in the left inferior frontal gyrus, left insula, and superior temporal gyrus in subjects with TN. Anatomic and functional alterations in the left inferior frontal gyrus were also reported in other pain-related disorders such as myofascial-type temporomandibular disorders (46), diabetic neuropathic pain (47), and affective disorders (48). The left inferior frontal gyrus is a subregion of the prefrontal cortex, which is one of the key regions involved in the affective, cognitive, and emotional aspects of pain, and can be activated by pain stimuli and has clear implications in pain perception and modulation (49). GM atrophy with hypoactivity in the left inferior frontal gyrus may lead to the loss of inhibitory control of the nociceptive transmission system (50) and may provide the neuroanatomical basis for the increased incidence of mood disorders reported in TN (3). The insula is involved not only in sensorimotor and affective processing, autonomic information, and high-level cognition but also in pain perception and its modulation according to prior neuroimaging studies (51, 52). Changes in cortical gyrification and associated altered functional connectivity were identified in the left insula and its critical roles in the pathophysiology of TN were emphasized (53). The superior temporal gyrus is critical for extracting meaningful linguistic features from speech input and is involved in managing perception in auditory, speech, emotions, and comprehension (54). The temporal lobe including superior temporal gyrus plays a critical role in the perception of chronic pain (9, 10). The decreased GMV and functional activity in the left superior temporal gyrus may indicate an adaptative mechanism initiated in response to chronic pain and activity-dependent dendritic changes.
Additionally, we found gray matter atrophy in the right amygdala in the unimodal meta-analysis. The amygdala is a pivotal brain center for the emotional-affective dimension of pain and pain modulation. Other chronic pain conditions in previous studies also detected changes in anatomic and functional activities within the amygdala (55, 56). The amygdala as an essential part of the emotion-related network involved in emotional processing, anxiety, stress regulation, reward learning, and motivation is associated with affective disorders, including anxiety and depression (57). The structural and functional alterations within the amygdala may also contribute to a significantly increased incidence of anxiety and depression in patients with TN (3). Gray matter atrophy and altered connectivity in the amygdala may be exhibited during the transition to chronic pain. Using the multimodal neuroimaging approach to assess TN-related structural and functional brain alterations in emotion-related and pain-related networks (13), reduced GMV in the bilateral amygdala and three significantly altered amygdala-related functional circuits were found and they supported the pain-related and emotion-related network deficits in TN patients. In this study, we found asymmetric changes in structural changes within the amygdala, which may be related to the hemispheric lateralization of pain processing. The left central nucleus of the amygdala showed lower neuronal activity than the right in models of inflammatory pain, independent of the side of the peripheral injury (58). Meanwhile, asymmetrical time-dependent activation of bilateral amygdala neurons was reported in rats with peripheral neuropathy, with enhanced evoked activity in the right central nucleus of the amygdala persisting compared to short-term increases in activity in the left, indicating that the right central nucleus of the amygdala was highly involved in the processing of sensitization related to neuropathy (59). The asymmetric findings in the amygdala may be the case that the hypotrophy in the right amygdala may be resulting from persistent activity.
Several brain regions showed anatomic or functional anomalies alone in subjects with TN in the current study. The anatomic abnormalities included GMV atrophy in the left putamen and left postcentral gyrus, all without functional brain activity changes. The putamen is connected with both cortical regions involved in sensory processing, attention, and memory. Widespread decreases in pain-related brain activation were identified in patients with putamen lesions, suggesting that the putamen may be pivotally involved in the shaping of the pain experience (60). GMV reduction in the putamen in the current meta-analysis was consistent with previous studies (11, 15, 25, 34, 61), supporting the hypothesis that the putamen contributed to sensory aspects of pain. The postcentral gyrus is associated with the anticipation, intensity, discrimination, spatial, and temporal summation aspects of pain processing and self-reported pain intensity (15).
The hyperactivity in the left cerebellum and hypoactivity in the left middle temporal gyrus and bilateral precuneus were not accompanied by GMV alterations. The cerebellum plays a crucial role in modulating motor control and is involved in a range of movement disorders. Also, a previous study found that the cerebellum can receive extensive somatosensory input via spinocerebellar pathways, underscoring its additional role as a sensory organ (62). Cerebellar functional changes may result from increased sensory input derived from long-term and high-frequency inputs related to patients with TN. The left middle temporal gyrus is involved in the perception of pain as previous neuroimaging studies reported pain-related activation within this brain region (63, 64). Both the precuneus and left middle temporal gyrus are crucial brain regions of the default mode network (DMN). The DMN is associated with mediating the recognition and rumination of pain and internal processing including autobiographical memory, self-reference, and stimulus-independent thought (65). DMN connectivity was identified to be related to pain frequency and intensity in adolescents (66), and alterations in connectivity between the DMN and the pain network were reported in adults with chronic pain (67). Extending prior works, current findings indicated that TN may be related to the desegregation of the DMN, and the hypoactivity of critical nodes within this network may represent altered maintenance of attention and vigilance of pain and reflect TN as a background pain sensation (27).
There were also some limitations in this study. First, the heterogeneity among the included studies in demographic data and methodologies could not be entirely ruled out. Second, for structural alterations, studies that investigated the cortical thickness alterations using surface-based morphometry (SBM) in TN were not included because the meta-analysis of these studies needed to use a thickness mask (68) that is different from the one used for VBM studies, and the limited number of published SBM studies in TN prevents the conducting of the vertex-based meta-analysis (12, 69, 70). Third, various physiological bases and underpinning assumptions of the included methods used in the fMRI studies may affect the results of the meta-analysis, hindering the comprehensive overview of whole-brain functional alterations in TN. Lastly, the current multimodal meta-analysis aims to identify overlapping brain regions showing both anatomic and functional changes; the directionality and causality of the findings cannot be directly answered.
Conclusion
Our multimodal meta-analysis demonstrated GM alterations in patients with TN, characterized by conjoint structural and functional alterations in the bilateral thalamus, left inferior frontal gyrus, insula and superior temporal gyrus, and right inferior temporal gyrus, and separative GMV reduction in the putamen, postcentral gyrus and amygdala, and altered function in cerebellum and nodes of the DMN. The pattern of conjoint and disassociated changes in the structure and function of GM prompted our understanding of the mechanisms underlying TN and may provide potential treatment biomarkers for TN in the future.
Author contributions
XH: conception, organization, execution, data acquisition, statistical analysis, and manuscript preparation. BL: organization, execution, data acquisition, statistical analysis, and manuscript preparation and revision. YL: project execution, data acquisition, manuscript review, and critique. JL and HS: manuscript review and critique. JY: conception, organization, statistical analysis, manuscript review, and critique; he was responsible for the overall content as the guarantor. All authors contributed to the article and approved the submitted version.
Funding
This study was supported by the National Natural Science Foundation of China (No. 81971071), the Applied Basic Research Programs of Science and Technology Department of Sichuan Province (No. 2021YJ0447), and the 1.3.5 project for disciplines of excellence-Clinical Research Incubation Project, West China Hospital, Sichuan University (No. 2021HXFH044).
Acknowledgments
The authors thank all authors of the included studies.
Conflict of interest
The authors declare that the research was conducted in the absence of any commercial or financial relationships that could be construed as a potential conflict of interest.
Publisher’s note
All claims expressed in this article are solely those of the authors and do not necessarily represent those of their affiliated organizations, or those of the publisher, the editors and the reviewers. Any product that may be evaluated in this article, or claim that may be made by its manufacturer, is not guaranteed or endorsed by the publisher.
Supplementary material
The Supplementary material for this article can be found online at: https://www.frontiersin.org/articles/10.3389/fneur.2023.1179896/full#supplementary-material
References
1. Headache Classification Committee of the International Headache Society (IHS) The International Classification of Headache Disorders, 3rd edition. Cephalalgia Rep. (2018) 38:1–211. doi: 10.1177/0333102417738202
2. Xu, R, Xie, ME, and Jackson, CM. Trigeminal neuralgia: current approaches and emerging interventions. J Pain Res. (2021) 14:3437–63. doi: 10.2147/JPR.S331036
3. Wu, TH, Hu, LY, Lu, T, Chen, PM, Chen, HJ, Shen, CC, et al. Risk of psychiatric disorders following trigeminal neuralgia: a nationwide population-based retrospective cohort study. J Headache Pain. (2015) 16:64. doi: 10.1186/s10194-015-0548-y
4. Antonini, G, Di Pasquale, A, Cruccu, G, Truini, A, Morino, S, Saltelli, G, et al. Magnetic resonance imaging contribution for diagnosing symptomatic neurovascular contact in classical trigeminal neuralgia: a blinded case-control study and meta-analysis. Pain. (2014) 155:1464–71. doi: 10.1016/j.pain.2014.04.020
5. Baliki, MN, Baria, AT, and Apkarian, AV. The cortical rhythms of chronic back pain. J Neurosci. (2011) 31:13981–90. doi: 10.1523/JNEUROSCI.1984-11.2011
6. Obermann, M, Yoon, MS, Ese, D, Maschke, M, Kaube, H, Diener, HC, et al. Impaired trigeminal nociceptive processing in patients with trigeminal neuralgia. Neurology. (2007) 69:835–41. doi: 10.1212/01.wnl.0000269670.30045.6b
7. Ashburner, J, and Friston, KJ. Voxel-based morphometry—the methods. NeuroImage. (2000) 11:805–21. doi: 10.1006/nimg.2000.0582
8. Obermann, M, Rodriguez-Raecke, R, Naegel, S, Holle, D, Mueller, D, Yoon, MS, et al. Gray matter volume reduction reflects chronic pain in trigeminal neuralgia. NeuroImage. (2013) 74:352–8. doi: 10.1016/j.neuroimage.2013.02.029
9. Li, M, Yan, J, Li, S, Wang, T, Zhan, W, Wen, H, et al. Reduced volume of gray matter in patients with trigeminal neuralgia. Brain Imaging Behav. (2017) 11:486–92. doi: 10.1007/s11682-016-9529-2
10. Wang, Y, Cao, DY, Remeniuk, B, Krimmel, S, Seminowicz, DA, and Zhang, M. Altered brain structure and function associated with sensory and affective components of classic trigeminal neuralgia. Pain. (2017) 158:1561–70. doi: 10.1097/j.pain.0000000000000951
11. Tsai, YH, Yuan, R, Patel, D, Chandrasekaran, S, Weng, HH, Yang, JT, et al. Altered structure and functional connection in patients with classical trigeminal neuralgia. Hum Brain Mapp. (2018) 39:609–21. doi: 10.1002/hbm.23696
12. Wu, M, Jiang, X, Qiu, J, Fu, X, and Niu, C. Gray and white matter abnormalities in primary trigeminal neuralgia with and without neurovascular compression. J Headache Pain. (2020) 21:136. doi: 10.1186/s10194-020-01205-3
13. Zhang, Y, Mao, Z, Pan, L, Ling, Z, Liu, X, Zhang, J, et al. Dysregulation of pain-and emotion-related networks in trigeminal neuralgia. Front Hum Neurosci. (2018) 12:107. doi: 10.3389/fnhum.2018.00107
14. Wang, Y, Yang, Q, Cao, D, Seminowicz, D, Remeniuk, B, Gao, L, et al. Correlation between nerve atrophy, brain grey matter volume and pain severity in patients with primary trigeminal neuralgia. Cephalalgia. (2019) 39:515–25. doi: 10.1177/0333102418793643
15. DeSouza, DD, Moayedi, M, Chen, DQ, Davis, KD, and Hodaie, M. Sensorimotor and pain modulation brain abnormalities in trigeminal neuralgia: a paroxysmal, sensory-triggered neuropathic pain. PLoS One. (2013) 8:e66340. doi: 10.1371/journal.pone.0066340
16. Zhu, P-W, Chen, Y, Gong, Y-X, Jiang, N, Liu, W-F, Su, T, et al. Altered brain network centrality in patients with trigeminal neuralgia: a resting-state fMRI study. Acta Radiol. (2020) 61:67–75. doi: 10.1177/0284185119847678
17. Chen, Y, Xiang, CQ, Liu, WF, Jiang, N, Zhu, PW, Ye, L, et al. Application of amplitude of low-frequency fluctuation to altered spontaneous neuronal activity in classical trigeminal neuralgia patients: a resting-state functional MRI study. Mol Med Rep. (2019) 20:1707–15. doi: 10.3892/mmr.2019.10404
18. Henssen, D, Dijk, J, Knepflé, R, Sieffers, M, Winter, A, and Vissers, K. Alterations in grey matter density and functional connectivity in trigeminal neuropathic pain and trigeminal neuralgia: a systematic review and meta-analysis. NeuroImage Clin. (2019) 24:102039. doi: 10.1016/j.nicl.2019.102039
19. Lansley, J, Mataix-Cols, D, Grau, M, Radua, J, and Sastre-Garriga, J. Localized grey matter atrophy in multiple sclerosis: a meta-analysis of voxel-based morphometry studies and associations with functional disability. Neurosci Biobehav Rev. (2013) 37:819–30. doi: 10.1016/j.neubiorev.2013.03.006
20. Wang, T, Liu, J, Zhang, J, Zhan, W, Li, L, Wu, M, et al. Altered resting-state functional activity in posttraumatic stress disorder: a quantitative meta-analysis. Sci Rep. (2016) 6:27131. doi: 10.1038/srep27131
21. Radua, J, and Mataix-Cols, D. Voxel-wise meta-analysis of grey matter changes in obsessive-compulsive disorder. Br J Psychiatry J Ment Sci. (2009) 195:393–402. doi: 10.1192/bjp.bp.108.055046
22. Radua, J, Mataix-Cols, D, Phillips, ML, El-Hage, W, Kronhaus, DM, Cardoner, N, et al. A new meta-analytic method for neuroimaging studies that combines reported peak coordinates and statistical parametric maps. Eur Psychiatry. (2012) 27:605–11. doi: 10.1016/j.eurpsy.2011.04.001
23. Radua, J, Borgwardt, S, Crescini, A, Mataix-Cols, D, Meyer-Lindenberg, A, McGuire, PK, et al. Multimodal meta-analysis of structural and functional brain changes in first episode psychosis and the effects of antipsychotic medication. Neurosci Biobehav Rev. (2012) 36:2325–33. doi: 10.1016/j.neubiorev.2012.07.012
24. Huang, X, Zhang, M, Li, B, Shang, H, and Yang, J. Structural and functional brain abnormalities in idiopathic cervical dystonia: a multimodal meta-analysis. Parkinsonism Relat Disord. (2022) 103:153–65. doi: 10.1016/j.parkreldis.2022.08.029
25. Gustin, SM, Peck, CC, Wilcox, SL, Nash, PG, Murray, GM, and Henderson, LA. Different pain, different brain: thalamic anatomy in neuropathic and non-neuropathic chronic pain syndromes. J Neurosci. (2011) 31:5956–64. doi: 10.1523/JNEUROSCI.5980-10.2011
26. Wang, Y, Zhang, X, Guan, Q, Wan, L, Yi, Y, and Liu, C-F. Altered regional homogeneity of spontaneous brain activity in idiopathic trigeminal neuralgia. Neuropsychiatr Dis Treat. (2015) 11:2659–66. doi: 10.2147/NDT.S94877
27. Wang, Y, Xu, C, Zhai, L, Lu, X, Wu, X, Yi, Y, et al. Spatial-temporal signature of resting-state BOLD signals in classic trigeminal neuralgia. J Pain Res. (2017) 10:2741–50. doi: 10.2147/JPR.S143734
28. Yuan, J, Cao, S, Huang, Y, Zhang, Y, Xie, P, Zhang, Y, et al. Altered spontaneous brain activity in patients with idiopathic trigeminal neuralgia: a resting-state functional MRI study. Clin J Pain. (2018) 34:600–9. doi: 10.1097/AJP.0000000000000578
29. Xiang, C-Q, Liu, W-F, Xu, Q-H, Su, T, Shu, Y-Q, Min, Y-L, et al. Altered spontaneous brain activity in patients with classical trigeminal neuralgia using regional homogeneity: a resting-state functional MRI study. Pain Pract. (2019) 19:397–406. doi: 10.1111/papr.12753
30. Yan, J, Li, M, Fu, S, Li, G, Wang, T, Yin, Y, et al. Alterations of dynamic regional homogeneity in trigeminal neuralgia: a resting-state fMRI study. Front Neurol. (2019) 10:1083. doi: 10.3389/fneur.2019.01083
31. Zhang, Y, Mao, Z, Pan, L, Ling, Z, Liu, X, Zhang, J, et al. Frequency-specific alterations in cortical rhythms and functional connectivity in trigeminal neuralgia. Brain Imaging Behav. (2019) 13:1497–509. doi: 10.1007/s11682-019-00105-8
32. Albano, L, Agosta, F, Basaia, S, Castellano, A, Messina, R, Parisi, V, et al. Alterations of brain structural MRI are associated with outcome of surgical treatment in trigeminal neuralgia. Eur J Neurol. (2022) 29:305–17. doi: 10.1111/ene.15105
33. Liu, H, Hou, H, Li, F, Zheng, R, Zhang, Y, Cheng, J, et al. Structural and functional brain changes in patients with classic trigeminal neuralgia: a combination of voxel-based morphometry and resting-state functional MRI study. Front Neurosci. (2022) 16:930765. doi: 10.3389/fnins.2022.930765
34. Shen, S, Zheng, H, Wang, J, Guo, W, Guo, X, Ji, H, et al. Gray matter volume reduction with different disease duration in trigeminal neuralgia. Neuroradiology. (2022) 64:301–11. doi: 10.1007/s00234-021-02783-y
35. Xu, H, Seminowicz, DA, Krimmel, SR, Zhang, M, Gao, L, and Wang, Y. Altered structural and functional connectivity of salience network in patients with classic trigeminal neuralgia. J Pain. (2022) 23:1389–99. doi: 10.1016/j.jpain.2022.02.012
36. Parise, M, Kubo, TT, Doring, TM, Tukamoto, G, Vincent, M, and Gasparetto, EL. Cuneus and fusiform cortices thickness is reduced in trigeminal neuralgia. J Headache Pain. (2014) 15:17. doi: 10.1186/1129-2377-15-17
37. Ter Minassian, A, Ricalens, E, Humbert, S, Duc, F, Aubé, C, and Beydon, L. Dissociating anticipation from perception: acute pain activates default mode network. Hum Brain Mapp. (2013) 34:2228–43. doi: 10.1002/hbm.22062
38. Vincent, MB, and Hadjikhani, N. Migraine aura and related phenomena: beyond scotomata and scintillations. Cephalalgia. (2007) 27:1368–77. doi: 10.1111/j.1468-2982.2007.01388.x
39. Yen, CT, and Lu, PL. Thalamus and pain. Acta Anaesthesiol Taiwan. (2013) 51:73–80. doi: 10.1016/j.aat.2013.06.011
40. Vos, BP, Benoist, JM, Gautron, M, and Guilbaud, G. Changes in neuronal activities in the two ventral posterior medial thalamic nuclei in an experimental model of trigeminal pain in the rat by constriction of one infraorbital nerve. Somatosens Mot Res. (2000) 17:109–22. doi: 10.1080/08990220050020535
41. Apkarian, AV, Sosa, Y, Sonty, S, Levy, RM, Harden, RN, Parrish, TB, et al. Chronic back pain is associated with decreased prefrontal and thalamic gray matter density. J Neurosci. (2004) 24:10410–5. doi: 10.1523/JNEUROSCI.2541-04.2004
42. Lim, M, Jassar, H, Kim, DJ, Nascimento, TD, and DaSilva, AF. Differential alteration of fMRI signal variability in the ascending trigeminal somatosensory and pain modulatory pathways in migraine. J Headache Pain. (2021) 22:4. doi: 10.1186/s10194-020-01210-6
43. Cifre, I, Sitges, C, Fraiman, D, Muñoz, M, Balenzuela, P, González-Roldán, A, et al. Disrupted functional connectivity of the pain network in fibromyalgia. Psychosom Med. (2012) 74:55–62. doi: 10.1097/PSY.0b013e3182408f04
44. Henderson, LA, Peck, CC, Petersen, ET, Rae, CD, Youssef, AM, Reeves, JM, et al. Chronic pain: lost inhibition? J Neurosci. (2013) 33:7574–82. doi: 10.1523/JNEUROSCI.0174-13.2013
45. Tseng, WT, Tsai, ML, Iwata, K, and Yen, CT. Long-term changes in trigeminal ganglionic and thalamic neuronal activities following inferior alveolar nerve transection in behaving rats. J Neurosci. (2012) 32:16051–63. doi: 10.1523/JNEUROSCI.1828-12.2012
46. Gerstner, G, Ichesco, E, Quintero, A, and Schmidt-Wilcke, T. Changes in regional gray and white matter volume in patients with myofascial-type temporomandibular disorders: a voxel-based morphometry study. J Orofac Pain. (2011) 25:99–106.
47. Cauda, F, Sacco, K, Duca, S, Cocito, D, D'Agata, F, Geminiani, GC, et al. Altered resting state in diabetic neuropathic pain. PLoS One. (2009) 4:e4542. doi: 10.1371/journal.pone.0004542
48. Fujino, J, Yamasaki, N, Miyata, J, Kawada, R, Sasaki, H, Matsukawa, N, et al. Altered brain response to others′ pain in major depressive disorder. J Affect Disord. (2014) 165:170–5. doi: 10.1016/j.jad.2014.04.058
49. Schmidt-Wilcke, T. Neuroimaging of chronic pain. Best Pract Res Clin Rheumatol. (2015) 29:29–41. doi: 10.1016/j.berh.2015.04.030
50. Fierro, B, De Tommaso, M, Giglia, F, Giglia, G, Palermo, A, and Brighina, F. Repetitive transcranial magnetic stimulation (rTMS) of the dorsolateral prefrontal cortex (DLPFC) during capsaicin-induced pain: modulatory effects on motor cortex excitability. Exp Brain Res. (2010) 203:31–8. doi: 10.1007/s00221-010-2206-6
51. Augustine, JR. Circuitry and functional aspects of the insular lobe in primates including humans. Brain Res Brain Res Rev. (1996) 22:229–44. doi: 10.1016/S0165-0173(96)00011-2
52. Frot, M, Faillenot, I, and Mauguière, F. Processing of nociceptive input from posterior to anterior insula in humans. Hum Brain Mapp. (2014) 35:5486–99. doi: 10.1002/hbm.22565
53. Wang, Y, Zhang, Y, Zhang, J, Wang, J, Xu, J, Li, J, et al. Structural and functional abnormalities of the insular cortex in trigeminal neuralgia: a multimodal magnetic resonance imaging analysis. Pain. (2018) 159:507–14. doi: 10.1097/j.pain.0000000000001120
54. Yi, HG, Leonard, MK, and Chang, EF. The encoding of speech sounds in the superior temporal gyrus. Neuron. (2019) 102:1096–110. doi: 10.1016/j.neuron.2019.04.023
55. Gagnon, CM, Scholten, P, Atchison, J, Jabakhanji, R, Wakaizumi, K, and Baliki, M. Structural MRI analysis of chronic pain patients following interdisciplinary treatment shows changes in brain volume and opiate-dependent reorganization of the amygdala and Hippocampus. Pain Med. (2020) 21:2765–76. doi: 10.1093/pm/pnaa129
56. Jiang, Y, Oathes, D, Hush, J, Darnall, B, Charvat, M, Mackey, S, et al. Perturbed connectivity of the amygdala and its subregions with the central executive and default mode networks in chronic pain. Pain. (2016) 157:1970–8. doi: 10.1097/j.pain.0000000000000606
57. Simons, LE, Moulton, EA, Linnman, C, Carpino, E, Becerra, L, and Borsook, D. The human amygdala and pain: evidence from neuroimaging. Hum Brain Mapp. (2014) 35:527–38. doi: 10.1002/hbm.22199
58. Carrasquillo, Y, and Gereau, RW. Hemispheric lateralization of a molecular signal for pain modulation in the amygdala. Mol Pain. (2008) 4:1744-8069-4-24. doi: 10.1186/1744-8069-4-24
59. Gonçalves, L, and Dickenson, AH. Asymmetric time-dependent activation of right central amygdala neurones in rats with peripheral neuropathy and pregabalin modulation. Eur J Neurosci. (2012) 36:3204–13. doi: 10.1111/j.1460-9568.2012.08235.x
60. Starr, CJ, Sawaki, L, Wittenberg, GF, Burdette, JH, Oshiro, Y, Quevedo, AS, et al. The contribution of the putamen to sensory aspects of pain: insights from structural connectivity and brain lesions. Brain J Neurol. (2011) 134:1987–2004. doi: 10.1093/brain/awr117
61. Tang, Y, Wang, M, Zheng, T, Yuan, F, Yang, H, Han, F, et al. Grey matter volume alterations in trigeminal neuralgia: a systematic review and meta-analysis of voxel-based morphometry studies. Prog Neuro-Psychopharmacol Biol Psychiatry. (2020) 98:109821. doi: 10.1016/j.pnpbp.2019.109821
62. Gao, JH, Parsons, LM, Bower, JM, Xiong, J, Li, J, and Fox, PT. Cerebellum implicated in sensory acquisition and discrimination rather than motor control. Science (New York, NY). (1996) 272:545–7. doi: 10.1126/science.272.5261.545
63. Freund, W, Klug, R, Weber, F, Stuber, G, Schmitz, B, and Wunderlich, AP. Perception and suppression of thermally induced pain: a fMRI study. Somatosens Mot Res. (2009) 26:1–10. doi: 10.1080/08990220902738243
64. Smallwood, RF, Laird, AR, Ramage, AE, Parkinson, AL, Lewis, J, Clauw, DJ, et al. Structural brain anomalies and chronic pain: a quantitative meta-analysis of gray matter volume. J Pain. (2013) 14:663–75. doi: 10.1016/j.jpain.2013.03.001
65. Kucyi, A, Moayedi, M, Weissman-Fogel, I, Goldberg, MB, Freeman, BV, Tenenbaum, HC, et al. Enhanced medial prefrontal-default mode network functional connectivity in chronic pain and its association with pain rumination. J Neurosci. (2014) 34:3969–75. doi: 10.1523/JNEUROSCI.5055-13.2014
66. Jones, SA, Morales, AM, Holley, AL, Wilson, AC, and Nagel, BJ. Default mode network connectivity is related to pain frequency and intensity in adolescents. NeuroImage Clin. (2020) 27:102326. doi: 10.1016/j.nicl.2020.102326
67. Farmer, MA, Chanda, ML, Parks, EL, Baliki, MN, Apkarian, AV, and Schaeffer, AJ. Brain functional and anatomical changes in chronic prostatitis/chronic pelvic pain syndrome. J Urol. (2011) 186:117–24. doi: 10.1016/j.juro.2011.03.027
68. Li, Q, Zhao, Y, Chen, Z, Long, J, Dai, J, Huang, X, et al. Meta-analysis of cortical thickness abnormalities in medication-free patients with major depressive disorder. Neuropsychopharmacology. (2020) 45:703–12. doi: 10.1038/s41386-019-0563-9
69. Li, M, Yan, J, Wen, H, Lin, J, Liang, L, Li, S, et al. Cortical thickness, gyrification and sulcal depth in trigeminal neuralgia. Sci Rep. (2021) 11:16322. doi: 10.1038/s41598-021-95811-z
Keywords: trigeminal neuralgia, functional MRI, voxel-based morphometry, multimodal, meta-analysis
Citation: Huang X, Li B, Li Y, Lin J, Shang H and Yang J (2023) A multimodal meta-analysis of gray matter alterations in trigeminal neuralgia. Front. Neurol. 14:1179896. doi: 10.3389/fneur.2023.1179896
Edited by:
Massimiliano Valeriani, Bambino Gesù Children's Hospital (IRCCS), ItalyReviewed by:
Yohannes W. Woldeamanuel, Stanford University, United StatesJuanwei Ma, Tianjin Medical University General Hospital, China
Copyright © 2023 Huang, Li, Li, Lin, Shang and Yang. This is an open-access article distributed under the terms of the Creative Commons Attribution License (CC BY). The use, distribution or reproduction in other forums is permitted, provided the original author(s) and the copyright owner(s) are credited and that the original publication in this journal is cited, in accordance with accepted academic practice. No use, distribution or reproduction is permitted which does not comply with these terms.
*Correspondence: Jing Yang, jingyang_cc@163.com
†ORCID: Jing Yang https://orcid.org/0000-0001-7512-6183
†These authors have contributed equally to this work