- 1Department of Neurobiology, Department of Neurology of Sir Run Run Shaw Hospital, Zhejiang University School of Medicine, Hangzhou, China
- 2NHC and CAMS Key Laboratory of Medical Neurobiology, MOE Frontier Science Center for Brain Research and Brain-Machine Integration, School of Brain Science and Brain Medicine, Zhejiang University, Hangzhou, China
- 3Department of Pharmacy, Sir Run Run Shaw Hospital, School of Medicine, Zhejiang University, Hangzhou, China
- 4Center for Sleep Medicine, Sir Run Run Shaw Hospital, School of Medicine, Zhejiang University, Hangzhou, China
Long-term drug treatment for Restless Legs Syndrome (RLS) patients can frequently result in augmentation, which is the deterioration of symptoms with an increased drug dose. The cause of augmentation, especially derived from dopamine therapy, remains elusive. Here, we review recent research and clinical progress on the possible mechanism underlying RLS augmentation. Dysfunction of the dopamine system highly possibly plays a role in the development of RLS augmentation, as dopamine agonists improve desensitization of dopamine receptors, disturb receptor interactions within or outside the dopamine receptor family, and interfere with the natural regulation of dopamine synthesis and release in the neural system. Iron deficiency is also indicated to contribute to RLS augmentation, as low iron levels can affect the function of the dopamine system. Furthermore, genetic risk factors, such as variations in the BTBD9 and MEIS1 genes, have been linked to an increased risk of RLS initiation and augmentation. Additionally, circadian rhythm, which controls the sleep–wake cycle, may also contribute to the worsening of RLS symptoms and the development of augmentation. Recently, Vitamin D deficiency has been suggested to be involved in RLS augmentation. Based on these findings, we propose that the progressive reduction of selective receptors, influenced by various pathological factors, reverses the overcompensation of the dopamine intensity promoted by short-term, low-dose dopaminergic therapy in the development of augmentation. More research is needed to uncover a deeper understanding of the mechanisms underlying the RLS symptom and to develop effective RLS augmentation treatments.
1. Introduction
Restless legs syndrome, also known as Willis-Ekbom disease (WED), is a sensorimotor disorder characterized by an irresistible urge to move the legs, worsening symptoms at night, and for some patients, unpleasant sensations in the legs. The disorder affects an estimated 1–15.3% of the population, with about 1–3% of people overall experiencing severe and frequent symptoms (1–7). Despite the prevalence of the syndrome, the cause of RLS syndrome remains ambiguous from both clinical and pathophysiological perspectives. Multiple pathological mechanisms, including dysfunction in dopamine-related systems, alterations in adenosine and glutamatergic pathways, brain iron deficiency, and genetic mutations, likely contribute to the etiopathogenesis of RLS (7). The effectiveness of drugs and therapies targeting these mechanisms supports their significant roles in RLS.
Despite the advancements in the development of drug targeting various transmitter pathways, dopaminergic agents(levodopa and dopamine agonists) have remained the mainstay of treatment for restless legs syndrome for the past four decades (7–10). The use of these agents in RLS therapy was initially reported in the 1980s, when Akpinar published a case study documenting the efficacy of levodopa. The long-term efficacy of levodopa and dopaminergic agonists, including pramipexole, ropinirole, and rotigotine, has been well established. Until recent years, they are widely considered the first-line pharmacological treatment for RLS (11–15). However, even with minimal doses of levodopa and dopamine agents, long-term treatment with these drugs leads to a progressive worsening of RLS symptoms (16, 17). This worsening can be differentiated from tolerance, early morning rebound, and the natural progression of RLS or fluctuations in disease severity (18). This deterioration in dopamine therapy, known as augmentation, is the leading cause of treatment discontinuation and failure in RLS (11, 19, 20).
“Augmentation” refers to an iatrogenic exacerbation of RLS symptoms, as characterized by earlier occurrence of symptoms in the afternoon than before treatment initiation, the spread of symptoms to the upper limbs, and a reduced latency until symptoms manifest during periods of rest. Another recognizable characteristic of augmentation is the paradoxical worsening of symptoms upon increasing the dose of dopamine agonists. The clinical phenomenon of augmentation was first observed during long-term levodopa treatment and was suggested to be a result of the treatment itself (21). Augmentation is especially common in patients undergoing levodopa treatment and was initially found in 73% of patient (16). The phenomenon of augmentation is not typically observed in long-term treatment with non-dopamine therapies. If such observations do exist, they are more likely considered a natural progression of RLS rather than an iatrogenic effect (22). However, evaluating the prevalence of augmentation poses challenges due to various influencing factors, including medication type and dosage, study duration and design, assessment criteria, and sample size (11). Specifically, augmentation rates have been found to rise with study duration: short-term studies report rates of 10% (22–26), studies lasting 2–3 years report rates of 15–30%, and studies lasting around 10 years report rates of 42–68% (6, 27, 28). Here, we provide a summary of mechanisms, including dopamine release efficiency, dopamine receptor sensitivity and interaction, genetic risk factors for RLS, iron deficiency, vitamin D deficiency and circadian rhythms. These factors are thought to play a role in the development of augmentation following dopamine agent administration. It can be speculated that the multi-dimensional integration of these pathological elements reverses the relief function of dopamine agents over time.
2. Dysfunction of dopamine system in RLS augmentation
2.1. The contribution of dopamine inefficiency in RLS augmentation
Several studies have supported the increased dopamine synthesis and secretion on the presynaptic surface in RLS patients (29–32). However, the synaptic concentration of endogenous dopamine and the complex postsynaptic signaling system in augmented RLS patients remains unclear. According to the physiological findings, synaptic dopamine concentrations are primarily influenced by presynaptic D2 and D3 autoreceptors and the dopamine transporter (DAT) (33). D2/D3 agonists lead to increased dopamine and serotonin levels in the prefrontal cortex of rats (34). Chronic administration of pramipexole can lead to desensitization of D3 autoreceptors and reduced dopamine uptake in the mouse striatum, which is apparently comparable to RLS patients (35, 36). Hypotheses suggest that augmentation is also linked to impaired dopamine function in the central nervous system. The therapeutic benefits of dopamine agonists on both sensory and motor symptoms, including periodic limb movement in sleep (PLMS), indicate the role of the dopaminergic system in the pathophysiology of RLS, consistent with a presynaptic hyperdopaminergic state. However, the paradoxical changes of receptor function in mild RLS patients, and potentially in augmented RLS patients, need further investigation.
2.2. The contribution of dopamine receptor interactions in RLS augmentation
The interaction between dopamine receptors within the dopamine receptor family and other neurotransmitter systems is implicated in the development of RLS augmentation. Specifically, high dopamine concentrations, possibly resembling the hyperdopaminergic state observed in patients with augmentation, target excitatory dopamine 1 receptors (D1Rs), which sustaining locomotor-like activity in the isolated spinal cord, potentially contributing to augmentation (37). Activation of dopamine 3 receptors (D3Rs) induce both overall sensory and motor excitability in the isolated spinal cord (38, 39), similar to the effect of dopamine agents. Actually, D3Rs and D1Rs form functional heterodimers and heterotetramers (40, 41). Long-term treatment with D3R agonists has also been shown to upregulate excitatory D1Rs in the spinal cord (42). Overall, the downregulation of inhibitory D3Rs and upregulation of excitatory D1Rs seem to contribute to the augmentation of RLS. This propose was partially supported by experiment that long-term treatment with D3R agonists in a rodent model could be reversed or rescued by adjuvant block of D1Rs in animals that no longer responded to the D3R agonist alone (42). The intricate balance of adenosine, dopamine, and glutamate in the striatum may be influenced by the heteromer formed by the combination of D1Rs and dopamine 2 receptors (D2Rs), which interact with adenosine A1 receptors (A1Rs) and A2 receptors (A2ARs), respectively (7). These findings suggest that the dynamic of the pathological network involved in dopamine system in augmented patients may be influenced by internal imbalances of dopamine and extensive neurotransmitter receptors.
2.3. Desensitization of dopamine receptors participate in RLS augmentation
Desensitization of dopamine receptors may also contribute to the development of augmentation. Like other G protein-coupled receptors (GPCRs), dopamine receptors regulate signal transduction by receptor desensitization (43). Recent studies revealed that D1 and D2R homo-oligomers undergo desensitization in response to selective agonists (44, 45). In addition, D3Rs are internalized through a mechanism of pharmacological sequestration after agonist-induced activation (46). It is ponderable that desensitization of dopamine receptors may progressively diminish the efficacy of dopamine agonists in augmented RLS patients, despite low-dose administration temporarily enhancing dopamine receptor signaling. This is especially relevant for RLS patients who receive nighttime treatment with dopamine agonists, as dopamine receptor sensitivity is highest during this time (47). It is considered that the treatment of RLS patients with dopamine agonists may induce pathological desensitization of dopamine receptors, resulting in a gradual reduction in the efficacy of these agents. This vulnerability can be confirmed in self-controlled patients undergoing long-term dopamine therapy.
3. Iron deficiency may contribute to augmentation
The role of iron in RLS pathogenesis has been established through numerous studies, as well as the positive effect of iron supplementation on RLS symptoms. However, the relationship between iron metabolism and RLS augmentation is still unclear. The severity of RLS symptoms at baseline and with augmentation has been associated with low ferritin levels, which suggests a deficiency in mobilizable iron stores (48, 49). Besides, iron supplementation can provide alleviate and remission of RLS symptoms in some patients (50–52) and prevents or reduces of augmentation during dopaminergic therapy (11). Mechanically, iron deficiency may exacerbate RLS severity through dysregulation of dopamine system. Iron serves as a cofactor for tyrosine hydroxylase, which converts tyrosine to dopamine, and oral iron supplement has been found to reduce dopamine transporter numbers in rat models (53). Iron deficiency is also associated with reduced levels of extracellular dopamine, dopamine transporters, D1Rs, and D2Rs (54). Nonetheless, additional research is required to elucidate the pathological mechanism linking iron deficiency, dopamine dysregulation, and RLS augmentation.
4. Genetic risk factors involved in augmentation
The contribution of genetic background to RLS augmentation is poorly understood. The significant physiological and pathological association between genetic risk factors, dopamine dysfunction, and iron metabolism suggests that RLS risk factors contribute to the development of augmentation. The MEIS1 and BTBD9 loci have a significant genetic association with RLS (55) and are involved in brain iron metabolism (56). Overexpression of BTBD9 in HEK cells increased ferritin expression in embryonic kidney cells (57). A previous study conducted in Denmark found that the rs9296249 variant in the BTBD9 gene was significantly associated with serum ferritin levels in female blood donors (58). On the other hand, an association between the MEIS1 gene and an increase in the expression of H-ferritin, L-ferritin, and divalent metal transporter-1 RNA has been uncovered in the thalamus (59).
BTBD9 and its homolog are involved in the transcriptional and cellular regulation of dopamine D2Rs and D3Rs, which are the main targets of dopamine agonists. The internalization of D2R is modulated by DNM-1 (60), which showed a significant increase in BTBD9 systematic knockout mice. The expression of DNM-1 was found to be enhanced in the BTBD9 complete knockout mice compared to wild-type mice that were euthanized at midnight (61). The symptoms of RLS patients and the thermal sensory deficit of BTBD9 complete knockout mice can be alleviated by D2/D3 agonists (62). A research using dBTBD9 mutant flies showed a significant decrease in brain dopamine levels and an incoordinate sleep phenotype, which was majorly rescued by giving pramipexole (57). In contrast, the increased sensory excitability and locomotor activity in D3KO mice suggest that both sensory and motor circuits are functionally upregulated in the spinal cord of this mouse (63). In both D3KO and iron-deprived mice, a significant increase of the D1R protein expression has been found in the lumbar spinal cord (64, 65). Under BTBD9 deficiency, the activity of the D1R-mediated dopamine system may have reached its peak, which indicated a weakened D2R signaling but an increased D1R signaling in RLS syndrome (61), similar to augmentation states. In addition, a reasonable viewpoint was that BTBD9 is involved in dopamine biosynthesis by unknown pathways (66). Therefore, RLS patients with risk BTBD9 loci may have a tendency toward augmentation in the pathological process.
5. Vitamin D and RLS augmentation
Vitamin D deficiency has been shown to correlate with RLS symptoms on serum vitamin D level (67, 68). Vitamin D is also associated with pathological factors of RLS, such as iron deficiency (68, 69) and dopamine dysfunction (70, 71). The effect of vitamin D on RLS augmentation has been reported. A case report showed that an 81-year-old woman’s dopaminergic augmentation significantly improved result from the co-treatment involved in vitamin D supplementation (72). Besides, an unpublished study revealed a high prevalence of vitamin D deficiency in 9 female RLS patients using dopaminergic drugs, and these females showed an improved response to vitamin D supplementation (73).
6. Circadian rhythms and RLS augmentation
RLS symptoms are more apparent at night, with a higher occurrence reported in the evening and night hours (74). Indeed, a phase advance of anticipated initiation of RLS symptoms, known as a feature of RLS augmentation, was possibly influenced by circadian rhythm. A study indicates that treatment with levodopa advances the dim light melatonin onset (DLMO) in RLS patients experiencing augmentation compared to those without augmentation (75). Moreover, considering the deterioration of RLS symptoms in cases of augmentation, the intensification of augmented symptoms is particularly evident at night. Specifically, unlike akathisia, paresthesias in RLS are typically localized, usually idiopathic, and have a periodic maximum of expression in dusk and night (76). Therefore, given the fluctuation of molecular expression in RLS and the evidence for chronobiotic mechanisms in the disease, the potential role of circadian mechanisms in the RLS deterioration cannot be ignored. (77, 78).
Pathological factors involved in augmentation, such as abnormal dopamine system dynamics, genetic risk factors expression, and iron concentration, display diurnal rhythmicity. This view suggests that the timing of dopamine agonist treatment may influence development of RLS augmentation through pathological networks. Dopamine release has an unambiguous circadian activity pattern of decreasing in the evening and night and increasing in the morning (79–83). In wild-type mice, D1R, D2L, and D2S mRNA levels were all decreased during the locomotion time compared with the sleep time (61). The level of D2R mRNA was increased in the striatum of BTBD9 complete knockout at midnight, indicating that the effect of BTBD9 deficiency on the D2R mRNA level is regulated by circadian rhythm (84). Arrhythmic transcription of dopamine receptor mRNAs may contribute to these findings. Additionally, diurnal variations of iron concentration have been observed in peripheral and regional brain areas (85). Significant diurnal changes in total iron concentrations in brain region were found in mice (86). Diurnal variations of iron-regulated proteins in CSF have been previously described in RLS patients (87). Research on fruit flies has found that the neural circuit connection from the small ventrolateral clock neurons to the protocerebrum are reduced when the iron is chelated from the diet (88). In terms of the genetic factors of RLS, although a study has shown that BTBD9 mutant mice exhibit abnormal sleep architecture, which is reminiscent of RLS patients (62), it is yet to be determined if BTBD9 variation impacts circadian. On the other hand, MEIS1 haploinsufficiency was associated with a sex-dependent increase in activity during the onset of rest, similar to the circadian rhythm of RLS symptoms observed in human patients (89).
7. Model of augmentation development
Overall, the effect of dopamine agents, potentially accompanied with iron deficiency, genetic risk factors, vitamin D deficiency and effect of circadian rhythms, contributes to the attenuation of dopamine signaling in patients with augmented RLS. RLS patients may exhibit impaired postsynaptic response to increased dopamine stimulation after long-term administration of low-dose dopamine agents, possibly due to dopamine receptor desensitization and interaction of dopamine receptors. When dopamine signaling is reduced during dusk and night, this insufficient postsynaptic dopamine signaling causes augmented RLS symptoms. More specifically, despite an overall increase in dopamine secretion in RLS patients, continuous treatment with dopamine agents may desensitize selective dopamine receptors, with a gradual decrease in peak dopamine efficiency over time. This leads to a deficit in dopamine signaling in the evening and at night compared with healthy people. As a result, the circadian attenuation of dopamine signaling in the evening and at night in augmented RLS patients disrupts normal sleep homeostasis, with the symptom of fragmented sleep at rest in these patients. The dopamine drug-induced incremental activation of dopamine signaling exacerbates the downregulation of overall post-synaptic dopamine receptors, thus worsening the RLS disease and expanding the symptoms (Figure 1).
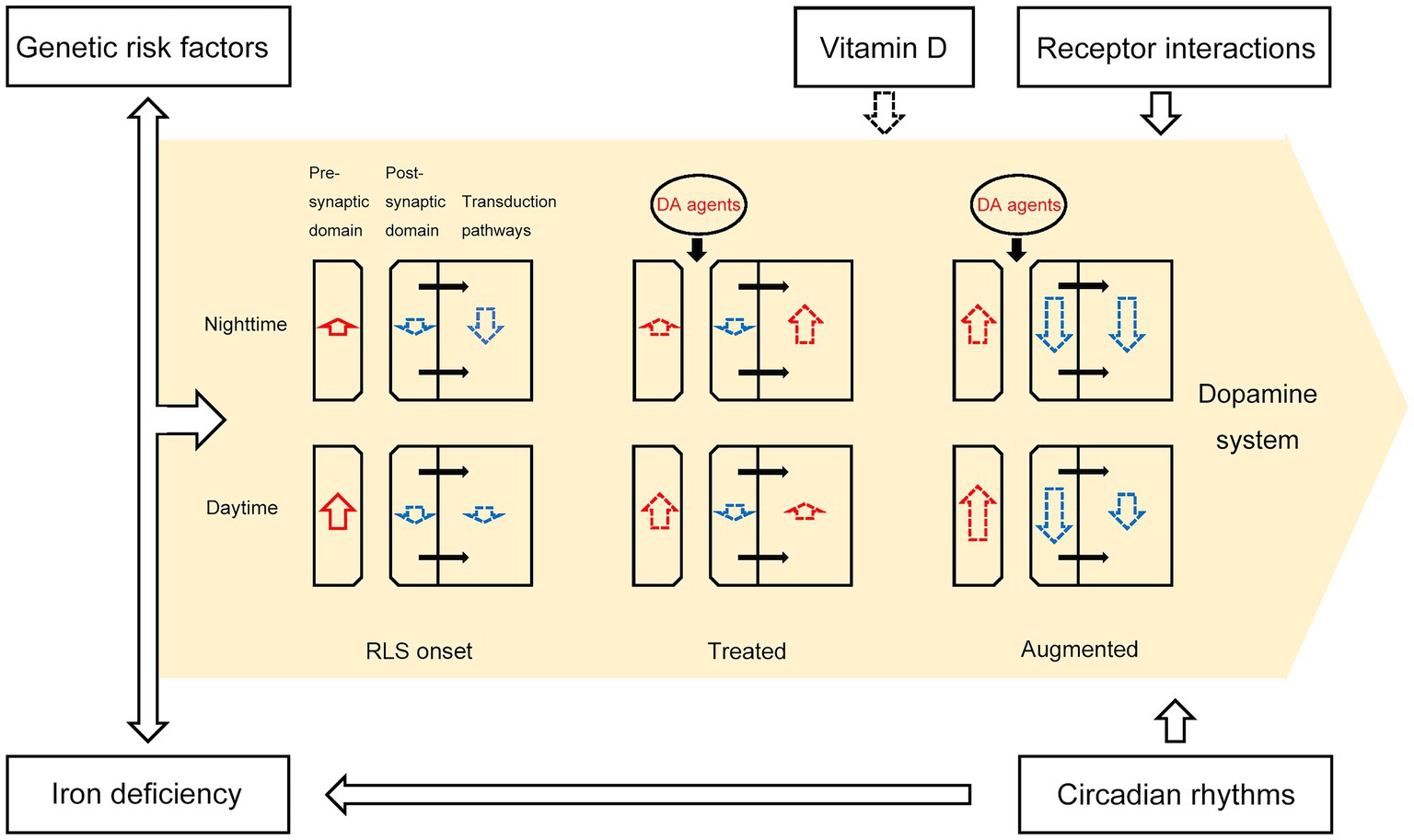
Figure 1. A schematic depicting the pathological model of RLS augmentation. The intensities of upregulation (length of red arrows) and downregulation (length of blue arrows) in presynaptic and postsynaptic DA signaling, as well as the signal strength of DAR downstream transduction pathways, changed during RLS augmentation. The black arrows within the yellow region represent biological processes between the post-synaptic domain and transduction pathways. Iron deficiency, genetic risk factors, receptor interactions, vitamin D, and circadian rhythms contribute to dopamine system dysfunction, as depicted by the black arrows outside the yellow region. Dopaminergic agents such as levodopa, pramipexole, ropinirole, and rotigotine temporarily increase the overall effect of dopamine on postsynaptic receptors, but irreversibly reduce the number of dopamine receptors. Dotted black or colored arrows indicate potential relationships between two entities.
8. Conclusion
Augmentation is one of the greatest threats to RLS patients in the context of the widespread usage of dopamine agents. The pathological mechanism behind this symptom is unclear and needs to be elucidated. Current clinical diagnoses and research findings highlight the potential mechanisms linking dopamine dysfunction, circadian regulation, iron deficiency, vitamin D deficiency and genetic risk factors to the development of augmentation. However, a multi-perspective investigation in the field is lacking. In addition, the role of other transmitter signaling pathways in the development of augmentation remains vague. Further careful and long-term research is necessary to fully understand the underlying mechanisms of augmentation in RLS patients.
Author contributions
PZ contributed to the original concept of the article, did the literature search, wrote the original version of the manuscript, drew the figure and reviewed it as it progressed. TW, LZ, and FG contributed to the original concept of the manuscript and reviewed the manuscript. All authors contributed to the article and approved the submitted version.
Conflict of interest
The authors declare that the research was conducted in the absence of any commercial or financial relationships that could be construed as a potential conflict of interest.
Publisher’s note
All claims expressed in this article are solely those of the authors and do not necessarily represent those of their affiliated organizations, or those of the publisher, the editors and the reviewers. Any product that may be evaluated in this article, or claim that may be made by its manufacturer, is not guaranteed or endorsed by the publisher.
References
1. Migueis, DP, Lopes, MC, Casella, E, Soares, PV, Soster, L, and Spruyt, K. Attention deficit hyperactivity disorder and restless leg syndrome across the lifespan: a systematic review and meta-analysis. Sleep Med Rev. (2023) 69:101770. doi: 10.1016/j.smrv.2023.101770
2. Allen, RP, Walters, AS, Montplaisir, J, Hening, W, Myers, A, Bell, TJ, et al. Restless legs syndrome prevalence and impact: REST general population study. Arch Intern Med. (2005) 165:1286–92. doi: 10.1001/archinte.165.11.1286
3. Cho, S-J, Hong, JP, Hahm, B-J, Jeon, HJ, Chang, SM, Cho, MJ, et al. Restless legs syndrome in a community sample of Korean adults: prevalence, impact on quality of life, and association with DSM-IV psychiatric disorders. Sleep. (2009) 32:1069–76. doi: 10.1093/sleep/32.8.1069
4. Trenkwalder, C, and Paulus, W. Restless legs syndrome: pathophysiology, clinical presentation and management. Nat Rev Neurol. (2010) 6:337–46. doi: 10.1038/nrneurol.2010.55
5. Yilmaz, K, Kilincaslan, A, Aydin, N, and Kor, D. Prevalence and correlates of restless legs syndrome in adolescents. Dev Med Child Neurol. (2011) 53:40–7. doi: 10.1111/j.1469-8749.2010.03796.x
6. Wang, T, Ying, M, Zhao, R, Zhu, D, and Zhang, L. Augmentation in patients with restless legs syndrome receiving pramipexole therapy: a retrospective study in a single center from China. Sleep Breath. (2022) 26:373–80. doi: 10.1007/s11325-021-02353-9
7. Manconi, M, Garcia-Borreguero, D, Schormair, B, Videnovic, A, Berger, K, Ferri, R, et al. Restless legs syndrome. Nat Rev Dis Primers. (2021) 7:80. doi: 10.1038/s41572-021-00311-z
8. Garcia-Borreguero, D, and Cano-Pumarega, I. New concepts in the management of restless legs syndrome. BMJ. (2017) 356:j104. doi: 10.1136/bmj.j104
9. Scholz, H, Trenkwalder, C, Kohnen, R, Riemann, D, Kriston, L, and Hornyak, M. Dopamine agonists for restless legs syndrome. Cochrane Database Syst Rev. (2011) 2011:CD006009. doi: 10.1002/14651858.CD006009.pub2
10. Winkelman, JW, Armstrong, MJ, Allen, RP, Chaudhuri, KR, Ondo, W, Trenkwalder, C, et al. Practice guideline summary: treatment of restless legs syndrome in adults: report of the guideline development, dissemination, and implementation Subcommittee of the American Academy of neurology. Neurology. (2016) 87:2585–93. doi: 10.1212/WNL.0000000000003388
11. Garcia-Borreguero, D, Silber, MH, Winkelman, JW, Högl, B, Bainbridge, J, Buchfuhrer, M, et al. Guidelines for the first-line treatment of restless legs syndrome/Willis–Ekbom disease, prevention and treatment of dopaminergic augmentation: a combined task force of the IRLSSG, EURLSSG, and the RLS-foundation. Sleep Med. (2016) 21:1–11. doi: 10.1016/j.sleep.2016.01.017
12. Fleishon, H, Mandel, S, and Arenas, A. Anterior spinal artery syndrome after cervical injection of heroin. Arch Neurol. (1982) 39:739. doi: 10.1001/archneur.1982.00510230065026
13. Garcia-Borreguero, D, Grunstein, R, Sridhar, G, Dreykluft, T, Montagna, P, Dom, R, et al. A 52-week open-label study of the long-term safety of ropinirole in patients with restless legs syndrome. Sleep Med. (2007) 8:742–52. doi: 10.1016/j.sleep.2006.09.009
14. Montplaisir, J, Karrasch, J, Haan, J, and Volc, D. Ropinirole is effective in the long-term management of restless legs syndrome: a randomized controlled trial. Mov Disord. (2006) 21:1627–35. doi: 10.1002/mds.21050
15. Silber, MH, Ehrenberg, BL, Allen, RP, Buchfuhrer, MJ, Earley, CJ, Hening, WA, et al. An algorithm for the Management of Restless Legs Syndrome. Mayo Clin Proc. (2004) 79:916–22. doi: 10.4065/79.7.916
16. Silber, MH, Buchfuhrer, MJ, Earley, CJ, Koo, BB, Manconi, M, Winkelman, JW, et al. The Management of Restless Legs Syndrome: an updated algorithm. Mayo Clin Proc. (2021) 96:1921–37. doi: 10.1016/j.mayocp.2020.12.026
17. Trenkwalder, C, and Paulus, W. Pharmacological treatments of augmentation in restless legs syndrome patients. Adv Pharmacol. (2019) 84:255–65. doi: 10.1016/bs.apha.2019.02.002
18. García-Borreguero, D. Dopaminergic augmentation in restless legs syndrome/Willis-Ekbom disease: identification and management. Sleep Med Clin. (2015):10:287–292, xiii. doi: 10.1016/j.jsmc.2015.05.020
19. Garcia-Borreguero, D, Cano-Pumarega, I, and Marulanda, R. Management of treatment failure in restless legs syndrome (Willis-Ekbom disease). Sleep Med Rev. (2018) 41:50–60. doi: 10.1016/j.smrv.2018.01.001
20. Khachatryan, SG, Ferri, R, Fulda, S, Garcia-Borreguero, D, Manconi, M, Muntean, M-L, et al. Restless legs syndrome: over 50 years of European contribution. J Sleep Res. (2022) 31:e13632. doi: 10.1111/jsr.13632
21. García-Borreguero, D, and Williams, A-M. Dopaminergic augmentation of restless legs syndrome. Sleep Med Rev. (2010) 14:339–46. doi: 10.1016/j.smrv.2009.11.006
22. Allen, RP, Chen, C, Garcia-Borreguero, D, Polo, O, DuBrava, S, Miceli, J, et al. Comparison of Pregabalin with Pramipexole for restless legs syndrome. N Engl J Med. (2014) 370:621–31. doi: 10.1056/NEJMoa1303646
23. Allen, RP, Ondo, WG, Ball, E, Calloway, MO, Manjunath, R, Higbie, RL, et al. Restless legs syndrome (RLS) augmentation associated with dopamine agonist and levodopa usage in a community sample. Sleep Med. (2011) 12:431–9. doi: 10.1016/j.sleep.2011.03.003
24. García-Borreguero, D, Högl, B, Ferini-Strambi, L, Winkelman, J, Hill-Zabala, C, Asgharian, A, et al. Systematic evaluation of augmentation during treatment with ropinirole in restless legs syndrome (Willis-Ekbom disease): results from a prospective, multicenter study over 66 weeks. Mov Disord. (2012) 27:277–83. doi: 10.1002/mds.24889
25. Högl, B, Garcia-Borreguero, D, Trenkwalder, C, Ferini-Strambi, L, Hening, W, Poewe, W, et al. Efficacy and augmentation during 6months of double-blind pramipexole for restless legs syndrome. Sleep Med. (2011) 12:351–60. doi: 10.1016/j.sleep.2010.12.007
26. Oertel, WH, Benes, H, Garcia-Borreguero, D, Geisler, P, Högl, B, Trenkwalder, C, et al. One year open-label safety and efficacy trial with rotigotine transdermal patch in moderate to severe idiopathic restless legs syndrome. Sleep Med. (2008) 9:865–73. doi: 10.1016/j.sleep.2008.04.012
27. Garcia-Borreguero, D, Cano-Pumarega, I, Garcia Malo, C, Cruz Velarde, JA, Granizo, JJ, and Wanner, V. Reduced response to gabapentin enacarbil in restless legs syndrome following long-term dopaminergic treatment. Sleep Med. (2019) 55:74–80. doi: 10.1016/j.sleep.2018.11.025
28. Silver, N, Allen, RP, Senerth, J, and Earley, CJ. A 10-year, longitudinal assessment of dopamine agonists and methadone in the treatment of restless legs syndrome. Sleep Med. (2011) 12:440–4. doi: 10.1016/j.sleep.2010.11.002
29. Earley, CJ, Hyland, K, and Allen, RP. CSF dopamine, serotonin, and biopterin metabolites in patients with restless legs syndrome. Mov Disord. (2001) 16:144–9. doi: 10.1002/1531-8257(200101)16:1<144::AID-MDS1009>3.0.CO;2-F
30. Earley, CJ, Connor, J, Garcia-Borreguero, D, Jenner, P, Winkelman, J, Zee, PC, et al. Altered brain iron homeostasis and dopaminergic function in restless legs syndrome (Willis-Ekbom disease). Sleep Med. (2014) 15:1288–301. doi: 10.1016/j.sleep.2014.05.009
31. Connor, JR, Wang, X-S, Allen, RP, Beard, JL, Wiesinger, JA, Felt, BT, et al. Altered dopaminergic profile in the putamen and substantia nigra in restless leg syndrome. Brain. (2009) 132:2403–12. doi: 10.1093/brain/awp125
32. Salminen, AV, Clemens, S, García-Borreguero, D, Ghorayeb, I, Li, Y, Manconi, M, et al. Consensus guidelines on the construct validity of rodent models of restless legs syndrome. Dis Model Mech. (2022) 15:dmm049615. doi: 10.1242/dmm.049615
33. Chang, P-K, Chien, K-Y, and Chen, J-C. Dopamine transporter is downregulated and its association with chaperone protein Hsc70 is enhanced by activation of dopamine D3 receptor. Brain Res Bull. (2020) 165:263–71. doi: 10.1016/j.brainresbull.2020.10.005
34. Chernoloz, O, El Mansari, M, and Blier, P. Long-term administration of the dopamine D3/2 receptor agonist pramipexole increases dopamine and serotonin neurotransmission in the male rat forebrain. J Psychiatry Neurosci. (2012) 37:113–21. doi: 10.1503/jpn.110038
35. Castro-Hernández, J, Afonso-Oramas, D, Cruz-Muros, I, Salas-Hernández, J, Barroso-Chinea, P, Moratalla, R, et al. Prolonged treatment with pramipexole promotes physical interaction of striatal dopamine D3 autoreceptors with dopamine transporters to reduce dopamine uptake. Neurobiol Dis. (2015) 74:325–35. doi: 10.1016/j.nbd.2014.12.007
36. Luis-Ravelo, D, Fumagallo-Reading, F, Castro-Hernandez, J, Barroso-Chinea, P, Afonso-Oramas, D, Febles-Casquero, A, et al. Prolonged dopamine D3 receptor stimulation promotes dopamine transporter ubiquitination and degradation through a PKC-dependent mechanism. Pharmacol Res. (2021) 165:105434. doi: 10.1016/j.phrs.2021.105434
37. Allen, RP. Restless leg syndrome/Willis-Ekbom disease pathophysiology. Sleep Med Clin. (2015) 10:207–14. doi: 10.1016/j.jsmc.2015.05.022
38. Keeler, BE, Baran, CA, Brewer, KL, and Clemens, S. Increased excitability of spinal pain reflexes and altered frequency-dependent modulation in the dopamine D3-receptor knockout mouse. Exp Neurol. (2012) 238:273–83. doi: 10.1016/j.expneurol.2012.09.002
39. Sharples, SA, Humphreys, JM, Jensen, AM, Dhoopar, S, Delaloye, N, Clemens, S, et al. Dopaminergic modulation of locomotor network activity in the neonatal mouse spinal cord. J Neurophysiol. (2015) 113:2500–10. doi: 10.1152/jn.00849.2014
40. Cruz-Trujillo, R, Avalos-Fuentes, A, Rangel-Barajas, C, Paz-Bermúdez, F, Sierra, A, Escartín-Perez, E, et al. D3 dopamine receptors interact with dopamine D1 but not D4 receptors in the GABAergic terminals of the SNr of the rat. Neuropharmacology. (2013) 67:370–8. doi: 10.1016/j.neuropharm.2012.11.032
41. Guitart, X, Navarro, G, Moreno, E, Yano, H, Cai, N-S, Sánchez-Soto, M, et al. Functional selectivity of allosteric interactions within G protein–coupled receptor oligomers: the dopamine D1-D3 receptor Heterotetramer. Mol Pharmacol. (2014) 86:417–29. doi: 10.1124/mol.114.093096
42. Dinkins, M-L, Lallemand, P, and Clemens, S. Long-term treatment with dopamine D3 receptor agonists induces a behavioral switch that can be rescued by blocking the dopamine D1 receptor. Sleep Med. (2017) 40:47–52. doi: 10.1016/j.sleep.2017.10.001
43. Gainetdinov, RR, Premont, RT, Bohn, LM, Lefkowitz, RJ, and Caron, MG. Desensitization of G protein-coupled receptors and neuronal functions. Annu Rev Neurosci. (2004) 27:107–44. doi: 10.1146/annurev.neuro.27.070203.144206
44. Lamey, M, Thompson, M, Varghese, G, Chi, H, Sawzdargo, M, George, SR, et al. Distinct residues in the carboxyl tail mediate agonist-induced desensitization and internalization of the human dopamine D1 receptor. J Biol Chem. (2002) 277:9415–21. doi: 10.1074/jbc.M111811200
45. Ng, GY, Varghese, G, Chung, HT, Trogadis, J, Seeman, P, O’Dowd, BF, et al. Resistance of the dopamine D2L receptor to desensitization accompanies the up-regulation of receptors on to the surface of Sf9 cells. Endocrinology. (1997) 138:4199–206. doi: 10.1210/endo.138.10.5433
46. Xu, W, Reith, MEA, Liu-Chen, L-Y, and Kortagere, S. Biased signaling agonist of dopamine D3 receptor induces receptor internalization independent of β-arrestin recruitment. Pharmacol Res. (2019) 143:48–57. doi: 10.1016/j.phrs.2019.03.003
47. Garcia-Borreguero, D, Larrosa, O, Granizo, JJ, de la Llave, Y, and Hening, WA. Circadian variation in neuroendocrine response to L-dopa in patients with restless legs syndrome. Sleep. (2004) 27:669–73. doi: 10.1093/sleep/27.4.669
48. Çurgunlu, A, Döventaş, A, Karadeniz, D, Erdinçler, DS, Oztürk, AK, Karter, Y, et al. Prevalence and characteristics of restless legs syndrome (RLS) in the elderly and the relation of serum ferritin levels with disease severity: hospital-based study from Istanbul, Turkey. Arch Gerontol Geriatr. (2012) 55:73–6. doi: 10.1016/j.archger.2011.06.002
49. Trenkwalder, C, Högl, B, Benes, H, and Kohnen, R. Augmentation in restless legs syndrome is associated with low ferritin. Sleep Med. (2008) 9:572–4. doi: 10.1016/j.sleep.2007.07.020
50. Avni, T, Reich, S, Lev, N, and Gafter-Gvili, A. Iron supplementation for restless legs syndrome - a systematic review and meta-analysis. Eur J Intern Med. (2019) 63:34–41. doi: 10.1016/j.ejim.2019.02.009
51. Bae, H, Cho, YW, Kim, KT, Allen, RP, and Earley, CJ. Randomized, placebo-controlled trial of ferric carboxymaltose in restless legs syndrome patients with iron deficiency anemia. Sleep Med. (2021) 84:179–86. doi: 10.1016/j.sleep.2021.05.036
52. Yang, X, Yang, B, Ming, M, Li, S, Wang, F, Zhu, Z, et al. Efficacy and tolerability of intravenous iron for patients with restless legs syndrome: evidence from randomized trials and observational studies. Sleep Med. (2019) 61:110–7. doi: 10.1016/j.sleep.2019.01.040
53. LaBarbera, V, Trotti, LM, and Rye, D. Restless legs syndrome with augmentation successfully treated with IV iron. Neurol Clin Pract. (2017) 7:e26–8. doi: 10.1212/CPJ.0000000000000276
54. Dauvilliers, Y, and Winkelmann, J. Restless legs syndrome: update on pathogenesis. Curr Opin Pulm Med. (2013) 19:594–600. doi: 10.1097/MCP.0b013e328365ab07
55. Schormair, B, Zhao, C, Bell, S, Tilch, E, Salminen, AV, Pütz, B, et al. Identification of novel risk loci for restless legs syndrome in genome-wide association studies in individuals of European ancestry: a meta-analysis. Lancet Neurol. (2017) 16:898–907. doi: 10.1016/S1474-4422(17)30327-7
56. Gonzalez-Latapi, P, and Malkani, R. Update on restless legs syndrome: from mechanisms to treatment. Curr Neurol Neurosci Rep. (2019) 19:54. doi: 10.1007/s11910-019-0965-4
57. Freeman, A, Pranski, E, Miller, RD, Radmard, S, Bernhard, D, Jinnah, HA, et al. Sleep fragmentation and motor restlessness in a Drosophila model of restless legs syndrome. Curr Biol. (2012) 22:1142–8. doi: 10.1016/j.cub.2012.04.027
58. Sørensen, E, Grau, K, Berg, T, Simonsen, AC, Magnussen, K, Erikstrup, C, et al. A genetic risk factor for low serum ferritin levels in Danish blood donors. Transfusion. (2012) 52:2585–9. doi: 10.1111/j.1537-2995.2012.03629.x
59. Catoire, H, Dion, PA, Xiong, L, Amari, M, Gaudet, R, Girard, SL, et al. Restless legs syndrome-associated MEIS1 risk variant influences iron homeostasis. Ann Neurol. (2011) 70:170–5. doi: 10.1002/ana.22435
60. Iwata, K, Ito, K, Fukuzaki, A, Inaki, K, and Haga, T. Dynamin and rab5 regulate GRK2-dependent internalization of dopamine D2 receptors. Eur J Biochem. (1999) 263:596–602. doi: 10.1046/j.1432-1327.1999.00549.x
61. Lyu, S, Doroodchi, A, Xing, H, Sheng, Y, DeAndrade, MP, Yang, Y, et al. BTBD9 and dopaminergic dysfunction in the pathogenesis of restless legs syndrome. Brain Struct Funct. (2020) 225:1743–60. doi: 10.1007/s00429-020-02090-x
62. DeAndrade, MP, Johnson, RL, Unger, EL, Zhang, L, van Groen, T, Gamble, KL, et al. Motor restlessness, sleep disturbances, thermal sensory alterations and elevated serum iron levels in Btbd9 mutant mice. Hum Mol Genet. (2012) 21:3984–92. doi: 10.1093/hmg/dds221
63. Meneely, S, Dinkins, M-L, Kassai, M, Lyu, S, Liu, Y, Lin, C-T, et al. Differential dopamine D1 and D3 receptor modulation and expression in the spinal cord of two mouse models of restless legs syndrome. Front Behav Neurosci. (2018) 12:199. doi: 10.3389/fnbeh.2018.00199
64. Brewer, KL, Baran, CA, Whitfield, BR, Jensen, AM, and Clemens, S. Dopamine D3 receptor dysfunction prevents anti-nociceptive effects of morphine in the spinal cord. Front Neural Circuits. (2014) 8:62. doi: 10.3389/fncir.2014.00062
65. Zhao, H, Zhu, W, Pan, T, Xie, W, Zhang, A, Ondo, WG, et al. Spinal cord dopamine receptor expression and function in mice with 6-OHDA lesion of the a11 nucleus and dietary iron deprivation. J Neurosci Res. (2007) 85:1065–76. doi: 10.1002/jnr.21207
66. Shaw, PJ, and Duntley, SP. Neurological disorders: towards a mechanistic understanding of restless legs syndrome. Curr Biol. (2012) 22:R485–6. doi: 10.1016/j.cub.2012.05.004
67. Wali, S, Shukr, A, Boudal, A, Alsaiari, A, and Krayem, A. The effect of vitamin D supplements on the severity of restless legs syndrome. Sleep Breath. (2015) 19:579–83. doi: 10.1007/s11325-014-1049-y
68. Wali, S, Alsafadi, S, Abaalkhail, B, Ramadan, I, Abulhamail, B, Kousa, M, et al. The association between vitamin D level and restless legs syndrome: a population-based case-control study. J Clin Sleep Med. (2018) 14:557–64. doi: 10.5664/jcsm.7044
69. Smith, EM, and Tangpricha, V. Vitamin D and anemia: insights into an emerging association. Curr Opin Endocrinol Diabetes Obes. (2015) 22:432–8. doi: 10.1097/MED.0000000000000199
70. Baksi, SN, and Hughes, MJ. Chronic vitamin D deficiency in the weanling rat alters catecholamine metabolism in the cortex. Brain Res. (1982) 242:387–90. doi: 10.1016/0006-8993(82)90331-6
71. Oran, M, Unsal, C, Albayrak, Y, Tulubas, F, Oguz, K, Avci, O, et al. Possible association between vitamin D deficiency and restless legs syndrome. Neuropsychiatr Dis Treat. (2014) 10:953–8. doi: 10.2147/NDT.S63599
72. Department of Neurology, Near East University School of Medicine, Nicosia, Cyprus, Diker S. Restless leg syndrome: role of Iron and vitamin D deficiencies. Cyprus. J Med Sci. (2018):114–6. doi: 10.5152/cjms.2018.347
73. Cederberg, KLJ, Silvestri, R, and Walters, AS. Vitamin D and restless legs syndrome: a review of current literature. Tremor Other Hyperkinet Mov (N Y). (2023) 13:12. doi: 10.5334/tohm.741
74. Garcia Borreguero, D, Winkelmann, J, and Allen, RP. Introduction: towards a better understanding of the science of RLS/WED. Sleep Med. (2017) 31:1–2. doi: 10.1016/j.sleep.2016.10.007
75. Garcia-Borreguero, D, Serrano, C, Larrosa, O, and Granizo, JJ. Circadian effects of dopaminergic treatment in restless legs syndrome. Sleep Med. (2004) 5:413–20. doi: 10.1016/j.sleep.2004.01.007
76. Hening, WA. Restless legs syndrome: a sensorimotor disorder of sleep/wake motor regulation. Curr Neurol Neurosci Rep. (2002) 2:186–96. doi: 10.1007/s11910-002-0029-y
77. Garcia-Borreguero, D, Larrosa, O, and de la Llave, Y. Circadian aspects in the pathophysiology of the restless legs syndrome. Sleep Med. (2002) 3:S17–21. doi: 10.1016/s1389-9457(02)00143-0
78. Michaud, M, Dumont, M, Selmaoui, B, Paquet, J, Livia Fantini, M, and Montplaisir, J. Circadian rhythm of restless legs syndrome: relationship with biological markers. Ann Neurol. (2004) 55:372–80. doi: 10.1002/ana.10843
79. Chung, S, Lee, EJ, Yun, S, Choe, HK, Park, S-B, Son, HJ, et al. Impact of circadian nuclear receptor REV-ERBα on midbrain dopamine production and mood regulation. Cells. (2014) 157:858–68. doi: 10.1016/j.cell.2014.03.039
80. Earley, CJ, Hyland, K, and Allen, RP. Circadian changes in CSF dopaminergic measures in restless legs syndrome. Sleep Med. (2006) 7:263–8. doi: 10.1016/j.sleep.2005.09.006
81. Khaldy, H, León, J, Escames, G, Bikjdaouene, L, García, JJ, and Acuña-Castroviejo, D. Circadian rhythms of dopamine and dihydroxyphenyl acetic acid in the mouse striatum: effects of pinealectomy and of melatonin treatment. Neuroendocrinology. (2002) 75:201–8. doi: 10.1159/000048238
82. Liang, X, Holy, TE, and Taghert, PH. Polyphasic circadian neural circuits drive differential activities in multiple downstream rhythmic centers (2022) 33:351–63. doi: 10.1101/2022.10.11.511837
83. Tang, Q, Assali, DR, Güler, AD, and Steele, AD. Dopamine systems and biological rhythms: Let’s get a move on. Front Integr Neurosci. (2022) 16:957193. doi: 10.3389/fnint.2022.957193
84. Akhisaroglu, M, Kurtuncu, M, Manev, H, and Uz, T. Diurnal rhythms in quinpirole-induced locomotor behaviors and striatal D2/D3 receptor levels in mice. Pharmacol Biochem Behav. (2005) 80:371–7. doi: 10.1016/j.pbb.2004.11.016
85. Unger, EL, Earley, CJ, and Beard, JL. Diurnal cycle influences peripheral and brain iron levels in mice. J Appl Physiol. ((1985) (2009)) 106:187–93. doi: 10.1152/japplphysiol.91076.2008
86. Unger, EL, Jones, BC, Bianco, LE, Allen, RP, and Earley, CJ. Diurnal variations in brain iron concentrations in BXD RI mice. Neuroscience. (2014) 263:54–9. doi: 10.1016/j.neuroscience.2013.12.056
87. Earley, CJ, Ponnuru, P, Wang, X, Patton, SM, Conner, JR, Beard, JL, et al. Altered iron metabolism in lymphocytes from subjects with restless legs syndrome. Sleep. (2008) 31:847–52. doi: 10.1093/sleep/31.6.847
88. Rudisill, SS, Martin, BR, Mankowski, KM, and Tessier, CR. Iron deficiency reduces synapse formation in the Drosophila clock circuit. Biol Trace Elem Res. (2019) 189:241–50. doi: 10.1007/s12011-018-1442-7
Keywords: restless legs syndrome, augmentation, dopamine agonists, iron deficiency, circadian rhythms
Citation: Zeng P, Wang T, Zhang L and Guo F (2023) Exploring the causes of augmentation in restless legs syndrome. Front. Neurol. 14:1160112. doi: 10.3389/fneur.2023.1160112
Edited by:
Anna Szucs, Queen Victoria Hospital, United KingdomReviewed by:
Ambra Stefani, Massachusetts General Hospital and Harvard Medical School, United StatesRoselyne Rijsman, Haaglanden Medical Center, Netherlands
Yuichi Inoue, Tokyo Medical University, Japan
Copyright © 2023 Zeng, Wang, Zhang and Guo. This is an open-access article distributed under the terms of the Creative Commons Attribution License (CC BY). The use, distribution or reproduction in other forums is permitted, provided the original author(s) and the copyright owner(s) are credited and that the original publication in this journal is cited, in accordance with accepted academic practice. No use, distribution or reproduction is permitted which does not comply with these terms.
*Correspondence: Fang Guo, gfang@zju.edu.cn; Lisan Zhang, zls09@zju.edu.cn