- 1Department of Ophthalmology, The First Affiliated Hospital of Nanchang University, Jiangxi Branch of National Clinical Research Center for Ocular Disease, Nanchang, China
- 2Department of Orthopaedics, The Second Affiliated Hospital of Shanxi Medical University, Taiyuan, China
Background: To date, no in-depth study has been conducted on the intrinsic pathological relationship between altered brain activity and related behavioral changes in patients with orbital fracture (OF).
Purpose: The present research aimed to explore the potential functional network cerebrum activities in patients with OF using resting state functional magnetic resonance imaging–fractional amplitude of low-frequency fluctuation (rsfMRI-fALFF). This technique can reveal dynamic functional changes in specific cerebrum areas.
Methods: Twenty patients with OF and 20 healthy controls (HCs) were included, closely matched in terms of gender, age, weight, and education level. To record spontaneous cerebral activity changes, the rsfMRI-fALFF tool was applied. Receiver operating characteristic (ROC) curves and Pearson's correlation analysis were used to analyze mean fALFF values in specific cerebrum regions and to explore changes of behavioral changes in patients with OF. The Hospital Depression and Anxiety scale was applied to reveal the relationship between emotional states and fALFF values of the right superior temporal gyrus in patients with OF.
Results: In comparison with HCs, significantly lower fALFF values were detected in the left anterior cingulate gyrus (LACG) and right superior temporal gyrus (RSTG) in patients with OF. ROC curve analysis showed excellent accuracy. The mean fALFF values of the RSTG negatively correlated with the depression score as well as the anxiety score.
Conclusion: The finding of abnormal spontaneous activities in cerebral regions may contribute to more comprehensive understanding of the potential neural network changes in patients with OF. The changes of fALFF value in patients with OF may help to gauge their emotional changes and clinical recovery levels.
Introduction
The orbital bones are fragile, with no protective surround, making them vulnerable to orbital wall fracture (1), of which trauma is the main cause. Previous research (2) has shown that about half of orbital fractures are isolated and are usually limited to one orbital wall, the orbital floor, and medial wall being the most frequently occurring fracture sites (3). Orbital fractures mostly occur in children and young people, and are more common in males than females (4, 5). Chi et al. reviewed 733 cases of orbital fractures, among which three quarters were male (6). Conservative treatment is often used for small orbital fractures without displacement (7), while, for large displaced fractures, surgical intervention is necessary (8, 9). Orbital fractures may cause exophthalmos (10), enophthalmos (11), diplopia (12), entropion (13), subconjunctival hemorrhage (14), and even blindness (15). Therefore, early monitoring and termination of adverse disease progression in patients with OF are very important. The use of modern imaging technology to study the brain activity of patients with OF may be important as a means by which to improve understanding of the mechanism of potential pathological changes in this condition, and may, therefore, be beneficial to the management of complications. Previous studies have confirmed that the changes of spontaneous brain activity in related brain regions can be used as an indicator of disease progression. Therefore, we tried to explore the value of using modern imaging techniques to explore the value of spontaneous brain activity changes as a marker of disease progression in patients with orbital fractures.
Magnetic resonance imaging (MRI), as a widely used auxiliary imaging technology, was developed in the 1980s and provides us a preliminary understanding of the anatomical structure and operating mechanism of the brain (16, 17). Hemodynamic changes caused by neuronal activity can be qualitatively measured with the help of MRI technology, known as functional magnetic resonance imaging (fMRI). This approach has been used in a variety of studies on the mechanism and effects of spontaneous neuronal activity in the brain, and has been helpful in exploring the pathophysiological changes and pathogenesis of various diseases (18, 19). The fractional amplitude of low frequency fluctuation (fALFF), a resting state fMRI method, has provided an index for the evaluation of spontaneous neural activity, and its accuracy and sensitivity have been widely confirmed (16). To our knowledge, the present experiment was the first attempt to explore the connection between spontaneous brain activity and behavioral performance in patients with OF using the fALFF method as well as to explore the value of fALFF in evaluating the pathological changes and severity of OF.
Subjects and Methods
Subjects
In total, 20 patients with OF (12 males, 8 females) and 20 matched healthy controls (HCs) participated in this research. The relevant inclusion criteria were: (1) with optic nerve injury; (2) with diplopia; (3) with orbital collapse; (4) with limited eye movement; (5) with surgical treatment; (6) no other ocular diseases (such as macular degeneration); (7) no brain disease (such as cerebral infarction); (8) no history of mental illness; (9) no organic diseases likely to affect MRI examination.
The 20 HCs (12 males, 8 females) were highly similar to the OF group in sex, age, weight, and education level. Our study met the ethical standards of the Medical Ethics Committee of the First Affiliated Hospital of Nanchang University as well as the principles of the Declaration of Helsinki. After materials, methods, purpose, and underlying risks of this experiment were explained, each participant signed a declaration of informed consents.
MRI Parameters
MRI scanning was conducted using a Trio 3-Tesla MR scanner (Trio; Siemens, Munich, Berlin, Germany) in all the participants. During the MRI scanning, other interference factors were excluded, and the subjects remained awake, breathing normally and with good vital signs. The whole-brainT1-weights were obtained with the application of the spoiled gradient-recalled echo sequence. Relevant corresponding parameter settings of structural images were as follows: echo time = 2.25 ms, repetition time = 1,800 ms, field of view = 250 × 250 mm2, layer interval = 0.5 mm, flip angle = 90°, matrix = 256 × 256, thickness = 1 mm. Functional images (n = 240) were captured with the following settings: echo time = 30 ms, repetition time = 2,000 ms, field of view = 220 × 220 mm2, flip angle = 90°, matrix = 64 × 64, thickness = 4 mm.
fMRI Data Processing
All data were pre-filtered using MRIcro (www.MRIcro.com) and then preprocessed the filtered data using SPM8 (https://www.fil.ion.ucl.ac.uk/spm/). In pre-filtering, the first 10 volumes were regarded as invalid data and excluded to ensure steady signals. The volumes were offset by no more than 2 mm in X, Y, or Z directions. On the basis of the standard echo planar imaging template, the images were resampled and normalized (with a standard setting of voxel size 3 × 3 × 3 mm, and were smoothed) to enhance the signal-to-noise ratio. This method has been described in detail previously (20).
fALFF Analysis
To calculate fALFF, a full-width Gaussian kernel (half maximum = 6 × 6 × 6 mm3) was used to smooth the remaining 230 images. Band-pass (0.01–0.08 Hz) filtering was used to control for movement artifacts and low frequency drift. A fast Fourier transform (FFT) algorithm was used to obtain the signal power spectrum, and fAFLL was calculated as the ratio of the amplitude at each value in the low frequency band (0.01–0.08 Hz) to full-band (0–0.25 Hz) power amplitude.
Brain-Behavior Correlation Analysis
To look for any associations between brain activity and behavioral performance, brain regions of interest were determined based on fALFF values, and Pearson's correlation analysis was used to explore the linear relationship between activities in these regions and clinical manifestations.
Statistical Analysis
Using SPSS software version 20.0 (IBM Corp, Armonk, NY, USA), an independent sample t-test was conducted on the common clinical variables and demographic data of patients with OF and HCs using a 5% significance level. A two-sample t-test was used to compare the functional data. Based on Gaussian random field theory, the statistical threshold of the voxel level in multiple comparisons was set at p < 0.05. Gaussian random field theory was used to determine the significance of the functional image at the 5% level with a cluster size > 40 voxels. Using the mean fALFF in various cerebral regions of HCs and patients with OF, the areas under the ROC curves (AUC) were obtained. In addition, Pearson correlation analysis was used to look for associations between the mean fALFF values in multiple cerebrum regions and characteristics of clinical behavior in patients with OF.
Results
Demographics and Visual Measurements
No significant differences were found between groups in terms of gender (p > 0.99), weight (p = 0.902), or age (OF, 51.21 ± 11.42; HC, 50.96 ± 10.82; p = 0.871). However, best corrected monocular visual parameters were significantly different between groups, as follows: visual acuities (p = 0.017, left, and 0.011, right eye), visual-evoked potential (VEP) latencies (p = 0.022, left, and 0.017, right eye), and amplitudes (p = 0.012, left, and 0.009, right eye) (Table 1).
Differences in fALFF
In comparison with HCs, the patients with OF showed significant lower fALFF values in the left anterior cingulate gyrus (LACG) and right superior temporal gyrus (RSTG) (Figure 1, Table 2). The mean fAFLL values are shown in Figure 2.
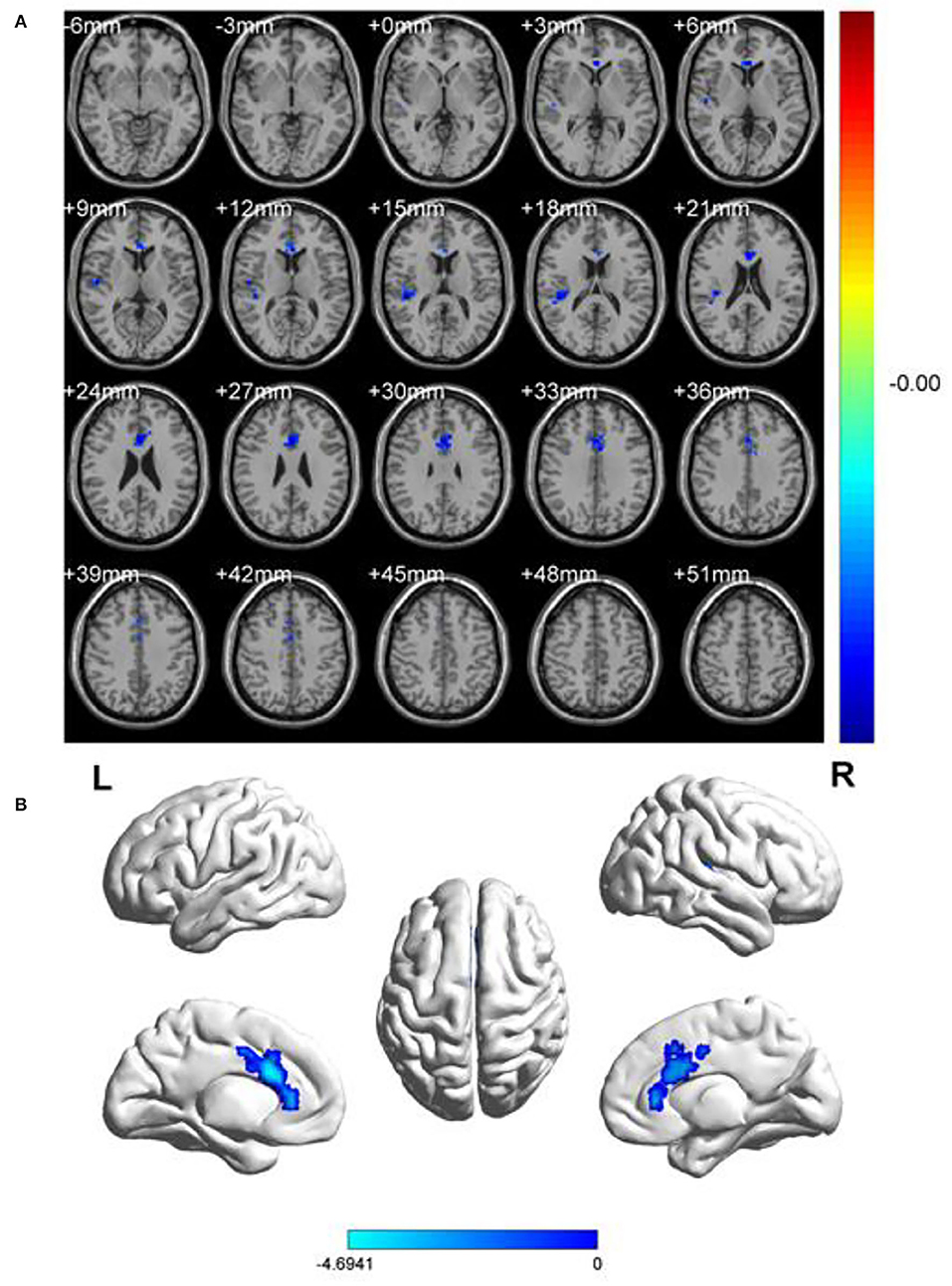
Figure 1. Spontaneous brain activities of and healthy controls. (A) Different fALFF areas in patients with OF. (B) The blue areas represented lower fALFF values. L, left; R, right.
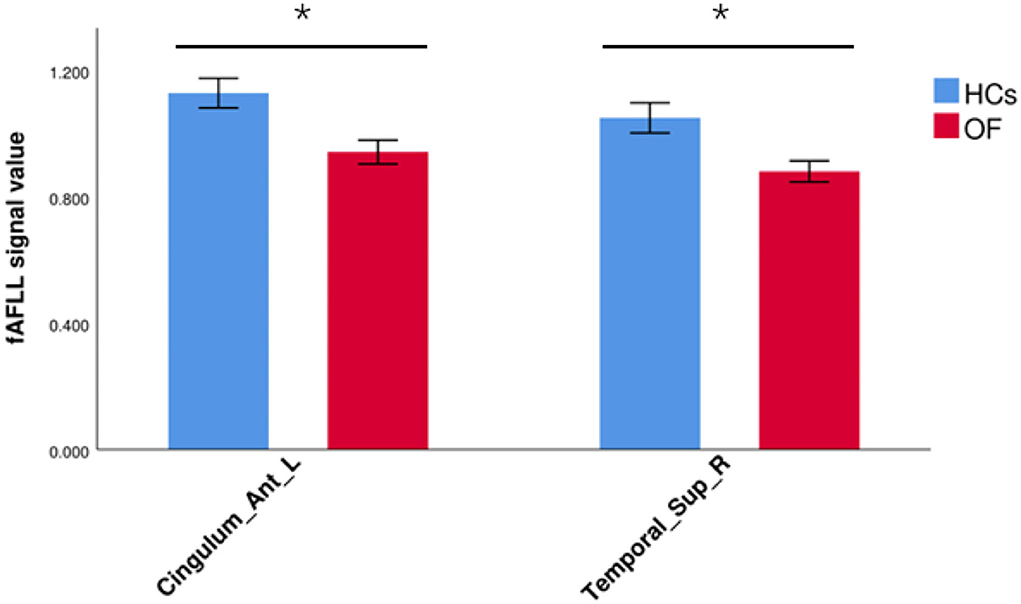
Figure 2. The average fALFF values in OF and HC groups. Cingulum_Ant_L, left anterior cingulate gyrus; Temporal_Sup_R, right superior temporal gyrus; fALFF, fractional amplitude of low-frequency fluctuation; OF, orbital fractures; HC, healthy control. “*” p < 0.05.
Receiver Operating Characteristic Curves
ROC curves were used to visualize the comparison between average fALFF values of patients with OF and HCs, and the areas under the curves (AUCs) were used as indicators of diagnostic accuracy. Using this approach, AUCs of the LATG and RSTG were found to be 0.983 and 1.000, respectively (Figure 3).
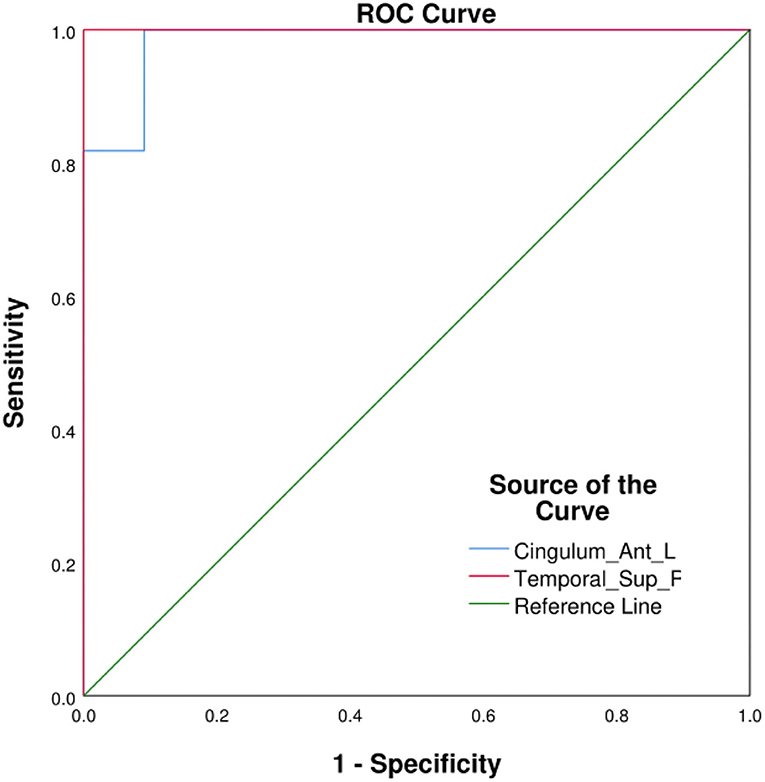
Figure 3. ROC curve analysis of the average fALFF values in different cerebrum areas. AUCs: Cingulum_Ant_L: 0.983, Temporal_Sup_R: 1.000. Cingulum_Ant_L, left anterior cingulate gyrus; Temporal_Sup_R, right superior temporal gyrus; AUC, area under the curve; ROC, receiver operating characteristic.
Correlation Analysis
In patients with OF, significant correlations were found between fALFF values in the RSTG and depression scores (negative correlation: r = −0.955, p < 0.01) and anxiety scores (negative correlation: r = −0.899, p < 0.01) (Figure 4).
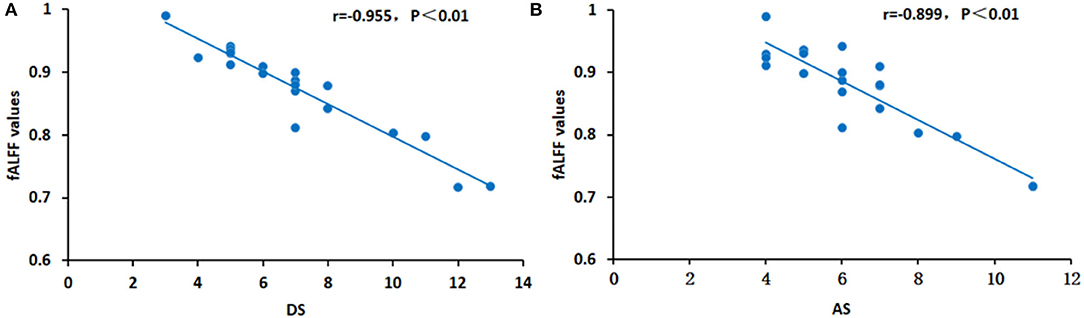
Figure 4. Correlations between the average fALFF values and clinical characteristics in the RSTG. In the RSTG, the DS (r = −0.955, p < 0.01) (A) and AS (r = −0.899, p < 0.01) (B) are both represented by a negative relationship with the fALFF values. fALFF, fractional amplitude of low-frequency fluctuation; DS, depression score; AS, anxiety score; RSTG, right superior temporal gyrus.
Discussion
To our knowledge, the ALFF method has not previously been used to study the potential relationship between brain activity changes and clinical manifestations in patients with OF. This study aimed to explore the cerebral neural changes after orbital fracture using the fALFF technique (Figure 5). The study found significantly lower fALFF values in the LACG and the RSTG in patients with OF (Figure 6). In previous studies, the fALFF method has been applied to a series of ophthalmological diseases, including normal-tension glaucoma (20), monocular blindness (21), retinal vein occlusion (22), diabetic retinopathy, and nephropathy (23) (Table 3), demonstrating its potential for clinical application.
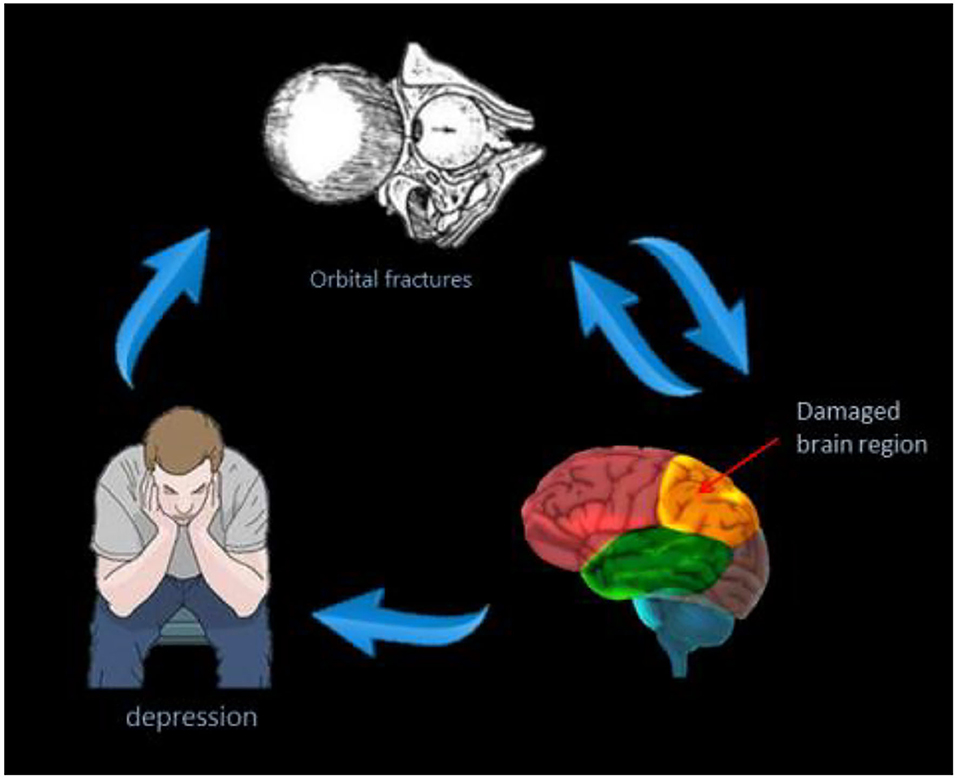
Figure 5. The correlations between average fALFF signal values and clinical manifestation of patients with OF. The patients with OF have lower fALFF values, and they are more likely to develop depressive symptoms. OF, orbital fractures.
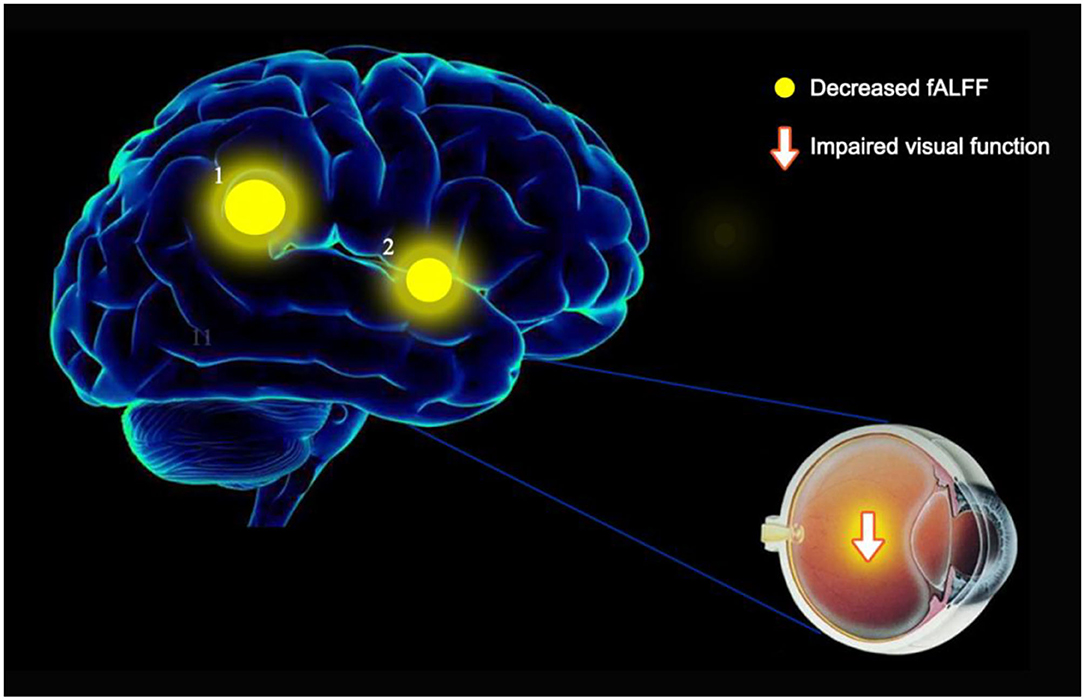
Figure 6. fALFF results of cerebral activity in the OF group. The fALFF values of the cerebral areas in the OF group were in the following: 1- left anterior cingulate gyrus (t = −4.6941), 2 - right superior temporal gyrus (t = −4.399). The intensity, as well as the frequency of brain activity, is reflected by the size of spots.
The anterior cingulate gyrus (ACG) is a functional area associated with many physiological functions, is located in the medial brain and passes longitudinally through the parietal lobe, and its main roles are in memory (24), action – outcome learning (25–27), emotion, and reward-related processing (28). The research of Hornak et al. (29) showed that, in some cases, the ACG plays an important part in voice and facial expression recognition, while Lane et al. (30–32) studied anterior cingulate injury in subjective emotional experience, and found that ventral ACG and Brodmann's area 9 may be activated during mood fluctuations. Based on the functions of the anterior cingulate gyrus, some researchers have explored its diagnostic value in Parkinson's disease (33), depression (34), and acute and chronic pain (35). In addition, a previous study has found that the prefrontal cingulate gyrus can respond to visual stimuli (36). In the present study, given the reduced visual responses in patients with OF, the results may indicate a compensatory mechanism for vision loss in patients with OF.
The superior temporal gyrus (STG) is a functional area of the brain located in the temporal lobe, closely related to emotional and speech processing (37, 38). The STG is a component of the default mode network, which is inhibited during brain activity and excited during rest. Liu et al. (39) used the rsMRI-fALFF method to study the STG in depression. They found that the lower fALFF values of STG correlated greater reductions on the Hamilton rating scale for depression, and inferred that STG neural changes were closely related to the effect of early treatment for depression. In addition, Wang et al. (40) measured functional connectivity density in STG and found that abnormal connectivity is negatively correlated with the treatment effect. In the present experiment, the fALFF value of the right STG in patients with OF was significantly lower than that in healthy controls, and we speculate that this decrease may be a compensatory mechanism for the recovery of brain function in patients with OF. The results suggest that the fALFF value may be used as a reliable index to gauge therapeutic effects of clinical treatment. Moreover, it was discovered that, in the patients with OF, fALFF values in the RSTG were negatively correlated with anxiety and depression scores, which may indicate a self-regulation mechanism in this brain area, with brain function being temporarily inhibited (Table 4).
This study has some limitations; one of which is the relatively small sample size, and the other is that the sample source was limited and not completely matched. Third, compared with the simple use of VEP, the use of pattern electro retino grams (PERGs) and pattern visual-evoked potentials (PVEPs) two checks can be more rigorous explanation of the problem. Therefore, future research should use larger and more closely matched samples to further clarify the neural changes in patients with orbital fractures, and to provide a more intuitive clinical efficacy index for treatment. In conclusion, this study has demonstrated that patients with OF have reduced fALFF values in specific cerebrum areas, indicating changes in spontaneous brain activity. Further research on the mechanism underpinning brain activity changes in patients with OF may be helpful to advance understanding of this condition.
Data Availability Statement
The original contributions presented in the study are included in the article/supplementary material, further inquiries can be directed to the corresponding author/s.
Ethics Statement
The studies involving human participants were reviewed and approved by Ethics Committee of Nanchang University. The patients/participants provided their written informed consent to participate in this study.
Author Contributions
YXG, SHX, PY, YCP, HYS, LJZ, RBL, and QYL: Data collation, data analysis, and paper writing and revision. SY: The funding and design of the project and the guidance of the article. All authors contributed to the article and approved the submitted version.
Conflict of Interest
The authors declare that the research was conducted in the absence of any commercial or financial relationships that could be construed as a potential conflict of interest.
Publisher's Note
All claims expressed in this article are solely those of the authors and do not necessarily represent those of their affiliated organizations, or those of the publisher, the editors and the reviewers. Any product that may be evaluated in this article, or claim that may be made by its manufacturer, is not guaranteed or endorsed by the publisher.
References
1. Ramponi DR, Astorino T, Bessetti-Barrett CR. Orbital Floor Fractures. Adv Emerg Nurs J. (2017) 39:240–7. doi: 10.1097/TME.0000000000000163
2. Dubois L, Steenen SA, Gooris PJ, Mourits MP, Becking AG. Controversies in orbital reconstruction–I. Defect-driven orbital reconstruction: a systematic review. Int J Oral Maxillofac Surg. (2015) 44:308–15. doi: 10.1016/j.ijom.2014.12.002
3. Joseph JM, Glavas IP. Orbital fractures: a review. Clin Ophthalmol. (2011) 5:95–100. doi: 10.2147/OPTH.S14972
4. Koenen L, Waseem M. Orbital Floor Fracture. StatPearls: StatPearls Internet Treasure Island (FL). (2020).
5. Phan LT, Jordan PW, McCulley TJ. Orbital trapdoor fractures. Saudi J Ophthalmol. (2012) 26:277–82. doi: 10.1016/j.sjopt.2012.05.008
6. Cope MR, Moos KF, Speculand B. Does diplopia persist after blow-out fractures of the orbital floor in children? Br J Oral Maxillofac Surg. (1999) 37:46–51. doi: 10.1054/bjom.1998.0382
7. Bolling JP, Wesley RE. Conservative treatment of orbital roof blow-in fracture. Ann Ophthalmol. (1987) 19:75–6.
8. Sullivan WG. Displaced orbital roof fractures: presentation and treatment. Plast Reconstr Surg. (1991) 87:657–61. doi: 10.1097/00006534-199104000-00008
9. Raveh J. Surgical techniques in orbital roof fractures: early treatment and results. J Craniomaxillofac Surg. (1995) 23:332–3. doi: 10.1016/S1010-5182(05)80166-0
10. Ha AY, Mangham W, Frommer SA, Choi D, Klinge P, Taylor HO, et al. Interdisciplinary management of minimally displaced orbital roof fractures: delayed pulsatile exophthalmos and orbital encephalocele. Craniomaxillofac Trauma Reconstr. (2017) 10:11–5. doi: 10.1055/s-0036-1584395
11. Koryczan P, Zapała J, Gontarz M, Wyszyńska-Pawelec G. Surgical treatment of enophthalmos in children and adolescents with pure orbital blowout fracture. J Oral Sci. (2020). doi: 10.2334/josnusd.20-0371
12. Roselló EG, Granado AM, Garcia MA, Martí SJ, Sala GL, Mármol BB, et al. Facial fractures: classification and highlights for a useful report. Insights Imaging. (2020) 11:49. doi: 10.1186/s13244-020-00847-w
13. Harish KM, Tulasidas G, Arthanari B, Bhagat JA. Aesthetic outcome of a case of orbital floor fracture treated using a retroseptal transconjunctival approach. Cureus. (2019) 11:e4063. doi: 10.7759/cureus.4063
14. Terrill SB, You H, Eiseman H, Rauser ME. Review of ocular injuries in patients with orbital wall fractures: a 5-year retrospective analysis. Clin Ophthalmol. (2020) 14:2837–42. doi: 10.2147/OPTH.S274567
15. Scolozzi P. Orbital fractures: enigmatic and insidious entities. Rev Med Suisse. (2020) 16:1281–6. doi: 10.53738/REVMED.2020.16.699.1281
16. Shi WQ, Tang LY, Lin Q, Li B, Jiang N, Zhu PW, et al. Altered spontaneous brain activity patterns in diabetic patients with vitreous hemorrhage using amplitude of lowfrequency fluctuation: a restingstate fMRI study. Mol Med Rep. (2020) 22:2291–9. doi: 10.3892/mmr.2020.11294
17. Yu YJ, Liang RB, Yang QC, Ge QM Li QY, Li B, et al. Altered spontaneous brain activity patterns in patients after lasik surgery using amplitude of low-frequency fluctuation: a resting-state functional MRI study. Neuropsychiatr Dis Treat. (2020) 16:1907–17. doi: 10.2147/NDT.S252850
18. Dai XJ, Liu CL, Zhou RL, Gong HH, Wu B, Gao L, et al. Long-term total sleep deprivation decreases the default spontaneous activity and connectivity pattern in healthy male subjects: a resting-state fMRI study. Neuropsychiatr Dis Treat. (2015) 11:761–72. doi: 10.2147/NDT.S78335
19. Bekiesinska-Figatowska M, Helwich E, Rutkowska M, Stankiewicz J, Terczyńska I. Magnetic resonance imaging of neonates in the magnetic resonance compatible incubator. Arch Med Sci. (2016) 12:1064–70. doi: 10.5114/aoms.2016.61913
20. Li HL, Chou XM, Liang Y, Pan T, Zhou Q, Pei CG, et al. Use of rsfMRI-fALFF for the detection of changes in brain activity in patients with normal-tension glaucoma. Acta Radiol. (2020) 62:414–22. doi: 10.1177/0284185120926901
21. Fang JW Yu YJ, Tang LY, Chen SY, Zhang MY, Sun T, et al. Abnormal fractional amplitude of low-frequency fluctuation changes in patients with monocular blindness: a functional magnetic resonance imaging (MRI) study. Med Sci Monit. (2020) 26:e926224. doi: 10.12659/MSM.926224
22. Tong Y, Huang X, Gao Q, Qi CX, Shen Y. Fractional amplitude of low-frequency fluctuations in retinal vein occlusion: a resting-state fMRI study. Zhonghua Yan Ke Za Zhi. (2020) 56:266–71. doi: 10.3760/cma.j.cn112142-20200904-00452
23. Tang ZH, Liu TT, Sun XH, Wu LJ, Xiao ZB. Altered spontaneous neuronal activity and functional connectivity pattern in primary angle-closure glaucoma: a resting-state fMRI study. Neurol Sci. (2021) 42:243–51. doi: 10.1007/s10072-020-04577-1
24. Rolls ET. The storage and recall of memories in the hippocampo-cortical system. Cell Tissue Res. (2018) 373:577–604. doi: 10.1007/s00441-017-2744-3
25. Rushworth MF, Kolling N, Sallet J, Mars RB. Valuation and decision-making in frontal cortex: one or many serial or parallel systems? Curr Opin Neurobiol. (2012) 22:946–55. doi: 10.1016/j.conb.2012.04.011
26. Kolling N, Wittmann MK, Behrens TE, Boorman ED, Mars RB, Rushworth MF. Value, search, persistence and model updating in anterior cingulate cortex. Nat Neurosci. (2016) 19:1280–5. doi: 10.1038/nn.4382
27. Rolls ET. The orbitofrontal cortex and emotion in health and disease, including depression. Neuropsychologia. (2019) 128:14–43. doi: 10.1016/j.neuropsychologia.2017.09.021
28. Rolls ET. The cingulate cortex and limbic systems for emotion, action, and memory. Brain Struct Funct. (2019) 224:3001–18. doi: 10.1007/s00429-019-01945-2
29. Hornak J, Bramham J, Rolls ET, Morris RG, O'Doherty J, Bullock PR, et al. Changes in emotion after circumscribed surgical lesions of the orbitofrontal and cingulate cortices. Brain. (2003) 126:1691–712. doi: 10.1093/brain/awg168
30. Lane RD, Reiman EM, Ahern GL, Schwartz GE, Davidson RJ. Neuroanatomical correlates of happiness, sadness, and disgust. Am J Psychiatry. (1997) 154:926–33. doi: 10.1176/ajp.154.7.926
31. Lane RD, Reiman EM, Bradley MM, Lang PJ, Ahern GL, Davidson RJ, et al. Neuroanatomical correlates of pleasant and unpleasant emotion. Neuropsychologia. (1997) 35:1437–44. doi: 10.1016/S0028-3932(97)00070-5
32. Lane RD, Frewen P, Neufeld RW, Densmore M, Stevens T, Lanius R. Neural correlates of levels of emotional awareness. Evidence of an interaction between emotion and attention in the anterior cingulate cortex. J Cogn Neurosci. (1998) 10:525–35. doi: 10.1162/089892998562924
33. Vogt BA. Cingulate cortex in Parkinson's disease. Handb Clin Neurol. (2019) 166:253–66. doi: 10.1016/B978-0-444-64196-0.00013-3
34. Godlewska BR, Browning M, Norbury R, Igoumenou A, Cowen PJ, Harmer CJ. Predicting treatment response in depression: the role of anterior cingulate cortex. Int J Neuropsychopharmacol. (2018) 21:988–96. doi: 10.1093/ijnp/pyy069
35. Bliss TV, Collingridge GL, Kaang BK, Zhuo M. Synaptic plasticity in the anterior cingulate cortex in acute and chronic pain. Nat Rev Neurosci. (2016) 17:485–96. doi: 10.1038/nrn.2016.68
36. Sidorov MS, Kim H, Rougie M, Williams B, Siegel JJ, Gavornik JP, et al. Visual sequences drive experience-dependent plasticity in mouse anterior cingulate cortex. Cell Rep. (2020) 32:108152. doi: 10.1016/j.celrep.2020.108152
37. Yi HG, Leonard MK, Chang EF. The encoding of speech sounds in the superior temporal gyrus. Neuron. (2019) 102:1096–110. doi: 10.1016/j.neuron.2019.04.023
38. Takahashi T, Yücel M, Lorenzetti V, Walterfang M, Kawasaki Y, Whittle S, et al. An MRI study of the superior temporal subregions in patients with current and past major depression. Prog Neuropsychopharmacol Biol Psychiatry. (2010) 34:98–103. doi: 10.1016/j.pnpbp.2009.10.005
39. Liu X, Hou ZH, Yin YY, Xie CM, Zhang HS, Zhang HX, et al. Dopamine multilocus genetic profile, spontaneous activity of left superior temporal gyrus, and early therapeutic effect in major depressive disorder. Front Psychiatry. (2020) 11:591407. doi: 10.3389/fpsyt.2020.591407
Keywords: orbital fractures, fALFF, spontaneous brain activity, resting state, image
Citation: Kang M, Gao Y, Zhang L, Liang R, Li Q, Shu H, Pan Y, Ying P, Xu S and Yi S (2022) Detection of Abnormal Spontaneous Brain Activity Patterns in Patients With Orbital Fractures Using Fractional Amplitude of Low Frequency Fluctuation. Front. Neurol. 13:874158. doi: 10.3389/fneur.2022.874158
Received: 11 February 2022; Accepted: 08 June 2022;
Published: 13 July 2022.
Edited by:
Yuzhen Xu, Tongji University, ChinaCopyright © 2022 Kang, Gao, Zhang, Liang, Li, Shu, Pan, Ying, Xu and Yi. This is an open-access article distributed under the terms of the Creative Commons Attribution License (CC BY). The use, distribution or reproduction in other forums is permitted, provided the original author(s) and the copyright owner(s) are credited and that the original publication in this journal is cited, in accordance with accepted academic practice. No use, distribution or reproduction is permitted which does not comply with these terms.
*Correspondence: Shao Yi, ZnJlZWJlZTk5QDE2My5jb20=
†These authors have contributed equally to this work