- 1Institute of Acupuncture and Moxibustion, China Academy of Chinese Medical Science, Beijing, China
- 2Institute of Basic Theory for Chinese Medicine, China Academy of Chinese Medical Science, Beijing, China
- 3Guang'anmen Hospital, China Academy of Chinese Medical Sciences, Beijing, China
Depression is a worldwide disease causing severe disability, morbidity, and mortality. Despite abundant studies, the precise mechanisms underlying the pathophysiology of depression remain elusive. Recently, cumulate research suggests that a disturbance of microbiota-gut-brain axis may play a vital role in the etiology of depression while correcting this disturbance could alleviate depression symptoms. The vagus nerve, linking brain and gut through its afferent and efferent branches, is a critical route in the bidirectional communication of this axis. Directly or indirectly, the vagus afferent fibers can sense and relay gut microbiota signals to the brain and induce brain disorders including depression. Also, brain changes in response to stress may result in gut hyperpermeability and inflammation mediating by the vagal efferents, which may be detrimental to depression. Notably, vagus nerve stimulation owns an anti-inflammatory effect and was proved for depression treatment. Nevertheless, depression was accompanied by a low vagal tone, which may derive from response to stress and contribute to pathogenesis of depression. In this review, we aim to explore the role of the vagus nerve in depression from the perspective of the microbiota-gut-brain axis, highlighting the relationship among the vagal tone, the gut hyperpermeability, inflammation, and depression.
Introduction
Depression is a leading mental disorder causing severe disability, morbidity, and mortality worldwide. Approximately 280 million people in the world have depression (1), with almost one in five people suffering from one episode at some point in their lifetime (2). Of the leading causes of Years Lived with Disability, depression was ranked second, the highest within mental disorders, and 13th among the top 25 leading causes of Disability Adjusted Life Years in 2019, which had increased from 1990 to 2019 (1, 3). The prevalence of depression was one of the major underlying reasons of self-harm and most depressive patients have suicidal thoughts (4). According to data from WHO, there are 785,000 suicides annually around the world, with an incidence of 10.6 per 100,000 population in 2016 (5), and up to 60% of them have major depression (6).
Conventionally, depression is viewed as a mental disease with physiological dysbiosis such as unbalanced neurotransmitters and neuronal circuitry, impaired neurogenesis, declined neuroplasticity, and neuroinflammation (7–9). Recently, growing studies identified the detrimental role of disturbed microbiota-gut-brain axis in the etiology of depression and that strategies targeting to restore the balance of this axis, namely probiotics, exert a curative effect on depression (10–12). The gut microbiota (GM), the gut, and the brain interact with one another reciprocally through various routes including the vagus nerve (VN) (13, 14). The VN, a mixed nerve composed of 80% afferent and 20% efferent fibers, is able to sense and relay GM signals to the brain via its afferents and also, deliver brain signals to peripheral organs including the gut via the efferents, modulating gut dysbiosis (14). A disturbed GM led to depression-like behaviors, which may be mediated by the VN (15). Inflammation is a crucial contributor to depression (16). Vagus nerve stimulation (VNS), approved for the treatment of treatment-resistant depression and refractory epilepsy, can induce anti-inflammatory cytokines and dampen inflammation (17, 18). Besides, stimulating the VN also protects against gut hyperpermeability, which may facilitate GM translocation and result in gut and system inflammation (19). Meanwhile, a depressed vagal tone was found in depressive patients (20). It is hypothesized that through the microbiota-gut-brain axis (MGBA), a low vagal tone-induced gut barrier deficit and system inflammation may have a close relation to depression. In this review, we aim to explore the role of the VN in depression from the perspective of MGBA, putting an emphasis on the relationship among vagal tone, gut hyperpermeability, inflammation, and depression.
Anti-depressive effect of VNS
Massive clinic research demonstrated the efficacy and safety of VNS in treating depression (C et al., 2008) (21, 22). In one multi-center study, 30 treatment-resistant depression patients with the median length of the current major depressive episode being 4.7 years, were recruited and results showed that using a ≥50% reduction in the baseline 28-item Hamilton Depression Rating Scale total score to define response, a 40% response rate along with sustained symptomatic responses were achieved after 10 weeks of VNS (23). Though another randomized, controlled acute phase trial showed tolerated results that after 10 weeks adjunctive VNS or sham treatment, 24-item Hamilton Rating Scale for Depression response rates were 15.2% for VNS group (n = 112) and 10.0% for sham group (n = 110), for which the relative shorter treatment duration may account as response rates showed a positive correlation with treatment duration (24–26). And similar accumulated antidepressant effects of VNS as mentioned above were found in a later European open-label study, in which response rates increased from 37% at 3 months to 53% at 12 months and remission rates almost doubled from 17% at 3 months to 33% at 12 months (27). Notably, in a recent 5-year, prospective, open-label, non-randomized, observational registry study, patients with treatment-resistant depression receiving adjunctive VNS with treatment as usual showed significantly better clinical outcomes compared to patients receiving treatment as usual, including a significantly higher 5-year cumulative response rate (67.6% compared with 40.9%) and a significantly higher remission rate (cumulative first-time remitters, 43.3% compared with 25.7%) (28). Intriguingly, to investigate dose-effect of VNS, another prospective, randomized, controlled trial conducted in 2013, in which participants were split into three groups: low (0.25 mA current, 130 μs pulse width), medium (0.5–1.0 mA, 250 μs), or high (1.25–1.5 mA, 250 μs), showed that though improvements of the medium and high-dose groups were better sustained at the end of the longer-term phase, the low group also had significant improvement in depressive symptoms at the end of the acute phase, suggesting that issues may exist when using low-dose VNS as a sham treatment because even low-dose VNS provided a substantial antidepressant effect (29). Besides, VNS treatment also showed promising therapeutic effects on conditions with comorbid depression including epilepsy (30), obesity (31), and migraine (32) and was capable of improving cognitive performance of treatment-resistant depression patients (33). Despite these benefits on depression and comorbid disorders, the high cost and invasiveness of VNS hampered its application and developments, both from a research and clinical perspective, and the field of a new, non-invasive VNS technique, transcutaneous auricular vagus nerve stimulation (taVNS), has drawn growing attention (34). Though in its infancy, several clinical studies had been conducted concerning taVNS in treating depression and all showed that taVNS alleviated depression symptoms with modest side effects reported (35–39). However, though evidence from various studies indicates that VNS may partly reverse the deficiency of monoaminergic neurotransmitters in the brain, peripheral and central inflammation, dysfunction of the HPA axis, abnormal neuroplasticity and neurogenesis, and disorder of functional connectivity which are implicated in depression, the antidepressant mechanism of VNS remains elusive and further efforts are warranted (2, 40–43).
Linking of the microbiota-gut-brain axis: The vagus nerve
Anatomy of the vagus nerve in the microbiota-gut-brain axis
Composed of 80% afferent and 20% efferent fibers, the VN transmits information and provides feedback by innervating different visceral organs including gastrointestinal tract, respiratory, and cardiovascular systems (44, 45). The gut is innervated by the hepatic and celiac branches of the VN with cell bodies of afferent neurons residing at nodose ganglia and terminating at the nucleus tractus solitarius (NTS), while the dorsal motor nucleus of the vagus nerve (DMNV), the origin of the efferent, is in close contact with the NTS (46, 47). In the gut, vagal afferents (VA) form three connections which end in muscle wall, mucosa, and of note, the recently defied neuropod cells which are a subset of enteroendocrine cells synapsing with vagal neurons (48). Attributing to their locations and a variety of receptors including mechanoreceptors and chemoreceptors expressed on them, VA are capable of detecting and responding to various sorts of signals including stretch, tension, or intestinal molecules such as bacterial by-products, gut hormones, or neurotransmitters (19, 49).
In the brain, the NTS together with the DMNV, form an autonomic brainstem loop regulating gastrointestinal motility, acid secretion, food intake, and satiety (50). Through projections from the NTS to several regions of the central nervous system (CNS), including the parabrachial nucleus, paraventricular nucleus of the hypothalamus, locus coeruleus, the amygdala, and the thalamus, the NTS form an autonomic brainstem loop which is modulated by the autonomic forebrain loop which comprises nuclei in the pons, the hypothalamus, the hippocampus, the amygdala, the anterior cingulate, the insular, and the prefrontal cortices, synthetically coordinating visceral information that includes neuroendocrine responses, emotions, cognition, and behaviors (50). Thus, the brain is in close communication with the gut through these two loops and the VN and stress, feelings, and thoughts may play a beneficial or detrimental role in gut homeostasis and vice versa.
From gut microbiota to brain: Role of vagus
Though the VN is unable to reach luminal contents, it can indirectly sense gut information through communication with enteroendocrine cells (EECs) (51). By releasing mediators including serotonin, cholecystokinin, glucagon-like peptide-1, and peptide YY, acting on corresponding receptors expressed on vagal afferent neurons, the EECs can modulate food intake and autonomic reflexes controlling gut motility, secretion, inflammatory responses, and mucosal defense (52). And the latest identified neuropods have demonstrated that apart from paracrine and endocrine, the EECs have the capability of forming synapses with neurons of the vagal nodose to transduce a sense from gut to brain with glutamate, to our knowledge, as the neurotransmitter (53). Most pivotally, as the first cell line facing the gastrointestinal contents, the EECs can detect signals from GM which may in turn influence the releasing of chemical stimuli including hormones, neurotransmitters, and metabolites produced by EECs (54, 55). Indeed, both in vitro and in vivo studies demonstrated that the EECs expressing toll-like receptors (TLRs) can sense microbe-associated molecular patterns (MAMPs) including lipopolysaccharide (LPS) and flagellin, which are constitutively released by bacteria (56, 57). In addition to secreted bacterial ligands by microbes, the EECs can also detect bacterial metabolites (58, 59). For example, short-chain fatty acids (SCFAs) can bind to G protein-coupled receptors (GPCRs) carried on EECs, including GPR40, GPR41, GPR43, GPR119, and GPR120, and in turn, stimulate the VN, regulating host metabolism and feeding behavior (60, 61). And in response to indole, the colonic enteroendocrine L-cells can secrete glucagon-like peptide-1 to stimulate colonic VA activity (62). Furthermore, certain microbes can directly infect enteroendocrine cells and upregulate glutamate transporters, indicating that these affected cells may be neuropods, directly synapsing with VA (63, 64). Taken together, these findings suggest that the EECs are a crucial interface for detecting GM signals and relaying these massages from gut luminal to the CNS via VA.
Apart from interchanging with EECs, another pathway transmitting intestinal canal information to the CNS relies on innervation of the enteric nervous system (ENS) by the VN (65). The ENS, known as the “second brain of the body,” is crucial for maintaining stable gut health, which requires both efforts from enteric neurons and the connections to the CNS, namely the VN and sympathetic nerves (66). Located in either the submucosal or myenteric plexus, enteric neurons, with mechanoreceptors and chemoreceptors, are responsive to molecular and mechanical aberrations of the gastrointestinal tract and, in turn, activate vagal afferent neurons (67). For example, the microbiota affect both developments and function of the ENS by activating pattern recognition receptors, including TLRs, especially, TLR2 and TLR4 (68). Indeed, intrinsic primary afferent neurons were activated by ingestion of Lactobacillus rhamnosus (JB-1) and in turn stimulated part of the VA (69). Other microbiota metabolites have also been shown to influence the ENS activity and regulated gut motility in rodents (70). In addition, by delivering neurotransmitters and neuropeptides, intrinsic neurons of the ENS can modulate immune cells and cytokines produced by immune cells which have a reciprocal effect on neurons (67).
Directly, the VA also express TLR4 and free fatty acid receptor 3, sufficiently detecting MAMPs and SCFAs by themselves (71, 72). TLR4 mRNA and protein was expressed in the rat nodose ganglion, suggesting that administration of LPS could activate VA at the level of nodose ganglion (73). Owing to the gut barrier, it seems unlikely for these neurons to be directly contacting these MAMPs in physiological condition. Nevertheless, once the integrity of this barrier is lesioned under circumstances such as confronting stress, burn injury, or gastrointestinal disorders, including irritable bowel syndrome (IBS) and functional constipation, chances for the translocation of GM and their metabolites crossing the gut epithelial wall soar, with upregulation of 5-hydroxytryptophan, over expression of the corticotropin releasing factor (CRF), excessive TLR activation, and decreasing level of SCFAs being the potential contributors for this deleterious process (74–80). The gut immune system is activated by antigens from intestinal lumen and consequently, immune active substances including cytokines are released by immune cells such as T cells, B cells, innate lymphoid cells, macrophages and dendritic cells (81, 82). For instance, when activated in response to pathogens and other injurious stimuli, macrophages, dendritic cells, and other cells in the mucosa can produce a cytokine named tumor necrosis factor alpha (TNF-α)(83, 84). When exposed to cytokines including TNF and interleukin-1 beta (IL-1β), VA from nodose ganglion expressing cytokine receptors sense the peripheral inflammation condition and propagate action potentials to the CNS which then activates anti-inflammation responses including the cholinergic anti-inflammatory pathway (85, 86).
Besides, certain species of intestine bacteria have the capacity to synthesize multiple neurotransmitters including γ-aminobutyric acid (GABA), noradrenaline, dopamine, and serotonin which can travel through portal circulation to affect the afferent pathway of the VN (87, 88). For example, when the culture pH condition was adjusted to the optimal pH of glutamate decarboxylase activity, JB-1 could produce GABA with a supplement of monosodium glutamate and pyridoxal phosphate and ingestion of Lactobacillus strain induced GABA receptors alteration in a region-dependent manner in the brain which were not observed in vagotomized mice (89, 90) (Figure 1).
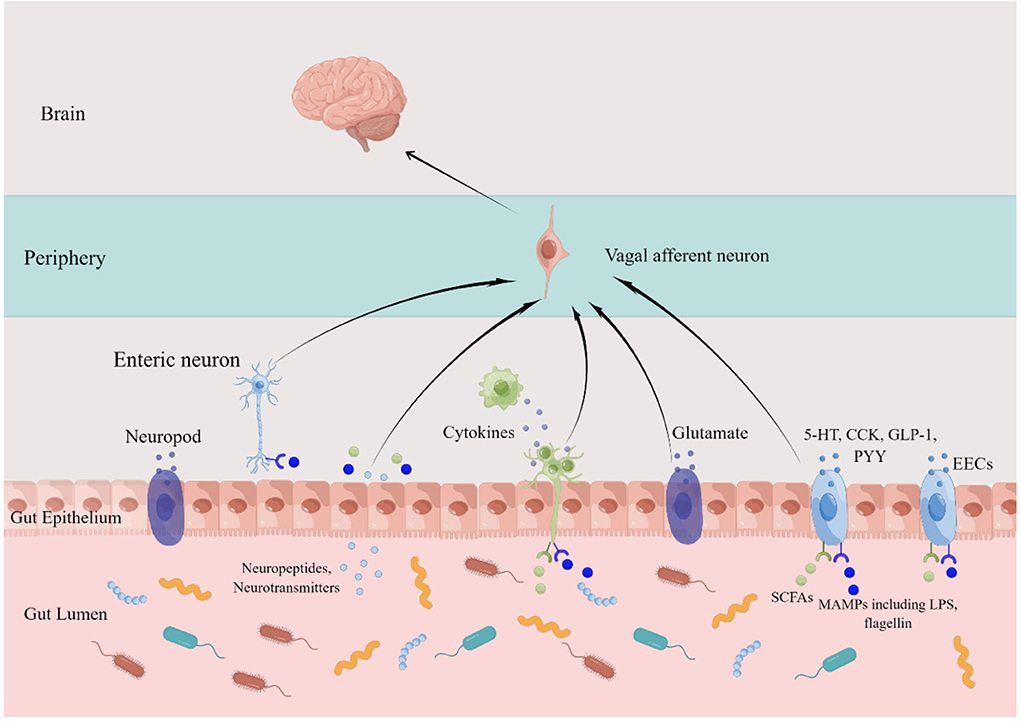
Figure 1. Vagal afferents transmit signals from gut microbiota to the central nervous system. 5-HT, serotonin; CCK, cholecystokinin; GLP-1, glucagon-like peptide-1; PYY, peptide YY; EECs, enteroendocrine cells; SCFAs, short-chain fatty acids; MAMPs, microbe-associated molecular patterns; LPS, lipopolysaccharide. By Figdraw.
Indeed, using an ex vivo intestinal jejunal segment mesenteric nerve recording preparation, Perez-Burgos et al. have shown that intra-luminally adding JB-1 could increase the firing rate of VA and these neurons may partially function as interneurons for stimulation by JB-1, receiving input from intrinsic primary afferent neurons, which synapse with VA and can be also activated by JB-1 (69, 91, 92).
Above all, on the one hand, the VA indirectly perceive GM signals through mutual communication with EECs, ENS, and the gut immune system, which interact with one another, with bioactive substances like neurotransmitters, neuropeptides, cytokines, and hormones as mediators. On the other hand, the GM and their metabolites, including SCFAs and neurotransmitters, may directly activate the VA. Together, these two pathways constitute the vagal afferent limb transmitting GM messages to the CNS, which then integrates these viscera messages and sends feedback to regulate gut homeostasis.
The microbiota-gut-brain axis and depression
Altered intestine microbiota composition in depression
In both clinical and animal models, the GM of depressed patients and animals with depressive behaviors were found to be significantly different from that of healthy controls. In depressive patients, both microbiota richness and diversity were declined in some studies (93, 94). Though there was no difference of gut microbiota composition in α-diversity in the majority of the major depressive disorder (MDD) studies between MDD patients and their healthy counterparts, a difference did exist in β-diversity (95, 96). In general, at the phylum level, the richness of Actinobacteria was higher in MDD while abundance of Bacteroidetes was lower; at the family level, the relative abundance of Bifidobacteriaceae, and Lachnospiraceae and Prevotellaceae was higher and lower, respectively; At the genus level, the abundance of Sutterella and Faecalibacterium was decreased, while that of Eggerthella, Olsenella, Collinsella, Lactobacillus, Oscillibacter increased (95). Even though distinctions of GM were observed between patients and controls in these studies, it is still debatable to determine the specific microbiota taxa which have the capacity to draw a line between the two groups (97, 98), and this uncertainty may be due to several factors such as psychiatric medication status, type of comparison group, medical comorbidities, and detection methods of fecal microbiota (99). Further research aiming to seek out the marker bacteria species that precisely discriminate the depressed patients and healthy individuals, moreover, even different types or periods of depression, are warranted to take these factors into account.
Animals with induced depressive behaviors also presented a loss of microbiota richness and diversity. The disturbance of GM composition was found in different animal models including the maternal separation model, chronic unpredictable stress model, chronic social defeat model, chronic restraint model, and the bilateral olfactory bulbectomy model (100–104). However, alterations of GM in animals with depression-like behaviors were different from that of depressed patients, with some changes even to be in the opposite direction, for example, the abundances of Bacteroidetes were lower in patients while increased in animals (101, 102).
Though we know that depressed patients are usually accompanied by disturbed GM, how this altered GM evolves and its role in depression warrant further investigations.
Fecal microbiota transplantation and depression
Depression, beyond our conventional appreciation of being a non-infectious neuropsychological disease, is transmissible through fecal microbiota transplantation (FMT) from depressed donors, either patients or animals, to recipient. Julie found that Flinders Resistant Line rats receiving FMT from MDD patients displayed significantly more depressive-like behaviors than those receiving FMT from healthy individuals (105). Moreover, intestine microbiota taxa were also transferred to recipients with the group receiving FMT from MDD patients carrying on certain taxa which was similar to their donors. The same results were found in another model in which recipient rats were depleted microbiota via an antibiotic cocktail treatment and presented depression-like symptoms including anhedonia and anxiety-like behaviors, as well as alterations in tryptophan metabolism, all of which resembled those of their counterpart providers (93). Besides, FMT from depressed animals to healthy recipients also induced depression phenotype, consistent with that of patients (106, 107). Fascinatingly, a recent study found that ingestion of FMT from rheumatoid arthritis patients resulted in the same depression-like phenotypes in antibiotic cocktail-treated mice (108). This transmissible characteristic of depression through FMT puts forward the hypothesis that a disturbed GM may play a causal rather than consequent role in its relationship with depression. However, more valid evidence is warranted to confirm this assumption.
Interestingly, in addition to these “depression-related microbiota,” some research indicated that the FMT of healthy individuals may possess a therapeutic effect. Kishimoto found that patients with either IBS, functional diarrhea, or functional constipation who received FMT of healthy relatives within the second degree of relationship (≥20 years of age) showed an improvement of depression and anxiety symptoms regardless of gastrointestinal symptom change (109). Other studies showed that both the gastrointestinal symptoms and depressive behaviors were alleviated after FMT, with this effect persisting over 4 weeks (110, 111).
Overall, it is possible for depression to transmit through fecal microbiota, though this is unlikely to happen in natural conditions. FMT may be effective adjunctive therapy in treating gastrointestinal or psychiatric disorders including IBS and depression, nevertheless, further FMT studies are worth pursuing to determine its efficacy and security.
Probiotics alleviate depression symptoms
Probiotics are generally defined as “live microorganisms that, when administered in adequate amounts, confer a health benefit on the host” (112). Beyond functioning locally in the gastrointestinal tract and treating gastrointestinal disorders including inflammatory bowel disease (IBD) (113) and IBS (114), more studies found that administration of probiotics can influence brain function and cure mental disorders (115) including Alzheimer's disease, Parkinson's disease, and depression, and the probiotics possessing beneficial effect on patients suffering from mental health issues are named psycho-biotics, first introduced by Dinan et al. (116). Indeed, clinical and animal studies have shown that probiotics supplementation could alleviate depression symptoms. In randomized, double-blind, placebo-controlled studies, probiotics significantly improved depression symptoms of depressive patients, though inconsistent results were reported concerning improvement of anxiety symptoms and serum inflammation markers (117–119, 193). Animal studies indicated that the anti-depressive effect of probiotics may attribute to mitigating gut microbial dysbiosis (120–122, 195), and this effect may not require the bacteria to be alive (112), suggesting a vital role of bacteria components in the effect. The most reported probiotics belong to lactic acid bacteria and Bifidobacterium, including strains JB-1 (123), Lactobacillus gasseri (124), Bifidobacterium longum NCC3001 (118) and Bifidobacterium breve CCFM1025 (112) and recently recognized Akkermansia muciniphila (121).
The vagus nerve plays a vital role linking gut microbiota to depression: Insight into the subdiaphragmatic vagotomy studies
Recent studies identified the essential role of the subdiaphragmatic VN in the transmission of depression through FMT. Wang et al. found that ingestion of fecal microbiota from chronic social defeated stress susceptible mice induced an anhedonia-like phenotype, higher plasma levels of interleukin-6, and decreased expression of synaptic proteins in the prefrontal cortex (PFC) in antibiotic-treated wild type mice and Ephx2 (coding soluble epoxide hydrolase) knockout (KO) mice but not in water-treated mice (125, 126). Further research found that Lactobacillus intestinalis, Lactobacillus reuteri and Faecalibaculum rodentium may, respectively, account for the anhedonia-like phenotype in antibiotic-treated wild type and Ephx2 KO mice after FMT, as merely ingestion of these microbiota duplicated these results. In another study, FMT from Chrna7 (coding α7 subtype of the nicotinic acetylcholine receptor, α7 nAChR) KO mice also resulted in depression-like phenotypes, systemic inflammation, and downregulation of synaptic proteins in the PFC in antibiotic cocktail-treated mice (127). Most importantly, subdiaphragmatic vagotomy significantly blocked the development of behavioral abnormalities, systemic inflammation, and downregulation of synaptic proteins in the PFC after ingestion of the three strain bacteria or receiving FMT from Chrna7 KO mice (125–127). These provide evidence supporting the hypothesis that the disturbance of intestine microbiota may have a causal effect on depression which is mediated by subdiaphragmatic VN and selectively deafferenting VN may be a target for effectively treating depression. Besides, subdiaphragmatic vagotomy also blocked the depression-like phenotype induced by intraperitoneal injection of LPS in rats (128). Further, a recent study found that LPS administration in mice also caused abnormal composition of gut microbiota, along with a depression-like phenotype, increasing of spleen weight, triggering of systemic inflammation and downregulating of synaptic proteins in the medial PFC in a subdiaphragmatic VN-dependent way (129). Most interestingly, they found a significant increase in the relative abundance of Lactobacillus reuteri after LPS administration which was consistent with the study of Wang et al. (125), that found positive correlations between the plasma levels of IL-6 (or TNF-α) and spleen weight and correlations between spleen weight and the abundance of the components of the microbiome. These results indicate that first, in addition to the “down to top” (from the gut to brain) effect, the subdiaphragmatic VN also mediates the “top to down” (from the peripheral or brain to gut) effect; second, the Lactobacillus reuteri may play a vital role in the pathogenesis of depression; third, as the biggest immune organ, the immune effect of the spleen in response to LPS from intraperitoneal administration or possibly leaking from the gut, may account for system inflammation and depression.
In addition, subdiaphragmatic VN dependent gut-brain signaling also contributes to the effects of oral selective serotonin reuptake inhibitors (SSRIs). After chronic or acute ingestion of sertraline or fluoxetine, two kinds of SSRIs, Karen-Anne found an increase in vagal fiber activity and the intactness of vagal signaling was required for this anti-depressive effect of chronic ingestion SSRI as subdiaphragmatic vagotomy abolished this effect, determined by the tail suspension test (130). Additionally, certain probiotics also needed this intact vagal signaling to play its anti-depressive role (124). Though, the precise mechanism remains unknown, these highlight the potential for pharmacologically stimulating VN to treat mood disorders even without the agents entering the circulation.
Vagal tone, gut permeability and inflammation, and depression
Stress as a risk factor for depression
From an evolutionary aspect, stress is a necessary response to stimulus that activates the fight-or-flight mechanism in the body which is essential for the survival of any organism (131). Proper stress enhances the acquisition and/or expression of immuno-protective responses such as wound healing, vaccination, anti-infectious agent, and anti-tumor, while inappropriate chronic stress is related to some psychiatric and gastrointestinal diseases including depression and IBD (131–133). Indeed, life stress, notably early life adversities including emotional maltreatment, physical abuse, and sexual abuse, was proved to be a risk factor for the onset of adulthood depression, which may be mediated by continued stress (134, 135). However, some indicated that those suffered childhood adversities without an early onset, seemed to gain benefit from childhood adversities, showing resilience to depression in high-stress, which suggests that it may be effective to distinguish between various types of reaction patterns based on the age at first onset of depression (136). In animals models, those that had undergone maternal separation and/or chronic stress such as chronic unpredictable stress, chronic restraint stress, and chronic mild stress, all developed depressive behaviors which were improved by administration of probiotics or prebiotics (137).
Compromised gut barrier function in depressed patients and stress induced depressed animals
Cumulative evidence demonstrates that stress can damage the gut barrier integrity in animals (137, 138). The HPA axis mediates the response to stress and activation of this axis results in the releasing of the CRF, which together with its receptors, mainly CRF1 and CRF2, plays a vital role in the gut permeability disturbance induced by stress exposure (139–141). Indeed, plasma zonulin and fatty acid-binding protein-2, the biomarkers of increased gut permeability, accompanied with plasma LPS, part of the bacteria wall of gram-negative bacteria, were found increased in subjects with a depressive disorder or an anxiety disorder, suggesting the existence of compromised gut epithelium barrier integrity and GM translocation in depressive patients (142). The gut hyperpermeability or the so-called leaky gut, may facilitate the translocation of GM and their metabolites through the intestinal epithelial barrier, further into the circulation system, promoting neural, endocrine, and immune responses (77). The serum immunoglobulin M and immunoglobulin A against LPS was also elevated in MDD, indicating an adaptive immune response to a gram-negative bacteria load and this may partly explain the increased relative abundance of gram positive cocci in rats that have undergone chronic early-life stress (143, 144). Further, this immune response was observed in Bipolar Disorder patients too, with these aberrations in the gut-brain axis most pronounced in Bipolar Type 1 and melancholia (145). Interestingly, the injection of LPS, even with minimal amounts, to human volunteers may decrease mood and induce anxiety (146).
Chronic inflammation induced by gut barrier dysfunction
System and neural inflammation play a crucial role in pathogenesis of depression while how this chronic inflammation condition forms and persists in depressive patients is unclear. The immune response to GM translocation induced by leaky gut may be responsible for the chronic inflammation condition in depression. The activation of immune cells by LPS binding to TLR4 activates nuclear factor kappa-B (NF-κB), one intracellular signaling molecule, which in turn promotes the production of pro-inflammatory cytokines, including TNF-α and IL-1 and cyclo-oxygenase-2 (147, 148). The same process also induces oxidative and nitrosative stress (O&NS) pathways, increasing expression of inducible nitric oxide and production of reactive oxygen species (ROS) by further activating nicotinamide adenine dinucleotide phosphate oxidase (149). The overload of ROS not only activates NF-κB, but leads to DNA damage and cell death, both processes aggravating the inflammatory state (150). Worse yet, cytokines including interferon-α, IL-6, IL-1β and TNF-α, and O&NS pathways may cause loosening of the tight junction barrier, forming a pro-inflammatory circle between intestine hyperpermeability and host immune response (149). This circle, at least partly, accounts for the chronic low-grade but sustained inflammation state of depressive patients. Therefore, adopting strategies targeted to protect or improve intestine epithelial integrity may exert benefits to diseases associated with the compromised gut barrier including depression while those, including stress, infection, poor diet, and antibiotics, undermine this barrier intactness resulting in deterioration of these diseases.
Gut inflammation and depression
Peripheral inflammation is closely connected to depression. Inflammation markers including serum IL-6, IL-1β, and C-reactive protein were elevated in depression patients (151, 152). Furthermore, administration of proinflammatory cytokines to humans or animals for treatment, has been found to induce depressive symptomatology, which was attenuated by treatment with antidepressants such as tricyclic antidepressants and SSRIs (153). Additionally, administration of LPS increased plasma concentration of cytokines and simultaneously induced depressive symptoms (146, 154). Most importantly, patients with gut inflammatory disorders have high co-occurrence of depressive behaviors. According to a recent Nature review, despite pronounced heterogeneity, the pooled prevalence of symptoms of depression in IBD, including Crohn's disease (CD) and ulcerative colitis, was reported over 20% (194). In addition, elevated depressive symptoms over time were associated with increased odds of active IBD (155). Besides, in studies of induced colitis in mice, behavior abnormality consistent with depression was observed, accompanied with an increase in circulating pro-inflammatory cytokines (156, 157). Interestingly, the anterior cingulate cortex (ACC), part of the autonomic forebrain loop, functioning as a relay hub and transmitting various input signals after evaluating requirements from other regions to guide adaptive behaviors, is particularly sensitive to cytokines (158, 159). Notably, both depression and CD are inflammation-related disorders and dysfunction of the ACC was reported in depression and CD (160, 161). Further, a recent study from Xu group found that patients with CD who were in the active phase exhibited higher amplitude of low frequency fluctuation (ALFF) in the left ACC and a positive correlation between mWavelet-ALFF values of the ACC and Hospital Anxiety and Depression Scale-depression scores in CD patients (162). These suggest that ACC may serve as an intersection in the brain, which senses gut inflammation and responses inappropriately, increasing risk of depression.
Indeed, through the leaky regions of the brain-blood barrier, the circumventricular organs or the neural routes, for instance the VN fibers, peripheral cytokines can reach the brain and stimulate the brain immune cells, mainly microglia (163, 164). Apart from this, immune cells like T cells, by upregulating various adhesion molecules and integrins, are attracted by chemokine to infiltrate the brain and produce cytokines in meninges or parenchyma (165). Then, the concentration of cytokines and activated microglia result in neuroinflammation, exerting detrimental effects on neurogenesis in the hippocampus which plays a direct role in the pathophysiology of depression (166–168). Also, by activation of indoleamine 2,3 dioxygenase and tryptophan 2,3 dioxygenase, enzymes catalyzing the conversion of tryptophan into N-formylkynurenine, pro-cytokines decreased tryptophan levels and increased production of kynurenine and other tryptophan-derived metabolites, resulting in less precursors for serotonin synthesis (169, 170). In addition, cytokines, in particular IL-1 through activation of p38 mitogen-activated kinase, inhibited glucocorticoid receptor function, sharing HPA-activating activity (171, 172).
Anti-inflammatory property and epithelial barrier protective role of VNS in the gut
The VN owns an anti-inflammatory property and was first introduced by Tracey (84), called “cholinergic anti-inflammatory pathway” (CAIP) (173). Through the vago-sympathetic reflex, VNS activates the splenic sympathetic nerve which releases norepinephrine binding to the β2 adrenergic receptor of splenic lymphocytes. And the acetylcholine (ACh) delivered by splenic lymphocytes in turn binds to α7 nAChRs of splenic macrophages to finally inhibit the release of TNF-α, a pro-inflammation cytokine (174). Further, the Janus kinase 2-Signal transducer and activator of the transcription 3 signaling pathway is implicated in this anti-inflammatory effect (175). In the gut, similarly, VNS also possesses an anti-inflammatory role depending on enteric neurons. When activated by systemic inflammation or local peripheral inflammation, the VA relay inflammation signals to the NTS, which through projection to the DMNV activates vagal efferents, which then stimulate enteric neurons and, in turn, the enteric neurons activate macrophages by releasing ACh, binding to α7 nAChRs, and inhibiting TNF-α releasing (85). The anti-inflammatory property in the gut of the VN was proved in an animal model of postoperative ileus, independent of the spleen and T cells (18). This effect was also observed in a number of studies of rodent colitis, such as dextran sulfate sodium, oxazolone-, and 2,4,6-trinitrobenzene sulfonic acid-colitis (176–178). Furthermore, in a recent animal study, rats were induced a small intestinal inflammation by indomethacin and VNS reduced the small bowel inflammation in a spleen-independent mechanism, suggesting a direct anti-inflammation effect of the VN in the gut (179).
In addition to the anti-inflammatory property, VNS also displays a direct protective role in intestinal epithelial barrier integrity. Male mice were subjected to a surface area steam burn to induce intestinal epithelial barrier breakdown and intestine inflammation, both of which were attenuated by VNS, either before or after the burn insult, and this preventive effect of VNS in intestine dysfunction was also observed in the traumatic brain injury model (180–182). Latter research identified a role of α7 nAChR and activation of enteric glia cells in this effect (183, 184). Several studies also indicated that electroacupuncture could protect against intestinal hyperpermeability through the VN, as vagotomy or intraperitoneal administration of an α7 nAChR inhibitor reversed this effect (185, 186). In another mice model challenged with LPS, VNS decreased expression of tight junction proteins occludin and zonula occludens through suppressing translocation of NF-κB p65 and downregulating myosin light-chain kinase (187). However, there is no publishing data concerning the effect of VNS on the intestinal epithelial barrier in depressive patients or animal models. Further studies are warranted to determine the relation between this effect and depression.
Linking the depression and gut inflammation: The vagal tone
Importantly, stress inhibits vagal tone and decreased heart rate variability, a marker of low vagal tone, was found in depressed patients, and also in IBD (20, 188, 189). Since increasing vagal tone by VNS displays an anti-inflammation effect in the gut mediated by macrophages, impaired vagal tone including in response to stress, may lead to a pro-inflammatory state in the gut. Indeed, Ghia and colleagues reported that impaired vagal function in mice following induced depression led to gut inflammation while restoration of parasympathetic function through administration of desmethylimipramine, a tricyclic antidepressant, protected against gut inflammation (190). In addition, Ghia et al. found that macrophages isolated from vagotomized mice showed an increase of proinflammatory cytokine release including IL-1β and IL-6 (191). Further, vagotomy reactivated inflammation in mice with chronic colitis. Additionally, in a nationwide register-based matched cohort study, Liu et al. found a positive association between vagotomy and later IBD, particularly CD (192). Together, these findings suggest that stress-induced depressed vagal tone leads to a pro-inflammatory state in the gut. Thus, stress, on the one side, directly wound the gut barrier, promoting gut bacteria translocation and gut inflammation; on the other side, through inhibition of vagal tone, prime immune system induces a pro-inflammatory state and aggravates gut and system inflammation, eventually leading to depression (142) (Figure 2).
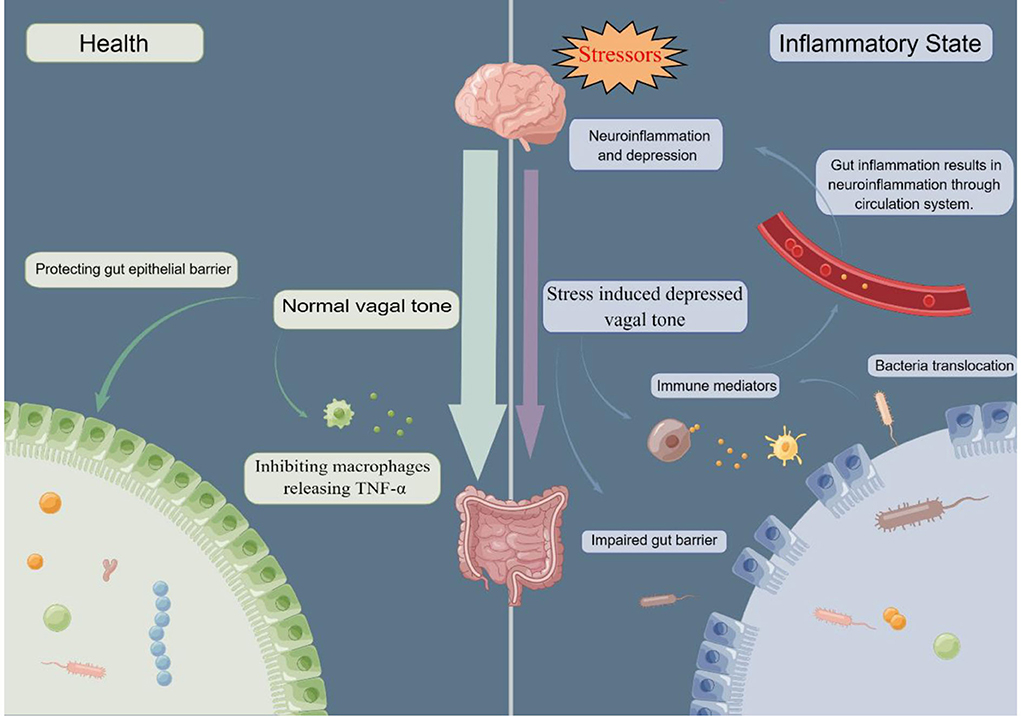
Figure 2. In healthy conditions, normal vagal tone can protect gut barrier and inhibit macrophages releasing pro-cytokine, TNF-α. While in response to stressors, vagal tone is depressed, promoting gut barrier impairment and releasing of TNF-α. Compromised gut barrier facilitate bacterial translocation, which activates immune system and induces immune mediators. Through the circulation system, gut and system inflammation ultimately results in neuroinflammation. TNF-α, tumor necrosis factor alpha. By Figdraw.
Contribution and limitations
In this review, we discussed the relationship between MGBA and depression and the role the VN plays in this relationship, hypothesizing that increasing vagal tone by stimulating the VN, electrically or chemically, may alleviate depressive symptoms through improving gut inflammation and permeability. This hypothesis links stress (risk factor for depression), gut hyperpermeability, and inflammation (found in depressed animals and patients) and the VN, partly explaining the origin of system and neuroinflammation and their contribution to depression. However, there are several limitations. First, to put forward a self-consistent hypothesis, we selectively put inflammation in the intersection and stressed the role of the VN in inflammation-mediator-induced depression models, while in non-inflammatory depression models, the anti-depressive effect of VNS may rely on other mechanisms rather than the anti-inflammatory and gut barrier protective role of the VN. Second, though it is clear that the VN mediates the contribution of disturbance of MGBA to depression, no unanimous conclusion that dysbiosis of GM precedes depressed brain or vice versa can be drawn. In future, more research is required to probe whether in non-inflammatory depression models, stimulating VN can still reverse the dysbiosis of GM to improve depression and to determine a causal relationship between gut and brain disturbances.
Conclusion
Depression is more than a mental disease but with substantial physiological and anatomic alterations including decreased neurogenesis and neuroplasticity, dysfunction of neural circuits, and unbalanced neurotransmitters. Disturbed MGBA was reported to be a contributor to depression and restoration of the disturbance of this axis by administration probiotics is effective in alleviating depressive symptoms. The VN is a pivotal route for bidirectional communication of MGBA, mediating the anti-depressive or pro-depressive effect of gut microbiota on depression. Nevertheless, further research is warranted to determine specific bacteria strains owning an anti-inflammatory role and its molecular basis via the VN. The VN possesses an anti-inflammatory and gut barrier protective role which is inhibited in response to stress, a risk factor of depression. It is likely that the depressed vagal tone may facilitate the gut bacteria translocation and system inflammation, promoting the onset and deterioration of depression. More clinic and pre-clinic studies are needed to test and improve this hypothesis.
Author contributions
YY, QY, JF, YM, and CT conceived the idea and revised extensively this review. QY, YM, and CT searched the literature. CT drafted the manuscript. All authors approve the final manuscript prior to submission.
Funding
This work was supported by China Academy of Chinese Medical Science Innovation Fund (No. CI2021A03301) and National Natural Science Foundation of China (No. 81273833).
Conflict of interest
The authors declare that the research was conducted in the absence of any commercial or financial relationships that could be construed as a potential conflict of interest.
Publisher's note
All claims expressed in this article are solely those of the authors and do not necessarily represent those of their affiliated organizations, or those of the publisher, the editors and the reviewers. Any product that may be evaluated in this article, or claim that may be made by its manufacturer, is not guaranteed or endorsed by the publisher.
References
1. Vos T, Lim SS, Abbafati C, Abbas KM, Abbasi M, Abbasifard M, et al. Global burden of 369 diseases and injuries in 204 countries and territories, 1990–2019: a systematic analysis for the Global Burden of Disease Study 2019. Lancet. (2020) 396:1204–22. doi: 10.1016/S0140-6736(20)30925-9
3. Santomauro DF, Mantilla Herrera AM, Shadid J, Zheng P, Ashbaugh C, Pigott DM, et al. Global prevalence and burden of depressive and anxiety disorders in 204 countries and territories in 2020 due to the COVID-19 pandemic. Lancet. (2021) 398:1700–12. doi: 10.1016/S0140-6736(21)02143-7
4. Ferrari AJ, Norman RE, Freedman G, Baxter AJ, Pirkis JE, Harris MG, et al. The burden attributable to mental and substance use disorders as risk factors for suicide: findings from the global burden of disease study 2010. PLoS ONE. (2014) 9:e91936. doi: 10.1371/journal.pone.0091936
5. Turecki G, Brent DA, Gunnell D, O'Connor RC, Oquendo MA, Pirkis J, et al. Suicide and suicide risk. Nat Rev Dis Primers. (2019) 5:74. doi: 10.1038/s41572-019-0121-0
6. Ng C, How C, Ng Y. Depression in primary care: assessing suicide risk. SMEDJ. (2017) 58:72–7. doi: 10.11622/smedj.2017006
7. Troubat R, Barone P, Leman S, Desmidt T, Cressant A, Atanasova B, et al. Neuroinflammation and depression: a review. Eur J Neurosci. (2021) 53:151–71. doi: 10.1111/ejn.14720
8. Tartt AN, Mariani MB, Hen R, Mann JJ, Boldrini M. Dysregulation of adult hippocampal neuroplasticity in major depression: pathogenesis and therapeutic implications. Mol Psychiatr. (2022) 27:2689–99. doi: 10.1038/s41380-022-01520-y
9. Zhang Z, Huang P, Li S, Liu Z, Zhang J, Li Y, et al. Neural mechanisms underlying the processing of emotional stimuli in individuals with depression: an ALE meta-analysis study. Psychiatry Res. (2022) 313:114598. doi: 10.1016/j.psychres.2022.114598
10. Palepu MSK, Dandekar MP. Remodeling of microbiota gut-brain axis using psychobiotics in depression. Eur J Pharmacol. (2022) 931:175171. doi: 10.1016/j.ejphar.2022.175171
11. Chang L, Wei Y, Hashimoto K. Brain–gut–microbiota axis in depression: a historical overview and future directions. Brain Res Bull. (2022) 182:44–56. doi: 10.1016/j.brainresbull.2022.02.004
12. Musazadeh V, Zarezadeh M, Faghfouri AH, Keramati M, Jamilian P, Jamilian P, et al. Probiotics as an effective therapeutic approach in alleviating depression symptoms: an umbrella meta-analysis. Critic Rev Food Sci Nutr. (2022) 2022:1–9. doi: 10.1080/10408398.2022.2051164
13. Cryan JF, O'Riordan KJ, Cowan CSM, Sandhu KV, Bastiaanssen TFS, Boehme M, et al. The microbiota-gut-brain axis. Physiol Rev. (2019) 99:1877–2013. doi: 10.1152/physrev.00018.2018
14. Breit S, Kupferberg A, Rogler G, Hasler G. Vagus nerve as modulator of the brain-gut axis in psychiatric and inflammatory disorders. Front Psychiatry. (2018) 9:44. doi: 10.3389/fpsyt.2018.00044
15. Munshi S. A depressed gut makes for a depressed brain via vagal transmission. Brain Behav Immun. (2021) 95:15–6. doi: 10.1016/j.bbi.2021.03.017
16. Beurel E, Toups M, Nemeroff CB. The bidirectional relationship of depression and inflammation: double trouble. Neuron. (2020) 107:234–56. doi: 10.1016/j.neuron.2020.06.002
17. Rosas-Ballina M, Olofsson PS, Ochani M, Valdes-Ferrer SI, Levine YA, Reardon C, et al. Acetylcholine-synthesizing T cells relay neural signals in a vagus nerve circuit. Science. (2011) 334:98–101. doi: 10.1126/science.1209985
18. Matteoli G, Gomez-Pinilla PJ, Nemethova A, Di Giovangiulio M, Cailotto C, van Bree SH, et al. A distinct vagal anti-inflammatory pathway modulates intestinal muscularis resident macrophages independent of the spleen. Gut. (2014) 63:938–48. doi: 10.1136/gutjnl-2013-304676
19. Bonaz B, Bazin T, Pellissier S. The vagus nerve at the interface of the microbiota-gut-brain axis. Front Neurosci. (2018) 12:49. doi: 10.3389/fnins.2018.00049
20. Koch C, Wilhelm M, Salzmann S, Rief W, Euteneuer F. A meta-analysis of heart rate variability in major depression. Psychol Med. (2019) 49:1948–57. doi: 10.1017/S0033291719001351
21. Daban C, Martinez-Aran A, Cruz N, Vieta E. Safety and efficacy of vagus nerve stimulation in treatment-resistant depression. A systematic review. J Affect Disord. (2008) 110:1–15. doi: 10.1016/j.jad.2008.02.012
22. Bottomley JM, LeReun C, Diamantopoulos A, Mitchell S, Gaynes BN. Vagus nerve stimulation (VNS) therapy in patients with treatment resistant depression: a systematic review and meta-analysis. Compr Psychiatry. (2020) 98:152156. doi: 10.1016/j.comppsych.2019.152156
23. Rush AJ, George MS, Sackeim HA, Marangell LB, Husain MM, Giller C, et al. Vagus nerve stimulation (VNS) for treatment-resistant depressions: a multicenter study. Biol Psychiatry. (2000) 47:276–86. doi: 10.1016/S0006-3223(99)00304-2
24. Rush AJ, Marangell LB, Sackeim HA, George MS, Brannan SK, Davis SM, et al. Vagus nerve stimulation for treatment-resistant depression: a randomized, controlled acute phase trial. Biol Psychiatry. (2005) 58:347–54. doi: 10.1016/j.biopsych.2005.05.025
25. Rush AJ, Sackeim HA, Marangell LB, George MS, Brannan SK, Davis SM, et al. Effects of 12 months of vagus nerve stimulation in treatment-resistant depression: a naturalistic study. Biol Psychiatry. (2005) 58:355–63. doi: 10.1016/j.biopsych.2005.05.024
26. Nahas Z, Marangell LB, Husain MM, Rush AJ, Sackeim HA, Lisanby SH, et al. Two-year outcome of vagus nerve stimulation (VNS) for treatment of major depressive episodes. J Clin Psychiatry. (2005) 66:1097–104. doi: 10.4088/JCP.v66n0902
27. Schlaepfer TE, Frick C, Zobel A, Maier W, Heuser I, Bajbouj M, et al. Vagus nerve stimulation for depression: efficacy and safety in a European study. Psychol Med. (2008) 38:651–61. doi: 10.1017/S0033291707001924
28. Aaronson ST, Sears P, Ruvuna F, Bunker M, Conway CR, Dougherty DD, et al. A 5-year observational study of patients with treatment-resistant depression treated with vagus nerve stimulation or treatment as usual: comparison of response, remission, and suicidality. Am J Psychiat. (2017) 174:640–8. doi: 10.1176/appi.ajp.2017.16010034
29. Aaronson ST, Carpenter LL, Conway CR, Reimherr FW, Lisanby SH, Schwartz TL, et al. Vagus nerve stimulation therapy randomized to different amounts of electrical charge for treatment-resistant depression: acute and chronic effects. Brain Stimul. (2013) 6:631–40. doi: 10.1016/j.brs.2012.09.013
30. Spindler P, Bohlmann K, Straub H-B, Vajkoczy P, Schneider UC. Effects of vagus nerve stimulation on symptoms of depression in patients with difficult-to-treat epilepsy. Seizure. (2019) 69:77–9. doi: 10.1016/j.seizure.2019.04.001
31. Pardo JV, Sheikh SA, Kuskowski MA, Surerus-Johnson C, Hagen MC, Lee JT, et al. Weight loss during chronic, cervical vagus nerve stimulation in depressed patients with obesity: an observation. Int J Obes. (2007) 31:1756–9. doi: 10.1038/sj.ijo.0803666
32. Cecchini AP, Mea E, Tullo V, Curone M, Franzini A, Broggi G, et al. Vagus nerve stimulation in drug-resistant daily chronic migraine with depression: preliminary data. Neurol Sci. (2009) 30:S101–4. doi: 10.1007/s10072-009-0073-3
33. Sackeim HA, Keilp JG, Rush AJ, George MS, Marangell LB, Dormer JS, et al. The effects of vagus nerve stimulation on cognitive performance in patients with treatment-resistant depression. Neuropsychiatr Neuropsychol Behav Neurol. (2001) 14:53–62.
34. Kong J, Fang J, Park J, Li S, Rong P. Treating depression with transcutaneous auricular vagus nerve stimulation: state of the art and future perspectives. Front Psychiatry. (2018) 9:20. doi: 10.3389/fpsyt.2018.00020
35. Hein E, Nowak M, Kiess O, Biermann T, Bayerlein K, Kornhuber J, et al. Auricular transcutaneous electrical nerve stimulation in depressed patients: a randomized controlled pilot study. J Neural Transm. (2013) 120:821–7. doi: 10.1007/s00702-012-0908-6
36. Liu J, Fang J, Wang Z, Rong P, Hong Y, Fan Y, et al. Transcutaneous vagus nerve stimulation modulates amygdala functional connectivity in patients with depression. J Affect Disord. (2016) 205:319–26. doi: 10.1016/j.jad.2016.08.003
37. Rong P, Liu J, Wang L, Liu R, Fang J, Zhao J, et al. Effect of transcutaneous auricular vagus nerve stimulation on major depressive disorder: a nonrandomized controlled pilot study. J Affect Disord. (2016) 195:172–9. doi: 10.1016/j.jad.2016.02.031
38. Trevizol AP, Shiozawa P, Taiar I, Soares A, Gomes JS, Barros MD, et al. Transcutaneous vagus nerve stimulation (taVNS) for major depressive disorder: an open label proof-of-concept trial. Brain Stimul. (2016) 9:453–4. doi: 10.1016/j.brs.2016.02.001
39. Li S, Zhang Z, Jiao Y, Jin G, Wu Y, Xu F, et al. An assessor-blinded, randomized comparative trial of transcutaneous auricular vagus nerve stimulation (taVNS) combined with cranial electroacupuncture vs. citalopram for depression with chronic pain. Front Psychiatry. (2022) 13:902450. doi: 10.3389/fpsyt.2022.902450
40. Das UN. Vagus nerve stimulation, depression, and inflammation. Neuropsychopharmacology. (2007) 32:2053–4. doi: 10.1038/sj.npp.1301286
41. Liu R-P, Fang J-L, Rong P-J, Zhao Y, Meng H, Ben H, et al. Effects of electroacupuncture at auricular concha region on the depressive status of unpredictable chronic mild stress rat models. Evid-based Complement Altern Med. (2013) 2013:789674. doi: 10.1155/2013/789674
42. Carreno FR, Frazer A. Vagal nerve stimulation for treatment-resistant depression. Neurotherapeutics. (2017) 14:716–27. doi: 10.1007/s13311-017-0537-8
43. Conway CR, Xiong W. The mechanism of action of vagus nerve stimulation in treatment-resistant depression: current conceptualizations. Psychiatric Clin North America. (2018) 41:395–407. doi: 10.1016/j.psc.2018.04.005
44. Prechtl JC, Powley TL. B-Afferents: a fundamental division of the nervous system mediating homeostasis? Behav Brain Sci. (1990) 13:289–300. doi: 10.1017/S0140525X00078729
45. Berthoud H-R, Neuhuber WL. Functional and chemical anatomy of the afferent vagal system. Auton Neurosci. (2000) 85:1–17. doi: 10.1016/S1566-0702(00)00215-0
46. Wang F-B, Powley TL. Vagal innervation of intestines: afferent pathways mapped with new en bloc horseradish peroxidase adaptation. Cell Tissue Res. (2007) 329:221–30. doi: 10.1007/s00441-007-0413-7
47. Bonaz B, Sinniger V, Pellissier S. Therapeutic potential of vagus nerve stimulation for inflammatory bowel diseases. Front Neurosci. (2021) 15:650971. doi: 10.3389/fnins.2021.650971
48. Kaelberer MM, Buchanan KL, Klein ME, Barth BB, Montoya MM, Shen X, et al. (2018). A gut-brain neural circuit for nutrient sensory transduction. Science. 361:eaat5236. doi: 10.1126/science.aat5236
49. Berthoud HR, Blackshaw LA, Brookes SJH, Grundy D. Neuroanatomy of extrinsic afferents supplying the gastrointestinal tract. Neurogastroenterol Motil. (2004) 16:28–33. doi: 10.1111/j.1743-3150.2004.00471.x
50. Bonaz B, Sinniger V, Pellissier S. Vagus nerve stimulation at the interface of brain–gut interactions. Cold Spring Harb Perspect Med. (2019) 9:a034199. doi: 10.1101/cshperspect.a034199
51. Raybould HE. Gut chemosensing: interactions between gut endocrine cells and visceral afferents. Autonomic Neurosci. (2010) 153:41–6. doi: 10.1016/j.autneu.2009.07.007
52. Dockray GJ. Enteroendocrine cell signalling via the vagus nerve. Curr Opin Pharmacol. (2013) 13:954–8. doi: 10.1016/j.coph.2013.09.007
53. Kaelberer MM, Rupprecht LE, Liu WW, Weng P, Bohorquez DV. Neuropod cells: the emerging biology of gut-brain sensory transduction. Annu. Rev. Neurosci. (2020) 43:337–53. doi: 10.1146/annurev-neuro-091619-022657
54. Muller PA, Schneeberger M, Matheis F, Wang P, Kerner Z, Ilanges A, et al. Microbiota modulate sympathetic neurons via a gut–brain circuit. Nature. (2020) 583:441–6. doi: 10.1038/s41586-020-2474-7
55. Fülling C, Dinan TG, Cryan JF. Gut microbe to brain signaling: what happens in vagus…. Neuron. (2019) 101:998–1002. doi: 10.1016/j.neuron.2019.02.008
56. Bogunovic M, Davé SH, Tilstra JS, Chang DTW, Harpaz N, Xiong H, et al. Enteroendocrine cells express functional toll-like receptors. Am J Physiol Gastrointest Liver Physiol. (2007) 292:G1770–83. doi: 10.1152/ajpgi.00249.2006
57. Furness JB, Rivera LR, Cho H-J, Bravo DM, Callaghan B. The gut as a sensory organ. Nat Rev Gastroenterol Hepatol. (2013) 10:729–40. doi: 10.1038/nrgastro.2013.180
58. Bala V, Rajagopal S, Kumar DP, Nalli AD, Mahavadi S, Sanyal AJ, et al. Release of GLP-1 and PYY in response to the activation of G protein-coupled bile acid receptor TGR5 is mediated by Epac/PLC-epsilon pathway and modulated by endogenous H2S. Front Physiol. (2014) 5:420. doi: 10.3389/fphys.2014.00420
59. Yu Y, Yang W, Li Y, Cong Y. Enteroendocrine cells: sensing gut microbiota and regulating inflammatory bowel diseases. Inflamm Bowel Dis. (2020) 26:11–20. doi: 10.1093/ibd/izz217
60. Cummings JH, Pomare EW, Branch WJ, Naylor CP, Macfarlane GT. Short chain fatty acids in human large intestine, portal, hepatic and venous blood. Gut. (1987) 28:1221–7. doi: 10.1136/gut.28.10.1221
61. Koh A, De Vadder F, Kovatcheva-Datchary P, Bäckhed F. From dietary fiber to host physiology: short-chain fatty acids as key bacterial metabolites. Cell. (2016) 165:1332–45. doi: 10.1016/j.cell.2016.05.041
62. Buckley MM, O'Brien R, Brosnan E, Ross RP, Stanton C, Buckley JM, et al. Glucagon-like peptide-1 secreting L-cells coupled to sensory nerves translate microbial signals to the host rat nervous system. Front Cell Neurosci. (2020) 14:95. doi: 10.3389/fncel.2020.00095
63. Dlugosz A, Zakikhany K, Muschiol S, Hultenby K, Lindberg G. Infection of human enteroendocrine cells with Chlamydia trachomatis: a possible model for pathogenesis in irritable bowel syndrome: C. trachomatis in enteroendocrine cells. Neurogastroenterol Motility. (2011) 23:928–34. doi: 10.1111/j.1365-2982.2011.01765.x
64. Dlugosz A, Muschiol S, Zakikhany K, Assadi G, D'Amato M, Lindberg G. Human enteroendocrine cell responses to infection with Chlamydia trachomatis: a microarray study. Gut Pathog. (2014) 6:24. doi: 10.1186/1757-4749-6-24
65. Furness JB. The enteric nervous system and neurogastroenterology. Nat Rev Gastroenterol Hepatol. (2012) 9:286–94. doi: 10.1038/nrgastro.2012.32
66. Rao M, Gershon MD. Enteric nervous system development: what could possibly go wrong? Nat Rev Neurosci. (2018) 19:552–65. doi: 10.1038/s41583-018-0041-0
67. Yoo BB, Mazmanian SK. The enteric network: interactions between the immune and nervous systems of the gut. Immunity. (2017) 46:910–26. doi: 10.1016/j.immuni.2017.05.011
68. Hyland NP, Cryan JF. Microbe-host interactions: influence of the gut microbiota on the enteric nervous system. Dev Biol. (2016) 417:182–7. doi: 10.1016/j.ydbio.2016.06.027
69. Perez-Burgos A, Mao Y, Bienenstock J, Kunze WA. The gut-brain axis rewired: adding a functional vagal nicotinic “sensory synapse.” FASEB J. (2014) 28:3064–74. doi: 10.1096/fj.13-245282
70. Golubeva AV, Joyce SA, Moloney G, Burokas A, Sherwin E, Arboleya S, et al. Microbiota-related changes in bile acid and tryptophan metabolism are associated with gastrointestinal dysfunction in a mouse model of autism. EBioMedicine. (2017) 24:166–78. doi: 10.1016/j.ebiom.2017.09.020
71. Goehler LE, Gaykema RPA, Nguyen KT, Lee JE, Tilders FJH, Maier SF, et al. Interleukin-1β in immune cells of the abdominal vagus nerve: a link between the immune and nervous systems? J. Neurosci. (1999) 19:2799–806. doi: 10.1523/JNEUROSCI.19-07-02799.1999
72. Cook TM, Gavini CK, Jesse J, Aubert G, Gornick E, Bonomo R, et al. Vagal neuron expression of the microbiota-derived metabolite receptor, free fatty acid receptor (FFAR3), is necessary for normal feeding behavior. Mol Metab. (2021) 54:101350. doi: 10.1016/j.molmet.2021.101350
73. Hosoi T, Okuma Y, Matsuda T, Nomura Y. Novel pathway for LPS-induced afferent vagus nerve activation: Possible role of nodose ganglion. Autonom Neurosci. (2005) 120:104–7. doi: 10.1016/j.autneu.2004.11.012
74. Geng S, Yang L, Cheng F, Zhang Z, Li J, Liu W, et al. Gut microbiota are associated with psychological stress-induced defections in intestinal and blood–brain barriers. Front Microbiol. (2020) 10:3067. doi: 10.3389/fmicb.2019.03067
75. Barreau F. Neonatal maternal deprivation triggers long term alterations in colonic epithelial barrier and mucosal immunity in rats. Gut. (2004) 53:501–6. doi: 10.1136/gut.2003.024174
76. Earley ZM, Akhtar S, Green SJ, Naqib A, Khan O, Cannon AR, et al. Burn injury alters the intestinal microbiome and increases gut permeability and bacterial translocation. PLoS ONE. (2015) 10:e0129996. doi: 10.1371/journal.pone.0129996
77. Kelly JR, Kennedy PJ, Cryan JF, Dinan TG, Clarke G, Hyland NP. Breaking down the barriers: the gut microbiome, intestinal permeability and stress-related psychiatric disorders. Front Cell Neurosci. (2015) 9:392. doi: 10.3389/fncel.2015.00392
78. Vanheel H, Vicario M, Vanuytsel T, Van Oudenhove L, Martinez C, Keita ÅV, et al. Impaired duodenal mucosal integrity and low-grade inflammation in functional dyspepsia. Gut. (2014) 63:262–71. doi: 10.1136/gutjnl-2012-303857
79. Vanuytsel T, van Wanrooy S, Vanheel H, Vanormelingen C, Verschueren S, Houben E, et al. Psychological stress and corticotropin-releasing hormone increase intestinal permeability in humans by a mast cell-dependent mechanism. Gut. (2014) 63:1293–9. doi: 10.1136/gutjnl-2013-305690
80. Overman EL, Rivier JE, Moeser AJ. CRF induces intestinal epithelial barrier injury via the release of mast cell proteases and TNF-α. PLoS ONE. (2012) 7:e39935. doi: 10.1371/journal.pone.0039935
81. Shi N, Li N, Duan X, Niu H. Interaction between the gut microbiome and mucosal immune system. Military Med Res. (2017) 4:14. doi: 10.1186/s40779-017-0122-9
82. Jiao Y, Wu L, Huntington ND, Zhang X. Crosstalk between gut microbiota and innate immunity and its implication in autoimmune diseases. Front Immunol. (2020) 11:282. doi: 10.3389/fimmu.2020.00282
83. Bonaz B, Sinniger V, Pellissier S. Vagus nerve stimulation: a new promising therapeutic tool in inflammatory bowel disease. J Intern Med. (2017) 282:46–63. doi: 10.1111/joim.12611
85. Matteoli G, Boeckxstaens GE. The vagal innervation of the gut and immune homeostasis. Gut. (2013) 62:1214–22. doi: 10.1136/gutjnl-2012-302550
86. Steinberg BE, Silverman HA, Robbiati S, Gunasekaran MK, Tsaava T, Battinelli E, et al. Cytokine-specific neurograms in the sensory vagus nerve. Bioelectron Med. (2016) 3:7–17.
87. Strandwitz P. Neurotransmitter modulation by the gut microbiota. Brain Res. (2018) 1693:128–33. doi: 10.1016/j.brainres.2018.03.015
88. Morais LH, Schreiber HL, Mazmanian SK. The gut microbiota–brain axis in behaviour and brain disorders. Nat Rev Microbiol. (2021) 19:241–55. doi: 10.1038/s41579-020-00460-0
89. Bravo JA, Forsythe P, Chew MV, Escaravage E, Savignac HM, Dinan TG, et al. Ingestion of Lactobacillus strain regulates emotional behavior and central GABA receptor expression in a mouse via the vagus nerve. Proc Natl Acad Sci USA. (2011) 108:16050–5. doi: 10.1073/pnas.1102999108
90. Qian L. Submerged fermentation of Lactobacillus rhamnosus YS9 for γ-aminobutyric acid (GABA) production. Braz J Microbiol. (2013) 44:183–7. doi: 10.1590/S1517-83822013000100028
91. Mao Y-K, Kasper DL, Wang B, Forsythe P, Bienenstock J, Kunze WA. Bacteroides fragilis polysaccharide A is necessary and sufficient for acute activation of intestinal sensory neurons. Nat Commun. (2013) 4:1465. doi: 10.1038/ncomms2478
92. Perez-Burgos A, Wang B, Mao Y-K, Mistry B, Neufeld K-AM, Bienenstock J, et al. Psychoactive bacteria Lactobacillus rhamnosus (JB-1) elicits rapid frequency facilitation in vagal afferents. Am J Physiol-Gastroint Liver Physiol. (2013) 304:G211–20. doi: 10.1152/ajpgi.00128.2012
93. Kelly JR, Borre Y, Brien CO, Patterson E, El Aidy S, Deane J, et al. Transferring the blues: Depression-associated gut microbiota induces neurobehavioural changes in the rat. J Psychiatr Res. (2016) 82:109–18. doi: 10.1016/j.jpsychires.2016.07.019
94. Knuesel T, Mohajeri MH. The role of the gut microbiota in the development and progression of major depressive and bipolar disorder. Nutrients. (2022) 14:37. doi: 10.3390/nu14010037
95. Simpson CA, Diaz-Arteche C, Eliby D, Schwartz OS, Simmons JG, Cowan CSM. The gut microbiota in anxiety and depression—a systematic review. Clin Psychol Rev. (2021) 83:101943. doi: 10.1016/j.cpr.2020.101943
96. McGuinness AJ, Davis JA, Dawson SL, Loughman A, Collier F, O'Hely M, et al. A systematic review of gut microbiota composition in observational studies of major depressive disorder, bipolar disorder and schizophrenia. Mol Psychiatry. (2022) 27:1920–35. doi: 10.1038/s41380-022-01456-3
97. Barandouzi ZA, Starkweather AR, Henderson WA, Gyamfi A, Cong XS. Altered composition of gut microbiota in depression: a systematic review. Front Psychiatry. (2020) 11:541. doi: 10.3389/fpsyt.2020.00541
98. Sanada K, Nakajima S, Kurokawa S, Barcelo-Soler A, Ikuse D, Hirata A, et al. Gut microbiota and major depressive disorder: a systematic review and meta-analysis. J Affect Disord. (2020) 266:1–13. doi: 10.1016/j.jad.2020.01.102
99. Cheung SG, Goldenthal AR, Uhlemann A-C, Mann JJ, Miller JM, Sublette ME. Systematic review of gut microbiota and major depression. Front Psychiatry. (2019) 10:34. doi: 10.3389/fpsyt.2019.00034
100. Park AJ, Collins J, Blennerhassett PA, Ghia JE, Verdu EF, Bercik P, et al. Altered colonic function and microbiota profile in a mouse model of chronic depression. Neurogastroenterol Motil. (2013) 25:733–E575. doi: 10.1111/nmo.12153
101. Xiao Q, Shu R, Wu C, Tong Y, Xiong Z, Zhou J, et al. Crocin-I alleviates the depression-like behaviors probably via modulating “microbiota-gut-brain” axis in mice exposed to chronic restraint stress. J Affect Disord. (2020) 276:476–86. doi: 10.1016/j.jad.2020.07.041
102. Karen C, Shyu DJH, Rajan KE. Lactobacillus paracasei supplementation prevents early life stress-induced anxiety and depressive-like behavior in maternal separation model-possible involvement of microbiota-gut-brain axis in differential regulation of microRNA124a/132 and glutamate receptors. Front Neurosci. (2021) 15:719933. doi: 10.3389/fnins.2021.719933
103. Zhang W, Qu W, Wang H, Yan H. Antidepressants fluoxetine and amitriptyline induce alterations in intestinal microbiota and gut microbiome function in rats exposed to chronic unpredictable mild stress. Transl Psychiatry. (2021) 11:131. doi: 10.1038/s41398-021-01254-5
104. Jiang R, Wang Y, Liu J, Wu Z, Wang D, Deng Q, et al. Gut microbiota is involved in the antidepressant effects of adipose-derived mesenchymal stem cells in chronic social defeat stress mouse model. Psychopharmacology. (2022) 239:533–49. doi: 10.1007/s00213-021-06037-w
105. Knudsen JK, Michaelsen TY, Bundgaard-Nielsen C, Nielsen RE, Hjerrild S, Leutscher P, et al. Faecal microbiota transplantation from patients with depression or healthy individuals into rats modulates mood-related behaviour. Sci Rep. (2021) 11:21869. doi: 10.1038/s41598-021-01248-9
106. Li N, Wang Q, Wang Y, Sun A, Lin Y, Jin Y, et al. Fecal microbiota transplantation from chronic unpredictable mild stress mice donors affects anxiety-like and depression-like behavior in recipient mice via the gut microbiota-inflammation-brain axis. Stress. (2019) 22:592–602. doi: 10.1080/10253890.2019.1617267
107. Lv W, Wu X, Chen W, Li Y, Zhang G, Chao L, et al. The gut microbiome modulates the changes in liver metabolism and in inflammatory processes in the brain of chronic unpredictable mild stress rats. Oxidative Med Cell Longev. (2019) 2019:7902874. doi: 10.1155/2019/7902874
108. Pu Y, Zhang Q, Tang Z, Lu C, Wu L, Zhong Y, et al. Fecal microbiota transplantation from patients with rheumatoid arthritis causes depression-like behaviors in mice through abnormal T cells activation. Transl Psychiatry. (2022) 12:223. doi: 10.1038/s41398-022-01993-z
109. Kurokawa S, Kishimoto T, Mizuno S, Masaoka T, Naganuma M, Liang K, et al. The effect of fecal microbiota transplantation on psychiatric symptoms among patients with irritable bowel syndrome, functional diarrhea and functional constipation: an open-label observational study. J Affect Disord. (2018) 235:506–12. doi: 10.1016/j.jad.2018.04.038
110. Lin H, Guo Q, Wen Z, Tan S, Chen J, Lin L, et al. The multiple effects of fecal microbiota transplantation on diarrhea-predominant irritable bowel syndrome (IBS-D) patients with anxiety and depression behaviors. Microb Cell Fact. (2021) 20:233. doi: 10.1186/s12934-021-01720-1
111. Doll JPK, Vazquez-Castellanos JF, Schaub A-C, Schweinfurth N, Kettelhack C, Schneider E, et al. Fecal microbiota transplantation (FMT) as an adjunctive therapy for depression-case report. Front Psychiatry. (2022) 13:815422. doi: 10.3389/fpsyt.2022.815422
112. Kosuge A, Kunisawa K, Arai S, Sugawara Y, Shinohara K, Iida T, et al. Heat-sterilized Bifidobacterium breve prevents depression-like behavior and interleukin-1 beta expression in mice exposed to chronic social defeat stress. Brain Behav Immun. (2021) 96:200–11. doi: 10.1016/j.bbi.2021.05.028
113. Ganji-Arjenaki M, Rafieian-Kopaei M. Probiotics are a good choice in remission of inflammatory bowel diseases: a meta analysis and systematic review. J Cell Physiol. (2018) 233:2091–103. doi: 10.1002/jcp.25911
114. McFarland L, Karakan T, Karatas A. Strain-specific and outcome-specific efficacy of probiotics for the treatment of irritable bowel syndrome: a systematic review and meta-analysis. EClinicalMedicine. (2021) 41:101154. doi: 10.1016/j.eclinm.2021.101154
115. Tong J, Satyanarayanan SK, Su H. Nutraceuticals and probiotics in the management of psychiatric and neurological disorders: a focus on microbiota-gut-brain-immune axis. Brain Behav Immun. (2020) 90:403–19. doi: 10.1016/j.bbi.2020.08.027
116. Dinan TG, Stanton C, Cryan JF. Psychobiotics: A Novel Class of Psychotropic. Biol Psychiatry. (2013) 74:720–6. doi: 10.1016/j.biopsych.2013.05.001
117. Akkasheh G, Kashani-Poor Z, Tajabadi-Ebrahimi M, Jafari P, Akbari H, Taghizadeh M, et al. Clinical and metabolic response to probiotic administration in patients with major depressive disorder: A randomized, double-blind, placebo-controlled trial. Nutrition. (2016) 32:315–20. doi: 10.1016/j.nut.2015.09.003
118. Pinto-Sanchez MI, Hall GB, Ghajar K, Nardelli A, Bolino C, Lau JT, et al. Probiotic Bifidobacterium longum NCC3001 reduces depression scores and alters brain activity: a pilot study in patients with irritable bowel syndrome. Gastroenterology. (2017) 153:448. doi: 10.1053/j.gastro.2017.05.003
119. Liu RT, Walsh RFL, Sheehan AE. Prebiotics and probiotics for depression and anxiety: a systematic review and meta-analysis of controlled clinical trials. Neurosci Biobehav Rev. (2019) 102:13–23. doi: 10.1016/j.neubiorev.2019.03.023
120. Dhaliwal J, Singh DP, Singh S, Pinnaka AK, Boparai RK, Bishnoi M, et al. Lactobacillus plantarum MTCC 9510 supplementation protects from chronic unpredictable and sleep deprivation-induced behaviour, biochemical and selected gut microbial aberrations in mice. J Appl Microbiol. (2018) 125:257–69. doi: 10.1111/jam.13765
121. Ding Y, Bu F, Chen T, Shi G, Yuan X, Feng Z, et al. A next-generation probiotic: Akkermansia muciniphila ameliorates chronic stress-induced depressive-like behavior in mice by regulating gut microbiota and metabolites. Appl Microbiol Biotechnol. (2021) 105:8411–26. doi: 10.1007/s00253-021-11622-2
122. Gao K, Farzi A, Ke X, Yu Y, Chen C, Chen S, et al. Oral administration of Lactococcus lactis WHH2078 alleviates depressive and anxiety symptoms in mice with induced chronic stress. Food Funct. (2022) 13:957–69. doi: 10.1039/d1fo03723d
123. Slykerman RF, Hood F, Wickens K, Thompson JMD, Barthow C, Murphy R, et al. Effect of Lactobacillus rhamnosus HN001 in pregnancy on postpartum symptoms of depression and anxiety: a randomised double-blind placebo-controlled trial. EBioMedicine. (2017) 24:159–65. doi: 10.1016/j.ebiom.2017.09.013
124. Yun S-W, Kim J-K, Lee K-E, Oh YJ, Choi H-J, Han MJ, et al. A probiotic Lactobacillus gasseri alleviates Escherichia coli-induced cognitive impairment and depression in mice by regulating IL-1 beta expression and gut microbiota. Nutrients. (2020) 12:3441. doi: 10.3390/nu12113441
125. Wang S, Ishima T, Zhang J, Qu Y, Chang L, Pu Y, et al. Ingestion of Lactobacillus intestinalis and Lactobacillus reutericauses depression- and anhedonia-like phenotypes in antibiotic-treated mice via the vagus nerve. J Neuroinflamm. (2020) 17:241. doi: 10.1186/s12974-020-01916-z
126. Wang S, Ishima T, Qu Y, Shan J, Chang L, Wei Y, et al. Ingestion of Faecalibaculum rodentium causes depression-like phenotypes in resilient Ephx2 knock-out mice: a role of brain-gut-microbiota axis via the subdiaphragmatic vagus nerve. J Affect Disord. (2021) 292:565–73. doi: 10.1016/j.jad.2021.06.006
127. Pu Y, Tan Y, Qu Y, Chang L, Wang S, Wei Y, et al. A role of the subdiaphragmatic vagus nerve in depression-like phenotypes in mice after fecal microbiota transplantation from Chrna7 knock-out mice with depression-like phenotypes. Brain Behav Immun. (2021) 94:318–26. doi: 10.1016/j.bbi.2020.12.032
128. Konsman JP, Luheshi GN, Bluthé RM, Dantzer R. The vagus nerve mediates behavioural depression, but not fever, in response to peripheral immune signals; a functional anatomical analysis. Eur J Neurosci. (2000) 12:4434–46. doi: 10.1046/j.0953-816x.2000.01319.x
129. Zhang J, Ma L, Chang L, Pu Y, Qu Y, Hashimoto K. A key role of the subdiaphragmatic vagus nerve in the depression-like phenotype and abnormal composition of gut microbiota in mice after lipopolysaccharide administration. Transl Psychiatr. (2020) 10:1–13. doi: 10.1038/s41398-020-00878-3
130. Neufeld K-AM, Bienenstock J, Bharwani A, Champagne-Jorgensen K, Mao Y, West C, et al. Oral selective serotonin reuptake inhibitors activate vagus nerve dependent gut-brain signalling. Sci Rep. (2019) 9:14290. doi: 10.1038/s41598-019-50807-8
131. Dhabhar FS. Effects of stress on immune function: the good, the bad, and the beautiful. Immunol Res. (2014) 58:193–210. doi: 10.1007/s12026-014-8517-0
132. Hammen C. Stress and depression. Annu Rev Clin Psychol. (2005) 1:293–319. doi: 10.1146/annurev.clinpsy.1.102803.143938
133. Konturek PC, Brzozowski T, Konturek SJ. Stress and the gut: pathophysiology, clinical consequences, diagnostic approach and treatment options. J Physiol Pharmacol. (2011) 62:591–9.
134. Hazel NA, Hammen C, Brennan PA, Najman J. Early childhood adversity and adolescent depression: the mediating role of continued stress. Psychol Med. (2008) 38:581–9. doi: 10.1017/S0033291708002857
135. Heim C, Binder EB. Current research trends in early life stress and depression: review of human studies on sensitive periods, gene–environment interactions, and epigenetics. Exp Neurol. (2012) 233:102–11. doi: 10.1016/j.expneurol.2011.10.032
136. Oldehinkel AJ, Ormel J, Verhulst FC, Nederhof E. Childhood adversities and adolescent depression: a matter of both risk and resilience. Dev Psychopathol. (2014) 26:1067–75. doi: 10.1017/S0954579414000534
137. Cruz-Pereira JS, Rea K, Nolan YM, O'Leary OF, Dinan TG, Cryan JF. Depression's unholy trinity: dysregulated stress, immunity, and the microbiome. Annu Rev Psychol. (2020) 71:49–78. doi: 10.1146/annurev-psych-122216-011613
138. Söderholm JD, Perdue MH II. Stress and intestinal barrier function. Am J Physiol Gastrointes Liver Physiol. (2001) 280:G7–G13. doi: 10.1152/ajpgi.2001.280.1.G7
139. Zhang L, Song J, Bai T, Qian W, Hou X-H. Stress induces more serious barrier dysfunction in follicle-associated epithelium than villus epithelium involving mast cells and protease-activated receptor-2. Sci Rep. (2017) 7:4950. doi: 10.1038/s41598-017-05064-y
140. Nozu T, Miyagishi S, Nozu R, Takakusaki K, Okumura T. Altered colonic sensory and barrier functions by CRF: roles of TLR4 and IL-1. J Endocrinol. (2018) 239:241–52. doi: 10.1530/JOE-18-0441
141. Nozu T, Okumura T. Pathophysiological commonality between irritable bowel syndrome and metabolic syndrome: role of corticotropin-releasing factor-toll-like receptor 4-proinflammatory cytokine signaling. J Neurogastroenterol Motil. (2022) 28:173–84. doi: 10.5056/jnm21002
142. Stevens BR, Goel R, Seungbum K, Richards EM, Holbert RC, Pepine CJ, et al. Increased human intestinal barrier permeability plasma biomarkers zonulin and FABP2 correlated with plasma LPS and altered gut microbiome in anxiety or depression. Gut. (2018) 67:1555–7. doi: 10.1136/gutjnl-2017-314759
143. Maes M, Kubera M, Leunis J-C. The gut-brain barrier in major depression: Intestinal mucosal dysfunction with an increased translocation of LPS from gram negative enterobacteria (leaky gut) plays a role in the inflammatory pathophysiology of depression. Neuroendocrinol Lett. (2008) 29:117–24.
144. Moussaoui N, Jacobs JP, Larauche M, Biraud M, Million M, Mayer E, et al. Chronic early-life stress in rat pups alters basal corticosterone, intestinal permeability, and fecal microbiota at weaning: influence of sex. J Neurogastroenterol Motil. (2017) 23:135–43. doi: 10.5056/jnm16105
145. Simeonova D, Stoyanov D, Leunis J, Carvalho AF, Kubera M, Murdjeva M, et al. Increased serum immunoglobulin responses to gut commensal gram-negative bacteria in unipolar major depression and bipolar disorder type 1, especially when melancholia is present. Neurotox Res. (2020) 37:338–48. doi: 10.1007/s12640-019-00126-7
146. Grigoleit J-S, Kullmann JS, Wolf OT, Hammes F, Wegner A, Jablonowski S, et al. Dose-dependent effects of endotoxin on neurobehavioral functions in humans. PLoS ONE. (2011) 6:e28330. doi: 10.1371/journal.pone.0028330
147. Feng L, Xia Y, Garcia GE, Hwang D, Wilson CB. Involvement of reactive oxygen intermediates in cyclooxygenase-2 expression induced by interleukin-1, tumor necrosis factor-alpha, and lipopolysaccharide. J Clin Invest. (1995) 95:1669–75. doi: 10.1172/JCI117842
148. Lin W-N, Lin C-C, Cheng H-Y, Yang C-M. Regulation of cyclooxygenase-2 and cytosolic phospholipase A2 gene expression by lipopolysaccharide through the RNA-binding protein HuR: involvement of NADPH oxidase, reactive oxygen species and mitogen-activated protein kinases: LPS-induced COX-2 and cPLA2 expression. Br J Pharmacol. (2011) 163:1691–706. doi: 10.1111/j.1476-5381.2011.01312.x
149. Berk M, Williams LJ, Jacka FN, O'Neil A, Pasco JA, Moylan S, et al. So depression is an inflammatory disease, but where does the inflammation come from? BMC Med. (2013) 11:200. doi: 10.1186/1741-7015-11-200
150. Blaser H, Dostert C, Mak TW, Brenner D. TNF and ROS Crosstalk in inflammation. Trends Cell Biol. (2016) 26:249–61. doi: 10.1016/j.tcb.2015.12.002
151. Lamers F, Milaneschi Y, Smit JH, Schoevers RA, Wittenberg G, Penninx BWJH. Longitudinal association between depression and inflammatory markers: results from the Netherlands study of depression and anxiety. Biol Psychiatry. (2019) 85:829–37. doi: 10.1016/j.biopsych.2018.12.020
152. Osimo EF, Baxter LJ, Lewis G, Jones PB, Khandaker GM. Prevalence of low-grade inflammation in depression: a systematic review and meta-analysis of CRP levels. Psychol Med. (2019) 49:1958–70. doi: 10.1017/S0033291719001454
153. Schiepers OJG, Wichers MC, Maes M. Cytokines and major depression. Prog Neuro-Psychopharmacol Biol Psychiatry. (2005) 29:201–17. doi: 10.1016/j.pnpbp.2004.11.003
154. Rodrigues FT, de Souza MR, de Carvalho Lima CN, da Silva FE, da Silva Costa DV, Dos Santos CC, et al. (2018). Major depression model induced by repeated and intermittent lipopolysaccharide administration: long-lasting behavioral, neuroimmune and neuroprogressive alterations. J Psychiatr Res. 107, 57–67. doi: 10.1016/j.jpsychires.2018.10.003
155. Marrie RA, Graff LA, Fisk JD, Patten SB, Bernstein CN. The relationship between symptoms of depression and anxiety and disease activity in IBD over time. Inflamm Bowel Dis. (2021) 27:1285–93. doi: 10.1093/ibd/izaa349
156. Heydarpour P, Rahimian R, Fakhfouri G, Khoshkish S, Fakhraei N, Salehi-Sadaghiani M, et al. Behavioral despair associated with a mouse model of Crohn's disease: role of nitric oxide pathway. Prog Neuro-Psychopharmacol Biol Psychiatry. (2016) 64:131–41. doi: 10.1016/j.pnpbp.2015.08.004
157. Haj-Mirzaian A, Amiri S, Amini-Khoei H, Hosseini M-J, Haj-Mirzaian A, Momeny M, et al. Anxiety- and depressive-like behaviors are associated with altered hippocampal energy and inflammatory status in a mouse model of Crohn's disease. Neuroscience. (2017) 366:124–37. doi: 10.1016/j.neuroscience.2017.10.023
158. Miller AH, Haroon E, Raison CL, Felger JC. Cytokine targets in the brain: impact on neurotransmitters and neurocircuits. Depress Anxiety. (2013) 30:297–306. doi: 10.1002/da.22084
159. Tsai P-J, Keeley RJ, Carmack SA, Vendruscolo JCM, Lu H, Gu H, et al. Converging structural and functional evidence for a rat salience network. Biol Psychiatry. (2020) 88:867–78. doi: 10.1016/j.biopsych.2020.06.023
160. Rolls ET, Cheng W, Gong W, Qiu J, Zhou C, Zhang J, et al. Functional connectivity of the anterior cingulate cortex in depression and in health. Cereb Cortex. (2019) 29:3617–30. doi: 10.1093/cercor/bhy236
161. Kong N, Gao C, Xu M, Gao X. Changes in the anterior cingulate cortex in Crohn's disease: a neuroimaging perspective. Brain Behav. (2021) 11:e02003. doi: 10.1002/brb3.2003
162. Kong N, Gao C, Zhang F, Zhang M, Yue J, Lv K, et al. Neurophysiological effects of the anterior cingulate cortex on the exacerbation of Crohn's disease: a combined fMRI-MRS study. Front Neurosci. (2022) 16:840149. doi: 10.3389/fnins.2022.840149
163. Vitkovic L, Konsman JP, Bockaert J, Dantzer R, Homburger V, Jacque C. Cytokine signals propagate through the brain. Mol Psychiatr. (2000) 5:604–15. doi: 10.1038/sj.mp.4000813
164. Miller AH, Raison CL. The role of inflammation in depression: from evolutionary imperative to modern treatment target. Nat Rev Immunol. (2016) 16:22–34. doi: 10.1038/nri.2015.5
165. Lewitus GM, Cohen H, Schwartz M. Reducing post-traumatic anxiety by immunization. Brain Behav Immun. (2008) 22:1108–14. doi: 10.1016/j.bbi.2008.05.002
166. Sahay A, Hen R. Adult hippocampal neurogenesis in depression. Nat Neurosci. (2007) 10:1110–5. doi: 10.1038/nn1969
167. Snyder JS, Soumier A, Brewer M, Pickel J, Cameron HA. Adult hippocampal neurogenesis buffers stress responses and depressive behaviour. Nature. (2011) 476:458–U112. doi: 10.1038/nature10287
168. Kim Y-K, Na K-S, Myint A-M, Leonard BE. The role of pro-inflammatory cytokines in neuroinflammation, neurogenesis and the neuroendocrine system in major depression. Prog Neuro-Psychopharmacol Biol Psychiatry. (2016) 64:277–84. doi: 10.1016/j.pnpbp.2015.06.008
169. Dantzer R, O'Connor JC, Freund GG, Johnson RW, Kelley KW. From inflammation to sickness and depression: when the immune system subjugates the brain. Nat Rev Neurosci. (2008) 9:46–57. doi: 10.1038/nrn2297
170. Le Floc'h N, Otten W, Merlot E. Tryptophan metabolism, from nutrition to potential therapeutic applications. Amino Acids. (2011) 41:1195–205. doi: 10.1007/s00726-010-0752-7
171. Wang X, Wu H, Miller AH. Interleukin 1 alpha (IL-1 alpha) induced activation of p38 mitogen-activated protein kinase inhibits glucocorticoid receptor function. Mol Psychiatr. (2004) 9:65–75. doi: 10.1038/sj.mp.4001339
172. Dunn AJ. Cytokine activation of the HPA axis. Ann NY Acad Sci. (2006) 917:608–17. doi: 10.1111/j.1749-6632.2000.tb05426.x
173. Borovikova LV, Ivanova S, Zhang M, Yang H, Botchkina GI, Watkins LR, et al. Vagus nerve stimulation attenuates the systemic inflammatory response to endotoxin. Nature. (2000) 405:458–62. doi: 10.1038/35013070
174. Bonaz B, Sinniger V, Pellissier S. Anti-inflammatory properties of the vagus nerve: potential therapeutic implications of vagus nerve stimulation: anti-inflammatory effect of vagus nerve stimulation. J Physiol. (2016) 594:5781–90. doi: 10.1113/JP271539
175. de Jonge WJ, van der Zanden EP, The FO Bijlsma MF, van Westerloo DJ, Bennink RJ. Stimulation of the vagus nerve attenuates macrophage activation by activating the Jak2-STAT3 signaling pathway. Nat Immunol. (2005) 6:844–51. doi: 10.1038/ni1229
176. Ghia JE, Blennerhassett P, Kumar-Ondiveeran H, Verdu EF, Collins SM. The vagus nerve: A tonic inhibitory influence associated with inflammatory bowel disease in a murine model. Gastroenterology. (2006) 131:1122–30. doi: 10.1053/j.gastro.2006.08.016
177. Bai A, Guo Y, Lu N. The effect of the cholinergic anti-inflammatory pathway on experimental colitis. Scand J Immunol. (2007) 66:538–45. doi: 10.1111/j.1365-3083.2007.02011.x
178. Meregnani J, Clarencon D, Vivier M, Peinnequin A, Mouret C, Sinniger V, et al. Anti-inflammatory effect of vagus nerve stimulation in a rat model of inflammatory bowel disease. Auton Neurosci-Basic Clin. (2011) 160:82–9. doi: 10.1016/j.autneu.2010.10.007
179. Caravaca AS, Levine YA, Drake A, Eberhardson M, Olofsson PS. Vagus nerve stimulation reduces indomethacin-induced small bowel inflammation. Front Neurosci. (2022) 15:730407. doi: 10.3389/fnins.2021.730407
180. Costantini TW, Bansal V, Peterson CY, Loomis WH, Putnam JG, Rankin F, et al. Efferent vagal nerve stimulation attenuates gut barrier injury after burn: modulation of intestinal occludin expression. J Trauma-Injury Infect Crit Care. (2010) 68:1349–54. doi: 10.1097/TA.0b013e3181dccea0
181. Krzyzaniak M, Peterson C, Loomis W, Hageny A-M, Wolf P, Reys L, et al. Postinjury vagal nerve stimulation protects against intestinal epithelial barrier breakdown. J Trauma-Injury Infect Crit Care. (2011) 70:1168–75. doi: 10.1097/TA.0b013e318216f754
182. Bansal V, Costantini T, Ryu SY, Peterson C, Loomis W, Putnam J, et al. Stimulating the central nervous system to prevent intestinal dysfunction after traumatic brain injury. J Trauma-Injury Infect Crit Care. (2010) 68:1059–64. doi: 10.1097/TA.0b013e3181d87373
183. Costantini TW, Bansal V, Krzyzaniak M, Putnam JG, Peterson CY, Loomis WH, et al. Vagal nerve stimulation protects against burn-induced intestinal injury through activation of enteric glia cells. Am J Physiol-Gastroint Liver Physiol. (2010) 299:G1308–18. doi: 10.1152/ajpgi.00156.2010
184. Costantini TW, Krzyzaniak M, Cheadle GA, Putnam JG, Hageny A-M, Lopez N, et al. Targeting alpha-7 nicotinic acetylcholine receptor in the enteric nervous system a cholinergic agonist prevents gut barrier failure after severe burn injury. Am J Pathol. (2012) 181:478–86. doi: 10.1016/j.ajpath.2012.04.005
185. Du M-H, Luo H-M, Hu S, Lv Y, Lin Z-L, Ma L. Electroacupuncture improves gut barrier dysfunction in prolonged hemorrhagic shock rats through vagus anti-inflammatory mechanism. World J Gastroenterol. (2013) 19:5988–99. doi: 10.3748/wjg.v19.i36.5988
186. Hu S, Du M-H, Luo H-M, Wang H, Lv Y, Ma L, et al. Electroacupuncture at zusanli (ST36) prevents intestinal barrier and remote organ dysfunction following gut ischemia through activating the cholinergic anti-inflammatory-dependent mechanism. Evid-Based Complement Altern Med. (2013) 2013:592127. doi: 10.1155/2013/592127
187. Zhou H, Liang H, Li Z-F, Xiang H, Liu W, Li J-G. Vagus nerve stimulation attenuates intestinal epithelial tight junctions disruption in endotoxemic mice through alpha 7 nicotinic acetylcholine receptors. Shock. (2013) 40:144–51. doi: 10.1097/SHK.0b013e318299e9c0
188. Pellissier S, Dantzer C, Canini F, Mathieu N, Bonaz B. Psychological adjustment and autonomic disturbances in inflammatory bowel diseases and irritable bowel syndrome. Psychoneuroendocrinology. (2010) 35:653–62. doi: 10.1016/j.psyneuen.2009.10.004
189. Pellissier S, Dantzer C, Mondillon L, Trocme C, Gauchez A-S, Ducros V, et al. Relationship between vagal tone, cortisol, tnf-alpha, epinephrine and negative affects in crohn's disease and irritable bowel syndrome. PLoS ONE. (2014) 9:e105328. doi: 10.1371/journal.pone.0105328
190. Ghia J-E, Blennerhassett P, Collins SM. Impaired parasympathetic function increases susceptibility to inflammatory bowel disease in a mouse model of depression. J Clin Invest. (2008) 118:2209–18. doi: 10.1172/JCI32849
191. Ghia J-E, Park AJ, Blennerhassett P, Khan WI, Collins SM. Adoptive transfer of macrophage from mice with depression-like behavior enhances susceptibility to colitis. Inflamm Bowel Dis. (2011) 17:1474–89. doi: 10.1002/ibd.21531
192. Liu B, Wanders A, Wirdefeldt K, Sjolander A, Sachs MC, Eberhardson M, et al. Vagotomy and subsequent risk of inflammatory bowel disease: a nationwide register-based matched cohort study. Aliment Pharmacol Ther. (2020) 51:1022–30. doi: 10.1111/apt.15715
193. Baiao R, Capitao LP, Higgins C, Browning M, Harmer CJ, Burnet PWJ. Multispecies probiotic administration reduces emotional salience and improves mood in subjects with moderate depression: a randomised, double-blind, placebo-controlled study. Psychol Med. (2022) 2022:PII S003329172100550X. doi: 10.1017/S003329172100550X
194. Bisgaard TH, Allin KH, Keefer L, Ananthakrishnan AN, Jess T. Depression and anxiety in inflammatory bowel disease: epidemiology, mechanisms and treatment. Nat Rev Gastroenterol Hepatol. (2022) 2022:1–10. doi: 10.1038/s41575-022-00634-6
Keywords: vagus nerve, depressive disorder, gut microbiota, microbiota-gut-brain axis, gut permeability
Citation: Tan C, Yan Q, Ma Y, Fang J and Yang Y (2022) Recognizing the role of the vagus nerve in depression from microbiota-gut brain axis. Front. Neurol. 13:1015175. doi: 10.3389/fneur.2022.1015175
Received: 09 August 2022; Accepted: 12 September 2022;
Published: 10 November 2022.
Edited by:
Hideki Ohira, Nagoya University, JapanReviewed by:
Ning Kong, Zhejiang Chinese Medical University, ChinaKenji Hashimoto, Chiba University, Japan
Copyright © 2022 Tan, Yan, Ma, Fang and Yang. This is an open-access article distributed under the terms of the Creative Commons Attribution License (CC BY). The use, distribution or reproduction in other forums is permitted, provided the original author(s) and the copyright owner(s) are credited and that the original publication in this journal is cited, in accordance with accepted academic practice. No use, distribution or reproduction is permitted which does not comply with these terms.
*Correspondence: Yongsheng Yang, yysh1950@sina.com