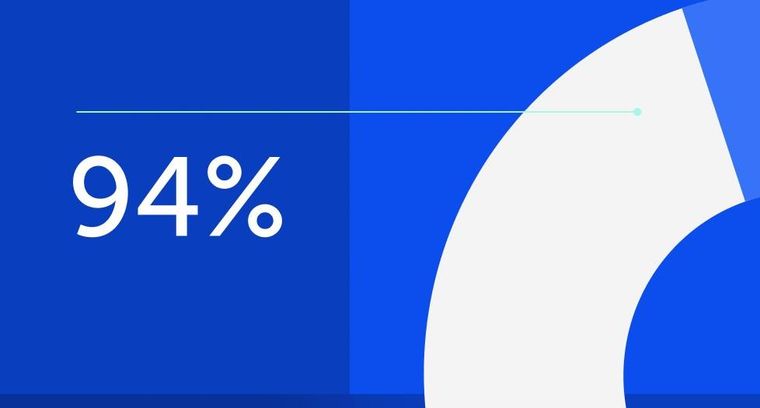
94% of researchers rate our articles as excellent or good
Learn more about the work of our research integrity team to safeguard the quality of each article we publish.
Find out more
ORIGINAL RESEARCH article
Front. Neurol., 12 November 2020
Sec. Neurotrauma
Volume 11 - 2020 | https://doi.org/10.3389/fneur.2020.539627
Objective: To investigate the effects of the apolipoprotein E gene (APOE) on the cerebral oxygen saturation of patients after traumatic brain injury (TBI).
Methods: Clinical data of 114 patients with TBI and 54 normal people were collected. The APOE genotypes of all subjects were determined by quantitative fluorescent polymerase chain reaction (QF-PCR). The regional cerebral oxygen saturation (rScO2) of TBI patients and normal people were monitored by near-infrared spectroscopy (NIRS).
Results: The mean rScO2 of patients was (55.06 ± 7.60)% in the early stage of TBI, which was significantly lower than that of normal people (67.21 ± 7.80)% (P < 0.05). Single-factor and multifactor logistic regression analyses showed APOEε4 was an independent risk factor that caused the early decline of rScO2 in TBI patients. Furthermore, in the TBI group, the rScO2 of APOEε4 carriers (52.23 ± 8.02)% was significantly lower than that of non-ε4 carriers (60.33 ± 7.12)% (P < 0.05). But in the normal group, no significant differences in rScO2 were found between APOEε4 carriers and non-carriers.
Conclusion: The rScO2 may be significantly decreased after TBI, and APOEε4 may be a risk factor for decreased rScO2 in the early stage of TBI.
Traumatic brain injury (TBI) is a common disease with high disability and mortality in the neurointensive care unit (NICU) (1–3). Cerebral blood flow often changes after TBI, which may lead to a series of pathological responses and irreversible brain damage (4, 5). As a result, TBI is often followed by ischemia and hypoxia, resulting in severe neurological impairment and death. Previous studies have shown that ischemia and hypoxia are strongly associated with poor outcomes (6, 7), and a decrease of cerebral oxygen saturation usually indicates the possibility of cerebral ischemia and hypoxia. Therefore, cerebral oxygen saturation monitoring is an important method to evaluate the condition of TBI patients during neurointensive care (8).
Near-infrared spectroscopy (NIRS) is a useful method to monitor regional cerebral oxygen saturation (rScO2) (9, 10). It has a good correlation with the jugular bulb oxygen saturation (SvjO2) which is considered a gold standard for brain oxygen metabolism (11) and is helpful for timely detection and correction of cerebral ischemia and hypoxia (12).
Apolipoprotein E gene (APOE) can affect the prognosis of TBI patients, and APOEε4, a subtype of APOE, is considered as a risk factor for exacerbations of TBI outcome (13–16). However, the mechanism through which APOEε4 influences the prognosis of TBI patients remains unclear. In previous studies, we have found that APOE may affect the blood-brain barrier permeability of mice after TBI (17), but it is unknown whether it affects cerebral blood flow and cerebral oxygen saturation. Therefore, as soon as we discovered the phenomenon by accident in our daily clinical work that some TBI patients with APOEε4 had lower cerebral oxygen saturation, it aroused our interest immediately. So we proposed the hypothesis APOEε4 might be related to lower rScO2 as compared with APOEε2 and APOEε3 after TBI. To verify our hypothesis, we explored the relationship between APOE and rScO2 in the early stage of TBI in this study. The rScO2 was measured by NIRS, and the APOE types of the subjects were determined by quantitative fluorescent polymerase chain reac (QF-PCR).
This is a retrospective study that complies with the ethical standards formulated by the Ethics Committee of the First Affiliated Hospital of Chongqing Medical University and has received its approval. The review batch number is 2019 Research Ethics (2019-026). Subjects included in this study were admitted to the hospital from March 2018 and to May 2019. For normal people and those patients who were conscious and cooperative, written informed consent were obtained from both patients and their legal guardians. For comatose patients, written informed consent was obtained from their legal guardians.
Inclusion criteria were patients with clear head injury history, admitted to hospital within 1–3 days after injury, and aged 15–65. Exclusion criteria were: (1) having a medical history that may affect cerebral oxygen saturation (e.g., TBI, cerebrovascular diseases, intracranial space-occupying diseases, encephalitis, psychosis or dementia, etc.); (2) with use of drugs that may affect cerebral oxygen saturation; (3) suffering from the respiratory circulatory system that seriously influences the cerebral oxygen saturation; (4) having serious scalp injury affecting the monitoring of cerebral oxygen saturation; (5) having serious multiple injuries complicated by severe dysfunction of other organs or systems (such as severe respiratory and circulatory dysfunction, electrolyte disorders, etc.).
According to the inclusion and exclusion criteria, TBI patients with medical histories that may affect cerebral oxygen saturation were excluded. All TBI patients were treated according to the guidelines of TBI (7, 18). In detail, for TBI patients, the possible factors that may influence the levels of rScO2 and blood oxygen saturation (SO2) were removed, such as cleaning and keeping the respiratory tract unobstructed, performing endotracheal intubation or tracheotomy, and utilizing a respirator. Meanwhile, oxygen inhalation was given to TBI patients to keep vital signs stable, partial pressure of oxygen (PO2), and partial pressure of carbon dioxide (PCO2) levels within a normal range.
Inclusion criteria were normal people without a history of TBI and aged 15–65. Exclusion criteria were: (1) suffering from nervous system diseases (e.g., cerebrovascular diseases, intracranial space-occupying lesions, encephalitis, psychosis, or dementia, etc.); (2) having respiratory and circulatory system diseases that severely affect cerebral oxygen saturation; (3) with use of drugs that may affect cerebral oxygen saturation.
Quantitative fluorescent polymerase chain reaction (QF-PCR) was used to determine the APOE genotype of the subjects. The steps were as follows: (1) 2 ml venous blood was collected from the subject to extract the DNA by using the QIAGEN DNA extraction kit; (2) 2 ul genomic DNA of the sample was added into the reaction tube containing 4 kinds of PCR reaction solutions by using the human APOE gene detection kit (Wuhan Youzhiyou Medical Technology Co., Ltd.); (3) the PCR reaction tube was removed to the nucleic acid amplification area for detection; and (4) determine the APOE genotype (Table 1 and Figure 1).
Figure 1. APOE genotype of the subjects was identified as ε2/ε4. (A) APOEε2 gene locus FAM channel (+) VIC channel (+), (B) APOEε4 gene locus FAM channel (+) VIC channel (+). Polymerase chain reaction multiple fluorescence quantitative determination (QF-PCR) was utilized in the present study.
MINR-P100 non-invasive cerebral blood oxygen monitor (Chongqing Mingxi Medical Equipment Co., Ltd.) was used in the present study to monitor rScO2. For TBI patients, the monitoring of rScO2 by NIRS was performed during 1–3 days after TBI. Meanwhile, NIRS was also used immediately to monitor the rScO2 of patients once their condition changed. For normal people, the monitoring of rScO2 by NIRS was performed under a relaxed and awake condition. The forehead skin was exposed and cleaned, and the probes were closely attached and fixed to bilateral forehead skin. The probes were located 1–2 cm from the upper edge of the eyebrow arch, then rScO2 data was detected and collected. The average monitoring time was 30 min (Standard division, ±0.5) for each patient, then an average rScO2 value was obtained from the NIRS machine.
The SPSS 25.0 statistical software was applied in the study. Between-group differences in rScO2 value were tested with the use of Student's t-tests, and differences in categorical outcomes with the use of Chi-square (χ2) tests. Single-factor and multifactor logistic regression analysis were applied to analyze independent risk factors affecting the change of rScO2, and P < 0.05 was considered statistically significant.
A total of 176 consecutive TBI patients were collected in this study, and 8 patients were excluded according to the inclusion and exclusion criteria. Therefore, 168 subjects including 114 TBI patients and 54 normal people were enrolled.
APOE has three alleles (APOEε2, APOEε3, and APOEε4), which encode six phenotypes including three homozygotes (ε2/2, ε3/ε3, ε4/ε4) and three heterozygotes (ε2/ε3, ε3/ε4, ε2/ε4). The gene distribution and allele frequency of the TBI group and the normal group in this study are consistent with Hardy-Weinberg's law (Table 2).
A total of 168 patients were included in this study, including 114 TBI patients and 54 normal people. The general clinical data, such as gender, age, smoking, alcohol-drinking, and hypertension, showed no significant difference between ε4 carriers and ε4 non-carriers in both the TBI group and normal group (P > 0.05 by Chi-square tests for differences in the composition of these data) (Tables 3, 4).
The rScO2 data were compared between the TBI group and the normal group by t-test. We found that the mean rScO2 of TBI patients was (55.06 ± 7.60)%, which was significantly lower than that ((67.21 ± 7.80)%) of the normal group (P < 0.001 by Student's t-test, Figure 2). This indicated that the rScO2 of patients in the early stage of TBI was significantly lower than that of normal people.
Figure 2. The rScO2 (55.06 ± 7.60)% of the TBI group was significantly lower than that of the normal group (67.21 ± 7.80)% (*P < 0.05). (A) Shows the distribution of data in two groups, (B) indicates the mean ± standard division.
In the present study, patients with rScO2 below 55% (29 patients) were considered having hypoxia (19), while patients with rScO2 above 55% were considered non-hypoxia (85 patients). Single-factor logistic regression analysis showed APOEε4 (P < 0.001), GCS (P = 0.001), Marshall CT Class (P = 0.013), and hypertension (P = 0.002) were independent risk factors for decreased rScO2 after TBI. Furthermore, multifactor logistic regression analysis also showed that APOEε4 (P = 0.013, OR = 6.742, 95% CI = 1.364–30.125), GCS (P = 0.041, OR = 4.591, 95% CI = 1.587–19.512), Marshall CT Class (P = 0.007, OR = 7.140, 95% CI = 0.775–22.145) and hypertension (P = 0.023, OR = 4.462, 95% CI = 1.228–16.239) were independent risk factors that related to the decrease of rScO2 after TBI (Table 5).
Table 5. Independent risk factors affecting rScO2 in the TBI group by single-factor and multi-factor logistic regression analysis.
According to Table 5, APOEε4 was an independent risk factor that affected rScO2 of patients in the early stage of TBI. To further study the influence of APOEε4 on rScO2, both TBI patients and normal people were divided into ε4 non-carriers group and ε4 carriers group. Statistical analysis showed that, in the early stage of TBI, the rScO2 [(52.23±8.02)%] of ε4 carriers was remarkably lower than that of ε4 non-carriers [(60.33 ± 7.12)%], which was statistically significant (P < 0.001 by Student's t-test). Meanwhile, the rScO2 of ε4 non-carriers and ε4 carriers in the normal group was (68.37 ± 5.56) and (68.75 ± 5.49)% (Table 6 and Figure 3) respectively, indicating no significant difference (P > 0.05 by Student's t-test).
Figure 3. The rScO2 of ε4 carriers in the TBI group was significantly lower than that of ε4 non-carriers, which was statistically significant (*P < 0.05). However, the rScO2 of ε4 carriers in the normal control group did not show a significant decrease compared with ε4 non-carriers (#P > 0.05) in (A,B).
Furthermore, in the TBI group, up to 57.89% (11 of 19) ε4 carriers had hypoxia, which was significantly higher than that (18.95%, 18 of 95) of ε4 non-carriers (P = 0.001 by Chi-square tests). However, in the normal group, only 11.11% (1of 9) ε4 carriers and 4.44% (2 of 45) ε4 non-carriers showed hypoxia, which showed no statistical significance (P = 0.428 by Chi-square tests) (Table 6).
As shown in the results, the mean rScO2 of TBI patients (55.06 ± 7.60)% in the early stage of TBI was significantly lower than that of normal people (67.21 ± 7.80)% (P < 0.05), which suggested that the rScO2 of patients significantly decreased in the early stage of TBI. Cerebral blood flow often decreased after TBI, which may lead to ischemia and hypoxia in brain tissue and result in irreversible brain damage. Relevant literature reported that more than 90% of people who died of TBI may have secondary cerebral ischemia and hypoxia (20). Similarly, the results of this study also showed the rScO2 of patients was significantly decreased in the early stage of TBI as compared with normal people. Furthermore, the rScO2 was considered abnormal when it was below 55%, and an over 12% decrease in rScO2 usually indicated the possibility of cerebral ischemia, which needs clinical intervention (7, 19, 21). Therefore, timely and accurate monitoring of rScO2 is extremely important for TBI patients in the NICU.
Presently, multiple methods including NIRS are used to monitor and evaluate the condition of TBI patients in the NICU, such as brain tissue oxygen tension (PbtO2), jugular venous oxygen saturation (SvjO2), cerebral microdialysis, thermal diffusion measurement of cerebral blood flow, and electroencephalogram (EEG) (22). As compared with PbtO2 and SvjO2, NIRS is non-invasive, continuous, more convenient and safe. In the NICU, the cerebral oxygen metabolism can be monitored by NIRS continuously, which shows not only the dynamic changes of rScO2 in real-time but also the mean rScO2 (23, 24). In our NICU, NIRS is a routine method to monitor the rScO2 of patients, which provides important information about cerebral oxygen metabolism to us in real-time. Proposed by Franz Jobsis in 1977, NIRS is based on the permeability of biological tissues to near-infrared spectrum (wavelength 700–1,000 nm) and different absorbed light waves for chromophores, such as hemoglobin and reduced hemoglobin, to achieve continuous and non-invasive monitoring of human tissue oxygenation saturation. It has been found that the optical properties of brain tissue will change when cerebral hemorrhage and cerebral ischemia occur. Zweifel et al. observed that the increase of rScO2 is consistent with the relief of vasospasm and the improvement of clinical symptoms through arterial imaging, and the arterial spasm is significantly related to the decrease of rScO2 on the same side, which suggested that changes of rScO2 may reflect the severity of TBI (25). In this study, we also found the level of GCS and Marshall CT Class were the risk factors affecting rScO2 in the early stage of TBI, indicating that rScO2 was related to the severity of TBI.
Previously, we have first shown that, in the cohort of mainland Chinese patients, APOEε4 carriers were more prone to clinical deterioration in the acute phase after TBI as compared with APOEε4 non-carriers (16). To further explore the influence of APOE on TBI outcomes, a series of studies were carried out in both the clinic and laboratory. In the present clinical study, we used NIRS to monitor rScO2 of TBI patients in the NICU. Through single-factor and multifactor logistic analysis, we found APOEε4 is an independent risk factor that caused the early decline of rScO2 in TBI patients. To further verify this speculation, the rScO2 of APOEε4 carriers and non-carriers both in the TBI group and the normal group were analyzed. The results showed that the mean rScO2 of APOEε4 carriers in TBI patients was significantly lower than that of APOEε4 non-carriers in the early stage of TBI. Furthermore, in TBI patients, decreased rScO2 was found in 57.89% APOEε4 carriers, but only in 18.95% APOEε4 non-carriers, indicating the rScO2 of patients with APOEε4 were more likely to decrease as compared to patients without APOEε4 in the early stage of TBI.
Meanwhile, by setting normal people as control, we found that there was no significant difference of rScO2 between APOEε4 carriers and non-carriers in the normal group [(68.75 ± 5.49)% vs. (68.37 ± 5.56)%, P = 0.851], indicating APOEε4 leads to a decrease of rScO2 in TBI patient but not in normal people. In another word, the negative effect of APOEε4 on rScO2 can be induced by TBI, which was similar to our previous results.
By monitoring the EEG of TBI patients in the NICU, we have found that APOEε4 is a risk factor for the worsening EEG activity at the acute phase (26). Additionally, we also found APOEε4 induced cerebral hypoperfusion which may directly cause impairment of cerebral oxygen metabolism in the early phase of aSAH (26). Furthermore, in the metabolic/hemodynamic model (MHM) coupling neuronal activity with EEG and hemodynamic responses, Sotero et al. (27) also confirmed that inhibitory or excitatory activity was accompanied by reductions or increase of oxygen consumption, cerebral blood flow (CBF), and blood oxygenation level-dependent (BOLD) responses, indicating EEG is very sensitive to cerebral ischemia and hypoxia. Studies on cerebral oxygen metabolism have also suggested the EEG had a positive correlation with cerebral oxygen saturation (28, 29), which was consistent with our studies.
In the laboratory researches, we found APOEε4 may affect intracellular calcium concentration, inflammatory response, excitatory amino acid release, and neuron apoptosis after mechanical injury (30, 31). Furthermore, we also found that by modulating NF-κB/MMP-9 pathway (17), APOEε4 may affect blood-brain barrier permeability which plays an important role in the brain edema and cerebral oxygen metabolism. Therefore, we speculate that APOEε4 may affect the cerebral oxygen saturation of TBI patients through the above process, and then result in worse EEG and clinical outcome eventually. Besides, some negative effects of APOEε4 will not be reflected under normal physiological conditions but will be induced under pathological conditions such as TBI, affecting the prognosis of TBI patients. However, more studies on the mechanism through which APOEε4 influences the rScO2 and outcome of TBI patients are still needed in the future.
We have to acknowledge, as a method monitoring the condition of cerebral oxygen saturation, NIRS has several limitations including, (1) the result of NIRS reflects the corresponding changes in the monitoring process, so the absolute value of rScO2 can't be obtained through NIRS; (2) the result of rScO2 monitored by NIRS may be influenced by factors including blood pressure, blood oxygen saturation, some narcotic drugs, etc.; (3) the interpretation of NIRS result may be influenced by the experience of the operators. Besides, this is a small sample and single-center clinical study, which has its limitations and requires large-scale and multiple centers for further verification. We will continue to carry out additional researches to explore the possible mechanism.
NIRS is a non-invasive and convenient method to evaluate rScO2 of TBI patients in the NICU. The rScO2 may be significantly decreased after TBI. Furthermore, APOEε4 may be a risk factor for decreased rScO2 in the early stage of TBI, which may be a possible basis to develop more precise and individualized treatments for different patients.
The data analyzed in this study is subject to the following licenses/restrictions: The datasets analyzed in this article are not publicly available. Requests to access these datasets should be directed to ZHJqaWFuZ2xpMjAxOUAxNjMuY29t.
The studies involving human participants were reviewed and approved by Ethics Committee of the First Affiliated Hospital of Chongqing Medical University. The patients/participants provided their written informed consent to participate in this study.
This study was designed and managed by YX and LJ, with data collected and processed by SX and ZW. Data were analyzed by WD and YZ. The manuscript was prepared by SX, ZW, YX, XS, QS, and LJ. All authors contributed to the article and approved the submitted version.
This work was supported by grants from the National Natural Science Foundation for Youth of China (No. 81701226).
The authors declare that the research was conducted in the absence of any commercial or financial relationships that could be construed as a potential conflict of interest.
The reviewer YW declared a shared affiliation with the authors to the handling editor at time of review.
We acknowledge YX for advice on this project, as well as LJ for assistance with data collection, processing and analysis.
1. Kowalski RG, Haarbauer-Krupa JK, Bell JM, Corrigan JD, Hammond FM, Torbey MT, et al. Acute ischemic stroke after moderate to severe traumatic brain injury. Stroke. (2017) 48:1802–9. doi: 10.1161/STROKEAHA.117.017327
2. Shao X, Hu Q, Chen S, Wang Q, Xu P, Jiang X. Ghrelin ameliorates traumatic brain injury by down-regulating bFGF and FGF-BP. Front Neurosci. (2018) 12:445. doi: 10.3389/fnins.2018.00445
3. Mohamadpour M, Whitney K, Bergold PJ. The importance of therapeutic time window in the treatment of traumatic brain injury. Front Neurosci. (2019) 13:07. doi: 10.3389/fnins.2019.00007
4. Veenith TV, Carter EL, Geeraerts T, Grossac J, Newcombe VFJ, Outtrim J, et al. Pathophysiologic mechanisms of cerebral ischemia and diffusion hypoxia in traumatic brain injury. JAMA Neurol. (2016) 73:542–50. doi: 10.1001/jamaneurol.2016.0091
5. Zhang L, Zhang L, Liu H, Jiang F, Wang H, Li D, et al. Inhibition of Epac2 attenuates neural cell apoptosis and improves neurological deficits in a rat model of traumatic brain injury. Front Neurosci. (2018) 12:263. doi: 10.3389/fnins.2018.00263
6. Robertson CS, Valadka AB, Hannay HJ, Contant CF, Gopinath SP, Cormio M, et al. Prevention of secondary ischemic insults after severe head injury. Crit Care Med. (1999) 27:2086–95. doi: 10.1097/00003246-199910000-00002
7. Maas AIR, Stocchetti N, Bullock R. Moderate and severe traumatic brain injury in adults. Lancet Neurol. (2008) 7:728–41. doi: 10.1016/S1474-4422(08)70164-9
8. Rosenthal G, Furmanov A, Itshayek E, Shoshan Y, Singh V. Assessment of a noninvasive cerebral oxygenation monitor in patients with severe traumatic brain injury. J Neurosurg. (2014) 120:901–7. doi: 10.3171/2013.12.JNS131089
9. Cooper RJ, Selb J, Gagnon L, Phillip D, Schytz HW, Iversen HK, et al. A systematic comparison of motion artifact correction techniques for functional near-infrared spectroscopy. Front Neurosci. (2012) 6:147. doi: 10.3389/fnins.2012.00147
10. Guhathakurta D, Dutta A. Computational pipeline for NIRS-EEG joint imaging of tDCS-evoked cerebral responses—an application in ischemic stroke. Front Neurosci. (2016) 10:261. doi: 10.3389/fnins.2016.00261
11. Naguib AN, Winch PD, Sebastian R, Gomez D, Guzman L, Rice J, et al. The correlation of two cerebral saturation monitors with jugular bulb oxygen saturation in children undergoing cardiopulmonary bypass for congenital heart surgery. J Intensive Care Med. (2017) 32:603–8. doi: 10.1177/0885066616663649
12. Goldman S, Sutter F, Ferdinand F, Trace C. Optimizing intraoperative cerebral oxygen delivery using noninvasive cerebral oximetry decreases the incidence of stroke for cardiac surgical patients. Heart Surgery Forum. (2004) 7:E376–81. doi: 10.1532/HSF98.20041062
13. Liaquat I, Dunn LT, Nicoll JA, Teasdale GM, Norrie JD. Effect of apolipoprotein E genotype on hematoma volume after trauma. J Neurosurg. (2002) 96:90–6. doi: 10.3171/jns.2002.96.1.0090
14. McCarron MO, Weir CJ, Muir KW, Hoffmann KL, Graffagnino C, Nicoll JA, et al. Effect of apolipoprotein E genotype on in-hospital mortality following intracerebral haemorrhage. Acta Neurol Scand. (2003) 107:106–9. doi: 10.1034/j.1600-0404.2003.01365.x
15. Millar K, Nicoll JA, Thornhill S, Murray GD, Teasdale GM. Long term neuropsychological outcome after head injury: relation to APOE genotype. J Neurol Neurosurg Psychiatr. (2003) 74:1047–52. doi: 10.1136/jnnp.74.8.1047
16. Jiang Y, Sun X, Xia Y, Tang W, Cao Y, Gu Y. Effect of APOE polymorphisms on early responses to traumatic brain injury. Neurosci Lett. (2006) 408:155–8. doi: 10.1016/j.neulet.2006.08.082
17. Teng Z, Guo Z, Zhong J, Cheng C, Huang Z, Wu Y, et al. ApoE Influences the blood-brain barrier through the NF-kappaB/MMP-9 pathway after traumatic brain injury. Sci Rep. (2017) 7:6649. doi: 10.1038/s41598-017-06932-3
18. Levin HS, Diaz-Arrastia RR. Diagnosis, prognosis, and clinical management of mild traumatic brain injury. Lancet Neurol. (2015) 14:506–17. doi: 10.1016/S1474-4422(15)00002-2
19. McCormick PW, Stewart M, Ray P, Lewis G, Dujovny M, Ausman JI. Measurement of regional cerebrovascular haemoglobin oxygen saturation in cats using optical spectroscopy. Neurol Res. (1991) 13:65–70. doi: 10.1080/01616412.1991.11739967
20. Ko SB. Multimodality monitoring in the neurointensive care unit: a special perspective for patients with stroke. J Stroke. (2013) 15:99–108. doi: 10.5853/jos.2013.15.2.99
21. Schewe JC, Thudium MO, Kappler J, Steinhagen F, Eichhorn L, Erdfelder F, et al. Monitoring of cerebral oxygen saturation during resuscitation in out-of-hospital cardiac arrest: a feasibility study in a physician staffed emergency medical system. Scand J Trauma Resusc Emerg Med. (2014) 22:58. doi: 10.1186/s13049-014-0058-y
22. Grinspan ZM, Pon S, Greenfield JP, Malhotra S, Kosofsky BE. Multimodal monitoring in the pediatric intensive care unit: new modalities and informatics challenges. Semin Pediatr Neurol. (2014) 21:291–8. doi: 10.1016/j.spen.2014.10.005
23. Brawanski A, Faltermeier R, Rothoerl RD, Woertgen C. Comparison of near-infrared spectroscopy and tissue p(O2) time series in patients after severe head injury and aneurysmal subarachnoid hemorrhage. J Cereb Blood Flow Metab. (2002) 22:605–11. doi: 10.1097/00004647-200205000-00012
24. Bhatia R, Hampton T, Malde S, Kandala NB, Muammar M, Deasy N, et al. The application of near-infrared oximetry to cerebral monitoring during aneurysm embolization: a comparison with intraprocedural angiography. J Neurosurg Anesthesiol. (2007) 19:97–104. doi: 10.1097/ANA.0b013e318031376d
25. Zweifel C, Castellani G, Czosnyka M, Helmy A, Manktelow A, Carrera E, et al. Noninvasive monitoring of cerebrovascular reactivity with near infrared spectroscopy in head-injured patients. J Neurotr. (2010) 27:1951–8. doi: 10.1089/neu.2010.1388
26. Jiang L, Yin X, Yin C, Zhou S, Dan W, Sun X. Different quantitative EEG alterations induced by TBI among patients with different APOE genotypes. Neurosci Lett. (2011) 505:160–4. doi: 10.1016/j.neulet.2011.10.011
27. Sotero RC, Trujillo-Barreto NJ. Biophysical model for integrating neuronal activity, EEG, fMRI and metabolism. Neuroimage. (2008) 39:290–309. doi: 10.1016/j.neuroimage.2007.08.001
28. Hoshi Y, Kosaka S, Xie Y, Kohri S, Tamura M. Relationship between fluctuations in the cerebral hemoglobin oxygenation state and neuronal activity under resting conditions in man. Neurosci Lett. (1998) 245:147–50. doi: 10.1016/S0304-3940(98)00197-9
29. Roche-Labarbe N, Wallois F, Ponchel E, Kongolo G, Grebe R. Coupled oxygenation oscillation measured by NIRS and intermittent cerebral activation on EEG in premature infants. Neuroimage. (2007) 36:718–27. doi: 10.1016/j.neuroimage.2007.04.002
30. Jiang L, Zhong J, Dou X, Cheng C, Huang Z, Sun X. Effects of ApoE on intracellular calcium levels and apoptosis of neurons after mechanical injury. Neuroscience. (2015) 301:375–83. doi: 10.1016/j.neuroscience.2015.06.005
Keywords: TBI, APOE, regional cerebral oxygen saturation (rScO2), cerebral oxygen saturation, near-infrared spectroscopy (NIRS)
Citation: Wu Z, Xiong S, Sun X, Shi Q, Dan W, Zhan Y, Xie Y and Jiang L (2020) Effects of Apolipoprotein E Polymorphism on Cerebral Oxygen Saturation After Traumatic Brain Injury. Front. Neurol. 11:539627. doi: 10.3389/fneur.2020.539627
Received: 02 April 2020; Accepted: 26 October 2020;
Published: 12 November 2020.
Edited by:
Gregory W. J. Hawryluk, University of Manitoba, CanadaReviewed by:
John K. Yue, University of California, San Francisco, United StatesCopyright © 2020 Wu, Xiong, Sun, Shi, Dan, Zhan, Xie and Jiang. This is an open-access article distributed under the terms of the Creative Commons Attribution License (CC BY). The use, distribution or reproduction in other forums is permitted, provided the original author(s) and the copyright owner(s) are credited and that the original publication in this journal is cited, in accordance with accepted academic practice. No use, distribution or reproduction is permitted which does not comply with these terms.
*Correspondence: Li Jiang, ZHJqaWFuZ2xpMjAxOUAxNjMuY29t; Yanfeng Xie, eHlmMzA1OEAxNjMuY29t
Disclaimer: All claims expressed in this article are solely those of the authors and do not necessarily represent those of their affiliated organizations, or those of the publisher, the editors and the reviewers. Any product that may be evaluated in this article or claim that may be made by its manufacturer is not guaranteed or endorsed by the publisher.
Research integrity at Frontiers
Learn more about the work of our research integrity team to safeguard the quality of each article we publish.