- Laboratory of Microbial Chemical Biology (LaBioQuiMi), Institute of Chemistry, State University of Campinas (UNICAMP), Campinas, Brazil
Citriculture has been one of the most important agricultural activities worldwide. Brazil is among the five major citrus producers in the world, together with China, the European Union (EU), the United States, and Mexico. Together, these five groups are responsible for about 50% of the global citrus fruit production and this industrial segment is responsible for generating direct and indirect jobs. However, several citric diseases such as citrus canker, huanglongbing, citrus variegated chlorosis, and citrus black spot have been associated with annual losses of tons of fruits and orange trees impacting the global economy for decades. Citrus canker is caused by the Gram-negative bacteria Xanthomonas citri subsp. citri. and is associated with symptoms such as the formation of chlorotic rings in the leaves, stems, and fruits. Huanglongbing (HLB) is mainly associated with the Gram-negative bacteria Candidatus Liberibacter spp. and its main symptoms are the appearance of yellowish branches and deformed small leaves with yellowish spots. Citrus variegated chlorosis (CVC) is associated with the bacterium Xylella fastidiosa and causes chlorotic spots with irregular edges on leaves and deformation of new leaves. Citrus black spot (CBS) is caused by the fungus Phyllosticta citricarpa and generates lesions on fruits and reddish-brown leaf lesions. Since citrus is important for global agriculture, the current review addresses the main features of these important diseases including their symptoms and transmission, as well as the diagnosis and control strategies that have been studied so far for application in the field.
Introduction
Citrus global production (2018) was around 158 million tons, including oranges, grapefruit, lemons, and tangerines (Food and Agriculture Organization of the United Nations, 2020). In this sense, citriculture is an important economic activity worldwide due to the adaptation of citrus varieties to all continents within the intertropical range (Passos, 1990; Caserta et al., 2020). According to data from the Food and Agriculture Organization of the United Nations, Brazil is the world’s second-largest producer of citrus fruits with an annual production of around 19 million tons and China comes first, with a production of around 43 million tons/year (Food and Agriculture Organization of the United Nations, 2020). In addition, Brazil is the world’s largest producer of sweet oranges, producing about 17 million tons of fruit annually (Food and Agriculture Organization of the United Nations, 2020), which represents more than 3/4 of the world’s orange juice exports (USDA/FAS Foreign Agricultural Service, 2021).
Given the importance of citriculture in many countries, some diseases have been representing a threat to this economic activity due to its high frequency of occurrence and damage to the production. Besides, there are several difficulties and high costs associated with citrus diseases control, such as citrus canker, citrus variegated chlorosis (CVC), Huanglongbing (HLB), and citrus black spot (CBS), which are diseases that have generated great economic impacts for the global citrus industry (Bassanezi et al., 2016; Mendonça et al., 2017).
Among these four diseases, citrus canker, HLB, and CVC are associated with the Gram-negative bacteria Xanthomonas citri, Candidatus Liberibacter spp., and Xylella fastidiosa, respectively, while the fungus Phyllosticta citricarpa is the causal agent of CBS (Figure 1). These diseases are responsible for great economic losses in the global citriculture, especially in Brazil, due to the severity of the symptoms, the drop in the production, susceptibility of commercial varieties to these diseases (Gottwald et al., 2001; Brunings and Gabriel, 2003; Teixeira et al., 2010; Li and Wang, 2014; Wetterich et al., 2017), and due to the precarious and inefficient control methods, in many cases.
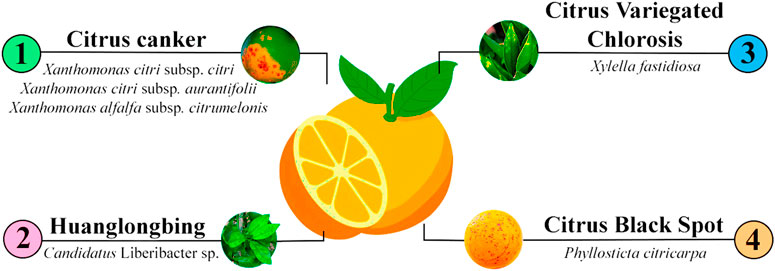
FIGURE 1. The four main citrus diseases addressed in this review, their respective pathogens. (1) Citrus canker is associated with three bacteria species of the genus Xanthomonas: X. citri subsp. citri; X. citri subsp. aurantifolii, and X. alfalfa subsp. citrumelonis. (2) HLB is mainly caused by the bacterium Candidatus Liberibacter sp. (3) CVC is caused by the Gram-negative bacterium Xylella fastidiosa. (4) CBS is caused by the heterothallic fungal pathogen Phyllosticta citricarpa.
Based on the above-mentioned concerns, this review describes the main diseases related to the worldwide citriculture: citrus canker, HLB, CVC, and CBS. Thus, here we present a historical overview and the geographic distribution of these diseases, the general disease characteristics such as the causal agent, transmission mode, and symptoms, besides addressing the disease control strategies, diagnosis and state of the art studies on alternative methods for their management.
Citrus canker
The citrus canker origin is not completely known, but some reports indicate that the disease originates from tropical areas in Asia, such as southern China, Indonesia, and India (Das, 2003).
Subsequently, this disease spread over several continents including Africa, North America, South America, and Oceania (Stall & Seymour, 1983; Jones et al., 1984; Feichtenberger et al., 1997; Braithwaite et al., 2002) (Table 1). Brazil was the first country in South America to detect a Xcc infection, then the disease was also observed in Paraguay (1967) and Argentina (1972) (Danós et al., 1984). The disease was first reported in Brazil in 1957 in São Paulo state and currently, citrus canker still affects several Brazilian states (Behlau, 2021a). Although citrus canker was officially declared eradicated in Florida (US), the disease reappeared in the region in 1997 and currently the state is a region that needs management strategies for citrus canker control (Gochez et al., 2020). The citrus canker eradication strategy was hampered due to factors such as Florida’s climate, the citriculture conditions, and the region’s economy. Through screening and surveillance actions, in 2019, citrus canker was declared eradicated from all Australian territories except the Northern Territory of Australia, where actions to eradicate the pathogen (X. citri subsp. citri) continue (IPPC, 2018).
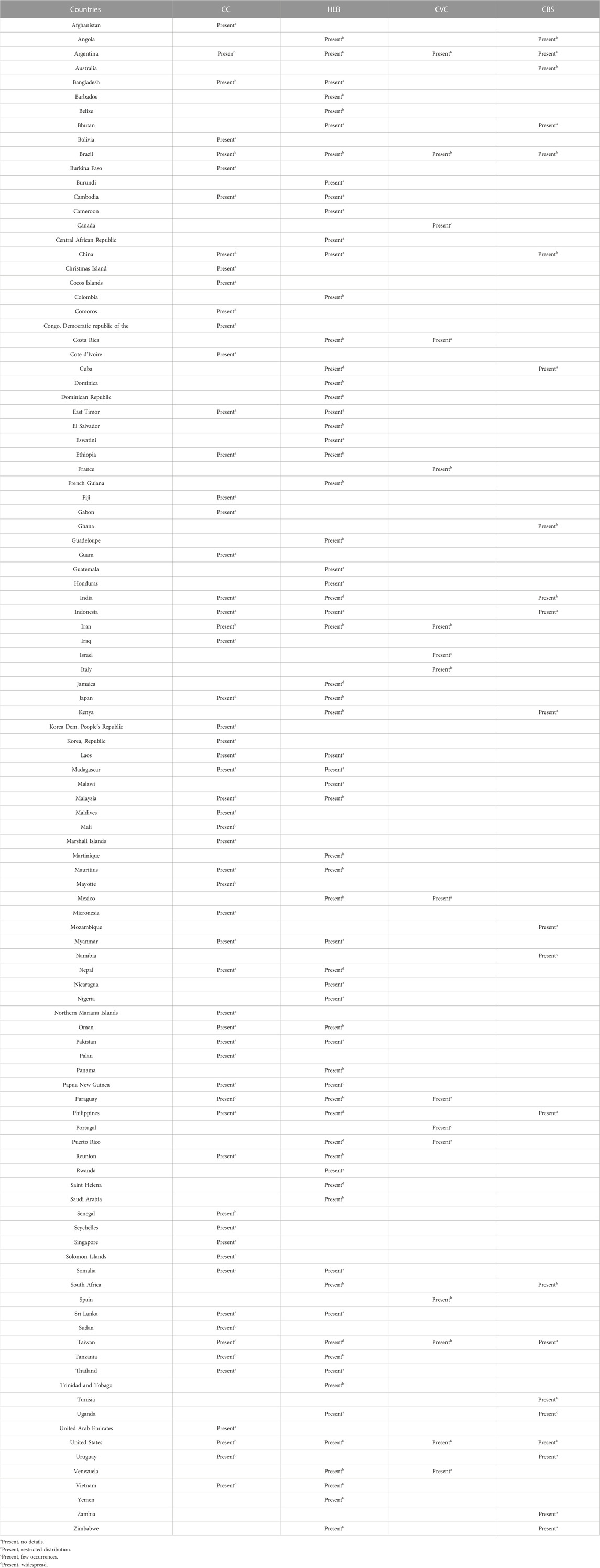
TABLE 1. Countries where there are reports of the presence of Citrus Canker (CC), Huanglongbing (HLB), Citrus Variegated Chlorosis (CVC), and Citrus Black Spot (CBS). Data: European and Mediterranean Plant Protection Organization, 2020.
Citrus canker is associated with three bacteria species of the genus Xanthomonas: X. citri subsp. citri (Xcc); X. citri subsp. aurantifolii pathotypes B and C (XauB and XauC, respectively); and X. alfalfa subsp. citrumelonis (Xacm) (Fonseca et al., 2019). Xcc, XauB, and XauC are the causative agents of citrus canker A, B, and C, respectively, and Xacm causes citrus bacterial spot disease, which induces similar symptoms to citrus canker (Fonseca et al., 2019). Citrus canker A is the most aggressive and, therefore, the main concern in Asia and South America. On the other hand, citrus canker B is less aggressive, occurring in Argentina, Paraguay, and Uruguay. Citrus canker C has only been reported in São Paulo state in Brazil since 2009 (Fonseca et al., 2019; Bahadur & Srivastava, 2022). The citrus canker management in Florida, one of the main citriculture region in the world, causes an increase of 3.84% in the total costs of its production (Gochez et al., 2020). The main characteristics of the citrus canker disease are summarized in Figure 2, such as the region of the first report and methods of detection, control, and spread of the disease.
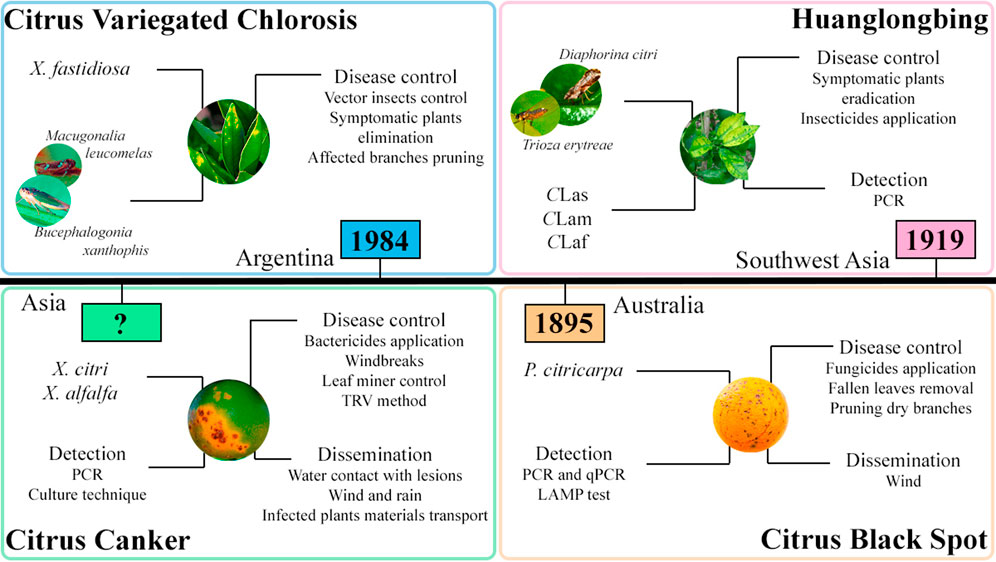
FIGURE 2. Summary of information on citrus canker (CC), citrus variegated chlorosis (CVC), huanglongbing (HLB), and citrus black spot (CBS) diseases addressed in this review. The figure shows the year and location of the first report, the detection, control, and spread methods, and their respective causal agents.
The biofilm formation by the bacterium Xcc is a crucial virulence factor, as it plays an important role in the infection initial stages and host colonization (Li & Wang, 2014; Pontes et al., 2020a). Xcc mutant strains that present impaired biofilm formation exhibit reduced growth in the plant and reduced ability to induce symptoms. The study indicated that d-leucine and 3-indolylacetonitrile (IAN) inhibited the production of the bacterium Xcc biofilm, even at a low concentration. The same study observed that D-leucine and IAN increased the pathogen’s sensitivity to copper bactericides, reducing the bacterial population growth and decreasing the disease symptoms development (Li & Wang, 2014).
The pathogen X. citri is disseminated through the contact of water droplets from rain and irrigation with lesions on branches, leaves, or fruits, thus, dispersing the inoculum in other citrus areas (Gottwald et al., 1988; Gottwald and Timmer, 1995; Yan and Wang, 2012). This mechanism is the main pathogen dissemination within a short distance (Li and Wang, 2014). The wind’s presence associated with rain is also a factor for the bacteria spread over larger areas (Gottwald et al., 1988; Gottwald and Timmer, 1995). Thus, pathogen dispersion is favoured by high temperatures and precipitation with the presence of constant wind (Koizumi et al., 1996).
Citrus canker symptoms are usually observed throughout the plant aerial part, such as branches, leaves, and fruits (Stall and Seymour, 1983). The typical symptoms are the formation of necrotic lesions with oily, water-soaked margins, and generating chlorotic rings in the leaves, stems, and fruits (Brunings and Gabriel, 2003; Li and Wang, 2014). An important disease symptom is citric tissue hyperplasia, that is excessive mitotic cell division that results in cankers (Gabriel et al., 2000; Das, 2003). Furthermore, severe infection by citrus canker can cause defoliation, deformation, and premature fruit drop (Das, 2003).
Despite the citrus canker economic importance, no efficient and environmental-friendly treatments have been developed so far. Consequently, copper spraying (Graham et al., 2010) the use of less susceptible species (Leite Jr and Mohan, 1990; Behlau et al., 2008), windbreaks (Gottwald et al., 2002; Behlau et al., 2008), systemic resistance inducers (Behlau et al., 2017), and leaf miners (Phyllocnistis citrella) control (Stein et al., 2007) are measures adopted for citrus canker management currently. Although they do not prevent the bacteria entry or elimination, these measures can significantly reduce the citrus canker incidence (Behlau et al., 2017). In addition, copper spraying prevents new infections due to the film formation on the leaves surface, reducing the Xcc population in the citrus leaves. However, in order to achieve an efficient control of the pathogen, this strategy requires multiple applications of copper-based bactericides in the field, increasing the negative effects for the environment and citrus plants (Francis et al., 2009; Behlau et al., 2017; Islam et al., 2019; Ferreira et al., 2022).
An alternative to the resistant Xcc populations’ issue would be the development of strategies able to reduce resistance to copper bactericides, increasing their effectiveness and decreasing the need for constant application (Pernezny et al., 2008; Li and Wang, 2014). In this sense, the tree row volume (TRV) methodology has indicated a reduction in the use of copper-based bactericides. First proposed by Byers et al. (1971), TRV aims to optimize the spray volume and the copper rate based on the estimated canopy volume in a given area (Behlau et al., 2021b). In previous studies by Scapin et al. (2015), the TRV method used in the citrus canker control showed a reduction in the water volume of 73% and a reduction in the copper rate by 50% compared to a standard rate. In a more recent study on the TRV method efficiency, Behlau et al. (2021b) demonstrated that it is possible to control citrus canker with the combination of 40 mL water volume and a copper rate of 36.8 mg/m3.
The use of other compounds’ application can also be effective in the citrus canker control, such as the combined use of copper oxychloride, streptocycline, and neem (Azadirachta indica) suspension (Das and Singh, 2000; Islam et al., 2019). Streptocycline has been used since 1955 in several treatments related to the Citrus genus species diseases (McManus et al., 2002; Islam et al., 2019), but the prolonged and excessive use of this antibiotic has caused the emergence of bacteria-resistant populations (Carter et al., 2000; Hyun et al., 2012; Islam et al., 2019). As mentioned previously, the use of resistant Xcc citrus varieties is an efficient method to control the pathogen, however, the development of these varieties is a costly and time-consuming process (Islam et al., 2019).
An alternative and ecological control method for citrus canker would be the use of endophytic bacteria as biological control. These endophytes are endosymbiotic microorganisms, which colonize the same niches of phytopathogens and may act as biocontrol agents (Islam et al., 2019). The endophytic bacteria used as biocontrol agents can increase resistance to diseases and, therefore, can be considered as a potential alternative to the use of chemical control strategies (Islam et al., 2019).
Islam et al. (2019) evaluated 134 endophytic bacteria species obtained from several plant species that were isolated from different angiosperm and gymnosperm tissues. Among these, 18 endophytic bacteria species showed antibacterial activity. Bacillus thuringiensis strains (TbL-22 and TbL-26) showed an expressive Xcc growth inhibition (Islam et al., 2019), which indicates a potential biocontrol alternative.
Another possibility to be potentially applied would be the Systemic Acquired Resistance (SAR) induction as an alternative to phytopathogens control (Walters et al., 2013). SAR is an induced defense mechanism against a broad spectrum of pathogens. This mechanism requires salicylic acid as a signal molecule and it is associated with the accumulation of proteins related to pathogenicity, consequently contributing to the plant’s resistance to the microorganisms’ attack (Francis et al., 2009). Other molecules (analogous to the salicylic acid, e.g. isonicotinic acid (INA) and acilbenzolar-S-methyl (ASM)), can also induce SAR and be used to control pathogens (Francis et al., 2009).
Francis et al. (2009) evaluated the response of Swingle citrumelo (C. paradisi x Poncirus trifoliata) to the soil application of imidacloprid, INA, and ASM compared to foliar application of ASM. The study evaluated the ability to reduce the number of lesions and the bacterial population after some weeks after application. The use of SAR inducers in the soil reduced the number of lesions and changed their phenotype: the lesions became smaller, darker, and less eruptive than on the untreated plants. This was reflected in the reduction of the Xcc population. The symptoms and results presented by the plants treated with the inducers were similar to the symptoms observed in resistant cultivars. Therefore, these results suggest that inducing SAR response in citrus species that are more susceptible to citrus canker is potentially interesting for Xcc control.
Another study concerning the development of new control methods for Citrus Canker disease has been performed through application of penicillic acid in citrus leaves. Vieira et al. (2022), observed a significant reduction of disease lesions, when applied the penicillic acid produced by Penicillium sp. The application of 25 μg mL−1 penicillic acid reduced 0.437 lesions/cm2, while 700 μg mL−1 metallic copper, used as control, reduced just 0.270 lesions/cm2. Therefore, penicillic acid is a potential agent to be used as control method of Citrus Canker disease. However, future studies are necessary to evaluate its environmental impact (Vieira et al., 2022), once a few studies describe its carcinogenic effect in rats (Dickens and Jones, 1961; Dickens 1962; 1967).
Currently, the detection of citrus canker depends on the bacteria culture (Abdulridha et al., 2019) and PCR techniques (Naqvi et al., 2022). The diagnosis can also be carried out through the observation of lesions on the fruit peels (Nasreen et al., 2020). Although PCR detection presents fast results, it also leads to possible false-negative results due to the presence of PCR inhibitors in the samples. While detection by culture techniques allows a more reliable result, the experiments are time-consuming (Abdulridha et al., 2019). Therefore, there is a need to develop an accurate and early detection method for the citrus canker diagnosis in the field.
Abdulridha et al. (2019) conducted a study with the remote sensing technique for citrus canker detection both in laboratory conditions and in orchards using an unmanned aerial vehicle. The hyperspectral imaging system achieved high overall accuracy for detecting canker citrus in infected leaves at asymptomatic developmental stages. Haji-Hashemi et al. (2018) studied the use of an electrochemical immunosensor to identify the effector protein PthA, to diagnose citrus canker. The immunosensor showed high selectivity, stability, good repeatability, and great potential for application in real samples.
Huanglongbing (HLB)
The disease popularly called greening was first identified in 1919 in southwest Asia. Subsequently, HLB spread to other continents, such as Africa and South America, being reported for the first time in these continents in the years 1928 and 2004, respectively (Gabriel et al., 2020).
In Brazil, the first HLB report occurred in São Paulo state in 2004. Then, the disease spread to Minas Gerais, in 2005, and Paraná, in 2006 (Coletta-Filho et al., 2004; Teixeira et al., 2005). During 2005–2019, 55.5 million trees were eliminated in Sao Paulo—Brazil due to HLB symptoms (Bassanezi et al., 2020). In Africa, HLB was first observed in 1928 in South Africa, under different names: yellow shoot and greening. However, only in 1937, there was the HLB first description, as it was still considered a mineral problem presented by the plant. In Florida, the disease was first detected in 2005, and later, it was detected in Texas and California states (Bové, 2006). HLB quickly spread across the Florida state. In 2015, it was estimated that 90% of citrus groves in the state were impacted by the disease (Singerman et al., 2018; Alquézar et al., 2022).
Some important information about HLB, such as the causal agents, main symptoms, detection protocols, control strategies, and dissemination forms are detailed in Figure 2. Currently, about 40 countries have trees affected by HLB, including countries in Asia, Africa, Oceania, and America (Dala-Paula et al., 2019; European and Mediterranean Plant Protection Organization, 2020) (Table 1).
HLB is caused by three Gram-negative bacteria species that colonize the plant phloem, which were named according to the continent on which they were first detected: Candidatus Liberibacter asiaticus (CLas), Candidatus L. americanus (CLam) e Candidatus L. africanus (CLaf) (Jagoueix and Bove, 1994; Garnier et al., 2000; Teixeira et al., 2005).
Two other Liberibacter species were identified between the years 2008 and 2011. Candidatus L. solanacearum was identified in 2008 in tomato and potato plants and was related to yellow psyllid and zebra chip diseases in New Zealand and American solanaceous plants (Hansen et al., 2008; Abad et al., 2009; Liefting et al., 2009; Secor et al., 2009). Raddadi et al. (2011) characterized a new Liberibacter species, which they called Candidatus L. europaeus. However, through this study, Raddadi et al. (2011) found that Ca. L. europaeus was not responsible for developing any specific symptoms in the infected plants, so they described Ca. L. europaeus as an endophytic bacterium rather than a pathogenic bacterium.
Posteriorly, in 2012, the non-pathogenic species of the genus Candidatus Liberibacter named Liberibacter crescens was isolated for the first time from the sap of Babaco papaya (Fagen et al., 2014). Furthermore, it had its genome completely sequenced (Leonard et al., 2012). L. crescens was isolated only once and it has served as a model organism for the study of other Candidatus Liberibacter spp. due to the high similarity of Average Nucleotide Identity (ANI) shown and overlaps related to functions encoded in its genome (Fagen et al., 2014; Sena-Vélez et al., 2019; Blacutt et al., 2020).
The Huanglongbing disease can be mainly disseminated through insect vectors by the inoculation of Candidatus Liberibacter spp. in the plant or during grafting processes (Batool et al., 2007). In the 1960s in South Africa and India, it was shown that two psyllid species, Diaphorina citri and Trioza erytreae are the vectors of HLB (Bové, 2006; Gabriel et al., 2020). The species D. citri commonly transmits CLas and CLam, while T. erytreae transmits CLaf (Wang, 2020), although this species can also transmit CLas (Cocuzza et al., 2017). The pathogen can be acquired through feeding by both psyllids in the nymph and adult phase, however Asian citrus psyllid adults inoculate huanglongbing bacterium more efficiently (Ammar et al., 2020). While the African species (T. erytreae) can spread the pathogen within 4 days after acquiring it (Cocuzza et al., 2017), the Asian species (D. citri) has a longer latency time, between 16 and 18 days (Canale et al., 2017). Once infected with the bacteria, both psyllid species remain infective for the life of the insect (Moll and Martin, 1973; Hung et al., 2004; Cocuzza et al., 2017). Both species prefer colder climates, however, the African species prefer more humid climates than the Asian species (Yang et al., 2006; Cocuzza et al., 2017).
After infection of the plant, the disease has a long latency period before the onset of symptoms (which can last from months to years). CLas suppresses the infection immune responses of its citrus host, which may explain the HLB long asymptomatic phase (Gabriel et al., 2020). In the asymptomatic stage of HLB, the infected plant by the Candidatus Liberibacter spp. becomes an inoculum source of the disease, spreading it through all orchards (Belasque Junior et al., 2009).
Among the major characteristic symptoms of HLB disease are the yellowish branches appearance, deformed small leaves with yellowish spots, fruit deformation, seed abortion, premature leaf, fruit drop, and sprouts death. The symptomatic leaves’ appearance is similar to those caused by zinc, manganese, and iron deficiency (Graça, 1991; Halbert and Majunath, 2004; Bové, 2006; Wetterich et al., 2017). Another symptom is fibrous root mass loss (Achor et al., 2020).
Due to the fact that CLas bacterium is found in the phloem of the host, only few virulence factors are known when compared to other phytopathogenic bacteria (Wang et al., 2017; Deng et al., 2019). The main known virulence factors are the secretion system (type I secretion systems—T1SSs and a general secretory pathway—Sec), putative effectors, lipopolysaccharides (LPS), and characteristics that can contribute to the disease development, such as flagella and the production of the enzyme salicylic acid hydroxylase that degrades salicylic acid (SA) (Wang et al., 2017; Wang, 2019; Pontes et al., 2020a). Also, there is a callose accumulation, which starts in the infection’s early stages and leads to more advanced stages of the disease. The callose accumulation during infection impairs the photoassimilates export in the leaf (Achor et al., 2020). The photosynthetic product transport inhibition leads to starch accumulation. This, on the other hand, leads to the appearance of mottled leaves due to the thylakoid chloroplast structure disruption (Deng et al., 2019). Such factors suggest that the symptoms observed in the leaves and fruits of HLB diseased citrus are correlated with phloem obstruction, affecting the photoassimilates transport (Achor et al., 2020). Photoassimilates are the products resulting from the photosynthesis process and, therefore, are used as an energy source by plants (Thomas, 2017).
The similarity of HLB symptoms with those of other citrus diseases, the knowledge lack about the mechanisms of infection, the irregular distribution of the pathogen in the host, and the inability to grow in vitro this bacterium, are reasons that make HLB a great challenge for scientists (Bové & Garnier, 2003; Bové, 2006; Wang, 2019; Tran et al., 2020).
The HLB detection is performed through the Polymerase Chain Reaction (PCR) technique (Innis et al., 1990). However, such method has limitations due to the CLas irregular distribution, which can generate false-negative results; in addition the technique presents a high cost and a time-consuming sample preparation, which makes its large-scale use in the citrus fields a difficult task to the producers (Li et al., 2009; Bassanezi et al., 2010; Wetterich et al., 2017; Tran et al., 2020).
In this sense, many researchers have developed studies to present an alternative for HLB detection in the field. Sanchez et al. (2019) studied Raman spectroscopy application in detecting HLB in citrus, comparing samples infected with CLas, healthy samples, and samples with nutritional deficiencies, verifying that this technique is highly sensitive and promising for diagnosing HLB, once it is based on the detection of molecules secreted by the infected cells in the initial infection stages, even when the CLas levels are still below the detection limits by PCR. Tran et al. (2020) studied the HLB detection based on the biomarkers secreted by CLas, such as sec-delivered effector 1 (SDE1), which is a unique biomarker of this pathogen and is a gene highly expressed in the infected citrus tissue in the disease early stages. These proteins are systematically distributed throughout the tree, which reduces false negatives and the sample number to be collected in each tree (Tran et al., 2020).
Recently, Pontes et al. (2020b) detected several metabolites in Citrus sinensis infected leaf tissues using desorption electrospray ionization coupled to mass spectrometer imaging (DESI-MSI). The putatively identified compounds were organic acids, phytohormones, sugars, amino acids, among others, which are metabolites related to the plant defense system. Furthermore, an increase in these metabolites concentration in both asymptomatic and symptomatic leaves was verified. In this study, DESI-MSI was used for the first time for HLB monitoring and proved to be a potential technique for the use in HLB disease diagnosis (Pontes et al., 2020b).
The main HLB management is carried out through psyllid control using insecticide application associated with a visual inspection of the symptomatic plants. The infected trees are then eradicated from the orchards (Belasque Junior et al., 2010a; Dorta et al., 2019; Alquézar et al., 2022). Vector control is fundamental for disease management, contributing both to the reduction of psyllid vector population and to avoid bacteria acquisition and inoculation in healthy plants (Belasque Junior et al., 2010b). However, the continuous application of neonicotinoid insecticides such as imidacloprid, acetamiprid, nitenpyram, clothianidin, thiamethoxam, and cyantraniliprole, which are often applied excessively, leads to increased production costs, resistant individual’s emergence, environmental contamination and may cause an environmental imbalance between pests and their natural enemies, causing outbreaks and pest resurgence (Naem et al., 2016; Dorta et al., 2019; Li et al., 2019; Uthman et al., 2022). The psyllid monitoring is carried out through shoots visual inspections, adhesive traps, stem-tap sampling, which is a common method in the United States; suction traps, sweep net samples, and vacuum samples per unit of time (Hall et al., 2012). To avoid the planting of already infected plants, healthy plant production must be strictly controlled using a restricted environment, which avoids vector access to plants, and with the controlled origin of the material, which prevents the introduction of bacteria in seedlings (Iwanami et al., 2013). Inspections for detecting symptomatic plants should be carried out frequently so that new symptomatic plants and symptomatic plants that have not been identified in previous inspections are detected. Symptomatic plants identified through visual inspection should be removed to reduce the inoculum sources (Belasque Junior et al., 2010a; Alquézar et al., 2022). In Brazil and the United States, a three-pronged system was implemented to prevent the HLB spread, which consists of planting healthy plants, eradicating infected trees, and applying insecticides (Alquézar et al., 2022).
In Brazil and China, citrus relocation is carried out in HLB-free areas (Zhang et al., 2019). However, these measures are not sufficiently effective due to the disease’s rapid progress, which causes productivity and fruit quality losses and, consequently, makes the affected orchard economically unfeasible within 7–10 years (Bassanezi et al., 2009). Over time, symptoms become more severe, with branches dying off. The orchards decline with HLB after years is evidenced by the presence of skeletonized trees (Gabriel et al., 2020). Therefore, other strategies have been studied, such as the use of chemotherapy, thermotherapy, antibiotics application, use of the disease vector natural enemies, and use of defense activators injection in the plant trunk (Dorta et al., 2019; Li et al., 2019; Zhang et al., 2019). However, thermotherapy only keeps the bacteria in low concentrations and, therefore, citrus remains an HLB disease inoculum source (Dorta et al., 2019; Zhang et al., 2019).
The antibiotics application, both in the form of leaf spray and injections in the affected tree’s trunk, presented efficiency in reducing the pathogen (Dorta et al., 2019). Recent studies have indicated that the application of oxytetracycline and streptomycin are inefficient to mitigate HLB disease (Li et al., 2019; Blacutt et al., 2020; Vincent et al., 2022), while other sulfonamide antibiotics such as sodium sulfadimethoxine (SDX) and sulfathiazole (STZ), are effective against CLas (Yang et al., 2020). However, the application of antibiotics in the field generates an increase in the production cost, and causes negative effects to human health and for the environment. Widespread application of insecticides can cause pest outbreaks, pest resurgence, the insecticide-resistant populations emergence, and can contaminate soil and water (Dorta et al., 2019; Li et al., 2019).
Citrus variegated chlorosis (CVC)
The first observation of trees with CVC symptoms occurred in Argentina, in 1984, and later, the disease was identified in the northern São Paulo state, Brazil, in 1987 (Rossetti & Negri, 2011; Gabriel et al., 2020). The CVC incidence already affected 200 million orange trees in the Sao Paulo State in 2005, but its incidence has declined significantly in 2019 (1.71%) (Coletta-Filho et al., 2020). However, new genotypes of X. fastidiosa have been reported in the Americas and Europe, as reported by Safady et al. (2019). CVC has also been detected in Paraguay and Costa Rica (Li et al., 2013) and 13 other countries around the world (Table 1).
In 2013, Xylella fastidiosa subspecies were found in ornamental coffee trees, in olive trees in Italy, and in ornamental olive trees in France, in 2019 (Giampetruzzi et al., 2017; Godefroid et al., 2019; Ministère de L’Agriculture et de L’Alimentation, 2019). In 2017, X. fastidiosa subspecies were detected in almond, grapevines, cherry, and plum trees. Researchers also detected X. fastidiosa subspecies in wild olive trees, lavender, oleander, and mimosa plants in the Islas Baleares and Ibiza, Spain (Govern Illes Balears, 2017; Godefroid et al., 2019). Although CVC-associated bacteria subspecies have been detected in European countries, the occurrence of disease in citrus has not been reported; however, X. fastidiosa have been identified in olive trees (Rapicavoli et al., 2018; Almeida et al., 2019; Santos et al., 2022).
Some important information about CVC, such as causal agent, main symptoms, control strategies, and dissemination forms, are indicated in Figure 2.
X. fastidiosa is a Gram-negative bacterium that colonizes the xylem vessels of economically important plant species, thus compromising plant development by blocking water and nutrients (Newman et al., 2003). This species has different pathotypes and, therefore, five subspecies have already been found: X. fastidiosa subsp. fastidiosa (Xff), X. fastidiosa subsp. pauca (Xfp), X. fastidiosa subsp. sandyi (Xfs), X. fastidiosa subsp. morus, and X. fastidiosa subsp. multiplex (Xfm) (Schaad et al., 2004; Schuenzel et al., 2005; Nunney et al., 2014; Gabriel et al., 2020).
Thereby, X. fastidiosa is associated with economic important diseases, such as Pierce’s disease in grapevines, citrus variegated chlorosis (CVC), coffee leaf scorch (CLS), plum leaf scorch, almond leaf scorch, oak leaf scorch, alfalfa dwarf, periwinkle wilt, and the rapid decline syndrome in olive trees (Purcell, 1997; Li et al., 2001; Gabriel et al., 2020). Xfp causes diseases in citrus (CVC) and coffee (coffee leaf scorch) in South and Central America (Godefroid et al., 2019). Despite the ability of X. fastidiosa to colonize a wide range of hosts (more than 500 plant species), the disease, however, has been found limited to Sweet orange (C. sinensis) (Gabriel et al., 2020; Landa et al., 2022), tangerines and their hybrids (He et al., 2000). Therefore, there is a limitation of host species that can be infected by Xylella sp., which is determined by the genome of the phytopathogen (Gabriel et al., 2020; Landa et al., 2022).
The X. fastidiosa transmission can occur through natural root grafts (He et al., 2000), but it can also be transmitted by sharpshooters (Gabriel et al., 2020). CVC vectors are insects that feed on citrus xylem vessels (Almeida et al., 2005), such as the leafhoppers of the Cicadellidae family, which include Bucephalogonia xanthophis and Macugonalia leucomelas that are more efficient at transmitting the pathogen. However, the transmission can also occur through spittlebugs (Aphrophoridae) (Lopes & Krugner, 2016; Marcus et al., 2022).
The genome of X. fastidiosa subsp. pauca was sequenced in 2000, allowing access to information about its pathogenic mechanisms (Simpson et al., 2000; Lindow, 2019). One described pathogenicity mechanism is attributed to the xylem occlusion by the bacteria aggregation and consequent biofilm formation, resulting in symptoms of water stress (Souza et al., 2004; Lorite et al., 2011).
As the xylem colonization by Xfp causes the sap flow to be obstructed, the infected trees present the symptoms initially in the leaves and, later, in the fruits (Gabriel et al., 2020). CVC symptoms include the appearance of yellow spots on the leave’s upper surface with the appearance of dark lesions on the underside. These lesions may coalesce and become necrotic. Young leaves are reduced in size and have a thin, twisted shape. Affected fruits have their development compromised, remaining small, hard and useless for commercialization (Feichtenberger et al., 1997). The branches usually show symptoms like zinc deficiency (Gabriel et al., 2020). Its fruits are hardened and have a reduced size and change in acidity, despite their high sugar content. Thus, fresh fruit consumption and the use of these fruits in juices are impaired (Gabriel et al., 2020). The fruits undergo early ripening and may be susceptible to sunburn (Feichtenberger et al., 1997; Gabriel et al., 2020). The fruits with a small size generate economic losses since a larger number of fruits is needed to reach the weight of a typical commercial box (Gabriel et al., 2020).
The CVC symptoms are in the great majority related to the blockage of the xylem vessels by the biofilm produced by X. fastidiosa. The main consequence of the impediment is the generation of water stress and a low supply of plant nutrients (Coletta-Filho et al., 2007; Mauricio et al., 2019). The disease progression is faster in younger plants that are exposed to seasonal water deficits, which can result in a fruit production decrease by about 75% when compared to the production of healthy or asymptomatic plants (Gabriel et al., 2020).
The CVC disease management is based on vector control through the use of insecticides, the symptomatic plants’ elimination, and the affected branches pruning (Gravena et al., 1997; Coletta-Filho et al., 2020; Lopes, 2020). However, branch pruning is not an efficient measure, especially in orchards with a high CVC incidence (Gabriel et al., 2020; Lopes, 2020).
Lopes (2020) studied the effectiveness of a new strategy to control CVC named “Scion Substitution.” The study compared the new methodology with the conventional pruning procedure for CVC infected branches. Using the traditional strategy, branches and trunks are removed. However, when they remove branches and trunks, the potential infection incidence, or even death, was 20%–30% and 10%–30%, respectively. Alternatively, applying the “Scion Substitution” strategy through the removal of the entire graft of lemon rootstocks (Citrus limonia Osbeck and Citrus reshni Hort. ex Tan.) with healthy shoots, the new shoots grow with no CVC infection (Lopes, 2020). Based on this method, plants highly affected by this disease do not need to be completely removed, which would reduce the infected plants’ eradication costs (Gabriel et al., 2020; Lopes, 2020).
Another strategy to mitigate CVC disease would be the use of resistant plants, however all commercially grown sweet orange varieties are susceptible to the disease. The citrus species that show resistance to Xfp are some mandarin, lemon, grapefruit, pummelo, and tangor genotypes, which do not show symptoms, although it is possible to detect the bacteria present in the xylem vessels (Mauricio et al., 2019).
Mauricio et al. (2019) studied the susceptibility of 264 Murcott tangor (C. reticulata Blanco x C. sinensis (L.) Osbeck) and Pera sweet orange (C. sinensis (L.) Osbeck) hybrids for 6 years. This study indicated that plants with high isoflavone reductase expression presented a great resistance to Xfp infection. Mauricio et al. (2019) evaluated the expression of 12 defense-related genes comparing resistant and susceptible varieties to CVC disease. Due to significantly higher expression in resistant varieties, the results suggest that some of these genes are involved in resistance against CVC. Resistance-related genes include disease resistance protein genes (RGA2 and DRP), protein kinase activity (LRR-RK and FLS2), a nucleotide binding protein (IFR2), and a transcription factor (b-Zip). This result indicates that the hybrids’ resistance against Xfp is not related to their anatomic system, but rather the defense mechanism presented by these citrus hybrids (Mauricio et al., 2019).
Recently, Pereira et al. (2020) found that the CrRAP2.2 gene found in C. reticulata is related to resistance to X. fastidiosa infection in this citrus variety. The gene is homologous to the Arabidopsis thaliana (AtRAP2.2) species, related to the transcriptional factor RAP2.2. This gene is associated with resistance to the pathogen Botrytis cinerea in A. thaliana (Zhao et al., 2012; Pereira et al., 2020). In this study, the CrRAP2.2 gene expression was responsible for inducing the CVC resistance in C. sinensis, which also reduces the disease symptoms (Pereira et al., 2020).
Diagnostic methods development for CVC during its asymptomatic phase is extremely important since it allows the citrus removal before becoming an inoculum source for the bacteria. In this sense, Soares et al. (2020) applied Liquid Chromatography coupled to Atmospheric Pressure Chemical Ionization-Mass Spectrometry-Selected Reaction Monitoring (LC/APCI-MS-SRM) method to detect CVC before the disease symptoms appeared in the affected trees. Then, in this study, the authors analysed samples of C. sinensis grafted on C. limonia, detecting high flavonoid concentrations in the leaves and coumarins in the roots, in symptomatic plants compared to asymptomatic plants and healthy plants. With these results, Soares et al. (2020) concluded that the LC/APCI-MS-SRM method could be applied for the detection of CVC before plants become symptomatic, being a simple and accurate detection method.
Citrus black spot (CBS)
CBS has been first seen in Australia nearly 120 years ago (Kotzé, 1981), and it is now distributed in Africa, Asia, Australia, the Americas (Serra et al., 2022) (Table 1). The CBS disease was responsible for ∼40% of production losses in South Africa, Brazil, Australia, and the USA (Savi et al., 2019; Tranet al., 2019; Franco et al., 2020). Figure 2 indicates some important information about CBS, such as causal agent, main symptoms, control strategies, and dissemination forms.
CBS is a citrus disease caused by the heterothallic fungal pathogen P. citricarpa (teleomorph Guignardia citricarpa). This pathogen is classified as a quarantine fungus in the United States, the European Union (Schirmacher et al., 2019), and citrus-producing areas that do not present the disease (Serra et al., 2022). The existence of two different, but morphologically identical, strains of P. citricarpa led to confusion in initial studies of this pathogen. One of the strains is the CBS causative agent, while the other is an endophytic microorganism (Kotzé, 1981). Differentiating two morphologically identical citrus pathogens, P. citricarpa and P. citriasiana, is also difficult, as P. citriasiana is a non-quarantine pathogen that causes CBS-like symptoms (Schirmacher et al., 2019).
P. citricarpa can infect through two inoculum forms: ascospores (a sexual propagule) and conidia (an asexual propagule), which are produced throughout the rainy season (Frare et al., 2019). Although both types are important to initiate an epidemic, the ascospores play an important role in introducing CBS to citrus orchards. Ascospores are formed in the dead leaves on the floor and disseminated by the wind. The conidia are formed in twigs, leaf litter, and, sometimes, in fruit lesions. They are dispersed over a short distance to receptive tissues, infecting nearby fruits and leaves and increasing the infection intensity in the tree. After infection, the pathogen initiates the infection and remains quiescent as subcuticular mycelium, maintaining the disease in a latency period. The infection peg invades the cuticle, enlarging into a mycelium small mass, settling between the cuticle and the epidermis wall. This infection’s longest period could also be explained by the slow fruit tissue colonization by the pathogen. In vitro studies show that P. citricarpa takes a long time to grow on artificial media, under controlled conditions (Kotzé, 1981; Frare et al., 2019).
In addition to the inoculum production increasing during rainy seasons, the CBS symptoms are more drastic on older trees than young ones, suggesting that age and stress could be linked to the disease advance (Kotzé, 1981; Frare et al., 2019). Some citrus species affected by the disease are lemons (Citrus limon), some limes (C. aurantiifolia and C. limettioides), mandarins/clementines (C. deliciosa, C. reticulata, and C. clementina), and sweet oranges (C. sinensis) (Frare et al., 2019).
The disease causes lesions on fruits and reddish-brown leaf lesions, but the quality inside the fruit is not altered. Nevertheless, fruits with lesions are not considered suitable for exporting. For that reason, the economic loss caused by the fungus is more associated with the human economic practice (Kotzé, 1981; Schubert et al., 2012).
The CBS symptoms can be classified as hard spot lesions, that normally appear when the fruit starts developing, with a yellow halo circling the lesion in green fruits and a green halo circling a dark brown lesion in mature fruits; freckle spot, that occurs when the fruit develops to an orange colour and can unite, forming a big lesion that can evolve into a virulent spot during storage; virulent spots, that occur when the fruit is mature, making the rind necrotic and becoming a great postharvest losses source; and false melanosis, that is an early pathogen expression, distinguish by small black spots (Kotzé, 1981; Frare et al., 2019). Commonly the symptoms that appear on varieties of citrus fruits (Hamlin, Pera, Valencia) are false melanosis, freckle spots, and hard spots. An important false melanosis aspect is the pycnidia absence, while both freckle spots and hard spots may have the presence of pycnidia in the lesion centre, and the existence of phenolic compounds around the stomata, suggesting that false melanosis symptom is a plant counterattack to the pathogen (Frare et al., 2019).
Symptomatic fruits with CBS are received in different ways on the domestic and foreign markets. For the domestic markets, the fruit peel damage causes an apprehension by the consumers in buying the product, while the foreign market, especially EU countries, has a strict policy regarding the fruit export with CBS symptoms. In Brazil, for example, the restrictions contributed to the decline in sweet orange exports to the EU between 1997 and 2015 (Silva Junior et al., 2016).
As the CBS symptoms only start to appear later, with the fruit formation, the pathogen detection must occur beforehand in order to implement strategies for its control. It is possible to detect the pathogen P. citricarpa and distinguish over other species in the genus Phyllosticta that are related to Citrus, through several molecular tests, such as polymerase chain reaction (PCR), real-time PCR, and loop-mediated isothermal amplification (LAMP) test (Meyer et al., 2012; Schirmacher et al., 2019). More recently, a real-time PCR test was developed to detect the P. citricarpa presence, with the advantage of being able to distinguish P. citricarpa from P. citriasiana, a pathogen that causes citrus tan spots on Citrus maxima. As P. citriasiana is a non-quarantine pathogen, the discovery was important to prevent the unnecessary quarantine on fruits with this fungus (Schirmacher et al., 2019).
The diseased orange tree diagnosis in the orchard allows the citrus grower to prevent the spread of the disease. The main measures to prevent the pathogen entry include the regulation of the production, transport and commercialization of seedlings and fruits, and the elimination of plant remains, for example. For areas already infected by the pathogens, the measures to be taken are the balanced chemical substances and fungicides use, removing fallen leaves from the surroundings (or accelerating their decomposition by artificial means), pruning their dry branches, harvesting in advance the fruits with CBS symptoms, and build a drip irrigation system (Silva Junior et al., 2016).
Various countries take rigorous measures to intercept the P. citricarpa propagation into CBS-free areas. However, so far the most effective way found for the CBS symptoms treatment is the fungicide application, preferably through the fruit susceptibility period (Kotzé, 1981; Silva Junior et al., 2016; Silva Junior et al., 2022). As each country has a unique climate, the period that each one applies the fungicide differs.
As the disease continues to cause damage and economic losses to citrus producers, the research on this area continues in development (Gomdola et al., 2022). Schreuder et al. (2018), tested various forms of postharvest treatments for CBS used in South Africa and concluded that cold storage, followed by packhouse treatment (a customary shipping protocol) improved the disease levels control in orange and lemons. More recently, Kupper et al. (2020) tested a potential use of antifungal compounds produced by Bacillus subtilis co-cultured with P. citricarpa at PDA medium for 10 days. LC-MS analyses detected antifungal compounds and two antibiotics, iturin and surfactin, which are compounds produced by bacteria to eliminate competitors (Kupper et al., 2020). Two strains of B. subtilis, separately, at 10% in the mineral oil applied as a spray were able to reduce the rate of the number of fruits that showed high disease severity (Kupper et al., 2020).
Another research indicated that the volatile organic compounds (VOCs) such as 3-methyl-1-butanol and 2-methyl-1-butanol produced by the Saccharomyces cerevisiae are able to inhibit the P. citricarpa growing (Toffano et al., 2017). Therefore, microbe metabolites have been an alternative increasingly explored as a CBS control method.
Fujimoto et al. (2022) evaluated the VOC production by two strains of Bacillus spp. (ACB-65 and ACB-73) in 5 different culture medium. The study verified a P. citricarpa colonies inhibition of up to 73% by VOC when tryptone soy agar (TSA) and tryptone soy agar (TSB) were used. GC-MS analysis showed the presence of 32 volatile metabolites, including alcohols, ketones, amines, ethers, aldehydes and carboxylic acids. Therefore, the results presented by Fujimoto et al. (2022) indicate a promising alternative to the use of synthetic fungicides to control CBS.
Final remarks and future perspectives
Citrus canker, CVC, HLB, and CBS are diseases that have impacted the world economy for decades (Bassanezi et al., 2016; Mendonça et al., 2017; Wetterich et al., 2017; Schirmacher et al., 2019). The mode of transmission occurs fast and cannot be immediately perceived by the citrus grower. Furthermore, there is not an efficient treatment for these diseases, leading to the death of infected plants (Yan & Wang, 2012; Frare et al., 2019; Mauricio et al., 2019; Gabriel et al., 2020).
The pathogenicity mechanisms of all these diseases are still not fully understood. Important reasons for the lack of knowledge are due to the great genetic variability of phytopathogens as the X. fastidiosa and Xanthomonas spp., the slow-growth of the fungus P. citricarpa and ineffective tentatives in culturing the bacterium Candidatus Liberibacter spp. Therefore, these challenges require deeper biochemical and chemical studies (Carvalho et al., 2005; Nunney et al., 2014; Frare et al., 2019; Merfa et al., 2019).
As a workaround to those limitations, current research has been dedicated to understand the mechanisms of infection during the disease’s initial stage using analytical approaches (Pontes et al., 2020b; Soares et al., 2020; Tran et al., 2020; Çetiner, 2022). With the development of biochemical methods and analytical platforms, we expect that the knowledge about the infection and pathogenicity mechanisms could promote the discovery of efficient strategies to diagnose and control the main citrus diseases.
Author contributions
HB, conducted the conceptualization of the manuscript and the major literature review. Furthermore, HB designed the figures; LF, reviewed and wrote about the Citrus Black Spot disease; JP, did considerations in the introduction, about huanglongbing disease, and wrote the final considerations and references; AP and TF, made considerations throughout the manuscript and critically reviewed the manuscript.
Funding
The authors acknowledge to the Coordenação de Aperfeiçoamento ,de Pessoal de Nível Superior—Brasil (CAPES)—Finance Code 001, Fundação de Amparo à Pesquisa no Estado de São Paulo [FAPESP Grant number 2019/06359-7, 2021/00728-0; FAPESP Grant number 2020/02805-0, 2021/08947-3; FAPESP Grant number 2019/17721-9], together with ABC and UNESCO in Brazil, and Serrapilheira Institute (grant number Serra-1912-31626).
Acknowledgments
We would like to thank Rodrigo Facchini Magnani and Nelson Arno Wulff from Fundecitrus company (Araraquara—Brazil) for their valuable discussions and partnership during the review writing.
Conflict of interest
The authors declare that the research was conducted in the absence of any commercial or financial relationships that could be construed as a potential conflict of interest.
Publisher’s note
All claims expressed in this article are solely those of the authors and do not necessarily represent those of their affiliated organizations, or those of the publisher, the editors and the reviewers. Any product that may be evaluated in this article, or claim that may be made by its manufacturer, is not guaranteed or endorsed by the publisher.
Abbreviations
ASM, acilbenzolar-S-methyl; CBS, citrus black spot; CVC, citrus variegated chlorosis; DESI-MSI, desorption electrospray ionization coupled to mass spectrometer imaging; EU, European Union; HLB, huanglongbing; IAN, 3-indolylacetonitrile; INA, isonicotinic acid; LAMP, loop-mediated isothermal amplification; LC-MS, liquid chromatography coupled to mass spectrometry; LPS, lipopolysaccharides; PCR, polymerase chain reaction; PDA, potato dextrose agar; RTX, repeats-in-toxin; SA, salicylic acid; SAR, systemic acquired resistance; TRV, tree row volume; VOCs, volatile organic compounds.
References
Abad, J. A., Bandla, M., French-Monar, R. D., Liefting, L. W., and Clover, G. R. G. (2009). First report of the detection of ‘Candidatus Liberibacter’ species in zebra chip disease-infected potato plants in the United States. Plant Dis. 93 (1), 108. doi:10.1094/PDIS-93-1-0108C
Abdulridha, J., Batuman, O., and Ampatzidis, Y. (2019). UAV-based remote sensing technique to detect citrus canker disease utilizing hyperspectral imaging and machine learning. Remote Sens. 11 (1), 1373. doi:10.3390/rs11111373
Achor, D., Welker, S., Ben-Mahmoud, S., Wang, C., Folimonova, S. Y., Dutt, M., et al. (2020). Dynamics of Candidatus Liberibacter asiaticus movement and sieve-pore plugging in citrus sink cells. Plant Physiol. 182, 882–891. doi:10.1104/pp.19.01391
Almeida, R. P. P., Blua, M. J., Lopes, J. R. S., and Purcell, A. H. (2005). Vector transmission of Xylella fastidiosa: Applying fundamental knowledge to generate disease management strategies. Ann. Entomol. Soc. Am. 98 (6), 775–786. doi:10.1603/0013-8746(2005)098[0775:VTOXFA]2.0.CO;2
Almeida, R. P. P., De La Fuente, L., Koebnik, R., Lopes, J. R. S., Parnell, S., and Scherm, H. (2019). Addressing the new global threat of Xylella fastidiosa. Phytopathology 109, 172–174. doi:10.1094/PHYTO-12-18-0488-FI
Alquézar, B., Carmona, L., Bennici, S., Miranda, M. P., Bassanezi, R. B., and Peña, L. (2022). Cultural management of Huanglongbing: Current status and ongoing research. Phytopathology 112, 11–25. doi:10.1094/PHYTO-08-21-0358-IA
Ammar, E.-D., George, J., Sturgeon, K., Stelinski, L. L., and Shatters, R. G. (2020). Asian citrus psyllid adults inoculate huanglongbing bacterium more efficiently than nymphs when this bacterium is acquired by early instar nymphs. Sci. Rep. 10, 18244. doi:10.1038/s41598-020-75249-5
Bahadur, A., and Srivastava, J. N. (2022). “Integrated management of citrus canker disease (Xanthomonas axonopodis pv. citri),” in Diseases of horticultural crops: Diagnosis and management. Editors J. N. Srivastava, and A. K. Singh (Boca Raton: Taylor & Francis Group), 170–183.
Bassanezi, R. B., Lopes, S. A., Belasque Júnior, J., Spósito, M. B., Yamamoto, P. T., Miranda, M. P., et al. (2010). Epidemiologia do huanglongbing e suas implicações para o manejo da doença. Citrus R&T 31 (1), 11–23. doi:10.5935/2236-3122.20100002
Bassanezi, R. B., Lopes, S. A., De Miranda, M. P., Wulff, N. A., Volpe, H. X. L., and Ayres, A. J. (2020). Overview of citrus huanglongbing spread and management strategies in Brazil. Trop. Plant Pathol. 45, 251–264. doi:10.1007/s40858-020-00343-y
Bassanezi, R. B., Montesino, L. H., and Stuchi, E. S. (2009). Effects of huanglongbing on fruit quality of sweet orange cultivars in Brazil. Eur. J. Plant Pathol. 125, 565–572. doi:10.1007/s10658-009-9506-3
Bassanezi, R. B., Silva Junior, G. S., Feichtenberger, E., Belasque Junior, J., Behlau, F., and Wulff, N. (2016). “A. Doenças dos Citros,” in Manual de fitopatologia: Doenças das plantas cultivadas. Editors L. Amorim, J. A. M. Rezende, A. Bergamim Filho, and L. E. A. Camargo (Ouro Fino: Agronômica Ceres), 271–306.
Batool, A., Iftikhar, Y., Mughal, S. M., Khan, M. M., Jaskani, M. J., Abbas, M., et al. (2007). Citrus greening disease – A major cause of citrus decline in the world: A review. Hort. Sci. 34, 159–166. doi:10.17221/1897-HORTSCI
Behlau, F. (2021a). An overview of citrus canker in Brazil. Trop. Plant Pathol. 46, 1–12. doi:10.1007/s40858-020-00377-2
Behlau, F., Belasque, J., Bergamin Filho, A., Graham, J. H., Leite, R. P., and Gottwald, T. R. (2008). Copper sprays and windbreaks for control of citrus canker on young orange trees in southern Brazil. Crop Prot. 27 (3-5), 807–813. doi:10.1016/j.cropro.2007.11.008
Behlau, F., Lanza, F. E., Scapin, M. da S., Scadelai, L. H. M., and Silva Júnior, G. J. (2021b). Spray volume and rate based on the tree row volume for a sustainable use of copper in the control of citrus canker. Plant Dis. 105, 183–192. doi:10.1094/PDIS-12-19-2673-RE
Behlau, F., Scandelai, L. H. M., da Silva Júnior, G. J., and Lanza, F. E. (2017). Soluble and insoluble copper formulations and metallic copper rate for control of citrus canker on sweet orange trees. Crop Prot. 94, 185–191. doi:10.1016/j.cropro.2017.01.003
Belasque Junior, J., Bassanezi, R. B., Yamamoto, P. T., Ayres, A. J., Tachibana, A., Violante, A. R., et al. (2010b). Lessons from huanglongbing management in São Paulo state, Brazil. J. Plant Pathol. 92 (2), 285–302. doi:10.4454/jpp.v92i2.171
Belasque Junior, J., Filho, A. B., Bassanezi, R. B., Barbosa, J. C., Fernandes, N. G., Yamamoto, P. T., et al. (2009). Base científica para a erradicação de plantas sintomáticas e assintomáticas de Huanglongbing (HLB, Greening) visando o controle efetivo da doença. Trop. Plant Pathol. 34 (3), 137–145. doi:10.1590/S1982-56762009000300001
Belasque Junior, J., Yamamoto, P. T., Miranda, M. P., Bassanezi, R. B., Ayres, A. J., and Bové, J. M. (2010a). Controle do huanglongbing no estado de São Paulo, Brasil. Citrus R&T 31 (1), 53–64. doi:10.5935/2236-3122.20100005
Blacutt, A., Ginnan, N., Dang, T., Bodaghi, S., Vidalakis, G., Ruegger, P., et al. (2020). An in vitro pipeline for screening and selection of citrus associated microbiota with potential anti-“Candidatus Liberibacter asiaticus” properties. Appl. Environ. Microbiol. 86 (8), e02883–19. doi:10.1128/AEM.02883-19
Bové, J. (2006). Huanglongbing: A destructive, newly-emerging, century-old disease of citrus. J. Plant Pathol. 88 (1), 7–37. doi:10.4454/jpp.v88il.828
Bové, J. M., and Garnier, M. (2003). Phloem-and xylem-restricted plant pathogenic bacteria. Plant Sci. 163 (6), 1083–1098. doi:10.1016/S0168-9452(02)00276-5
Braithwaite, M., Leite Júnior, R. P., Smith, J. J., Boa, E., and Saddler, G. S. (2002). First report of citrus canker by Xanthomonas campestris pv. citri on Citri sinensis in Bolivia. Plant Pathol. 51 (3), 383. doi:10.1046/j.1365-3059.2002.00696.x
Brunings, A. M., and Gabriel, D. W. (2003). Xanthomonas citri: Breaking the surface. Mol. Plant Pathol. 4 (3), 141–157. doi:10.1046/j.1364-3703.2003.00163.x
Byers, R. E., Hickey, K. D., and Hill, C. H. (1971). Base gallonage per acre. Va. Fruit. 60 (8), 19–23.
Canale, M. C., Tomaseto, A. F., Haddad, M. L., Coletta-Filho, H. D., and Lopes, J. R. S. (2017). Latency and persistence of ‘Candidatus Liberibacter asiaticus’ in its psyllid vector, Diaphorina citri (Hemiptera: Liviidae). Phytopathology 107, 264–272. doi:10.1094/PHYTO-02-16-0088-R
Carter, A. P., Clemons, W. M., Brodersen, D. E., Morgan-Warren, R. J., Wimberly, B. T., and Ramakrishnan, V. (2000). Functional insights from the structure of the 30S ribosomal subunit and its interactions with antibiotics. Nature 407, 340–348. doi:10.1038/35030019
Carvalho, F. M. S., Caramori, L. P. C., and Júnior, R. P. L. (2005). Genetic diversity of Xanthomonas axonopodis pv. citri based on plasmid profile and pulsed field gel electrophoresis. Genet. Mol. Biol. 28 (3), 446–451. doi:10.1590/S1415-47572005000300020
Caserta, R., Teixeira-Silva, N. S., Granato, L. M., Dorta, S. O., Rodrigues, C. M., Mitre, L. K., et al. (2020). Citrus biotechnology: What has been done to improve disease resistance in such an important crop? Biotechnol. Res. Innov. 3, 95–109. doi:10.1016/j.biori.2019.12.004
Çetiner, H. (2022). Citrus disease detection and classification using based on convolution deep neural network. Microprocess. Microsyst. 95, 104687. doi:10.1016/j.micpro.2022.104687
Cocuzza, G. E. M., Alberto, U., Hernández-Suárez, E., Silverio, F., Silvestro, S. D., Tena, A., et al. (2017). A review on Trioza erytreae (African citrus psyllid), now in mainland Europe, and its potential risk as vector of huanglongbing (HLB) in citrus. J. Pest Sci. 90, 1–17. doi:10.1007/s10340-016-0804-1
Coletta-Filho, H. D., Castillo, A. I., Laranjeira, F. F., de Andrade, E. C., Silva, N. T., de Souza, A. A., et al. (2020). Citrus variegated chlorosis: An overview of 30 years of research and disease management. Trop. Plant Pathol. 45, 175–191. doi:10.1007/s40858-020-00358-5
Coletta-Filho, H. D., Pereira, E. O., Souza, A. A., Takita, M. A., Cristofani-Yale, M., and Machado, M. A. (2007). Analysis of resistance to Xylella fastidiosa within a hybrid population of Pera sweet orange x Murcott tangor. Planto Pahotology 45 (4), 661–668. doi:10.1111/j.1365-3059.2007.01605.x
Coletta-Filho, H. D., Targon, M. L. P. N., Takita, M. A., De Negri, J. D., Pompeu Junior, J., Machado, M. A., et al. (2004). First report of the causal agent of Huanglonbing (“Candidatus Liberibacter asiaticus”) in Brazil. Plant Dis. 88 (12), 1382. doi:10.1094/PDIS.2004.88.12.1382C
Dala-Paula, B. M., Plotto, A., Bai, J., Manthey, J. A., Baldwin, E. A., Ferrarezi, R. S., et al. (2019). Effect of huanglongbing or greening disease on orange juice quality, a review. Front. Plant Sci. 9, 1976. doi:10.3389/fpls.2018.01976
Danós, E., Berger, R. D., and Stall, R. E. (1984). Temporal and spatial spread of Citrus Canker within groves. Phytopathology 74 (8), 904–908. doi:10.1094/phyto-74-904
Das, A. K. (2003). Citrus canker – a review. J. Appl. Hortic. 5 (1), 52–60. doi:10.37855/jah.2003.v05i01.15
Das, A. K., and Singh, S., 2000. Management of acid lime canker by using chemicals with compatible cultural practices. In: Hitech citrus management: Proceedings of the international symposium citriculture, eds. by S. P. Ghosh, and S. Singh, pp. 1054–1056. Indian Society of Citriculture and National Research Centre for Citrus, Nagpur, India.
Deng, H., Achor, D. S., Etxeberria, E., Yu, Q., Du, D., Stanton, D., et al. (2019). Phloem regeneration is a mechanism for Huanglongbing-tolerance of ‘Bearss’ lemon and ‘LB8-9’ sugar belle Mandarin. J. Front. Plant Sci. 10 (277), 277. doi:10.3389/fpls.2019.00277
Dickens, F. (1967). Carcinogenesis: A broad critique. Baltimore, Maryland: Williams & Wilkins, 447–470.
Dickens, F., and Jones, H. E. (1961). Carcinogenic activity of a series of reactive lactones and related substances. Br. J. Cancer 15 (1), 85–100. doi:10.1038/bjc.1961.10
Dickens, F., and Jones, H. E. H. (1962). Carcinogenic Action of certains lactones and chemically related substances. Br. J. Cancer 15, 85. doi:10.1038/bjc.1961.10
Dorta, S. O., Machado, M. A., and Freitas-Astúa, J. (2019). Desenvolvimento de estratégias alternativas visando ao controle do Huanglongbing. Citrus R&T 40, e1045. doi:10.4322/crt.17519
European and Mediterranean Plant Protection Organization (EPPO) (2020). EPPO global database. Available at: https://gd.eppo.int. [Accessed 27 October, 2022].
Fagen, J. R., Leonard, M. T., Coyle, J. F., McCullough, C. M., Davis-Richardson, A. G., Davis, M. J., et al. (2014). Liberibacter crescens gen. nov., sp. nov., the first cultured member of the genus Liberibacter. Int. J. Syst. Evol. 64, 2461–2466. doi:10.1099/ijs.0.063255-0
Feichtenberger, E., Muller, G. W., and Guirado, N. (1997). “Doenças dos citros (Citrus spp.),” in Manual de fitopatologia. Doenças das plantas cultivadas. Editors H. Kimati, L. Amorim, A. Bergamin Filho, L. E. A. Camargo, and J. A. M. Rezende (São Paulo: Agronômica Ceres), 247–280.
Ferreira, D. H., Moreira, R. R., Junior, G. J. S., and Behlau, F. (2022). Copper rate and spray interval for joint management of citrus canker and citrus black spot in orange orchards. Eur. J. Plant Pathol. 163, 891–906. doi:10.1007/s10658-022-02527-5
Fonseca, N. P., Patané, J. S. L., Varani, A. M., Felestrino, É. B., Caneschi, W. L., Sanchez, A. M., et al. (2019). Analyses of seven new genomes of Xanthomonas citri pv. Aurantifolii strains, causative agents of citrus canker B and C, show reduced repertoire of pathogenicity-related genes. Front. Microbiol. 10, 2361. doi:10.3389/fmicb.2019.02361
Food and Agriculture Organization of the United Nations (2020). Faostat. Available at: http://www.fao.org/faostat/en/#data/QC. [Accessed 04 January 2022].
Francis, M. I., Redondo, A., Burns, J. K., and Graham, J. H. (2009). Soil application of imidacloprid and related SAR-inducing compounds produces effective and persistent control of citrus canker. Eur. J. Plant Pathol. 124, 283–292. doi:10.1007/s10658-008-9415-x
Franco, D., de Goes, A., and Pereira, F. D. (2020). Sources and concentrations of cupric fungicides for the control of Citrus Black Spot. Rev. Caatinga 33 (1), 1–8. doi:10.1590/1983-21252020v33n101rc
Frare, G. F., Silva Junior, G. J., Lanza, F. E., Bassanezi, R. B., Ramires, T. G., and Amorim, L. (2019). Sweet orange fruit age and inoculum concentration affect the expression of Citrus Black Spot symptoms. Plant Dis. 103 (5), 913–921. doi:10.1094/PDIS-03-18-0492-RE
Fujimoto, A., Augusto, F., Fill, T. P., Moretto, R. K., and Kupper, K. C. (2022). Biocontrol of Phyllosticta citricarpa by Bacillus spp.: Biological and chemical aspects of the microbial interaction. World J. Microbiol. Biotechnol. 38, 53. doi:10.1007/s11274-021-03214-z
Gabriel, D. W., Duane, Y. P., and Ramadugu, C. (2000). “The molecular mechanism of citrus canker pathogenicity and a gene engineering approach to control,” in International Society of Citriculture, Orlando, FL, December 3–7, 2000, 51.
Gabriel, D., Gottwald, T. R., Lopes, S. A., and Wulff, N. A. (2020). Bacterial pathogens of citrus: Citrus canker, citrus variegated chlorosis and Huanglongbing. Genus Citrus 2020, 371–389. doi:10.1016/B978-0-12-812163-4.00018-8
Garnier, M., Bové, J. M., Sanders, G. M., Korsten, L., and Le Roux, H. F. (2000). “Presence of “Candidatus liberibacter africanus” in the western cape province of South Africa,” in Proceedings of 14th Conference of International Organization of Citrus Virologists, Campinas, São Paulo State, Brazil, 13-18 September 1998, 369–372.
Giampetruzzi, A., Saponari, M., Loconsole, G., Boscia, D., Savino, V. N., Almeida, R. P. P., et al. (2017). Genome-Wide analysis provides evidence on the genetic relatedness of the emergent Xylella fastidiosa genotype in Italy to isolates from Central America. Phytopathology 107 (7), 816–827. doi:10.1094/PHYTO-12-16-0420-R
Gochez, A. M., Behlau, F., Singh, R., Ong, K., Whilby, L., and Jones, J. B. (2020). Panorama of citrus canker in the United States. Trop. Plant Pathol. 45, 192–199. doi:10.1007/s40858-020-00355-8
Godefroid, M., Cruaud, A., Streito, J. C., Rasplus, J. Y., and Rossi, J. P. (2019). Xylella fastidiosa: Climate suitability of European continent. Sci. Rep. 9, 8844. doi:10.1038/s41598-019-45365-y
Gomdola, D., Bhunjun, C. S., Hyde, K. D., Jeewon, R., Pem, D., and Jayawardena, R. S. (2022). Ten important forest fungal pathogens: A review on their emergence and biology. Mycosphere 13, 612–671. doi:10.5943/mycosphere/13/1/6
Gottwald, T. R., Graham, J. H., and Schubert, T. S. (2002). Citrus Canker: The pathogen and its impact. Plant Health Prog. 3, 1. doi:10.1094/PHP-2002-0812-01-RV
Gottwald, T. R., Hughes, G., Graham, J. H., Sun, X., and Riley, T. (2001). The citrus canker epidemic in Florida: The scientific basis of regulatory eradication policy for an invasive species. Phytopathology 91 (1), 30–34. doi:10.1094/PHYTO.2001.91.1.30
Gottwald, T. R., McGuire, R. G., and Garran, S. (1988). Asiatic citrus canker: Spatial and temporal spread in simulated new planting situations in Argentina. Phytopathology 78 (6), 739–745. doi:10.1094/Phyto-78-739
Gottwald, T. R., and Timmer, L. W. (1995). The efficacy of windbreaks in reducing the spread of citrus canker caused by Xanthomonas campesiris pv. Citri. Trop. Agric. 72 (3), 194–201.
Govern Illes Balears (2017). Confirmats 219 positius per Xylella fastidiosa a les Illes Balears. Available at: https://www.caib.es/pidip2front/jsp/es/fitxa-convocatoria/8982890. [Accessed 04 January 2022].
Graça, J. V. (1991). Citrus greening disease. Ann. Rev. Pythopathol. 29, 109–136. doi:10.1146/annurev.py.29.090191.000545
Graham, J. H., Dewdney, M. M., and Myers, M. E. (2010). Streptomycin and copper formulations for control of citrus canker grapefruit. Proc. Fla. State Hort. Soc. 123, 32–99.
Gravena, S., Lopes, J. R. S., Paiva, P. E. B., Yamamoto, P. T., and Roberto, S. R. (1997). “Os vetores de Xylella fastidiosa,” in Clorose variegada dos citros. L. C. Donadio, and C. S. Moreira (Bebedouro: EECB/Fundecitrus), 37–119.
Haji-Hashemi, H., Norouzi, P., Safarnejad, M. R., Larijani, B., Habibi, M. M., Raeisi, H., et al. (2018). Sensitive electrochemical immunosensor for citrus bacterial disease detection using fast Fourier transformation square-wave voltammetry method. J. Electroanal. Chem. 820, 111–117. doi:10.1016/j.jelechem.2018.04.062
Halbert, S. E., and Manjunath, K. L. (2004). Asian citrus psyllids (sternorrhyncha: Psyllidae) and citrus greening disease of citrus: A literature review and assessment of risk in Florida. Fla. Entomol. 87 (3), 330–353. doi:10.1653/0015-4040(2004)087[0330:acpspa]2.0.co;2
Hall, D. G., Richardson, M. L., Ammar, E., and Halbert, S. E. (2012). Asian citrus psyllid, Diaphorina citri, vector of citrus huanglongbing disease. Entomol. Exp. 146 (2), 207–223. doi:10.1111/eea/12025
Hansen, A. K., Trumble, J. T., Stouthamer, R., and Paine, T. D. (2008). New Huanglongbing species, ‘Candidatus Liberibacter psyllaurous’ found to infect tomato and potato, is vectored by the psyllid Bactericera cockerelli (Sulc). Appl. Environ. Microbiol. 74 (18), 5862–5865. doi:10.1128/AEM.01268-08
He, C. X., Li, W. B., Ayres, A. J., Hartung, J. S., Miranda, V. S., and Teixeira, D. C. (2000). Distribution of Xylella fastidiosa in citrus rootstocks and transmission of citrus variegated chlorosis between sweet orange plants through natural root grafts. Plant Dis. 84 (6), 622–626. doi:10.1094/PDIS.2000.84.6.622
Hung, T. H., Hung, S. C., Chen, C. N., Hsu, M. H., and Su, H. J. (2004). Detection by PCR of Candidatus liberibacter asiaticus, the bacterium causing citrus huanglongbing in vector psyllids: Application to the study of vector-pathogen relationships. Plant Pathol. 53, 96–102. doi:10.1111/j.1365-3059.2004.00948.x
Hyun, J. W., Kim, H. J., Yi, P. H., Hwang, R. Y., and Park, E. W. (2012). Mode of action of streptomycin resistance in the citrus canker pathogen (Xanthomonas smithii subsp. citri) in Jeju Island. Plant Pathol. J. 28, 207–211. doi:10.5423/PPJ.2012.28.2.207
Innis, M. A., Gelfand, D. H., Sninsky, J. J., and White, T. J. (1990). PCR protocols: A guide to methods and applications. San Diego: Academic Press.
International Plant Protection Convention (IPPC) (2018). 2018 IPPC annual report. Available at: https://www.fao.org/3/ca3783en/ca3783en.pdf. [Accessed: 04 January, 2022].
Islam, M. N., Ali, M. S., Choi, S. J., Hyun, J. W., and Baek, K. H. (2019). Biocontrol of citrus canker disease caused by Xanthomonas citri subsp. Citri using an endophytic Bacillus thuringiensis. Plant Pathol. J. 35 (5), 486–497. doi:10.5423/PPJ.OA.03.2019.0060
Iwanami, T., Tuan, D. H., and Ichinose, K. (2013). Asian Citrus Greening and assessment of current techniques for reducing disease occurrences. J. Agric. Sci. 2 (1), 13–21. doi:10.14511/jasa.2013.020103
Jagoueix, S., Bové, J. M., and Garnier, M. (1994). The phloem-limited bacterium of greening disease of citrus is a member of the subdivision of the proteobacteria. Proteobacteria Int. J. Syst. Bacteriol. 44 (3), 379–386. doi:10.1099/00207713-44-3-379
Jones, D. R., Moffett, M. L., and Navaratnam, S. J. (1984). Citrus canker on thursday island. Plant Pathol. 13, 64–65. doi:10.1071/APP9840064a
Koizumi, M., Kimijima, E., Tsukamoto, T., Togawa, M., and Masui, S. (1996). “Dispersal of citrus canker bacteria in droplets and prevention with windbreaks,” in International citrus congress, 8, sun city. Procedings. Riverside (Spain: International Society of Citriculture), 340–344.
Kotzé, J. M. (1981). Epidemiology and control of citrus black spot in South Africa. Plant Dis. 65 (12), 945–950. doi:10.1094/PD-65-945
Kupper, K. C., Moretto, R. K., and Fujimoto, A. (2020). Production of antifungal compounds by Bacillus spp. isolates and its capacity for controlling citrus black spot under field conditions. World J. Microbiol. Biotechnol. 36 (7), 7–10. doi:10.1007/s11274-019-2772-0
Landa, B. B., Saponari, M., Feitosa-Junior, O. R., Giampetruzzi, A., Vieira, F. J. D., Mor, E., et al. (2022). Xylella fastidiosa’s relationships: The bacterium, the host plants, and the plant microbiome. New Pathol. 234, 1598–1605. doi:10.1111/nph.18089
Leite, R. P., and Mohan, S. K. (1990). Integrated management of the citrus bacterial canker disease caused by Xanthomonas campestris pv. citri in the State of Paraná. Braz. Crop Prot. 9 (1), 3–7. doi:10.1016/0261-2194(90)90038-9
Leonard, M. T., Fagen, J. R., Davis-Richardson, A. G., Davis, M. J., and Triplett, E. W. (2012). Complete genome sequence of Liberibacter crescens BT-1. Stand. genom. Sci. 7, 271–283. doi:10.4056/sigs.3326772
Li, J., Pang, Z., Duan, S., Lee, D., Kolbasov, V. G., and Wang, N. (2019). The in Planta effective concentration of oxytetracycline against ‘Candidatus Liberibacter asiaticus’ for suppression of citrus Huanglongbing. Phytopathology 109 (12), 2046–2054. doi:10.1094/PHYTO-06-19-0198-R
Li, J., and Wang, N. (2014). Foliar application of biofilm formation-inhibiting compounds enhances control of citrus canker caused by Xanthomonas citri subsp. Citri. Phytopathol. 104 (2), 134–142. doi:10.1094/PHYTO-04-13-0100-R
Li, W. B., Pria, W. D., Teixeira, D. C., Miranda, V. S., Ayres, A. J., Franco, C. F., et al. (2001). Coffee Leaf Scorch caused by a strain of Xylella fastidiosa from citrus. Plant Dis. 85 (5), 501–505. doi:10.1094/PDIS.2001.85.5.501
Li, W., Levy, L., and Hartung, J. S. (2009). Quantitative distribution of ‘Candidatus Liberibacter asiaticus’ in citrus plants with citrus huanglongbing. Phytopathology 99 (2), 139–144. doi:10.1094/PHYTO-99-2-0139
Li, W., Teixeira, D. V., Hartung, J. S., Huang, Q., Duan, Y., Zhou, L., et al. (2013). Development and systematic validation of qPCR assays for rapid and reliable differentiation of Xylella fastidiosa strains causing citrus variegated chlorosis. J. Microbiol. Methods 92, 79–89. doi:10.1016/j.mimet.2012.10.008
Liefting, L. W., Weir, B. S., Pennycook, S. R., and Clover, G. R. G. (2009). ‘Candidatus Liberibacter solanacearum’, associated with plants in the family Solanaceae. Int. J. Syst. Evol. 59, 2274–2276. doi:10.1099/ijs.0.007377-0
Lindow, S. (2019). Money matters: Fueling rapid recent insight into Xylella fastidiosa – an important and expanding global pathogen. Phytopathology 109, 210–212. doi:10.1094/PHYTO-09-18-0325-PER
Lopes, J. R. S., and Krugner, R. (2016). “Transmission ecology and epidemiology of the citrus variegated chlorosis strain of Xylella fastidiosa,” in Vector-mediated transmission of plant pathogens. Editor J. K. Brown (American Phytopathological Society Press), 195–208. doi:10.1094/9780890545355.014
Lopes, S. A. (2020). Scion substitution: A new strategy to control citrus variegated chlorosis disease. Plant Dis. 104 (1), 239–245. doi:10.1094/PDIS-02-19-0302-RE
Lorite, G. S., Rodrigues, C. M., Souza, A. A., Kranz, C., Mizaikoff, B., and Cotta, M. A. (2011). The role of conditioning film formation and surface chemical changes on Xylella fastidiosa adhesion and biofilm evolution. J. Colloid Interface Sci. 359 (1), 289–295. doi:10.1016/j.jcis.2011.03.066
Marcus, I. M., White, D., Backus, E. A., Walker, S. L., and Roper, M. C. (2022). Fluid dynamic simulations at the interface of the blue-green sharpshooter functional foregut and grapevine xylem sap with implications for transmission of Xylella fastidiosa. PLOS ONE 17, e0265762. doi:10.1371/journal.pone.0265762
Mauricio, F. N., Soratto, T. A. T., Diogo, J. A., Boscariol-Camargo, R. L., De Souza, A. A., Coletta-Filho, H. D., et al. (2019). Analysis of defense-related gene expression in citrus hybrids infected by Xylella fastidiosa. Xylella fastidiosa Phytopathol. 109, 301–306. doi:10.1094/phyto-09-18-0366-fi
McManus, P. S., Stockwell, V. O., Sundin, G. W., and Jones, A. L. (2002). Antibiotic use in plant agriculture. Annu. Rev. Phytopathol. 40, 443–465. doi:10.1146/annurev.phyto.40.120301.093927
Mendonça, L. B. P., Zambolim, L., and Badel, J. L. (2017). Bacterial citrus diseases: Major threats and recent progress. J. Bacteriol. Mycol. 5 (4), 340–350. doi:10.15406/jbmoa.2017.05.00143
Merfa, M. V., López, E. P., Naranjo, E., Jain, M., Gabriel, D. W., and De La Fuente, L. (2019). Progress and obstacles in culturing ‘Candidatus Liberibacter asiaticus’, the bacterium associated with huanglongbing. Phytopathology 109 (7), 1092–1101. doi:10.1094/PHYTO-02-19-0051-RVW
Meyer, L., Jacobs, R., Kotzé, J. M., Truter, M., and Korsten, L. (2012). Detection and molecular identification protocols for Phyllosticta citricarpa from citrus matter. S. Afr. J. Sci. 108 (3/4), 1–6. doi:10.4102/sajs.v108i3/4.602
Ministère DE L’Agriculture et de L’Alimentation (2019). La contamination par Xylella fastidiosa de 2 oliviers confirmée en PACA. Available at: https://agriculture.gouv.fr/la-contamination-par-xylella-fastidiosa-de-2-oliviers-confirmee-en-paca. [Accessed 04 January 2022].
Moll, J. N., and Martin, M. M. (1973). Electron microscope evidence that citrus psylla (Trioza erytreae) is a vector of greening disease in South Africa). Phytophylactica 5, 41–44.
Naem, A., Freed, S., Jin, F. L., Akmal, M., and Mehmood, M. (2016). Monitoring of insecticide resistance in Diaphorina citri Kuwayama (Hemiptera: Psyllidae) from citrus groves of Punjab, Pakistan. Crop Prot. 86, 62–68. doi:10.1016/j.cropro.2016.04.010
Naqvi, S. A. H., Wang, J., Malik, M. T., Umar, U. U. D., Rehman, A. U., Hasnain, A., et al. (2022). Citrus canker—distribution, taxonomy, epidemiology, disease cycle, pathogen biology, detection, and management: A critical review and future research agenda. Agronomy 12, 1075. doi:10.3390/agronomy12051075
Nasreen, R., Bora, P., and Medhi, K. K. (2020). Citrus canker: Developments down the lane. Ann. Plant Soil Res. 22 (4), 396–404. doi:10.47815/apsr.2020.10011
Newman, K. L., Almeida, R. P. P., Purcell, A. H., and Lindow, S. E. (2003). Use of a green fluorescent strain for analysis of Xylella fastidiosa colonization of Vitis vinifera. Appl. Environ. Microbiol. 69 (12), 7319–7327. doi:10.1128/AEM.69.12.7319-7327.2003
Nunney, L., Schuenzel, E. L., Scally, M., Bcromley, R. E., and Stouthamer, R. (2014). Large-Scarle intersubspecific recombination in the plant pathogenic bacterium Xylella fastidiosa is associated with the host shift to mulberry. Appl. Environ. Microbiol. 80 (10), 3025–3033. doi:10.1128/AEM.04112-13
Pereira, W., Takita, M., Souza, M. M. A., and de Souza, A. (2020). Citrus reticulata CrRAP2.2 transcriptional factor similar functions to the Arabidopsis homolog and increases resistance to Xylella fastidiosa. Mol. Plant-Microbe Interact. 33 (3), 519–527. doi:10.1094/MPMI-10-19-0298-R
Pernezny, K., Nagata, R., Havranek, N., and Sanchez, J. (2008). Comparison of two culture media for determination of the copper resistance of Xanthomonas strains and their usefulness for prediction of control with copper bactericides. Crop Prot. 27 (2), 256–262. doi:10.1016/j.cropro.2007.05.012
Pontes, J. G. M., Fernandes, L. S., dos Santos, R. V., Tasic, L., and Fill, T. P. (2020a). Virulence factors in the phytopathogen−host interactions: An overview. J. Agr. Food Chem. 68 (29), 7555–7570. doi:10.1021/acs.jafc.0c02389
Pontes, J. G. M., Vendramini, P. H., Fernandes, L. S., de Souza, F. H., Pilau, E. J., Eberlin, M. N., et al. (2020b). Mass spectrometry imaging as a potential technique for diagnostic of Huanglongbing disease using fast and simple sample preparation. Sci. Rep. 10, 13457. doi:10.1038/s41598-020-70385-4
Purcell, A. H. (1997). Xylella fastidiosa, a regional problem or global threat? J. Plant Pathol. 79 (2), 99–105.
Raddadi, N., Gonella, E., Camerota, C., Pizzinat, A., Tedeschi, R., Crotti, E., et al. (2011). ‘Candidatus Liberibacter europaeus’ sp. nov. that is associated with and transmitted by the psyllid Cacopsylla pyri apparently behaves as an endophyte rather than a pathogen. Environ. Microbiol. 13 (2), 414–426. doi:10.1111/j.1462-2920.2010.02347.x
Rapicavoli, J., Ingel, B., Blanco-Ulate, B., Cantu, D., and Roper, C. (2018). Xylella fastidiosa: An examination of a re-emerging plant pathogen. Mol. Plant Pathol. 19 (4), 786–800. doi:10.1111/mpp.12585
Rossetti, V., and Negri, J. D. (2011). Clorose variegada dos citros - revisão. Citrus R&T 32 (1), 61–66. doi:10.5935/2236-3122.20110007
Safady, N. G., Lopes, J. R. S., Francisco, C. S., and Coletta-Filho, H. D. (2019). Distribution and genetic diversity of Xylella fastidiosa subsp. Pauca associated with olive quick syndrome symptoms in Southeastern Brazil. Phytopathology 103 (2), 257–264. doi:10.1094/PHYTO-07-18-0273-FI
Sanchez, L., Pant, S., Xing, Z., Mandadi, K., and Kurouski, D. (2019). Rapid and noninvasive diagnostics of Huanglongbing and nutrient deficits on citrus trees with a handheld Raman spectrometer. Anal. Bioanal. Chem. 411, 3125–3133. doi:10.1007/s00216-019-01776-4
Santos, B. N. G., Anguita-Maeso, M., and Coletta-Filho, H. D. (2022). Transmission and distribution of Xylella fastidiosa subsp. pauca in olive trees as a parameter for managing olive quick decline syndrome. Plant Pathol. 71, 1849–1858. In Press. doi:10.1111/ppa.13627
Savi, D. C., Shaaban, K. A., Mitra, P., Ponomareva, L. V., Thorson, J. S., Glienke, C., et al. (2019). Secondary metabolites produced by the citrus phytopathogen Phyllosticta citricarpa. J. Antibiot. Res. 72, 306–310. doi:10.1038/s41429-019-0154-3
Scapin, M. S., Behlau, F., Scandelai, L. H. M., Fernandes, R. S., Silva Júnior, G. J., and Ramos, H. H. (2015). Tree-row-volume-based sprays of copper bactericide for control of citrus canker. Crop Prot. 77, 119–126. doi:10.1016/j.cropro.2015.07.007
Schaad, N. W., Postnikova, E., Lacy, G., Fatmi, M., and Chang, C. J. (2004). Xylella fastidiosa subspecies: X. fastidiosa subsp. piercei, subsp. nov., X. fastidiosa subsp. multiplex, subsp. nov., and X. fastidiosa subsp. pauca, subsp. nov. Syst. Appl. Microbiol. 27 (3), 290–300. doi:10.1078/0723-2020-00263
Schirmacher, A. M., Tomlinson, J. A., Barnes, A. V., and Barton, V. C. (2019). Species-specific real-time PCR for diagnosis of Phyllosticta citricarpa on Citrus species. Bull. OEPP 49 (2), 306–313. doi:10.1111/epp.12555
Schreuder, W., du Plooy, W., Erasmus, A., Savage, C., Basson, E., Lennox, C., et al. (2018). Postharvest fungicide treatments and cold storage control citrus black spot infections. Crop Prot. 112, 332–342. doi:10.1016/j.cropro.2018.06.020
Schubert, T. S., Dewdney, M. M., Peres, N. A., Palm, M. E., Jeyaprakash, A., Sutton, B., et al. (2012). First report of Guignardia citricarpa associated with citrus black spot on sweet orange (Citrus sinensis) in North America. Plant Dis. 96 (8), 1225. doi:10.1094/PDIS-01-12-0101-PDN
Schuenzel, E. L., Scally, M., Stouthamer, R., and Nunney, L. (2005). A multigene phylogenetic study of clonal diversity and divergence in North American strains of the plant pathogen Xylella fastidiosa. Xylella fastidiosa Appl. Environ. Microbiol. 71 (7), 3832–3839. doi:10.1128/AEM.71.7.3832-3839.2005
Secor, G. A., Rivera, V. V., Abad, J. A., Lee, I. M., Clover, G. R. G., Liefting, L. W., et al. (2009). Association of ‘Candidatus Liberibacter solanacearum’ with zebra chip disease of potato established by graft and psyllid transmission, electron microscopy, and PCR. Plant Dis. 93 (6), 574–583. doi:10.1094/PDIS-93-6-0574
Sena-Vélez, M., Holland, S. D., Aggarwal, M., Cogan, N. G., Jain, M., Gabriel, D. W., et al. (2019). Growth dynamics and survival of Liberibacter crescens BT-1, an important model organism for the citrus Huanglongbing pathogen “Candidatus Liberibacter asiaticus. Appl. Environ. Microbiol. 85 (21), 016566–e1719. doi:10.1128/AEM.01656-19
Serra, W., Álvarez, M. B. L., Rodríguez, D. G., Alonso-Oliva, E., Llorente, A. S., Guarnaccia, V., et al. (2022). Polyphasic identifcation and MAT1-2 isolates of Phyllosticta citricarpa in Cuba. Eur. J. Plant Pathol. 162, 995–1003. doi:10.1007/s10658-021-02453-y
Silva Junior, G. J., Feichtenberger, E., Spósito, M. B., Amorim, L., Bassanezi, R. B., and Goes, A. (2016). Pinta preta dos citros: A doença e seu manejo. Araraquara, São PauloBrasil: Fundecitrus.
Silva Junior, G. J., Moraes, M. R., Moreira, R. R., and Behlau, F. (2022). Tree age and cultivar-oriented use of mineral oil added to fungicide tank mixture for the control of citrus black spot in sweet orange orchards. Pest Manag. Sci. 78, 488–498. doi:10.1002/ps.6652
Simpson, A. J. G., Reinach, F. C., Arruda, P., Abreu, F. A., Acencio, M., Alvarenga, R., et al. (2000). The genome sequence of the plant pathogen Xylella fastidiosa. Nature 406, 151–157. doi:10.1038/35018003
Singerman, A., Burani-Arouca, M., and Futch, S. H. (2018). The profitability of new citrus plantings in Florida in the Era of Huanglongbing. HortScience 53 (11), 1655–1663. doi:10.21273/HORTSCI13410-18
Soares, M. S., da Silva, D. F., Amaral, J. C., da Silva, M. M., Forim, M. R., Rodrigues-Filho, E., et al. (2020). Rapid differentiation of graft Citrus sinensis with and without Xylella fastidiosa infection by mass spectrometry. Rapid Commun. Mass Spectrom. 34, e8745. doi:10.1002/rcm.8745
Souza, A. A., Takita, M. A., Coletta-Filho, H. D., Caldana, C., Yanai, G. M., Muto, N. H., et al. (2004). Gene expression. Profile of the plant pathogen Xylella fastidiosa during biofilm formation in vitro. FEMS Microbiol. Lett. 237 (2), 341–353. doi:10.1016/j.femsle.2004.06.055
Stall, R. E., and Seymour, C. P. (1983). Canker, a threat to citrus in the gulf-coast states. Plant Dis. 67, 581–585. doi:10.1094/PD-67-581
Stein, B., Ramallo, J., Foguet, L., and Graham, J. H. (2007). Citrus Leafminer control and copper sprays for management of citrus canker on lemon in Tucumán, Argentina. Proc. Fla. State Hort. Soc. 120, 127–131.
Teixeira, D. C., Danet, J. L., Eveillard, S., Martins, E. C., Jesus Junior, W. C., Yamamoto, P. T., et al. (2005). Citrus huanglongbing in São Paulo state, Brazil: PCR detection of the ‘Candidatus’ liberibacter species associated with the disease. Mol. Cell. Probes 19 (3), 173–179. doi:10.1016/j.mcp.2004.11.002
Teixeira, D. C., Wulff, N. A., Lopes, S. A., Yamamoto, P. T., Miranda, M. O., Spósito, M. B., et al. (2010). Caracterização e etiologia das bactérias associadas ao huanglongbing. Citrus R&T 31, 115–128. doi:10.5935/2236-3122.20100012
Thomas, B. (2017). “Sources and sinks,” in Life Sciences - encyclopedia of applied plant Sciences B. Thomas, B. G. Murray, and D. J Murphy. (Amsterdam, Netherlands: Elsevier), 119–127. doi:10.1016/B978-0-12-394807-6.00096-4
Toffano, L., Fialho, M. B., and Pascholati, S. F. (2017). Potential of fumigation of orange fruits with volatile organic compounds produced by Saccharomyces cerevisiae to control citrus black spot disease at postharvest. Biol. Control 108, 77–82. doi:10.1016/j.biocontrol.2017.02.009
Tran, N. T., Miles, A. K., Dietzgen, R. G., and Drenth, A. (2019). Phyllosticta capitalensis and P. paracapitalensis are endophytic fungi that show potential to inhibit pathogenic P. citricarpa on citrus. Australas. Plant Pathol. 48, 281–296. doi:10.1007/s13313-019-00628-0
Tran, T. T., Clark, K., Ma, W., and Mulchandani, A. (2020). Detection of a secreted protein biomarker for citrus Huanglongbing using a single-walled carbon nanotubes-based chemiresistive biosensor. Biosens. Bioelectron. 147 (111766), 111766–111769. doi:10.1016/j.bios.2019.111766
USDA/FAS Foreign Agricultural Services (2021). Citrus: World markets and trade. Availabe at: https://apps.fas.usda.gov/psdonline/circulars/citrus.pdf. [Accessed: 04 January, 2022].
Uthman, Q. O., Atta, A. A., Kadyampakeni, D. M., Qureshi, J. A., Morgan, K. T., and Nkedi-Kizza, P. (2022). Integrated water, nutrient, and pesticide management of Huanglongbing-affected sweet oranges on Florida sandy soils - a Review. Plants 11, 1850. doi:10.3390/plants11141850
Vieira, G., Khalil, Z. G., Capon, R. J., Sette, L. D., Ferreira, H., and Sass, D. C. (2022). Isolation and agricultural potential of penicillic acid against citrus canker. J. Appl. Microbiol. 132, 3081–3088. doi:10.1111/jam.15413
Vincent, C. I., Hijaz, F., Pierre, M., and Killiny, N. (2022). Systemic uptake of oxytetracycline and streptomycin in Huanglongbing-affected citrus groves after foliar application and trunk injection. Antibiotics 11, 1092. doi:10.3390/antibiotics11081092
Walters, D. R., Ratsep, J., and Havis, N. D. (2013). Controlling crop diseases using induced resistance: Challenges for the future. J. Exp. Bot. 64, 1263–1280. doi:10.1093/jxb/ert026
Wang, N. (2020). A perspective of citrus Huanglongbing in the context of the Mediterranean basin. J. Plant Pathol. 102, 635–640. doi:10.1007/s42161-020-00555-w
Wang, N., Pierson, E. A., Setubal, J. C., Xu, J., Levy, J. G., Zhang, Y., et al. (2017). The Candidatus Liberibacter-Host interface: Insights into pathogenesis mechanisms and disease control. Ann. Rev. Phytopathol. 55, 451–482. doi:10.1146/annurev-phyto-080516-035513
Wang, N. (2019). The citrus Huanglongbing crisis and potential solutions. Mol. Plant 12, 607–609. doi:10.1016/j.molp.2019.03.008
Wetterich, C. B., Neves, R. F. O., Belasque, J., Ehsani, R., and Marcassa, L. G. (2017). Detection of Huanglongbing in Florida using fluorescence imaging spectroscopy and machine-learning methods. J. Appl. Opt. 56 (1), 15–23. doi:10.1364/AO.56.000015
Yan, Q., and Wang, N. (2012). High-Throughput screening and analysis of genes of Xanthomonas citri subsp. citri involved in citrus canker symptom development. Mol. Plant Microbe Interact. 1, 69–84. doi:10.1094/mpmi-05-11-0121
Yang, C., Powell, C. A., Duan, Y., Ancona, V., Huang, J., and Zhang, M. (2020). Transcriptomic analysis reveals root metabolic alteration and induction of huanglongbing resistance by sulphonamide antibiotics in huanglongbing-affected citrus plants. Plant Pathol. 69, 733–743. doi:10.1111/ppa.13154
Yang, Y., Huang, M., Beattie, G. A. C., Xia, Y., Ouyang, G., and Xiong, J. (2006). Distribution, biology, ecology and control of the psyllid Diaphorina citri kuwayana, a major pest of citrus: A status report for China. Int. J. Pest Manag. 52 (4), 343–352. doi:10.1080/09670870600872994
Zhang, M., Yang, C., Powell, C. A., Avery, P. B., Wang, J., Huang, Y., et al. (2019). Field evaluation of integrated management for mitigating citrus Huanglongbing in Florida. Front. Plant Sci. 9, 1890. doi:10.3389/fpls.2018.01890
Keywords: citriculture, diseases, control strategies, diagnostic, citrus
Citation: Barbieri HB, Fernandes LS, Pontes JGdM, Pereira AK and Fill TP (2023) An overview of the most threating diseases that affect worldwide citriculture: Main features, diagnose, and current control strategies. Front. Nat. Prod. 2:1045364. doi: 10.3389/fntpr.2023.1045364
Received: 15 September 2022; Accepted: 06 January 2023;
Published: 24 January 2023.
Edited by:
Dulce Helena Siqueira Silva, São Paulo State University, BrazilReviewed by:
Massuo Jorge Kato, University of São Paulo, BrazilCaixiang Liu, University of Chinese Academy of Sciences, China
Copyright © 2023 Barbieri, Fernandes, Pontes, Pereira and Fill. This is an open-access article distributed under the terms of the Creative Commons Attribution License (CC BY). The use, distribution or reproduction in other forums is permitted, provided the original author(s) and the copyright owner(s) are credited and that the original publication in this journal is cited, in accordance with accepted academic practice. No use, distribution or reproduction is permitted which does not comply with these terms.
*Correspondence: Taicia Pacheco Fill, dGFpY2lhQHVuaWNhbXAuYnI=