- Spine Division, Departments of Orthopaedic and Neurological Surgery, Duke University Health System, Durham, NC, United States
Introduction: Although autologous iliac crest bone graft (ICBG) has long been the gold standard for spinal fusion, complications related to its harvest and availability issues with local bone autograft have encouraged the development of bone graft substitutes that provide safer alternatives with consistent clinical efficacy and potential for applications across musculoskeletal health, including spinal fusion. This study evaluates the initial safety and efficacy of a novel integrative bone matrix (IBM) in spinal fusion procedures.
Methods: The charts of twenty patients who underwent spinal fusion surgery at 1–5 contiguous interbody levels and/or 1–15 contiguous posterolateral levels with the novel IBM between November 2022 and May 2023 were retrospectively evaluated for safety and efficacy endpoints at standard of care 3, 6, and 12 months postoperative follow up visits. Radiographic fusion rate was evaluated by plain radiographs using the Bridwell interbody fusion grading system and/or the Glassman posterolateral fusion grading system, as appropriate. Subjective pain, disability, and quality of life assessments included the EuroQoL 5 Dimensions Visual Analogue Scale (EQ-5D VAS), Oswestry Disability Index (ODI), and the revised Scoliosis Research Society Score (SRS-22r).
Results: No adverse events occurred that were related to the graft, and no subjects required unplanned revision surgery. Radiographic fusion was achieved in all (100%) of the interbody and posterolateral levels by 12 months. There was no significant difference in overall fusion rate between subjects receiving short vs. long segment constructs. At 3, 6, and 12 month follow up, significant (p < 0.001) improvements were observed compared to baseline values for all patient reported outcome measures, and the cohort reached the minimum clinically significant mean improvements.
Discussion: This study highlights the potential of this novel IBM as a safe and effective bone graft substitute in spinal arthrodesis procedures. Patients had a high rate of fusion without any graft-related adverse events. Larger, controlled studies with longer-term follow-up are warranted for further validation.
Introduction
Spinal fusion plays a pivotal role in managing pain from a diverse range of spinal disorders, including degenerative disc disease, spinal stenosis, spondylolisthesis, and spinal fractures. From 1998 to 2008, the frequency of spinal fusion discharges was reported to have increased by 137%, which further increased between the years 2002 and 2014 to 276% for elective lumbar fusion procedures (1–3). The use of autologous iliac crest bone graft (ICBG) has been considered the gold standard for bone grafting in spinal fusion surgery, due to its natural osteoconductive, osteoinductive, and osteogenic properties. However, harvesting ICBG can lead to complications such as pain, blood loss, and infection (4, 5).
One systematic review and meta-analysis compared ICBG to local autologous bone in spinal fusion procedures and found that the use of local bone resulted in significantly higher fusion rates compared to ICBG (5). However, although autologous local bone from the operative site may serve as an alternative, its limited availability and variable quality present challenges in achieving consistent surgical outcomes (6). Thus, there is an unmet need for bone graft substitutes that can offer comparable efficacy in supporting bone healing while circumventing the limitations of autologous grafting (5, 7). Bone graft substitutes, such as those from either allogeneic (donor) or synthetic sources, can fulfill this need, however not all bone graft substitutes are biomimetic alternatives for autologous bone (4, 5, 7, 8).
Specifically in the field of regenerative medicine, extensive research efforts have focused on identifying novel osteobiologics capable of enhancing bone healing and integration in spinal fusion procedures (4, 5, 7). Bone graft substitutes with osteoconductive and osteoinductive characteristics accelerate bone healing by releasing growth factors and cytokines, which facilitates cell proliferation, angiogenesis, and extracellular matrix deposition for optimal bone regeneration and integration (4, 7). A recent advancement in bone grafting technology was the development of cellular bone matrices (CBMs) or viable bone matrices (VBMs) that preserve viable cells, aiming to enhance osteogenesis and improve patient outcomes (4, 9). As with many advancements, improvements in grafting technology often come with compromise. The focus on preserving viable cell populations in CBMs and VBMs often comes at the price of reducing inductive factors and/or suboptimal handling of the bone graft during manufacturing. However, the latest advancement in bone grafting technology (integrative bone matrix, IBM) provides viable and functional cells while including improved graft handling and the preservation of a greater concentration of inherent osteoinductive and angiogenic proteins compared to what has been previously reported for manufactured demineralized bone (10–12). Due to the critical role of angiogenesis in bone healing, there is an unmet need for an advanced viable bone graft that can provide high levels of both osteoinductive and angiogenic growth factors to support clinical success.
This article provides preliminary observational data on the use of a novel integrative bone matrix (IBM) in spinal fusion procedures and summarizes the regenerative properties of advanced viable bone grafts such as IBM.
Materials and methods
This study was reviewed and approved by our institutional IRB review process. A waiver of Informed Consent and HIPAA was obtained due to the retrospective nature of the work performed. This study was conducted in accordance with the ethical principles in the Declaration of Helsinki and conducted according to U.S. and international standards of Good Clinical Practice in accordance with applicable Federal regulations, International Council for Harmonization guidelines, and institutional research policies and procedures.
Subject population
This retrospective observational study was performed to evaluate initial safety and efficacy outcomes of INFLUX™ SPARC integrative bone matrix (IBM) implanted between November 2022 and May 2023 in patients aged 18–80 years undergoing spinal fusion surgery. Only patients receiving the novel IBM as a bone graft were included in the study. Enrolled subjects included those with spinal fusion surgery at 1–5 contiguous interbody levels and/or 1–15 contiguous posterolateral levels. Subjects with fusion constructs from 1 to 3 interbody or posterolateral levels were defined as short segment, and those that had greater than 3 interbody or posterolateral levels in their fusion constructs were defined as long segment.
Endpoints
The primary endpoint was evaluation of fusion of the treated levels, as assessed by the operative surgeon through radiographic examination of plain films (and CT imaging when available) collected at baseline (preoperative) and 3, 6, and 12 months postoperatively utilizing the Bridwell interbody fusion grading system and the Glassman posterolateral fusion grading system as appropriate (Tables 1, 2) (13, 14). Grades I and II on the Bridwell system and Grades 4 and 5 on the Glassman system were classified as successful fusion based on established literature (14, 15).
The operative physician's standard of care patient-reported assessments collected at baseline (preoperative) and 3-, 6-, and 12-months postoperatively for pain, function, and quality of life were the secondary endpoints of the study: EuroQoL 5 Dimensions Visual Analogue Scale (EQ-5D VAS), Oswestry Disability Index (ODI), and the revised Scoliosis Research Society Score (SRS-22r). The EQ-5D VAS scale ranges from 0 to 100, with 0 indicating “the worst health you can imagine” and 100 indicating “the best health you can imagine” (16). ODI scores can range from 0% (no disability) to 100% (most severe disability) (17). SRS-22r has a minimum score of 1 and a maximum score of 5, with higher scores indicating better patient quality of life (18). Minimal clinically significant improvements were defined as an increase of 12 points for EQ-5D VAS, a 10% decrease in points for ODI, and a mean increase of 0.4 for SRS-22r, as established in the literature (19–21). Safety evaluation included incidence of pseudoarthrosis or unplanned reoperation related to the use of IBM.
Statistical analysis
Continuous variables are presented as means with standard deviations and categorical data are represented as counts with percentages. Fusion rates were considered noncontinuous variables, whereas pain and disability scores were treated as ordinal variables. The number of levels treated was correlated with preoperative quality of life scale scores using Spearman correlations. Independent median samples tests were used to compare median time to fusion for short vs. long segment subjects, and those with vs. without comorbidities. The nonparametric, related-samples Friedman's Analysis of Variance was used to examine change in quality-of-life outcomes over time. The Bonferroni correction was applied to pairwise post hoc analyses. Statistical significance was set at p < .05. SPSS software version 27 (IBM Corp.; Armonk, New York, USA) was used for statistical analysis.
Results
Patient and procedural details
A total of 20 patients met the inclusion criteria for this study, 50% (n = 10) of which were male. Table 3 provides a summary of subject demographics and health profiles. Subjects had a mean age of 60.4 years (range 45–79) and a mean body mass index (BMI) of 31.9 kg/m2. A quarter (25.0%) of the subjects were current smokers and 35.0% were currently taking steroids. Diagnoses within patient charts revealed a range of health conditions: 45.0% of subjects were obese (BMI > 30), 30.0% had cardiovascular disease, 20.0% had kidney disease, 5.0% had Type II diabetes, and 35.0% were undergoing a revision surgery.
On average, each subject had 3.6 ± 1.4 indications for surgery (Table 4). The most common indications were stenosis (90.0%), followed by herniated nucleus pulposus (HNP) (75.0%), spondylolisthesis (60.0%), and spondylosis (50.0%). There were no cases of degenerative disc disease (DDD). Other indications (85.0%) included scoliosis, radiculitis/radiculopathy, kyphosis, and revision. Most subjects underwent interbody with posterior spinal fusion (90.0%), but some underwent posterolateral fusion alone (10.0%). The cohort was split evenly between short (50.0%) vs. long segment (50.0%) fusion constructs. The average number of levels treated per interbody subject was 2.1 (ranging from T8 to S1), and 5.0 for posterolateral subjects (ranging from C2 to S1). However as expected, the most treated levels were between L3 – S1 (70.2% of interbody and 32.3% of posterolateral). The number of levels treated was not associated with preoperative EQ-5D [r(19) = −0.075, p = 0.752], ODI [r(19) = 0.123, p = 0.604], or SRS-22r [r(19) = 0.004, p = 0.985] scores.
Approximately half of the procedures were performed using minimally invasive approaches (55.0% TLIF or LLIF). All interbody subjects (100.0%) received an expandable titanium interbody device (Globus Medical, Audubon, Pennsylvania) and bilateral percutaneous pedicle screw constructs with posterolateral grafting. A total of 5.0cc of IBM was used per fusion area (i.e., 5.0cc for posterolateral fusion alone, 10.0cc for both interbody and posterolateral), and all posterior fusions also included the use of available local autograft (approximately 6cc per level). All subjects who underwent ALIF, LLIF, or TLIF also underwent PSF. When preparing the fusion graft for implantation during intervertebral fusion, the expandable cage was first packed with bone graft and implanted in a collapsed state, then was expanded to the desired height and backfilled with bone graft. No patients required harvesting of ICBG.
Clinical and radiographic outcomes
Radiographic fusion rates per level are summarized in Table 5. For interbody fusion (n = 37 levels), assessed using the Bridwell grading system (Table 1) and literature definition for successful fusion (grade I or II), fusion rates steadily increased over time, with 77.8% at 3 months, 94.6% at 6 months, reaching 100.0% at 12 months post-surgery (13, 15). The median time to interbody fusion was 3.0 months (range 3.0–4.5). Posterior fusion rates (n = 99 levels), evaluated via the Glassman grading system (Table 2) and literature definition for successful fusion (grade 4 or 5), showed a slower progression with only 2.0% achieving fusion at 3 months, increasing to 57.6% at 6 months, and eventually reaching 100.0% by 12 months, with a median fusion time of 6.0 months (range 6.0–12.0) (14). The combined fusion rate, i.e., scores of Bridwell grade I or II and Glassman grade 4 or 5 for subjects with interbody and posterolateral grafting (n = 37 levels), was 2.7% at 3 months, 62.2% at 6 months, and 100.0% at 12 months, with a median fusion time of 6.0 months (range 6.0–12.0). Although excellent reference images can be found in the original publications, example radiographs representing the interbody and posterolateral scoring systems from our subject cohort are shown in Figure 1 (13, 14).
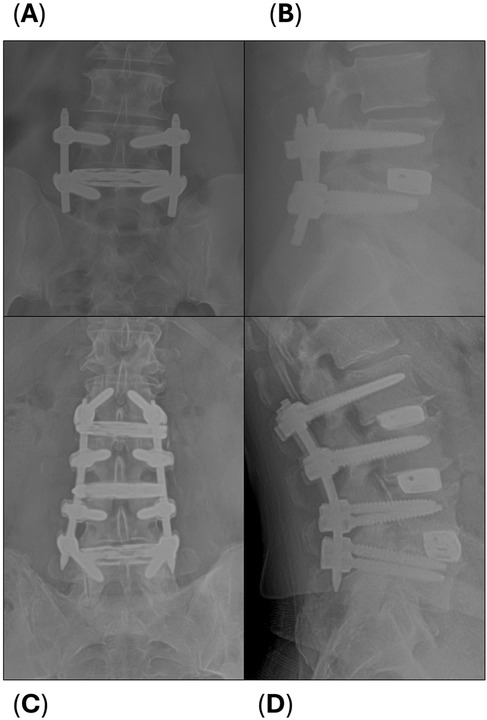
Figure 1. Example radiographs of interbody (Bridwell) and posterolateral (Glassman) scoring. (A) At 3 months, this single level LLIF subject was scored as Bridwell Grade III (not fused) and (B) Glassman Grade 2 (not fused). (C) At 12 months, this 3-level LLIF subject was scored as Bridwell Grade I (fused) and (D) Glassman Grade 5 (fused) at each level.
Radiographs and CT imaging that display baseline and successful fusion at 6- and 12-month follow-up are provided of two subjects in Figure 2: a low-risk subject undergoing a single level interbody and posterior fusion, and a high-risk subject undergoing an 11-level revision posterior fusion. One subject achieved their single level fusion on both scales at only 3 months: a high risk 77-year-old obese female who was a current smoker, hypertensive, with coronary artery and peripheral vascular disease, and was currently taking corticosteroids. No patients experienced pseudarthrosis or required unplanned revision surgery. When fusion rates between subjects receiving short vs. long segment constructs were compared, no significant differences were found (p > 0.05 for interbody, posterolateral only, and combined median times to fusion), and there were no differences in comorbid risk factors between these groups. Further, no significant differences were found in median time to fusion for subjects who were current smokers, currently on steroids, or obese (>30 kg/m2), however obesity trended with a shorter time to fusion (p = 0.057).
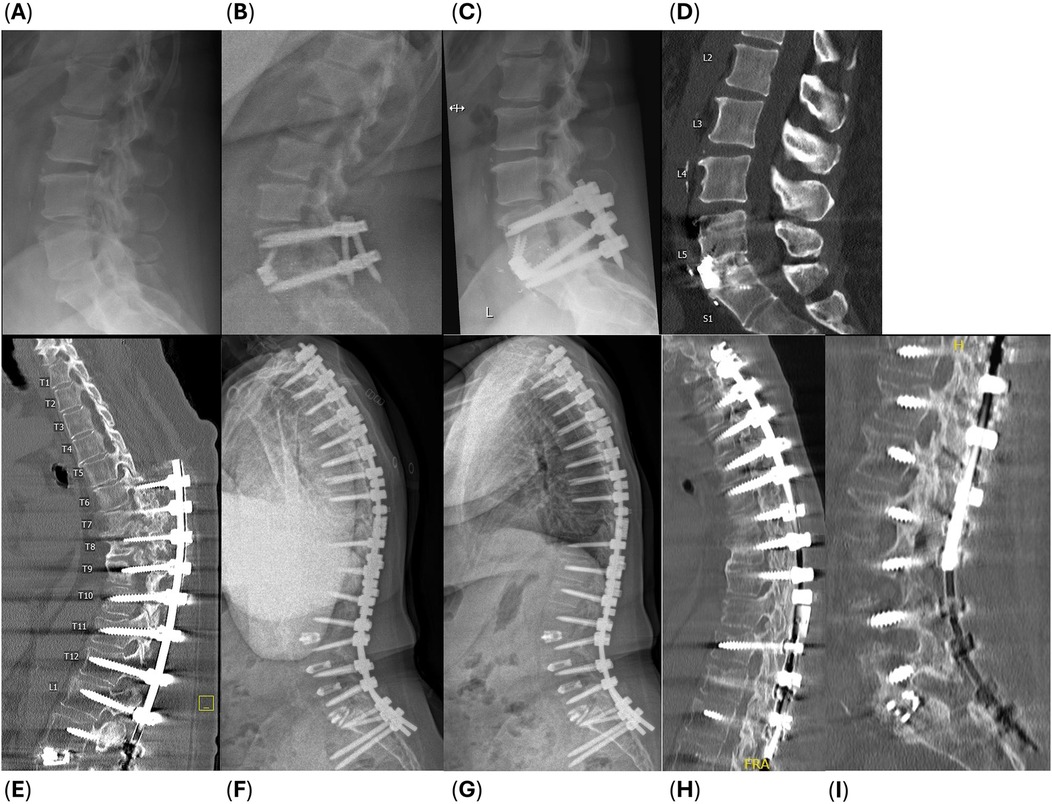
Figure 2. Example radiographs of two subjects: (A–D) a 51-year-old obese female who underwent a single level ALIF from L5 to S1, and (E–I) a 61-year-old obese, current smoker female with hypertension who underwent a revision T2 to L1 posterolateral fusion after a L1 to L3 TLIF with T6 to pelvis posterior fusion 8 months prior, and a L3 to L5 PLIF 6 years prior (subject experienced PJF and pseudarthrosis in the thoracic spine and underwent hardware removal with revision in the latest procedure). (A,E) Baseline radiographic imaging and successful fusion apparent at (B,F) 6-month follow up, (C,G) 12-month follow-up, and (D,H,I) CT images at 12 months postoperative.
Subjective clinical outcomes
Subject-reported outcomes, including: EuroQoL 5 Dimensions Visual Analogue Scale (EQ-5D VAS) for pain, Oswestry Disability Index (ODI) for disability, and Scoliosis Research Society Score (SRS-22r) for quality of life demonstrated statistically significant main effects (p < 0.001). Additionally, post-hoc comparisons at 3-, 6-, and 12 months were significantly improved compared to baseline (p < 0.001 for all comparisons) (Table 6, Figures 3–5).
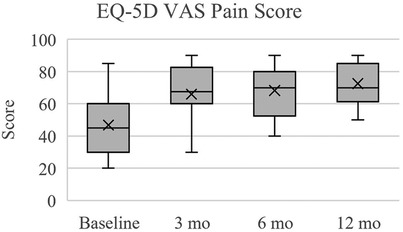
Figure 3. EuroQoL 5 dimensions visual analogue scale (EQ-5D VAS) for pain. Scores reported here and in Table 6 are presented as median (IQR, 25th – 75th percentile). At each follow up timepoint, mean scores were: 46.8 ± 19.8 (baseline), 66.0 ± 16.6 (3 months), 68.3 ± 16.3 (6 months), and 72.5 ± 12.7 (12 months).
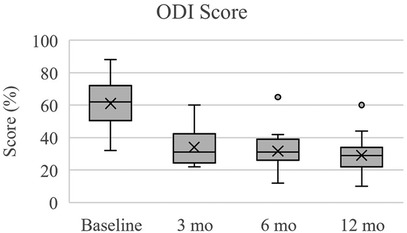
Figure 4. Oswestry disability Index (ODI) for disability. Scores reported here and in Table 6 are presented as median (IQR, 25th – 75th percentile). At each follow up timepoint, mean scores were: 61.1 ± 14.0 (baseline), 34.2 ± 11.0 (3 months), 31.8 ± 10.8 (6 months), and 29.1 ± 11.4 (12 months).
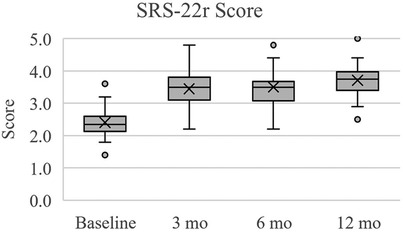
Figure 5. Scoliosis research society score (SRS-22r) for quality of life. Scores reported here and in Table 6 are presented as median (IQR, 25th – 75th percentile). At each follow up timepoint, mean scores were: 2.4 ± 0.5 (baseline), 3.4 ± 0.6 (3 months), 3.5 ± 0.6 (6 months), and 3.7 ± 0.5 (12 months).
In terms of pain perception measured by EQ-5D VAS, subjects exhibited significant improvements over the follow up times (Table 6, Figure 3). At baseline, the median pain score was 45.0 (IQR: 30.0–60.0), which notably increased to 67.5 (IQR: 60.0–82.5) at 3 months (p < 0.001), further improving to 70.0 (IQR: 52.5–80.0) at 6 months (p < 0.001), and 70.0 (IQR: 61.3–85.0) at 12 months (p < 0.001). Regarding disability levels assessed by ODI, subjects experienced a significant reduction in disability from baseline (median score: 62.0, IQR: 50.5–72.0, p < 0.001) to 3 months (median score: 31.0, IQR: 24.5–42.3, p < 0.001), remaining relatively stable at both 6 months (median score: 31.0, IQR: 26.0–39.0, p < 0.001) and 12 months (median score: 29.0, IQR: 22.0–34.0, p < 0.001) (Table 6, Figure 4). Quality of life, as measured by SRS-22r, also showed significant improvement throughout the study period: the median score increased from 2.4 (IQR: 2.1–2.6) at baseline to 3.5 (IQR: 3.1–3.8) at 3 months (p < 0.001) and 6 months (p < 0.001), reaching 3.8 (IQR: 3.4–4.0) at 12 months (p < 0.001), indicating a consistent enhancement in patients' overall well-being over time (Table 6, Figure 5).
The most substantial improvements compared to baseline were at the 12-month assessment, however the cohort reached the minimum clinically significant mean improvements in EQ-5D VAS (+12 points), ODI (−10%), and SRS-22r (+0.4 points) by 3 months (19–21). The mean changes in EQ-5D VAS scores at 3, 6, and 12 months were +19.3, +21.5, and +25.8, respectfully. At 3, 6, and 12 months, the mean change in ODI percentage points were −26.9%, −29.3, and −32.0%, respectively. For SRS-22r, the mean score changes at 3, 6, and 12 months were +1.0, +1.1, and +1.3. Overall, from baseline to 12 months there was a 54.9% improvement in EQ-5D VAS, 52.4% in ODI, and 54.2% in SRS-22r.
Safety
No adverse events related to the graft were reported. Only 1 report of mild, procedure-related proximal junctional kyphosis (PJK) was found in an obese 78-year-old white female with chronic kidney and cardiovascular disease.
Discussion
Expanding capabilities in regenerative medicine research and manufacturing have resulted in osteobiologic substitutes that reduce dependence on autologous bone grafts, and therefore effectively lower the risk of related complications (4, 5, 7, 8). The clinical success of these grafts is mainly attributed to the inclusion of osteoconductive, osteoinductive, and/or osteogenic properties that mimic those of autologous bone (5, 12). During bone healing, tissue remodeling and neovascularization are conducted by osteogenic cells and cytokines such as insulin-like growth factors (IGFs), bone morphogenetic proteins (BMPs), platelet-derived growth factor (PDGF), transforming growth factors (TGF-βs), vascular endothelial growth factor (VEGF), and endothelial growth factor (EGF) (22). Thus, bone grafts that preserve viable bone forming cells and a high concentration of these supportive growth factors and cytokines may provide clinical benefit over bone grafts which only provide osteoconductive properties.
Currently in the United States, synthetic scaffolds, bone morphogenetic proteins (BMPs), demineralized bone matrices (DBMs), bone marrow aspirate (BMA) byproducts, and viable bone matrices (VBMs) are available for use. Each osteobiologic has shown promising potential to enhance fusion rates and alleviate pain associated with autologous harvesting in musculoskeletal fusion procedures, however some provide more limited characteristics compared to others which could affect clinical outcomes (23). Synthetic bone graft scaffolds provide consistent structure but have lower reported fusion rates (77% to 90%) when used alone compared to using as an extender with iliac crest autograft (78% to 100%) (24, 25). Demineralized bone matrix (DBM) was designed to enhance fusion with osteoinductive properties, however growth factor concentration variability between individual DBMs and within lots is thought to be responsible for the differing reported rates of fusion (52% to 100%) (23, 26, 27). Bone morphogenetic proteins (BMPs) have high reported fusion rates (93% to 99%) due to strong osteoinductive effects but carry risks such as inflammation and malignancy (23, 28–30). Bone marrow aspirate (BMA) relies on osteogenic cells and growth factors to drive osteogenesis, and depending on which scaffold it is combined with, has similar reported arthrodesis rates to iliac crest autograft (76% to 100%) (31, 32). Currently there is a lack of evidence supporting the utilization of DBM or BMA derivatives as standalone orthobiologics (4). The only class of osteobiologic that incorporates all three required components to support bone healing are VBMs or IBMs (Table 7).
VBMs have shown equivalent or greater clinical success compared to ICBG even in complex cases and with patients with higher risk factors in spinal fusion (33, 34), foot and ankle arthrodesis (35–38), and craniomaxillofacial repairs (39, 40). The ability of advanced grafts to overcome potential healing deficiencies caused by these risk factors could explain the lack of significant differences in fusion rates between short vs. long segment arthrodesis and low vs. high comorbid risk factors in this dataset, however larger studies are needed to confirm these relationships. Recent studies have also suggested that VBMs may be more cost-effective compared to other alternatives such as rhBMP2, in part due to their lower complication rates which potentially can lead to lower healthcare costs over a 2-year period postoperatively (41, 42). In fact, the higher rates of complications from using rhBMP2 could explain the 50% decrease in utilization during thoracolumbar spine fusion surgeries observed between the years 2008–2014 (43). There are many different VBMs commercially available, yet differences exist between products in manufacturing processes which can affect the quantity and quality of cells and growth factors, shelf life, thaw time, use of carrier(s), and time of post-thaw viability (44). Differences in the presence and bioavailability of these factors may explain the differences in clinical fusion success, as reported by others (44, 45). Integrative bone matrix (IBM) includes the same three bone-supportive characteristics as VBM yet provides a greater concentration of native osteoinductive proteins compared to what has been previously reported for demineralized bone (10–12).
As this is the first reporting of results for this application, the purpose of this study was to assess the initial safety and efficacy of a novel IBM in spinal fusion procedures. The use of SPARC IBM was considered safe, as it led to zero related adverse events and circumvented the need for harvesting iliac crest autograft. The only procedure-related complication was proximal junctional kyphosis (PJK) in a patient with obesity, which is a well-known risk factor for PJK (46). Increasing fusion levels also heightens the risk of PJK, nonunion, and mechanical strain, with severe cases requiring revision surgery. However, these outcomes are highly dependent upon differing surgical techniques, patient factors, and follow-up protocols. It is worth noting that the PJK in this study was asymptomatic and did not require any subsequent intervention by 1-year follow up. Efficacy outcomes included radiographic fusion success, which occurred in 100% of interbody and posterolateral levels by 12 months, and subjective clinical evaluations for pain, function, and quality of life which reached the minimum clinically significant mean improvements by 3 months and was maintained through 12 months of follow up.
Previously published VBM spinal fusion rates and pain, disability, and quality of life score improvements align with those reported in this IBM study. A recent systematic review including VBM studies published through January 2023 reported lumbar segment fusion rates of 68% – 99% at 12 months (9). However, the reported rate of reoperation (up to 8.7% in one study with a similar number of enrolled subjects) (47) is significantly higher than our observed rate of 0%. Only one VBM study was identified that included similar subjective outcome measures (48), reporting a 57.1% improvement in mean ODI scores at 12 months, similar to the improvements reported in this study (52.4%).
This innovative IBM combines cancellous chips and cortical DBM fibers to optimize both handling and osteoconduction, and is processed using a gentle manufacturing protocol that preserves viable osteoprogenitor cells with viability for up to 5 h post-thaw, per the IFU. Mitigation of the risk of infection and disease transmission are due to advanced screening of donors and aseptic processing methods. Unlike what has been previously reported for conventional DBM grafts, the IBM retains greater levels of native growth factors extracted from the bone lining cells (Figure 6) (10–12). These include a diverse array of bioavailable osteoinductive, angiogenic, and mitogenic growth factors such as: BMPs, PDGF, TGF-β3, VEGF, and EGF. Unlike VBMs, the IBM thaws quickly in approximately 10 min in room temperature sterile water or saline and is dimethyl sulfoxide (DMSO)-free, which eliminates the need to rinse and decant prior to implantation. The graft comes in a surgeon-friendly ready-to-use syringe.
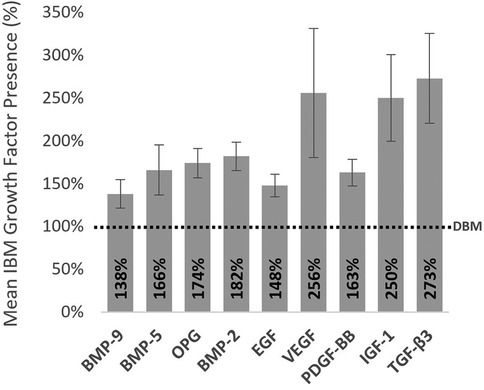
Figure 6. Advanced proprietary processing resulted in heightened growth factor preservation and bioavailability for the IBM compared to demineralized bone matrix (DBM) concentrations. These preserved growth factors can promote osteoinduction, angiogenesis, and cellular proliferation. Values were assessed from donor matched samples across 8 donors (lots). Proteins were extracted from bone samples using standard guanidine hydrochloride protein extraction methods, then each target protein was quantified via a commercially available custom multiplex fluorescent ELISA. *Dashed line represents normalized level of growth factors measured from a common DBM.
These favorable radiographic fusion results combined with early improvements in pain, function, and quality of life highlight the potential of this novel IBM as a promising bone graft extender and substitute in spinal fusion procedures. Aside from its retrospective nature, limitations for this study include lack of a control group, a small cohort size, short-term follow up, use of local autograft alongside the IBM, radiographic evaluation by the operative surgeon, lack of CT fusion evaluation for all subjects, and use of a Ti cage which may have contributed to challenges in fusion determination. Larger, controlled studies with longer-term follow-up are needed to further validate these results.
Data availability statement
The raw data supporting the conclusions of this article will be made available by the authors, without undue reservation.
Ethics statement
The studies involving humans were approved by New York University Institutional Review Board. The studies were conducted in accordance with the local legislation and institutional requirements. The Ethics Committee/institutional review board waived the requirement of written informed consent for participation from the participants or the participants' legal guardians/next of kin because of the retrospective nature of the work performed.
Author contributions
PP: Conceptualization, Data curation, Funding acquisition, Investigation, Methodology, Supervision, Writing – original draft, Writing – review & editing, Formal Analysis, Project administration, Validation. OO: Formal Analysis, Methodology, Project administration, Validation, Writing – review & editing. AD: Formal Analysis, Methodology, Project administration, Validation, Writing – review & editing. JM: Formal Analysis, Methodology, Project administration, Validation, Writing – review & editing. AY: Data curation, Formal Analysis, Project administration, Validation, Writing – review & editing. MF: Data curation, Formal Analysis, Project administration, Validation, Writing – review & editing.
Funding
The author(s) declare financial support was received for the research, authorship, and/or publication of this article. The authors declare that this study received funding from ISTO Biologics, Inc. The funder had the following involvement with the study: study design, data collection and analysis, and supported preparation of the manuscript.
Acknowledgments
The authors would like to thank TruSci, LLC for their medical writing and editorial work, and Kristina Chapple, PhD for her biostatistical expertise.
Conflict of interest
PGP reports that they are a consultant for ISTO Biologics, Inc. (manufacturer of SPARC IBM).
The remaining authors declare that the research was conducted in the absence of any commercial or financial relationships that could be construed as a potential conflict of interest.
Publisher's note
All claims expressed in this article are solely those of the authors and do not necessarily represent those of their affiliated organizations, or those of the publisher, the editors and the reviewers. Any product that may be evaluated in this article, or claim that may be made by its manufacturer, is not guaranteed or endorsed by the publisher.
References
1. Rajaee SS, Bae HW, Kanim LEA, Delamarter RB. Spinal fusion in the United States: analysis of trends from 1998 to 2008. Spine (Phila Pa 1976). (2012) 37(1):67–76. doi: 10.1097/BRS.0b013e31820cccfb
2. Martin BI, Mirza SK, Spina N, Spiker WR, Lawrence B, Brodke DS. Trends in lumbar fusion procedure rates and associated hospital costs for degenerative spinal diseases in the United States, 2004 to 2015. Spine (Phila Pa 1976). (2019) 44(5):369–76. doi: 10.1097/BRS.0000000000002822
3. Deng H, Yue JK, Ordaz A, Suen catherine G, Sing DC. Elective lumbar fusion in the United States: national trends in inpatient complications and cost from 2002 to 2014. J Neurosurg Sci. (2021) 65(5):503–12. doi: 10.23736/S0390-5616.19.04647-2
4. Khan SN, Shahzad H. Osteobiologics and value-based care: challenges and opportunities. Int J Spine Surg. (2023) 17(S3):S44–52. doi: 10.14444/8560
5. Tavares WM, de França SA, Paiva WS, Teixeira MJ. A systematic review and meta-analysis of fusion rate enhancements and bone graft options for spine surgery. Sci Rep. (2022) 12(1):7546. doi: 10.1038/s41598-022-11551-8
6. Park DK, Roberts R, Arnold P, Kim DH, Sasso R, Baker KC, et al. Lumbar spine fusion rates with local bone in posterolateral and combined posterolateral and interbody approaches. J Am Acad Orthop Surg Glob Res Rev. (2019) 3(11):e018. doi: 10.5435/JAAOSGlobal-D-18-00018
7. Roberts TT, Rosenbaum AJ. Bone grafts, bone substitutes and orthobiologics: the bridge between basic science and clinical advancements in fracture healing. Organogenesis. (2012) 8(4):114–24. doi: 10.4161/org.23306
8. Hsieh PC, Buser Z, Skelly AC, Brodt ED, Brodke D, Meisel HJ, et al. Allogenic stem cells in spinal fusion: a systematic review. Global Spine J. (2019) 9(1 Suppl):22S–38. doi: 10.1177/2192568219833336
9. Lambrechts MJ, Issa TZ, Mazmudar A, Lee Y, Toci GR, D’Antonio ND, et al. Cellular bone matrix in spine surgery—are they worth the risk: a systematic review. Global Spine J. (2024) 14(3):1070–81. doi: 10.1177/21925682231205099
10. Martin GJ, Boden SD, Titus L, Scarborough NL. New formulations of demineralized bone matrix as a more effective graft alternative in experimental posterolateral lumbar spine arthrodesis. Spine (Phila Pa 1976). (1999) 24(7):637–45. doi: 10.1097/00007632-199904010-00005
11. Wildemann B, Kadow-Romacker A, Haas NP, Schmidmaier G. Quantification of various growth factors in different demineralized bone matrix preparations. J Biomed Mater Res A. (2007) 81(2):437–42. doi: 10.1002/jbm.a.31085
12. Blum B, Moseley J, Miller L, Richelsoph K, Haggard W. Measurement of bone morphogenetic proteins and other growth factors in demineralized bone matrix. Orthopedics. (2004) 27(1 Suppl):s161–5. doi: 10.3928/0147-7447-20040102-17
13. Bridwell KH, Lenke LG, McEnery KW, Baldus C, Blanke K. Anterior fresh frozen structural allografts in the thoracic and lumbar spine. Do they work if combined with posterior fusion and instrumentation in adult patients with kyphosis or anterior column defects? Spine (Phila Pa 1976). (1995) 20(12):1410–8. doi: 10.1097/00007632-199506020-00014
14. Glassman SD, Dimar JR, Carreon LY, Campbell MJ, Puno RM, Johnson JR. Initial fusion rates with recombinant human bone morphogenetic protein-2/compression resistant matrix and a hydroxyapatite and tricalcium phosphate/collagen carrier in posterolateral spinal fusion. Spine (Phila Pa 1976). (2005) 30(15):1694–8. doi: 10.1097/01.brs.0000172157.39513.80
15. Kim SY, Park KS, Jung SS, Chung SY, Kim SM, Park MS, et al. An early comparative analysis of the use of autograft versus allograft in anterior cervical discectomy and fusion. Korean J Spine. (2012) 9(3):142. doi: 10.14245/kjs.2012.9.3.142
16. Rabin R, De Charro F. EQ-5D: a measure of health status from the EuroQol group. Ann Med. (2001) 33(5):337–43. doi: 10.3109/07853890109002087
17. Fairbank JCT, Davies JB, Couper J, O’Brien JP. The oswestry low back pain disability questionnaire. Physiotherapy. (1980) 66(8):271–3.6450426
18. Asher MA, Lai SM, Glattes RC, Burton DC, Alanay A, Bago J. Refinement of the SRS-22 health-related quality of life questionnaire function domain. Spine (Phila Pa 1976). (2006) 31(5):593–7. doi: 10.1097/01.brs.0000201331.50597.ea
19. Joelson A, Nerelius F, Sigmundsson FG, Karlsson J. The minimal important change for the EQ VAS based on the SF-36 health transition item: observations from 25772 spine surgery procedures. Qual Life Res. (2022) 31(12):3459. doi: 10.1007/s11136-022-03182-3
20. Hägg O, Fritzell P, Nordwall A. The clinical importance of changes in outcome scores after treatment for chronic low back pain. Eur Spine J. (2003) 12(1):12–20. doi: 10.1007/s00586-002-0464-0
21. Crawford CH, Glassman SD, Bridwell KH, Berven SH, Carreon LY. The minimum clinically important difference in SRS-22R total score, appearance, activity and pain domains after surgical treatment of adult spinal deformity. Spine (Phila Pa 1976). (2015) 40(6):377–81. doi: 10.1097/BRS.0000000000000761
22. Oliveira ÉR, Nie L, Podstawczyk D, Allahbakhsh A, Ratnayake J, Brasil DL, et al. Advances in growth factor delivery for bone tissue engineering. Int J Mol Sci. (2021) 22(2):1–33. doi: 10.3390/ijms22020903
23. D’Souza M, Macdonald NA, Gendreau JL, Duddleston PJ, Feng AY, Ho AL. Graft materials and biologics for spinal interbody fusion. Biomedicines. (2019) 7(4):75. doi: 10.3390/biomedicines7040075
24. Buser Z, Brodke DS, Youssef JA, Meisel HJ, Myhre SL, Hashimoto R, et al. Synthetic bone graft versus autograft or allograft for spinal fusion: a systematic review. J Neurosurg Spine. (2016) 25(4):509–16. doi: 10.3171/2016.1.SPINE151005
25. Nickoli MS, Hsu WK. Ceramic-based bone grafts as a bone grafts extender for lumbar spine arthrodesis: a systematic review. Global Spine J. (2014) 4(3):211–6. doi: 10.1055/s-0034-1378141
26. Cammisa FP, Lowery G, Garfin SR, Geisler FH, Klara PM, McGuire RA, et al. Two-Year fusion rate equivalency between grafton® DBM gel and autograft in posterolateral spine fusion. Spine (Phila Pa 1976). (2004) 29(6):660–6. doi: 10.1097/01.BRS.0000116588.17129.B9
27. Buser Z, Brodke DS, Youssef JA, Rometsch E, Park JB, Yoon ST, et al. Allograft versus demineralized bone matrix in instrumented and noninstrumented lumbar fusion: a systematic review. Global Spine J. (2018) 8(4):396–412. doi: 10.1177/2192568217735342
28. Simmonds MC, Brown JVE, Heirs MK, Higgins JPT, Mannion RJ, Rodgers MA, et al. Safety and effectiveness of recombinant human bone morphogenetic protein-2 for spinal fusion. Ann Intern Med. (2013) 158(12):877. doi: 10.7326/0003-4819-158-12-201306180-00005
29. Fu R, Selph S, McDonagh M, Peterson K, Tiwari A, Chou R, et al. Effectiveness and Harms of recombinant human bone morphogenetic protein-2 in spine fusion. Ann Intern Med. (2013) 158(12):890. doi: 10.7326/0003-4819-158-12-201306180-00006
30. Galimberti F, Lubelski D, Healy AT, Wang T, Abdullah KG, Nowacki AS, et al. A systematic review of lumbar fusion rates with and without the use of rhBMP-2. Spine (Phila Pa 1976). (2015) 40(14):1132–9. doi: 10.1097/BRS.0000000000000971
31. Kitchel SH. A preliminary comparative study of radiographic results using mineralized collagen and bone marrow aspirate versus autologous bone in the same patients undergoing posterior lumbar interbody fusion with instrumented posterolateral lumbar fusion. Spine J. (2006) 6(4):405–11. doi: 10.1016/j.spinee.2005.09.013
32. Noh T, Zakaria H, Massie L, Ogasawara CT, Lee GA, Chedid M. Bone marrow aspirate in spine surgery: case series and review of the literature. Cureus. (2021) 13(12):e20309. doi: 10.7759/cureus.20309
33. Hall JF, McLean JB, Jones SM, Moore MA, Nicholson MD, Dorsch KA. Multilevel instrumented posterolateral lumbar spine fusion with an allogeneic cellular bone graft. J Orthop Surg Res. (2019) 14(1):372. doi: 10.1186/s13018-019-1424-2
34. Elgafy H, Wetzell B, Gillette M, Semaan H, Rowland A, Balboa CA, et al. Lumbar spine fusion outcomes using a cellular bone allograft with lineage-committed bone-forming cells in 96 patients. BMC Musculoskelet Disord. (2021) 22(1):699. doi: 10.1186/s12891-021-04584-z
35. Moran TE, Sequeira S, Cooper MT, Park J. A retrospective analysis of outcomes from foot and ankle arthrodesis and open reduction and internal fixation using cellular bone allograft augmentation. Foot Ankle Spec. (2022) 15(4):312–20. doi: 10.1177/1938640020952301
36. Roukis TS, Samsell B. A new approach to ankle and foot arthrodesis procedures using a living cellular bone matrix: a case series. Clin Res Foot Ankle. (2018) 6(3):1–6. doi: 10.4172/2329-910X.1000274
37. Roukis TS. Use of living cellular bone matrix for treating a failed ankle arthroplasty: an abbreviated technique and case study. Clin Res Foot Ankle. (2018) 6(4):1–6. doi: 10.4172/2329-910X.1000282
38. Donaghue P, Gokcen E. Radiographical outcomes of a cellular based allograft following foot/ankle arthrodesis in patients with risk for non-union. Orthop Rev (Pavia). (2024) 16:115603. doi: 10.52965/001c.115603
39. Alfi DM, Hassan A, East SM, Gianulis EC. Immediate mandibular reconstruction using a cellular bone allograft following tumor resection in a pediatric patient. Face. (2021) 2(4):490–5. doi: 10.1177/27325016211057287
40. Marschall JS, Kushner GM, Flint RL, Jones LC, Alpert B. Immediate reconstruction of segmental mandibular defects with nonvascular bone grafts: a 30-year perspective. J Oral Maxillofac Surg. (2020) 78(11):2099.e1–.e9. doi: 10.1016/j.joms.2020.03.035
41. Wetzell B, McLean JB, Moore MA, Kondragunta V, Dorsch K. A large database study of hospitalization charges and follow-up re-admissions in US lumbar fusion surgeries using a cellular bone allograft (CBA) versus recombinant human bone morphogenetic protein-2 (rhBMP-2). J Orthop Surg Res. (2020) 15(1):544. doi: 10.1186/s13018-020-02078-7
42. Wetzell B, McLean JB, Dorsch K, Moore MA. A 24-month retrospective update: follow-up hospitalization charges and readmissions in US lumbar fusion surgeries using a cellular bone allograft (CBA) versus recombinant human bone morphogenetic protein-2 (rhBMP-2). J Orthop Surg Res. (2021) 16(1):680. doi: 10.1186/s13018-021-02829-0
43. Beschloss AM, Dicindio CM, Lombardi JS, Shillingford JN, Laratta JL, Holderread B, et al. The rise and fall of bone morphogenetic protein 2 throughout the United States. Clin Spine Surg. (2022) 35(6):264–9. doi: 10.1097/BSD.0000000000001301
44. Venuti N, Mohan V. Spinal fusion with mesenchymal stem cell bone matrices: a review on product comparison. Spine Research. (2019) 5(1):2. doi: 10.21767/2471-8173.100047
45. Lin C, Zhang N, Waldorff EI, Punsalan P, Wang D, Semler E, et al. Comparing cellular bone matrices for posterolateral spinal fusion in a rat model. JOR Spine. (2020) 3(2):e1084. doi: 10.1002/jsp2.1084
46. Han X, Ren J. Risk factors for proximal junctional kyphosis in adult spinal deformity after correction surgery: a systematic review and meta-analysis. Acta Orthop Traumatol Turc. (2022) 56(3):158. doi: 10.5152/j.aott.2022.21255
47. Ammerman JM, Libricz J, Ammerman MD. The role of osteocel plus as a fusion substrate in minimally invasive instrumented transforaminal lumbar interbody fusion. Clin Neurol Neurosurg. (2013) 115(7):991–4. doi: 10.1016/j.clineuro.2012.10.013
48. Lee DD, Kim JY. A comparison of radiographic and clinical outcomes of anterior lumbar interbody fusion performed with either a cellular bone allograft containing multipotent adult progenitor cells or recombinant human bone morphogenetic protein-2. J Orthop Surg Res. (2017) 12(1):126. doi: 10.1186/s13018-017-0618-8
Keywords: spinal fusion, bone graft, spine surgery, biologics, regenerative medicine, integrative bone matrix, IBM
Citation: Passias PG, Onafowokan OO, Das A, Mir JM, Yung A and Fisher M (2025) An innovative advance in bone grafting: initial clinical results using a novel integrative bone graft in spinal fusion. Front. Musculoskelet. Disord. 2:1459266. doi: 10.3389/fmscd.2024.1459266
Received: 3 July 2024; Accepted: 17 December 2024;
Published: 6 January 2025.
Edited by:
Ashim Gupta, Future Biologics, United StatesReviewed by:
Hiroshi Noguchi, University of Tsukuba, JapanNathan Kucko, Kuros Biosciences, Netherlands
John Von Benecke, Locate Bio, United Kingdom
Copyright: © 2025 Passias, Onafowokan, Das, Mir, Yung and Fisher. This is an open-access article distributed under the terms of the Creative Commons Attribution License (CC BY). The use, distribution or reproduction in other forums is permitted, provided the original author(s) and the copyright owner(s) are credited and that the original publication in this journal is cited, in accordance with accepted academic practice. No use, distribution or reproduction is permitted which does not comply with these terms.
*Correspondence: Peter G. Passias, cGV0ZXIucGFzc2lhc0BkdWtlLmVkdQ==