- 1Postgraduate Program in Microbiology, Department of Pathology, Federal University of Paraná (UFPR), Centro Politécnico, Curitiba, Paraná, Brazil
- 2Center for Pharmaceutical Research and Innovation, College of Pharmacy, University of Kentucky, Lexington, KY, United States
- 3Department of Pharmaceutical Sciences, College of Pharmacy, University of Kentucky, Lexington, KY, United States
- 4Postgraduate Program in Genetics, Department of Genetics, Federal University of Paraná (UFPR), Centro Politécnico, Curitiba, Paraná, Brazil
Introduction: The exploration of new bioactive compounds for agricultural applications is critical for sustainable development. Endophytic fungi, particularly those from underexplored biomes in Brazil, represent a promising source of natural compounds. This study focused on isolation and bioprospecting endophytic fungi from the medicinal plant Vochysia divergens (Pohl), grown in Serra do Amolar (Brazilian Pantanal Biome), with an additional emphasis on conserving microbial biodiversity.
Methods and results: Leaves and petioles were collected from 18 V. divergens specimens, from which 293 endophytes were isolated and grouped by morphological characteristics into 91 phenotypes. One representative of each phenotype was selected for secondary metabolite extraction and taxonomic identification. Fungi belonging to 27 families and 32 different genera were identified, with Diaporthe, Phyllosticta, and Pseudofusicoccum as the most predominant. We also introduce and describe a new endophytic species, Diaporthe amolarensis. Multiple extracts inhibited mycelial growth of the phytopathogenic fungus Colletotrichum abscissum, with a superior effect compared to the fungicide control. These extracts were produced by Diaporthe amolarensis, Xylaria arbuscula, and Nemania primolutea. Additionally, the extract from one X. arbuscula isolate displayed moderate activity against the phytopathogen Phyllosticta citricarpa. HPLC-UV and HPLC-MS analyses of these most inhibitory extracts revealed natural products with beneficial potential that need characterization and to have their modes of action elucidated.
Discussion: Finally, a very important contribution of this study was the ex situ conservation of the biodiversity of the Serra do Amolar, allowing future studies and biotechnological applications involving endophytes from this region.
1 Introduction
One of the biggest challenges facing global agriculture is the control of diseases caused by pathogenic microorganisms. Their rapid dissemination capacity and ability to affect crop production have a severe impact on national agriculture, leading to significant economic losses (Fontes and Valadares-Inglis, 2020). Phytosanitary management involves various practices, with the application of chemical pesticides being one of them. Despite the increase in pesticide use over the recent decades, this has not resulted in a proportional reduction in crop losses (Tan et al., 2018; Sang and Kim, 2020). The large-scale use of pesticides and fungicides has led to its own sets of problems, including the resistance of some pathogenic microorganisms (Morandi et al., 2009; Braga et al., 2022; Torres-Rodriguez et al., 2022). Among these harmful pathogens of most concern are fungi from several genera, such as Colletotrichum (Lima et al., 2011; Silva et al., 2017), Phyllosticta (Baldassari et al., 2006; Tonial et al., 2017), and Fusarium (Brown et al., 2012; Paccanaro et al., 2017). Therefore, species of these genera were used as targets for testing new compounds with activity against phytopathogens.
Considering the risk of pathogen resistance to commonly used fungicides, it has become increasingly necessary to control these pathogens and their negative impacts with new chemical classes that have modes of action different from existing fungicides (Javed et al., 2021; Afzal et al., 2023). Biotechnological advances improve biosynthetic processes, including the production of bioactive compounds of diverse natural substances that can significantly support human, animal, and plant health (Faria et al., 2023). Such compounds, derived from plants, animals, and microorganisms, are gaining considerable attention (Rafiq et al., 2024). Our research group has been dedicated to the prospection and discovery of secondary metabolites (SMs) produced by endophytic microorganisms of medicinal plants from underexplored Brazilian biomes (Savi et al., 2015; Gos et al., 2017; Noriler et al., 2018; Savi et al., 2018; Iantas et al., 2021; Glienke et al., 2024). Endophytes are a dependable source of natural products, as they colonize the internal tissues of plants without causing damage to their host, an interaction based on the exchange of signals and compounds (Petrini et al., 1993; Araújo et al., 2014). In this symbiotic relationship, the endophytes receive protection and nutrients and in return produce SMs that protect the host by increasing its resistance to pathogens (Savi D. et al., 2019).
Brazil is a country with enormous biodiversity, comprising six different biomes, some of which are understudied. One of them is the Pantanal (Glienke et al., 2024), the largest continuous wetland in the world, located in the states of Mato Grosso and Mato Grosso do Sul (in addition to Bolivia and Paraguay), experiencing drastic seasonal fluctuations, with alternating periods of floods and droughts. This biome has an enormous richness of species, and its diversity is strongly related to the seasonality of the region, offering the potential to discover an abundance of natural products. The plant Vochysia divergens (Cambará) belongs to a group of approximately 5% of the tree species of the Pantanal biome that is capable of surviving in regions long flooded and therefore became dominant in that area. In addition, it has medicinal properties and is used in teas and syrups for the treatment of colds, coughs, pneumonia, and gastrointestinal diseases (Arieira and Da Cunha, 2006).
The Serra do Amolar, an environmental preservation area, managed by the Serra do Amolar Protection and Conservation Network, in the Pantanal biome, is located in the north of the state of Mato Grosso do Sul, on the border with the Mato Grosso state and Bolivia. It is one of the largest biological heritage sites in Brazil. Our research group has been studying endophytes from medicinal plants located in other regions of the Pantanal and found several new endophytic natural products (Savi et al., 2015; Gos et al., 2017; Noriler et al., 2018; Savi et al., 2018; Iantas et al., 2021). Because the Serra do Amolar region is difficult to access and consequently little studied, it is much more native than other areas of the Pantanal (Fundação Ecotrópica, 2003; Moreira, 2011). Our hypothesis is that the plants in this region harbor a greater diversity of endophytes with greater potential to produce undiscovered compounds with beneficial properties. Additionally, this region has been recently suffering from wildfires, placing its biodiversity at risk (Pessi et al., 2023). Therefore, this study aimed to extend our knowledge of endophytic fungi present in the Pantanal Biome through bioprospecting the medicinal plant Vochysia divergens from the Serra do Amolar. To evaluate its potential for producing SMs with biological activities, we isolated and identified the endophytic community, extracted SMs, and tested them against three phytopathogens. This study contributes not only to discover new SMs with biotechnological applications but also to explore the diversity of endophytes present in this underexplored region and, in addition, conducts an ex situ conservation of the isolates.
2 Materials and methods
2.1 Sampling of plant material
Sampling of plant material occurred in February 2019 with assistance from the Serra do Amolar Institute.1 We sampled the leaves and petioles of 18 V. divergens trees along a section of the Paraguay River (Figure 1; Supplementary Table S1) within the Serra do Amolar. The collected samples were stored in plastic bags and kept under refrigeration until processing.
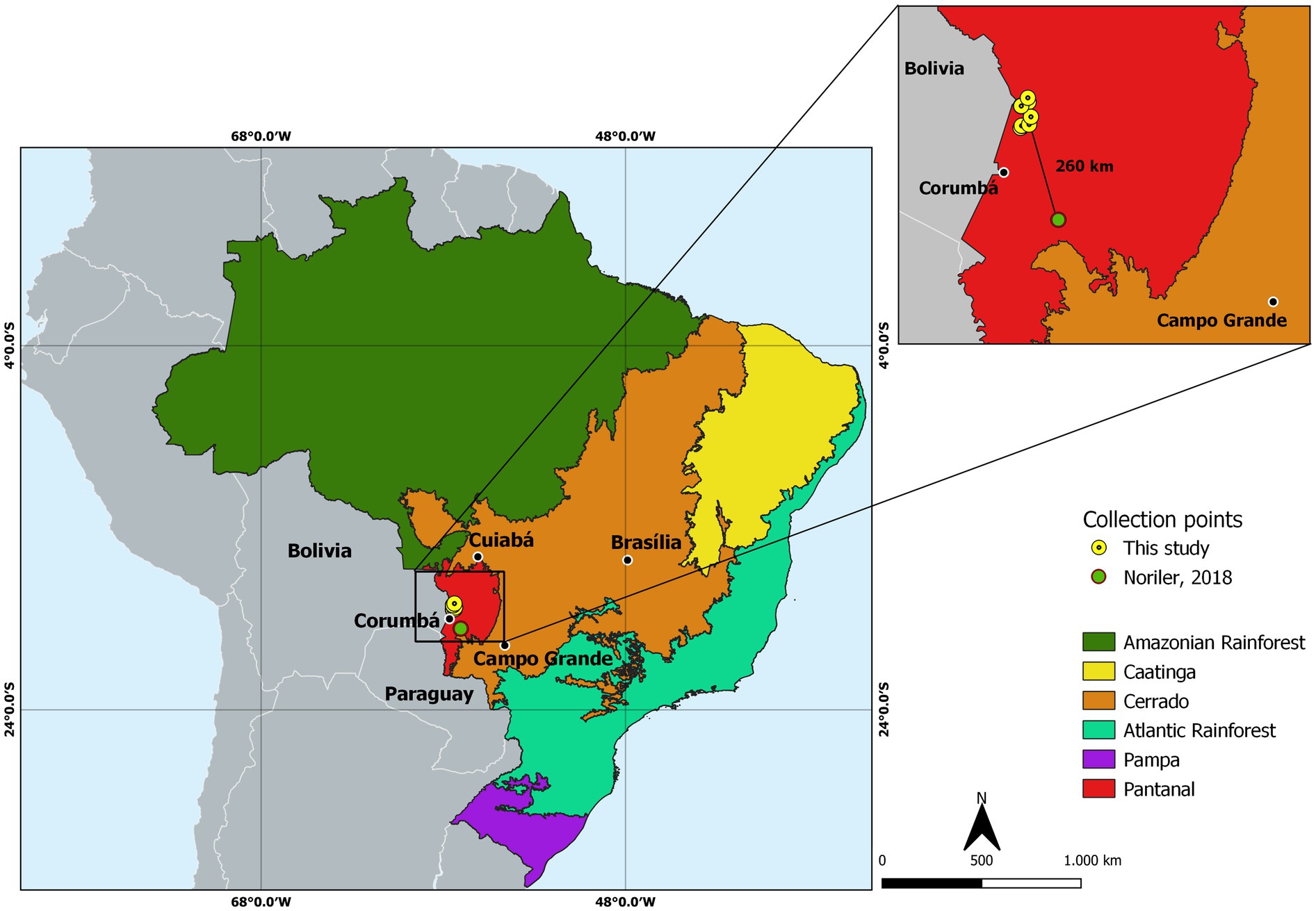
Figure 1. Map of Brazil showing the division by biomes and the sample collection place. The magnified box shows the points of collection of leaves and petioles of Vochysia divergens used in this study (yellow dots) in contrast to the point of collection of leaves and petioles of the same plant in the previous study carried out by Noriler et al. (2018) (red dot) with approximately 260 km of distance between them. Source: The authors.
2.2 Isolation of endophytes
After collection, five leaves and five petioles from each plant without marks or injuries were used to isolate endophytic fungi. To eliminate the epiphytic microorganisms, a surface disinfection protocol was performed as described by Petrini (1986) and adapted by Noriler et al. (2018). Then, the leaves and petioles were fragmented into five pieces (8-mm squares), each of which was placed onto Petri dishes containing potato dextrose agar (PDA), pH 5.8, with the addition of tetracycline (50 μg/mL) to inhibit bacterial growth. The plates were incubated at 28°C for up to 30 days, and fungal growth was checked daily. The emerging mycelia were transferred to fresh PDA plates at pH 5.8 and stored for later use.
The isolates were grouped according to their macromorphological characteristics, such as colony color, hyphal texture, and growth rate. A pure culture of each representative phenotype was generated, using the single spore culture method according to Gilchrist-Saavedra et al. (2006). The pure cultures were used for molecular identification and bioprospecting and were all deposited in the Coleções Microbiológicas da Rede Paranaense (CMRP) culture collection2 at the Federal University of Paraná, Brazil. The access to genetic heritage isolated in this study was registered in SISGEN (National System for the Management of Genetic Heritage and Associated Traditional Knowledge) under number A74A5EE in compliance with Brazilian Law 13.123/2015, and the Nagoya Protocol. The collection of biological material used in this study was authorized by the Instituto Chico Mendes de Conservação da Biodiversidade (ICMBio), according to authorization SISBIO (Biodiversity Authorization and Information System) No. 85130.
2.3 Identification of endophytes
Genomic DNA was extracted from mycelia following previously published protocols (Raeder and Broda, 1985; Glienke, 1999). Subsequently, partial regions of six loci were amplified according to the specificities of each genus and species. The list of primers used for each amplification is shown in Table 1.
The PCRs were performed for a final volume of 12.5 μL (1X reaction buffer, 0.2 μM of forward primer, 0.2 μM of reverse primer, 1.5 mM of MgCl2, 0.2 mM of dNTPs, and 0.05 U/μL of Taq Polymerase). The PCR conditions used for each gene and taxonomic group are listed in Table 2. The PCR products were purified using the enzymes Exo1 and FastAP (GE Healthcare, USA), and the BigDye® Terminator Kit v3.1 was used for the sequencing reaction. The product of this reaction was purified by gel filtration (Sephadex G50), and the sequencing was determined with an automatic sequencer (ABI3500®, Applied Biosystems, Foster City, CA, USA).
The obtained chromatograms were inspected using MEGA X (Tamura et al., 2011) and BioEdit (Hall, 1999). The sequences were compared with those available in the NCBI/GenBank database3 using the BLAST Tool and compared with type strains obtained from the MycoBank4 and Westerdijk Fungal Biodiversity Institute5 databases (Supplementary Tables S2–S4, S11–S49). Phylogenetic analyses were performed with the sequences that correspond to the type or authentic strains and those generated by this study. The alignments of the DNA sequences were made using Mafft software (Katoh and Toh, 2008)6 and verified manually with MEGA X software. Bayesian inference analysis was inferred using MrBayes v3.2.6 ×86 (Ronquist et al., 2012) via CIPRES Science Gateway (Miller et al., 2011). This analysis was performed using two parallel runs with one cold and three heated chains each, using the number of generations needed to split frequencies ≤0.01 and a sampling frequency set to every 100 generations. The posterior probability values were calculated after discarding the first 25% of the generated trees as burn-in. The resulting trees are plotted in FigTree v.1.4.2.7 The substitution modes were selected for each gene using JModelTest (Darriba et al., 2012). All sequences obtained were deposited at GenBank, and the access codes are listed in Supplementary Table S5.
2.4 Morphological characterization/taxonomy
The descriptions provided here were based on macromorphological characteristics in different culture media and micromorphological characteristics from sporulated colonies, which formed asexual structures. These observations were conducted for the isolates to which the new species descriptions apply. For the isolate CMRP4997, the following culture conditions were used: PDA pH 5.5, oatmeal agar (OA), and 2% malt extract (MEA) with and without the addition of sterile pine needles and autoclaved leaves of Schinus terebinthifolius, incubated at 25°C over a period of 12 h of light and 12 h of darkness as described by Gomes et al. (2013). Colony diameter measurements were determined at 25°C in the dark in PDA, pH 5.5, OA, and MEA media, with measurements performed 3, 4, 5, and 7 days after inoculation. After 15 days, colony colors were described (verse and reverse) using color charts of Ranyer (1970). Alpha and beta conidia were measured to calculate the mean size and standard deviation using ImageJ software. The micromorphological characteristics were examined and captured using a BX51 Olympus microscope equipped with an SC30 camera from the microscopy center (Centro de Tecnologias Avançadas em Microscopia—UFPR).
2.5 Extraction and evaluation of endophyte SMs
2.5.1 SM extractions
Endophytic isolates representing each phenotype were selected for small-scale fermentations in liquid medium and extract production. The isolates were cultured for 7 days in PDA at pH 5.8 and 28°C. Three mycelial discs (6 mm) were added into Erlenmeyer flasks (250 mL) containing 100 mL of liquid malt extract medium (Schulz et al., 2002) and incubated under constant agitation (180 rpm) for 10 days at 28°C. The cultures were filtered using Whatman no. 4 filter paper to remove the mycelium, and the remaining broth was mixed with 4% of Amberlite® XAD-16 polymer and kept overnight under constant agitation (180 rpm), followed by centrifugation. The resin was washed three times with distilled water and extracted three times with methanol (MeOH). The solvent was evaporated in vacuo at 45°C to obtain the dry crude extract and then diluted in MeOH to a final concentration of 10 mg/mL (Iantas et al., 2021).
2.5.2 SM mycelial growth inhibition of pathogenic Fungi
The phytopathogenic fungus C. abscissum CMRP704 was used for the initial screening of the antifungal activity of the crude extracts. The experiment was carried out with 100 μL of each extract (10 mg/mL) added to a Petri dish containing PDA pH 5.8 and spread using a Drigalski spatula, followed by a pathogen mycelial disc (6 mm) placed in the center of the plate. The fungicide Carbendazim (Derosal®; 1.0 mg/mL) and pure methanol were used as positive and negative controls, respectively. The plates were incubated at 24°C for 7 days, and the diameter of the colonies was measured and compared with that of both control plates (Savi, 2011). The percentage of mycelial growth inhibition was calculated with the following formula: Pi = (Cd – Td)/Cd, where Pi = percent inhibition; Cd = control growth diameter; Td = treatment growth diameter. The experiments were carried out in triplicate.
The extracts that presented mycelial growth inhibition higher than the positive control (fungicide Carbendazim) in the previous analysis with C. abscissum were also evaluated against the phytopathogens F. graminearum LGMF1703 and P. citricarpa CMRP06 (Glienke et al., 2011), using the same methodology, with the following modifications: (1) the incubation temperature used for F. graminearum was 28°C, (2) the growth inhibition rates were analyzed at 4 and 21 days after inoculation for F. graminearum and P. citricarpa, respectively. The experiments were performed in triplicate.
2.5.3 Chemical analyses of SMs
To identify the potential compounds in the prioritized bioactive fungal extracts, we conducted chromatography analyses using HPLC-UV/MS. HPLC-UV/MS analyses were accomplished with an Agilent InfinityLab LC/MSD mass spectrometer (MS Model G6125B; Agilent Technologies, Santa Clara, CA, USA) equipped with an Agilent 1260 Infinity II Series Quaternary LC system and a Phenomenex NX-C18 column (250 × 4.6 mm, 5 μm) [method: solvent A: H2O/0.1% formic acid, solvent B: CH3CN; flow rate: 0.5 mL min−1; 0–30 min, 5–100% B (linear gradient); 30–35 min, 100% B; 35–36 min, 100–5% B; 36–40 min, 5% B]. All solvents used were of ACS grade and purchased from Pharmco-AAPER (Brookfield, CT). A549, PC3, and HEL299 cells were obtained from ATCC (Manassas, VA). All other reagents used were reagent grade and purchased from Sigma-Aldrich (Saint Louis, MO).
2.5.4 Cytotoxicity assays for SM extracts
The cytotoxicity of crude extracts was evaluated against A549 (non-small-cell lung carcinoma), PC3 (prostate adenocarcinoma), and HEL299 (normal lung fibroblast) human cell lines. The assays were accomplished in triplicate following our previously reported protocols (Savi et al., 2018; Shaaban et al., 2013, 2015; Wang et al., 2013) actinomycin D (A549, PC3, and HEL299) was used as a positive control.
3 Results
3.1 Endophyte diversity observed with Vochysia divergens from the Serra do Amolar
A total of 293 cultivable endophytic fungi were isolated from leaf and petiole fragments collected from 18 trees of V. divergens. The isolates were grouped into 91 phenotypes according to their morphological characteristics (Supplementary Figure S1). A representative isolate of each phenotype was identified at the genus and/or species level using phylogenetic analyses (Figures 2–4; Supplementary Figures S2–S36).
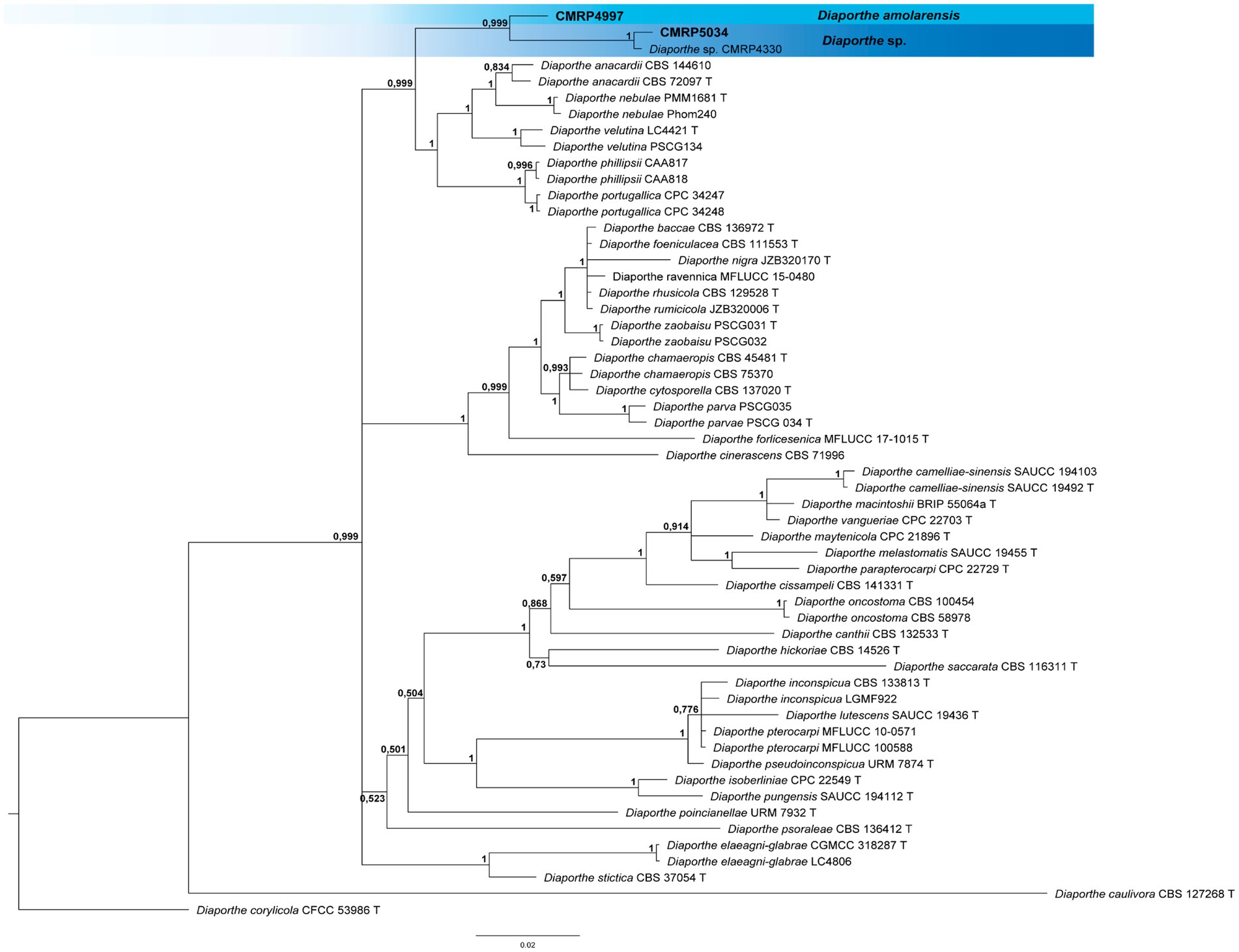
Figure 2. Bayesian inference phylogenetic tree of Diaporthe oncostoma species complex based on multiple alignments of tef1, tub2, and his3 partial sequences. The data matrix had 56 taxa and 1750 characters. The species Diaporthe corylicola (CFCC 53986) was used as an outgroup. Strains marked with a “T” correspond to type sequences. Bayesian posterior probabilities equal to or greater than 0.50 are presented next to each node. The scale bar of 0.02 represents the number of changes. The sequence of the isolates here studied is presented with their isolation codes (CMRP4997 and CMRP5034) highlighted in bold.
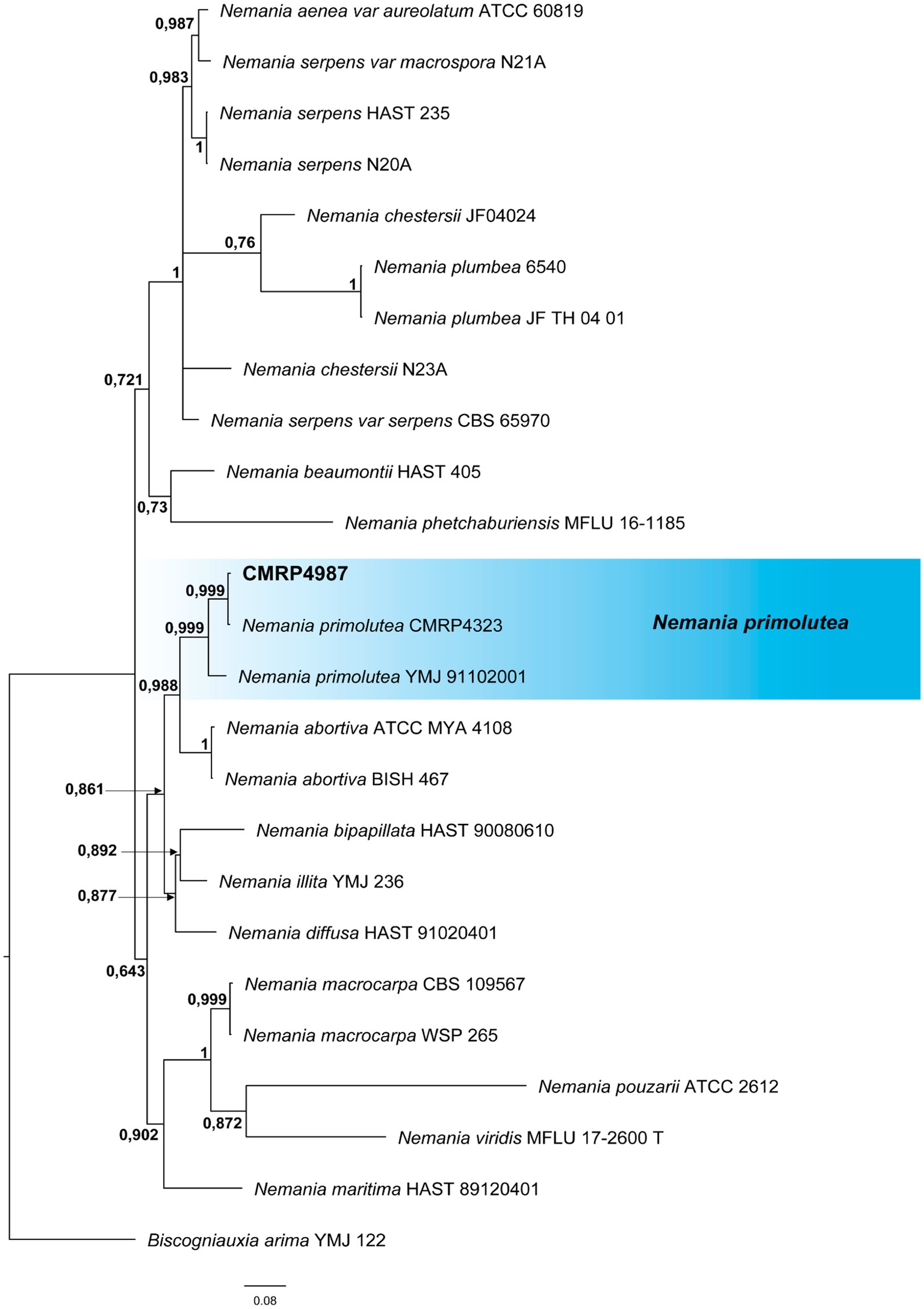
Figure 3. Bayesian inference phylogenetic tree of Nemania species based on multiple alignments of ITS, act, and tub2 partial sequences. The data matrix had 25 taxa and 2,846 characters. The species Biscogniauxia arima (YMJ 122) was used as an outgroup. Strains marked with a “T” correspond to type sequences. Bayesian posterior probabilities equal to or greater than 0.50 are presented next to each node. The scale bar of 0.08 represents the number of changes. The sequence of the isolate here studied is presented with its isolation code (CMRP4987) highlighted in bold.
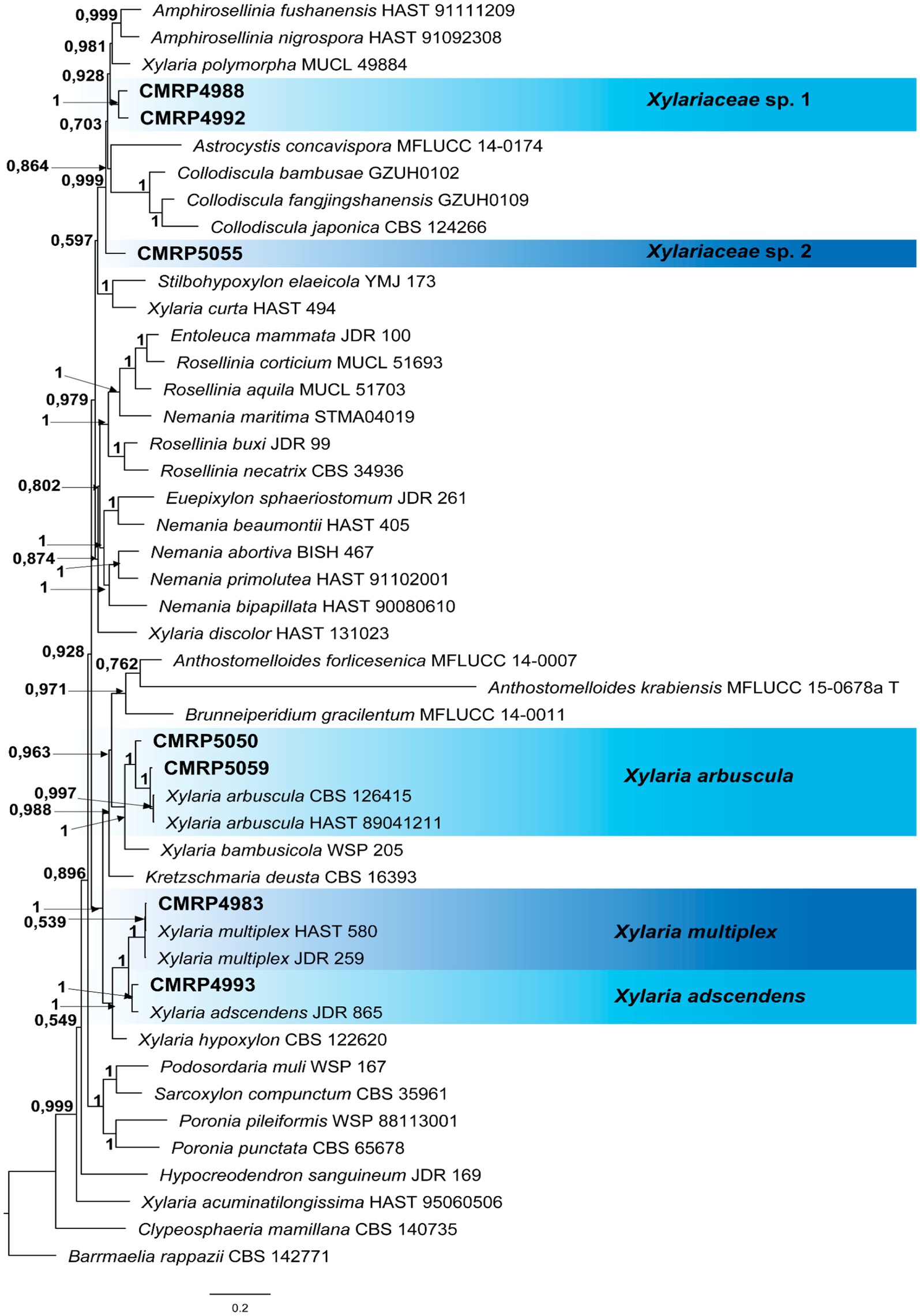
Figure 4. Bayesian Inference phylogenetic tree of species from Xylariaceae family based on multiple alignments of ITS, LSU, and tub2 partial sequences. The data matrix had 47 taxa and 5,647 characters. The species Barrmaelia rappazii (CBS 142771) was used as an outgroup. Strains marked with a “T” correspond to type sequences. Bayesian posterior probabilities equal to or greater than 0.50 are presented next to each node. The scale bar of 0.2 represents the number of changes. The sequences of the isolates here studied are presented with their isolation codes (CMRP4988, CMRP4992, CMRP5055, CMRP5050, CMRP5059, CMRP4983, and CMRP4993) highlighted in bold.
All the identified phenotypes belong to the Phylum Ascomycota within three classes: Eurotiomycetes, Dothideomycetes, and Sordariomycetes. Sordariomycetes was the dominant class corresponding to 69% of the isolates, and the dominant orders in this class were Diaporthales (25%, with 56 isolates belonging to three families and three genera), Glomerellales (21%, with 24 isolates of one family), and Xylariales (21% with 27 isolates of six genera of four families). The 293 isolates belong to 32 different genera of 27 families (Figure 5; Supplementary Table S6). All isolates were included in the same phenotype as the representative isolate used in the identification analysis belong to the same family/genera. Diaporthe and Phyllosticta were the most frequent genera isolated, with 50 isolates obtained from each of one, followed by Pseudofusicoccum with 44 isolates (Figure 5; Supplementary Table S6). It was also observed that among the most isolated genera the individuals were predominantly obtained by both leaf and petiole tissues, while in the least frequent genera, the host tissue specificity was more evident (Figure 5).
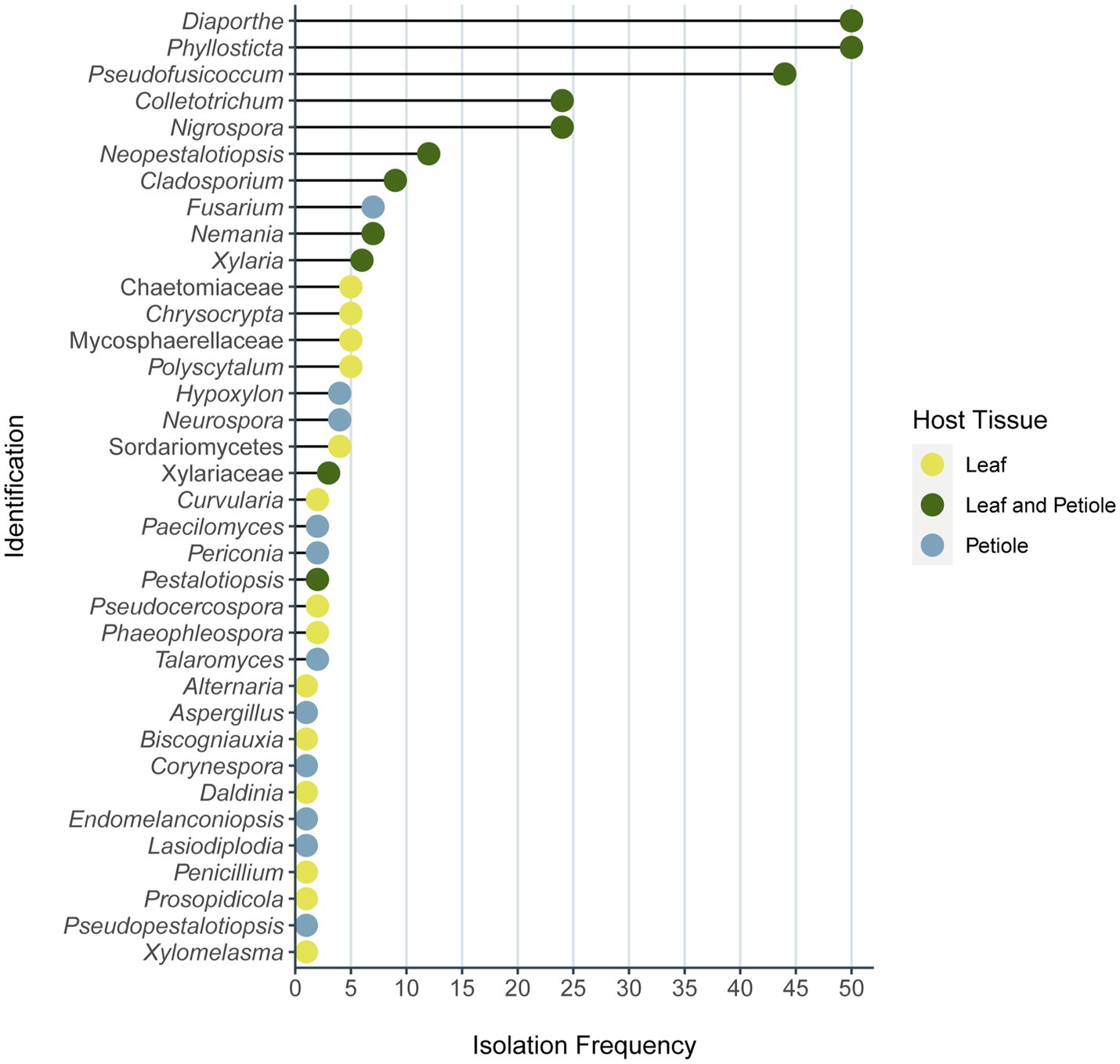
Figure 5. Frequency of endophyte isolates identified of each genus or family. Number of endophytes of each genus and family isolated from leaves, petioles, or both of plant Vochysia divergens in the present study. This result considers the molecular identification of each phenotype representative isolates. The color of each dot represents the host tissue origin of each genera/family, the isolates that came only from leaves are indicated in yellow, the ones from petiole only are indicated in blue, and the green dots mean that the isolates came from both plant tissues.
Thirteen isolates did not group with any type genera from the families Chaetomiaceae (5), Mycosphaerellaceae (5), and Xylariaceae (3). These are families not yet very well resolved phylogenetically (Supplementary Figures S2, S3; Figure 4). We suggest that additional gene sequences need to be obtained to identify these 13 isolates at a more restricted taxonomic level. Thus, in the present study, these isolates were assigned only at the family level. Other four isolates did not cluster with any family of the Onygenales order, then with an analysis based on a reevaluation study of this order, those isolates are currently located in an incertae sedis clade in Onygenales (Kandemir et al., 2022; Supplementary Figure S4). Therefore, those isolates remain identified on an Order level until further resolution studies.
All identifications were performed based on phylogenetic analysis individually for each genus or family and the trees are shown in the Supplementary material (Supplementary Figures S2–S36). The three dominant genera isolated in this study (Diaporthe, Phyllosticta, and Pseudofusicoccum) are discussed below. To identify the four strains producing bioactive SMs at the species level (i.e., Diaporthe amolarensis CMRP4997, Nemania primolutea CMRP4987, and Xylaria arbuscula CMRP5050 and CMRP5059), more robust analyses were performed using multilocus alignments. These analyses are also presented below.
3.1.1 Identification of isolates
3.1.1.1 The genus Diaporthe
Diaporthe was the dominant genus with 50 isolates obtained as endophytes that were grouped into 13 phenotypes (Supplementary Table S6; Supplementary Figure S1). Based on a phylogeny using the partial sequence of tef1 with 1,104 pb and 316 taxa (corresponding to the type and representative strains), these 13 phenotypes were identified as belonging to five clades (data not shown). Each clade was submitted to a new analysis based on Diaporthe species complex, following a recently reappraisal by Norphanphoun et al. (2022). The results are described below.
3.1.1.1.1 Clade 1 Diaporthe oncostoma species complex: Diaporthe amolarensis CMRP4997, and Diaporthe sp. CMRP5034
Bayesian inference analysis of the Diaporthe oncostoma species complex comprising 1750 pb of tef1, tub2, and his3 partial sequences showed the strains CMRP4997 and CMRP5034 clustered with CMRP4330 (Diaporthe sp.) in a single branch (supported by 0.999 probability), different from the other species present in the clade (Supplementary Tables S2, S7–S10). These three strains are phylogenetically close to the species D. anacardii, D. macadamiae, D. nebulae, D. velutia, D. phillipsii, and D. portugallica, and not distant from the species D. inconspicua and D. pterocarpi (Figure 2). The CMRP4330 strain was isolated by our group in a previous study (Iantas et al., 2021) also as an endophyte of V. divergens from the Pantanal Biome, however, from a different region than the Serra do Amolar. The strain CMRP5034 is very similar to CMRP4330 and was named Diaporthe sp. However, the strain CMRP4997 is a separate branch. Due to the long length of the branch, these strains probably do not belong to the same species (Figure 2).
The D. amolarensis CMRP4997 strain was the only one that showed sporulation in culture, and therefore, it was possible to describe this new species, which was designated Diaporthe amolarensis, named after its isolation region, the Serra do Amolar (Pantanal, Brazil). The macro and micromorphology were described using different culture media (Figure 6). Below, we describe this new species.
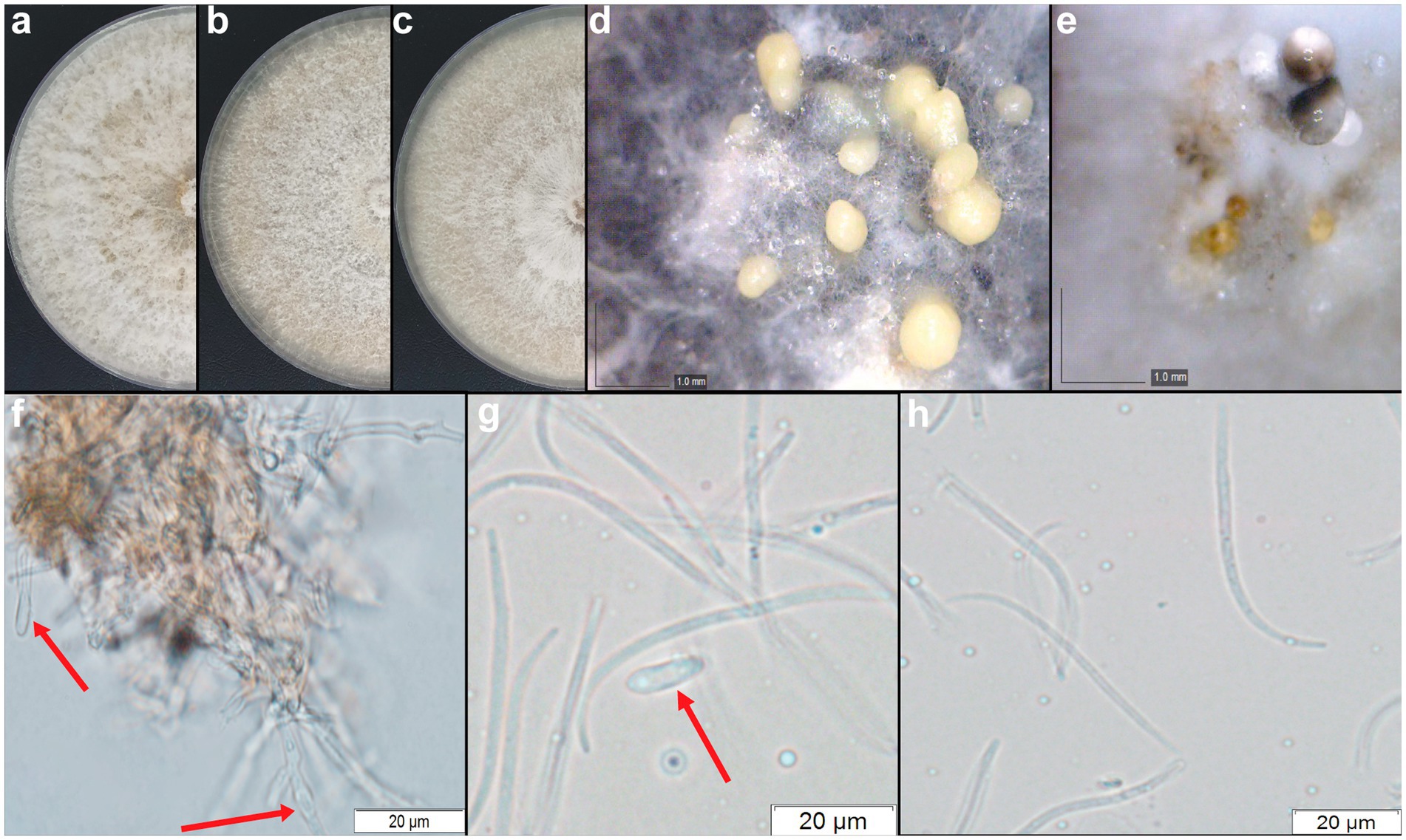
Figure 6. Diaporthe amolarensis (CMRP4997). (A–C) Colonies at 15 days on PDA, MEA, and OA, respectively. (D–E) Conidiomata sporulating on OA and MEA. (F) Conidiogenous cells. (G) Alpha conidia. (H) Beta conidia. Bars: (F–H) 20 μm.
Species description.
Diaporthe amolarensis CMRP4997.
Diaporthe amolarensis: Mayrhofer & Glienke, sp. nov. Mycobank 843837 (Figure 6).
Etymology: Named after the Pantanal region where it was collected, Serra do Amolar.
Sporulation on PDA and OA culture media. Conidiomata pycnidial globose, conical or irregular, solitary or aggregated, exposed on PDA medium surface, dark brown to black, cream translucent conidial drops exuded from the ostioles, 170–350 μm diameter. Conidiogenous cells hyaline and subcylindrical, tapering toward the apex 12.2–15.1 2–3.5 μm. Alpha conidia common, hyaline, fusiform, biguttulate, 12.8–16.1 3.3–4.9 μm, mean ± SD = 14.5 ± 1.8 4.29 ± 0.44 (n = 50). Beta conidia spindle-shaped, aseptate, smooth, hyaline, mostly curved toward one end 26.4–35.1 0.3–1.5 μm, mean ± SD = 30.26 ± 2.50 0.89 ± 0.29 (n = 50), and gamma conidia absent (Figure 6).
Culture characteristics: Colonies covering a dish after 15 days in the dark at 25°C. Colonies on flat PDA, aerial mycelium with cotton texture, white to pale yellow on the surface, colonies reaching 79 mm in diameter after 7 days at 25°C; reverse brown. In flat OA, aerial mycelium with fluffy texture in the center, white on the surface, colonies reaching 79 mm in diameter; reverse light yellow. In the flat MEA, aerial mycelium with cotton texture, white to pale yellow on the surface, colonies reaching 73 mm diameter; reverse yellow to brown forming concentric rings (Figure 6).
Specimen examined: Brazil, Serra do Amolar, Pantanal, Mato Grosso do Sul (18°15′37.8”S 57°27′37.4”W), endophytic species isolated from petiole of Vochysia divergens (popular name Cambará), February 2019, C. Glienke. Holotype: UPCB 98095 (Herbarium of the Department of Botany code, Federal University of Paraná), ex-type culture CMRP4997 (Microbiological Collections of Paraná Network at Federal University of Paraná).
Notes—Endophytic isolate of a medicinal plant in Brazil.
3.1.1.1.2 Clade 2 Diaporthe sojae species complex part 1: Diaporthe vochysiae (CMRP4977, CMRP4978, CMRP4994, CMRP4996, CMRP5036, and CMRP5220) and Diaporthe infertilis (CMRP5061)
Based on phylogenetic analysis of the Diaporthe sojae partial species complex using multiple alignments of the partial sequences of ITS, tef1, tub2, and his3 (comprising 2,273 characters), these six strains clustered with the type strain of D. vochysiae LGMF1583 (Supplementary Figure S5; Supplementary Table S14). This type strain was isolated by our group as an endophyte of V. divergens from the Pantanal Biome, however, from a different region than the Serra do Amolar (Noriler et al., 2019).
Moreover, based on the same phylogenetic tree, one strain CMRP5061 was clustered with the type strain (CBS 230.52) and two other representative strains (CBS 19939 and CPC 203.22) of the species Diaporthe infertilis (Supplementary Figure S5).
3.1.1.1.3 Clade 3 Diaporthe sojae species complex part 2: Diaporthe cerradensis (CMRP4985)
Based on the phylogenetic analysis of the Diaporthe sojae partial species complex based on the multiple alignment of ITS and tef1 partial sequences the strain CMRP4985 belongs to D. cerradensis species (Supplementary Figure S6; Supplementary Table S15).
3.1.1.1.4 Clade 4 Diaporthe rudis species complex: Diaporthe cf. heveae 1 (CMRP4989, CMRP5038)
Bayesian Inference analysis of the Diaporthe rudis species complex based on the alignment of tef1 partial sequence revealed strains CMRP4989 and CMRP5038 clustered with CBS 852.97 Diaporthe cf. heveae 1 (Supplementary Figure S7; Supplementary Table S16). This species still needs to be better resolved, as there is also Diaporthe cf. heveae 2, which belongs to a completely different clade (Gomes et al., 2013).
3.1.1.1.5 Clade 5 Diaporthe arecae species complex: Diaporthe podocarpi-macrophylli (CMRP4990)
Bayesian Inference analysis of the Diaporthe arecae species complex based on the alignment of tef1 partial sequence showed the strain CMRP4990 clustered with the CGMCC 3.18281 D. podocarpi-macrophylli type strain and another representative strain (LC6229) from the same species (Supplementary Figure S8; Supplementary Table S17).
3.1.1.2 The genus Phyllosticta (CMRP4971, CMRP4972, CMRP5048)
Phyllosticta together with Diaporthe was also the dominant genus with 50 isolates obtained as endophytes, but they were grouped into only three phenotypes. Based on a phylogenetic analysis using ITS and the partial sequences of tef1, act, and gapdh with 2038 characters and 81 taxa (corresponding to the type and representative strains), these three phenotypes were identified as belonging to the species P. capitalensis (Supplementary Figure S9; Supplementary Table S18). In the same clade are the strains CMRP4583 and CMRP4660 isolated by Iantas et al. (2021) as endophytes of V. divergens and previously identified as P. capitalensis. Despite the low support of this branch (0.66 posterior probability), the identity was confirmed based on the nucleotide differences between P. capitalensis and P. paracapitalensis according to Guarnaccia et al. (2017).
Phyllosticta species usually have low morphological diversity, and as phenotypes were assigned based on morphology, we cannot be sure that all 50 Phyllosticta isolates belong to the P. capitalensis species, although we sequenced the three most morphologically different strains (Supplementary Figure S1).
3.1.1.3 The genus Pseudofusicoccum (CMRP4982, CMRP4976, CMRP5047, and CMRP5219)
Pseudofusicoccum was one of the most abundant genera, with 44 isolates obtained as endophytes in this study. Based on morphology, we selected four strains for sequencing, and despite representing different phenotypes (Supplementary Figure S1), all were identified as P. stromaticum (Supplementary Figure S10; Supplementary Table S19). These isolates clustered with P. stromaticum CMRP4328 and P. stromaticum LGMF1608, both isolated by our group (Iantas et al., 2021; Noriler et al., 2018) as endophytes from Stryphnodendron adstringens and Vochysia divergens. Furthermore, an isolate previously identified as Pseudofusicoccum sp. LGMF1611 (Noriler et al., 2018) is also located in the same branch with high support, being currently identified as Pseudofusicoccum stromaticum.
3.1.1.4 Nemania primolutea CMRP4987
Due to the high bioactivities detected in the extract produced by this strain, the isolate of the genus Nemania was identified at the species level by multilocus analysis that comprised 2,868 pb of the partial sequences of ITS, act and tub2 of all type and representative strains with GenBank available sequences. The Bayesian Inference analysis (Figure 3; Supplementary Table S3) showed the strain CMRP4987 in the same branch (0.999 posterior probability) as the species N. primolutea (YMJ 91102001), sharing 96% similarity (identities = 540/565) and the strain CMRP4328 previously identified as N. primolutea with 99% similarity (identities = 586/589). Therefore, the CMRP4987 isolate was identified as N. primolutea.
3.1.1.5 The family Xylariaceae
Despite the low number of isolates obtained as endophytes in our study (6) belonging to the family Xylariaceae, we performed a more robust phylogenetic analysis due to the activity observed by secondary metabolites of the strains CMRP5050 and CMRP5059. Due to uncertainties regarding the taxonomic resolution within Xylariaceae sensu stricto, we decided to identify the isolates of this family according to Voglmayr et al. (2018). A multilocus analysis was carried out, comprising 47 taxa and 5,647 characters of the partial sequences of ITS, LSU, rpb2, and tub3 of the strains used in the Voglmayr et al. (2018) study (Supplementary Table S4). The Bayesian inference analysis (Figure 4) showed that our endophyte isolates belong to the Xylariaceae sensu stricto family (Voglmayr et al., 2018), which also corresponds to Xylariaceae clade xy1 (Samarakoon et al., 2022).
Two isolates were identified as Xylaria multiplex (CMRP4983) and Xylaria adscendens (CMRP4993) in the Xylariaceae sensu stricto. Three isolates (CMRP4988, CMRP4992, and CMRP5055) remain identified only at the family level because they did not cluster with any genus present in the analysis (Figure 4).
3.1.1.5.1 Xylaria arbuscula CMRP5050 and CMRP5059
The isolates CMRP5050 and CMRP5059 were selected for further analysis, due to the biological activity of their secondary metabolites. These two isolates are in the same branch (1 posterior probability) as the species X. arbuscula (CBS 126415 and HAST 89041211) (Figure 4). The strain CMRP5050 shares 95% similarity (identities = 511/538) and 95% similarity (Identities = 533/564) with CBS 126415 and HAST 89041211, respectively, and CMRP5059 shares 99% similarity (identities = 530/537) and 98% similarity (identities = 554/563) with CBS 126415 and HAST 89041211, respectively.
3.2 Endophytic fungi from Serra do Amolar produce SMs bioactive against phytopathogenic fungi
In the antifungal activity screening test of the 91 extracts produced by the endophytes, most treatments exhibited low inhibition of C. abscissum, approximately 10%. However, a few extracts stood out, appearing as outliers in the density plot (Figure 7). Among the extracts with higher activity, we highlight four (red points) that demonstrated equal or greater growth inhibition than the commercial fungicide Carbendazim (yellow point): Diaporthe amolarensis CMRP4997 (MGI: 96.65%), N. primolutea CMRP4987 (MGI: 92.99%), X. arbuscula CMRP5059 (MGI: 81.85%), and X. arbuscula CMRP5050 (MGI: 48.67%) (Figure 7).
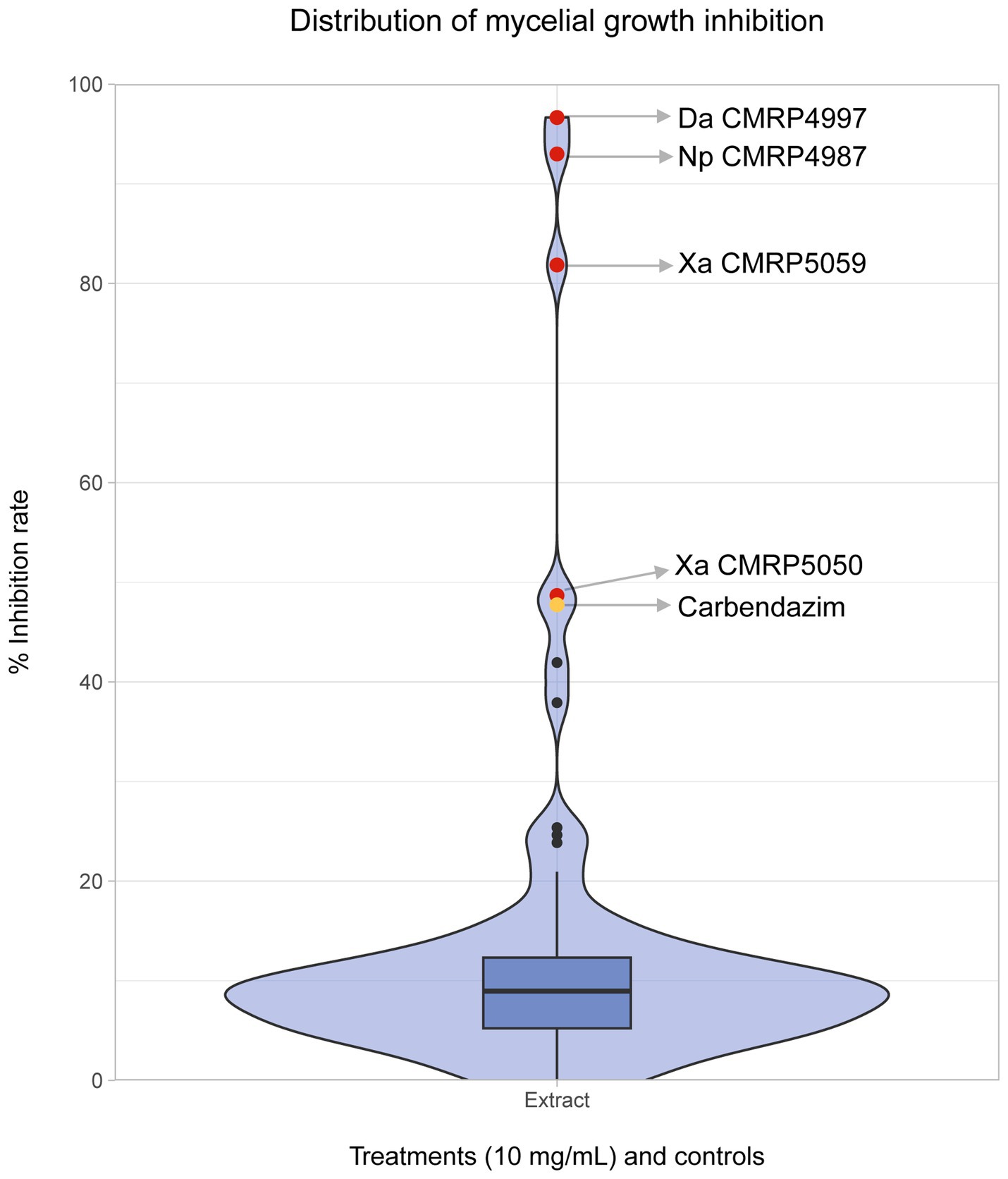
Figure 7. Distribution of mycelial growth inhibition of phytopathogen Colletotrichum abscissum (CMRP704) After exposure to extracts produced by endophytes. Distribution of mycelial growth inhibition (in %) of the phytopathogen Colletotrichum abscissum in the presence of 100 μL of the extracts at 10 mg/mL obtained by the cultivation of 91 endophytic fungi. The outside points colored in red represent the extracts produced by the isolates D. amolarensis (Da) CMRP4997, N. primolutea (Np) CMRP4987, X. arbuscula (Xa) CMRP5059, and X. arbuscula (Xa) CMRP5050, which had higher inhibition activity than the Carbendazim (showed in yellow).
Based on these results, four extracts were further evaluated for their antifungal activity against the phytopathogenic fungi F. graminearum and P. citricarpa (Figure 8). Against P. citricarpa, the extract produced by strain CMRP5059 X. arbuscula stood out, showing the highest mycelial growth inhibition (MGI: 68.64%). Furthermore, the extracts produced by the strains CMRP5050 (X. arbuscula) and CMRP4987 (N. primolutea) exhibited moderate activity against P. citricarpa, with MGI values of 55.38% and MGI: 49.91%, respectively (Figure 8). The extract from CMRP5050 (X. arbuscula) also demonstrated the highest inhibition rate against F. graminearum (MGI: 20.64%) (Figure 8). As the initial screening test was performed only against C. abscissum, we cannot infer about the antifungal activity of the non-evaluated extracts against P. citricarpa and F. graminearum. Therefore, it is possible that we could observe more active extracts against those two pathogens.
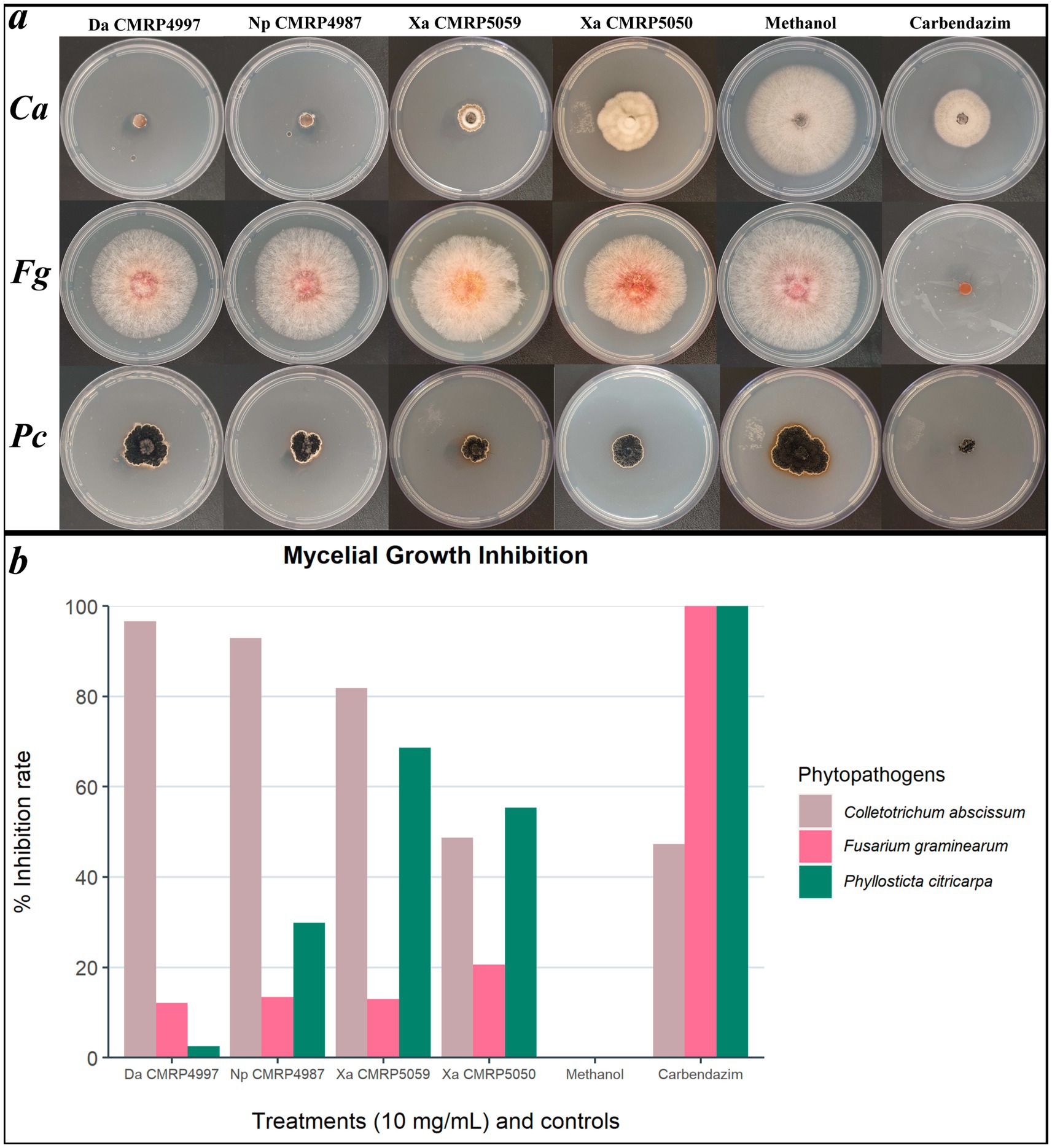
Figure 8. Mycelial growth inhibition of phytopathogens Colletotrichum abscissum (CMRP704), Fusarium graminearum (LGMF1703), and Phyllosticta citricarpa (CMRP06) after exposure to extracts selected in screening test. Mycelial growth inhibition test plates (A) and mean of mycelial growth inhibition (in %) (B) of the phytopathogens C. abscissum (Ca), F. graminearum (Fg), and P. citricarpa (Pc) in presence of 100 μL of the extracts from strains Diaporthe amolarensis (Da) CMRP4997 (MGI: 12.16%), Nemania primolutea (Np) CMRP4987 (MGI: 13.40%), Xylaria arbuscula (Xa) CMRP5059 (MGI: 12.96%), and Xylaria arbuscula (Xa) CMRP5050 (MGI: 20.64%), compared to the controls (Methanol and Carbendazim).
3.3 Cytotoxicity
The cytotoxicity of the crude extracts produced by the most interesting four endophytic fungi strains, Diaporthe amolarensis CMRP4997, Nemania primolutea CMRP4987, Xylaria arbuscula CMRP5059, and Xylaria arbuscula CMRP5050, were evaluated using A549 (non-small cell lung), PC3 (prostate), and HEL299 (human lung fibroblast). The extracts CMRP4997 and CMRP5059 displayed the highest cytotoxicity assays against all tested cell lines (with less than 10% cell viability). CMRP5050 demonstrated also high cytotoxicity in an assay against all three tested cell lines (~25% cell viability), and CMRP4987 showed low cytotoxicity against A549 and PC3, respectively, but was highly toxic against HEL299 (Supplementary Figure S41).
3.4 HPLC-UV/MS analysis of selected fungal extracts
The bioactive extracts produced by the most interesting four endophytic fungi strains, Diaporthe amolarensis CMRP4997, Nemania primolutea CMRP4987, Xylaria arbuscula CMRP5059, and Xylaria arbuscula CMRP5050, have been subjected for chemical screening analysis including HPLC-UV and HPLC-MS analyses. The extracts generated from the small-scale fermentations of these fungal strains have been dissolved in MeOH and subjected to HPLC-UV/MS analysis and compared with our microbial natural products database (AntiBase) (Laatsch, 2017) for compound dereplication/identification. The HPLC-UV/MS analysis of the obtained extracts from these four fungal strains displayed several interesting UV/vis and MS peaks (Supplementary Figures S42–S66). The HPLC-UV/MS analysis of the extract produced by the fungal strain, Diaporthe amolarensis CMRP4997, displayed five major peaks [HPLC Rt = 12.91, 27.3, 30.62, 31.25, and 32.45 min] with various UV/vis and molecular weights ranges (MW = 196–414 Daltons) (Supplementary Figures S42–S46). No mass and UV/vis match were found in the AntiBase search, which indicates the potential of this strain to produce new natural products. In the same manner, the HPLC-UV/MS analysis of the extract produced by the endophytic, Nemania primolutea CMRP4987, displayed several interesting peaks [HPLC Rt = 16.04 (MW 431), 21.83 (MW 478) 22.53 (MW 325), 27.19 (MW 332), 29.29 (MW 666), 30.62 (MW 290) and 31.26 min (no clear mass detected), 32.46 min (no clear mass detected)] with various UV/vis and molecular weights ranges (Supplementary Figures S47–S52). The two major LC-UV/MS peaks detected at 31.26 and 32.46 min in the fungal extract of Nemania primolutea CMRP4987 were the same as those peaks (31.26 and 32.46 min) detected in the extract of Diaporthe amolarensis CMRP4997. AntiBase search for the LC-UV/MS peaks detected from this strain extract indicated no hits and suggests the potential of the endophytic fungus, Nemania primolutea CMRP4987, to produce new natural products.
The HPLC-UV/MS metabolic profiles of the extracts produced by the two fungal strains, Xylaria arbuscula CMRP5059 and Xylaria arbuscula CMRP5050, indicate interesting compounds with diversity in their chemical structures (various UV/vis chromophores, with over 25 different major metabolites) (Supplementary Figures S53–S66). AntiBase search using the detected molecular weights and UV/vis resulted in the presence of several cytochalasin-analogs as well as halorosellinic acid analogs that match with MS and UV/vis, including 19,20-epoxycytochalasin C (MW 523), 19,20-epoxycytochalasin D (MW 523), cytochalasin N (MW 523), cytochalasin C (MW 507), cytochalasin D (MW 507), 6,7-dihydro-7-oxo-cytochalasin C (MW 507), halorosellinic acid (MW 432), and 17-dehydroxyhalorosellinic acid (MW 416), all of them have been reported previously from fungi (Edwards et al., 1989; Fujii et al., 2000; Chinworrungsee et al., 2001; Chinworrungsee et al., 2002; Chen et al., 2011; Wang et al., 2019; Figure 9). All the remaining LC-UV/MS peaks identified from these two fungal extracts did not match any UV/vis and mass data in AntiBase, which indicates the potential of these two fungal strains to produce interesting bioactive new natural products including new cytochalasins- and halorosellinic acid analogs. Based on the aforementioned LC-UV/MS and bioactivity data results, this study highlights that some of these selected strains including Xylaria arbuscula CMRP5059 and Xylaria arbuscula CMRP5050 are considered promising strains to produce new bioactive natural products, and have been selected for future studies (including scale-up fermentation, isolation, structure elucidation/compound identification, and bioactivities of the new bioactive compounds produced by these endophytic fungi) and will be published later.
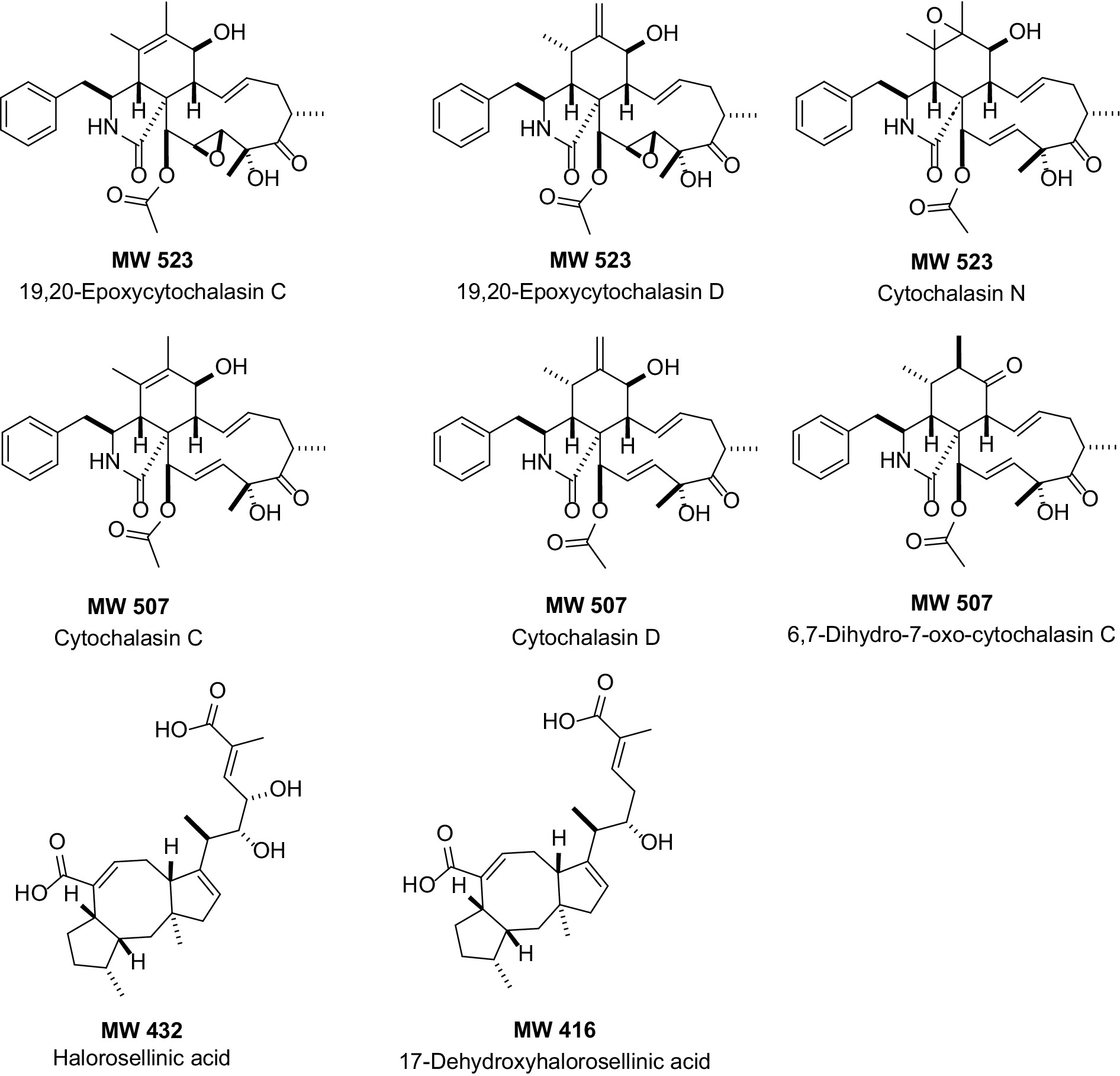
Figure 9. Chemical structures of the compounds identified from the selected endophytic fungal extracts (determined through HPLC-UV/vis and HPLC-ESI-MS profiling, and AntiBase search). MW, Molecular weight.
4 Discussion
Considering the importance of discovering new bioactive compounds for agriculture applications, the potential of endophytes to produce novel natural products from an underexplored biome in Brazil combined with the need for microbial biodiversity conservation, we conducted a bioprospecting study on endophytic fungi of the medicinal plant V. divergens, from the Serra do Amolar to produce SMs with biological activity against phytopathogens. We also acted on the ex situ conservation of the endophytes, preserving them in the CMRP Culture Collection of the TaxOnline Network.
The isolation data presented in this study indicate a high diversity of endophytic fungi associated with the medicinal plant V. divergens from the Serra do Amolar region of the Pantanal wetlands. Phylogenetic analysis revealed that we isolated at least 54 different species from 27 families and 32 genera and at least 19 new species of endophytic fungi. We consider this richness to be very high, given that we used only 18 plant specimens of the same species collected from the same region in the Pantanal Biome. The extensive fire that occurred in 2020 affected more than 90% of this environmental conservation region, with an estimated loss of 742,000 trees and approximately 17 million vertebrate animals, mainly reptiles (Pletsch et al., 2021). Some of the new species reported in this study, which were isolated in 2019, may no longer be present in situ, however, were preserved ex-situ at the CMRP culture collection. This underscores the importance of isolating and preserving endophytes in culture collections, where they will be stored and available for future research (Smith and Ryan, 2008).
These results corroborate the findings of previous studies that also isolated endophytes from the plant V. divergens (Noriler et al., 2018; Iantas et al., 2021). Noriler et al. (2018) isolated 777 endophytic fungi from leaves and petioles of the plant V. divergens, also collected in the Pantanal, but in a different region, approximately 260 km from the sample collection area of this study, near the Miranda River. The isolates belonged primarily to the Phylum Ascomycota, although some were Basidiomycota. The class Sordariomycetes was dominant, similar to what was observed in the present study, with Diaporthales being the most dominant order among main orders. In addition, as in the present study, Diaporthe was the most dominant genus. The genus Diaporthe belongs to the family Diaporthaceae and comprises hundreds of species (Chepkirui and Stadler, 2017), being recently considered a paraphyletic genus (Gao et al., 2017). This genus is distributed worldwide and its species are able to colonize a wide range of hosts in various associations, occurring as endophytes, plant pathogens, and saprobes (Udayanga et al., 2012; Gomes et al., 2013). Among endophytic fungi, the genus Diaporthe is one of the most commonly isolated from various host plants and is frequently associated with the production of secondary metabolites with diverse biological activities (Chepkirui and Stadler, 2017; Gomes et al., 2013). Most of the bioactive SMs recently described from isolates of this genus were obtained from endophytes associated with medicinal plants (Chepkirui and Stadler, 2017), with some recent studies demonstrating antifungal activity against citrus phytopathogens such as C. abscissum and P. citricarpa (Tonial et al., 2017; Noriler et al., 2018; Savi et al., 2020; Iantas et al., 2021). Investigations of this study support the findings of high antifungal activity obtained by Diaporthe amolarensis, which was also reported as a new species.
Over the years, due to the need to explore compounds from natural sources, our group has conducted numerous bioprospecting studies, reporting the production of SMs of endophytic microorganisms (Savi et al., 2015; Gos et al., 2017; Noriler et al., 2018; Savi D. C. et al., 2019). In this study, we report four extracts produced by endophytic fungi that inhibited the mycelial growth of the phytopathogen C. abscissum: Diaporthe amolarensis CMRP4997 (MGI: 96.65%), N. primolutea CMRP4987 (MGI: 92.99%), Xylaria arbuscula CMRP5059 (MGI: 81.85%), and X. arbuscula CMRP5050 (MGI: 48.67%). The first three extracts inhibited the mycelial growth of the phytopathogen levels higher than the positive control, the fungicide Carbendazim. C. abscissum is the causal agent of postbloom fruit drop (PFD), a disease that affects citrus production (Silva et al., 2017), in which necrotic lesions on the petals and premature fruit drop and calyx retention are the main symptoms (Pinho et al., 2015). In Brazil, PFD causes significant production losses, especially in the state of São Paulo, where much of citrus cultivation is concentrated (Pinho et al., 2015; Silva et al., 2017). Therefore, finding endophytic isolates that produce SMs active against this pathogen is of great importance. Additionally, studying endophyte extracts at the compound level can help to characterize the biological activity observed in the crude extract, and provide knowledge for a future application of these compounds.
In addition to the good results observed against C. abscissum by the extract of Diaporthe amolarensis CMRP4997, those obtained with the metabolites of the isolates Nemania primolutea CMRP4987, Xylaria arbuscula CMRP5059, and X. arbuscula CMRP5050 also showed promising activities. These three isolates belong to the family Xylariaceae, known as an important source of SMs with biological activity (Petrini, 1986; Stadler, 2011; Kuhnert et al., 2014). Members of the Xylariaceae family are normally of cosmopolitan distribution and are mainly endophytes, although can also be found as phytopathogens. However, little is known about the species N. primolutea and X. arbuscula and their bioactive metabolites. The species N. primolutea was described by Ju et al. (2005) been isolated from a dead trunk of Artocarpus communis in Taiwan. To date, few studies have demonstrated the biological activity of isolates of this species, such as antibacterial (Tan et al., 2020) and lignolytic (Idris et al., 2019) activities. Recently, a study from our group reported antifungal activity from extracts of this species, with mycelial growth inhibition of C. abscissum (MGI: 80%), and P. citricarpa (MGI: 76%), as well as inhibition of symptoms development in citrus flowers and leaves (Iantas et al., 2021). X. arbuscula species was described by Saccardo (1878), and recently, it was related to the production of the compound cytochalasin B. This compound is a mycotoxin with various biological activities including nuclear cell extrusion, inhibition of HIV-1 protease, and antibiotic and cytotoxic effects, and was also related to the symbiotics endophyte/host interaction (Amaral, 2009; Amaral et al., 2014; Amaral et al., 2017). All these data support the results presented in this study and reaffirm the diverse biological potential of the SMs produced by those strains, especially those isolated from medicinal plants from Brazilian biomes.
The bioactive metabolites produced by the four most promising endophytic fungi strains were subjected to chemical screening analysis, including HPLC-UV and HPLC-MS. These analyses revealed several UV/vis and MS peaks, indicating the production of compounds with great chemical structural diversity. An AntiBase search for the LC-UV/MS peaks detected from some of these metabolites indicated no hits, suggesting that these endophytic fungi may produce new natural products.
Furthermore, we evaluated the cytotoxic activities of the four most promising extracts against A549 (non-small cell lung), PC3 (prostate), and HEL299 (human lung fibroblast) cell lines. The crude extracts produced by Diaporthe amolarensis CMRP4997 and Xylaria arbuscula CMRP5059 displayed high cytotoxicity across all tested cell lines. The high activity of the extract from Nemania primolutea CMRP4987 against HEL299 suggests that the antifungal activity against the phytopathogens observed may be related to the high cytotoxicity against the cancer cell lines. In contrast, the crude extract of CMRP4987 showed promising antifungal activity, but only low or moderate cytotoxicity, indicating a potentially different mechanism of antifungal action (Supplementary Figure S41). Because all biological evaluations were conducted using crude extracts, the detected cytotoxic activity may not be caused by the same pure compounds, which produce the antifungal activity, particularly as the LC-UV/MS data of the crude extracts indicate that these extracts may produce different classes of chemical structures.
Although no extract demonstrated strong activity against F. graminearum, the causal agent of Fusarium head blight (FHB), the observed rates of mycelial growth inhibition rates are not negligible, given the difficulty in controlling this pathogen. The best result obtained in this study against this pathogen was achieved with the extract produced from the fermentation of the X. arbuscula CMRP5050 (MGI: 23.0%). Additionally, a common strategy for managing this pathogen is the search for compounds that reduce the production of mycotoxins, called anti-mycotoxigenic (Pagnussatt et al., 2014), which should be an approach in future studies. Furthermore, it is important to note that the present study is the first to demonstrate the antifungal activity of extracts produced by an endophyte of the genus Xylaria against the phytopathogens C. abscissum, P. citricarpa, and F. graminearum.
In conclusion, this study highlights the Serra do Amolar as an important region of the Pantanal in Brazil, with a rich diversity of endophytes, including the newly described species D. amolarensis. Furthermore, extracts from four endophytic fungi demonstrated promising SMs with biological activity against phytopathogens, such as C. abscissum, P. citricarpa, and F. graminearum and should be further explored (scale-up fermentation, isolation of compounds, and purification) to identify bioactive new natural products and their mode of action. Finally, a significant contribution of this study was the ex situ conservation of the Serra do Amolar biodiversity, supporting future research and potential biotechnological applications of these endophytes. Such studies add value to Brazilian biodiversity and underscore the importance of conserving these biomes.
Data availability statement
The datasets presented in this study can be found in online repositories. The names of the repository/repositories and accession number(s) can be found in the article/Supplementary material.
Ethics statement
Ethical approval was not required for the studies on humans in accordance with the local legislation and institutional requirements because only commercially available established cell lines were used.
Author contributions
BM: Data curation, Formal analysis, Investigation, Methodology, Software, Visualization, Writing – original draft, Writing – review & editing. JI: Data curation, Formal analysis, Investigation, Writing – review & editing. SN: Formal analysis, Methodology, Resources, Writing – original draft. LP: Formal analysis, Investigation, Methodology, Writing – review & editing. JT: Formal analysis, Funding acquisition, Methodology, Writing – review & editing. JR: Conceptualization, Data curation, Funding acquisition, Investigation, Methodology, Project administration, Supervision, Writing – original draft, Writing – review & editing. KS: Conceptualization, Data curation, Formal analysis, Funding acquisition, Investigation, Methodology, Supervision, Validation, Writing – original draft, Writing – review & editing. CG: Conceptualization, Data curation, Funding acquisition, Project administration, Resources, Supervision, Writing – original draft, Writing – review & editing.
Funding
The author(s) declare that financial support was received for the research, authorship, and/or publication of this article. This study was supported by the INCT Citrus CNPq 465440/2014-2 Brazil and CNPq grant 309971/2016-0 and 424738/2016-3 and 310887/2019-4 to CG, CAPES-Brazil—grant to BM. This study was also supported by National Institutes of Health grant R37 AI052218 (JT), the Center of Biomedical Research Excellence (COBRE) for Translational Chemical Biology (CTCB, NIH P20 GM130456), the National Institute of Food and Agriculture (USDA-NIFA-CBGP, Grant No. 2023-38821-39584; KS and JT), the University of Kentucky College of Pharmacy, the University of Kentucky Markey Cancer Center and the National Center for Advancing Translational Sciences (UL1TR000117 and UL1TR001998), as well as through a University Professorship in Pharmacy, and NIH grant 5R01CA243529-05 (to JR).
Conflict of interest
The authors declare that the research was conducted in the absence of any commercial or financial relationships that could be construed as a potential conflict of interest.
The author(s) declared that they were an editorial board member of Frontiers, at the time of submission. This had no impact on the peer review process and the final decision.
Publisher’s note
All claims expressed in this article are solely those of the authors and do not necessarily represent those of their affiliated organizations, or those of the publisher, the editors and the reviewers. Any product that may be evaluated in this article, or claim that may be made by its manufacturer, is not guaranteed or endorsed by the publisher.
Supplementary material
The Supplementary material for this article can be found online at: https://www.frontiersin.org/articles/10.3389/fmicb.2024.1501182/full#supplementary-material
Footnotes
1. ^https://institutoserradoamolar.org.br
2. ^https://www.cmrp-taxonline.com
3. ^National Center for Biotechnology Information—http://www.ncbi.nlm.nih.gov/BLAST/.
5. ^http://www.westerdijkinstitute.nl/
References
Afzal, M., Ahmed, E., Sharif, A., and Javaid, A. (2023). Antifungal potential of two new triterpenoidal glycosides from the Albizia kalkora against fusarium oxysporum f. sp. lycopersici. Allelopath. J. 60, 83–92. doi: 10.26651/allelo.j/2023-60-1-1455
Amaral, L. S. (2009). Análise de metabólitos secundários produzidos por fungos endofíticos associados à Cupressus lusitanica [dissertation]. São Carlos: Universidade Federal de São Carlos.
Amaral, L. S., Fill, T. P., Santos, L. F. A., and Rodrigues-Filho, E. (2017). Biosynthesis and mass spectral fragmentation pathways of 13C and 15N labeled cytochalasin D produced by Xylaria arbuscula. J. Mass Spectrom. 52, 239–247. doi: 10.1002/jms.3922
Amaral, L. S., Rodrigues-Filho, E., Santos, C. A. A., Abreu, L. M., and Pfenning, L. H. (2014). An HPLC evaluation of cytochalasin D biosynthesis by Xylaria arbuscula cultivated in different media. Nat. Prod. Commun. 9, 1279–1282. doi: 10.1177/1934578X1400900914
Araújo, W. L., Quecine, M. C., Lacava, P. T., Aguilar-Vildoso, C. I., Marcon, J., Lima, A. O. S., et al. (2014). Microrganismos endofíticos: Aspectos teóricos e práticos de isolamento e caracterização. 2nd Edn. Santarém: UFOPA.
Arieira, J., and Da Cunha, C. N. (2006). Fitossociologia de uma floresta inundável monodominante de Vochysia divergens Pohl (Vochysiaceae), no Pantanal Norte, MT, Brasil. Acta Botanica Brasilica 20, 569–580. doi: 10.1590/S0102-33062006000300007
Baldassari, R. B., De Reis, R. F., and Goes, A. (2006). Susceptibility of fruits of the “Valência” and “Natal” sweet orange varieties to Guignardia citricarpa and the influence of the coexistence of healthy and symptomatic fruits. Fitopatol. Bras. 31, 337–341. doi: 10.1590/S0100-41582006000400002
Braga, G. Ú. L., Silva-Junior, G. J., Brancini, G. T. P., Hallsworth, J. E., and Wainwright, M. (2022). Photoantimicrobials in agriculture. J. Photochem. Photobiol. B Biol. 235:112548. doi: 10.1016/j.jphotobiol.2022.112548
Brown, N. A., Antoniw, J., and Hammond-Kosack, K. E. (2012). The predicted secretome of the plant pathogenic fungus fusarium graminearum: a refined comparative analysis. PLoS One 7:4. doi: 10.1371/journal.pone.0033731
Carbone, I., and Kohn, L. M. A. (1999). Method for designing primer sets for speciation studies in filamentous ascomycetes. Mycologia 91, 553–556. doi: 10.1080/00275514.1999.12061051
Chen, Z., Huang, H., Chen, Y., Wang, Z., Ma, J., Wang, B., et al. (2011). New Cytochalasins from the marine-derived fungus Xylaria sp. SCSIO 156. Helvetica Chimica Acta 94:51. doi: 10.1002/hlca.201100051
Chepkirui, C., and Stadler, M. (2017). The genus Diaporthe: a rich source of diverse and bioactive metabolites. Mycol. Prog. 16, 477–494. doi: 10.1007/s11557-017-1288-y
Chinworrungsee, M., Kittakoop, P., Isaka, M., Chanphen, R., Tanticharoen, M., and Thebtaranonth, Y. (2002). Halorosellins a and B, unique isocoumarin glucosides from the marine fungus Halorosellinia oceanica. J. Chamical Soc. Perkin Trans. 1, 2473–2476. doi: 10.1039/B207887M
Chinworrungsee, M., Kittakoop, P., Isaka, M., Rungrod, A., Tanticharoen, M., and Thebtaranonth, Y. (2001). Antimalarial halorosellinic acid from the marine fungus Halorosellinia oceanica. Bioorg. Med. Chem. Lett. 11, 1965–1969. doi: 10.1016/s0960-894x(01)00327-4
Crous, P. W., Groenewald, J. Z., Risède, J. M., Simoneau, P., and Hyde, K. D. (2004). Calonectria species and their Cylindrocladium anamorphs: species with sphaeropedunculate vesicles. Stud. Mycol. 55, 213–226. doi: 10.3114/sim.55.1.213
Daranagama, D. A., Camporesi, E., Tian, Q., Liu, X., Chamyuang, S., Marc Stadler, M., et al. (2015). Anthostomella is polyphyletic comprising several genera in Xylariaceae. Fungal Divers. 73, 203–238. doi: 10.1007/s13225-015-0329-6
Darriba, D., Taboada, G., Doallo, R., and Posada, D. (2012). JModelTest 2: more models, new heuristics and parallel computing. Nat. Methods 9:772. doi: 10.1038/nmeth.2109
De Hoog, G. S., and Gerrits van den Ende, A. H. G. (1998). Molecular diagnostics of clinical strains of filamentous Basidiomycetes. Mycoses 41, 183–189. doi: 10.1111/j.1439-0507.1998.tb00321.x
Edwards, R., Maitland, D. J., and Whalley, A. J. S. (1989). Metabolites of the higher fungi. Part 24. Cytochalasin N, O, P, Q, and R. New cytochalasins from the fungus Hypoxylon terricola mill. J. Chamical Soc. Perkin Trans. 1, 57–65.
Faria, D. J., Carvalho, A. P. A. D., and Conte-Junior, C. A. (2023). Valorization of fermented food wastes and byproducts: bioactive and valuable compounds, bioproduct synthesis, and applications. Fermentation 9:920. doi: 10.3390/fermentation9100920
Fontes, E. M. G., and Valadares-Inglis, M. C. (2020). Controle biológico de pragas da agricultura. Brasilia, DF: Embrapa.
Fujii, Y., Tani, H., Ichinoe, M., and Nakajima, H. (2000). Zygosporin D and two new Cytochalasins produced by the fungus Metarrhizium anisopliae. J. Nat. Prod. 63, 132–135. doi: 10.1021/np990331t
Fundação Ecotrópica (2003). Plano de Manejo das RPPN’S Acurizal, Penha e Dorochê. Cuiabá, MT: Fundação de Apoio à Vida nos Trópicos.
Gao, Y., Liu, F., Duan, W., Crous, P. W., and Cai, L. (2017). Diaporthe is paraphyletic. IMA Fungus 8, 153–187. doi: 10.5598/imafungus.2017.08.01.11
Gilchrist-Saavedra, L., Fuentes-Dávila, G., Martínez-Cano, C., López-Atilano, R. M., Duveiller, E., and Singh, R. P. (2006). Practical guide to the identification of selected diseases of wheat and barley. 2nd Edn. Mexico: CIMMYT.
Glass, N. L., and Donaldson, G. C. (1995). Development of primer sets designed for use with the PCR to amplify conserved genes from filamentous ascomycetes. Appl. Environ. Microbiol. 61, 1323–1330. doi: 10.1128/aem.61.4.1323-1330.1995
Glienke, C. (1999). Guignardia citricarpa Kiely: Análise Genética, Cariotípica e Interação com o Hospedeiro. [dissertation]. Piracicaba, SP: Escola Superior de Agricultura Luiz de Queiroz.
Glienke, C., Pereira, O. L., Stringari, D., Fabris, J., Kava-Cordeiro, V., Galli-Terasawa, L., et al. (2011). Endophytic and pathogenic Phyllosticta species, with reference to those associated with Citrus black spot. Persoonia 26, 47–56. doi: 10.3767/003158511X569169
Glienke, C., Petters-Vandresen, D. A. L., Souto, A. D. S. S., Marinoni, L., and Da Silva, M. (2024). Microbiological collections in Brazil: current status and perspectives. Diversity 16:116. doi: 10.3390/d16020116
Gomes, R. R., Glienke, C., Videira, S. I. R., Lombard, L., Groenewald, J. Z., and Crous, P. W. (2013). Diaporthe: a genus of endophytic, saprobic and plant pathogenic fungi. Persoonia 31, 1–41. doi: 10.3767/003158513X666844
Gos, F. M. W. R., Savi, D. C., Shaaban, K. A., Thorson, J. S., Aluizio, R., Possiede, Y. M., et al. (2017). Antibacterial activity of endophytic Actinomycetes isolated from the medicinal plant Vochysia divergens (Pantanal, Brazil). Front. Microbiol. 8:1642. doi: 10.3389/fmicb.2017.01642
Guarnaccia, V., Groenewald, J. Z., Li, H., Glienke, C., Carstens, E., Hattingh, V., et al. (2017). First report of Phyllosticta citricarpa and description of two new species, P. Paracapitalensis and P. Paracitricarpa, from citrus in Europe. Stud. Mycol. 87, 161–185. doi: 10.1016/j.simyco.2017.05.003
Hall, T. A. (1999). BioEdit: a user-friendly biological sequence alignment editor and analysis program for windows 95/98/NT. Nucleic Acids Symp. Ser. 41, 95–98.
Hsieh, H. M., Ju, Y. M., and Rogers, J. D. (2005). Molecular phylogeny of Hypoxylon and closely related genera. Mycologia 97, 844–865. doi: 10.3852/mycologia.97.4.844
Iantas, J., Savi, D. C., Schibelbein, R. S., Noriler, S. A., Assad, B. M., Dilarri, G., et al. (2021). Endophytes of Brazilian medicinal plants with activity against Phytopathogens. Front. Cell. Infect. Microbiol. 12, 1–18. doi: 10.3389/fmicb.2021.714750
Idris, I. R., Kanti, A., and Sudiana, I. M. (2019). Screening of potential lignin-degrading fungi from the tropical forest for lignocellulose biotreatment. IOP Conf. Series 308, 12014–12017. doi: 10.1088/1755-1315/308/1/012014
Javed, S., Mahmood, Z., Khan, K. M., Sarker, S. D., Javaid, A., Khan, I. H., et al. (2021). Lupeol acetate as a potent antifungal compound against opportunistic human and phytopathogenic mold Macrophomina phaseolina. Sci. Rep. 11, 8411–8417. doi: 10.1038/s41598-021-87725-7
Ju, Y. M., Rogers, J. D., and Hsieh, H. M. (2005). New Hypoxylon and Nemania species from Costa Rica and Taiwan. Mycologia 97, 562–567. doi: 10.3852/mycologia.97.2.562
Kandemir, H., Dukik, K., Teixeira, M. M., Stielow, J. B., Delma, F. Z., Al-Hatmi, A., et al. (2022). Phylogenetic and ecological reevaluation of the order Onygenales. Fungal Divers. 115, 1–72. doi: 10.1007/s13225-022-00506-z
Katoh, K., and Toh, H. (2008). Recent developments in the MAFFT multiple sequence alignment program. Brief. Bioinform. 9, 286–298. doi: 10.1093/bib/bbn013
Kuhnert, E., Fournier, J., Persoh, D., Luangsa-ard, J. J. D., and Stadler, M. (2014). New Hypoxylon species from Martinique and new evidence on the molecular phylogeny of Hypoxylon based on ITS rDNA and β-tubulin data. Fungal Divers. 64, 181–203. doi: 10.1007/s13225-013-0264-3
Lima, W. G., Spósito, M. B., Amorim, L., Gonçalvez, F. P., and Filho, P. A. M. (2011). Colletotrichum gloeosporioides, a new causal agent of citrus post-bloom fruit drop. Eur. J. Plant Pathol. 131, 157–165. doi: 10.1007/s10658-011-9795-1
Miller, M.A., Pfeiffer, W., and Schwartz, T. (2011). The CIPRES science gateway: a community resource for phylogenetic analyses. Proceedings of the TeraGrid 2011 Conference: Extreme Digital Discovery, TG’11. 41, 1–8.
Morandi, M. A. B., Paula Júnior, T. J., Bettiol, W., and Teixeira, H. (2009). Controle biológico de fungos fitopatogênicos. Informe Agropecuário. 30, 73–82.
Moreira, V. F. (2011). Rede de Proteção e Conservação da Serra do Amolar: Rompendo fronteiras para a conservação do Pantanal. [dissertation]. Corumbá, MS: Universidade Federal do Mato Grosso do Sul.
Noriler, S. A., Savi, D. C., Aluizio, R., Palácio-Cortes, A. M., Possiede, Y. M., and Glienke, C. (2018). Bioprospecting and structure of fungal endophyte communities found in the Brazilian biomes, pantanal, and Cerrado. Front. Microbiol. 9:1526. doi: 10.3389/fmicb.2018.01526
Noriler, S. A., Savi, D. C., Ponomareva, L. V., Rodrigues, R., Rohr, J., Thorson, J. S., et al. (2019). Vochysiamides a and B: two new bioactive carboxamides produced by the new species Diaporthe vochysiae. Fitoterapia 138:104273. doi: 10.1016/j.fitote.2019.104273
Norphanphoun, C., Gentekaki, E., Hongsanan, S., Jayawardena, R., Senanayake, I. C., Manawasinghe, I. S., et al. (2022). Diaporthe: formalizing the species-group concept. Mycosphere 13, 752–819. doi: 10.5943/mycosphere/13/1/9
O’Donnell, K., and Cigelnik, E. (1997). Two divergent intragenomic rDNA ITS2 types within a monophyletic lineage of the fungus fusarium are nonorthologous. Mol. Phylogenet. Evol. 7, 103–116. doi: 10.1006/mpev.1996.0376
Paccanaro, M. C., Sella, L., Castiglioni, C., Giacomello, F., Martínez-Rocha, A. L., D’Ovidio, R., et al. (2017). Synergistic effect of different plant Cell Wall–degrading enzymes is important for virulence of fusarium graminearum. Mol. Plant-Microbe Interact. 30, 886–895. doi: 10.1094/MPMI-07-17-0179-R
Pagnussatt, F. A., Spier, F., Bertolin, T. E., Costa, J. A. V., and Gutkoski, L. C. (2014). Technological and nutritional assessment of dry pasta with oatmeal and the microalga Spirulina platensis. Brazilian J. Food Technol. 17, 296–304. doi: 10.1590/1981-6723.1414
Pessi, D. D., Silva, N. M., Mioto, C. L., Barbosa, D. S., Moreira, R. M., Gomes, M. A. G., et al. (2023). Aplicação de Sensoriamento Remoto na análise das mudanças da vegetação de campos de altitude no Pantanal usando dados multitemporais Landsat. Terr Plural. 17:e2321497. doi: 10.5212/TerraPlural.v.17.2321497.003
Petrini, O. (1986). Taxonomy of endophytic Fungi of aerial plant tissues. Microbiology of the Phyllosphere. Cambridge: Cambridge University Press, 175–187.
Petrini, O., Sieber, T. N., Toti, L., and Viret, O. (1993). Ecology, metabolite production, and substrate utilization in endophytic fungi. Nat. Toxins 1, 185–196. doi: 10.1002/nt.2620010306
Pinho, D. B., Lopes, U. P., and Pereira, O. L. (2015). Fungal Planet description sheets: 320-370. Persoonia 34, 167–266. doi: 10.3767/003158515X688433
Pletsch, M. A. J. S., Junior, C. H. L. S., Penha, T. V., Körting, T. S., Silva, M. E. S., Pereira, G., et al. (2021). The 2020 Brazilian pantanal fires. Anais da Academia Brasileira de Ciencias. 93:e20210077. doi: 10.1590/0001-3765202120210077
Raeder, U., and Broda, P. (1985). Rapid preparation of DNA from filamentous fungi. Lett. Appl. Microbiol. 1, 17–20. doi: 10.1111/j.1472-765X.1985.tb01479.x
Rafiq, M., Javaid, A., Kanwal, A., Anwar, A., Khan, I. H., Kanwal, Q., et al. (2024). GC-MS analysis and antifungal potential of flower extract of Acacia nilotica subsp. Indica against Macrophomina phaseolina. Microbial Pathogenesis 194, 1–9. doi: 10.1016/j.micpath.2024.106819
Ronquist, F., Teslenko, M., Mark, P., Ayres, D. L., Darling, A., Höhna, S., et al. (2012). Mrbayes 3.2: efficient bayesian phylogenetic inference and model choice across a large model space. Syst. Biol. 61, 539–542. doi: 10.1093/sysbio/sys029
Saccardo, P. A. (1878). Fungi Veneti novi vel critici vel mycologiae Venetae addendi. Series VIII. Michelia 1, 239–275.
Samarakoon, M. C., Hyde, K. D., Maharachchikumbura, S. S. N., Stadler, M., Jones, E. B. G., Promputtha, I., et al. (2022). Taxonomy, phylogeny, molecular dating and ancestral state reconstruction of Xylariomycetidae (Sordariomycetes). Fungal Divers. 112, 1–88. doi: 10.1007/s13225-021-00495-5
Sang, H., and Kim, J. (2020). Advanced strategies to control plant pathogenic fungi by host-induced gene silencing (HIGS) and spray-induced gene silencing (SIGS). Plant Biotechnol. Rep. 14, 1–8. doi: 10.1007/s11816-019-00588-3
Savi, D. C. (2011). Biodiversidade e bioprospecção de actinomicetos da planta Vochysia divergens (Cambará) [dissertation]. Curitiba, PR: Universidade Federal do Paraná.
Savi, D. C., Shaaban, K. A., Mitra, P., Ponomareva, L. V., Thorson, J. S., Glienke, C., et al. (2019). Secondary metabolites produced by the citrus phytopathogen Phyllosticta citricarpa. Journal of Antibiotics. 72:, 306–310. doi: 10.1038/s41429-019-0154-3
Savi, D. C., Haminiuk, C. W. I., Sora, G. T. S., Adamoski, D. M., Kenski, J., Winnischofer, S. M. B., et al. (2015). Antitumor, antioxidant and antibacterial activities of secondary metabolites extracted by endophytic Actinomycetes isolated from Vochysia Divergens. Int. J. Pharmaceut. Chem. Biol. Sci. 5, 347–356.
Savi, D. C., Noriler, S. A., Ponomareva, L. V., Thorson, J. S., Rohr, J., Glienke, C., et al. (2020). Dihydroisocoumarins produced by Diaporthe cf. heveae LGMF1631 inhibiting citrus pathogens. Folia Microbiol. 65, 381–392. doi: 10.1007/s12223-019-00746-8
Savi, D. C., Shaaban, K. A., Gos, F. M. W. R., Ponomareva, L. V., Thorson, J. S., and Glienke, C. (2018). Phaeophleospora vochysiae Savi & Glienke sp. nov. isolated from Vochysia divergens found in the Pantanal, Brazil, produces bioactive secondary metabolites. Sci. Rep. 8:3122. doi: 10.1038/s41598-018-21400-2
Savi, D., Aluizio, R., and Glienke, C. (2019). Brazilian Plants: An Unexplored Source of Endophytes as Producers of Active Metabolites. Planta Medica. 85:, 619–636. doi: 10.1055/a-0847-1532
Schulz, B., Boyle, C., Draeger, S., and Römmert, A. K. (2002). Endophytic fungi: a source of novel biologically active secondary metabolites. Mycol. Res. 106, 996–1004. doi: 10.1017/S0953756202006342
Shaaban, K. A., Elshahawi, S. I., Wang, X., Horn, J., Kharel, M. K., Leggas, M., et al. (2015). Cytotoxic Indolocarbazoles from Actinomadura melliaura ATCC 39691. J. Nat. Prod. 78, 1723–1729. doi: 10.1021/acs.jnatprod.5b00429
Shaaban, K. A., Wang, X., Elshahawi, S. I., Ponomareva, L. V., Sunkara, M., Copley, G. C., et al. (2013). Herbimycins D-F, ansamycin analogues from Streptomyces sp. RM-7-15. J. Nat. Prod. 76, 1619–1626. doi: 10.1021/np400308w
Silva, A. O., Savi, D. C., Gomes, F. B., Gos, F. M. W. R., Silva, G. J. Jr., and Glienke, C. (2017). Identification of Colletotrichum species associated with postbloom fruit drop in Brazil through GAPDH sequencing analysis and multiplex PCR. Eur. J. Plant Pathol. 147, 731–748. doi: 10.1007/s10658-016-1038-z
Smith, D., and Ryan, M.J. (2008). The ex situ conservation of microorganisms: Aiming at a certified quality management. Biotechnology. Encyclopedia of Life Support Systems. Biotechnology, XV.
Stadler, M. (2011). Importance of secondary metabolites in the Xylariaceae as parameters for assessment of their taxonomy, phylogeny, and functional biodiversity. Curr. Res. Environ. Appl. Mycol. 1, 75–133. doi: 10.5943/cream/1/2/1
Tamura, K., Peterson, D., Peterson, N., Stecher, G., Nei, M., and Kumar, S. (2011). MEGA5: molecular evolutionary genetics analysis using maximum likelihood, evolutionary distance, and maximum parsimony methods. Mol. Biol. Evol. 28, 2731–2739. doi: 10.1093/molbev/msr121
Tan, M. A., Castro, S. G., Oliva, P. M. P., Yap, P. R. J., Nakayama, A., Magpantay, H. D., et al. (2020). Biodiscovery of antibacterial constituents from the endolichenic fungi isolated from Parmotrema rampoddense. 3 Biotech 10, 212–217. doi: 10.1007/s13205-020-02213-5
Tan, X., Zhou, Y., Zhou, X., Xia, X., Wei, Y., He, L., et al. (2018). Diversity and bioactive potential of culturable fungal endophytes of Dysosma versipellis; a rare medicinal plant endemic to China. Sci. Rep. 8:5929. doi: 10.1038/s41598-018-24313-2
Tonial, F., Maia, B. H. N. S., Sobottka, A. M., Savi, D. C., Vicente, V. A., Gomes, R. R., et al. (2017). Biological activity of Diaporthe terebinthifolii extracts against Phyllosticta citricarpa. FEMS Microbiol. Lett. 364, 1–7. doi: 10.1093/femsle/fnx026
Torres-Rodriguez, J. A., Reyes-Pérez, J. J., Quinones-Aguilar, E. E., and Hernandez-Montiel, L. G. (2022). Actinomycete potential as biocontrol agent of Phytopathogenic Fungi: mechanisms, source, and applications. Plan. Theory 11:3201. doi: 10.3390/plants11233201
Udayanga, D., Liua, X., Mckenzie, E. H. C., Chukeatirote, E., and Hyde, K. D. (2012). Multi-locus phylogeny reveals three new species of Diaporthe from Thailand. Cryptogam. Mycol. 33, 295–309. doi: 10.7872/crym.v33.iss3.2012.295
Vilgalys, R., and Hester, M. (1990). Rapid genetic identification and mapping of enzymatically amplified ribosomal DNA from several Cryptococcus species. J. Bacteriol. 172, 4238–4246. doi: 10.1128/jb.172.8.4238-4246.1990
Voglmayr, H., Friebes, G., Gardiennet, A., and Jaklitsch, W. M. (2018). Barrmaelia and Entosordaria in Barrmaeliaceae (fam. Nov., Xylariales) and critical notes on Anthostomella-like genera based on multigene phylogenies. Mycol. Prog. 17, 155–177. doi: 10.1007/s11557-017-1329-6
Wang, W. X., Li, Z. H., He, J., Feng, T., Li, J., and Liu, J. K. (2019). Cytotoxic cytochalasans from fungus Xylaria longipes. Fitoterapia 137:104278. doi: 10.1016/j.fitote.2019.104278
Wang, X., Shaaban, K. A., Elshahawi, S. I., Ponomareva, L. V., Sunkara, M., Zhang, Y., et al. (2013). Frenolicins C-G, Pyranonaphthoquinones from Streptomyces sp. RM-4-15. J. Nat. Prod. 76, 1441–1447. doi: 10.1021/np400231r
Keywords: Diaporthe , Xylaria , natural products, Vochysia divergens , Pantanal, Serra do Amolar, secondary metabolites
Citation: Mayrhofer BF, Iantas J, Noriler SA, Ponomareva LV, Thorson JS, Rohr J, Shaaban KA and Glienke C (2024) Highly diverse endophytic fungi from Serra do Amolar-Pantanal (Brazil) producing bioactive secondary metabolites against phytopathogens. Front. Microbiol. 15:1501182. doi: 10.3389/fmicb.2024.1501182
Edited by:
Ravinder Kumar, Indian Agricultural Research Institute (ICAR), IndiaReviewed by:
Geromy G. Moore, Agricultural Research Service (USDA), United StatesArshad Javaid, University of the Punjab, Pakistan
Copyright © 2024 Mayrhofer, Iantas, Noriler, Ponomareva, Thorson, Rohr, Shaaban and Glienke. This is an open-access article distributed under the terms of the Creative Commons Attribution License (CC BY). The use, distribution or reproduction in other forums is permitted, provided the original author(s) and the copyright owner(s) are credited and that the original publication in this journal is cited, in accordance with accepted academic practice. No use, distribution or reproduction is permitted which does not comply with these terms.
*Correspondence: Jürgen Rohr, anJvaHIyQHVreS5lZHU=; Khaled A. Shaaban, a2hhbGVkX3NoYWFiYW5AdWt5LmVkdQ==; Chirlei Glienke, Y2guZ2xpZW5rZUBnbWFpbC5jb20=