- 1Key Laboratory of National Forestry and Grassland Administration on Biodiversity Conservation in Southwest China, Southwest Forestry University, Kunming, China
- 2Forestry College, Southwest Forestry University, Kunming, China
- 3Key Laboratory for Forest Resources Conservation and Utilization in the Southwest Mountains of China, Ministry of Education, Southwest Forestry University, Kunming, China
Background: Botrytis cinerea a blueberry gray mold, is one of the main diseases affecting postharvest storage, causing significant losses. Several studies have shown that Bacillus tequilensis can prevent the growth of plant pathogens by producing various antibacterial substances, and can induce plant resistance. However, research on the biological management of post-harvest gray mold in blueberries using B. tequilensis remains unclear.
Methods: To better control the postharvest gray mold of blueberry, the effects of B. tequilensis KXF6501 fermentation solution (YY) and KXF6501 cell-free supernatant (SQ) on the induction of disease resistance in blueberry fruits were studied using biochemical and transcriptomic analyses.
Results: We found that YY controlled the conidial germination and mycelial growth of B. cinerea in vitro, followed by SQ. After 3 d of culture, the lesion diameter and incidence of gray mold in blueberry fruits inoculated with YY and SQ were smaller than those in the control group. Therefore, gray mold in blueberries was effectively controlled during the prevention period, and the control effect of YY was better than that of SQ. Transcription spectrum analysis of blueberry peel tissue showed that the YY- and SQ-induced phenylpropane metabolic pathways had more differentially expressed genes (DEGs) than other biological pathways. In addition, biochemical analyses showed that YY treatment effectively enhanced the activity of enzymes related to the phenylpropane pathway (phenylalanine ammonialyase [PAL], cinnamate 4-hydroxylase [C4H], 4-coumarate CoA ligase [4CL], and polyphenol oxidase [PPO]) and stimulated the synthesis of lignin, total phenols, and flavonoids, followed by SQ. Compared with the control, the YY and SQ treatments reduced the weight loss rate and better maintained the appearance and nutritional quality of the blueberry fruits.
Conclusion: Our findings suggest that B. tequilensis KXF6501 is potentially useful as a suitable bio-control agent in harvested blueberries.
1 Introduction
Blueberries, classified within the genus Vaccinium of the family Ericaceae (Bell et al., 2021). Blueberries are known as the “king of berries”, due to their abundance of amino acids, trace elements and its unique compounds with high biological activity (anthocyanins, tannins, flavonoids and phenolic acids, etc.), so it is highly beneficial to the liver, as it helps to prevent cancer, prolong life, and protect the heart (Chen et al., 2015, 2017; Miller et al., 2019; Shi et al., 2019a). Gray mold caused by Botrytis cinerea is one of the most important diseases affecting blueberries, limiting the storage, transportation, and sales of blueberries (Rivera et al., 2013; Ji et al., 2019). B. cinerea causes fruit tissue death by activating toxin production, degrading enzyme production, oxygen-active substances, and programmed cell death, which seriously affects the economic value of blueberries (Bell et al., 2021; Bi et al., 2023). At present, chemical control is the oldest and most common method of blueberry postharvest storage and is based on the use of chemicals, such as chlorine, chlorine dioxide, sulfur dioxide, and some synthetic fungicides, to reduce postharvest diseases (Bell et al., 2021). Although chemical treatments can effectively control blueberry diseases, they can easily lead to drug residues and environmental pollution (Bell et al., 2021). Therefore, it is of great significance to seek safe and efficient storage and preservation technologies for the postharvest preservation of fruits, vegetables, and human health.
Biological control technologies for antagonistic microorganisms have become a significant focus of research in the field of fruit preservation because of their high efficiency, lack of pesticide residues, and easy degradation (Alvarez et al., 2019; Chen et al., 2020). Bacillus spp. strains attracted considerable attention as biological control agents (BCAs) because they produce endogenous spores, enzymes, antibiotics, and toxins (Kiesewalter et al., 2020). Simultaneously, they compete for limited food and space, obviating disease-causing pathogenic fungi from growing in their hosts (Panebianco et al., 2015; Papoutsis et al., 2019). Kurniawan et al. (2018) showed that several Bacillus spp. have antifungal properties against B. cinerea of blueberries. Bacillus subtilis KLBC BS6 was effective in inhibiting B. cinerea conidia germination and mycelial growth, remarkably reducing gray mold outbreaks and enhancing the disease-resistant enzymatic activities of phenylalanine ammonia-lyase [PAL], polyphenol oxidase [PPO], Chitinase [CHI], and superoxide dismutase [SOD] in blueberry fruits (Lu et al., 2021). Bacillus tequilensis JN-369 induces and improves the SOD, CAT, and POD activities in rice, thus enabling resistance against rice blast infection (Zhou et al., 2021).
Research has shown that B. tequilensis can prevent the growth of plant pathogens by producing various antibacterial substances, such as biosurfactants and lipopeptides, and can induce plant resistance (Singh and Sharma, 2020). However, research on the biological management of post-harvest gray mold in blueberries using B. tequilensis remains unclear. Therefore, the primary objectives of this study were to (a) evaluate the antimicrobial properties of B. tequilensis KXF6501 on B. cinerea in post-harvest blueberry fruits, (b) investigate the changes in RNA-Seq transcription patterns in blueberry fruits induced by fermentation solution (YY) and cell-free supernatant (SQ), and (c) explore the impact of YY and SQ in preventive treatments on enzyme activities related to the phenylpropane pathway in blueberry fruits.
2 Materials and methods
2.1 Fruits and microorganism sources
Jewelry blueberries were hand harvested from a mercantile orchard in Chengjiang, Yuxi, Yunnan Province, China (24°38’32” N latitude, 102°53’28” E longitude). The orchard has superior natural environment, large-scale planting, rich varieties (such as jewelry, Emerald and Lexie) and high-level planting and management technology. Select blueberries that are uniformly mature, evenly sized, free from diseases and pests, and without mechanical damage, and bring them back to the laboratory immediately. Immersed in 2% (v/v) sodium hypochlorite for 30 s and drying at 22°C for disinfection. Subsequently, they were shared among different groups based on a completely randomized block design.
B. cinerea was isolated from diseased blueberries, cultured on PDA for 5 d at 25 °C until sporulation occurred. Subsequently, spore suspensions were produced by flooding B. cinerea cultures with sterile distilled water, and spore concentrations were controlled to 1 × 105 conidia mL–1 using a haemocytometer.
Strawberries that appeared healthy and showed no physical damage were used to isolate the B. tequilensis strain KXF6501. The strawberry fruit was soaked in a 250 mL beaker containing 100 mL of PBS buffer for 2 h to prepare a stock solution. Subsequently, 1 mL of the stock solution was diluted to 10–4, 10–5, and 10–6, and 100 μL of each diluted sample was spread onto nutrient agar (NA) plates, respectively. Plates were incubated at 28 ° for 24 h, and a single colony was transferred and purified on NA medium. The isolated B. tequilensis strain KXF6501 was identified using the 16S rDNA gene. Meanwhile, it is maintained at −80 °C in a nutrient broth with glycerol’s by the Plant Pathology Laboratory of Southwest Forestry University. The strain was activated and grown for 24 h at 28 °C on nutrient agar.
2.2 Preparation of KXF6501 fermentation solution and cell-free supernatant
Culture suspension of KXF6501 (1 × 107 CFU mL–1) incubated for 24 h was prepared into the following two kinds of treatment solutions: (1) KXF6501 fermentation solution (YY): culture suspension of KXF6501 (1 × 107 CFU mL–1) incubated for 24 h; (2) KXF6501 cell-free supernatant (SQ): culture suspension of KXF6501 (1 × 107 CFU mL–1) was incubated for 24 h, filtered by sterile filter membrane with a diameter of 0.22 micron, and the supernatant was collected for later use.
2.3 Effects of KXF6501 fermentation solution and cell-free supernatant against B. cinerea in vitro
The antagonistic effects of YY and SQ on B. cinerea were assessed using the dual-culture plate approach described by Shi et al. (2014). In a plate containing fresh PDA (diameter, 90 mm), a 6 mm diameter plug of a 5-d B. cinerea mycelial culture was centered. Then, four pieces of sterile filter paper with a diameter of 6 mm were inoculated with 5 μL treating fluids (YY or SQ) at a distance of 3 cm away from fungal inoculums. PDA inoculated with only the pathogenic fungus served as the control. Both inoculated and controls plates were incubated for 5 d at 25 °C, and colonies were measured and recorded. Each test was performed in triplicate by three plates.
2.4 Efficacy of KXF6501 fermentation solution and cell-free supernatant on the B. cinerea conidia germination in vitro
According to Wang F. et al. (2019), conidial germination and germ tube elongation of B. cinerea were measured in potato glucose broth to further assess the antagonistic action of YY and SQ. We mixed 1 mL of a suspension of fungal spores (1 × 107 conidia mL–1) with 1 mL of YY or SQ supplemented with 10 mL of PDB in 50 mL Erlenmeyer flasks, and the fungal spore suspension (1 × 107 conidia mL–1) alone served as a control (CK). After inoculation, it was cultured in a shaking table (28 °C, 120 rpm/min) for 2 and 4 h, and the germination rate and germ tube lengths were observed using a microscope (a minimum of 100 B. cinerea spores per repetition).
2.5 Effects of KXF6501 fermentation solution and cell-free supernatant against blueberry gray mold in vivo
The pretreated blueberry fruits were divided into three groups and placed in empty sterile Petri dishes. With a sterile needle, a 3 mm deep wound was made on each blueberry fruits, and each wound was treated with 10 μL of YY, SQ or sterile distilled water (CK). After 2 h, 10 μL of B. cinerea spore suspension (1 × 105 Conidia mL–1) was inoculated into the same wound (Shi et al., 2019b). After the spore suspension is completely absorbed, fruits were stored at 22 °C and 85–95%. Disease incidence and lesion diameter were observed and recorded daily (Wu et al., 2022; Wang et al., 2024). The lesion diameter was measured using the criss-cross method. The incidence rate was calculated using the following formula: incidence rate (%) = (number of diseased fruits / total number of fruits) × 100%. Each treatment had three independent replicates, with eight blueberries per replicate and 72 blueberries per trial. All the experiments were performed twice.
2.6 Transcriptomic analysis of blueberry fruits treated with KXF6501 fermentation solution and cell-free supernatant
2.6.1 Extraction of RNA, RNA sequencing, and identification of DEGs
Blueberry fruits were soaked in YY (YY-1, YY-2, YY-3), SQ (SQ-1, SQ-2, SQ-3), or sterile distilled water (CK-1, CK-2, CK-3). After 24 h of storage, peel samples were frozen in liquid nitrogen and total RNA was extracted using the MirVana miRNA Isolation Kit. The RNA content was measured using a NanoDrop ND-2000 spectrophotometre, and cDNA libraries were created using a TruSeq Stranded mRNA Sample Preparation Kit. PANOMIX Biotech Co., Ltd. (Sichuan, China) conducted a transcriptomic analysis to remove low-quality reads to ensure high-quality data. DEG-seq was used to identify DEGs with thresholds of | log2FC| > 1 and P-value < 0.05.
2.6.2 GO and KEGG analysis on DEGs
For gene function annotation, the DEGs were classified using the GO database1 and metabolic pathways enriched for DEGs were identified using the KEGG database (KEGG).2
2.6.3 RNA-seq verification using real-time quantitative reverse transcription-polymerase chain reaction (RT-qPCR)
Fruit peel RNA was extracted and cDNA was synthesized as described previously (Shi et al., 2019c). To validate the results of our transcriptomic analysis, RT-qPCR was performed using the TIB8600 Real-Time PCR System (Triplex International Biosciences, Xiamen, China) with primers specific for Differentially Expressed Genes (DEGs) identified through bioinformatics analysis (Supplementary Table 1).
2.7. Effects of KXF6501 fermentation solution and cell-free supernatant on phenylpropanoid metabolism related enzymes and substances in blueberry fruits
Blueberries were subjected to treatments, with YY, SQ, and sterile distilled water as a control (CK) for 5 min. Subsequently, fruits were air-dried, placed in plastic containers, and stored at 22 °C with 85–95% relative humidity for 10 d. Each treatment was replicated three times, and the test was repeated twice. The effects of YY and SQ on the enzymatic activity of PAL, 4CL, PPO, and C4H, as well as the levels of flavonoid, total phenols, and lignin in fruit samples collected at 0, 2, 4, 6, 8, and 10 d post-treatment were assessed. The PAL activity was determined following the protocol outlined by Zhou et al. (2014), whereas the PPO activity was measured according to the method described by Shi et al. (2018). According to methods described by Deng et al. (2016) for determining the activity of C4H and 4CL. The results are expressed in units per gram of fresh fruit weight. A Folin-Ciocalteu colorimetric approach was used to determine total phenols and flavonoids (Ozgen et al., 2010; Yihui et al., 2018; Lu et al., 2019; Zhang et al., 2019), whereas Toscano et al. (2018) approach determined lignin content of fruits.
2.8 Effects of KXF6501 fermentation solution and cell-free supernatant on blueberry quality
To assess the effects of YY and SQ on the quality of blueberries, the fruits were subjected to individual soaking treatments: (1) YY, (2) SQ, or (3) sterile distilled water (CK) for 5 min. Subsequently, the fruits were placed in plastic containers and stored at 22 °C with a relative humidity of 85–95% for 10 d. Fruit samples (0, 2, 4, 6, 8, and 10 d) were collected after treatment.
Each 2-d weight of the fruit (in grams) was measured, and the percent loss in weight was computed by applying the formula (weight loss rate % = (A–B)/A), where A is the initial weight of the fruit before treatment, and B is the weight of the fruit at a specific time point. The levels of total soluble solids and reducing sugars in fruits were quantified using a portable refractometer and the anthrone reagent method, while the ascorbic acid and titratable acidity content were determined using 2,6-dichlorophenol indophenol and titration with 0.1 M NaOH, respectively. The color characteristics (L, a, b, c, and h0) of the fruits were assessed using a colorimeter.
2.9 Statistical approach
Data analysis was conducted using Tukey’s multiple range tests in SPSS22.0 software (IBM Company, Armonk, NY, USA), with significance observed at P < 0.05.
3 Results
3.1 The effect of KXF6501 fermentation solution and cell-free supernatant against B. cinerea in vitro
As shown in Table 1, the effects of YY and SQ on the germ tube length and conidial germination rate of B. cinerea in vitro were showed. Following the application of YY and SQ, a significant decrease (P < 0.05) in the conidial germination rate of B. cinerea was noticed in the YY treatment group after 2 h, whereas SQ initially reduced the germination rate of the conidia, and the inhibitory effect gradually decreases over time. For germ tube length, no significant differences were observed between the treatment and control group at the 2-h. However, after 4 h of treatment, the length of B. cinerea germinal tubes in the YY group reduced to 51.58 μm, which was noticeably lower compared to both the control group (79.63 μm) and the SQ group (52.60 μm) (P < 0.05).

Table 1. Effects of KXF6501 fermentation solution and cell-free supernatant on germ tube lengths and conidia germination rate of B. cinerea grown on PDB over 2 and 4 h.
The dual-culture plate result indicated that YY demonstrated antagonistic effects on B. cinerea mycelial growth, followed by SQ (Supplementary Figure 1A). Following a 5-d incubation period, the colony diameters of the YY and SQ groups measured 2.03 and 5.27 cm, respectively (Figure 1). These values were noticeably lower than those in the control fruits, with inhibition rates of 75.30% and 36.03% (P < 0.05), respectively.
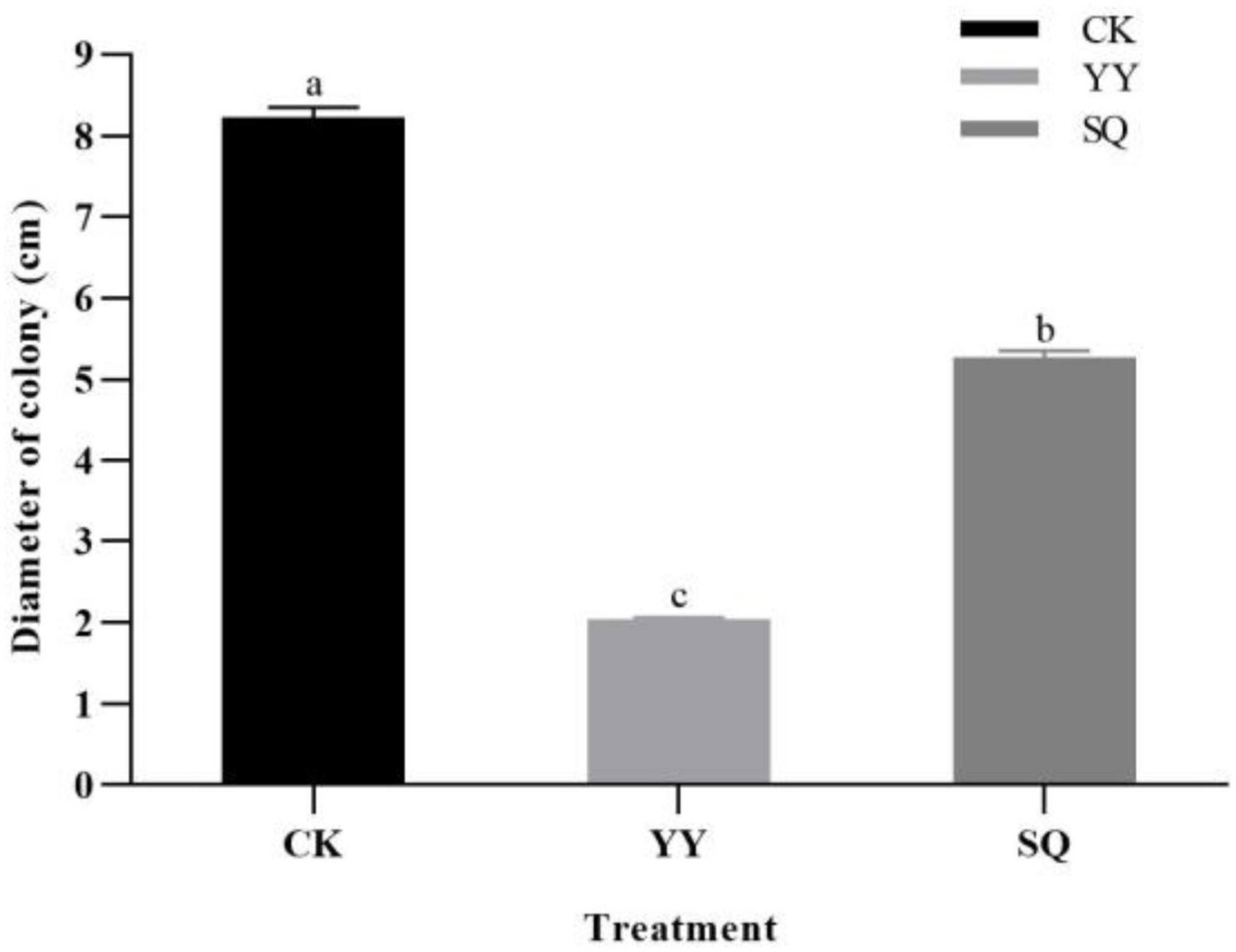
Figure 1. Inhibitory effect of KXF6501 fermentation solution and cell-free supernatant on B. cinerea after 5 d. Based on the Tukey’s multiple range test, there are significant (P < 0.05) differences between all variables followed by letters. All units are reported as means of three distinct values ± SE. Different lowercase letters indicate significant (P < 0.05) differences between treatments by the Tukey’s multiple range test.
3.2 Efficacy of KXF6501 fermentation solution and cell-free supernatant in controlling blueberry gray mold in vivo
As shown in Table 2, the application of YY reduced gray mold decay attributed to B. cinerea, followed by SQ (Supplementary Figure 1B). Following 2-d inoculation, the incidence rate of the YY treatment group was notably the lowest at 13.33%, a statistically significant decrease (P < 0.05) compared to that of the control fruits, with the SQ treatment fruits at 20.00%. In the YY and SQ treatment groups, morbidity decreased (P < 0.05) by 40% and 20%, respectively, on the 3rd day, compared to that in the control fruits (100%). After 3 d, the lesion diameters in the three groups of infected blueberries exhibited noticeable variation. Blueberries treated with YY after storage for 3, 4, and 5 d had lesion diameters of 0.63, 0.83, and 0.97 cm, respectively, which were notably smaller than those of the SQ treatment group (0.76, 0.91, and 1.07 cm) and the control group (0.96, 1.34, and 1.50 cm) (P < 0.05).
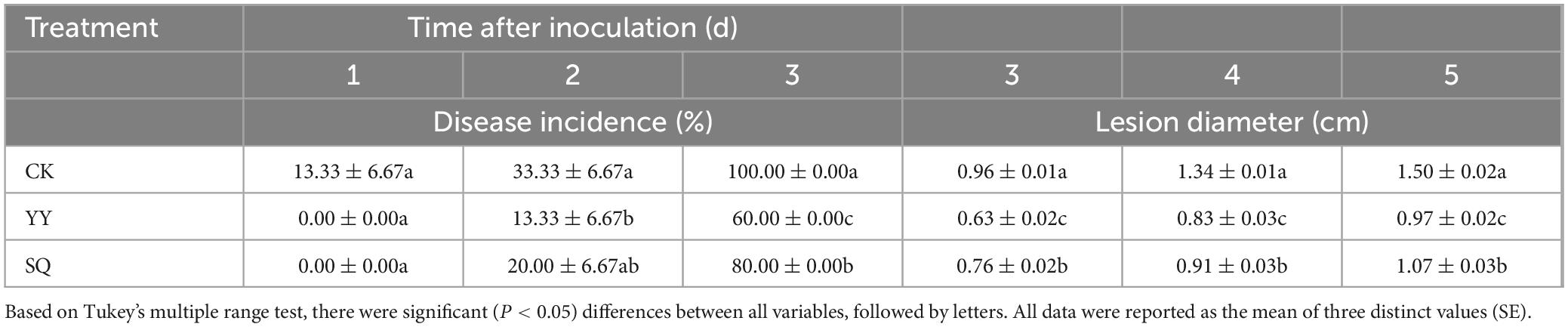
Table 2. The effect of KXF6501 fermentation solution and cell-free supernatant on lesion diameter and disease incidence in blueberries infected with B. cinerea.
3.3 Transcriptional analyses of blueberry fruits treated with KXF6501 fermentation solution and cell-free supernatant
3.3.1 Analysis of biological pathway enrichment and differential expression
In the current study, the R2 values between biological replicates ranged 0.92–1.00, indicating the reliability of the transcriptome data (Figure 2A). A comparative analysis was conducted on three sets of sequencing data to elucidate the variations in gene expression among fruits subjected to the YY, SQ, and CK treatments during storage: CK vs. YY, CK vs. SQ, and YY vs. SQ. Differentially expressed genes were identified and assessed based on their expression levels (FPKM) for each gene |log2FC| > 1, P-value < 0.05). As illustrated in Figure 2B, 1328 DEGs were detected in the CK vs. YY comparison, with 926 DEGs showing upregulation and 402 DEGs showing downregulation. A total of 1081 DEGs were detected in the comparison between CK and SQ, with 695 upregulated and 386 downregulated genes (Figure 2C). In total, 840 DEGs were identified in the comparison between YY and SQ, with 318 upregulated and 522 downregulated DEGs (Figure 2D). More DEGs were detected in the CK vs. YY comparison group, suggesting a greater difference in quality between blueberries treated with CK and YY. At the same time, volcano plots can visually represent each DEG’s distribution and expression. The differentially expressed genes observed in the comparison between the CK and YY groups exhibited a pronounced level of divergence, suggesting more conspicuous variation among the genes (Figure 2B). The disparity in the quantity and distribution of DEGs in the volcano plot indicates a greater distinction between the blueberries in the CK and YY groups.
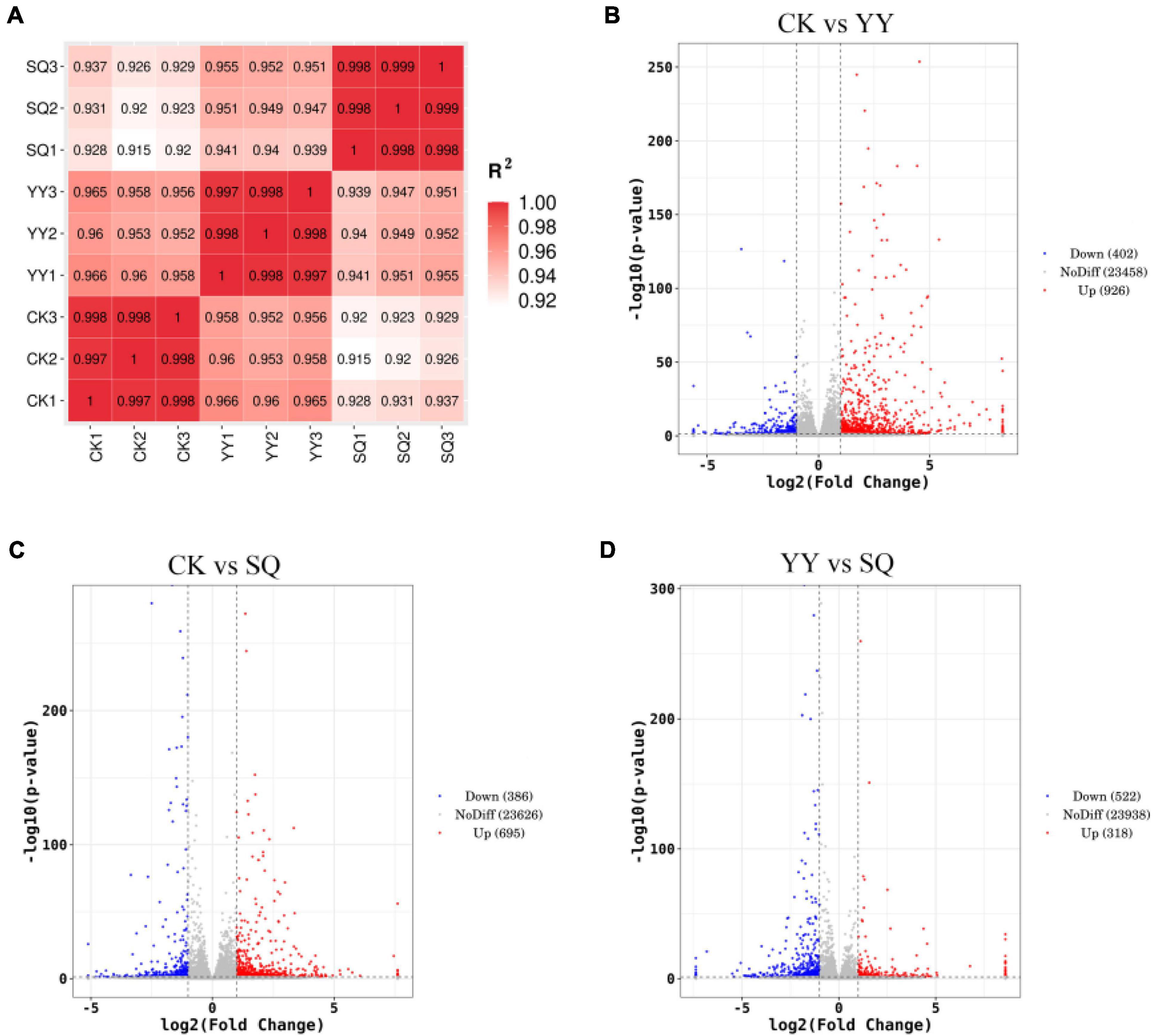
Figure 2. Sample correlation test chart (A), volcano map distribution of DEGs in CK vs. YY (B), CK vs. SQ (C), and YY vs. SQ (D).
Among the 1328, 1081, and 840 DEGs of blueberry fruits in the CK vs. YY, CK vs. SQ, and YY vs. SQ groups, 359, 286, and 199 DEGs with pathway annotations were distributed across 93, 86, and 76 pathways, respectively. The top 20 enriched biological pathways in the CK vs. YY, CK vs. SQ, and YY vs. SQ blueberry fruits are depicted in Figure 3. Of these 20 pathways, “phenylpropanoid biosynthesis” emerged as the most important enrichment pathway in the three groups, and it had a small FDR compared with the other pathways.
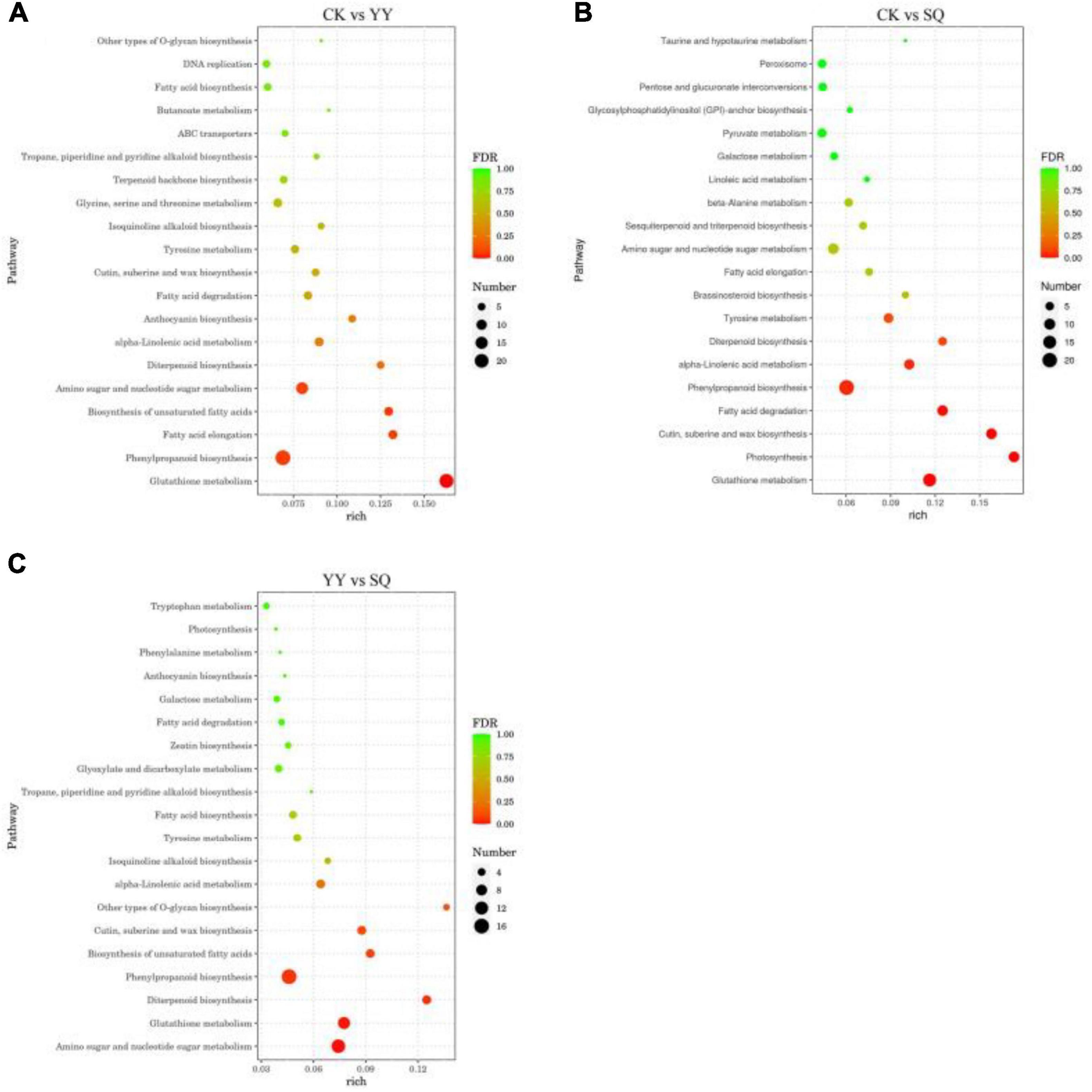
Figure 3. Scatter diagram of DEGS biological pathway enrichment in CK vs YY (A), CK vs SQ (B) and YY vs SQ (C) fruits.
3.3.2 Transcriptome analyzed and identified consistently DEGs by treated with KXF6501 fermentation solution and cell-free supernatant
Two groups of DEGs (CK vs. YY and CK vs. SQ) were compared to further investigate the alterations in gene expression within the blueberry peel tissue following YY and SQ treatments. Of these, 481 were differentially expressed in both treatments (Figure 4A) (Supplementary Table 2). Of the 481 DEGs, 94 had path annotations. These 94 genes were categorized into seven groups according biological pathways, including carbohydrate metabolism (14 genes, 14.89%), metabolism of other amino acids (14 genes, 14.89%), biosynthesis of other secondary metabolites (11 genes, 11.70%), lipid metabolism (11 genes, 11.70%), metabolism of terpenoids and polyketides (11 genes, 11.70%), environmental adaptation (seven genes, 7.45%), and others (26 genes, 27.66%) (Figure 4B). Further analysis divided the 11 genes implicated in secondary metabolic biosynthesis into four categories: phenylpropanoid biosynthesis (seven genes, 63.64%), isoquinoline alkaloid biosynthesis (two genes, 18.18%), flavonoid biosynthesis (one gene, 9.09%), and anthocyanin biosynthesis (one gene, 9.09%) (Figure 4C). Figure 4D shows the hierarchical clustering of the differentially expressed profiles of genes involved in phenylpropanoid biosynthesis (Supplementary Table 3). The phenylpropanoid biosynthesis pathway encompassed several differentially expressed genes, including five peroxidase genes (g282, g6517, g37110, g46030, and g45681), a probable mannitol dehydrogenase gene (g41069), and a beta-glucosidase 12 gene (g4457). Two differentially expressed genes (DEGs) were downregulated, whereas five DEGs were upregulated. Furthermore, the DEGs that were down-regulated or up-regulated in both treatments were consistent.
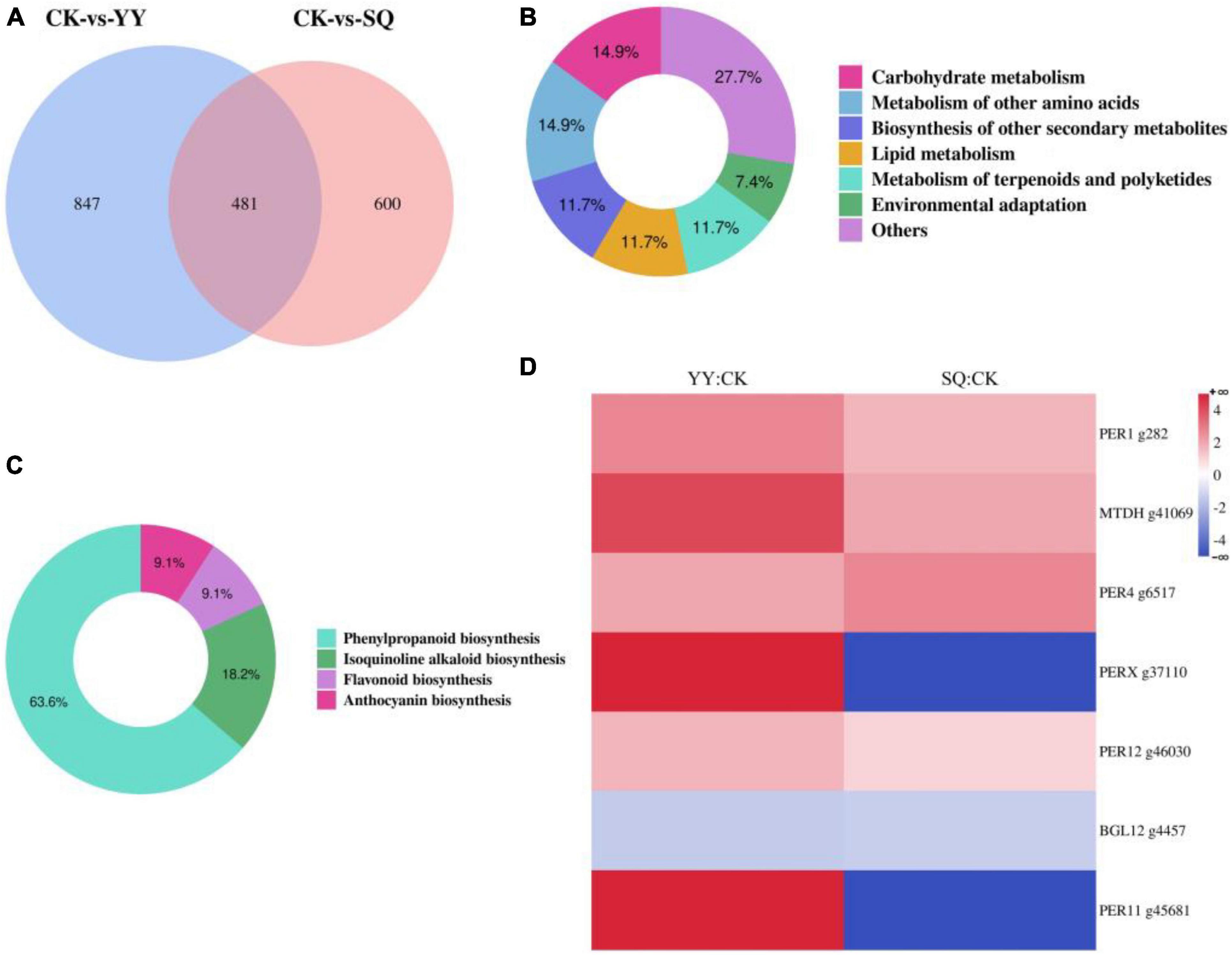
Figure 4. The consistently differentially expressed genes (DEGs) identified through transcriptome analysis in fruits treated with YY and SQ. (A) Venn diagram illustrating the non-overlap or overlap of DEGs between the two treatments; (B) Categorizes the consistently DEGs in both treatments according to biological pathways; (C) A KEGG pathway-based classification of DEGs involved in secondary metabolic biosynthesis in both treatments; (D) A cluster analysis of phenylpropanoid biosynthesis-related DEGs expression profiles in both treatments YY and SQ.
3.3.3 Inconsistently DEGs identified by transcriptome analysis treated with KXF6501 fermentation solution and cell-free supernatant
Compared with the control, the phenylpropanoid biosynthesis pathway in YY includes the following DEGs: six peroxidase genes (g45983, g46519, g20528, g9789, g41892, and g33343),three beta-glucosidase genes (g18328, g7883, g4459), a Cinnamoyl-CoA reductase gene (g33301), a Raucaffricine-O-beta-D-glucosidase gene (g38065), a Probable mannitol dehydrogenase (g41068), a Stemmadenine O-acetyltransferase gene (g2690), a Caffeic acid 3-O-methyltransferase gene (g25476), a Feruloyl CoA ortho-hydroxylase F6H1-3 (g38995), a Cytochrome P450 84A1 gene (g26210), a Acetyl-CoA-benzylalcohol acetyltransferase (g43274). Among the DEGs (Figure 5A), 12 had upregulated expression while 5 had downregulated expression.
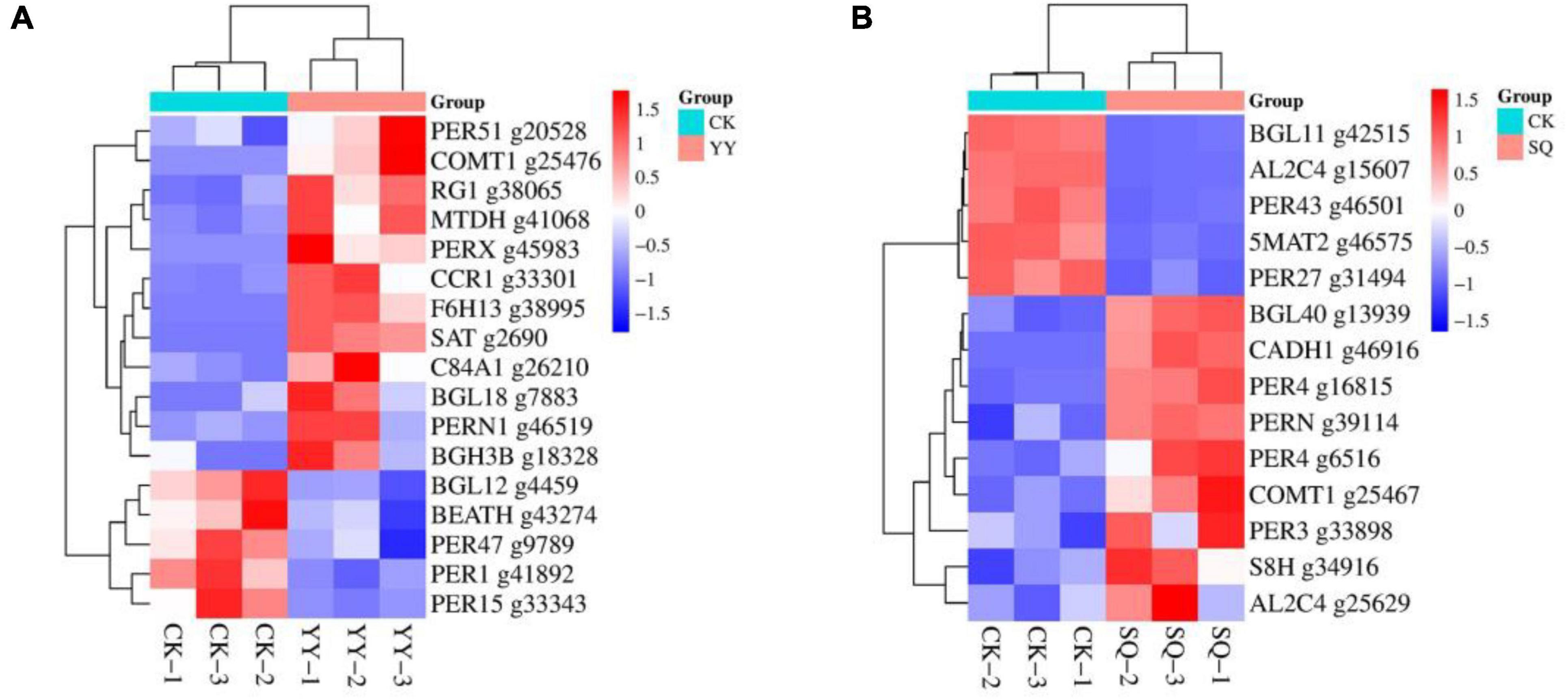
Figure 5. Cluster analysis was conducted on the expression profiles of specific genes associated with phenylpropanoid biosynthesis in CK-vs-YY (A) and CK-vs-SQ (B).
Compared with the control, the phenylpropanoid biosynthesis pathway in SQ exhibits differential expression of several key genes, including six peroxidase genes (g6516, g16815, g33898, g39114, g46501, g31494), two beta-glucosidase genes (g13939, g42515), a Cinnamyl alcohol dehydrogenase gene (g46916), two Aldehyde dehydrogenase genes (g25629, g15607), a Scopoletin 8-hydroxylase gene (g34916), a Caffeic acid 3-O-methyltransferase gene (g25467), and a Pelargonidin 3-O-(6-caffeoylglucoside) 5-O-(6-O-malonylglucoside) malonyltransferase gene (g46575). Nine DEGs were upregulated and five DEGs were downregulated (Figure 5B).
3.3.4 RT-qPCR analysis
To assess the credibility of our transcriptome data, we randomly selected ten genes associated with phenylpropane metabolism, transcription factors, and plant hormone signal transduction in fruits. The findings shown in Figure 6 indicate that the expression levels of these genes in the CK, YY, and SQ samples aligned with the transcriptome data, indicating the repeatability and consistency of the RNA-seq data.
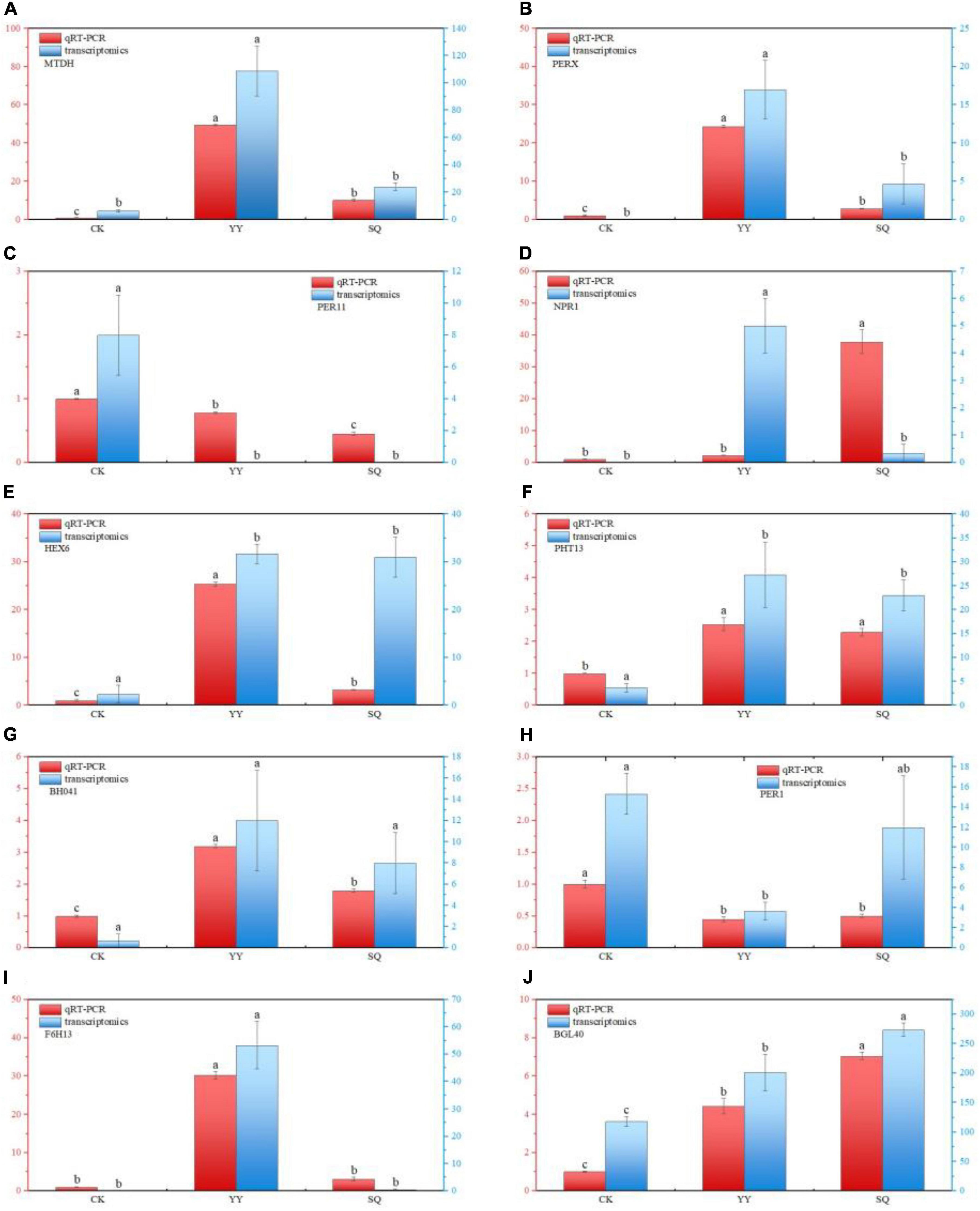
Figure 6. DEGs validation using RT-qPCR for MTDH (A), PERX (B), PER11 (C), NPR1 (D), HEX6 (E), PHT13 (F), BHO41 (G), PER1 (H), F6H13 (I), and BGL40 (J). Based on the Tukey’s multiple range test, there are significant (P < 0.05) differences between all variables followed by letters. All datas are reported as means of three distinct values ± SE. Different lowercase letters indicate significant (P < 0.05) differences between treatments by the Tukey’s multiple range test.
3.4 Effects of KXF6501 fermentation solution and cell-free supernatant on phenylpropanoid metabolism related enzymes and substances in harvested blueberries
Compared to the control fruits, the application of YY and SQ to blueberry fruits triggered PAL, C4H, 4CL, and PPO activities, as well as an increase in total phenol, flavonoid, and lignin content. PAL activity in all groups exhibited an overall upward trend (Figure 7A), with YY-treated fruits reaching peak activity on the 6th day and displayed significantly higher (P < 0.05) PAL activity than that of the control fruits except on the 10th day. SQ-treated fruits maintained higher PAL activity on days 4–10 compared the control. The C4H activity of control fruits increased slightly during the entire storage period (Figure 7B). During storage, the C4H activity was significantly increased by YY and SQ (P < 0.05). The peak activity of C4H in YY and SQ fruits was observed 4 d post-treatment, with the enzyme activity being 2.63- and 1.92 times higher (P < 0.05) in these fruits than in the control fruits. As shown in Figure 7C, the 4CL enzyme induced by YY showed significantly higher (P < 0.05) activity than that induced by SQ and the control during the entire storage period. On the 6th day, the 4CL activity of the YY and SQ treatments reached a peak that was 40.80% and 16.17% higher than that of the control, respectively. All treatment groups showed similar changes in PPO activity (Figure 7D). YY treatment rapidly induced PPO activity, which peak on day 4. All treatments significantly increased PPO activity (P < 0.05) during days 6–10, compared with the control. As shown in Figure 7E, YY and SQ notably enhanced lignin levels in blueberry fruits (P < 0.05) during days 2–10. The lignin content of YY-treated- and SQ-treated fruits was 67.66% and 22.71% higher, respectively, than that of the control fruits on day 8. The phenol levels of the blueberry fruits across all groups exhibited a similar pattern during storage (Figure 7F). Compared to the control, YY- and SQ-treated fruits had higher levels of total phenols, which increased significantly (P < 0.05) during days 6–10. Peak total phenol levels were reached on the 6th day and subsequently declined. The change in flavonoid content (Figure 7G) was consistent with that of total phenols. The YY and SQ treatment groups exhibited significantly higher flavonoid content than in the control fruits on days 4–10 (P < 0.05).
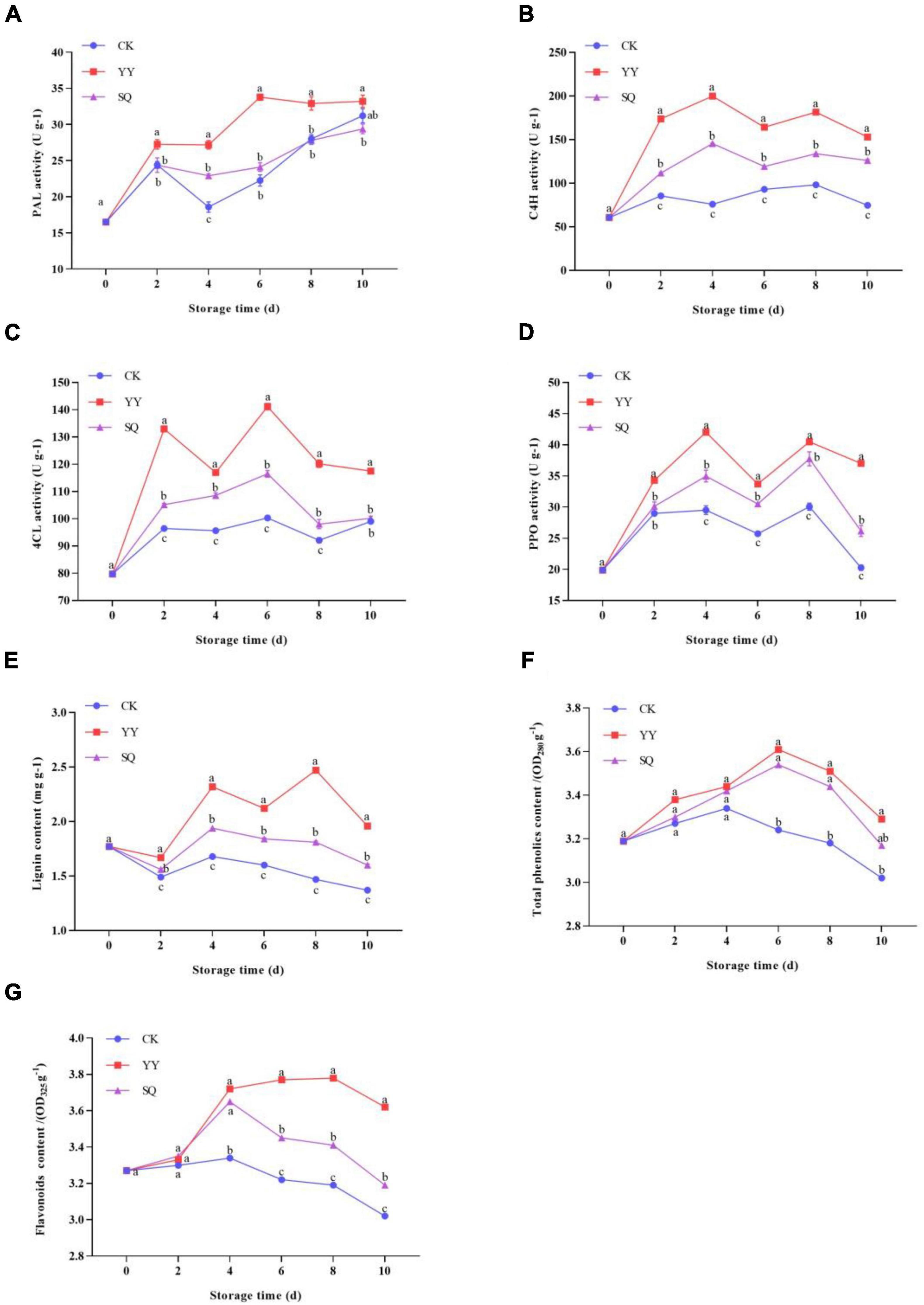
Figure 7. Effects of different treatments (YY and SQ) compared to control (CK) on PAL (A), C4H (B), 4CL (C), PPO (D), lignin content (E), phenol content (F), and flavonoid content (G) of blueberries. Different lowercase letters indicate significant (P < 0.05) differences between treatments by the Tukey’s multiple range test.
3.5 Effect of KXF6501 fermentation solution and cell-free supernatant on the postharvest quality of blueberry fruits
As shown in Table 3, the rate of weight loss in the fruits increased with the storage time elongation, with the YY treatment group demonstrating the lowest weight loss rate throughout the storage period, followed by the SQ treatment group. The TSS, TA, AsA and Reducing sugar content in fruits treated with YY were consistently higher than in the control fruits, except at 2 d. Simultaneously, the contents of TSS, TA, AsA and Reducing sugars in fruits treated with SQ in the late storage period (6, 8, and 10 d) were significantly (P < 0.05) higher than in the control fruits.
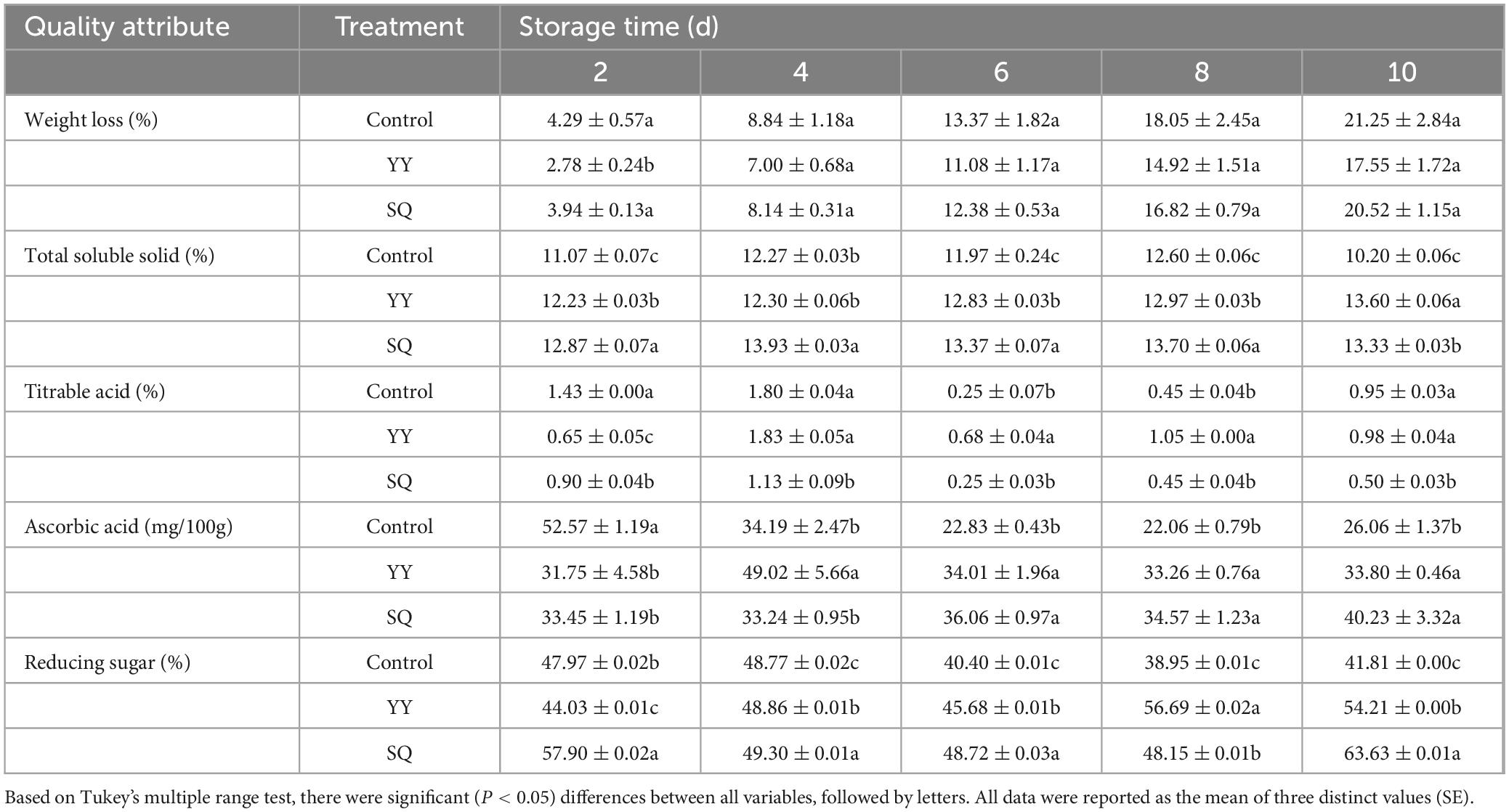
Table 3. Effect of KXF6501 fermentation solution and cell-free supernatant on the quality parameters of postharvest blueberries.
According to Table 4, there is no significant difference in L, a, b, c, and h0 values of blueberries between the YY and SQ treatment groups and the control groups throughout the duration of storage. However, the a/b value over the storage period exhibited a statistically remarkable (P < 0.05) increase in the SQ experimental group, with the exception of day 8.
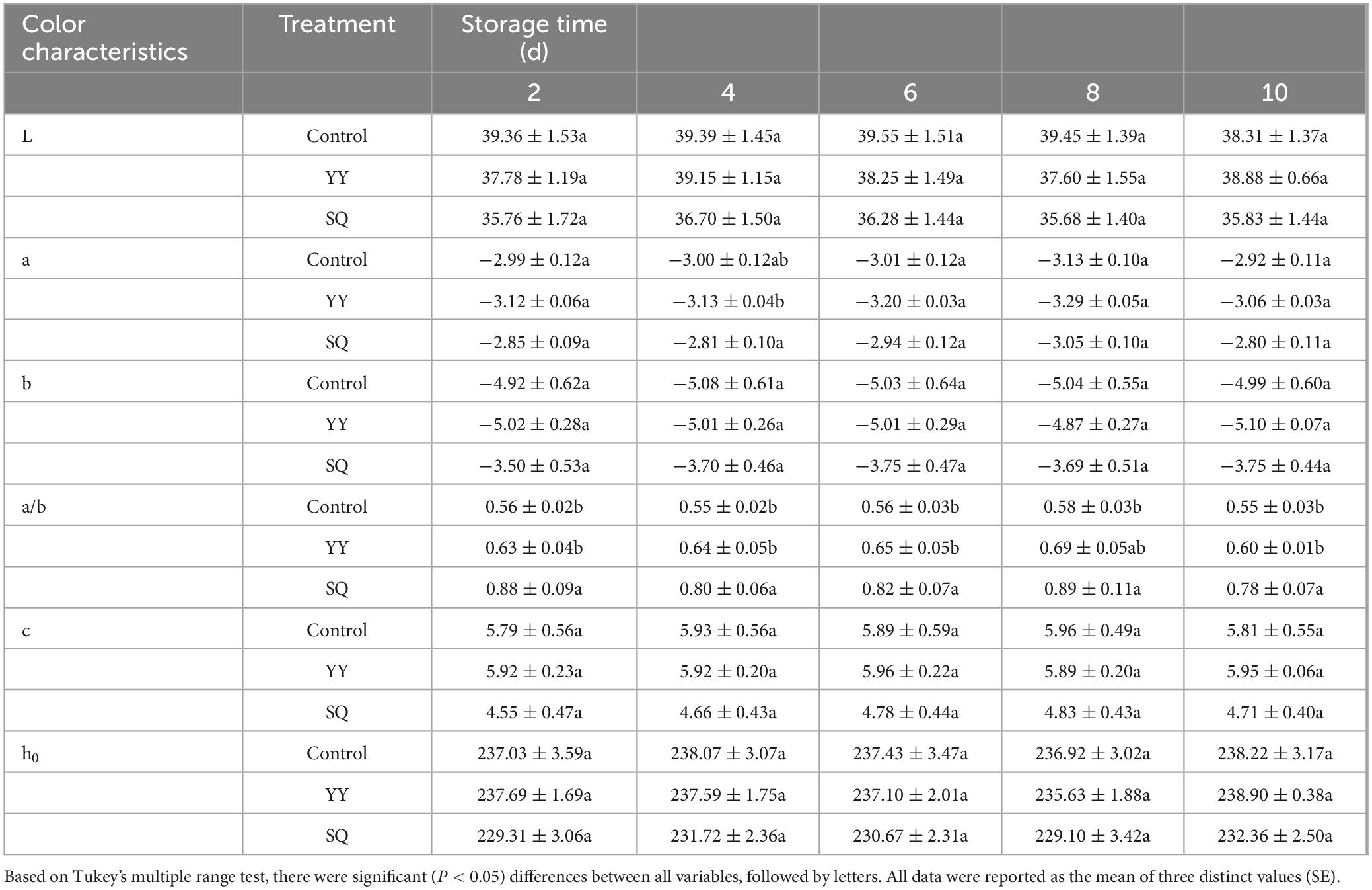
Table 4. Effects of KXF6501 fermentation solution and cell-free supernatant on color variation in postharvest blueberries.
4 Discussion
Post-harvest blueberry fruits are easily infected with B. cinerea during storage and transportation, leading to the decay of blueberry fruits, shortening their storage period, and affecting their flavor and nutritional value (Bell et al., 2021). Therefore, decay emerged as the predominant factor influencing blueberry quality during storage, necessitating the development of treatment strategies to prolong storage life (Ding et al., 2023). In recent years, microbial antagonists derived from the Bacillus genus have been recognized as environmentally friendly and biologically safe alternatives to conventional chemical fungicides (Lastochkina et al., 2019). The use of Bacillus spp. as biocontrol formulations to mitigate in vegetables and fruits postharvest diseases has gained increasing attention (Koh et al., 2014; Arroyave-Toro et al., 2017). The B. velezensis SL-6 strain has an inhibitory effect on B. cinerea in pears and can significantly reduce the incidence of B. cinerea and the expansion of lesion diameter during pear storage (Cozzolino et al., 2020). Bacillus strains M9-20 decreased the growth of B. cinerea spores and mycelia, inhibited blueberry fruit rot caused by pathogenic bacteria, and reduced the incidence and severity of rot by 50% (Kurniawan et al., 2018). Bacillus spp. are recognized as safe microorganisms suitable for the food industry (Algburi et al., 2016), and some Bacillus strains are recognized as probiotic bacteria (Khochamit et al., 2015). In this study, a fermentation solution (YY) of the BCA B. tequilensis KXF6501 which was isolated from healthy strawberries, significantly inhibited spore germination and reduced germ tube length in B. cinerea, followed by the cell-free supernatant (SQ). We also analyzed the antagonistic effects of KXF6501 (YY and SQ) on B. cinerea mycelium growth in vitro. Indicating that both YY and SQ exhibited inhibitory effects, with YY demonstrating a greater efficacy against B. cinerea than SQ. The YY and SQ treatments reduced the incidence of fruits and lesion diameter, thus effectively controlling the gray mold of blueberries in vivo. The control effect of YY was better than that of SQ. This may be due to competition for nutrients and space between microbial antagonists and phytopathogenic microorganisms (Carmona-Hernandez et al., 2019; Bell et al., 2021). At the same time, the attachment of microbial antagonists to the hyphae of pathogens significantly slows pathogen growth and spore germination, as antagonists have a higher nutrient absorption rate than the target pathogens (Sharma et al., 2009; Singh et al., 2022). Moreover, certain antifungal compounds may be essential for protection against pathogenic infections (Koh et al., 2014; Arroyave-Toro et al., 2017).
The biological control mechanism of Bacillus spp. involves the production of antimicrobial compounds that hinder the growth of plant pathogens (Guardado-Valdivia et al., 2018). Little is known, nevertheless, about the basic process of fruit disease resistance induction, which is limited to a single metabolite, enzyme, or gene (Lu et al., 2015). We conducted an analysis of the transcriptome of fruits treated with YY and SQ to further study the possible defense responses of fruits induced by YY and SQ using Illumina sequencing technology. The results showed that YY and SQ caused substantial changes in mRNAs levels compared to the control fruits (Figures 2B–D). Furthermore, according to the biological pathway enrichment analysis, the blueberries resistance response to YY and SQ was influenced by the biosynthesis of secondary metabolites (Figure 3). These findings are consistent with the results obtained from the analysis of biological pathways that included consistently differentially expressed genes (DEGs) in both treatments (Figure 4B). Simultaneously, the consistent DEGs linked to secondary metabolite biosynthesis underscore the significance of phenylpropanoid biosynthesis as a key secondary metabolic process in fruit resistance induction (Figure 4). The proportion of consistent DEGs involved in phenylpropanoid biosynthesis was the highest, displaying consistent expression patterns across both treatments. In particular, the expression levels of cationic peroxidase 1, probable mannitol dehydrogenase, lignin-forming anionic peroxidase, peroxidase 4, and peroxidase 12, all of which play roles in the synthesis of crucial enzymes, were elevated in fruits treated with YY and SQ compared to those in control fruits. In addition, we analyzed the inconsistent DEGs related to phenylpropane metabolism in the YY and SQ treatments. In the YY treatment group, 12 DEGs were downregulated, and 12 DEGs were upregulated. Similarly, in the SQ treatment group, 5 DEGs were downregulated, whereas 9 DEGs were upregulated. The majority of Differentially Expressed Genes (DEGs) are actively involved in critical enzymes PAL, POD, C4H, and 4CL. This finding aligns with the results studied by Lu et al. (2015), who discovered the upregulation of critical genes related to phenylpropanoid metabolism and biosynthesis in yeast-treated fruits.
The phenylpropanoid pathway is one of important plant secondary metabolism, and is beneficial for wound healing and resistance enhancement in fruits and vegetables (Wang B. et al., 2019). The primary products of this pathway include antifungal plant antitoxins, phenolic acids (associated with the biosynthesis of secondary cell walls), lignin, and essential precursors like chalcones that serve as initiators of the flavonoid pathway (Tan et al., 2016; Zhou et al., 2018). Antifungal plant antitoxins, phenolic acids (associated with the biosynthesis of secondary cell walls), lignin, and essential precursors like chalcones (initiating flavonoid biosynthesis) are the primary products of this pathway. Lignin primarily consists of phenylpropanoid units resulting from the oxidative polymerization of hydroxycinnamoyl alcohol derivatives. Its biosynthesis plays a crucial role in enhancing the structural integrity of the cell wall, and serves as a physical barrier against pathogens and their reproduction (Ferrer et al., 2008; Liu et al., 2014; Zhou et al., 2018). Vital enzymes such as PAL, C4H, and 4CL in the phenylpropanoid pathway are essential for regulating the smooth progression of this biosynthetic pathway (Muro-Villanueva et al., 2019). PAL facilitates the transformation of l-phenylalanine into trans-cinnamic acid, followed by cinnamate C4H, which catalyzes the transformation of trans-cinnamic acid into hydroxycinnamic acid to produce lignin biosynthesis precursors, including coumaric acid, ferulic acid, and caffeic acid. Subsequently, 4CL catalyzes the synthesis of coenzyme A thioesters, which are converted to flavonoids. Simultaneously, p-coumaroyl coenzyme A is catalyzed by lignin (Du et al., 2023). In addition, phenols are oxidized to quinones by PPO, which are directly toxic to pathogens (Shadle et al., 2003). Flavonoids exhibit antioxidant properties and anti-bacterial action, such as the inhibition of spore germination and mycelium growth (Liu et al., 2014).
To verify the upregulation of genes in the phenylpropane pathway at the biochemical level, we measured enzyme activity (PAL, C4H, 4CL, and PPO) and the content of three metabolites (flavonoids, total phenols and lignin) at various time points in blueberry peel. The findings showed that treatment with YY and SQ significantly increased the activities of the defense-related enzymes PAL, C4H, 4CL, and PPO and promoted the accumulation of total phenols, flavonoids, and lignin in fruits. By comparing with the treatment with SQ, YY proved to be more effective in accumulating higher levels of enzyme activities and substance related to the phenylpropane pathway. This may be attributed to the fact that in addition to the antimicrobial substances in the supernatant, cells in B. tequilensis fermentation broth also play an important role (Carmona-Hernandez et al., 2019). The Bacillus cells colonize the surface of the fruit, which act as inducers to stimulate the enzymes activity and substances related to the phenylpropanoid metabolism pathway, thus protecting the fruit from pathogen attack. The accumulation of lignin, flavonoids, and total phenols in postharvest fruits following treatment with Bacillus may be attributed to the upregulation of key enzymes in the phenylpropanoid pathway (Wang et al., 2023). Moreover, postharvest blueberry fruits treated with YY and SQ exhibited significantly stable quality during storage compared to the control groups, highlighting the potential of KXF6501 to preserve the appearance and nutritional quality of postharvest blueberry fruits. These results show that the BCA of B. tequilensis KXF6501 can serve as a viable approach for mitigating postharvest fruit diseases.
5 Conclusion
B. tequilensis KXF6501 can reduce incidence and lesion diameter caused by B. cinerea gray mold during blueberries storage, thereby preserving postharvest fruit quality. Application of B. tequilensis KXF6501 (YY and SQ) resulted in significant upregulation of genes associated with phenylpropanoid biosynthesis, leading to the elevated enzyme activities and accumulation of antifungal compounds in phenylpropanoid pathway. That enhanced disease resistance in blueberries. When compared to the SQ treatment, YY proved to be more effective in controlling gray mold disease by accumulating higher levels of enzyme activity as well as substances related to the phenylpropane pathway. Therefore, B. tequilensis KXF6501 may be a potential BCA for blueberry gray mold. However, we need to further explore the mechanism of action of B. tequilensis KXF6501 in controlling postharvest diseases of fruits for assessing the safety of both B. tequilensis KXF6501 and its metabolites on consumer health. These results of this research offer insights into the use of B. tequilensis KXF6501 for the biological management of postharvest diseases in blueberries.
Data availability statement
The datasets presented in this study can be found in online repositories. The names of the repository/repositories and accession number(s) can be found in this article/Supplementary material.
Author contributions
QD: Data curation, Methodology, Resources, Writing – original draft. RL: Formal analysis, Visualization, Writing – review and editing. LL: Data curation, Visualization, Writing – review and editing. LC: Formal analysis, Methodology, Writing – review and editing. JT: Methodology, Resources, Writing – review and editing. JD: Data curation, Methodology, Resources, Writing – review and editing. FW: Data curation, Funding acquisition, Project administration, Supervision, Validation, Writing – review and editing.
Funding
The authors declare that financial support was received for the research, authorship, and/or publication of this article. This study was supported by the National Natural Science Foundation of China (Nos. 32160395), “High-level Talents Training Support Program” of Yunnan Province (XDYC-QNRC-2022-0226), and Joint Special Project for Agriculture of Yunnan Province (202301BD070001-102).
Conflict of interest
The authors declare that the research was conducted in the absence of any commercial or financial relationships that could be construed as a potential conflict of interest.
Publisher’s note
All claims expressed in this article are solely those of the authors and do not necessarily represent those of their affiliated organizations, or those of the publisher, the editors and the reviewers. Any product that may be evaluated in this article, or claim that may be made by its manufacturer, is not guaranteed or endorsed by the publisher.
Supplementary material
The Supplementary Material for this article can be found online at: https://www.frontiersin.org/articles/10.3389/fmicb.2024.1455008/full#supplementary-material
Footnotes
References
Algburi, A., Volski, A., Cugini, C., Walsh, E., Chistyakov, V., Mazanko, M., et al. (2016). Safety properties and probiotic potential of Bacillus subtilis KATMIRA1933 and Bacillus amyloliquefaciens B-1895. Adv. Microbiol. 6, 432–452. doi: 10.4236/aim.2016.66043
Alvarez, A., Gelezoglo, R., Garmendia, G., González, M. L., Magnoli, A. P., Arrarte, E., et al. (2019). Role of Antarctic yeast in biocontrol of Penicillium expansum and patulin reduction of apples. Environ. Sustain. 2, 277–283. doi: 10.1007/s42398-019-00081-1
Arroyave-Toro, J. J., Mosquera, S., and Villegas-Escobar, V. (2017). Biocontrol activity of Bacillus subtilis EA-CB0015 cells and lipopeptides against postharvest fungal pathogens. Biol. Control 114, 195–200. doi: 10.1016/j.biocontrol.2017.08.014
Bell, S. R., Hernández Montiel, L. G., González Estrada, R. R., and Gutiérrez Martínez, P. (2021). Main diseases in postharvest blueberries, conventional and eco-friendly control methods: A review. LWT 149:112046. doi: 10.1016/j.lwt.2021.112046
Bi, K., Liang, Y., Mengiste, T., and Sharon, A. (2023). Killing softly: A roadmap of Botrytis cinerea pathogenicity. Trends Plant Sci. 28, 211–222. doi: 10.1016/j.tplants.2022.08.024
Carmona-Hernandez, S., Reyes-Pérez, J. J., Chiquito-Contreras, R. G., Rincon-Enriquez, G., Cerdan-Cabrera, C. R., and Hernandez-Montiel, L. G. (2019). Biocontrol of postharvest fruit fungal diseases by bacterial antagonists: A review. Agronomy 9:121. doi: 10.3390/agronomy9030121
Chen, H., Cao, S., Fang, X., Mu, H., Yang, H., Wang, X., et al. (2015). Changes in fruit firmness, cell wall composition and cell wall degrading enzymes in postharvest blueberries during storage. Sci. Horticult. 188, 44–48. doi: 10.1016/j.scienta.2015.03.018
Chen, K., Tian, Z., He, H., Long, C.-A., and Jiang, F. (2020). Bacillus species as potential biocontrol agents against citrus diseases. Biol. Control 151:104419. doi: 10.1016/j.biocontrol.2020.104419
Chen, Y., Hung, Y.-C., Chen, M., and Lin, H. (2017). Effects of acidic electrolyzed oxidizing water on retarding cell wall degradation and delaying softening of blueberries during postharvest storage. LWT 84, 650–657. doi: 10.1016/j.lwt.2017.06.011
Cozzolino, M. E., Distel, J. S., García, P. A., Mascotti, M. L., Ayub, M. J., Benazzi, L. M., et al. (2020). Control of postharvest fungal pathogens in pome fruits by lipopeptides from a Bacillus sp. isolate SL-6. Sci. Horticult. 261:108957. doi: 10.1016/j.scienta.2019.108957
Deng, L., Yin, B., Yao, S., Wang, W., and Zeng, K. (2016). Postharvest application of oligochitosan and chitosan reduces calyx alterations of citrus fruit induced by ethephon degreening treatment. J. Agric. Food Chem. 64, 7394–7403. doi: 10.1021/acs.jafc.6b02534
Ding, J., Liu, C., Huang, P., Zhang, Y., Hu, X., Li, H., et al. (2023). Effects of thymol concentration on postharvest diseases and quality of blueberry fruit. Food Chem. 402:134227. doi: 10.1016/j.foodchem.2022.134227
Du, R., Deng, J., Huang, E., Chen, L., Tang, J., Liu, Y., et al. (2023). Effects of salicylic acid-grafted bamboo hemicellulose on gray mold control in blueberry fruit: The phenylpropanoid pathway and peel microbial community composition. Int. J. Biol. Macromol. 251:126303. doi: 10.1016/j.ijbiomac.2023.126303
Ferrer, J. L., Austin, M. B., Stewart, C., and Noel, J. P. (2008). Structure and function of enzymes involved in the biosynthesis of phenylpropanoids. Plant Physiol. Biochem. 46, 356–370. doi: 10.1016/j.plaphy.2007.12.009
Guardado-Valdivia, L., Tovar-Pérez, E., Chacón-López, A., López-García, U., Gutiérrez-Martínez, P., Stoll, A., et al. (2018). Identification and characterization of a new Bacillus atrophaeus strain B5 as biocontrol agent of postharvest anthracnose disease in soursop (Annona muricata) and avocado (Persea americana). Microbiol. Res. 210, 26–32. doi: 10.1016/j.micres.2018.01.007
Ji, Y., Hu, W., Jiang, A., Xiu, Z., Liao, J., Yang, X., et al. (2019). Effect of ethanol treatment on the quality and volatiles production of blueberries after harvest. J. Sci. Food Agric. 99, 6296–6306. doi: 10.1002/jsfa.9904
Khochamit, N., Siripornadulsil, S., Sukon, P., and Siripornadulsil, W. (2015). Antibacterial activity and genotypic–phenotypic characteristics of bacteriocin-producing Bacillus subtilis KKU213: Potential as a probiotic strain. Microbiol. Res. 170, 36–50. doi: 10.1016/j.micres.2014.09.004
Kiesewalter, H. T., Lozano-Andrade, C. N., Maróti, G., Snyder, D., Cooper, V. S., Jørgensen, T. S., et al. (2020). Complete Genome sequences of 13 Bacillus subtilis soil isolates for studying secondary metabolite diversity. Microbiol. Resour. Announc. 9, 10–1128. doi: 10.1128/mra.01406-19
Koh, Y., Jung, J., Hur, J., and Kim, G. (2014). Control of postharvest fruit rot diseases of kiwifruit by antagonistic bacterium Bacillus subtilis. Int. Symp. Kiwifruit 1096, 377–382. doi: 10.17660/ActaHortic.2015.1096.44
Kurniawan, O., Wilson, K., Mohamed, R., and Avis, T. J. (2018). Bacillus and Pseudomonas spp. provide antifungal activity against gray mold and Alternaria rot on blueberry fruit. Biol. Control 126, 136–141. doi: 10.1016/j.biocontrol.2018.08.001
Lastochkina, O., Seifikalhor, M., Aliniaeifard, S., Baymiev, A., Pusenkova, L., Garipova, S., et al. (2019). Bacillus Spp.: Efficient biotic strategy to control postharvest diseases of fruits and vegetables. Plants 8:97. doi: 10.3390/plants8040097
Liu, Y., Ge, Y., Bi, Y., Li, C., Deng, H., Hu, L., et al. (2014). Effect of postharvest acibenzolar-S-methyl dipping on phenylpropanoid pathway metabolism in muskmelon (Cucumis melo L.) fruits. Sci. Horticult. 168, 113–119. doi: 10.1016/j.scienta.2014.01.030
Lu, L., Ji, L., Shi, R., Li, S., Zhang, X., Guo, Q., et al. (2019). Dextran as an elicitor of phenylpropanoid and flavonoid biosynthesis in tomato fruit against gray mold infection. Carbohydr. Polym. 225:115236. doi: 10.1016/j.carbpol.2019.115236
Lu, L., Wang, J., Zhu, R., Lu, H., Zheng, X., and Yu, T. (2015). Transcript profiling analysis of Rhodosporidium paludigenum-mediated signalling pathways and defense responses in mandarin orange. Food Chem. 172, 603–612. doi: 10.1016/j.foodchem.2014.09.097
Lu, Y., Ma, D., He, X., Wang, F., Wu, J., Liu, Y., et al. (2021). Bacillus subtilis KLBC BS6 induces resistance and defence-related response against Botrytis cinerea in blueberry fruit. Physiol. Mol. Plant Pathol. 114:101599. doi: 10.1016/j.pmpp.2020.101599
Miller, K., Feucht, W., and Schmid, M. (2019). Bioactive compounds of strawberry and blueberry and their potential health effects based on human intervention studies: A brief overview. Nutrients 11:1510. doi: 10.3390/nu11071510
Muro-Villanueva, F., Mao, X., and Chapple, C. (2019). Linking phenylpropanoid metabolism, lignin deposition, and plant growth inhibition. Curr. Opin. Biotechnol. 56, 202–208. doi: 10.1016/j.copbio.2018.12.008
Ozgen, M., Scheerens, J., Reese, N., and Miller, R. (2010). Total phenolic, anthocyanin contents and antioxidant capacity of selected elderberry (Sambucus canadensis L.) accessions. Pharmacogn. Magaz. 6:198. doi: 10.4103/0973-1296.66936
Panebianco, S., Vitale, A., Polizzi, G., Scala, F., and Cirvilleri, G. (2015). Enhanced control of postharvest citrus fruit decay by means of the combined use of compatible biocontrol agents. Biol. Control 84, 19–27. doi: 10.1016/j.biocontrol.2015.02.001
Papoutsis, K., Mathioudakis, M. M., Hasperué, J. H., and Ziogas, V. (2019). Non-chemical treatments for preventing the postharvest fungal rotting of citrus caused by Penicillium digitatum (green mold) and Penicillium italicum (blue mold). Trends Food Sci. Technol. 86, 479–491. doi: 10.1016/j.tifs.2019.02.053
Rivera, S. A., Zoffoli, J. P., and Latorre, B. A. (2013). Determination of optimal sulfur dioxide time and concentration product for postharvest control of gray mold of blueberry fruit. Postharvest Biol. Technol. 83, 40–46. doi: 10.1016/j.postharvbio.2013.03.007
Shadle, G. L., Wesley, S. V., Korth, K. L., Chen, F., Lamb, C., and Dixon, R. A. J. P. (2003). Phenylpropanoid compounds and disease resistance in transgenic tobacco with altered expression of L-phenylalanine ammonia-lyase. Phytochemistry 64, 153–161. doi: 10.1016/S0031-9422(03)00151-1
Sharma, R., Singh, D., and Singh, R. (2009). Biological control of postharvest diseases of fruits and vegetables by microbial antagonists: A review. Biol. Control 50, 205–221. doi: 10.1016/j.biocontrol.2009.05.001
Shi, C., Yan, P., Li, J., Wu, H., Li, Q., and Guan, S. (2014). Biocontrol of Fusarium graminearum growth and deoxynivalenol production in wheat kernels with bacterial antagonists. Int. J. Environ. Res. Public Health 11, 1094–1105. doi: 10.3390/ijerph110101094
Shi, Z., Deng, J., Wang, F., Liu, Y., Jiao, J., Wang, L., et al. (2019a). Individual and combined effects of bamboo vinegar and peach gum on postharvest grey mould caused by Botrytis cinerea in blueberry. Postharvest Biol. Technol. 155, 86–93. doi: 10.1016/j.postharvbio.2019.05.016
Shi, Z., Yang, H., Jiao, J., Wang, F., Lu, Y., and Deng, J. (2019b). Effects of graft copolymer of chitosan and salicylic acid on reducing rot of postharvest fruit and retarding cell wall degradation in grapefruit during storage. Food Chem. 283, 92–100. doi: 10.1016/j.foodchem.2018.12.078
Shi, Z., Wang, F., Lu, Y., and Deng, J. (2018). Combination of chitosan and salicylic acid to control postharvest green mold caused by Penicillium digitatum in grapefruit fruit. Sci. Horticult. 233, 54–60. doi: 10.1016/j.scienta.2018.01.039
Singh, A. K., and Sharma, P. (2020). Disinfectant-like activity of lipopeptide biosurfactant produced by Bacillus tequilensis strain SDS21. Colloids Surf. B Biointerf. 185:110514. doi: 10.1016/j.colsurfb.2019.110514
Singh, S. K., Singh, V. K., Singh, P. K., Modi, A., and Kumar, A. (2022). Microbial antagonists in postharvest management of fruit. Res. Technol. Adv. Food Sci. 2022, 333–346. doi: 10.1016/B978-0-12-824369-5.00005-1
Tan, B. A., Daim, L. D. J., Ithnin, N., Ooi, T. E. K., Md-Noh, N., Mohamed, M., et al. (2016). Expression of phenylpropanoid and flavonoid pathway genes in oil palm roots during infection by Ganoderma boninense. Plant Gene 7, 11–20. doi: 10.1016/j.plgene.2016.07.003
Toscano, S., Ferrante, A., Leonardi, C., and Romano, D. (2018). PAL activities in asparagus spears during storage after ammonium sulfate treatments. Postharvest Biol. Technol. 140, 34–41. doi: 10.1016/j.postharvbio.2018.02.010
Wang, B., Jiang, H., Bi, Y., He, X., Wang, Y., Li, Y., et al. (2019). Preharvest multiple sprays with sodium nitroprusside promote wound healing of harvested muskmelons by activation of phenylpropanoid metabolism. Postharvest Biol. Technol. 158:110988. doi: 10.1016/j.postharvbio.2019.110988
Wang, F., Deng, J., Jiao, J., Lu, Y., Yang, L., and Shi, Z. (2019). The combined effects of Carboxymethyl chitosan and Cryptococcus laurentii treatment on postharvest blue mold caused by Penicillium italicum in grapefruit fruit. Sci. Horticult. 253, 35–41. doi: 10.1016/j.scienta.2019.04.031
Wang, R., Zhang, Q., Li, B., Weng, Q., and Liu, P. (2024). Dimethyl trisulfide (DMTS) fumigation enhances fruit quality and reduces postharvest incidence of bacterial canker in tomato. Postharvest Biol. Technol. 216:113038. doi: 10.1016/j.postharvbio.2024.113038
Wang, X., Xie, S., Mu, X., Guan, B., Hu, Y., and Ni, Y. (2023). Investigating the resistance responses to Alternaria brassicicola in ‘Korla’ fragrant pear fruit induced by a biocontrol strain Bacillus subtilis Y2. Postharvest Biol. Technol. 199:112293. doi: 10.1016/j.postharvbio.2023.112293
Wu, C., Wang, Y., Ai, D., Li, Z., and Wang, Y. (2022). Biocontrol yeast T-2 improves the postharvest disease resistance of grape by stimulation of the antioxidant system. Food Sci. Nutr. 10, 3219–3229. doi: 10.1002/fsn3.2940
Yihui, G., Song, J., Du, L., Vinqvist, M., Palmer, L. C., Fillmore, S., et al. (2018). Characterization of laccase from apple fruit during postharvest storage and its response to diphenylamine and 1-methylcyclopropene treatments. Food Chem. 253, 314–321. doi: 10.1016/j.foodchem.2018.01.142
Zhang, L., Wang, L., Zeng, X., Chen, R., Yang, S., and Pan, S. (2019). Comparative transcriptome analysis reveals fruit discoloration mechanisms in postharvest strawberries in response to high ambient temperature. Food Chem. X 2:100025. doi: 10.1016/j.fochx.2019.100025
Zhou, H., Zhu, H., Ren, Z., Li, X., Zhong, J., and Liu, E. (2021). Efficacy of Bacillus tequilensis strain JN-369 to biocontrol of rice blast and enhance rice growth. Biol. Control 160:104652. doi: 10.1016/j.biocontrol.2021.104652
Zhou, Y., Ma, J., Xie, J., Deng, L., Yao, S., and Zeng, K. (2018). Transcriptomic and biochemical analysis of highlighted induction of phenylpropanoid pathway metabolism of citrus fruit in response to salicylic acid, Pichia membranaefaciens and oligochitosan. Postharvest Biol. Technol. 142, 81–92. doi: 10.1016/j.postharvbio.2018.01.021
Keywords: Bacillus tequilensis, blueberry, induced resistance, phenylpropanoids, postharvest disease, biological control
Citation: Du Q, Li R, Liu L, Chen L, Tang J, Deng J and Wang F (2024) Application of Bacillus tequilensis for the control of gray mold caused by Botrytis cinerea in blueberry and mechanisms of action: inducing phenylpropanoid pathway metabolism. Front. Microbiol. 15:1455008. doi: 10.3389/fmicb.2024.1455008
Received: 26 June 2024; Accepted: 06 August 2024;
Published: 30 August 2024.
Edited by:
Ajay Kumar, Amity University, IndiaReviewed by:
Qiushui Wang, Beijing Academy of Science and Technology (Beijing Center for Physical and Chemical Analysis), ChinaUgo De Corato, Italian National Agency for New Technologies, Energy and Sustainable Economic Development (ENEA), Italy
Copyright © 2024 Du, Li, Liu, Chen, Tang, Deng and Wang. This is an open-access article distributed under the terms of the Creative Commons Attribution License (CC BY). The use, distribution or reproduction in other forums is permitted, provided the original author(s) and the copyright owner(s) are credited and that the original publication in this journal is cited, in accordance with accepted academic practice. No use, distribution or reproduction is permitted which does not comply with these terms.
*Correspondence: Jia Deng, dengjia1983@163.com; Fang Wang, 35445230@qq.com